- 1MRC Weatherall Institute of Molecular Medicine, University of Oxford, Oxford, United Kingdom
- 2Nuffield Department of Women’s and Reproductive Health, University of Oxford, Oxford, United Kingdom
- 3Developmental Immunology Research Group, Department of Paediatrics, University of Oxford, Oxford, United Kingdom
- 4Gene Regulatory Networks in Development and Disease Laboratory, MRC Weatherall Institute of Molecular Medicine, Radcliffe Department of Medicine, University of Oxford, Oxford, United Kingdom
- 5Department of Biochemistry, Faculty of Science, King Abdulaziz University, Jeddah, Saudi Arabia
The conduits of life; the animal oviducts and human fallopian tubes are of paramount importance for reproduction in amniotes. They connect the ovary with the uterus and are essential for fertility. They provide the appropriate environment for gamete maintenance, fertilization and preimplantation embryonic development. However, serious pathologies, such as ectopic pregnancy, malignancy and severe infections, occur in the oviducts. They can have drastic effects on fertility, and some are life-threatening. Despite the crucial importance of the oviducts in life, relatively little is known about the molecular drivers underpinning the embryonic development of their precursor structures, the Müllerian ducts, and their successive differentiation and maturation. The Müllerian ducts are simple rudimentary tubes comprised of an epithelial lumen surrounded by a mesenchymal layer. They differentiate into most of the adult female reproductive tract (FRT). The earliest sign of Müllerian duct formation is the thickening of the anterior mesonephric coelomic epithelium to form a placode of two distinct progenitor cells. It is proposed that one subset of progenitor cells undergoes partial epithelial-mesenchymal transition (pEMT), differentiating into immature Müllerian luminal cells, and another subset undergoes complete EMT to become Müllerian mesenchymal cells. These cells invaginate and proliferate forming the Müllerian ducts. Subsequently, pEMT would be reversed to generate differentiated epithelial cells lining the fully formed Müllerian lumen. The anterior Müllerian epithelial cells further specialize into the oviduct epithelial subtypes. This review highlights the key established molecular and genetic determinants of the processes involved in Müllerian duct development and the differentiation of its upper segment into oviducts. Furthermore, an extensive genome-wide survey of mouse knockout lines displaying Müllerian or oviduct phenotypes was undertaken. In addition to widely established genetic determinants of Müllerian duct development, our search has identified surprising associations between loss-of-function of several genes and high-penetrance abnormalities in the Müllerian duct and/or oviducts. Remarkably, these associations have not been investigated in any detail. Finally, we discuss future directions for research on Müllerian duct development and oviducts.
Introduction
The paramesonephric or Müllerian ducts are the embryonic anlagen of most of the female reproductive tract. They give rise to the oviducts (human fallopian tubes), uterine horns (human uterus and chicken shell gland), cervix and upper vagina, in an anterior-to-posterior fashion. The mammalian FRT is of paramount importance in female biology due to its role in promoting a successful fertilization and pregnancy. Concretely, the oviducts provide the environment for gametes nutrition and transport, fertilization and the development and transport of the preimplantation blastocyst (Killian, 2004; Li and Winuthayanon, 2017). Later processes such as blastocyst implantation and fetal development occur in the uterine horns (Kim and Kim, 2017). The reproductive pattern is similar in chickens (Bellairs and Osmond, 2005). Furthermore, the FRT is the site of origin of serious human diseases such as ovarian (George et al., 2016; Zhang et al., 2019), endometrial (Bansal et al., 2009; Morice et al., 2016) and cervical cancer (Cohen et al., 2019); ectopic pregnancy (Rana et al., 2013); and pelvic inflammatory diseases such as endometriosis (Zondervan et al., 2018), salpingitis (Owhor et al., 2019), and hydrosalpinx (Ng and Cheong, 2019). Many of these diseases are life-threatening and can cause infertility.
Despite the crucial importance of this system in female life and disease, reproductive adult organs and the molecular mechanisms underlying their homeostasis, hormonal cycles, pregnancy and associated diseases have been poorly characterized. The lack of knowledge of developmental processes orchestrating FRT formation is, among other reasons, a causative factor and an increasing interest in the field has been detected in recent years (Mullen and Behringer, 2014; Cunha et al., 2018; Roly et al., 2018; Zhao and Yao, 2019). To address this gap, Müllerian duct development is reviewed in this work. A special focus is placed on the anterior Müllerian duct portion differentiating into oviducts, as they are the least studied of the FRT organs and yet are the site of origin of high-grade serous ovarian cancer (George et al., 2016; Zhang et al., 2019), the deadliest gynecological malignancy; tubal causes of infertility; ectopic pregnancy (Rana et al., 2013); and pelvic inflammatory disease (Ng and Cheong, 2019; Owhor et al., 2019).
During embryonic development, the intermediate mesoderm of the gastrula gives rise to two urogenital mesonephroi that will host the female (Müllerian) and male (Wolffian) primitive genital tracts. These reproductive tubes are essential during embryonic sex determination and both ducts provisionally develop regardless of the genetic sex (female: mammal XX and avian ZW; male: mammal XY and avian ZZ). The Wolffian or mesonephric ducts originate directly from the intermediate mesoderm, followed by Müllerian duct development from the anterior mesonephric coelomic epithelium (Gruenwald, 1941; Jacob et al., 1999; Guioli et al., 2007; Orvis and Behringer, 2007; Yoshino et al., 2014; Atsuta and Takahashi, 2016). In XY/ZZ embryos, Wolffian ducts are maintained and Müllerian ducts regress, and vice versa in XX/ZW embryos. These mechanisms are conserved among species, except in class Aves females, where the right gonad and Müllerian duct additionally regress whereas the left equivalents become fully functional (Gruenwald, 1941; Dohr and Tarmann, 1984; Jacob et al., 1999; Guioli et al., 2007; Orvis and Behringer, 2007; Atsuta and Takahashi, 2016). Müllerian ducts are histomorphologically similar during ontogeny and birth, comprising a simple columnar luminal epithelium surrounded by mesenchymal stroma (Komatsu and Fujita, 1978; Yamanouchi et al., 2010; Ghosh et al., 2017). Although certain degree of differentiation is accomplished during this early life period, it is only during the first 2 weeks of life that the primitive tube undergoes an intense morphological change governed by genetic programmes in which every Müllerian section terminally differentiates into an adult reproductive organ (Cunha, 1976; Komatsu and Fujita, 1978; Kurita et al., 2001; Yamanouchi et al., 2010; Ghosh et al., 2017).
The scope of this review focuses on the cellular mechanisms and genetic/molecular entities involved in Müllerian development and successive differentiation into oviducts. This review is structured in two sections: (1) the chronological three-step Müllerian development and oviduct differentiation as well as (2) advanced insights into the Müllerian genetics and developmental pathways. These developmental genetic families mainly comprise master embryonic transcriptional regulators in the homeobox and paired box families, secreted proteins in the WNT [wingless-type mouse mammary tumor virus (MMTV) integration site family] family, RARs (retinoic acid receptors), cofactors, kinases and a G protein-coupled receptor. The bone morphogenic protein (BMP), WNT, transforming growth factor beta (TFG-β), phosphatidylinositol 3-kinase (PI3K)/protein kinase B (Akt) pathway, G-protein coupled receptor and fibroblast growth factor (FGF) signaling pathways are also involved in Müllerian/oviduct biology. Furthermore, literature on cell populations forming the Müllerian ducts is discussed and novel theories on cell lineages, hierarchies and dynamics throughout Müllerian and oviduct life are proposed. This review also includes a thorough genome-wide interrogation of knockout mouse databases searching Müllerian/oviduct phenotypes, which identified new candidate genes that might play an important role in their biology.
Sections
Müllerian Ducts Originate From the Mesonephric Coelomic Epithelium, Remain in XX/ZW Embryos and Anteriorly Differentiate Into the Oviducts
Embryonic Müllerian development is highly conserved among avian and mammalian species and it is executed in three phases (Gruenwald, 1941; Dohr and Tarmann, 1984; Jacob et al., 1999; Guioli et al., 2007; Orvis and Behringer, 2007; Atsuta and Takahashi, 2016) (Figures 1A–C). In mouse it occurs between E11.75-13.5 (Kobayashi et al., 2004; Guioli et al., 2007; Orvis and Behringer, 2007), in human between Carnegie stages 18–23 (Hashimoto, 2003) and in chicken between HH16-HH30/32 (Guioli et al., 2007; Atsuta and Takahashi, 2016). After Müllerian development, the Wolffian ducts regress in females (Figure 1D) and the Müllerian ducts differentiate into the adult FRT (Figures 1E,F).
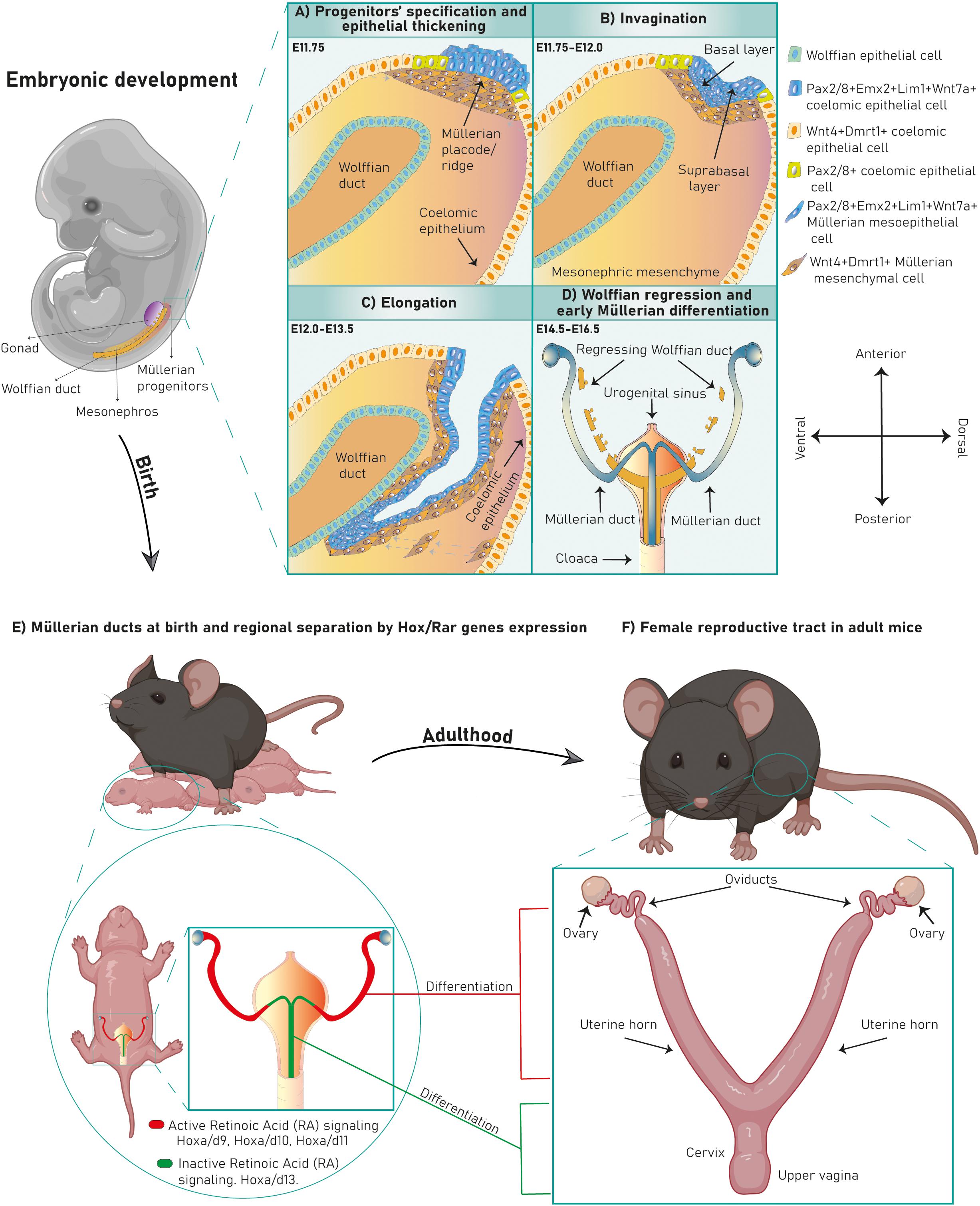
Figure 1. Murine FRT development. Mouse Müllerian ducts originate between E11.75–13.5. The first developmental phase consists of Müllerian progenitors’ specification and placode formation in the mesonephric coelomic epithelium. Pax2+/8+/Emx2+/Lim1+/Wnt7a+ epithelial cells (mesoepithelial progenitors) give rise to the tubal lumen whereas Wnt4+/Dmrt1+ epithelial cells are mesenchymal progenitors (A). Subsequently, epithelial invagination occurs through apical constriction of suprabasal epithelial cells in the placode, ending when the proliferative Müllerian tip establishes physical contact with the Wolffian duct at E12.0 (B). Afterward, the Müllerian ducts elongate following the anterior–posterior axis until E13.5 (C). Finally, the Wolffian ducts regress (D) and the Müllerian ducts undertake an initial regional differentiation marked by a Hox/RAR axis (E). During the first 2 weeks of life, every FRT section completely differentiates, giving rise to the functional female reproductive tract (F).
Embryonic Development of Müllerian Ducts: Cellular and Molecular Mechanisms
The Müllerian ducts develop from the anterior mesonephric coelomic epithelium and elongate along the anteroposterior axis between the Wolffian duct and the coelomic epithelium. After elongation they fuse with the urogenital sinus and differentiate into the FRT. The Müllerian developmental process is divided into three stages marked by diverse cellular and molecular mechanisms (Figure 2A).
