- 1Laboratory of Molecular Cell Biology, Department of Biology, Institute of Botany and Microbiology, KU Leuven, Leuven, Belgium
- 2VIB-KU Leuven Center for Microbiology, Leuven, Belgium
Candida albicans and Staphylococcus species are, respectively, the most common fungal and bacterial agents isolated from bloodstream infections, worldwide. Moreover, it has been shown that 20% of all C. albicans bloodstream infections are polymicrobial in nature, with Staphylococcus epidermidis and Staphylococcus aureus being the first and third most common co-isolated organisms, respectively. These species are part of the commensal microbial flora but can cause hospital-acquired infections with an extreme ability to inhabit diverse host niches, especially in immunocompromised patients. They are well known for their ability to form persistent biofilms in the host or on abiotic surfaces such as indwelling medical devices. Interactions within these biofilm communities can lead to increased virulence, drug tolerance, and immune evasion. This can ultimately impact morbidity and infection outcome, often leading to an increased mortality. Therefore, characterizing the interactions between these species could lead to the development of novel therapeutic approaches that target polymicrobial infections. In this mini review, we briefly highlight the current knowledge and most recent insights into the complex interspecies interactions of C. albicans with Staphylococcus bacteria.
Introduction
Candida albicans is a commensal fungus that colonizes the oral cavity, vagina, and gastro-intestinal tract in most humans (Nobile and Johnson, 2015). It is however also an opportunistic pathogen, able to cause both superficial and systemic infections, the latter mainly in immunocompromised patients. In certain niches of the host, C. albicans co-exists with commensal bacteria, including Staphylococcus species (Tsui et al., 2016). Staphylococcus epidermidis and Staphylococcus aureus are, respectively, the first and third most co-isolated pathogens with C. albicans in systemic blood stream infections. Additionally, more than one in four cases of candidemia is estimated to be polymicrobial, whereas systemic Staphylococcus infections without Candida are less common (Klotz et al., 2007a).
The significance of polymicrobial infections caused by a mixture of bacteria and fungi is increasingly recognized in medical settings (Brogden et al., 2005). Furthermore, a vast number of infections, including those of C. albicans and Staphylococcus species, originate from biofilms and they are often associated with high mortality rates (Tsui et al., 2016). C. albicans and Staphylococcus species were co-isolated from various biofilm-associated diseases, including periodontitis, cystic fibrosis, denture stomatitis, urinary tract, burn wound infections, and infections of medical devices such as central venous catheters (Adam et al., 2002; Baena-Monroy et al., 2005; Gupta et al., 2005; Valenza et al., 2008; Cuesta et al., 2010). The complexity of these polymicrobial infections poses an additional challenge to find efficient treatment strategies (Koo et al., 2017).
This mini review elaborates on the current knowledge and recent insights in the interactions, altered drug tolerance and infection outcome, and possible treatment strategies of polymicrobial C. albicans-Staphylococcus species infections.
Molecular Interactions of C. Albicans With Staphylococcus Species
Physical Interactions
Different research groups have focused on the characterization of the interaction between C. albicans and Staphylococcus species at a molecular level. An early study by El-Azizi and colleagues showed enhanced adhesion and biofilm formation of C. albicans upon addition of S. epidermidis, indicating a possible symbiotic interaction between both species (El-Azizi et al., 2004). Peters et al. observed that staphylococci, with S. aureus in particular, predominantly adhere to the hyphae of C. albicans resulting in a unique biofilm architecture (Peters et al., 2010).
Later, the importance of C. albicans Als membrane proteins in C. albicans-Staphylococcus species aggregation was recognized (Klotz et al., 2007b). While Als5, Als6, and Als7 adhesins are associated with adhesion to human endothelium, the Als3 protein was identified as a C. albicans hyphae specific receptor which binds S. aureus (Peters et al., 2012) and S. epidermidis (Beaussart et al., 2013). These hyphae specific associations of S. aureus (Ovchinnikova et al., 2012) and S. epidermidis (Beaussart et al., 2013) further stresses the importance of C. albicans yeast-to-hyphae morphogenesis in mixed species biofilm formation. However, it was observed that even in the C. albicans als3 mutant strain, S. aureus cells attach to the fungal hyphae, suggesting that either additional cell surface molecules, biofilm extracellular matrix (ECM) components or non-specific hydrophobic and electrostatic interactions may play a role in interspecies aggregation as well (Peters et al., 2012). A single-cell force spectroscopy study suggested that C. albicans Als1 and cell surface O-mannosylations interact with specific peptide ligands containing an “τφ+” motif and lectin binding sites on the S. epidermidis surface, respectively (Beaussart et al., 2013). Specific and direct interspecies cell surface interactions between C. albicans and Staphylococcus species might at least partly explain the enhanced biofilm growth (El-Azizi et al., 2004), drug tolerance (Adam et al., 2002; Harriott and Noverr, 2010; Kong et al., 2016), and increased morbidity (Kong et al., 2015; Nash et al., 2016) in polymicrobial infections (Figure 1A).
