- 1Department of Physiological Sciences, Faculty of Veterinary Medicine, Warsaw University of Life Sciences, Warsaw, Poland
- 2Department of Biophysics, Warsaw University of Life Sciences, Warsaw, Poland
Process of inflammation and complex interactions between immune and cancer cells within tumor microenvironment are known to drive and shape the outcome of the neoplastic disease. Recent studies increasingly show that ion channels can be used as potential targets to modulate immune response and to treat inflammatory disorders and cancer. The action of both innate and adaptive immune cells is tightly regulated by ionic signals provided by a network of distinct ion channels. TRPV1 channel, known as a capsaicin receptor, was recently documented to be expressed on the cells of the immune system but also aberrantly expressed in the several tumor types. It is activated by heat, protons, proinflammatory cytokines, and associated with pain and inflammation. TRPV1 channel is not only involved in calcium signaling fundamental for many cellular processes but also takes part in cell-environment crosstalk influencing cell behavior. Furthermore, in several studies, activation of TRPV1 by capsaicin was associated with anti-cancer effects. Therefore, TRPV1 provides a potential link between the process of inflammation, cancer and immunity, and offers new treatment possibilities. Nevertheless, in many cases, results regarding TRPV1 are contradictory and need further refinement. In this review we present the summary of the data related to the role of TRPV1 channel in the process of inflammation, cancer and immunity, limitations of the studies, and directions for future research.
Introduction
Cancer is the second cause of death in the world according to the World Health Organization. Recently, more attention is paid to the correlation between the process of inflammation and crosstalk between immune and tumor cells. Presence of specific immune cells that produce anti- or proinflammatory cytokines shapes the tumor microenvironment (TME) and have a great impact on tumor development outcome (1, 2). Therefore, current therapeutic approaches more often focus on targeting TME and harnessing the immune system to eradicate tumors. Consequently, the knowledge about specific molecules or their ligands that can hamper tumor growth, induce apoptosis, modulate immune response or influence tumor microenvironment, are of special interest for the medical field.
Ion channels are widely known to be involved in many biological processes in both excitatory and non-excitatory cells. They exhibit broad tissue distribution and their expression is not limited solely to the plasma membrane. Some of the ion channels are expressed in the inner compartments of the cells such as mitochondria where they play a fundamental role in the regulation of cell death and cytoprotection (3). Furthermore, increasing evidence indicates that ion channels have implications in cancer cell proliferation, survival and migration (4, 5). For this reason, ion channels emerged recently as potential pharmacological targets for the treatment of several disorders including neoplastic disease.
Transient Receptor Potential (TRP) ion channels are a family of non-selective cation channels that in vertebrates are represented by 28 different members divided into six subfamilies namely: Canonical (TRPC), Vanilloids (TRPV), Melastatins (TRPM), Mucolipins (TRPML), Polycystins (TRPP), and Ankyrin (TRPA1). They are receptors of multiple different stimuli such as temperature, pH, ROS, osmotic stress, and bacterial toxins. Furthermore, their activity is regulated by phytochemical compounds such as capsaicin, piperine, geraniol, or menthol (6). TRP ion channels were primarily associated with sensory nerve cells however recent findings showed that their expression is also present in other cell types including immune cells such as dendritic cells, macrophages, or T lymphocytes (7).
TRPV1 was the first identified member of the vanilloid receptor subfamily of TRP ion channels and to date is the most extensively studied. It was characterized in 1997 by Julius group as a receptor of capsaicin, a pungent compound from chili peppers (8). Further studies revealed that TRPV1 is also activated by temperature (with threshold ~43°C), protons and bacterial toxins such as lipopolysaccharide (LPS) (9, 10). For this reason, TRPV1 was associated with thermoception and nociception. Additionally, it is important to underline that several factors can influence activation threshold of TRPV1. For instance, in the presence of the divalent ions such as Mg2+ from the extracellular side, TRPV1 was shown to be activated at lower temperatures (11).
Expression in a wide range of tissues, such as skin, airways, gastrointestinal tract, urinary epithelial cells, pancreatic B cells and in the immune cells, underlines the important role of TRPV1. Currently it is known, that TRPV1 channel is not only involved in the thermal and pain sensation but also in other processes such as T cells activation, urinary bladder functions, insulin sensitivity, or airway hypersensitivity (12–19). TRPV1 was shown to be implicated in neurogenic inflammation, neuropathic pain, autoimmune disorders, cancer and immune cells functioning. To date, TRPV1 agonist, capsaicin or resiniferatoxin, and antagonists such as capsazepine (CPZ), BCTC, SB-705498, or NEO6860 were tested to treat migraine, osteoarthritis, overactive bladder, atopic dermatitis, and neuropathic pain (clinicaltrials.gov). Furthermore, in some studies capsaicin-induced TRPV1 activation was also associated with anti-inflammatory and anticancer effects. Thus, identification of novel modulators of TRPV1 activity might be beneficial to medical field.