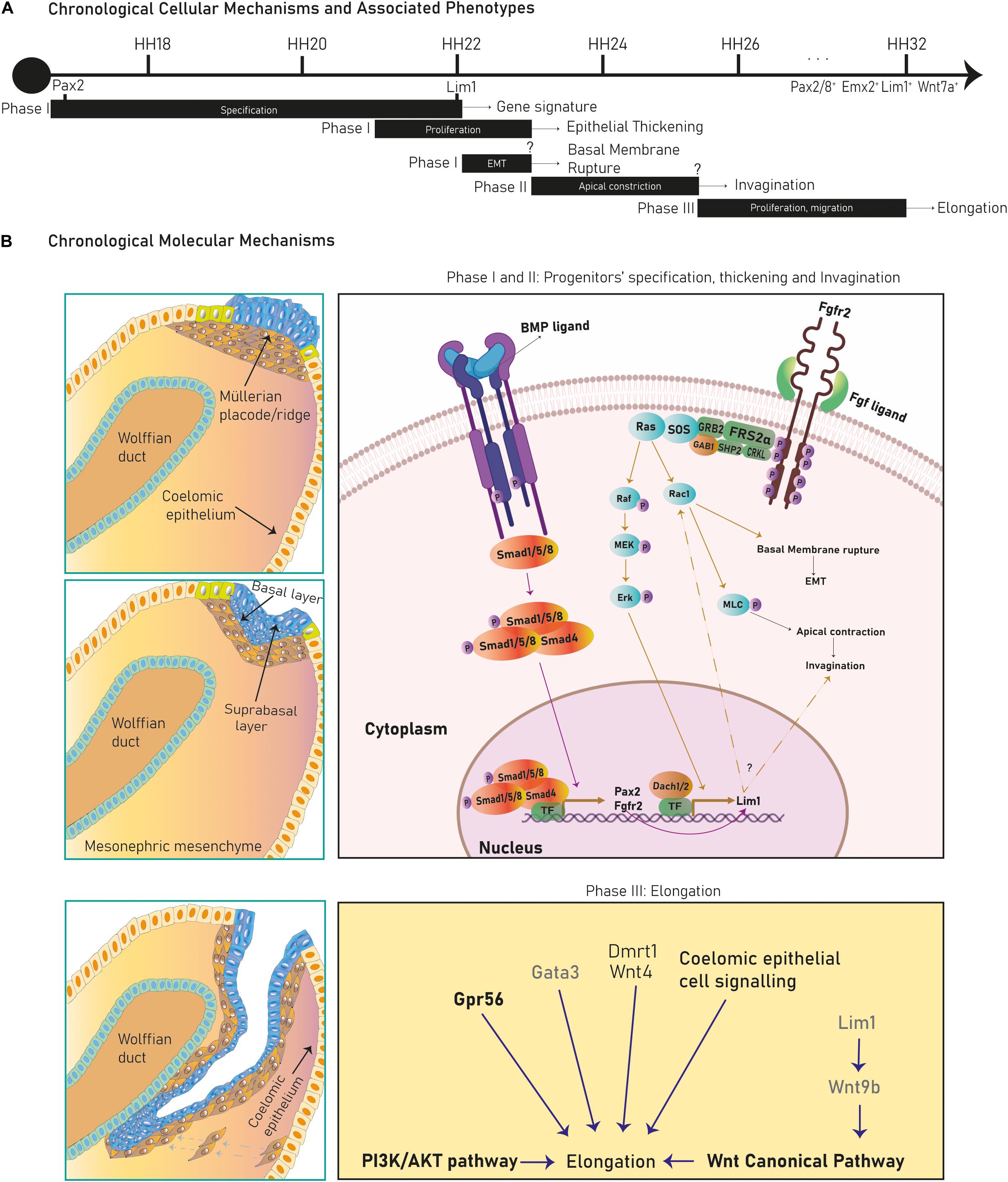
Figure 2. Cellular mechanisms and molecular pathways in Müllerian duct development. Several cellular mechanisms chronologically succeed during chicken Müllerian duct development (A). In (B), early stages in Müllerian duct development are governed by the BMP pathway regulating Pax2, activating the FGF/RAS/ERK pathway and promoting Lim1 expression and apical constriction control. Dach1/2 and Pax2 also regulate Lim1. Later, development proceeds through rostral-caudal elongation depending on Wolffian paracrine signals, such as Lim1-regulated Wnt9b, which activates the Wnt Canonical pathway. Besides, the PI3k/Akt pathway, coelomic epithelium signaling and Wnt4, Gata3, Gpr56, and Dmrt1 regulate elongation. Genes/pathways in bold are expressed/intrinsic to Müllerian epithelial cells, genes in light are expressed by mesonephric mesenchymal cells and genes in gray are expressed by Wolffian epithelial cells.
Phase I: progenitors’ specification and epithelial thickening
During this stage, cells from the cranial coelomic epithelial surface on both mesonephroi undertake fate specification into Müllerian progenitors, and a placode/ridge forms close to the Wolffian duct (Gruenwald, 1941, Dohr and Tarmann, 1984, Jacob et al., 1999, Kobayashi et al., 2004, Guioli et al., 2007, Orvis and Behringer, 2007 and Atsuta and Takahashi, 2016) (Figure 1A). This process is conserved across most species. A placode is a multi-layered epithelial thickening forming basal and supra-basal strata of cells and created by cell proliferation (Figure 1B). The Müllerian ridge contains Müllerian mesenchymal and mesoepithelial progenitors and BMP signaling is essential for its development in chicken embryos (Atsuta and Takahashi, 2016). Moreover, an independent bulk-RNA study on chicken Müllerian ducts found that genes related to positive regulation of pathway-restricted SMAD protein phosphorylation and SMAD protein signal transduction were enriched at this stage, supporting a role for the BMP pathway (Roly et al., 2020b). The BMP effector molecule/s is currently unknown, although expression of Bmp2/3/4/7 has been documented during chicken Müllerian development (Atsuta and Takahashi, 2016; Roly et al., 2020b). Regarding Müllerian progenitors, the term “mesoepithelial” is adopted across species as these progenitors give rise to cells negative for epithelial markers (negative: Ck8 and E-cadherin; weakly positive: pan-cytokeratin) and positive for a mesenchymal marker (vimentin) while showing mesenchymal morphology and arrangement upon the basement membrane (BM), which is an epithelial feature (Gruenwald, 1941; Dohr and Tarmann, 1984; Jacob et al., 1999; Orvis and Behringer, 2007; Prunskaite-Hyyryläinen et al., 2016; Ghosh et al., 2020; Roly et al., 2020a). On the other hand, mesenchymal progenitors differentiate into cells of a true mesenchymal phenotype. This suggests that mesoepithelial progenitors undergo partial epithelial-mesenchymal transition (pEMT), whereas mesenchymal progenitors fully complete an EMT (Figure 3). The first EMT step observed in this context is the rupture of the placode BM, positively regulated by the FGF pathway in chickens. When the FGF receptor Fgfr2 and the intracellular modulator Rac1 are downregulated, the placode BM fails to break (Atsuta and Takahashi, 2016) (Figure 2B). The role of the FGF/ERK (extracellular signal-related kinase)/MAPK (mitogen-activated protein kinase) pathway is reaffirmed due to the expression of several pathway genes during chicken Müllerian development (Roly et al., 2020b). The FGF molecular regulator is unknown, although upregulated expression of Fgf10/16/18 has been reported during chicken Müllerian development (Roly et al., 2020b). Interestingly, the BMP pathway positively regulates the FGF/RAS/MAPK pathway in chicken Müllerian ducts (Atsuta and Takahashi, 2016).
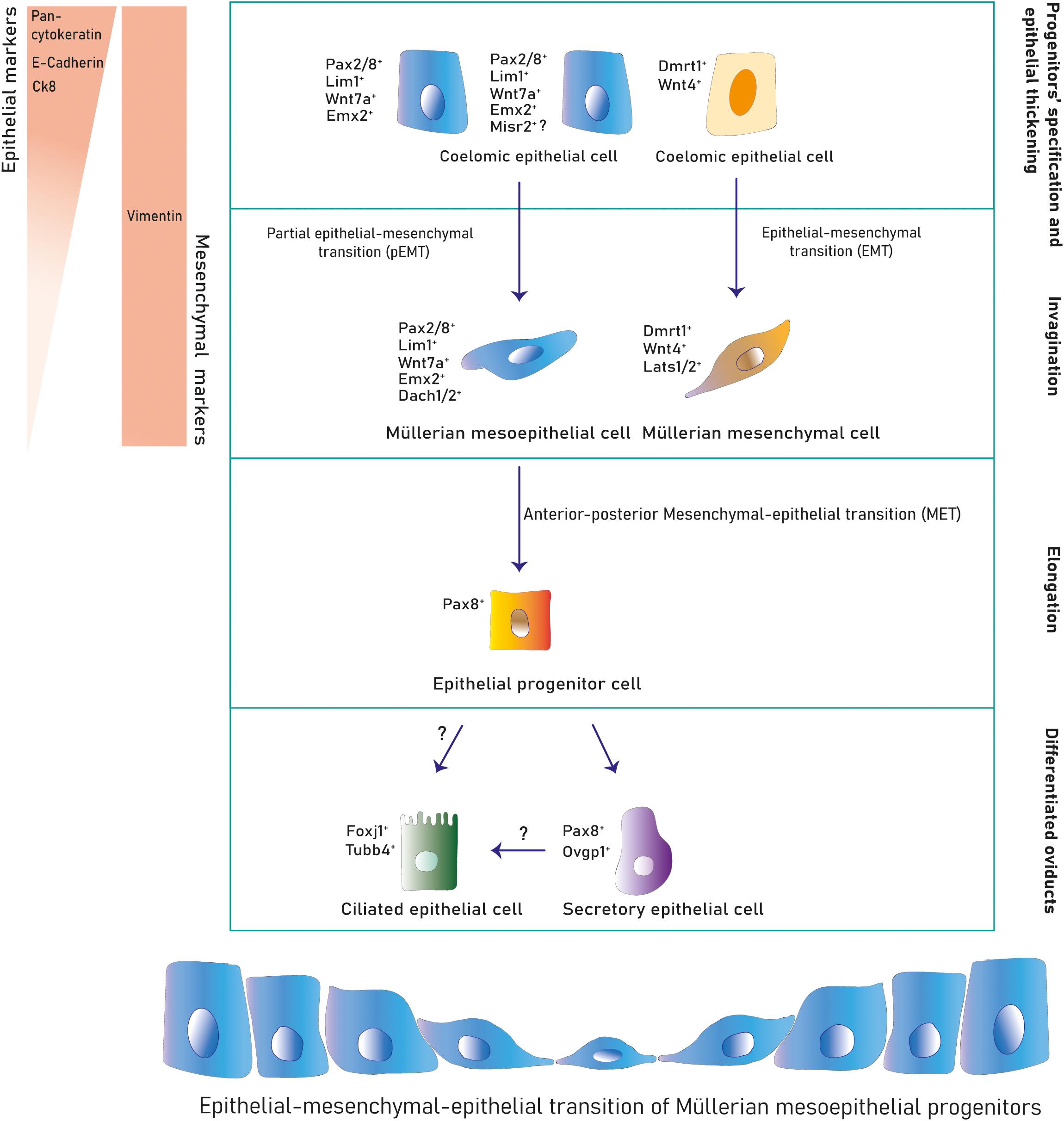
Figure 3. Cell lineages during Müllerian development and oviduct differentiation. Two primitive populations expressing distinct markers in the mesonephric coelomic epithelium give rise to the Müllerian epithelial and mesenchymal lineages. Mesoepithelial progenitors execute a pEMT into the mesoepithelial type forming the lumen and mesenchymal progenitors undergo EMT differentiating into mesenchyme. After Müllerian duct formation, the mesoepithelium reverses to an epithelial identity that differentiates into secretory and ciliated oviduct epithelial subtypes postnatally.
The murine Müllerian luminal epithelium is exclusively derived from mesoepithelial progenitors in the placode expanding along the anterior–posterior axis (Ghosh et al., 2020). Conversely, in mouse and chicken, mesenchymal cells derive from progenitors in the placode migrating anteroposteriorly and from progenitors along the coelomic mesonephric epithelium undergoing local delamination and dorsal-ventral migration (Guioli et al., 2007; Ayers et al., 2015; Prunskaite-Hyyryläinen et al., 2016) (Figures 1A–C, 3). Mesoepithelial progenitors sequentially upregulate the embryonic transcription factors Pax2, Emx2, and Lim1 (also Pax8, Pbx1, Hnf1b, and Wnt7a in mouse), while mesenchymal precursors express markers such as Wnt4 (and Dmrt1 in chicken) (Jacob et al., 1999; Vainio et al., 1999; Schnabel et al., 2003; Kobayashi et al., 2004; Guioli et al., 2007; Orvis and Behringer, 2007; Lokmane et al., 2010; Ayers et al., 2015; Atsuta and Takahashi, 2016; Prunskaite-Hyyryläinen et al., 2016). Expression of the Dach1/2 transcriptional cofactors has also been reported in murine mesoepithelial progenitors (Davis et al., 2008). Some mouse mesoepithelial progenitors have been shown to express Misr2, although this putative subpopulation has not been further studied (Ghosh et al., 2020).
In chicken mesoepithelial progenitors, Pax2 expression is regulated by the BMP pathway (Figure 2B) and controls Lim1 expression (Atsuta and Takahashi, 2016). Lim1 expression is also governed by the FGF/ERK/MAPK pathway as cell electroporation with a dominant-negative Fgfr2 and Ras inhibition decreased phosphorylated ERK (pERK) levels and abolished Lim1 expression (Atsuta and Takahashi, 2016) (Figure 2B). Additionally, Lim1 expression in chickens requires unknown Wolffian duct signals (Atsuta and Takahashi, 2016), although this specification is not clear in mammals.
Phase II: invagination
After progenitor specification and placode formation, Wnt4+/Dmrt1+ progenitors differentiate into mesenchymal cells, whereas Pax2+/Emx2+/Lim1+ progenitors give rise to mesoepithelial cells (Figures 1B, 3). They invaginate until the growing duct tip establishes physical contact with the Wolffian duct (Gruenwald, 1941; Dohr and Tarmann, 1984; Jacob et al., 1999; Hashimoto, 2003; Kobayashi et al., 2004; Guioli et al., 2007; Orvis and Behringer, 2007). The cellular mechanism behind Müllerian epithelial invagination is cell intercalation-mediated apical contraction of supra-basal cells (Atsuta and Takahashi, 2016; Pearl et al., 2017), which transmits tension to the BM, resulting in bending. Apical constriction is executed by an actomyosin skeleton of F-actin filaments and non-muscle myosin II, a motor protein activated by its phosphorylated myosin light chain (pMLC) (Sellers, 1991; Bresnick, 1999; Martin and Goldstein, 2014) (Figure 2B). pMLC is apically detected in chicken Pax2+/Lim1+ cells before Müllerian invagination (Atsuta and Takahashi, 2016). Fgfr2 and Rac1 downregulation decreases pMLC and disrupts invagination, indicating a possible role for FGF signaling in chicken (Atsuta and Takahashi, 2016) (Figure 2B). Additionally, the PI3K/Akt pathway is activated at this stage in rodents although its specific role has not been studied (Fujino et al., 2009). Müllerian invagination is controlled by Lim1 and Wnt4, expressed by mesoepithelial and mesenchymal progenitors respectively (Vainio et al., 1999; Kobayashi et al., 2004; Orvis and Behringer, 2007; Atsuta and Takahashi, 2016). In Lim1-null and Wnt4-null knock-out mice, epithelial invagination is blocked, although Lim1+/Wnt7a+ progenitors are detected in Wnt4-nulls suggesting that Wnt4 does not control mesoepithelial progenitors’ specification and that, likely, a two-axes mesenchymal-epithelial signaling is required for invagination (Kobayashi and Behringer, 2003; Kobayashi et al., 2004; Guioli et al., 2007; Prunskaite-Hyyryläinen et al., 2016). In chicken embryos, loss of Lim1 also blocks invagination (Atsuta and Takahashi, 2016).