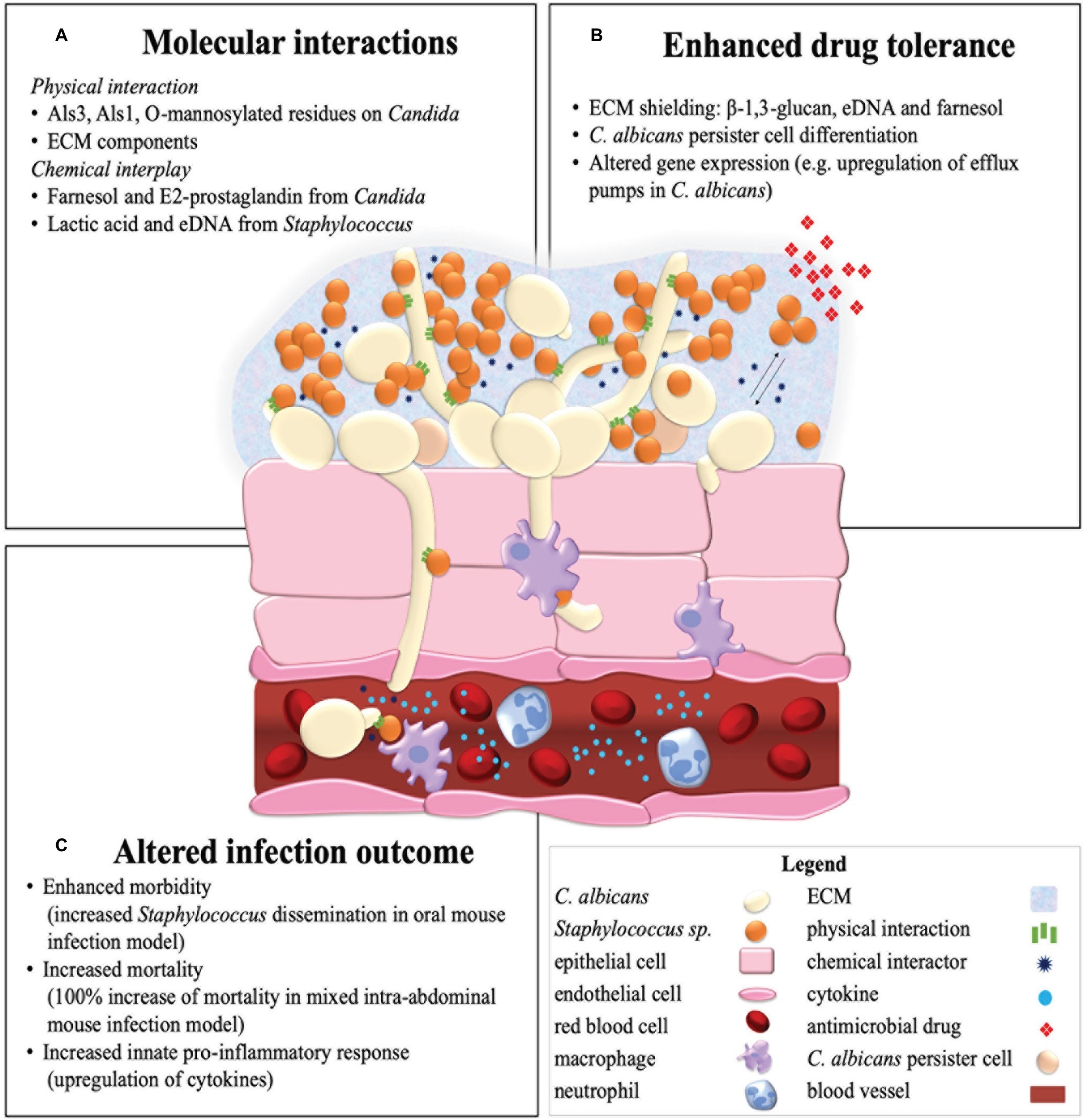
Figure 1. Summary illustration of a mixed C. albicans and Staphylococcus species biofilm in a host environment. Represented are the molecular interactions of C. albicans and Staphylococcus species (A) in an invasive endothelial infection with an enhanced antimicrobial drug tolerance (B) and altered infection outcome (C) as a result.
Chemical Interplay
In one of the earliest studies of C. albicans-S. aureus interactions, it was suggested that secreted products of C. albicans may be the cause of the enhanced growth of S. aureus in co-infection (Staib et al., 1976). Besides physical interactions, secreted effectors such as quorum sensing (QS) molecules and small secreted metabolites indeed prove to be involved in interspecies communication. Quorum sensing, i.e., density dependent intercellular signaling through small secreted molecules, has been found in both Staphylococcus (Kong et al., 2006) and Candida species (Albuquerque and Casadevall, 2012). During aggregation or biofilm formation, inter and intra-kingdom QS intensifies and might be able to act as a significant biofilm developmental regulator (Morales and Hogan, 2010). The most widely described QS molecule of C. albicans is farnesol (Hornby et al., 2001). Farnesol reduces C. albicans yeast-to-hyphae morphogenesis and so consequently reduces biofilm growth (Ramage et al., 2002; Piispanen et al., 2011). Moreover, it was found that farnesol reduced S. aureus viability and impedes S. aureus biofilm development when present in high concentrations (>100 μM) (Jabra-Rizk et al., 2006). On the contrary, it is shown that low concentrations of farnesol (0.5–5 nM) promote S. aureus biofilm formation (Krause et al., 2015). Moreover, intermediate concentrations of farnesol (40 μM) that are physiologically relevant within the C. albicans biofilm were found to enhance drug tolerance of S. aureus toward vancomycin (Kong et al., 2017). It was hypothesized that farnesol induces oxidative stress in S. aureus, which is sensed by the multiple gene regulator MgrA that in term upregulates drug efflux pumps in a general stress response (Kong et al., 2017). Recently, this effect of farnesol on S. aureus was studied in more detail. Vila et al. found that S. aureus that is repeatedly exposed to farnesol loses its typically yellow pigment staphyloxanthin, which is an important virulence factor. Moreover, farnesol exposure induced an accumulation of intracellular reactive oxygen species (ROS) and alterations in the expression of redox-sensors and major virulence regulators. Interestingly, this activated stress-response led to an enhanced tolerance to H2O2 and phagocytic killing (Vila et al., 2019). Finally, it was discovered that the increased host mortality of co-infections by C. albicans and S. aureus was associated with C. albicans-mediated induction of the agr QS system of S. aureus, with increased alpha and delta-toxin production and hemolytic activity as a result (Todd et al., 2019). It is likely that other, yet unidentified QS molecules may also play a stimulatory role in the interkingdom interaction of Candida and Staphylococcus species. This hypothesis is strengthened by the observation that conditioned medium of S. aureus biofilms dramatically increases C. albicans biofilm growth (Lin et al., 2013).
