- 1Center for Vaccine Development and Global Health, University of Maryland School of Medicine, Baltimore, MD, United States
- 2Department of Medicine, University of Maryland School of Medicine, Baltimore, MD, United States
- 3Department of Pediatrics, University of Maryland School of Medicine, Baltimore, MD, United States
- 4Department of Pathology, University of Maryland School of Medicine, Baltimore, MD, United States
- 5Centre pour le Développement des Vaccins, Bamako, Mali
- 6Division of Infectious Diseases, Duke University Medical Center, Durham, NC, United States
- 7Department of Medicine, Division of Infectious Diseases and International Health, Duke University School of Medicine, Durham, NC, United States
- 8Duke Clinical Research Institute, Durham, NC, United States
- 9Colegio de Ciencias de la Salud e Instituto de Microbiologia, Universidad San Francisco de Quito, Quito, Ecuador
- 10Département de Bactériologie, Centre d’Immunologie et des Maladies Infectieuses-Paris, Cimi-Paris, INSERM U1135, AP-HP, Sorbonne Université, Hôpitaux Universitaires Est Parisien, Paris, France
- 11Statens Serum Institut, Copenhagen, Denmark
- 12Department of Microbiology, Tumor and Cell Biology, Division of Clinical Microbiology, Karolinska Institutet, Karolinska University Hospital, Stockholm, Sweden
- 13Department of Microbiology, AHEPA University Hospital, Thessaloniki, Greece
- 14Department of Medicine, AHEPA University Hospital, Thessaloniki, Greece
- 15Medical School, University of Cyprus, Nicosia, Cyprus
- 16Department of Respiratory Medicine, Graduate School of Medicine, Nagoya University, Nagoya, Japan
- 17Division of Infectious Diseases, Department of Medicine, Taipei Veterans General Hospital, Taipei, Taiwan
- 18Institute of Emergency and Critical Care Medicine, National Yang-Ming University, Taipei, Taiwan
- 19Department of Pharmacy, Singapore General Hospital, Singapore, Singapore
- 20Emerging Infectious Diseases, Duke-National University of Singapore Medical School, Singapore, Singapore
- 21Department of Pharmacy, Faculty of Science, National University of Singapore, Singapore, Singapore
- 22Departments of Pathology and Pediatrics, Aga Khan University, Karachi, Pakistan
- 23National Institute for Communicable Diseases, A Division of the National Health Laboratory Service, School of Pathology, Faculty of Health Sciences, University of the Witwatersrand, Johannesburg, Johannesburg, South Africa
- 24Department of Clinical Sciences, Institute of Tropical Medicine Antwerp, Antwerp, Belgium
- 25Department of Microbiology, Immunology and Transplantation, KU Leuven, Leuven, Belgium
- 26Department of Clinical Microbiology and Microbiology, National Institute for Biomedical Research, University Hospital of Kinshasa, Kinshasa, Democratic Republic of Congo
Klebsiella pneumoniae is a common cause of sepsis and is particularly associated with healthcare-associated infections. New strategies are needed to prevent or treat infections due to the emergence of multi-drug resistant K. pneumoniae. The goal of this study was to determine the diversity and distribution of O (lipopolysaccharide) and K (capsular polysaccharide) antigens on a large (>500) global collection of K. pneumoniae strains isolated from blood to inform vaccine development efforts. A total of 645 K. pneumoniae isolates were collected from the blood of patients in 13 countries during 2005–2017. Antibiotic susceptibility was determined using the Kirby-Bauer disk diffusion method. O antigen types including the presence of modified O galactan types were determined by PCR. K types were determined by multiplex PCR and wzi capsular typing. Sequence types of isolates were determined by multilocus sequence typing (MLST) targeting seven housekeeping genes. Among 591 isolates tested for antimicrobial resistance, we observed that 19.3% of isolates were non-susceptible to carbapenems and 62.1% of isolates were multidrug resistant (from as low as 16% in Sweden to 94% in Pakistan). Among 645 isolates, four serotypes, O1, O2, O3, and O5, accounted for 90.1% of K. pneumoniae strains. Serotype O1 was associated with multidrug resistance. Fifty percent of 199 tested O1 and O2 strains were gmlABC-positive, indicating the presence of the modified polysaccharide subunit D-galactan III. The most common K type was K2 by both multiplex PCR and wzi capsular typing. Of 39 strains tested by MLST, 36 strains were assigned to 26 known sequence types of which ST14, ST25, and ST258 were the most common. Given the limited number of O antigen types, diverse K antigen types and the high multidrug resistance, we believe that an O antigen-based vaccine would offer an excellent prophylactic strategy to prevent K. pneumoniae invasive infection.
Introduction
Klebsiella pneumoniae is a common Gram-negative bacterial cause of sepsis that contributes to high mortality and morbidity in hospitalized, immunosuppressed, and chronically ill patients. Klebsiella spp. were the third most common cause of healthcare-associated infections (9.9%) in acute-care hospitals in United States in 2011 after Clostridioides difficile (12.1%) and Staphylococcus aureus (10.7%) (Magill et al., 2014). A follow-up study was performed in 2015 and showed that the prevalence of healthcare-associated infections was decreased compared to 2011. Further, Klebsiella spp. were responsible for ∼5% of these infections with C. difficile (15%), S. aureus (11%) and Escherichia coli (10%) being the most common (Magill et al., 2018). Similarly, K. pneumoniae was responsible for 12% of healthcare-associated pneumonia infections in a point prevalence survey in acute care hospitals in the European Union in 2011–2012 (Walter et al., 2018). Many additional studies have highlighted the importance of K. pneumoniae as a cause of healthcare-associated infections (Meatherall et al., 2009; Folgori et al., 2016; Cai et al., 2017; Baykara et al., 2018).
The occurrence of multidrug-resistant (MDR) K. pneumoniae has been considered as an urgent threat worldwide (Lee et al., 2017). Limited therapeutic options for infection with MDR K. pneumoniae results in increased mortality, longer hospital stays and inflated healthcare costs (Cerceo et al., 2016). Given the emergence of multidrug resistant isolates, prevention of K. pneumoniae infections has become of paramount importance. Several groups have developed vaccines to prevent K. pneumoniae infection and these target capsular polysaccharide, extracellular vesicles and outer membrane proteins (Cryz et al., 1986a; Edelman et al., 1994; Chhibber et al., 2005; Lundberg et al., 2013; Lee et al., 2015). Likewise, we are developing a quadrivalent conjugate vaccine that targets the O1, O2, O3, and O5 polysaccharides of K. pneumoniae (Hegerle et al., 2018). We and others have shown that antibodies directed against the O polysaccharide are protective against wild-type K. pneumoniae in lethal infection models (Clements et al., 2008; Hegerle et al., 2018).
Klebsiella pneumoniae capsular polysaccharide (K antigen) and lipopolysaccharide (O antigen) are important virulence factors that can activate the innate immune system (Simoons-Smit et al., 1986; Shankar-Sinha et al., 2004). Additionally, these two antigens are used to differentiate K. pneumoniae isolates. More than 77 K antigens have been defined to date (Follador et al., 2016). Trautmann et al. (1997) determined that over 80% of K. pneumoniae isolates from Denmark belong to O1, O2, O3, and O5. This finding is corroborated by Follador et al. (2016) who sequenced more than 500 human and environmental isolates from United States, United Kingdom, Australia, Singapore, Indonesia, Laos, Nepal and Vietnam. Of these, only 134 out of the 500 isolates were from humans; 47 strains were from urine, 67 strains were isolated from blood, and 20 strains were from sputum (Follador et al., 2016). Nine serotypes have been proposed but in different combinations by separate groups O1, O2ab (O2a), O2a/O9 (O2aeh), O3, O4, O5, O7, O11, and O12 was proposed by Trautmann et al. (1997) whereas O1, O2, O2ac, O3, O4, O5, O7, O8, and O12 was proposed by Hansen et al. (1999). The O8 polysaccharide is similar to the O1 antigen and O9 is a subtype of the O2 antigen (Ørskov and Ørskov, 1984; Kelly et al., 1993). Serotyping O2 isolates is particularly complex due to the various O2 subtypes (O2a, O2ac, O2aeh, and O2afg) (Clarke et al., 2018). LPS O antigens are composed of D-galactans in O1 and O2 and mannans in O3 and O5 strains. Among galactans, D-galactan I was found in O1 (with D-galactan II), O2a, and O2ac isolates (with 2c polysaccharide) (Whitfield et al., 1991, 1992). Recently, D-gal III has been identified as a component of the LPS of O1 and O2 strains; D-gal III is a product of conversion of the D-gal I disaccharide by the gmlABC operon (Stojkovic et al., 2017). More recently, multilocus sequence typing (MLST) has replaced O and K typing as the most common method used to type K. pneumoniae isolates (Diancourt et al., 2005).