TRPV1 channel is involved in the regulation of calcium signaling, crucial for many cellular processes including proliferation, apoptosis, secretion of cytokines or T cell activation. Furthermore, TRPV1 appears as a polymodal receptor that takes part in cell-environment crosstalk. Consequently, it can influence not only cell behavior but also cell fate and potentially can shape the antitumor response.
Therefore, TRPV1 might offer new therapeutic option in clinical applications against neoplastic diseases and pain treatment. Nevertheless, its involvement in the immune and cancer cells functioning is still under investigation. Herein, we present a review regarding the role of the TRPV1 channel in the process of inflammation, cancer, and immunity.
Role of the TRPV1 Channel in the Process of Inflammation
Inflammation is a process characterized by pain, swelling, increased temperature, and redness that can be induced by pathogen infection or tissue damage. The main role of the inflammation is to stimulate the cells to fight against pathogens and regenerate destroyed tissue. It is tightly correlated with the action of immune cells and secretion of pro-inflammatory factors (cytokines, chemokines) (20). Currently, the process of inflammation is gaining more attention as it became linked to many pathological conditions including metabolic, neurogenerative, autoimmunological disorders but also tumorigenesis and progression of cancer (21–24).
TRPV1, as a pain and heat sensor expressed at high level in C-fibers associated with neurogenic pain, was primarily associated with neurogenic inflammation (25). However, expression of TRPV1 on both mRNA and protein level was also found on non-neural cell types and for this reason, recent studies address the role of vanilloid receptor in other inflammatory disorders such as asthma, rheumatoid arthritis or inflammatory bowel diseases (26–28).
The role of TRPV1 in the process of inflammation is vague. Primarily TRPV1 activation was associated with the induction of inflammatory response but recent studies demonstrated also its anti-inflammatory properties. TRPV1 was associated with the process of inflammation based on the studies that showed: (1) overexpression of V1 in inflamed tissues, (2) alleviation of inflammation by use of TRPV1 antagonist or genetic ablation or (3) correlation between TRPV1 activation and expression of proinflammatory cytokines.
Association of TRPV1 Activation With Proinflammatory Responses
Increased expression of TRPV1 channel on both mRNA and protein level has been reported in several chronic inflammatory diseases for instance in the lung tissue of murine model of chronic asthma (29, 30), in synovial fibroblasts from patients with rheumatoid arthritis and osteoarthritis (31) or in esophageal mucosa in the course of non-erosive reflux disease (NERD) and erosive esophagitis (32, 33). Interestingly, in many studies inflammatory response was alleviated by administration of TRPV1 antagonists or TRPV1 siRNA which further underscore its role in the process. Nevertheless, further studies should also address the cell type in which TRPV1 is expressed in order to decode the true role of TRPV1 in the process of inflammation. Studies by Engler et al. (31) showed the increased expression of TRPV1 by qPCR and Western Blot analysis in the synovial fibroblasts isolated from patients with osteoarthritis and rheumatoid arthritis. Yet, in many studies the analysis was performed on entire organs (e.g., lung tissue) which contains a mixed cell population. Thus, the overall effect should be considered as a result of neuronal, immune and other cell types interactions.
In the studies by Ma et al. (34) exposure of human esophageal epithelial cells (HET-1A) to acidic pH (5.0) increased TRPV1 expression and led to ATP release and upregulation of inflammatory cytokines such as IL-8, Monocyte Chemoattractant Protein 1 (MCP-1), and Macrophages Inflammatory Protein-1α (MIP-1α). Silva et al. (33) showed that the development of esophageal inflammation could be blocked by TRPV1 antagonist SB366791 in the mouse model of NERD. These results suggest that TRPV1 is a potential pharmacological target in patients with NERD.
Also in mouse model of chronic asthma, administration of TRPV1 antagonist capsazepine (CPZ) or TRPV1 siRNA attenuated airway inflammation, hypersensitiveness, as well as reduced levels of cytokines IL-4, IL-5, IL-13 (type 2 T helper cytokines), and Thymic Stromal Lymphopoietin (TSLP), IL-25, IL-33 (epithelial cell-derived cytokines) (30). In urban particle matter and formaldehyde (FA) induced murine asthma model upregulated expression of TRPV1 was associated with enhanced release of pro-inflammatory neuropeptides such as substance P and Calcitonin Gene–Related Peptide (CGRP), which contributed to neurogenic inflammation. CPZ treatment, in turn, effectively reduced the level of pro-inflammatory neuropeptides (29).