Besides quorum sensing molecules, other effectors such as secreted metabolites and macromolecules take part in C. albicans-Staphylococcus species interactions. Prostaglandin E2 (PGE2) is, in contrast to farnesol, a secreted metabolite of C. albicans that increases S. aureus biofilm growth in a dose-dependent manner (Krause et al., 2015). Similarly, extracellular DNA (eDNA) from S. epidermidis and to a lesser extent from C. albicans, which is released into the ECM by autolysis or active secretion, enhances biofilm adhesion, aggregation, and development (Pammi et al., 2013). Downregulation of the bacterial repressors of autolysis (the lrg operon) in the mixed C. albicans-S. epidermidis biofilm might be the cause of enhanced eDNA levels in this condition (Pammi et al., 2013). Moreover, lactate, which is a small secreted metabolite present in the human host but also produced by anaerobic fermentation in bacteria including staphylococci, can inhibit yeast-to-hyphae morphogenesis (Liang et al., 2016) and induce β-glucan masking (Ballou et al., 2016) in C. albicans, both strategies of immune evasion. To conclude, Xu et al. (2008) impose a role of bacterial peptidoglycans in the induction of C. albicans hyphae formation (Xu et al., 2008) and thus, potentially, biofilm development. Further research can potentially reveal the role of chemical interspecies communication in mixed species biofilms with the ultimate goal of impeding commensal or symbiotic molecular interactions within this polymicrobial relationship (Figure 1A).
Enhanced Antimicrobial Drug Tolerance
A significant amount of evidence shows that single species biofilms of fungi and bacteria are characterized by increased drug tolerance levels, compared to their planktonic counterparts. This biofilm specific enhanced tolerance is mostly due to various biofilm specific traits, including ECM production, persister cell differentiation and upregulation of drug efflux pumps (Van Acker et al., 2014). Mixed C. albicans-Staphylococcus species biofilms display an additional, polymicrobial specific enhanced drug tolerance toward various antimicrobials, compared to their single species biofilm format (Adam et al., 2002; Harriott and Noverr, 2009, 2010; Kong et al., 2016; Kean et al., 2017). Multiple authors show enhanced tolerance of S. aureus toward the antibiotic vancomycin in the presence of C. albicans (Harriott and Noverr, 2009, 2010; Kong et al., 2016). This effect is mediated by C. albicans ECM (Harriott and Noverr, 2009; Kong et al., 2016) and by the transcriptional regulator of biofilm formation Bcr1, but independent from C. albicans Als1–7, Als9, and Hwp1 adhesins (Harriott and Noverr, 2010). Similarly, C. albicans ECM increases vancomycin tolerance of S. epidermidis in the mixed C. albicans-S. epidermidis biofilm (Adam et al., 2002). Vancomycin remains one of the few effective antibiotics against the clinically relevant methicillin-resistant S. aureus (MRSA), stressing the importance of this polymicrobial commensal effect (Kong et al., 2016). Also C. albicans can benefit from the polymicrobial interaction during treatment. Biofilms of C. albicans with S. epidermidis and S. aureus show enhanced tolerance toward the antifungals fluconazole (Adam et al., 2002) and miconazole (Kean et al., 2017), respectively.
Two mechanisms can possibly explain the protective effect of ECM in polymicrobial interactions: the matrix binds drug components and thus physically limiting penetration of drugs into the biofilm, or the matrix material itself induces drug tolerance associated gene expression (Adam et al., 2002; Harriott and Noverr, 2009). This first hypothesis is supported by Kong et al., showing the significant importance of C. albicans matrix β-1,3-glucan in polymicrobial tolerance toward vancomycin of S. aureus by decreasing penetration of the drug inside the biofilm (Kong et al., 2016). A similar role might exist for eDNA, another important ECM constituent of C. albicans-Staphylocoocus species biofilms (Pammi et al., 2013; Kean et al., 2017). Furthermore, it was shown that degradation of eDNA by DNAse treatment increased the sensitivity of miconazole by two-fold in the mixed C. albicans-S. epidermidis biofilm but not in mono-species biofilms, while ECM chitin and β-1,3-glucan degradation had no such effect (Kean et al., 2017). The polymicrobial biofilm produces significantly more matrix material. It is hypothesized that most of it is made by C. albicans, which than coats S. aureus (Harriott and Noverr, 2009). However, it is also possible that in a mixed culture, S. aureus upregulates its own ECM production. Besides the protection by polymeric ECM constituents, other mechanisms can play a role in the enhanced polymicrobial drug tolerance. An example of this is farnesol of C. albicans, which enhances drug tolerance of S. aureus toward vancomycin (Kong et al., 2017). Further research into the molecular mechanisms involved in the polymicrobial enhanced drug tolerance can possibly lead to improved treatment of polymicrobial C. albicans-Staphylococcus infections (Figure 1B).