Phase III: caudal elongation
Finally, the Müllerian architecture is achieved by two-dimensional growth. First, the developing Müllerian tip undergoes caudal elongation along the anteroposterior axis through apical constriction, cell proliferation and cranial-caudal cell migration (Gruenwald, 1941; Jacob et al., 1999; Kobayashi et al., 2004; Guioli et al., 2007; Orvis and Behringer, 2007; Fujino et al., 2009; Atsuta and Takahashi, 2016). Second, the deposited cells proliferate to expand the tube on the dorsoventral axis (Jacob et al., 1999; Guioli et al., 2007; Orvis and Behringer, 2007) (Figure 1C). Initially, the BM is shared between the Wolffian ducts and the Müllerian mesoepithelial tip. Subsequently, as the Müllerian duct elongates, the number of mesenchymal cells between the ducts increases, establishing a physical separation regulated by PI3k in rodents, which splits the female and male BMs (Gruenwald, 1941; Jacob et al., 1999; Hashimoto, 2003; Fujino et al., 2009). However, the Wolffian ducts remain in physical contact with the Müllerian proliferative tip, guiding and supporting its growth during the whole process, although Wolffian ducts do not contribute cells to the paramesonephric ducts (Gruenwald, 1941; Dohr and Tarmann, 1984; Hashimoto, 2003; Carroll et al., 2005; Kobayashi et al., 2005; Guioli et al., 2007; Orvis and Behringer, 2007). Murine Müllerian elongation depends on physical and Wnt9b- and Gata3-mediated paracrine interactions with the Wolffian duct. Both genes are expressed by the Wolffian epithelium. In mouse, Wnt9b controls Müllerian elongation through the canonical WNT pathway and the loss of Gata3 prompts elongation arrest (Carroll et al., 2005; Grote et al., 2008). Elongation also depends on the Gpr56 receptor expressed in the mesoepithelial progenitors (chicken), the mesenchymal expression of Wnt4 and Dmrt1 (chicken), the PI3K/Akt pathway (rodents) and coelomic epithelium-derived paracrine signals (chicken) (Gruenwald, 1941; Carroll et al., 2005; Kobayashi et al., 2005; Pedersen et al., 2005; Fujino et al., 2009; Atsuta and Takahashi, 2016; Prunskaite-Hyyryläinen et al., 2016; Roly et al., 2020a) (Figure 2B). This crosstalk between the coelomic epithelium and the elongating tube is evident, given that Wnt4 expression is activated along the chicken coelomic epithelium as elongation advances and that physical disruption of the coelomic epithelium blocks Müllerian development at the mechanical incision level (Fujino et al., 2009; Prunskaite-Hyyryläinen et al., 2016).
Overall, Müllerian duct development is a complex three-stage process encompassing key cellular mechanisms and its successful execution requires the coordinated action of several developmental pathways and genetic entities. Recently, a high-throughput bulk-RNA sequencing study of developing chicken Müllerian ducts, confirmed the aforementioned cellular mechanisms (Roly et al., 2020b). In this study, genes related to transcription and cell differentiation were differentially expressed during the early and the last stages of development, coinciding with the progenitors’ specification and the initial post-developmental differentiation respectively. Cell migration and negative regulation of apoptosis were upregulated at elongation whereas cell adhesion and cell proliferation mechanisms were enriched during all the developmental stages. After Müllerian development, the ducts merge on their caudal end and with the endoderm-derived urogenital sinus. It has been demonstrated that a transmembrane protein of unknown function, Lhfpl2 (lipoma HMGIC fusion partner-like 2), is behind the scenes as a point mutation in this gene prevents murine Müllerian tips to fuse with the urogenital sinus (Zhao et al., 2016).
Sex-Specified Wolffian Duct Regression, Müllerian Duct Maintenance and Anterior Differentiation Into Oviducts in Female Embryos
At the end of Müllerian development, the embryo displays dual gender phenotypic identity. Complete sex determination occurs afterward in two fundamental steps: (1) regression of the genetically unmatched reproductive tubes (Figure 1D) and (2) maintenance of the sex-matched ducts with their subsequent differentiation into adult reproductive organs (Figures 1E,F).
Throughout mammalian male sex differentiation, MIS (Müllerian-inhibiting substance) is secreted and binds to Misr2 (MIS type II receptor), activating the MIS signaling pathway that promotes Müllerian regression (Josso et al., 1993; Mishina et al., 1996; Kobayashi et al., 2004; Renfree et al., 2009). Misr2 is expressed in mouse Müllerian mesenchyme in both genders and is regulated by mesoepithelial-secreted Wnt7a (Baarends et al., 1994; Hacker et al., 1995; Parr and Mcmahon, 1998; Swain and Lovell-Badge, 1999; Kobayashi et al., 2004; Arango et al., 2008). Male mice bearing null mutations for MIS, Misr2 or Wnt7a impair Müllerian duct regression (Behringer et al., 1994; Mishina et al., 1996; Parr and Mcmahon, 1998). A recent study investigating these Misr2+cells in female rodents has shown that they are kept in the postnatal uterine stroma where they are hypothesized to function as progenitors (Saatcioglu et al., 2019). This would differ from the human given that these cells disappear from the Müllerian mesenchyme by week 37 (Saatcioglu et al., 2019). In XX mouse embryos, Müllerian ducts persist in the absence of MIS whereas Wolffian ducts are cleared through the action of the transcription factor COUP-TFII (or NR2F2), expressed by the mouse Wolffian mesenchyme (Zhao et al., 2017) (Figure 1D).
After female sex determination, the Müllerian ducts are simple tubes comprised of an immature lumen and mesenchymal layers that starting a differentiation program. Post-development, a mesenchymal signature of Hox genes (mouse, human, chicken) and retinoic acid (RA) signaling (mouse) segmentally marking the ducts can be observed (Taylor et al., 1997; Ma et al., 1998; Nakajima et al., 2016, 2019; Ghosh et al., 2017) (Figure 1E). The Hox/RA signature consists of differentially expressed RA-related and Hox genes (Hoxa9,10,11,13) subluminally segmenting the proximal and distal Müllerian duct (Raines et al., 2013; Nakajima et al., 2016, 2019). Each specified Müllerian section gives rise to a different FRT organ (Figures 1E,F) with the most anterior differentiating into the oviducts.
The differential retinoic acid signaling consists of a decreasing gradient from the proximal (highest signal) to the caudal (signal inhibition) Müllerian mesenchyme where inhibition is mediated by the RA-degrading protein Cyp26a1 in mouse (Nakajima et al., 2016, 2019). Tuned activation and absence of the RA-signaling pathway determines oviduct-uterine and vaginal differentiation, respectively (Nakajima et al., 2016, 2019). In the former, RA signaling is active from E14.5 (post-Müllerian development) to P0 in mouse, when gradually decreases until disappearance by P15 (Nakajima et al., 2016). Hox genes are first detected at E15.5 in mouse, although Hoxa10 has been documented in the middle duct at E14.5 (Taylor et al., 1997; Ma et al., 1998; Nakajima et al., 2016). At E16.5, Hoxa9/10/11 have a similar expression pattern, marking the upper and middle segments of the Müllerian duct; however, Hoxa9 extends further into half of the segment that will develop into oviducts. Conversely, Hoxa13 is upregulated in the caudal region, which differentiate into the cervix and upper vagina. Within the first 2 weeks of mouse life the Hox genes restrict their expression to their determined organ; Hoxa9 shows strong expression in the oviduct and low expression in the uterine horns, Hoxa10 is robustly expressed in the uterine horns, Hoxa11 is strongly expressed in the lower uterine horns and endocervix, and Hoxa13 is moderately expressed in the uterine cervix and highly expressed in the ectocervix and upper vagina (Taylor et al., 1997; Ma et al., 1998) (Figure 1F). The HoxD cluster (Hoxd9/10/11) follows a similar fashion to their Hoxa paralogs (Dollé et al., 1991). This gene signature is conserved during adulthood in mouse, chicken and human. Additionally, Müllerian luminal differentiation has also been shown to be marked by mesoepithelial–epithelial transitions (MOETs). After female Müllerian development (where luminal cells retain a mesothelial phenotype), the epithelial marker E-cadherin is gradually expressed in female mouse luminal cells and they acquire epithelial apicobasal polarity (Orvis and Behringer, 2007; Stewart et al., 2013; Prunskaite-Hyyryläinen et al., 2016; Ghosh et al., 2017). These processes represent conserved MOETs observed in many species such as the human, mouse, chicken, quail, duck, goose, and rat (Dohr and Tarmann, 1984; Jacob et al., 1999). It is currently unknown whether this mechanism is derived from mesenchymal-specification signaling or is a cell-autonomous process that the Müllerian mesothelium undertakes.
Specific oviduct differentiation has been shown to rely on epithelial–mesenchymal interactions as demonstrated by mouse heterotypic recombinant assays at postnatal stages, where the epithelial identity of each Müllerian segment is determined by the associated mesenchyme (Yamanouchi et al., 2010). Mouse oviduct epithelium which has undertaken fate-decision endpoints has been detected at 3/4 weeks based on electron microscopy experiments and heterotypic recombinant assays (Komatsu and Fujita, 1978; Yamanouchi et al., 2010). Recombinant studies have also been performed with the other reproductive organs, leading to the same conclusions (Cunha, 1976; Kurita et al., 2001).
The proximal mouse Müllerian section with oviduct fate accounts for the highest RA activity (Nakajima et al., 2016), which suggests a role for this pathway in the differentiation mechanism. Cultured proximal Müllerian ducts at E14.5 differentiate into oviduct-like epithelial cells whereas middle Müllerian ducts differentiate into uterine-like epithelium (Nakajima et al., 2016). Inhibition of RA signaling through the pan-RAR antagonist AGN193109 in cultured anterior mouse Müllerian ducts induced differentiation of Trp63+vagina-like epithelia, and treatment with RA and AGN193109 rescued the oviduct epithelium phenotype and blocked the vagina-like induction (Nakajima et al., 2016). Taken together, it seems that a reversible fate decision has been made in the ducts at this embryonic point, although further research would be necessary to support this hypothesis. Regarding the specific role of Hox genes in oviduct patterning, no reproductive tract aberrations have been reported in mice lacking Hoxa9/d9 (Fromental-Ramain et al., 1996; Raines et al., 2013). Hoxa10-null mice display anteriorization of the proximal first quarter of the uterine horns into oviducts, as well as homozygous Hoxa11 knockouts (Satokata et al., 1995; Benson et al., 1996; Gendron et al., 1997). The expression of several genetic identities has been reported to influence oviduct differentiation. Reduction in Wnt4 expression leads to aberrant mouse oviduct formation, whereas lack of Wnt7a prompts oviductal posteriorization with abnormal elongation and lack of coiling (Miller et al., 1998; Parr and Mcmahon, 1998; Vainio et al., 1999; Prunskaite-Hyyryläinen et al., 2016). Ctnnb1 knocked-out Müllerian mesenchymal cells also trigger uncoiled oviducts, and its continuous stabilization prompts a failure in oviduct differentiation by impeding the expression of E-cadherin in the differentiating mesoepithelial Müllerian lumen (Deutscher and Hung-Chang Yao, 2007; Stewart et al., 2013). This suggests that fine tuning of the canonical WNT pathway is required for oviduct differentiation and that given the identical knockout phenotypes between Wnt7a knockouts and mesenchymal-specific Ctnnb1 knockouts, Wnt7a could be a WNT effector candidate in this context. The cofactors Dach1/2 have also been proposed as contributors to oviductal differentiation given the hypoplastic oviduct in Dach2–/–; Dach1+/– females (Davis et al., 2008). Furthermore, an interesting event deserving additional investigation could be the differential epithelial Lim1 expression that extendedly remains in the anterior mouse Müllerian region when it has disappeared from the rest of the duct (Kobayashi et al., 2004).
At birth, mouse oviductal epithelial cells are distinguished by the expression of Pax8 (Ghosh et al., 2017, 2020) (Figure 3). These Pax8+cells can self-renew and differentiate into Pax8– ciliated cells by P3–P4 (Shi et al., 2014; Ghosh et al., 2017). After the first 2 weeks of life, the adult mouse oviducts become fully distinguishable and mature exhibiting four differentiated regions conserved across species; the isthmus, ampulla, infundibulum, and fimbriae (Figure 4). The mature human and mouse epithelium is composed of Pax8+secretory, Pax8– Caps+ ciliated and intermediate secretory-ciliated cell subtypes (Hu et al., 2020). The Wnt non-canonical planar cell polarity (PCP) pathway has been reported to control diverse aspects of the mouse oviduct epithelial polarity and organ morphology through the action of Celsr1 from P2 to adulthood (Shi et al., 2014). In this study, the oviduct expression of other PCP pathway-related genes (Celsr2, Vangl1/2, Dvl1/2, Pk2/3, Fz1-3/6/8 and Ptk7) was detected and further investigation on these entities could contribute to determine this pathways’ complete effector network. The role of Vangl2 controlling cell polarity in the mouse uterine horns has been previously shown, although the effects in the oviduct epithelial polarity has not been reported (Vandenberg and Sassoon, 2009). However, the authors did report a lack of oviduct coiling in mice with mutated Vangl2, which could be potentially linked to a collateral 50% reduction in Wnt7a expression (Vandenberg and Sassoon, 2009). Also, it would be valuable to study if this PCP pathway-dependent epithelial polarization develops at earlier stages, for example, at the differentiation time of Müllerian luminal MOETs.