The goal of this study was to determine the global diversity and distribution of O and K antigens on a large (>500) global collection of K. pneumoniae strains isolated from blood. This data will help to determine whether a glycoconjugate vaccine that targets a limited number of O antigens would provide coverage against the majority of K. pneumoniae bacteremic strains that are currently circulating worldwide. We are particularly interested in evaluating prevalence amongst blood culture isolates as our intended primary endpoint for a Phase 3 clinical trial would be prevention of systemic infections.
Materials and Methods
Bacterial Strains
All K. pneumoniae isolates were collected from the blood of patients in 13 countries during 2005–2017 (Table 1). All isolates were identified using standard microbiological procedures. A random sample of K. pneumoniae strains collected from the Americas (United States and Ecuador), Europe (France, Denmark, and Sweden), Asia (Japan, Taiwan, Singapore, and Pakistan) and Africa (South Africa, DR Congo, and Mali), irrespective of antimicrobial resistance, was selected for analysis. However, isolates from Greece were inadvertently selected from a multi drug resistance collection. The number of isolates collected each year is shown for each country in Supplementary Figure S1. Altogether, 645 K. pneumoniae isolates were shipped to the Center for Vaccine Development and Global Health at the University of Maryland School of Medicine for subsequent testing and analysis. K. pneumoniae B5055 (O1), 7380 (O2), 390 (O3), Mich.61 (O4), 4425/51 (O5), 889 (O8), 1205 (O9), and 708 (O12) were used as reference strains for the Ørskov and Ørskov O typing scheme (Ørskov and Ørskov, 1984; Trautmann et al., 2004). K. pneumoniae strains were grown in animal product-free HY-Soy (HS) media [0.5% sodium chloride, 1% Hy-Soy (TEKnova, Hollister, CA, United States), 0.5% Hy-Yest 444 (Kerry Biosciences, Beloit, WI, United States) at 37°C. Genomic DNA was extracted using the GenElute Bacterial Genomic DNA kit (Sigma-Aldrich, St. Louis, MO, United States) following the manufacturer’s instructions. DNA was extracted from 1.5 ml of overnight bacterial culture and eluted into 200 μl of elution solution (10 mM Tris–HCl, 0.5 mM EDTA, pH 9.0) and 2 μl of DNA was used for polymerase chain reaction (PCR).
Antimicrobial Susceptibility Test
Antimicrobial susceptibility was determined by the Kirby-Bauer disk diffusion method. The following antibiotics were tested in this study: amikacin, ampicillin/sulbactam, aztreonam, cefazolin, cefepime, ceftriaxone, ertapenem, gentamicin, levofloxacin, meropenem, piperacillin-tazobactam, and tetracycline. Antimicrobial impregnated disks (BD BBLTM Sensi-DiskTM) were purchased from Becton, Dickinson and Company (Franklin Lakes, NJ, United States). Antimicrobial susceptibility was determined by measuring the diameter of the zone of inhibition and the results were interpreted according to Clinical and Laboratory Standards Institute (CLSI) guidelines (M100-S29). MDR K. pneumoniae was defined as non-susceptible to at least one agent in three or more antibiotic classes. Non-MDR was defined as non-susceptible to less than three antimicrobial categories or fully susceptible (Magiorakos et al., 2012). Isolates from Greece were part of an MDR collection and were thus excluded from all analyses involving antimicrobial resistance to avoid bias from non-randomly selected strains.
Typing O Antigens and Genetic Analysis of D-Galactan Types
O typing was performed by PCR, using a method described by Fang et al. (2016) except that we did not differentiate O2ac from O2a. O1, O2, O3, O4, O5, O8, O9, and O12 were determined (identification of O7 which is rare was not done). Briefly, genomic DNA (>10 ng) was used as the template, Dreamtaq Green PCR Master Mix (Thermo Fisher Scientific, Waltham, MA, United States) was used for PCR, with 0.4 μM primers and 2 mM Mg2+ in a final reaction volume of 50 μl. The following cycling conditions were used: 95°C for 10 min, followed by 30 cycles of 95°C for 30 s, 60°C for 30 s, and 72°C for 1 min, then a final extension at 72°C for 10 min. DNA fragment size was determined on a 1% agarose gel. D-galactan III-possessing strains were identified by screening O1 and O2 strains randomly selected from different regions for the gmlABC locus by PCR (Szijártó et al., 2016). The geographic distribution of O types was visualized using an online tool1.
K Typing
Two methods were used to K type K. pneumoniae: multiplex PCR and wzi gene sequencing. K1, K2, K5, K20, K54, and K57 were identified by multiplex PCR described by Turton et al. (2010). Briefly, multiplex PCR was performed using the Qiagen Multiplex PCR kit (Qiagen, Hilden, Germany) with 0.2 μM primers and 3 mM Mg2+ in a final reaction volume of 25 μl. Cycling parameters were 95°C for 15 min, followed by 35 cycles of 94°C for 30 s, 58°C for 90 s and 72°C for 90 s, and a final extension at 72°C for 10 min. Amplicons were separated on a 1.5% agarose gel.
The wzi gene was sequenced and K types determined as described by Brisse et al. (2013). PCR amplification of wzi was achieved by using PCR buffer containing 2 mM dNTP’s, 0.4 μM primers, and 1 U of Green Taq DNA polymerase (Genscript, Piscataway, NJ, United States). Cycling conditions were as follows: 95°C for 3 min, followed by 40 cycles of 95°C for 30 s, 56°C for 45 s and 72°C for 40 s, and a final extension at 72°C for 5 min. After DNA purification using a QIAquick PCR Purification kit (Qiagen), amplicons were sequenced (Genewiz, South Plainfield, NJ, United States) and chromatograms were reviewed and sequences trimmed using Geneious software (Biomatters Ltd., Auckland, New Zealand). The wzi alleles and K types were identified from the K. pneumoniae sequence typing database at http://bigsdb.web.pasteur.fr. Phylogenetic trees of wzi alleles were constructed using MEGA version 7.0 based on the neighbor-joining method (100 bootstrap replicates) and Jukes-Cantor distance (Brisse et al., 2013; Martin et al., 2016).
Multilocus Sequence Typing (MLST) Analysis
Multilocus sequence typing with seven genes (gapA, infB, mdh, pgi, phoE, rpoB, and tonB) was performed on strains according to the protocol described on the K. pneumoniae MLST website2 (Diancourt et al., 2005). The MLST database was used to assign alleles and sequence types (STs). Clonal complexes (CCs) were defined as groups for which MLST profiles showed only one allelic mismatch with at least one other member of the group (Bialek-Davenet et al., 2014).
Statistical Analysis
The proportion of susceptible versus resistant strains for each O type was analyzed by Chi-square analysis with Yate’s correction using GraphPad Prism version 6.05 software (GraphPad Software, San Diego, United States). The significance level was p < 0.05.
Results
Antimicrobial Resistance
All 54 MDR isolates from Greece were resistant to ampicillin-sulbactam, cefazolin, cefepime, ceftriaxone, ertapenem, meropenem, and piperacillin-tazobactam (data not shown). These isolates were excluded from subsequent analyses (Supplementary Figure S2). High resistance rates to ampicillin-sulbactam (60.9%) and cefazolin (77.3%) were observed among 591 global isolates (see Supplementary Table S2). Resistance to carbapenems, which are used as a last resort for serious multidrug resistant infections (Meletis, 2016), was observed to be 19.3% (Table 2). The highest resistance rate against carbapenems was observed in Asia (51.2% of Asian isolates were resistant).