Altered Infection Outcome
Enhanced Morbidity and Mortality
Co-infections of C. albicans with Staphylococcus species are among the best examples of “lethal synergism,” meaning that the co-infection mediates the increased mortality of otherwise “survivable” monomicrobial infections. Several studies by Carlson et al. show that peritoneal infections of C. albicans with S. aureus are lethal, while the monomicrobial infections are not (Carlson, 1982, 1983, 1988). Other studies using an intra-abdominal infection mouse model found an increased mortality rate of 80–100% at 48–72 h post-inoculation with C. albicans and S. aureus, while the single species infections were nonlethal (Nash et al., 2014, 2016; Esher et al., 2019; Todd et al., 2019). In addition, this co-infection resulted in both local and systemic inflammation and dissemination and a significant increase in the infection rates and microbial burden. Mice infected with S. aureus had on average 1 × 104 CFU (colony forming units) in the kidney and spleen which increased with a median 1-log-unit or 2-log-unit, respectively, when co-infected with C. albicans. A 4-log-unit CFU increase was observed both in the spleen and kidneys for C. albicans in the mixed infections, compared to the single species infection (Peters and Noverr, 2013). In a murine model of oral candidiasis, a co-infection of C. albicans with S. aureus showed on average a 5-log-unit increase in S. aureus colonization on the tongues of mice which were co-infected with C. albicans, compared to mice infected with S. aureus alone (Kong et al., 2015). In addition, it was shown that oral candidiasis predisposes for secondary systemic bacterial infections since S. aureus was recovered from the kidneys of co-infected mice, but not from single species infections. C. albicans was recovered in 30% of the co-infected mice. Furthermore, these mice showed signs of severe morbidity with a mortality rate up to 20%, while the monomicrobial infections were nonlethal (Kong et al., 2015; Schlecht et al., 2015). The ability to form hyphae was found to be crucial in the murine model of oral candidiasis, in contrast to the intra-abdominal infection model, where C. albicans morphogenesis is not required to induce synergistic lethality (Nash et al., 2014). Since C. albicans forms hyphae which can invade and penetrate human tissues (Sudbery et al., 2004) and staphylococci adhere specifically to these hyphae (Peters et al., 2010), C. albicans hyphae possibly taxi staphylococci during invasion (Peters et al., 2012).
Enhanced mortality is also observed during co-infection of C. albicans with S. epidermidis. Clinical studies in neonates with concurrent bloodstream infections of C. albicans and coagulase-negative staphylococci, such as S. epidermidis, showed an increased mortality and morbidity compared to the single species infections (Kaufman et al., 2014). Additionally, Holt et al. used a polymicrobial biofilm model of S. epidermidis and C. albicans in the nematode Caenorhabditis elegans to show that S. epidermidis exhibits a significantly increased virulence and induced mortality in dual infections. Extracellular polymeric substances (EPSs) produced by S. epidermidis may play a role in this enhanced virulence (Holt et al., 2017). The infectious synergism of these species highlights the importance of both the treatment and surveillance of mixed species infections (Figure 1C).
Effect on the Host Immune Response
Little is known about how multispecies communities differentially affect the host immune response compared to monomicrobial infections (Peters and Noverr, 2013). In vitro, mixed C. albicans-methicillin sensitive S. aureus (MSSA) biofilms contain secreted aspartyl proteases (SAP) and phospholipase C (PL-C) (Zago et al., 2015). Moreover, soluble factors produced in mixed C. albicans-MSSA biofilms are more damaging to epithelial cells compared to the factors produced in the respective single species biofilms (De Carvalho Dias et al., 2017). In vivo, C. albicans and S. aureus exhibit distinct immunomodulatory properties (Seidl et al., 2012), and during co-infection, an altered host immune response is observed, compared to the response in mono-species infections (Lin et al., 2009). For example, in an intra-abdominal catheter biofilm infection in mice, significantly more neutrophils were recruited to the peritoneum 24 h after co-infection with C. albicans and S. aureus compared to the monomicrobial infections. These results indicate a fast response and mobilization of neutrophils during mixed infection (Rogiers et al., 2018). Nash et al. studied the early stages of both local and systemic inflammatory events during mixed C. albicans-S. aureus intra-abdominal infection (Nash et al., 2014). They found that the synergistic lethality induced by co-infection is associated with host factors, including elevated local cytokines (IL-6, TNF-α, and IL-1β) (Nash et al., 2014). In the murine peritonitis model, a unique subset of innate pro-inflammatory cytokines (interleukin-6, granulocyte colony-stimulating factor, keratinocyte chemoattractant, monocyte chemoattractant protein-1, and macrophage inflammatory protein-1α) was identified to significantly increase during C. albicans-S. aureus co-infection, which leads to an increased inflammatory infiltration to the peritoneum and other target organs, compared to the monomicrobial infection (Peters and Noverr, 2013). These five cytokines were increased in both the kidneys and spleen during co-infections and are all involved in the innate proinflammatory response, activated very shortly after pathogen exposure. Additionally, IL-6, G-CSF, and KC are important for recruitment of neutrophils to the site of infection (Peters and Noverr, 2013). Recently, a novel role for the host immune response was suggested in facilitating C. albicans-S. aureus infectious synergy. Using time-lapse microscopy, it was demonstrated that macrophages are attracted to the hyphae of C. albicans and that they selectively engulf S. aureus cells attached to these hyphae, mediating S. aureus dissemination in vitro (Allison et al., 2019). Nevertheless, there is still a lot to discover in how co-infections of C. albicans with Staphylococcus species affect the host immune response or whether the host immune response against one microorganism affects the interaction with the other microorganism. Since insight into host-pathogen interactions are of fundamental concern, this topic should be addressed in future research (Figure 1C; Morales and Hogan, 2010).