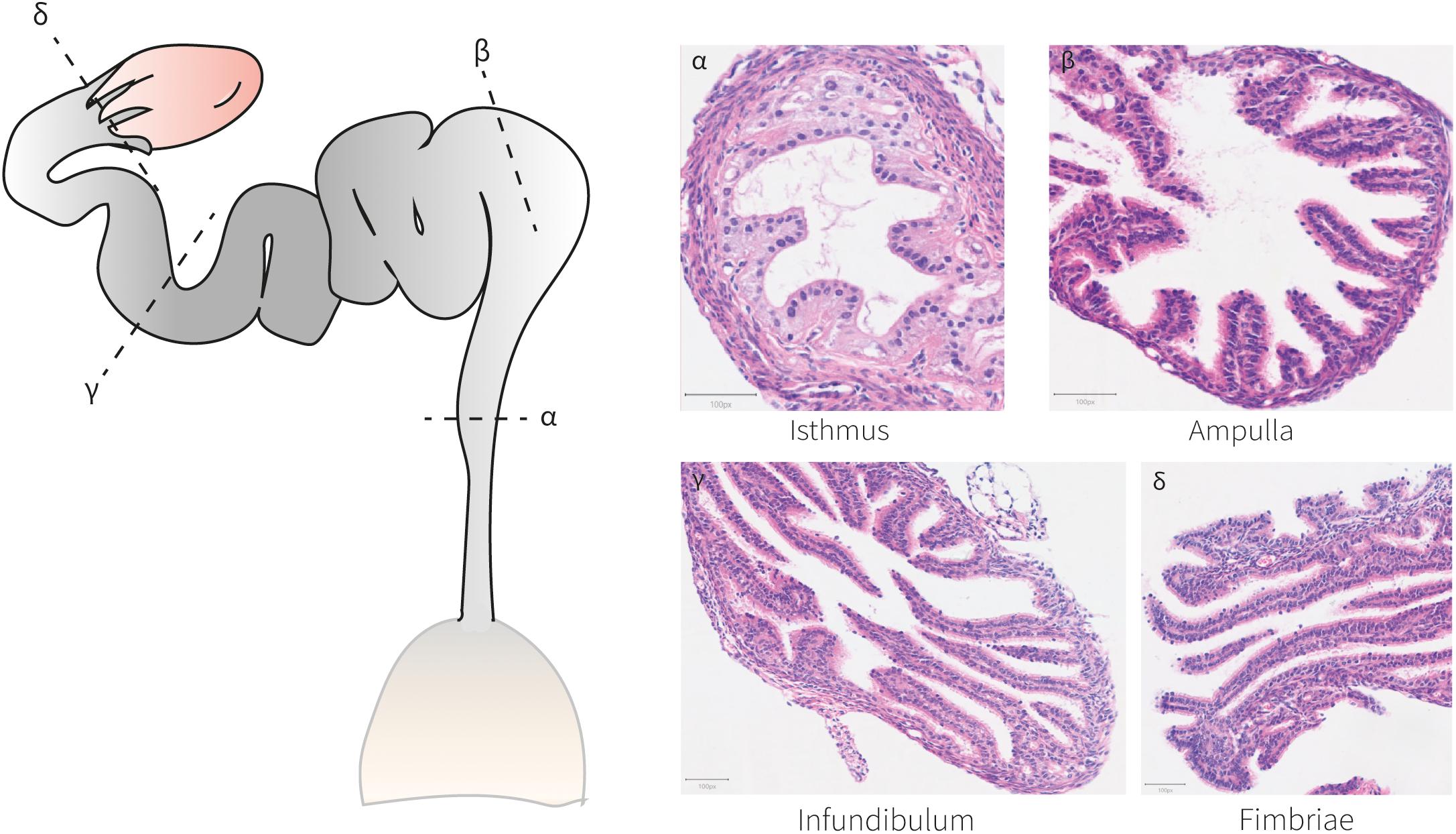
Figure 4. Histo-anatomical characterization of murine oviducts. Hematoxylin and eosin staining of oviduct sections shows the histo-anatomical pattern of the isthmus, ampulla, infundibulum and fimbriae following the posterior-anterior axis. The epithelium establishes luminal protrusions surrounded by a stromal and a smooth muscle layer.
Genetics and Molecular Biology of Müllerian Duct Development and Differentiation
Several gene families regulate Müllerian duct development and oviduct differentiation. In this section, published genes with a role/phenotype in these processes are presented and discussed (Table 1). Additionally, our genome-wide survey of mouse knockouts identified novel genes potentially involved in Müllerian duct development and oviduct physiology and/or integrity (Supplementary Table 1).
Pax2/8
Pax2/8 are master embryonic factors from the highly conserved Pax family, characterized by a paired box DNA-binding domain. Pax2/8 belong to the Pax2/5/8 (group II) subfamily which share a highly similar sequence containing a paired box domain, a partial homeodomain lacking a DNA-binding sequence and an octapeptide domain. Previous to coelomic mesoepithelial progenitor specification, Pax2 is expressed in some mesonephric coelomic cells in mouse and chicken (Torres et al., 1995; Vainio et al., 1999; Pedersen et al., 2005; Guioli et al., 2007; Davis et al., 2008; Atsuta and Takahashi, 2016). In chickens, high levels of Pax2 expression have been documented during the three developmental stages (Roly et al., 2020b). Pax8 is expressed in the Müllerian duct from its initial development to later adult stages and mice bearing Pax8 mutations present a normal Müllerian phenotype (Vainio et al., 1999; Bouchard et al., 2004; Carroll et al., 2005). In Pax2-null mice, Müllerian progenitor cells undergo initial invagination (Torres et al., 1995; Kobayashi et al., 2004) but do not elongate due to the absence of Wolffian ducts. In chickens, Pax2 downregulation blocks Müllerian duct invagination (Atsuta and Takahashi, 2016). These opposite phenotypes are discussed later when data involving Pax8 and Lim1 are presented. In humans, the PAX2 landscape is more complex. A PAX2 polymorphism and a synonymous mutation have been linked to different Müllerian duct anomalies and a patient carrying a bicorporeal uterus and a double cervix, respectively (Wang et al., 2012; Xu et al., 2017). Furthermore, increased expression of this gene related to its hypomethylation was correlated to a septate uterus phenotype (Wang et al., 2020). This suggests that PAX2 might have a role in Müllerian development/differentiation in humans although this has not been conclusively proved as no mutations in this gene have been reported in Müllerian agenesis cases to date.
Lim1 and Hnf1b
Lim1 is a homeobox transcription factor with two amino-terminal cysteine-histidine-containing LIM domains that bind iron and zinc and a DNA-binding homeodomain (Barnes et al., 1994). In mice, its transcript levels peak in the embryo at E11.5 chronologically corresponding to Lim1-mediated coelomic progenitor specification (Barnes et al., 1994; Kobayashi et al., 2004). Lim1 expression strongly persists during chicken and mouse Müllerian development (Kobayashi et al., 2004; Roly et al., 2020b). From E16.5–17.5, Lim1 expression is restricted to the Müllerian anterior axis before complete disappearance (Kobayashi et al., 2004; Davis et al., 2008). In Lim1-null mouse and Lim1-downregulated chicken embryos, Müllerian invagination is blocked (Kobayashi et al., 2004; Atsuta and Takahashi, 2016). In humans, the same deletion in chromosome 17 encompassing LIM1 causes Müllerian agenesis, also known as Mayer-Rokitansky-Küster-Hauser (MRKH) syndrome (Bernardini et al., 2009; Ledig et al., 2011; Nik-Zainal et al., 2011; Sandbacka et al., 2013; McGowan et al., 2015; Waschk et al., 2016). The phenotypes within MRKH patients are variable, for example, two different patients in the same study presented, the first, uterine and vaginal agenesis and the second, absence of the upper vagina with unicornuate uterus and bilaterally multicystic kidneys (Bernardini et al., 2009). This deletion is also the cause of a case of uterine fusion anomaly (Ledig et al., 2018). These are typical examples of mutations with incomplete penetrance and variable expressivity, which suggests that additional genetic and non-genetic factors control full penetrance in the human phenotypes. This deletion also includes the geneHNF1β, a POU homeodomain transcription factor expressed in the mesoepithelial progenitors during mouse Müllerian duct development as well as in the adult oviducts and uterine horns. Mice with homozygotic mutations in Hnf1b do not develop Müllerian ducts (Lokmane et al., 2010). Human mutations in LIM1 have been associated with MRKH syndrome (Ledig et al., 2011, 2012; Sandbacka et al., 2013) whereas mutations in HNF1β have been related to fusion anomalies (Bingham et al., 2002).
In chicken embryos with shRNA-mediated Pax2 knockdown, Lim1 expression is dramatically decreased, hindering Müllerian duct invagination (Atsuta and Takahashi, 2016). In contrast, Lim1 is still expressed in Pax2-null mouse Müllerian progenitor cells, and invagination occurs (Torres et al., 1995; Kobayashi et al., 2004). Additionally, Lim1 mRNA levels are greatly reduced in the mesonephros of Pax2–/–/Pax8+/– mice (Boualia et al., 2013), suggesting that Pax2/8 might regulate Lim1. This potential regulation mechanism has not been elucidated but direct interaction between Lmx1b, another Lim-homeodomain family member, and Pax2 was proven by yeast two-hybrid assays and co-immunoprecipitation (Marini et al., 2005). The homeodomain was found to be essential for this event. Moreover, Pax2 directly binds Lim1 enhancer regions in a Wolffian cell line model (Boualia et al., 2013). The apparent contradictory conclusions from the murine and chicken Pax2-deficient models, i.e., invagination/non-invagination outcomes upon Lim1 regulation, could be explained by Pax8 compensation. The mouse Pax2 phenotype could be rescued by Pax8 acting redundantly, whereas unlike in mammals, the chicken genome does not contain Pax8. This theory could be supported by two facts, (1) Pax2 and Pax8 are paralogous genes in the same subfamily and share the highest percentage of sequence similarity within Pax members, (2) Pax2/8 functional redundancy and synergistic cooperation with Lim1 was previously shown in renal system development in Xenopus and mice (Carroll and Vize, 1999; Bouchard et al., 2002).
Emx2
Emx2 is a homeobox gene expressed in the mouse mesonephric coelomic epithelium at E10-11, although by E12-13 is restricted to the forming Müllerian mesoepithelium (Miyamoto et al., 1997; Pellegrini et al., 1997; Grote et al., 2008). In chicken, Emx2 is highly expressed in the Müllerian ducts during their development (Roly et al., 2020b). Emx2-null mice completely lack Müllerian ducts (Miyamoto et al., 1997). In the Wolffian ducts, Pax2 is a direct transcriptional regulator of Emx2 (Pedersen et al., 2005; Boualia et al., 2011), which could potentially be conserved in the Müllerian ducts. Emx2 expression persists into mouse and human adulthood, is regulated by HoxA10 and is involved in the reproductive hormone cycle, blastocyst implantation and endometriosis (Du and Taylor, 2004, 2015).
Wnt4/Wnt7a/Wnt5a/Wnt9b/Ctnnb1
The WNT family of secreted glycoproteins is extensively involved in embryonic developmental processes, adult tissue differentiation and homeostasis. Concretely, Wnt4, Wnt5a, Wnt7a, and Wnt9b are WNT elicitors with an important role in Müllerian development and oviduct differentiation. In mouse and chicken, Wnt4 is expressed in the Müllerian ridge before invagination and, subsequently, in the coelomic epithelium and Müllerian mesenchymal cells where the expression is maintained throughout fetal life (Vainio et al., 1999; Ayers et al., 2015; Prunskaite-Hyyryläinen et al., 2016). Wnt4-null mice show no Müllerian duct invagination and lack Müllerian structures (Vainio et al., 1999; Kobayashi et al., 2004; Orvis and Behringer, 2007; Prunskaite-Hyyryläinen et al., 2016), although Wnt4 is also required during elongation (Prunskaite-Hyyryläinen et al., 2016). Conditional knockout of Wnt4 and Wnt5a from mouse mesenchymal cells occasionally causes Müllerian aplasia as well (St-Jean et al., 2019a).
Mutations in WNT4 have been found in patients with MRKH and hyperandrogenism (Biason-Lauber et al., 2004, 2007; Philibert et al., 2008, 2011). However, the individual role of this gene is not well delimited as WNT4 mutations in MRKH patients not exhibiting hyperandrogenism could not be found (Ravel et al., 2009; Gervasini et al., 2010; Chang et al., 2012) and not all MRKH patients with hyperandrogenism have WNT4 mutations.
Wnt7a is expressed in the murine Müllerian duct mesoepithelium throughout and after development, being restricted to the oviduct and uterine epithelium after birth (Miller et al., 1998; Vainio et al., 1999; Davis et al., 2008; Prunskaite-Hyyryläinen et al., 2016). In Wnt7a-null mice, the Müllerian duct develops, although the oviducts and uterine horns suffer posteriorization, where oviducts resemble uterine horns, and these acquire vaginal characteristics, promoting the postnatal loss of Hoxa10/11 (Parr and Mcmahon, 1998; Miller et al., 1998). Oviductal regional differentiation occurs in Wnt7a-nulls, but coiling and normal elongation do not (Parr and Mcmahon, 1998). Its interaction with other Müllerian effectors is unknown but Wnt7a regulation of Lim1 in the Müllerian duct has been discarded (Kobayashi et al., 2004). Furthermore, Wolffian mouse epithelium expresses Wnt9b during E9.5–14.5 to control Müllerian duct elongation by canonical WNT signaling (Carroll et al., 2005; Pedersen et al., 2005). In Wnt9b mutants, normal Müllerian development takes place until elongation arrest (Carroll et al., 2005). In Wolffian-specific Lim1 conditional knockout embryos, Cdh1 (E-cadherin) and Wnt9b expression is significantly reduced, triggering the same phenotype, and suggesting Wolffian Lim1-regulation of these genes (Pedersen et al., 2005). In humans, the importance of WNT9B in Müllerian formation and differentiation has proved inconclusive. On one hand, several WNT9B mutations in patients with Müllerian duct anomalies (Wang et al., 2014; Waschk et al., 2016) and a polymorphism linked to MRKH risk (Ma et al., 2015) have been reported. On the other hand, WNT9B was ruled out as a causative factor in 542 Chinese patients with Müllerian aberrations (Tang et al., 2014).
Furthermore, the expression of Wnt16, Wnt6, Wnt7b, Wnt2, and Wnt10a has been documented in the chicken developing Müllerian duct and they represent potential WNT candidates to be further explored (Roly et al., 2020b).