Overall, we observed 62.1% MDR globally (Table 2). Multidrug resistance varied by region with higher proportions observed in Africa (80.7%) and Asia (72.7%) than in the Americas (48.7%) or Europe (25.7%). In the United States, 53.3 and 38.4% of isolates collected from University of Maryland Medical Center and Duke University Medical Center were multidrug-resistant, respectively. MDR K. pneumoniae isolates were observed across Europe with the percentage ranging from 16 to 32.4%. The percentage of MDR isolates collected from Japan (31.6%) and Taiwan (16.7%) were lower than that of Singapore (56%) and Pakistan (94.4%).
O Types and D-Galactan Types
Among 645 K. pneumoniae isolates, 44.8% were O1, 19.5% were O2, 14.9% were O3, and 10.9% were O5. Collectively, these four O types accounted for 90.1% of K. pneumoniae strains in 13 countries (Figure 1). One O9 isolate in United States and 3 O12 isolates in two countries (United States and Sweden) were found while 14 O4 isolates were found globally. No O8 isolates were identified. Although we observed diverse profiles of O types between countries, the total percent of O1, O2, O3, and O5 isolates collectively ranged from 72% in Sweden to as high as 100% in Greece. We also evaluated the serotype distribution in the United States over time and found that the prevalence of O1, O2, O3, and O5 remained fairly stable between 2011 and 2015 (Supplementary Figure S3). We observed an increase in the percentage of O1 in the United States from 44% in 2011 to 50% in 2015 and in O5 from 2.2% in 2011 to 10.7% in 2015. In contrast, serotype O2 decreased from 20 to 3.6% though statistically significant differences were not reached due to the small numbers. Some K. pneumoniae O1 and O2 strains have been shown to possess a modified galactan O-antigen type (Stojkovic et al., 2017). We screened 199 O1 and O2 isolates for the presence of gmlABC which encodes this D-galactan III antigen. We detected gmlABC in 49.7% of isolates tested. Our data suggests that D-galactan I is more common in Europe than in other regions (Table 3).
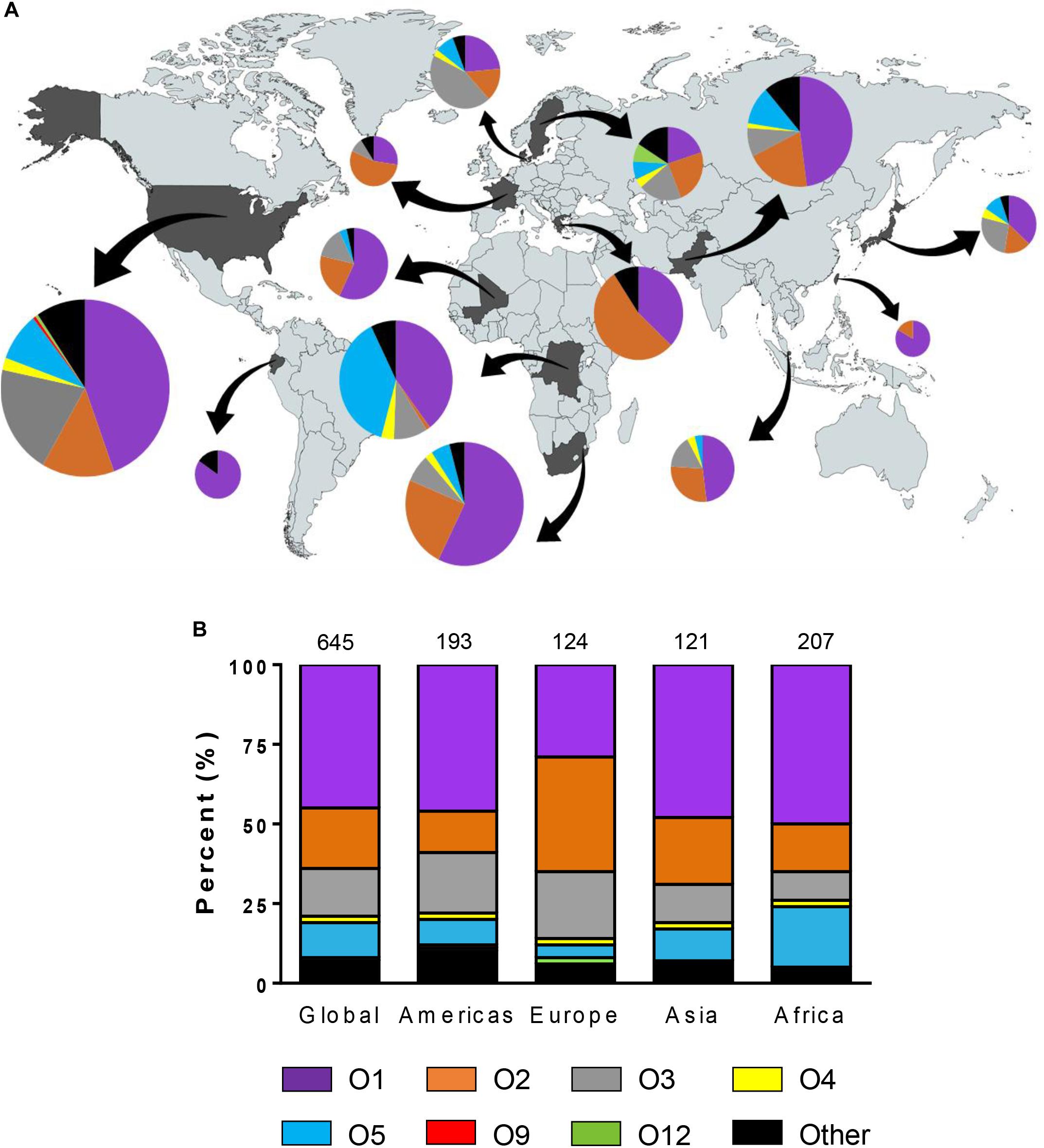
Figure 1. Geographic distribution of Klebsiella pneumoniae O types. (A) Diversity of O types by country. The size of each pie chart represents the number of isolates tested from each country. Arrows indicate each country from which strains were isolated. (B) Diversity of O types by region. The numbers on the top of the graph represent the number of isolates belonging to each region. No O8 strains were found in this study.
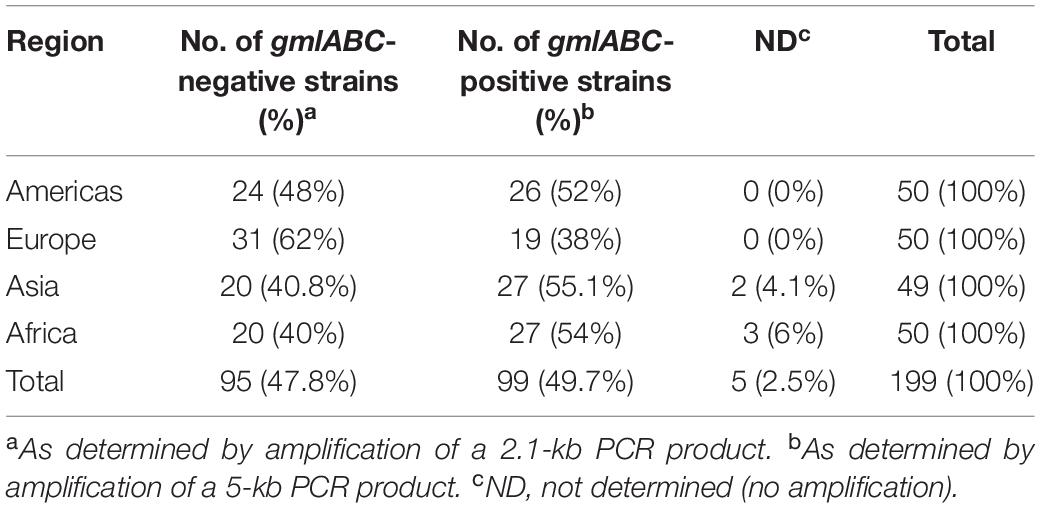
Table 3. Distribution of D-galactan I (gmlABC-negative) and III (gmlABC-positive) in O1 and O2 Klebsiella pneumoniae in 13 countries.