Treatment Strategies
Finding an efficient treatment strategy for polymicrobial infections is a major challenge since traditional therapies most often only target individual causative agents within specific kingdoms (Brogden et al., 2005). Combination therapy is being studied as a possible alternative to overcome this challenge. It was demonstrated that anidulafungin and tigecycline act synergistically on S. aureus in mixed C. albicans-S. aureus intra-abdominal catheter biofilm infections in mice (Rogiers et al., 2018). Other potential drug sources are also being investigated for combination therapy against polymicrobial infections. An example of such is the diverse group of “essential oils.” It was shown that in vitro, a combination of fluconazole and mupirocin with clove oil is, respectively, 10 and 4 times more effective against mixed biofilms than fluconazole or mupirocin alone (Budzynska et al., 2017). Recently, other important components of mixed communities of fungi and bacteria, such as quorum sensing and the production of ECM in biofilms, are being explored as possible targets. The effect of quorum quenching enzymes against C. albicans-S. epidermidis biofilms was recently studied (Weiland-Brauer et al., 2019). Two promising quorum quenching enzymes (QQ-5 and QQ-7), identified through functional screening of metagenomic large insert libraries, were found able to inhibit C. albicans morphogenesis, leading to an impaired biofilm formation. Moreover, they both inhibited S. epidermidis biofilm formation, probably by repression of polysaccharide intracellular adhesin (PIA), which plays a key role in biofilm formation of Staphylococcus species. Such quorum quenching molecules are promising drugs to combat mixed species infections (Weiland-Brauer et al., 2019). Small amphiphilic peptides are another class of antimicrobial proteins explored for their activity against (poly)microbial biofilms (Gupta et al., 2019). Recently, valine-glycine dipeptide-derived cholic acid, or CAP3, was found to exhibit broad-spectrum antimicrobial activity against both single and mixed C. albicans-S. aureus biofilms in vitro and in vivo. Its effect was attributed to both biofilm structure disruption and membrane permeabilization (Gupta et al., 2019). Furthermore, the activity of guanylated polymethacrylates, a new class of antimicrobial agents, was assessed against polymicrobial C. albicans-S. aureus biofilms (Qu et al., 2016). Guanylated polymethacrylates are synthetic structural mimics of antimicrobial peptides. They were found to be effective against established polymicrobial biofilms and they outperformed multiple other antimicrobial drug combinations. Finally, the sterilization of catheters against the formation of polymicrobial biofilms is also under investigation. Treatment of catheters with 50% ethanol for 4 h appeared to be sufficient to kill and inhibit the growth of C. albicans-S. aureus mixed biofilms (Peters et al., 2013). Unfortunately, effective measures to deal with polymicrobial infections consisting of C. albicans with Staphylococcus species are still lacking.
Conclusion
Mixed infections of C. albicans with Staphylococcus species are of great importance in clinical settings. The enhanced antimicrobial tolerance, increased virulence, and altered infection outcome due to the interaction of both species makes it even more challenging to find efficient treatment strategies.
Although our knowledge on polymicrobial infections has been greatly expanded recently, there are still questions which remain to be solved in the future. The effect of mixed species infections on the host immune response is still not clear and should be further addressed. In addition, since candidemia is increasingly caused by non-albicans Candida species, it is interesting to study the interaction of Staphylococcus species with other Candida species such as C. glabrata, C. auris, C. parapsilosis, and C. tropicalis. Since our current knowledge on biofilms is still predominantly based on studies concerning monomicrobial or dual species biofilms, it would be interesting to include even more than two microorganisms. An environment where this is particularly important is within the oral cavity since it has a rich and diverse microbial flora. Understanding the complex interspecies communication and the microbial behavior when two or more species live together could help to improve our knowledge on polymicrobial infections and to develop effective treatment strategies.
Author Contributions
HC and KD contributed equally to this publication. All authors contributed to the writing of this manuscript.
Funding
HC was funded by a personal grant from KU Leuven (Internal Funds, KU Leuven; grant number DB/18/009/BM). KD was supported by a personal research grant from the Fund for Scientific Research Flanders (FWO; grant number 1181818N). Original research in the lab is supported by a grant from the National Institute of Allergy and Infectious Diseases (NIAID) under award number R01AI130170 (NIAID) and from the Fund for Scientific Research Flanders (FWO; WO.009.16N).
Conflict of Interest Statement
The authors declare that the research was conducted in the absence of any commercial or financial relationships that could be construed as a potential conflict of interest.
Acknowledgments
We acknowledge Nico Vangoethem for help with the figure.
References
Adam, B., Baillie, G. S., and Douglas, L. J. (2002). Mixed species biofilms of Candida albicans and Staphylococcus epidermidis. J. Med. Microbiol. 51, 344–349. doi: 10.1099/0022-1317-51-4-344
Albuquerque, P., and Casadevall, A. (2012). Quorum sensing in fungi – a review. Med. Mycol. 50, 337–345. doi: 10.3109/13693786.2011.652201
Allison, D. L., Scheres, N., Willems, H. M. E., Bode, C. S., Krom, B. P., and Shirtliff, M. E. (2019). The host immune system facilitates disseminated Staphylococcus aureus disease due to phagocytic. Attraction to Candida albicans during co-infection: a case of bait and switch. Infect. Immun. 87. doi: 10.1128/IAI.00137-19
Baena-Monroy, T., Moreno-Maldonado, V., Franco-Martinez, F., Aldape-Barrios, B., Quindos, G., and Sanchez-Vargas, L. O. (2005). Candida albicans, Staphylococcus aureus and Streptococcus mutans colonization in patients wearing dental prosthesis. Med. Oral Patol. Oral Cir. Bucal. 10(Suppl. 1), E27–E39.
Ballou, E. R., Avelar, G. M., Childers, D. S., Mackie, J., Bain, J. M., Wagener, J., et al. (2016). Lactate signalling regulates fungal beta-glucan masking and immune evasion. Nat. Microbiol. 2:16238. doi: 10.1038/nmicrobiol.2016.238
Beaussart, A., Herman, P., El-Kirat-Chatel, S., Lipke, P. N., Kucharikova, S., Van Dijck, P., et al. (2013). Single-cell force spectroscopy of the medically important Staphylococcus epidermidis-Candida albicans interaction. Nanoscale 5, 10894–10900. doi: 10.1039/c3nr03272h
Brogden, K. A., Guthmiller, J. M., and Taylor, C. E. (2005). Human polymicrobial infections. Lancet 365, 253–255. doi: 10.1016/S0140-6736(05)70155-0
Budzynska, A., Rozalska, S., Sadowska, B., and Rozalska, B. (2017). Candida albicans/Staphylococcus aureus dual-species biofilm as a target for the combination of essential oils and fluconazole or mupirocin. Mycopathologia 182, 989–995. doi: 10.1007/s11046-017-0192-y
Carlson, E. (1982). Synergistic effect of Candida albicans and Staphylococcus aureus on mouse mortality. Infect. Immun. 38, 921–924.