Despite that in many studies CPZ was used as a TRPV1 antagonist, the results has to be treated with caution. CPZ recently was found to activate TRPA1 channel, which along with TRPV1 is involved in nociception and inflammation. Kistner et al. (35) found that CPZ administration led to attenuation of inflammation in the model of murine colitis. Nevertheless, authors demonstrated that the effect was TRPV1-independent and rather associated with desensitization of TRPA1. Furthermore, CPZ was also documented to block voltage-activated calcium channels (36). Thus, the use of CPZ to assess the role of TRPV1 in the process of inflammation might be speculative. To note, FA is known TRPA1 activator, thus the assessment of the TRPV1 role in the model of FA-induced inflammation should be done with caution. McNamara et al. (37) clearly demonstrated that formalin exerts its effect through TRPA1 not TRPV1 since the effect was abolished in TRPA1−/− KO mice.
In the studies by Hoffmeister et al. (38) TRPV1 was linked with nociception and inflammation in the acute gout attacks. Injection of monosodium urate into rat joints induced hyperalgesia, allodynia, leukocyte infiltration and IL-1β production, which were inhibited by the co-administration of TRPV1 antagonist SB366791. Injection of TRPV1 antagonist also reduced nociception and associated pain-like behavior. Interestingly, monosodium urate crystals increased expression of TRPV1 in the articular tissue, which was correlated the peak of inflammatory response and nociception (38). Furthermore, deletion of TRPV1 gene was shown to attenuate synovial inflammation, bone erosion, cartilage damage in the course of rheumatoid arthritis (26).
In hairless mice with induced atopic dermatitis TRPV1 antagonist PAC-14028 administered as a cream improved skin barrier functions and restored expression of epidermal differentiation markers. Additionally, it significantly inhibited inflammation by decreasing the expression of IL-4 and IL-13 as well as expression of serum IgE (39).
A systemic inflammatory response in many cases is associated with a pathological condition known as sepsis. To date, the best models to study sepsis is cecal ligation and puncture (CLP) and LPS intravenous administration model. CLP is based on perforation of the cecum followed by microbial infection and subsequent severe immune response. TRPV1 was shown to be involved in the course of sepsis which was firstly proven by Bryant et al. (40) who suggested an important role of nociceptive system in response to infection. In the studies by Ninomiya et al. (41) pre-treatment of LPS-activated macrophages with TRPV1 antagonists AMG 9810 and CPZ reduced production of pro-inflammatory cytokines IL-6, IL-1β, and IL-18 and COX-2 expression. In mice, with LPS-induced lung injury, CPZ pre-treatment prevented the increase in respiratory system resistance, tissue damping, and decreased the area of collapsed lung parenchyma (42).
LPS was shown to activate TRPV1 but with lower potency than TRPA1. Furthermore, also other TRP ion channels (for instance TRPV4) were shown to be affected by LPS treatment (10). Thus, it has to be taken into consideration that the effects of LPS and TRPV1 pharmacological blockers might be the result of the interaction of several overlapping factors. The data on potential proinflammatory effect of TRPV1 activation or blockade is summarized in Table 1.