To determine if particular serotypes were associated with antibiotic resistance, we evaluated the serotype distribution amongst susceptible and non-susceptible strains for each antibiotic class and for MDR versus non-MDR strains. The serotype distribution observed among 367 MDR isolates was 49% for O1, 17% for O2, 11% for O3, and 14% for O5, respectively (Figure 2A). There were significantly more O1 and fewer O3 strains in the MDR group compared to the non-MDR group (p < 0.05). The percentage of O serotypes in the susceptible and non-susceptible groups to each antibiotic is shown in Figure 2B. There were significantly more O1 strains that were non-susceptible to aminoglycosides and tetracycline compared to susceptible (p < 0.05). Likewise, there were more O2 strains that were not susceptible to extended spectrum cephalosporins, carbapenems, monobactams, and fluoroquinolones than susceptible. There were more O5 strains that were non-susceptible to aminoglycosides and monobactams than susceptible. In contrast, O3 strains were more likely to be susceptible than non-susceptible (to all classes except carbapenems).
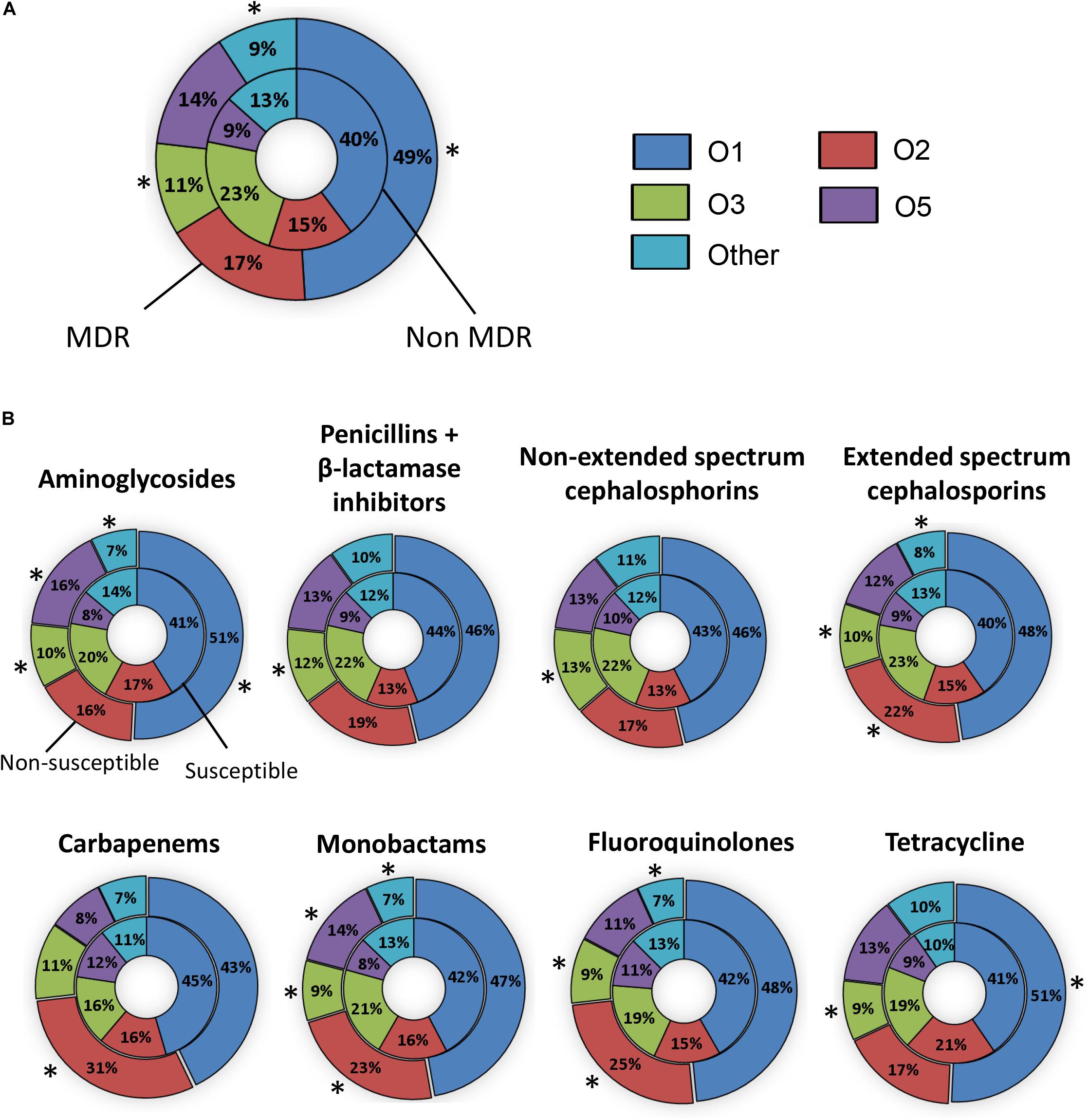
Figure 2. The prevalence of O types in invasive Klebsiella pneumoniae that are susceptible or resistant to antibiotics. (A) The percentage of O types amongst non-MDR (inner pie chart) and MDR isolates (outer pie chart). (B) The percentage of O types amongst isolates susceptible (inner pie chart) and non-susceptible (outer pie chart) to each antibiotic. Statistically significant differences (P ≤ 0.05) between susceptible and non-susceptible isolates are indicated by asterisks (∗). Isolates collected from Greece were not included.
K Types and Phylogenetic Diversity of wzi Sequences
The most common K type by multiplex PCR among 474 strains was K2 (7%). However, 88.4% of isolates were negative for K1, K2, K5, K20, K54, and K57 and therefore could not be typed using this method (Figure 3A). Of 519 isolates, 451 possessed known wzi alleles (149 wzi alleles), of which 298 isolates had known K types (47 K types). Eight new alleles were deposited into public repositories3. The most common K types were K2 (6%) and K24 (7%) (Supplementary Figure S4). A phylogenetic tree of wzi types from 265 randomly selected isolates is shown in Figure 3B. 89.4% of the strains included in this analysis were O1, O2, O3, and O5 and possessed diverse wzi sequences. K2 was identified in two clades according to different wzi sequences, wzi72 and wzi2.