Retinoic Acid Receptors
Retinoic acid is a molecule derived from vitamin A that plays a crucial role in embryonic development (Mendelsohn et al., 1994). RA signaling is transduced by RARs and RXRs (retinoid X receptors), which are important during Müllerian duct development. Rarα−/−/Rarβ2–/– mice block Müllerian development at E12.5 even though Wolffian ducts developed (Mendelsohn et al., 1994). The genotype Rarα−/−/Rarγ–/– triggers the loss of the mouse Müllerian caudal end due to loss of the Wolffian caudal end (Mendelsohn et al., 1994). Furthermore, Rxrα−/−/Rarα–/– lead to a loss of Müllerian ducts (Kastner et al., 1997). These observations suggest functional redundancy. Furthermore, the Rara and Rarb genes were found to be highly expressed in the developing Müllerian duct at constant levels along the three stages (Roly et al., 2020b). RA signaling has also been shown to play a role in Müllerian differentiation and the retinol dehydrogenase Rdh10 as well as the RA-synthesis enzyme Aldh1a2 regulated by the transcription factor C/ebpδ have been proposed to be the molecules acting in this pathway (Nakajima et al., 2016, 2019). More research to support these findings would be needed to unravel the complete RA signaling regulatory network. At post-birth stages, Rara, Rarg, Rxra, and Rxrb are expressed in the mouse uterus and vagina and it would be interesting to test whether this is conserved in the oviducts (Nakajima et al., 2016). Furthermore, some level of indirect Rar regulation on Hoxa10 has been proposed, given that Rar does not bind to the Hoxa10 promoter but RA-Rar signaling increased Hoxa10 expression (Nakajima et al., 2016).
Hox Genes
Hox genes are master embryonic regulators across animals. In vertebrates, they are organized into four unlinked genomic clusters, HoxA/B/C/D, located on chromosomes 6/11/15/2 in mouse; 7/17/12/2 in human; and 2/3/1/7 in chicken. They display temporal-spatial collinearity, meaning they are expressed in a regulated manner in time and space in the embryonic body according to their physical organization on the chromosome: genes nearer the 3′ end are expressed earlier and more anteriorly in the embryo than the next gene in the cluster. In adult mice, chickens, and humans, the Hoxa9/10/11/13 and Hoxd9/10/11/13 genes are expressed in the FRT mesenchyme following this spatial-specific pattern (Hoxa9/d9 in oviducts, Hoxa10/d10/a11/d11 in uterine horns and Hoxa13/d13 cervix and vagina) (Taylor et al., 1997; Ma et al., 1998; Raines et al., 2013). These genes have been shown to control the FRT segmentation (Satokata et al., 1995; Benson et al., 1996; Gendron et al., 1997; Raines et al., 2013; Ashary et al., 2020). Functional redundancy has been suggested for the A and D clusters in the FRT, with stronger contribution from the HoxA cluster (Fromental-Ramain et al., 1996; Raines et al., 2013). Furthermore, redundancy and inter-regulation have been suggested among the Hoxa10 and Hoxa11 genes (Branford et al., 2000). HoxA genes are mainly expressed in the reproductive mesenchyme, although epithelial expression occurs during pregnancy initiation and the steroid hormone cycle (Benson et al., 1996; Ma et al., 1998). Hox genes are regulated by steroids through direct interaction with estrogen and progesterone receptors, which bind Hox regulatory elements (Gendron et al., 1997; Ma et al., 1998). Studies on Hoxa10/11 genes reported a role not only during Müllerian duct differentiation but also blastocyst implantation and development, the hormone cycle, endometriosis and hydrosalpinx (Benson et al., 1996; Gendron et al., 1997; Du and Taylor, 2004, 2015; Xu et al., 2014; Ashary et al., 2020). Furthermore, a single-cell RNA sequencing study of Hox-mutant developing uterine horns has suggested a possible mechanism of action for these genes through the control of the Wnt and Cxcl12/Cxcr4 ligand/receptor pathways (Mucenski et al., 2019).
Other Genes
The Dach/dachshund genes are a conserved family of transcriptional cofactors. At E12.5–13.5, Dach1/Dach2 are strongly expressed in the mouse Müllerian duct mesoepithelium (Davis et al., 2008). Dach1 or Dach2-null mutants display a normal phenotype, but when both genes are mutated, Müllerian duct development is abnormal, suggesting functional redundancy as double heterozygotes appear normal (Davis et al., 2008). Dach1/2 regulate Lim1 and Wnt7a, as their expression is nearly depleted in Dach2–/–; Dach1+/– and Dach2+/–; Dach1–/– females, and hypoplasia is evident in the FRT, with increasing severity along the anterior-posterior axis (Davis et al., 2008).
Dmrt1 is a transcription factor containing a zinc finger-like DNA-binding domain (DM domain) expressed in the chicken Müllerian coelomic placode. As duct development advances, it becomes expressed in Müllerian mesenchymal cells until ∼HH35 (Ayers et al., 2015). When Dmrt1 is knocked down, Müllerian ridge thickening and mesenchymal cell production are compromised, often blocking elongation owing to the lack of mesenchymal cells (Ayers et al., 2015).
Lats1/2 (Large tumor suppressor kinase 1/2) are Ser/Thr kinases of the Hippo pathway acting as repressors of the transcriptional cofactors Yap and Taz (St-Jean et al., 2019b). The Hippo pathway, through the action of Lats1/2, is essential for maintaining Müllerian mesenchymal cell pluripotency. Conditional deletion of these genes results in increased aberrant expression of Yap, Taz and the Ctgf transcription factor, activating the myofibroblast differentiation program and causing aberrations in the Müllerian ducts and oviducts (St-Jean et al., 2019b). The gross defect in Müllerian ducts is an abnormal coiling during elongation which provokes the creation of multi-lumens in adult organs (St-Jean et al., 2019b). Defects in adult oviducts involved severe dilatation, caudal cysts that ruptured by adulthood breaking the oviduct in pieces (St-Jean et al., 2019b). However, a controlled expression of Taz and Yap might be necessary given that conditional inactivation of the Müllerian mesenchymal Taz and Yap triggers postnatal cystic aberrations on the oviduct smooth muscle layer impairing their reproductive function (Godin et al., 2020).
Gpr56 is an adhesion G protein-coupled receptor critical for chicken Müllerian elongation (Roly et al., 2020a). This protein is coded by the Adgrg1 gene, is first expressed in chicken coelomic epithelium progenitors during invagination, and its expression is maintained in Müllerian luminal cells until later post-developmental stages (Roly et al., 2020a). When its expression is knocked down, the number of proliferative cells decreases in vivo, whereas its overexpression in a chicken fibroblastic cell line prompts an increase in cell proliferation and migration (Roly et al., 2020a), which suggest Gpr56 control on cell proliferation and migration during Müllerian duct elongation. Furthermore, this receptor family could play further or the same roles through different family members given that expression of G protein-coupled receptors is upregulated during Müllerian duct formation, with Adgrd1 showing one of the highest expression levels at the last stages of development (Roly et al., 2020b).
Tgfbr1 is the type 1 receptor for TGF-β ligands controlling the transduction of the TGF-β signaling pathway. This pathway is transduced through intracellular SMAD proteins. In mouse oviducts, Tgfbr1 is expressed in the epithelium, mesenchymal and smooth muscle cells (Li et al., 2011). Conditional knockout of Tgfbr1 in Amhr2+ mesenchymal Müllerian cells produces adult oviducts with cystic structures as a result of defects in smooth muscle development (Li et al., 2011). These structures prevented the reproductive function as seen by unsuccessful preimplantation embryo development and transport to the uterine horns. Furthermore, members of the TGF-β signaling pathway (Tgf-β1/2/3 and their corresponding receptors) have been identified in the developing chicken Müllerian duct and further investigation would reveal whether this pathway plays a role at early developmental stages as well (Roly et al., 2020b).
The gene Dicer1 encodes an RNase III (Dicer) that processes microRNAs that modulate gene expression in the cytoplasm. In mice with Amhr2-Cre mediated conditional deletion of Dicer1 (this RNase is missing in Müllerian mesenchymal cells), the oviducts present aberrations related to anomalies in the smooth muscle layer (Hong et al., 2008; Gonzalez and Behringer, 2009). In this model, the oviducts are shortened, cystic and the epithelium lacks organization. Furthermore, the isthmus presents a great loss of the smooth muscle layer and absence of epithelial folds. Successful reproduction is halted in these mice and most of the blastocysts were found near the ampulla, where fertilization happens, and never made it to establish a pregnancy in the uterine horns. Dicer regulates several microRNAs that are algorithm-predicted to regulate the expression of several genes related to Müllerian development and qPCR analysis demonstrated that the genes Hoxa9, Hoxa10, Wnt5a, Wnt7a were upregulated after Dicer1 deletion in the Müllerian mesenchyme (Nagaraja et al., 2008).
Genome-Wide Screening of Gene Knockouts
Lastly, we carried out an extensive genome-wide interrogation of mouse knockout lines reporting mutant phenotypes in Müllerian ducts/oviducts using the MGI (Mouse Genome Informatics) and DMDD (Deciphering the Mechanisms of Developmental Disorders) databases (Mohun et al., 2013; Smith et al., 2019). Ninety-five mouse knockout lines registered Phenotype Ontology Annotations (POA) affecting the Müllerian ducts (69.5%) and oviducts (30.5%) (Figure 5A). The mutant phenotypes with the highest incidence are abnormal Müllerian duct and oviduct morphology, abnormal Müllerian duct topology and absence of Müllerian ducts and oviducts (Figure 5B). Although the penetrance of most mutations was unknown, several genes previously unreported displayed significant penetrance, ranging from 33.3% for Cir1 (Corepressor interacting with Rbpj, 1) and Rpgrip1 (Rpgrip1-like) to 50% for Actn4 (Alpha-actinin-4) and Anks6 (Ankyrin repeat and sterile alpha motif domain containing 6), 72.7% for Sh3pxd2a (Sh3 and Px domains 2a) and 100% for B9d2 (B9 domain containing 2), Greb1l (Greb1-like retinoic acid receptor coactivator), and Nxn (Nucleoredoxin) (Supplementary Table 1). Wolffian duct abnormalities might be behind the Cir1, Anks6, and Greb1l Müllerian phenotypes. We used the Database for Annotation, Visualization and Integrated Discovery (DAVID) v6.8 (Huang et al., 2009) for extracting the biological meaning of these genetic entities. The analysis showed that Sh3pxd2a, B9d2, Rpgrip1, and Anks6 are cilium-related proteins involved in cell projection whereas Cir1 is a repressor of the Notch signaling pathway. Although literature search of the possible relationship of these genes to Müllerian/oviduct biology was mostly unsuccessful, a link for Greb1l was found. In a Greb1l knock-out mouse, Müllerian ducts are missing (De Tomasi et al., 2017) and a study using whole-genome sequencing in patients with Müllerian agenesis found possible causative mutations in the GREB1L gene (Jacquinet et al., 2020). Greb1l has been proposed as a coactivator of RA-RAR mediated transcription in a study using zebrafish and mouse (Brophy et al., 2017) and a similar protein, GREB1, has been found to form a chromatin complex with estrogen receptor (ER) and RARs, among others, and to be indispensable for ER-mediated transcription in human cells (Mohammed et al., 2013).
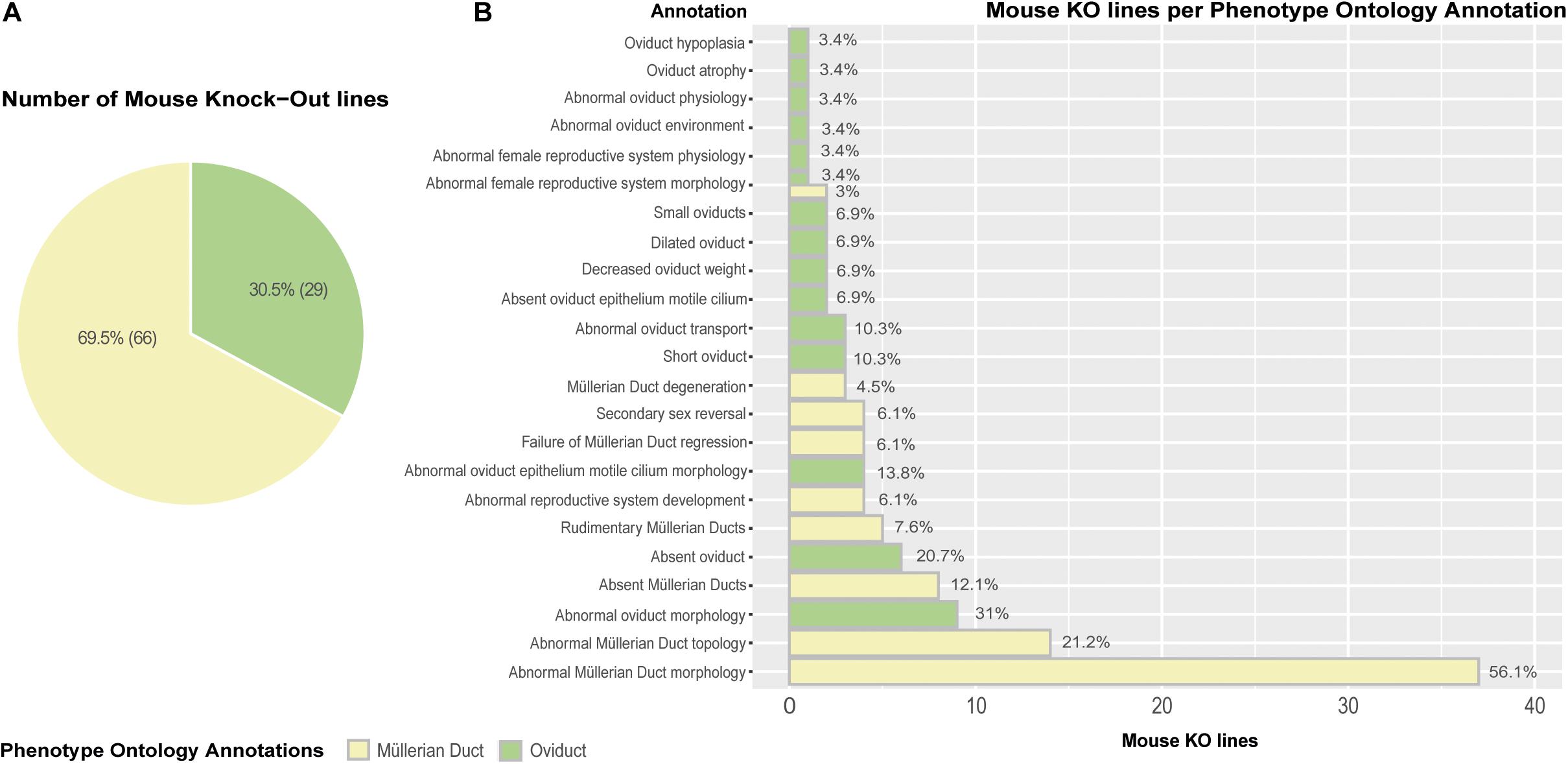
Figure 5. Mouse knock-out (KO) lines expressing Phenotype Ontology Annotations (POA) associated to Müllerian ducts/oviducts. (A) Pie chart representing KO lines in the MGI database registering at least one POA related to Müllerian duct/oviduct. (B) Barplot depicting the number of KO lines per annotation. Percentages are calculated as the number of mouse KO lines displaying a Phenotype Ontology Annotation out of the total number of mouse KO lines per POA type.