Carlson, E. (1983). Effect of strain of Staphylococcus aureus on synergism with Candida albicans resulting in mouse mortality and morbidity. Infect. Immun. 42, 285–292.
Carlson, E. (1988). Synergism of Candida albicans and delta toxin producing Staphylococcus aureus on mouse mortality and morbidity: protection by indomethacin. Zentralbl. Bakteriol. Mikrobiol. Hyg. A. 269, 377–386. doi: 10.1016/S0176-6724(88)80181-0
Cuesta, A. I., Jewtuchowicz, V., Brusca, M. I., Nastri, M. L., and Rosa, A. C. (2010). Prevalence of Staphylococcus spp and Candida spp in the oral cavity and periodontal pockets of periodontal disease patients. Acta Odontol. Latinoam. 23, 20–26.
De Carvalho Dias, K., Barbugli, P. A., De Patto, F., Lordello, V. B., De Aquino Penteado, L., Medeiros, A. I., et al. (2017). Soluble factors from biofilm of Candida albicans and Staphylococcus aureus promote cell death and inflammatory response. BMC Microbiol. 17:146. doi: 10.1186/s12866-017-1031-5
El-Azizi, M. A., Starks, S. E., and Khardori, N. (2004). Interactions of Candida albicans with other Candida spp. and bacteria in the biofilms. J. Appl. Microbiol. 96, 1067–1073. doi: 10.1111/j.1365-2672.2004.02213.x
Esher, S. K., Fidel, P. L. Jr., and Noverr, M. C. (2019). Candida/staphylococcal polymicrobial intra-abdominal infection: pathogenesis and perspectives for a novel form of trained innate immunity. J. Fungi 5. doi: 10.3390/jof5020037
Gupta, N., Haque, A., Mukhopadhyay, G., Narayan, R. P., and Prasad, R. (2005). Interactions between bacteria and Candida in the burn wound. Burns 31, 375–378. doi: 10.1016/j.burns.2004.11.012
Gupta, S., Thakur, J., Pal, S., Gupta, R., Mishra, D., Kumar, S., et al. (2019). Cholic acid-peptide conjugates (CAPs) as potent antimicrobials against interkingdom polymicrobial biofilms. Antimicrob. Agents Chemother. 63. doi: 10.1128/AAC.00520-19
Harriott, M. M., and Noverr, M. C. (2009). Candida albicans and Staphylococcus aureus form polymicrobial biofilms: effects on antimicrobial resistance. Antimicrob. Agents Chemother. 53, 3914–3922. doi: 10.1128/AAC.00657-09
Harriott, M. M., and Noverr, M. C. (2010). Ability of Candida albicans mutants to induce Staphylococcus aureus vancomycin resistance during polymicrobial biofilm formation. Antimicrob. Agents Chemother. 54, 3746–3755. doi: 10.1128/AAC.00573-10
Holt, J. E., Houston, A., Adams, C., Edwards, S., and Kjellerup, B. V. (2017). Role of extracellular polymeric substances in polymicrobial biofilm infections of Staphylococcus epidermidis and Candida albicans modelled in the nematode Caenorhabditis elegans. Pathog. Dis. 75. doi: 10.1093/femspd/ftx052
Hornby, J. M., Jensen, E. C., Lisec, A. D., Tasto, J. J., Jahnke, B., Shoemaker, R., et al. (2001). Quorum sensing in the dimorphic fungus Candida albicans is mediated by farnesol. Appl. Environ. Microbiol. 67, 2982–2992. doi: 10.1128/AEM.67.7.2982-2992.2001
Jabra-Rizk, M. A., Meiller, T. F., James, C. E., and Shirtliff, M. E. (2006). Effect of farnesol on Staphylococcus aureus biofilm formation and antimicrobial susceptibility. Antimicrob. Agents Chemother. 50, 1463–1469. doi: 10.1128/AAC.50.4.1463-1469.2006
Kaufman, D. A., Brown, A. T., Eisenhuth, K. K., Yue, J., Grossman, L. B., and Hazen, K. C. (2014). More serious infectious morbidity and mortality associated with simultaneous candidemia and coagulase-negative staphylococcal bacteremia in neonates and in vitro adherence studies between Candida albicans and Staphylococcus epidermidis. Early Hum. Dev. 90(Suppl. 1), S66–S70. doi: 10.1016/S0378-3782(14)70021-0
Kean, R., Rajendran, R., Haggarty, J., Townsend, E. M., Short, B., Burgess, K. E., et al. (2017). Candida albicans mycofilms support Staphylococcus aureus colonization and enhances miconazole resistance in dual-species interactions. Front. Microbiol. 8:258. doi: 10.3389/fmicb.2017.00258
Klotz, S. A., Chasin, B. S., Powell, B., Gaur, N. K., and Lipke, P. N. (2007a). Polymicrobial bloodstream infections involving Candida species: analysis of patients and review of the literature. Diagn. Microbiol. Infect. Dis. 59, 401–406. doi: 10.1016/j.diagmicrobio.2007.07.001
Klotz, S. A., Gaur, N. K., De Armond, R., Sheppard, D., Khardori, N., Edwards, J. E. Jr., et al. (2007b). Candida albicans Als proteins mediate aggregation with bacteria and yeasts. Med. Mycol. 45, 363–370. doi: 10.1080/13693780701299333
Kong, E. F., Kucharikova, S., Van Dijck, P., Peters, B. M., Shirtliff, M. E., and Jabra-Rizk, M. A. (2015). Clinical implications of oral candidiasis: host tissue damage and disseminated bacterial disease. Infect. Immun. 83, 604–613. doi: 10.1128/IAI.02843-14