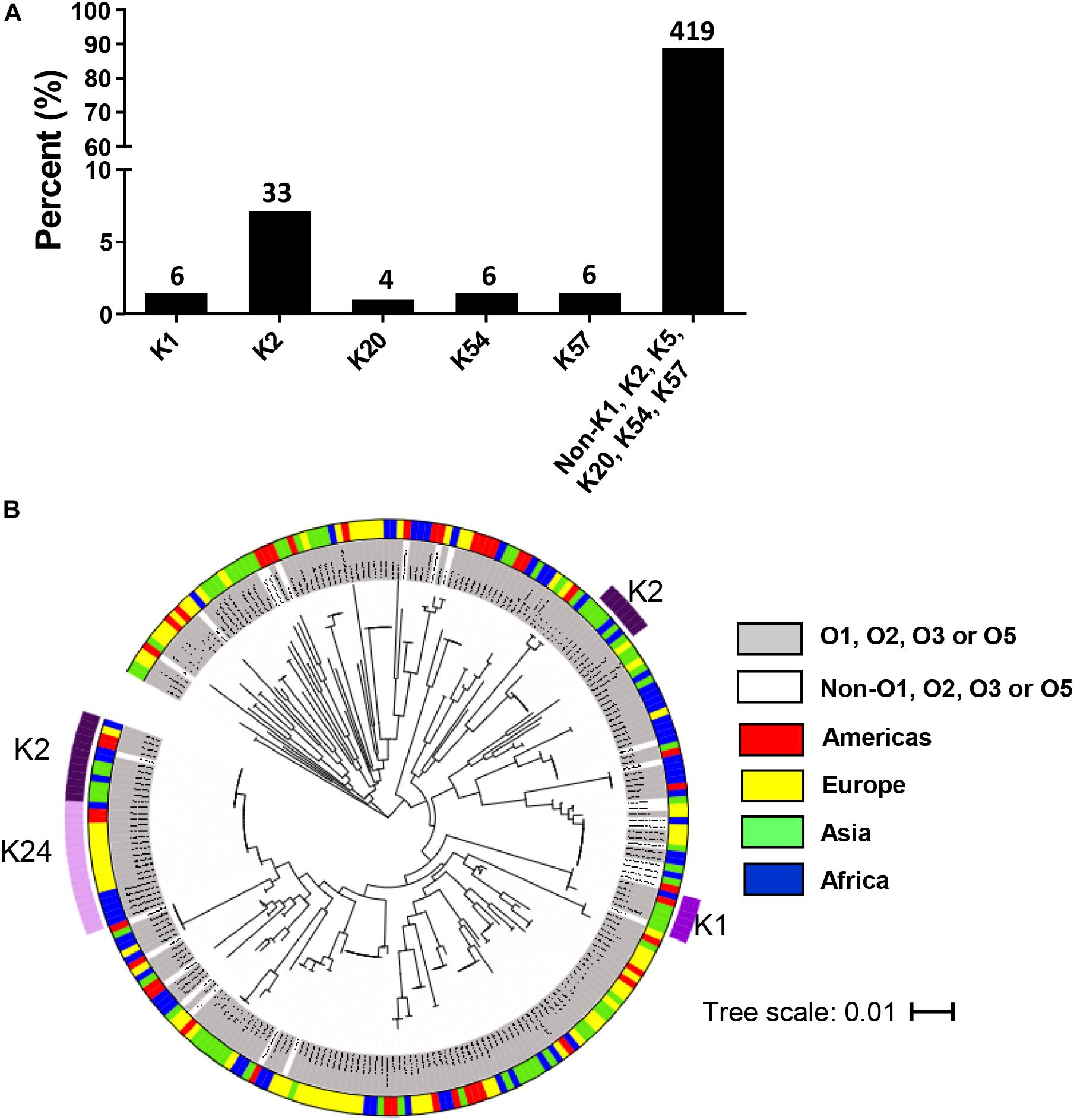
Figure 3. Distribution of K types in invasive Klebsiella pneumoniae isolates in the multi-country collection. (A) K types by multiplex PCR among 474 isolates. The numbers on top of the bars represent the number of isolates belonging to each K type. (B) Phylogenetic tree for wzi sequence of 265 invasive randomly selected strains. The scale bar represents the amount of genetic change; 0.01 equals 1 change per 100 nucleotide sites. The categories of O serotypes and the geographical origins are represented by colors as indicated.
Sequence Types
Sequence types were determined among 10 randomly selected O1:K2 isolates to assess whether K. pneumoniae O1:K2, which are highly pathogenic, share common ancestry lineages worldwide (Yeh et al., 2016). The most common ST among 10 O1:K2 serotype isolates was ST14 (50%, 5 isolates), followed by ST25 (40%, 4 isolates) and ST86 (10%, 1 isolate) (Table 4). All O1:K2 serotype isolates collected from America and Asia were ST14 and ST25. Three different STs were found in four O1:K2 serotype isolates collected from Africa.
To determine if particular STs are associated with a particular serotype from a single geographic location, 29 randomly selected O1, O2, O3, and O5 isolates from University of Maryland Medical Center were tested by MLST and 22 known STs were identified (see Supplementary Table S1). Of the 29 strains, 9 were O1 (31%), 6 were O2 (21%), 8 were O3 (28%), 2 were O4 (7%) and 4 were O5 (14%). While CC14 (2 ST15 isolates) and CC258 (3 ST258 and 1 ST1458) were found, another seven ST singletons were also identified among O1 and O2 isolates. All O3 and O5 isolates were singletons. Two new STs (ST2589 and ST2590) in O1 isolates and one ST2591 in O5 isolates were identified, respectively. We observed multiple STs for each O type.
Discussion
The objective of this study was to determine whether a vaccine that targets K. pneumoniae O1, O2, O3, and O5 antigens would provide coverage against the majority of bacteremic strains circulating worldwide. In this study, we examined rates of antibiotic resistance, the distribution and diversity of O antigens, K antigens, and STs and among 645 invasive clinical isolates collected from 13 countries. This is the largest study of invasive K. pneumoniae that has been performed to date with isolates coming from diverse geographic locations.
Carbapenems have been considered as a treatment of last choice for multidrug-resistant K. pneumoniae (Meletis, 2016; Pennini et al., 2017). Recently, the increased emergence of K. pneumoniae resistant to those antibiotics has become a serious problem worldwide. We observed the high rate of non-susceptibility to carbapenems (Table 2), suggesting that antibiotic treatment options for multidrug-resistant K. pneumoniae are becoming limited. We observed higher multidrug resistance (62.1%) than Hackel et al. (2016) who found 30.1% MDR out of 9,098 K. pneumoniae isolates collected in 2012–2014 from 176 geographic sites. These strains were isolated predominantly from intra-abdominal infections, urinary tract infections, skin and soft tissue infections and lower respiratory tract infections. Unlike our collection, only 3.8% of the isolates were from blood. Further, the higher proportion of multidrug resistant organisms that we observed in our study may be due to inclusion of isolates from sub-Saharan Africa and South Asia which show high rates of MDR (Investigators of the Delhi Neonatal Infection Study (DeNIS) collaboration, 2016; Henson et al., 2017; Musicha et al., 2017). Hackel et al. described isolates from South Africa but no other African countries and no isolates from India, Bangladesh or Pakistan (Hackel et al., 2016).
We found that 90.1% of the K. pneumoniae isolates were O1, O2, O3, or O5, consistent with previous reports (Figure 1; Trautmann et al., 1997; Follador et al., 2016). O1 and O2 K. pneumoniae isolates have been considered important pathogens due to their high prevalence, virulence and rate of antibiotic resistance (Fang et al., 2016; Szijártó et al., 2016; Pennini et al., 2017). While D-galactan I antigen was first identified as a repeat unit of O polysaccharide in O1 and O2 isolates, some O1 and O2 strains were recently reported to possess a modified D-galactan I, called D-galactan III (Whitfield et al., 1992; Szijártó et al., 2016; Stojkovic et al., 2017). In this study, 49.7% isolates carried the gmlABC operon which is associated with D-gal III synthesis. Similarly, Szijártó et al. (2016) reported that 40% of O1 isolates and 68% of O2 isolates possess the gmlABC operon (Szijártó et al., 2016). These results imply that an O-polysaccharide-based vaccine would need to ensure that antibodies recognize both the D-gal III epitope as well as the D-gal I epitope to achieve maximal coverage (Stojkovic et al., 2017).
Although O1 and O2 strains are the most common serotypes globally (64.3%), 25.7% of strains tested were determined to be O3 or O5. These findings suggest that vaccines for preventing infection caused by O3 and O5 K. pneumoniae are also necessary. No O8 isolate was found among 645 K. pneumoniae in line with that seen in studies by other investigators (Follador et al., 2016). It should be noted that K. pneumoniae O1 and O8 O polysaccharide are serologically similar and O1 OPS would likely protect against O8 strains (Trautmann et al., 1997). O12 isolates have been isolated from several locations in Europe including Spain, Denmark, and United Kingdom (Hansen et al., 1999; Follador et al., 2016). We found only 3 O12 isolates from United States and Sweden. Thus, targeting O1, O2, O3, and O5, but not O12, would be important for development of a broad spectrum vaccine against K. pneumoniae. In the current study, the most common O type was O1, regardless of antibiotic resistance, which is associated with high bacterial dissemination and colonization into organs (Hsieh et al., 2014). Pennini et al. (2017) reported that O2 was the dominant serotype of MDR K. pneumoniae and associated with ß-lactam and carbapenem-resistance (Pennini et al., 2017). In our study, although O2 was associated with resistance to extended spectrum cephalosporins, carbapenems, monobactams, and fluoroquinolones (Figure 2B), O1 was the dominant serotype of MDR K. pneumoniae and associated with resistance to aminoglycosides and tetracycline. O5 strains were associated with non-susceptibility to aminoglycosides, and monobactams. In the Pennini et al. (2017) study, 57.7% of isolates were from respiratory sites and only 9.2% were from blood/peritoneum. All of the isolates examined in the current study were from blood culture. In addition, the distribution of isolates from geographic sites was different in the Pennini et al. (2017) study compared to our study. A higher proportion of isolates were from Europe (38% for Pennini et al. versus 19% for our study) and a lower proportion of strains were from Africa (5% for Pennini et al. versus 32% for our study). This may explain the difference in serotype distribution that we observed amongst MDR strains compared to Pennini et al. (2017). Collectively, the data suggests that it is important to prevent K. pneumoniae O1 infections due to their high prevalence and virulence and serotypes O2 and O5 due to their association with antibiotic resistance.