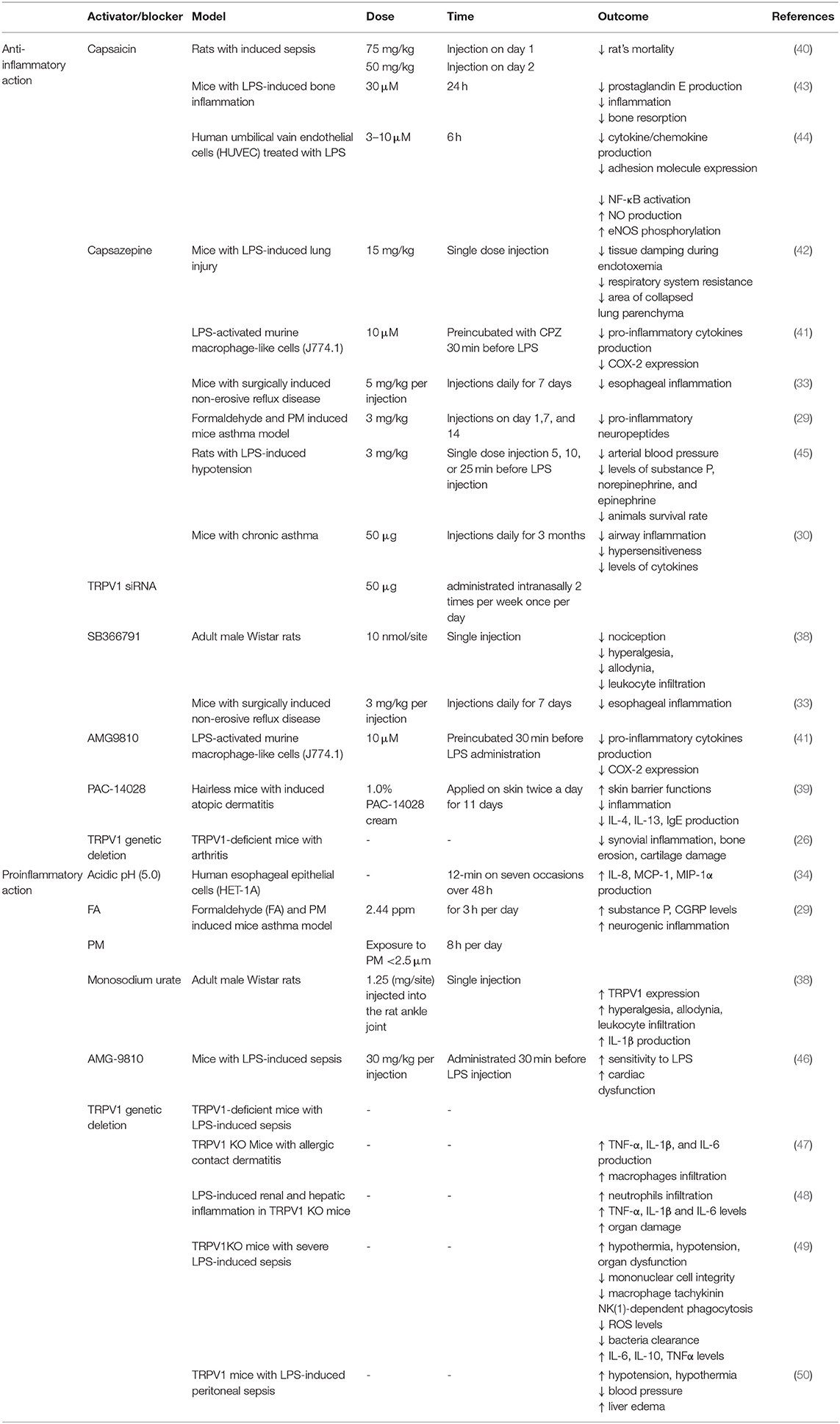
Table 1. Effects of TRPV1 activation (capsaicin) and blocking/genetic deletion on the process of inflammation.
Anti-inflammatory Role of TRPV1 Channel
Surprisingly, recent studies on the role of TRPV1 in the process of inflammation showed that pharmacological or genetic ablation of TRPV1 channel actually might aggravate the symptoms of inflammation.
Feng et al. (47) showed that lack of TRPV1 channel leads to cutaneous inflammation in the mouse model of allergic contact dermatitis. Authors demonstrated that TRPV1 deficiency was associated with upregulation of proinflammatory cytokines expression such as TNF-α, IL-1β, and IL-6 increased macrophages infiltration and simultaneous inflammation (47).
In rats injected with LPS, TRPV1 blockage with CPZ lowered arterial blood pressure, and levels of substance P, norepinephrine, and epinephrine, as a result lowering survival rate at 24 and 48 h after LPS administration (45). Nevertheless, in the light of more recent studies, such effect could be a result of CPZ influence on TRPA1 not TRPV1 (35).
Also, in the model of LPS-induced renal and hepatic inflammation, TRPV1 KO mice exhibited significantly higher neutrophils infiltration, higher serum TNF-α, IL-1β, and IL-6 cytokines levels, more severe inflammatory response and subsequent exaggerated organ damage during endotoxic shock (48). This indicates that TRPV1 might actually have protective and anti-inflammatory role (Figure 1).