In our search, knockout mice for widely reported Pax2/8, Lhx1, Emx2, Hox genes, Wnt4/7a/9b, and RARs, were also found. These mutant phenotypes correspond with literature reports, and they are among those genes registering mutant annotations of highest incidence and impact (Supplementary Table 1).
Discussion
The Müllerian ducts form during embryonic development and anteriorly differentiates into the oviducts. In this review, a plausible model for Müllerian development and oviduct differentiation is proposed. In this model, Pax2+/8+/Emx2+/Lim1+ mesonephric coelomic epithelial cells undergo pEMT into Müllerian mesoepithelial cells, whereas Dmrt1+/Wnt4+ populations undergo EMT into Müllerian mesenchyme. The mesoepithelial progenitors give rise to the lumen through invagination controlled by cell intercalation-mediated apical contraction and subsequent rostral-caudal elongation in intimate contact with the Wolffian ducts. The BMP and FGF/RAS/MAPK signaling pathways have been shown to regulate, at least, early Müllerian developmental stages, but it would be necessary to establish their role in later developmental, differentiation and homeostatic stages. Elongation is regulated by several factors including mesonephric coelomic epithelium signaling, Wolffian-dependent canonical WNT pathway, PI3K/Akt pathway and Müllerian expressed genes. Signaling mediated by G protein-coupled receptors has been reported at these stages in chickens (Roly et al., 2020a, b). Furthermore, the TGF-β signaling pathway has been shown to play a role in Müllerian differentiation.
After Müllerian duct formation, mesoepithelial progenitors undergo MOETs, differentiating into true uncommitted epithelium before birth during a similar time window in which the mesenchymal Hox/RA signaling axis is established. RA signaling is required for oviduct and uterine differentiation and blocked for the establishment of vaginal epithelium. The primitive oviduct-committed epithelium further differentiates, as seen in the new-born mouse oviduct, where epithelial Pax8+ progenitors can self-renew and differentiate into ciliated and secretory epithelial cell subtypes. Committed oviduct cell types appear within the first weeks of life when full regional differentiation has been undertaken. The non-canonical Wnt PCP pathway has been established as the regulator of the epithelial polarization at these differentiation and adult stages. Interestingly, when smooth muscle differentiation is perturbed in models involving genes truncated in the Müllerian mesenchyme (e.g., Lats1/2, Taz and Yap, Tgfbr1, Dicer), there seems to be a common phenotype in the oviducts exhibiting fluid-filled cysts impeding the reproductive function.
A preliminary gene regulatory network (GRN) underlying Müllerian development and differentiation into oviducts has been described, and the components of this network have been hierarchically related. Furthermore, genome-wide survey of mouse knockout lines identified new genes involved in Müllerian and oviduct biology, which would benefit from further investigation. Some interesting questions to answer regarding the role of these new candidates in Müllerian/oviduct biology would be: (1) Do these genes interact with any of the genes in the Müllerian GRN? (2) What are their expression patterns and functions in Müllerian ducts development and oviduct differentiation and adult homeostasis? (3) Are there some gyneacological pathologies related to the identified genes in the female reproductive tract?
More research is also needed on the factors that trigger Müllerian placode formation and later developmental stages, molecular candidates in these networks, upstream regulators, downstream effector circuits, and the relationships among members. The current lack-of-function models and experiments could prove very informative for potential future studies. Furthermore, there is limited research on prenatal Müllerian differentiation into oviducts. In more studied reproductive organs such as the vagina, important investigations of the mesenchymal-epithelial interactions during Müllerian differentiation have allowed the discovery of the GRN underlying this process. In the vagina, the Bmp4/Smad, ActA/Runx1, and Fgf7/10/Fgfr2IIIb/Mapk pathways independently specify Müllerian differentiation into the vaginal epithelium (Laronda et al., 2013; Terakawa et al., 2016, 2020). This is an elegant example of mesenchymal-epithelial interactions where the mesenchymal-secreted factors BMP4, FGF7/10 and Activin A specify the immature caudal Müllerian epithelium into vaginal epithelium. Studies like this in the oviducts would greatly advance our understanding of oviduct-specific Müllerian differentiation. Investigation on postnatal oviduct morphogenesis and genetic programs, cell identities and signaling pathways underlying these changes are scarce and would represent an exciting new direction on the field. Additionally, information on the differentiation/maintenance programs executed by adult oviduct epithelial cells is also lacking. Finally, taken together, this review could serve as a guide to future research choices aimed at improving our understanding of Müllerian developmental processes, oviduct differentiation, FRT biology and their associated pathologies.
Author Contributions
LS: conceptualization, methodology, investigation, and writing – original draft. IR, MM, MA, ZH, NW, AbA, AsA, TS-S, and AAA: writing – review and editing. LS and IR: visualization. AAA: supervision. All authors contributed to the article and approved the submitted version.
Funding
This work was supported by funding from the Biotechnology and Biological Sciences Research Council (BBSRC) (grant number BB/M011224/1), the Ovarian Cancer Action (OCA), and Oxford Biomedical Research Centre (OxBRC), National Institute for Health Research.
Conflict of Interest
The authors declare that the research was conducted in the absence of any commercial or financial relationships that could be construed as a potential conflict of interest.
Acknowledgments
We thank BioRender.com for their help in creating some figures sections. We also thank Dr. Massimo Masiero for his revision and corrections on the figures and Dr. Mohammad Karami Nejad Ranjbar for his input and help.
Supplementary Material
The Supplementary Material for this article can be found online at: https://www.frontiersin.org/articles/10.3389/fcell.2021.605301/full#supplementary-material
References
Arango, N. A., Kobayashi, A., Wang, Y., Jamin, S. P., Lee, H. H., Orvis, G. D., et al. (2008). A mesenchymal perspective of Müllerian duct differentiation and regression in Amhr2-lacZ mice. Mol. Reproduct. Dev. 75, 1154–1162. doi: 10.1002/mrd.20858
Ashary, N., Laheri, S., and Modi, D. (2020). Homeobox genes in endometrium: from development to decidualization. Int. J. Dev. Biol. 64, 227–237. doi: 10.1387/ijdb.190120dm
Atsuta, Y., and Takahashi, Y. (2016). Early formation of the Müllerian duct is regulated by sequential actions of BMP/Pax2 and FGF/Lim1 signaling. Development 143, 3549–3559. doi: 10.1242/dev.137067
Ayers, K. L., Cutting, A. D., Roeszler, K. N., Sinclair, A. H., and Smith, C. A. (2015). DMRT1 is required for Müllerian duct formation in the chicken embryo. Dev. Biol. 400, 224–236. doi: 10.1016/j.ydbio.2015.02.001
Baarends, W. M., van Helmond, M. J., Post, M., van der Schoot, P. J., Hoogerbrugge, J. W., de Winter, J. P., et al. (1994). A novel member of the transmembrane serine/threonine kinase receptor family is specifically expressed in the gonads and in mesenchymal cells adjacent to the Müllerian duct. Development 120, 189–197.
Bansal, N., Yendluri, V., and Wenham, R. M. (2009). The molecular biology of endometrial cancers and the implications for pathogenesis, classification, and targeted therapies. Cancer Control. 16, 8–13. doi: 10.1177/107327480901600102
Barnes, J. D., Crosby, J. L., Jones, C. M., Wright, C. V., and Hogan, B. L. (1994). Embryonic expression of Lim-1, the mouse homolog of Xenopus Xlim-1 suggests a role in lateral mesoderm differentiation and neurogenesis. Dev. Biol. 161, 168–178. doi: 10.1006/dbio.1994.1018
Behringer, R. R., Finegold, M. J., and Cate, R. L. (1994). Müllerian-inhibiting substance function during mammalian sexual development. Cell 79, 415–425. doi: 10.1016/0092-8674(94)90251-8
Benson, G. V., Lim, H., Paria, B. C., Satokata, I., Dey, S. K., and Maas, R. L. (1996). Mechanisms of reduced fertility in Hoxa-10 mutant mice: uterine homeosis and loss of maternal Hoxa-10 expression. Development 122, 2687–2696.
Bernardini, L., Gimelli, S., Gervasini, C., Carella, M., Baban, A., Frontino, G., et al. (2009). Recurrent microdeletion at 17q12 as a cause of Mayer-Rokitansky-Küster-Hauser (MRKH) syndrome: two case reports. Orphanet J. Rare Dis. 4:25. doi: 10.1186/1750-1172-4-25
Biason-Lauber, A., De Filippo, G., Konrad, D., Scarano, G., Nazzaro, A., and Schoenle, E. J. (2007). WNT4 deficiency–a clinical phenotype distinct from the classic Mayer-Rokitansky-Küster-Hauser syndrome: a case report. Hum. Reprod. 22, 224–229. doi: 10.1093/humrep/del360
Biason-Lauber, A., Konrad, D., Navratil, F., and Schoenle, E. J. (2004). A WNT4 mutation associated with Müllerian-duct regression and virilization in a 46,XX woman. New Eng. J. Med. 351, 792–798. doi: 10.1056/nejmoa040533
Bingham, C., Ellard, S., Cole, T. R., Jones, K. E., Allen, L. I., Goodship, J. A., et al. (2002). Solitary functioning kidney and diverse genital tract malformations associated with hepatocyte nuclear factor-1beta mutations. Kidney Int. 61, 1243–1251. doi: 10.1046/j.1523-1755.2002.00272.x
Boualia, S. K., Gaitan, Y., Murawski, I., Nadon, R., Gupta, I. R., and Bouchard, M. (2011). Vesicoureteral reflux and other urinary tract malformations in mice compound heterozygous for Pax2 and Emx2. PLoS One 6:e21529. doi: 10.1371/journal.pone.0021529
Boualia, S. K., Gaitan, Y., Tremblay, M., Sharma, R., Cardin, J., Kania, A., et al. (2013). A core transcriptional network composed of Pax2/8, Gata3 and Lim1 regulates key players of pro/mesonephros morphogenesis. Dev. Biol. 382, 555–566. doi: 10.1016/j.ydbio.2013.07.028
Bouchard, M., Souabni, A., and Busslinger, M. (2004). Tissue-specific expression of cre recombinase from the Pax8 locus. Genesis 38, 105–109. doi: 10.1002/gene.20008
Bouchard, M., Souabni, A., Mandler, M., Neubüser, A., and Busslinger, M. (2002). Nephric lineage specification by Pax2 and Pax8. Genes Dev. 16, 2958–2970. doi: 10.1101/gad.240102
Branford, W. W., Benson, G. V., Ma, L., Maas, R. L., and Potter, S. S. (2000). Characterization of Hoxa-10/Hoxa-11 transheterozygotes reveals functional redundancy and regulatory interactions. Dev. Biol. 224, 373–387. doi: 10.1006/dbio.2000.9809
Bresnick, A. R. (1999). Molecular mechanisms of nonmuscle myosin-II regulation. Curr. Opin. Cell Biol. 11, 26–33. doi: 10.1016/s0955-0674(99)80004-0
Brophy, P. D., Rasmussen, M., Parida, M., Bonde, G., Darbro, B. W., Hong, X., et al. (2017). A gene implicated in activation of retinoic acid receptor targets is a novel renal agenesis gene in humans. Genetics 207, 215–228. doi: 10.1534/genetics.117.1125
Carroll, T. J., Park, J. S., Hayashi, S., Majumdar, A., and McMahon, A. P. (2005). Wnt9b plays a central role in the regulation of mesenchymal to epithelial transitions underlying organogenesis of the mammalian urogenital system. Dev. Cell 9, 283–292. doi: 10.1016/j.devcel.2005.05.016
Carroll, T. J., and Vize, P. D. (1999). Synergism between Pax-8 and Lim-1 in embryonic kidney development. Dev. Biol. 214, 46–59. doi: 10.1006/dbio.1999.9414
Chang, X., Qin, Y., Xu, C., Li, G., Zhao, X., and Chen, Z. J. (2012). Mutations in WNT4 are not responsible for Müllerian duct abnormalities in Chinese women. Reprod. Biomed. Online 24, 630–633. doi: 10.1016/j.rbmo.2012.03.008
Cunha, G. R. (1976). Stromal induction and specification of morphogenesis and cytodifferentiation of the epithelia of the Mullerian ducts and urogenital sinus during development of the uterus and vagina in mice. J. Exp. Zool. 196, 361–370. doi: 10.1002/jez.1401960310
Cunha, G. R., Robboy, S. J., Kurita, T., Isaacson, D., Shen, J., Cao, M., et al. (2018). Development of the human female reproductive tract. Differentiation 103, 46–65.
Davis, R. J., Harding, M., Moayedi, Y., and Mardon, G. (2008). Mouse Dach1 and Dach2 are redundantly required for Müllerian duct development. Genesis 46, 205–213. doi: 10.1002/dvg.20385
De Tomasi, L., David, P., Humbert, C., Silbermann, F., Arrondel, C., Tores, F., et al. (2017). Mutations in GREB1L cause bilateral kidney agenesis in humans and mice. Am. J. Hum. Genet. 101, 803–814. doi: 10.1016/j.ajhg.2017.09.026
Deutscher, E., and Hung-Chang Yao, H. (2007). Essential roles of mesenchyme-derived beta-catenin in mouse Müllerian duct morphogenesis. Dev. Biol. 307, 227–236. doi: 10.1016/j.ydbio.2007.04.036
Dohr, G., and Tarmann, T. (1984). Contacts between Wolffian and Müllerian cells at the tip of the outgrowing Müllerian duct in rat embryos. Acta Anatomica 120, 123–128. doi: 10.1159/000145905
Dollé, P., Izpisúa-Belmonte, J. C., Brown, J. M., Tickle, C., and Duboule, D. (1991). HOX-4 genes and the morphogenesis of mammalian genitalia. Genes Dev. 5, 1767–1776. doi: 10.1101/gad.5.10.1767
Du, H., and Taylor, H. S. (2004). Molecular regulation of Müllerian development by Hox genes. Ann. N. Y. Acad. Sci. 1034, 152–165. doi: 10.1196/annals.1335.018
Du, H., and Taylor, H. S. (2015). The role of Hox genes in female reproductive tract development, adult function, and fertility. Cold Spring Harbor Perspect. Med. 6:a023002. doi: 10.1101/cshperspect.a023002
Fromental-Ramain, C., Warot, X., Lakkaraju, S., Favier, B., Haack, H., Birling, C., et al. (1996). Specific and redundant functions of the paralogous Hoxa-9 and Hoxd-9 genes in forelimb and axial skeleton patterning. Development 122, 461–472.