A high prevalence of K2 isolates among 519 invasive strains was similarly observed by Paczosa and Mecsas (2016). K24 was the most common capsule type in our study. The hypervirulent K1 capsule type (Siu et al., 2011) was found amongst isolates collected from United States, Taiwan, South Africa, DR Congo, and Pakistan by wzi capsular typing. Diverse K types were identified as has been previously reported (Cryz et al., 1986b), indicating that a vaccine that targets only 4 O antigens would be less difficult and less expensive to construct and provide broad coverage compared to a vaccine that targets the capsular polysaccharide (that would need 24 K antigens to provide the same coverage).
Our data showed that clonally diverse K. pneumoniae caused bacteremia in patients in 13 countries. Additionally, there was no association between particular O serotypes and sequence types. Therefore, MLST data cannot be used as a proxy for O typing. Furthermore, a strategy to target antigens specific for certain STs might be insufficient to prevent infections caused by diverse lineages of K. pneumoniae.
In summary, we determined that there are 4 major O antigen types among 645 strains and 49 K antigens among 519 strains collected from 13 countries. In addition, diverse STs and a high rate of MDR K. pneumoniae was determined. Therefore, we believe that a K. pneumoniae O antigen-based vaccine would offer an excellent alternative strategy to a vaccine based on capsular polysaccharide which has previously been proposed, developed and evaluated in a Phase 1 study (Edelman et al., 1994). Furthermore, by preventing K. pneumoniae infections from occurring in the first place, this will eliminate the need for antibiotics and will therefore remove selective pressure for resistance (Klugman and Black, 2018). Additionally, prevention is far superior to treatment as those patients who survive sepsis suffer multiple long-term complications. Our data suggests that our proposed quadrivalent vaccine (Hegerle et al., 2018) could potentially prevent 90.1% of K. pneumoniae bacteremic infections worldwide.
Data Availability Statement
The original contributions presented in the study are included in the article/Supplementary Material. Further inquiries can be directed to the corresponding author.
Author Contributions
ST, RS, and AC designed the study. MC, ST, RS, and AC performed the analysis and wrote the manuscript. MT, JKJ, SM, JT, VF, AA, ET, DD, FM, KK, EC, YS, Y-TL, SaS, OP, AS-M, JJ, and OL collected the strains. NH, JN, SuS, SJ, SN, ShS, JP-B, and JS performed the assays. All authors listed have made a substantial, direct and intellectual contribution to the work, and approved it for publication.
Funding
The authors declare that this study received funding from Nosocomial Vaccine Corporation. The funder was not involved in the study design, collection, analysis, interpretation of data, the writing of this article or the decision to submit it for publication.
Conflict of Interest
ST, RS, and AC are holders of US patent 9,988,426 titled “Broad spectrum conjugate vaccine to prevent Klebsiella pneumoniae and Pseudomonas aeruginosa infections.”
The remaining authors declare that the research was conducted in the absence of any commercial or financial relationships that could be construed as a potential conflict of interest.
Acknowledgments
We thank Ghazala Shaheen and Humaira Shafaq from Department of Pathology and Pediatrics, the Aga Khan University, Karachi, Pakistan; Efthimia Protonotariou and Lemonia Skoura at Department of Microbiology, AHEPA University Hospital, Greece; Marie-France Phoba from Department of Clinical Microbiology, National Institute for Biomedical Research, and Department of Microbiology, University Hospital of Kinshasa, Kinshasa, Democratic Republic of the Congo; Barbara Barbé at Department of Clinical Sciences, Institute of Tropical Medicine, Antwerp, Belgium; Jorge Reyes from Instituto de Microbiologia, Universidad San Francisco de Quito, Quito, Ecuador; Jose Eduardo Villacis at Instituto Nacional de Investigación en Salud Pública, Ministerio de Salud Publica del Ecuador, Quito, Ecuador; Tze-Peng Lim at Department of Pharmacy, Singapore General Hospital, Singapore, Singapore and Emerging Infectious Diseases, Duke-National University of Singapore Graduate Medical School, Singapore, Singapore; Samba O. Sow, Adama Mamby Keita, Boubou Tamboura, and Aliou Toure from Centre pour le Développement des Vaccins, Mali, Bamako, Mali; Karen L. Kotloff from Center for Vaccine Development and Global Health and Department of Pediatrics, University of Maryland School of Medicine, Baltimore, MD, United States. We also thank the team of curators of the Institute Pasteur MLST and whole genome MLST databases for curating the data and making them publicly available at http://bigsdb.pasteur.fr/.
Supplementary Material
The Supplementary Material for this article can be found online at: https://www.frontiersin.org/articles/10.3389/fmicb.2020.01249/full#supplementary-material
FIGURE S1 | The number of K. pneumoniae isolates analyzed by year and country.
FIGURE S2 | The number of K. pneumoniae isolates tested by each method.
FIGURE S3 | Trends in O antigens of invasive K. pneumoniae collected from University of Maryland Medical Center in 2011–2015.
FIGURE S4 | Distribution of K types by wzi gene sequencing among 519 isolates. Allele# represents wzi alleles which have been assigned an allele number by the wzi database at http://bigsdb.web.pasteur.fr but which do not correspond to a K type.
TABLE S1 | Sequence types of 39 isolates with O1, O2, O3, O4, and O5 antigens.
TABLE S2 | Resistance of 591 K. pneumoniae isolated from blood to 12 different antibiotics.
Footnotes
- ^ https://mapchart.net/
- ^ www.pasteur.fr/mlst
- ^ https://bigsdb.pasteur.fr/klebsiella/klebsiella.html
References
Baykara, N., Akalın, H., Arslantaş, M. K., Hancı, V., Çağlayan, Ç, Kahveci, F., et al. (2018). Epidemiology of sepsis in intensive care units in Turkey: a multicenter, point-prevalence study. Crit. Care 22:93. doi: 10.1186/s13054-018-2013-1
Bialek-Davenet, S., Criscuolo, A., Ailloud, F., Passet, V., Jones, L., Delannoy-Vieillard, A. S., et al. (2014). Genomic definition of hypervirulent and multidrug-resistant Klebsiella pneumoniae clonal groups. Emerg. Infect. Dis. 20, 1812–1820. doi: 10.3201/eid2011.140206
Brisse, S., Passet, V., Haugaard, A. B., Babosan, A., Kassis-Chikhani, N., Struve, C., et al. (2013). wzi gene sequencing, a rapid method for determination of capsular type for Klebsiella strains. J. Clin. Microbiol. 51, 4073–4078. doi: 10.1128/jcm.01924-13
Cai, Y., Venkatachalam, I., Tee, N. W., Tan, T. Y., Kurup, A., Wong, S. Y., et al. (2017). Prevalence of healthcare-associated infections and antimicrobial use among adult inpatients in Singapore acute-care hospitals: results from the first national point prevalence survey. Clin. Infect. Dis. 64, S61–S67. doi: 10.1093/cid/cix103
Cerceo, E., Deitelzweig, S. B., Sherman, B. M., and Amin, A. N. (2016). Multidrug-resistant gram-negative bacterial infections in the hospital setting: overview, implications for clinical practice, and emerging treatment options. Microb. Drug. Resist. 22, 412–431. doi: 10.1089/mdr.2015.0220
Chhibber, S., Rani, M., and Vanashree, Y. (2005). Immunoprotective potential of polysaccharide-tetanus toxoid conjugate in Klebsiella pneumoniae induced lobar pneumonia in rats. Indian. J. Exp. Biol. 43, 40–45.