Kong, E. F., Tsui, C., Kucharikova, S., Andes, D., Van Dijck, P., and Jabra-Rizk, M. A. (2016). Commensal protection of Staphylococcus aureus against antimicrobials by Candida albicans biofilm matrix. MBio 7, 1–12. doi: 10.1128/mBio.01365-16
Kong, E. F., Tsui, C., Kucharikova, S., Van Dijck, P., and Jabra-Rizk, M. A. (2017). Modulation of Staphylococcus aureus response to antimicrobials by the Candida albicans quorum sensing molecule farnesol. Antimicrob. Agents Chemother. 61. doi: 10.1128/AAC.01573-17
Kong, K. F., Vuong, C., and Otto, M. (2006). Staphylococcus quorum sensing in biofilm formation and infection. Int. J. Med. Microbiol. 296, 133–139. doi: 10.1016/j.ijmm.2006.01.042
Koo, H., Allan, R. N., Howlin, R. P., Stoodley, P., and Hall-Stoodley, L. (2017). Targeting microbial biofilms: current and prospective therapeutic strategies. Nat. Rev. Microbiol. 15, 740–755. doi: 10.1038/nrmicro.2017.99
Krause, J., Geginat, G., and Tammer, I. (2015). Prostaglandin E2 from Candida albicans stimulates the growth of Staphylococcus aureus in mixed biofilms. PLoS One 10:e0135404. doi: 10.1371/journal.pone.0135404
Liang, W., Guan, G., Dai, Y., Cao, C., Tao, L., Du, H., et al. (2016). Lactic acid bacteria differentially regulate filamentation in two heritable cell types of the human fungal pathogen Candida albicans. Mol. Microbiol. 102, 506–519. doi: 10.1111/mmi.13475
Lin, Y. J., Alsad, L., Vogel, F., Koppar, S., Nevarez, L., Auguste, F., et al. (2013). Interactions between Candida albicans and Staphylococcus aureus within mixed species biofilms. Bios 84, 30–39. doi: 10.1893/0005-3155-84.1.30,
Lin, L., Ibrahim, A. S., Xu, X., Farber, J. M., Avanesian, V., Baquir, B., et al. (2009). Th1-Th17 cells mediate protective adaptive immunity against Staphylococcus aureus and Candida albicans infection in mice. PLoS Pathog. 5:e1000703. doi: 10.1371/journal.ppat.1000703
Morales, D. K., and Hogan, D. A. (2010). Candida albicans interactions with bacteria in the context of human health and disease. PLoS Pathog. 6:e1000886. doi: 10.1371/journal.ppat.1000886
Nash, E. E., Peters, B. M., Fidel, P. L., and Noverr, M. C. (2016). Morphology-independent virulence of Candida species during polymicrobial intra-abdominal infections with Staphylococcus aureus. Infect. Immun. 84, 90–98. doi: 10.1128/IAI.01059-15
Nash, E. E., Peters, B. M., Palmer, G. E., Fidel, P. L., and Noverr, M. C. (2014). Morphogenesis is not required for Candida albicans-Staphylococcus aureus intra-abdominal infection-mediated dissemination and lethal sepsis. Infect. Immun. 82, 3426–3435. doi: 10.1128/IAI.01746-14
Nobile, C. J., and Johnson, A. D. (2015). Candida albicans biofilms and human disease. Annu. Rev. Microbiol. 69, 71–92. doi: 10.1146/annurev-micro-091014-104330
Ovchinnikova, E. S., Krom, B. P., Busscher, H. J., and Van Der Mei, H. C. (2012). Evaluation of adhesion forces of Staphylococcus aureus along the length of Candida albicans hyphae. BMC Microbiol. 12:281. doi: 10.1186/1471-2180-12-281
Pammi, M., Liang, R., Hicks, J., Mistretta, T. A., and Versalovic, J. (2013). Biofilm extracellular DNA enhances mixed species biofilms of Staphylococcus epidermidis and Candida albicans. BMC Microbiol. 13:257. doi: 10.1186/1471-2180-13-257
Peters, B. M., Jabra-Rizk, M. A., Scheper, M. A., Leid, J. G., Costerton, J. W., and Shirtliff, M. E. (2010). Microbial interactions and differential protein expression in Staphylococcus aureus-Candida albicans dual-species biofilms. FEMS Immunol. Med. Microbiol. 59, 493–503. doi: 10.1111/j.1574-695X.2010.00710.x
Peters, B. M., and Noverr, M. C. (2013). Candida albicans-Staphylococcus aureus polymicrobial peritonitis modulates host innate immunity. Infect. Immun. 81, 2178–2189. doi: 10.1128/IAI.00265-13
Peters, B. M., Ovchinnikova, E. S., Krom, B. P., Schlecht, L. M., Zhou, H., Hoyer, L. L., et al. (2012). Staphylococcus aureus adherence to Candida albicans hyphae is mediated by the hyphal adhesin Als3p. Microbiology 158, 2975–2986. doi: 10.1099/mic.0.062109-0
Peters, B. M., Ward, R. M., Rane, H. S., Lee, S. A., and Noverr, M. C. (2013). Efficacy of ethanol against Candida albicans and Staphylococcus aureus polymicrobial biofilms. Antimicrob. Agents Chemother. 57, 74–82. doi: 10.1128/AAC.01599-12,
Piispanen, A. E., Bonnefoi, O., Carden, S., Deveau, A., Bassilana, M., and Hogan, D. A. (2011). Roles of Ras1 membrane localization during Candida albicans hyphal growth and farnesol response. Eukaryot. Cell 10, 1473–1484. doi: 10.1128/EC.05153-11
Qu, Y., Locock, K., Verma-Gaur, J., Hay, I. D., Meagher, L., and Traven, A. (2016). Searching for new strategies against polymicrobial biofilm infections: guanylated polymethacrylates kill mixed fungal/bacterial biofilms. J. Antimicrob. Chemother. 71, 413–421. doi: 10.1093/jac/dkv334