Fujino, A., Arango, N. A., Zhan, Y., Manganaro, T. F., Li, X., MacLaughlin, D. T., et al. (2009). Cell migration and activated PI3K/AKT-directed elongation in the developing rat Müllerian duct. Dev. Biol. 325, 351–362. doi: 10.1016/j.ydbio.2008.10.027
Gendron, R. L., Paradis, H., Hsieh-Li, H. M., Lee, D. W., Potter, S. S., and Markoff, E. (1997). Abnormal uterine stromal and glandular function associated with maternal reproductive defects in Hoxa-11 null mice. Biol. Reprod. 56, 1097–1105. doi: 10.1095/biolreprod56.5.1097
George, S. H., Garcia, R., and Slomovitz, B. M. (2016). Ovarian cancer: the Fallopian tube as the site of origin and opportunities for prevention. Front. Oncol. 6:108. doi: 10.3389/fonc.2016.00108
Gervasini, C., Grati, F. R., Lalatta, F., Tabano, S., Gentilin, B., Colapietro, P., et al. (2010). SHOX duplications found in some cases with type I Mayer-Rokitansky-Küster-Hauser syndrome. Genetic Med. 12, 634–640. doi: 10.1097/gim.0b013e3181ed6185
Ghosh, A., Syed, S. M., Kumar, M., Carpenter, T. J., Teixeira, J. M., Houairia, N., et al. (2020). In vivo cell fate tracing provides no evidence for mesenchymal to epithelial transition in adult fallopian tube and uterus. Cell Rep. 31:107631. doi: 10.1016/j.celrep.2020.107631
Ghosh, A., Syed, S. M., and Tanwar, P. S. (2017). In vivo genetic cell lineage tracing reveals that oviductal secretory cells self-renew and give rise to ciliated cells. Development 144, 3031–3041. doi: 10.1242/dev.149989
Godin, P., Tsoi, M., Paquet, M., and Boerboom, D. (2020). YAP and TAZ are required for the postnatal development and the maintenance of the structural integrity of the oviduct. Reproduction 160, 307–318. doi: 10.1530/rep-20-0202
Gonzalez, G., and Behringer, R. R. (2009). Dicer is required for female reproductive tract development and fertility in the mouse. Mol. Reprod. Dev. 76, 678–688. doi: 10.1002/mrd.21010
Grote, D., Boualia, S. K., Souabni, A., Merkel, C., Chi, X., Costantini, F., et al. (2008). Gata3 acts downstream of beta-catenin signaling to prevent ectopic metanephric kidney induction. PLoS Genet. 4:e1000316. doi: 10.1371/journal.pgen.1000316
Gruenwald, P. (1941). The relation of the growing Müllerian duct to the Wolffian duct and its importance for the genesis of malformations. Anatom. Record 81, 1–19. doi: 10.1002/ar.1090810102
Guioli, S., Sekido, R., and Lovell-badge, R. (2007). The origin of the Müllerian duct in chick and mouse. Dev. Biol. 302, 389–398. doi: 10.1016/j.ydbio.2006.09.046
Hacker, A., Capel, B., Goodfellow, P., and Lovell-Badge, R. (1995). Expression of Sry, the mouse sex determining gene. Development 121, 1603–1614.
Hashimoto, R. (2003). Development of the human Müllerian duct in the sexually undifferentiated stage. Anatom. Record 272A, 514–519. doi: 10.1002/ar.a.10061
Hong, X., Luense, L. J., McGinnis, L. K., Nothnick, W. B., and Christenson, L. K. (2008). Dicer1 is essential for female fertility and normal development of the female reproductive system. Endocrinology 149, 6207–6212. doi: 10.1210/en.2008-0294
Hu, Z., Artibani, M., Alsaadi, A., Wietek, N., Morotti, M., Shi, T., et al. (2020). The repertoire of serous ovarian cancer non-genetic heterogeneity revealed by single-cell sequencing of normal fallopian tube epithelial cells. Cancer Cell 37, 226–242. doi: 10.1016/j.ccell.2020.01.003
Huang, D. W., Sherman, B. T., and Lempicki, R. A. (2009). Systematic and integrative analysis of large gene lists using DAVID bioinformatics resources. Nat. Protocols 4, 44–57. doi: 10.1038/nprot.2008.211
Jacob, M., Konrad, K., and Jacob, H. J. (1999). Early development of the Müllerian duct in avian embryos with reference to the human. an ultrastructural and immunohistochemical study. Cells Tissues Organs 164, 63–81. doi: 10.1159/000016644
Jacquinet, A., Boujemla, B., Fasquelle, C., Thiry, J., Josse, C., Lumaka, A., et al. (2020). GREB1L variants in familial and sporadic hereditary urogenital adysplasia and Mayer-Rokitansky-Küster-Hauser syndrome. Clin. Genet. 98, 126–137. doi: 10.1111/cge.13769
Josso, N., Cate, R. L., Picard, J. Y., Vigier, B., di Clemente, N., Wilson, C., et al. (1993). Anti-Müllerian hormone: the Jost factor. Recent Prog. Hormone Res. 48, 1–59.
Kastner, P., Mark, M., Ghyselinck, N., Krezel, W., Dupé, V., Grondona, J. M., et al. (1997). Genetic evidence that the retinoid signal is transduced by heterodimeric RXR/RAR functional units during mouse development. Development 124, 313–326.
Killian, G. J. (2004). Evidence for the role of oviduct secretions in sperm function, fertilization and embryo development. Animal Reprod. Sci. 8, 141–153. doi: 10.1016/j.anireprosci.2004.04.028
Kim, S. M., and Kim, J. S. (2017). A review of mechanisms of implantation. Dev. Reprod. 21, 351–359. doi: 10.12717/dr.2017.21.4.351
Kobayashi, A., and Behringer, R. R. (2003). Developmental genetics of the female reproductive tract in mammals. Nat. Rev. Genet. 4, 969–980. doi: 10.1038/nrg1225
Kobayashi, A., Kwan, K. M., Carroll, T. J., McMahon, A. P., Mendelsohn, C. L., and Behringer, R. R. (2005). Distinct and sequential tissue-specific activities of the LIM-class homeobox gene Lim1 for tubular morphogenesis during kidney development. Development 132, 2809–2823. doi: 10.1242/dev.01858
Kobayashi, A., Shawlot, W., Kania, A., and Behringer, R. R. (2004). Requirement of Lim1 for female reproductive tract development. Development 131, 539–549. doi: 10.1242/dev.00951
Komatsu, M., and Fujita, H. (1978). Electron-microscopic studies on the development and aging of the oviduct epithelium of mice. Anatomy Embryol. 152, 243–259. doi: 10.1007/bf00350523
Kurita, T., Cooke, P. S., and Cunha, G. R. (2001). Epithelial-stromal tissue interaction in paramesonephric (Müllerian) epithelial differentiation. Dev. Biol. 240, 194–211. doi: 10.1006/dbio.2001.0458
Laronda, M. M., Unno, K., Ishi, K., Serna, V. A., Butler, L. M., Mills, A. A., et al. (2013). Diethylstilbestrol induces vaginal adenosis by disrupting SMAD/RUNX1-mediated cell fate decision in the Müllerian duct epithelium. Dev. Biol. 381, 5–16. doi: 10.1016/j.ydbio.2013.06.024
Ledig, S., Brucker, S., Barresi, G., Schomburg, J., Rall, K., and Wieacker, P. (2012). Frame shift mutation of LHX1 is associated with Mayer-Rokitansky-Küster-Hauser (MRKH) syndrome. Hum. Reprod. 27, 2872–2875. doi: 10.1093/humrep/des206
Ledig, S., Schippert, C., Strick, R., Beckmann, M. W., Oppelt, P. G., and Wieacker, P. (2011). Recurrent aberrations identified by array-CGH in patients with Mayer-Rokitansky-Küster-Hauser syndrome. Fertil. Steril. 95, 1589–1594. doi: 10.1016/j.fertnstert.2010.07.1062
Ledig, S., Tewes, A. C., Hucke, J., Römer, T., Kapczuk, K., Schippert, C., et al. (2018). Array-comparative genomic hybridization analysis in patients with Müllerian fusion anomalies. Clin. Genet. 93, 640–646. doi: 10.1111/cge.13160
Li, Q., Agno, J. E., Edson, M. A., Nagaraja, A. K., Nagashima, T., and Matzuk, M. M. (2011). Transforming growth factor β receptor type 1 is essential for female reproductive tract integrity and function. PLoS Genet. 7:e1002320. doi: 10.1371/journal.pgen.1002320
Li, S., and Winuthayanon, W. (2017). Oviduct: roles in fertilization and early embryo development. J. Endocrinol. 232, R1–R26.
Lokmane, L., Heliot, C., Garcia-Villalba, P., Fabre, M., and Cereghini, S. (2010). vHNF1 functions in distinct regulatory circuits to control ureteric bud branching and early nephrogenesis. Development 137, 347–357. doi: 10.1242/dev.042226
Ma, L., Benson, G. V., Lim, H., Dey, S. K., and Maas, R. L. (1998). Abdominal B (AbdB) Hoxa genes: regulation in adult uterus by estrogen and progesterone and repression in Müllerian duct by the synthetic estrogen diethylstilbestrol (DES). Dev. Biol. 197, 141–154. doi: 10.1006/dbio.1998.8907
Ma, W., Li, Y., Wang, M., Li, H., Su, T., Li, Y., et al. (2015). Associations of polymorphisms in WNT9B and PBX1 with Mayer-Rokitansky-Küster-Hauser syndrome in chinese han. PLoS One 10:e0130202. doi: 10.1371/journal.pone.0130202
Marini, M., Giacopelli, F., Seri, M., and Ravazzolo, R. (2005). Interaction of the LMX1B and PAX2 gene products suggests possible molecular basis of differential phenotypes in nail–patella syndrome. Eur. J. Hum. Genet. 13, 789–792. doi: 10.1038/sj.ejhg.5201405
Martin, A. C., and Goldstein, B. (2014). Apical constriction: themes and variations on a cellular mechanism driving morphogenesis. Development 141, 1987–1998. doi: 10.1242/dev.102228
McGowan, R., Tydeman, G., Shapiro, D., Craig, T., Morrison, N., Logan, S., et al. (2015). DNA copy number variations are important in the complex genetic architecture of Müllerian disorders. Fertil. Steril. 103, 1021–1030. doi: 10.1016/j.fertnstert.2015.01.008
Mendelsohn, C., Lohnes, D., Décimo, D., Lufkin, T., LeMeur, M., Chambon, P., et al. (1994). Function of the retinoic acid receptors (RARs) during development (II). Multiple abnormalities at various stages of organogenesis in RAR double mutants. Development 120, 2749–2771.
Miller, C., Pavlova, A., and Sassoon, D. A. (1998). Differential expression patterns of Wnt genes in the murine female reproductive tract during development and the estrous cycle. Mechan. Dev. 76, 91–99. doi: 10.1016/s0925-4773(98)00112-9
Mishina, Y., Rey, R., Finegold, M. J., Matzuk, M. M., Josso, N., Cate, R. L., et al. (1996). Genetic analysis of the Müllerian-inhibiting substance signal transduction pathway in mammalian sexual differentiation. Genes Dev. 10, 2577–2587. doi: 10.1101/gad.10.20.2577
Miyamoto, N., Yoshida, M., Kuratani, S., Matsuo, I., and Aizawa, S. (1997). Defects of urogenital development in mice lacking Emx2. Development 124, 1653–1664.
Mohammed, H., DSantos, C., Serandour, A. A., Ali, H. R., Brown, G. D., Atkins, A., et al. (2013). Endogenous purification reveals GREB1 as a key estrogen receptor regulatory factor. Cell Reports 3, 342–349. doi: 10.1016/j.celrep.2013.01.010
Mohun, T., Adams, D. J., Baldock, R., Bhattacharya, S., Copp, A. J., Hemberger, M., et al. (2013). Deciphering the Mechanisms of Developmental Disorders (DMDD): a new programme for phenotyping embryonic lethal mice. Dis. Models Mechan. 6, 562–566. doi: 10.1242/dmm.011957
Morice, P., Leary, A., Creutzberg, C., Abu-Rustum, N., and Darai, E. (2016). Endometrial cancer. Lancet 387, 1094–1108.
Mucenski, M. L., Mahoney, R., Adam, M., Potter, A. S., and Potter, S. S. (2019). Single cell RNA-seq study of wild type and Hox9,10,11 mutant developing uterus. Sci. Rep. 9:4557.
Mullen, R. D., and Behringer, R. R. (2014). Molecular genetics of Müllerian duct formation, regression and differentiation. Sexual Dev. 8, 281–296. doi: 10.1159/000364935
Nagaraja, A. K., Andreu-Vieyra, C., Franco, H. L., Ma, L., Chen, R., Han, D. Y., et al. (2008). Deletion of Dicer in somatic cells of the female reproductive tract causes sterility. Mol. Endocrinol. 22, 2336–2352. doi: 10.1210/me.2008-0142
Nakajima, T., Iguchi, T., and Sato, T. (2016). Retinoic acid signaling determines the fate of uterine stroma in the mouse Müllerian duct. PNAS 113, 14354–14359. doi: 10.1073/pnas.1608808113
Nakajima, T., Yamanaka, R., and Tomooka, Y. (2019). Elongation of Müllerian ducts and connection to urogenital sinus determine the borderline of uterine and vaginal development. Biochem. Biophys. Rep. 17, 44–50. doi: 10.1016/j.bbrep.2018.10.013
Ng, K. Y. B., and Cheong, Y. (2019). Hydrosalpinx- salpingostomy, salpingectomy or tubal occlusion. Best Pract. Res. Clin. Obstet. Gynaecol. 59, 41–47. doi: 10.1016/j.bpobgyn.2019.01.011
Nik-Zainal, S., Strick, R., Storer, M., Huang, N., Rad, R., Willatt, L., et al. (2011). High incidence of recurrent copy number variants in patients with isolated and syndromic Müllerian aplasia. J. Med. Genet. 48, 197–204. doi: 10.1136/jmg.2010.082412
Orvis, G. D., and Behringer, R. R. (2007). Cellular mechanisms of Müllerian duct formation in the mouse. Dev. Biol. 306, 493–504. doi: 10.1016/j.ydbio.2007.03.027
Owhor, L. E., Reese, S., and Kölle, S. (2019). Salpingitis impairs bovine tubal function and sperm-oviduct interaction. Sci. Rep. 9:10893.