Clarke, B. R., Ovchinnikova, O. G., Kelly, S. D., Williamson, M. L., Butler, J. E., Liu, B., et al. (2018). Molecular basis for the structural diversity in serogroup O2-antigen polysaccharides in Klebsiella pneumoniae. J. Biol. Chem. 293, 4666–4679. doi: 10.1074/jbc.RA117.000646
Clements, A., Jenney, A. W., Farn, J. L., Brown, L. E., Deliyannis, G., Hartland, E. L., et al. (2008). Targeting subcapsular antigens for prevention of Klebsiella pneumoniae infections. Vaccine 26, 5649–5653. doi: 10.1016/j.vaccine.2008.07.100
Cryz, S. J. Jr., Mortimer, P., Cross, A. S., Furer, E., and Germanier, R. (1986a). Safety and immunogenicity of a polyvalent Klebsiella capsular polysaccharide vaccine in humans. Vaccine 4, 15–20. doi: 10.1016/0264-410x(86)90092-7
Cryz, S. J. Jr., Mortimer, P. M., Mansfield, V., and Germanier, R. (1986b). Seroepidemiology of Klebsiella bacteremic isolates and implications for vaccine development. J. Clin. Microbiol. 23, 687–690. doi: 10.1128/jcm.23.4.687-690.1986
Diancourt, L., Passet, V., Verhoef, J., Grimont, P. A., and Brisse, S. (2005). Multilocus sequence typing of Klebsiella pneumoniae nosocomial isolates. J. Clin. Microbiol. 43, 4178–4182. doi: 10.1128/jcm.43.8.4178-4182.2005
Edelman, R., Taylor, D. N., Wasserman, S. S., McClain, J. B., Cross, A. S., Sadoff, J. C., et al. (1994). Phase 1 trial of a 24-valent Klebsiella capsular polysaccharide vaccine and an eight-valent Pseudomonas O-polysaccharide conjugate vaccine administered simultaneously. Vaccine 12, 1288–1294. doi: 10.1016/s0264-410x(94)80054-4
Fang, C. T., Shih, Y. J., Cheong, C. M., and Yi, W. C. (2016). Rapid and accurate determination of lipopolysaccharide O-antigen types in Klebsiella pneumoniae with a novel PCR-based O-genotyping method. J. Clin. Microbiol. 54, 666–675. doi: 10.1128/jcm.02494-15
Folgori, L., Bernaschi, P., Piga, S., Carletti, M., Cunha, F. P., Lara, P. H., et al. (2016). Healthcare-associated infections in pediatric and neonatal intensive care units: impact of underlying risk factors and antimicrobial resistance on 30-day case-fatality in Italy and Brazil. Infect. Control. Hosp. Epidemiol. 37, 1302–1309. doi: 10.1017/ice.2016.185
Follador, R., Heinz, E., Wyres, K. L., Ellington, M. J., Kowarik, M., Holt, K. E., et al. (2016). The diversity of Klebsiella pneumoniae surface polysaccharides. Microb. Genom. 2:e000073. doi: 10.1099/mgen.0.000073
Hackel, M., Kazmierczak, K. M., Hoban, D. J., Biedenbach, D. J., Bouchillon, S. K., de Jonge, B. L., et al. (2016). Assessment of the in vitro activity of ceftazidime-avibactam against multidrug-resistant Klebsiella spp. Collected in the INFORM global surveillance study, 2012 to 2014. Antimicrob. Agents Chemother. 60, 4677–4683. doi: 10.1128/aac.02841-15
Hansen, D. S., Mestre, F., Alberti, S., Hernandez-Alles, S., Alvarez, D., Domenech-Sanchez, A., et al. (1999). Klebsiella pneumoniae lipopolysaccharide O typing: revision of prototype strains and O-group distribution among clinical isolates from different sources and countries. J. Clin. Microbiol. 37, 56–62. doi: 10.1128/jcm.37.1.56-62.1999
Hegerle, N., Choi, M., Sinclair, J., Amin, M. N., Ollivault-Shiflett, M., Curtis, B., et al. (2018). Development of a broad spectrum glycoconjugate vaccine to prevent wound and disseminated infections with Klebsiella pneumoniae and Pseudomonas aeruginosa. PLoS One 13:e0203143. doi: 10.1371/journal.pone.0203143
Henson, S. P., Boinett, C. J., Ellington, M. J., Kagia, N., Mwarumba, S., Nyongesa, S., et al. (2017). Molecular epidemiology of Klebsiella pneumoniae invasive infections over a decade at Kilifi County Hospital in Kenya. Int. J. Med. Microbiol. 307, 422–429. doi: 10.1016/j.ijmm.2017.07.006
Hsieh, P. F., Wu, M. C., Yang, F. L., Chen, C. T., Lou, T. C., Chen, Y. Y., et al. (2014). D-galactan II is an immunodominant antigen in O1 lipopolysaccharide and affects virulence in Klebsiella pneumoniae: implication in vaccine design. Front. Microbiol. 5:608. doi: 10.3389/fmicb.2014.00608
Investigators of the Delhi Neonatal Infection Study (DeNIS) collaboration (2016). Characterisation and antimicrobial resistance of sepsis pathogens in neonates born in tertiary care centres in Delhi, India: a cohort study. Lancet Glob. Health 4, e752–e760. doi: 10.1016/s2214-109x(16)30148-6
Kelly, R. F., Severn, W. B., Richards, J. C., Perry, M. B., MacLean, L. L., and Tomás, J. M. (1993). Structural variation in the O-specific polysaccharides of Klebsiella pneumoniae serotype O1 and O8 lipopolysaccharide: evidence for clonal diversity in rfb genes. Mol. Microbiol. 10, 615–625. doi: 10.1111/j.1365-2958.1993.tb00933.x
Klugman, K. P., and Black, S. (2018). Impact of existing vaccines in reducing antibiotic resistance: primary and secondary effects. Proc. Natl. Acad. Sci. U.S.A. 115, 12896–12901. doi: 10.1073/pnas.1721095115
Lee, C. R., Lee, J. H., Park, K. S., Jeon, J. H., Kim, Y. B., Cha, C. J., et al. (2017). Antimicrobial resistance of hypervirulent Klebsiella pneumoniae: epidemiology, hypervirulence-associated determinants, and resistance mechanisms. Front. Cell. Infect. Microbiol. 7:483. doi: 10.3389/fcimb.2017.00483
Lee, W. H., Choi, H. I., Hong, S. W., Kim, K. S., Gho, Y. S., and Jeon, S. G. (2015). Vaccination with Klebsiella pneumoniae-derived extracellular vesicles protects against bacteria-induced lethality via both humoral and cellular immunity. Exp. Mol. Med. 47:e183. doi: 10.1038/emm.2015.59
Lundberg, U., Senn, B. M., Schuler, W., Meinke, A., and Hanner, M. (2013). Identification and characterization of antigens as vaccine candidates against Klebsiella pneumoniae. Hum. Vaccin. Immunother. 9, 497–505. doi: 10.4161/hv.23225
Magill, S. S., Edwards, J. R., Bamberg, W., Beldavs, Z. G., Dumyati, G., Kainer, M. A., et al. (2014). Multistate point-prevalence survey of health care-associated infections. N. Engl. J. Med. 370, 1198–1208. doi: 10.1056/NEJMoa1306801
Magill, S. S., O’Leary, E., Janelle, S. J., Thompson, D. L., Dumyati, G., Nadle, J., et al. (2018). Changes in prevalence of health care-associated infections in U.S. hospitals. N. Engl. J. Med. 379, 1732–1744. doi: 10.1056/NEJMoa1801550
Magiorakos, A. P., Srinivasan, A., Carey, R. B., Carmeli, Y., Falagas, M. E., Giske, C. G., et al. (2012). Multidrug-resistant, extensively drug-resistant and pandrug-resistant bacteria: an international expert proposal for interim standard definitions for acquired resistance. Clin. Microbiol. Infect. 18, 268–281. doi: 10.1111/j.1469-0691.2011.03570.x