Ramage, G., Saville, S. P., Wickes, B. L., and Lopez-Ribot, J. L. (2002). Inhibition of Candida albicans biofilm formation by farnesol, a quorum-sensing molecule. Appl. Environ. Microbiol. 68, 5459–5463. doi: 10.1128/AEM.68.11.5459-5463.2002
Rogiers, O., Holtappels, M., Siala, W., Lamkanfi, M., Van Bambeke, F., Lagrou, K., et al. (2018). Anidulafungin increases the antibacterial activity of tigecycline in polymicrobial Candida albicans/Staphylococcus aureus biofilms on intraperitoneally implanted foreign bodies. J. Antimicrob. Chemother. 73, 2806–2814. doi: 10.1093/jac/dky246
Schlecht, L. M., Peters, B. M., Krom, B. P., Freiberg, J. A., Hansch, G. M., Filler, S. G., et al. (2015). Systemic Staphylococcus aureus infection mediated by Candida albicans hyphal invasion of mucosal tissue. Microbiology 161, 168–181. doi: 10.1099/mic.0.083485-0
Seidl, K., Solis, N. V., Bayer, A. S., Hady, W. A., Ellison, S., Klashman, M. C., et al. (2012). Divergent responses of different endothelial cell types to infection with Candida albicans and Staphylococcus aureus. PLoS One 7:e39633. doi: 10.1371/journal.pone.0039633
Staib, F., Grosse, G., and Mishra, S. K. (1976). Staphylococcus aureus and Candida albicans infection (animal experiments). Zentralbl. Bakteriol. Orig. A. 234, 450–461. PMID:
Sudbery, P., Gow, N., and Berman, J. (2004). The distinct morphogenic states of Candida albicans. Trends Microbiol. 12, 317–324. doi: 10.1016/j.tim.2004.05.008,
Todd, O. A., Fidel, P. L. Jr., Harro, J. M., Hilliard, J. J., Tkaczyk, C., Sellman, B. R., et al. (2019). Candida albicans augments Staphylococcus aureus virulence by engaging the staphylococcal agr quorum sensing system. MBio 10. doi: 10.1128/mBio.00910-19
Tsui, C., Kong, E. F., and Jabra-Rizk, M. A. (2016). Pathogenesis of Candida albicans biofilm. Pathog. Dis. 74:ftw018. doi: 10.1093/femspd/ftw018
Valenza, G., Tappe, D., Turnwald, D., Frosch, M., Konig, C., Hebestreit, H., et al. (2008). Prevalence and antimicrobial susceptibility of microorganisms isolated from sputa of patients with cystic fibrosis. J. Cyst. Fibros. 7, 123–127. doi: 10.1016/j.jcf.2007.06.006
Van Acker, H., Van Dijck, P., and Coenye, T. (2014). Molecular mechanisms of antimicrobial tolerance and resistance in bacterial and fungal biofilms. Trends Microbiol. 22, 326–333. doi: 10.1016/j.tim.2014.02.001
Vila, T., Kong, E. F., Ibrahim, A., Piepenbrink, K., Shetty, A. C., Mccracken, C., et al. (2019). Candida albicans quorum-sensing molecule farnesol modulates staphyloxanthin production and activates the thiol-based oxidative-stress response in Staphylococcus aureus. Virulence 10, 625–642. doi: 10.1080/21505594.2019.1635418
Weiland-Brauer, N., Malek, I., and Schmitz, R. A. (2019). Metagenomic quorum quenching enzymes affect biofilm formation of Candida albicans and Staphylococcus epidermidis. PLoS One 14:e0211366. doi: 10.1371/journal.pone.0211366
Xu, X. L., Lee, R. T., Fang, H. M., Wang, Y. M., Li, R., Zou, H., et al. (2008). Bacterial peptidoglycan triggers Candida albicans hyphal growth by directly activating the adenylyl cyclase Cyr1p. Cell Host Microbe 4, 28–39. doi: 10.1016/j.chom.2008.05.014
Zago, C. E., Silva, S., Sanita, P. V., Barbugli, P. A., Dias, C. M., Lordello, V. B., et al. (2015). Dynamics of biofilm formation and the interaction between Candida albicans and methicillin-susceptible (MSSA) and -resistant Staphylococcus aureus (MRSA). PLoS One 10:e0123206. doi: 10.1371/journal.pone.0123206
Keywords: Candida albicans, Staphylococcus aureus, Staphylococcus epidermidis, polymicrobial infections, biofilms, interspecies interactions, interkingdom interactions
Citation: Carolus H, Van Dyck K and Van Dijck P (2019) Candida albicans and Staphylococcus Species: A Threatening Twosome. Front. Microbiol. 10:2162. doi: 10.3389/fmicb.2019.02162
Edited by:
Leonardo Nimrichter, Federal University of Rio de Janeiro, BrazilReviewed by:
Clarissa J. Nobile, University of California, Merced, United StatesAllan J. Guimaraes, Universidade Federal Fluminense, Brazil
Copyright © 2019 Carolus, Van Dyck and Van Dijck. This is an open-access article distributed under the terms of the Creative Commons Attribution License (CC BY). The use, distribution or reproduction in other forums is permitted, provided the original author(s) and the copyright owner(s) are credited and that the original publication in this journal is cited, in accordance with accepted academic practice. No use, distribution or reproduction is permitted which does not comply with these terms.
*Correspondence: Patrick Van Dijck, cGF0cmljay52YW5kaWpja0BrdWxldXZlbi52aWIuYmU=
†These authors have contributed equally to this work