Parr, B. A., and Mcmahon, A. P. (1998). Sexually dimorphic development of the mammalian reproductive tract requires Wnt7-a. Nature 395, 707–710. doi: 10.1038/27221
Pearl, E. J., Li, J., and Green, J. B. (2017). Cellular systems for epithelial invagination. Phil. Trans. R. Soc. B 372:20150526. doi: 10.1098/rstb.2015.0526
Pedersen, A., Skjong, C., and Shawlot, W. (2005). Lim1 is required for nephric duct extension and ureteric bud morphogenesis. Dev. Biol. 288, 571–581. doi: 10.1016/j.ydbio.2005.09.027
Pellegrini, M., Pantano, S., Lucchini, F., Fumi, M., and Forabosco, A. (1997). Emx2 developmental expression in the primordia of the reproductive and excretory systems. Anatomy Embryol. 196, 427–433. doi: 10.1007/s004290050110
Philibert, P., Biason-Lauber, A., Gueorguieva, I., Stuckens, C., Pienkowski, C., Lebon-Labich, B., et al. (2011). Molecular analysis of WNT4 in four adolescent girls with Müllerian duct abnormality and hyperandrogenism (atypical Mayer-Rokitansky-Küster-Hauser syndrome). Fertil. Steril. 95, 2683–2686. doi: 10.1016/j.fertnstert.2011.01.152
Philibert, P., Biason-Lauber, A., Rouzier, R., Pienkowski, C., Paris, F., Konrad, D., et al. (2008). Identification and functional analysis of a new WNT4 gene mutation among 28 adolescent girls with primary amenorrhea and Müllerian duct abnormalities: a french collaborative study. J. Clin. Endocrinol. Metab. 93, 895–900. doi: 10.1210/jc.2007-2023
Prunskaite-Hyyryläinen, R., Skovorodkin, I., Xu, Q., Miinalainen, I., Shan, J., and Vainio, S. J. (2016). Wnt4 coordinates directional cell migration and extension of the Müllerian duct essential for ontogenesis of the female reproductive tract. Hum. Mol. Genet. 25, 1059–1073. doi: 10.1093/hmg/ddv621
Raines, A. M., Adam, M., Magella, B., Meyer, S. E., Grimes, H. L., Dey, S. K., et al. (2013). Recombineering-based dissection of flanking and paralogous Hox gene functions in mouse reproductive tracts. Development 140, 2942–2952. doi: 10.1242/dev.092569
Rana, P., Kazmi, I., Singh, R., Afzal, M., Al-Abbasi, F. A., Aseeri, et al. (2013). Ectopic pregnancy: a review. Arch. Gynecol. Obstet. 288, 747–757.
Ravel, C., Lorenço, D., Dessolle, L., Mandelbaum, J., McElreavey, K., Darai, E., et al. (2009). Mutational analysis of the WNT gene family in women with Mayer-Rokitansky-Küster-Hauser syndrome. Fertil. Steril. 91:4Sul.
Renfree, M. B., Fenelon, J., Wijayanti, G., Wilson, J. D., and Shaw, G. (2009). Wolffian duct differentiation by physiological concentrations of androgen delivered systemically. Dev. Biol. 334, 429–436. doi: 10.1016/j.ydbio.2009.07.040
Roly, Z. Y., Backhouse, B., Cutting, A., Tan, T. Y., Sinclair, A. H., Ayers, K. L., et al. (2018). The cell biology and molecular genetics of Müllerian duct development. Wiley Interdiscipl. Rev. Dev. Biol. 7:e310.
Roly, Z. Y., Godini, R., Estermann, M. A., Major, A. T., Pocock, R., and Smith, C. A. (2020a). Transcriptional landscape of the embryonic chicken Müllerian duct. BMC Genom. 21:688. doi: 10.1186/s12864-020-07106-8
Roly, Z. Y., Major, A. T., Fulcher, A., Estermann, M. A., Hirst, C. E., and Smith, C. A. (2020b). Adhesion G-protein-coupled receptor, GPR56, is required for Müllerian duct development in the chick. J. Endocrinol. 244, 395–413. doi: 10.1530/joe-19-0419
Saatcioglu, H. D., Kano, M., Horn, H., Zhang, L., Samore, W., Nagykery, N., et al. (2019). Single-cell sequencing of neonatal uterus reveals an Misr2+ endometrial progenitor indispensable for fertility. eLife 8:e46349.
Sandbacka, M., Laivuori, H., Freitas, É, Halttunen, M., Jokimaa, V., Morin-Papunen, L., et al. (2013). TBC6, LHX1 and copy number variations in the complex genetics of Müllerian aplasia. Orphanet J. Rare Dis. 8:125. doi: 10.1186/1750-1172-8-125
Satokata, I., Benson, G., and Maas, R. (1995). Sexually dimorphic sterility phenotypes in Hoxa10-deficient mice. Nature 374, 460–463. doi: 10.1038/374460a0
Schnabel, C. A., Selleri, L., and Cleary, M. L. (2003). Pbx1 is essential for adrenal development and urogenital differentiation. Genesis 37, 123–130. doi: 10.1002/gene.10235
Sellers, J. R. (1991). Regulation of cytoplasmic and smooth muscle myosin. Curr. Opin. Cell Biol. 3, 98–104. doi: 10.1016/0955-0674(91)90171-t
Shi, D., Komatsu, K., Hirao, M., Toyooka, Y., Koyama, H., Tissir, F., et al. (2014). Celsr1 is required for the generation of polarity at multiple levels of the mouse oviduct. Development 141, 4558–4568. doi: 10.1242/dev.115659
Smith, C. M., Hayamizu, T. F., Finger, J. H., Bello, S. M., McCright, I. J., Xu, J., et al. (2019). The mouse Gene Expression Database (GXD): 2019 update. Nucleic Acids Res. 47, D774–D779.
Stewart, C. A., Wang, Y., Bonilla-Claudio, M., Martin, J. F., Gonzalez, G., Taketo, M. M., et al. (2013). CTNNB1 in mesenchyme regulates epithelial cell differentiation during Müllerian duct and postnatal uterine development. Mol. Endocrinol. 27, 1442–1454. doi: 10.1210/me.2012-1126
St-Jean, G., Boyer, A., Zamberlam, G., Godin, P., Paquet, M., and Boerboom, D. (2019a). Targeted ablation of Wnt4 and Wnt5a in Müllerian duct mesenchyme impedes endometrial gland development and causes partial Müllerian agenesis. Biol. Reprod. 100, 49–60. doi: 10.1093/biolre/ioy160
St-Jean, G., Tsoi, M., Abedini, A., Levasseur, A., Rico, C., Morin, M., et al. (2019b). Lats1 and Lats2 are required for the maintenance of multipotency in the Müllerian duct mesenchyme. Development 146:dev180430. doi: 10.1242/dev.180430
Swain, A., and Lovell-Badge, R. (1999). Mammalian sex determination: a molecular drama. Genes Dev. 13, 755–767. doi: 10.1101/gad.13.7.755
Tang, R., Dang, Y., Qin, Y., Zou, S., Li, G., Wang, Y., et al. (2014). WNT9B in 542 chinese women with Müllerian duct abnormalities: mutation analysis. Reprod. Biomed. Online 28, 503–507. doi: 10.1016/j.rbmo.2013.11.011
Taylor, H. S., Vanden Heuvel, G. B., and Igarashi, P. A. (1997). A conserved Hox axis in the mouse and human female reproductive system: late establishment and persistent adult expression of the Hoxa cluster genes. Biol. Reprod. 57, 1338–1345. doi: 10.1095/biolreprod57.6.1338
Terakawa, J., Rocchi, A., Serna, V. A., Bottinger, E. P., Graff, J. M., and Kurita, T. (2016). FGFR2IIIb-MAPK activity is required for epithelial cell fate decision in the lower Müllerian duct. Mol. Endocrinol. 30, 783–795. doi: 10.1210/me.2016-1027
Terakawa, J., Serna, V. A., Nair, D. M., Sato, S., Kawakami, K., Radovick, S., et al. (2020). SIX1 cooperates with RUNX1 and SMAD4 in cell fate commitment of Müllerian duct epithelium. Cell Death Diff. 27, 3307–3320. doi: 10.1038/s41418-020-0579-z
Torres, M., Gómez-Pardo, E., Dressler, G. R., and Gruss, P. (1995). Pax-2 controls multiple steps of urogenital development. Development 121, 4057–4065.
Vainio, S., Heikkilä, M., Kispert, A., Chin, N., and Mcmahon, A. P. (1999). Female development in mammals is regulated by Wnt-4 signalling. Nature 397, 405–409. doi: 10.1038/17068
Vandenberg, A. L., and Sassoon, D. A. (2009). Non-canonical Wnt signaling regulates cell polarity in female reproductive tract development via van gogh-like 2. Development 136, 1559–1570. doi: 10.1242/dev.034066
Wang, C., Xing, Q., Song, B., Li, G., Xu, Z., Wang, T., et al. (2020). Aberrant DNA methylation in the Pax2 promoter is associated with Müllerian duct anomalies. Arch. Gynecol. Obstet. 301, 1455–1461. doi: 10.1007/s00404-020-05539-w
Wang, M., Li, Y., Ma, W., Li, H., He, F., Pu, D., et al. (2014). Analysis of WNT9B mutations in chinese women with Mayer-Rokitansky-Küster-Hauser syndrome. Reprod. Biomed. Online 28, 80–85. doi: 10.1016/j.rbmo.2013.09.022
Wang, P., Zhao, H., Sun, M., Li, Y., and Chen, Z. J. (2012). PAX2 in 192 chinese women with Müllerian duct abnormalities: mutation analysis. Reprod. Biomed. Online 25, 219–222. doi: 10.1016/j.rbmo.2012.04.010
Waschk, D. E., Tewes, A. C., Römer, T., Hucke, J., Kapczuk, K., Schippert, C., et al. (2016). Mutations in WNT9B are associated with Mayer-Rokitansky-Küster-Hauser syndrome. Clin. Genet. 89, 590–596.
Xu, B., Geerts, D., Bu, Z., Ai, J., Jin, L., Li, Y., et al. (2014). Regulation of endometrial receptivity by the highly expressed HOXA9, HOXA11 and HOXD10 HOX-class homeobox genes. Hum. Reprod. 29, 781–790. doi: 10.1093/humrep/deu004
Xu, Z., Wu, S., Xing, Q., Wang, X., Xiang, H., Xu, Y., et al. (2017). Genetic association between PAX2 and Müllerian duct anomalies in Han Chinese females. J. Assisted Reprod. Genet. 34, 125–129. doi: 10.1007/s10815-016-0807-0
Yamanouchi, H., Umezu, T., and Tomooka, Y. (2010). Reconstruction of oviduct and demonstration of epithelial fate determination in mice. Biol. Reprod. 82, 528–533. doi: 10.1095/biolreprod.109.078329
Yoshino, T., Saito, D., Atsuta, Y., Uchiyama, C., Ueda, S., Sekiguchi, K., et al. (2014). Interepithelial signaling with nephric duct is required for the formation of overlying coelomic epithelial cell sheet. PNAS 111, 6660–6665. doi: 10.1073/pnas.1316728111
Zhang, S., Igor Dolgalev, I., Zhang, T., Ran, H., Levine, D. A., and Neel, B. G. (2019). Both fallopian tube and ovarian surface epithelium are cells-of-origin for high-grade serous ovarian carcinoma. Nat. Commun. 10: 5367.
Zhao, F., Franco, H. L., Rodriguez, K. F., Brown, P. R., Tsai, M. J., Tsai, S. Y., et al. (2017). Elimination of the male reproductive tract in the female embryo is promoted by COUP-TFII in mice. Science 357, 717–720. doi: 10.1126/science.aai9136
Zhao, F., and Yao, H. H. (2019). A tale of two tracts: history, current advances, and future directions of research on sexual differentiation of reproductive tracts. Biol. Reprod. 101, 602–616. doi: 10.1093/biolre/ioz079
Zhao, F., Zhou, J., Li, R., Dudley, E. A., and Ye, X. (2016). Novel function of LHFPL2 in female and male distal reproductive tract development. Sci. Rep. 6:23037.
Keywords: Müllerian ducts, oviducts, embryonic development, differentiation, gene regulatory networks, epithelial-mesenchymal transition (EMT), genome-wide survey
Citation: Santana Gonzalez L, Rota IA, Artibani M, Morotti M, Hu Z, Wietek N, Alsaadi A, Albukhari A, Sauka-Spengler T and Ahmed AA (2021) Mechanistic Drivers of Müllerian Duct Development and Differentiation Into the Oviduct. Front. Cell Dev. Biol. 9:605301. doi: 10.3389/fcell.2021.605301
Received: 11 September 2020; Accepted: 28 January 2021;
Published: 08 March 2021.
Edited by:
Mohit Kumar Jolly, Indian Institute of Science (IISc), IndiaReviewed by:
Sagar S. Varankar, University of Cambridge, United KingdomDeepak Modi, National Institute for Research in Reproductive Health (ICMR), India
Copyright © 2021 Santana Gonzalez, Rota, Artibani, Morotti, Hu, Wietek, Alsaadi, Albukhari, Sauka-Spengler and Ahmed. This is an open-access article distributed under the terms of the Creative Commons Attribution License (CC BY). The use, distribution or reproduction in other forums is permitted, provided the original author(s) and the copyright owner(s) are credited and that the original publication in this journal is cited, in accordance with accepted academic practice. No use, distribution or reproduction is permitted which does not comply with these terms.
*Correspondence: Ahmed A. Ahmed, YWhtZWQuYWhtZWRAd3JoLm94LmFjLnVr