Martin, R. M., Cao, J., Brisse, S., Passet, V., Wu, W., Zhao, L., et al. (2016). Molecular epidemiology of colonizing and infecting isolates of Klebsiella pneumoniae. mSphere 1:e00261-16. doi: 10.1128/mSphere.00261-16
Meatherall, B. L., Gregson, D., Ross, T., Pitout, J. D., and Laupland, K. B. (2009). Incidence, risk factors, and outcomes of Klebsiella pneumoniae bacteremia. Am. J. Med. 122, 866–873. doi: 10.1016/j.amjmed.2009.03.034
Meletis, G. (2016). Carbapenem resistance: overview of the problem and future perspectives. Ther. Adv. Infect. Dis. 3, 15–21. doi: 10.1177/2049936115621709
Musicha, P., Cornick, J. E., Bar-Zeev, N., French, N., Masesa, C., Denis, B., et al. (2017). Trends in antimicrobial resistance in bloodstream infection isolates at a large urban hospital in Malawi (1998-2016): a surveillance study. Lancet Infect. Dis. 17, 1042–1052. doi: 10.1016/s1473-3099(17)30394-8
Ørskov, I., and Ørskov, F. (1984). Serotyping of Klebsiella. Methods Microbiol. 14, 143–164. doi: 10.1016/s0580-9517(08)70449-5
Paczosa, M. K., and Mecsas, J. (2016). Klebsiella pneumoniae: going on the offense with a strong defense. Microbiol. Mol. Biol. Rev. 80, 629–661. doi: 10.1128/mmbr.00078-15
Pennini, M. E., De Marco, A., Pelletier, M., Bonnell, J., Cvitkovic, R., and Beltramello et al. (2017). Immune stealth-driven O2 serotype prevalence and potential for therapeutic antibodies against multidrug resistant Klebsiella pneumoniae. Nat. Commun. 8:1991. doi: 10.1038/s41467-017-02223-7
Shankar-Sinha, S., Valencia, G. A., Janes, B. K., Rosenberg, J. K., Whitfield, C., Bender, R. A., et al. (2004). The Klebsiella pneumoniae O antigen contributes to bacteremia and lethality during murine pneumonia. Infect. Immun. 72, 1423–1430. doi: 10.1128/iai.72.3.1423-1430.2004
Simoons-Smit, A. M., Verweij-van Vught, A. M., and MacLaren, D. M. (1986). The role of K antigens as virulence factors in Klebsiella. J. Med. Microbiol. 21, 133–137. doi: 10.1099/00222615-21-2-133
Siu, L. K., Fung, C. P., Chang, F. Y., Lee, N., Yeh, K. M., Koh, T. H., et al. (2011). Molecular typing and virulence analysis of serotype K1 Klebsiella pneumoniae strains isolated from liver abscess patients and stool samples from noninfectious subjects in Hong Kong, Singapore, and Taiwan. J. Clin. Microbiol. 29, 3761–3765. doi: 10.1128/JCM.00977-11
Stojkovic, K., Szijártó, V., Kaszowska, M., Niedziela, T., Hartl, K., Nagy, G., et al. (2017). Identification of d-Galactan-III as part of the lipopolysaccharide of Klebsiella pneumoniae serotype O1. Front. Microbiol. 8:684. doi: 10.3389/fmicb.2017.00684
Szijártó, V., Guachalla, L. M., Hartl, K., Varga, C., Banerjee, P., Stojkovic, K., et al. (2016). Both clades of the epidemic KPC-producing Klebsiella pneumoniae clone ST258 share a modified galactan O-antigen type. Int. J. Med. Microbiol. 306, 89–98. doi: 10.1016/j.ijmm.2015.12.002
Trautmann, M., Held, T. K., and Cross, A. S. (2004). O antigen seroepidemiology of Klebsiella clinical isolates and implications for immunoprophylaxis of Klebsiella infections. Vaccine 22, 818–821. doi: 10.1016/j.vaccine.2003.11.026
Trautmann, M., Ruhnke, M., Rukavina, T., Held, T. K., Cross, A. S., Marre, R., et al. (1997). O-antigen seroepidemiology of Klebsiella clinical isolates and implications for immunoprophylaxis of Klebsiella infections. Clin. Diagn. Lab. Immunol. 4, 550–555. doi: 10.1128/cdli.4.5.550-555.1997
Turton, J. F., Perry, C., Elgohari, S., and Hampton, C. V. (2010). PCR characterization and typing of Klebsiella pneumoniae using capsular type-specific, variable number tandem repeat and virulence gene targets. J. Med. Microbiol. 59, 541–547. doi: 10.1099/jmm.0.015198-0
Walter, J., Haller, S., Quinten, C., Kärki, T., Zacher, B., Eckmanns, T., et al. (2018). Healthcare-associated pneumonia in acute care hospitals in European Union/European economic area countries: an analysis of data from a point prevalence survey, 2011 to 2012. Euro. Surveill. 23:1700843. doi: 10.2807/1560-7917.ES.2018.23.32.1700843
Whitfield, C., Perry, M. B., MacLean, L. L., and Yu, S. H. (1992). Structural analysis of the O-antigen side chain polysaccharides in the lipopolysaccharides of Klebsiella serotypes O2(2a), O2(2a,2b), and O2(2a,2c). J. Bacteriol. 174, 4913–4919. doi: 10.1128/jb.174.15.4913-4919.1992
Whitfield, C., Richards, J. C., Perry, M. B., Clarke, B. R., and MacLean, L. L. (1991). Expression of two structurally distinct D-galactan O antigens in the lipopolysaccharide of Klebsiella pneumoniae serotype O1. J. Bacteriol. 173, 1420–1431. doi: 10.1128/jb.173.4.1420-1431.1991
Keywords: Klebsiella pneumoniae, multidrug resistance, vaccine, O antigen, K antigen
Citation: Choi M, Hegerle N, Nkeze J, Sen S, Jamindar S, Nasrin S, Sen S, Permala-Booth J, Sinclair J, Tapia MD, Johnson JK, Mamadou S, Thaden JT, Fowler VG Jr, Aguilar A, Terán E, Decre D, Morel F, Krogfelt KA, Brauner A, Protonotariou E, Christaki E, Shindo Y, Lin Y-T, Kwa AL, Shakoor S, Singh-Moodley A, Perovic O, Jacobs J, Lunguya O, Simon R, Cross AS and Tennant SM (2020) The Diversity of Lipopolysaccharide (O) and Capsular Polysaccharide (K) Antigens of Invasive Klebsiella pneumoniae in a Multi-Country Collection. Front. Microbiol. 11:1249. doi: 10.3389/fmicb.2020.01249
Received: 22 October 2019; Accepted: 15 May 2020;
Published: 12 June 2020.
Edited by:
Miklos Fuzi, Semmelweis University, HungaryReviewed by:
Sylvain Brisse, Institut Pasteur, FranceKwan Soo Ko, Sungkyunkwan University School of Medicine, South Korea
David Gordon, The Australian National University, Australia
Copyright © 2020 Choi, Hegerle, Nkeze, Sen, Jamindar, Nasrin, Sen, Permala-Booth, Sinclair, Tapia, Johnson, Mamadou, Thaden, Fowler, Aguilar, Terán, Decre, Morel, Krogfelt, Brauner, Protonotariou, Christaki, Shindo, Lin, Kwa, Shakoor, Singh-Moodley, Perovic, Jacobs, Lunguya, Simon, Cross and Tennant. This is an open-access article distributed under the terms of the Creative Commons Attribution License (CC BY). The use, distribution or reproduction in other forums is permitted, provided the original author(s) and the copyright owner(s) are credited and that the original publication in this journal is cited, in accordance with accepted academic practice. No use, distribution or reproduction is permitted which does not comply with these terms.
*Correspondence: Sharon M. Tennant, c3Rlbm5hbnRAc29tLnVtYXJ5bGFuZC5lZHU=
†These authors have contributed equally to this work and share senior authorship