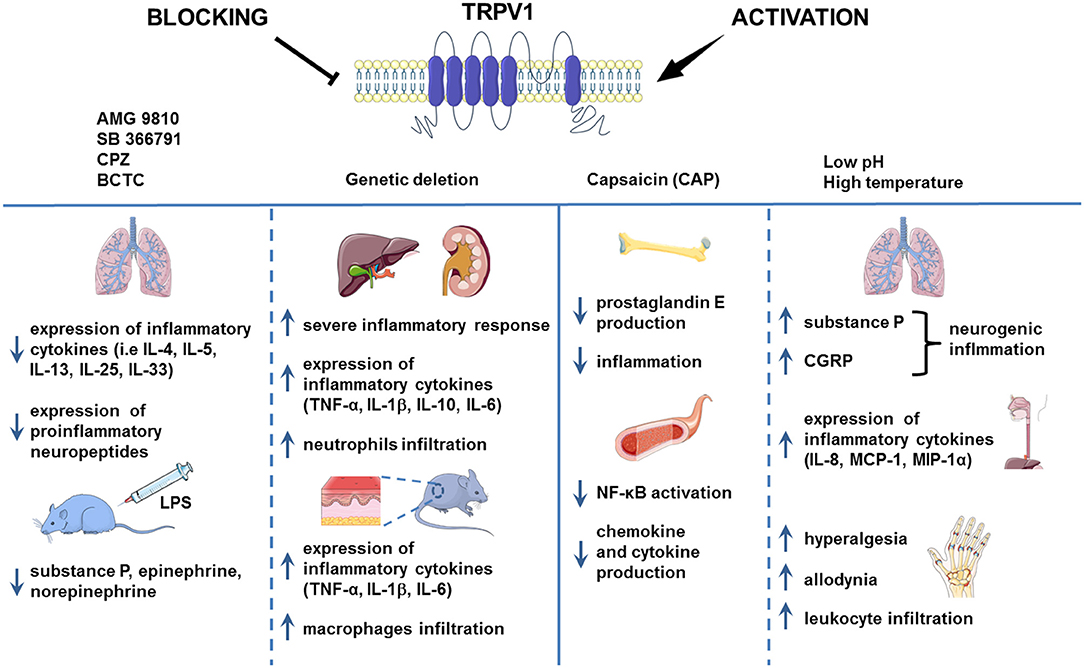
Figure 1. TRPV1 in inflammation. TRPV1 is widely known to be implicated in inflammation. However, the results of the studies regarding the role of TRPV1 channel in the process of inflammation are contradictory. In the chronic asthma model and mouse injected with LPS, the pharmacological blockade of TRPV1 decreased the level of proinflammatory cytokines. Genetic deletion of TRPV1, however, resulted in severe inflammation in the mouse model of LPS-induced renal and hepatic inflammation and allergic contact dermatitis. Activation of TRPV1 channel by H+ or formaldehyde was associated with aggravation of inflammation in the mouse model of asthma and human esophageal epithelial cells (HET-1A). Nevertheless, capsaicin administration led to the alleviation of inflammation symptoms as it was shown on the HUVEC cell culture and mouse LPS-induced bone inflammation model. Images adapted from Smart Servier Medical Art.
In the CLP sepsis model, TRPV1 knockout mice (TRPV1 KO) exhibited more severe LPS-induced sepsis compared with wild type. CLP TRPV1 KO mice showed significant hypothermia, hypotension, and organ dysfunction caused by decreased mononuclear cell integrity associated with apoptosis, macrophage tachykinin NK(1)-dependent phagocytosis, level of reactive oxygen species and bacteria clearance but increased IL-6, IL-10, and TNFα levels (49). Also, Clark et al. (50) showed that genetic deletion of TRPV1 in the murine model of LPS-induced peritoneal sepsis led to enhanced hypotension, hypothermia, decreased blood pressure and liver edema, thus, indicating that TRPV1 is involved in response regulation to sepsis and has an overall protective role.
Also, administration of TRPV1 agonists was shown to reduce inflammation. Bryant et al. (40) showed that injection of capsaicin reduced rat's mortality in the model of abdominal sepsis. Mice with LPS-induced bone inflammation treated with capsaicin exhibited suppressed prostaglandin E production, inflammation and subsequent bone resorption (43). Presence of capsaicin in HUVEC cells culture before LPS stimulation lowered cytokine and chemokine production, adhesion molecule expression, and NF-κB activation but increased nitric oxide (NO) production and endothelial nitric oxide synthase (eNOS) phosphorylation. Authors suggested that TRPV1 activation in endothelial cells is associated with activation of eNOS/NO pathway and consequent suppression of inflammation (44). Moreover, endogenous activation of cardiac TRPV1 during sepsis and endotoxemia led to the release of CGRP minimizing cardiac dysfunction (46). Summarizing, anti-inflammatory action of TRPV1 activation or blockade is presented in Table 1.
TRPV1 is widely known to have pro-inflammatory role, but growing evidence points to its anti-inflammatory and protective role as well. It is important to underline that involvement of TRPV1 in the process of inflammation is regulated at several levels starting from gene expression to post-transcriptional modifications and cellular compartmentalization. The process is also associated with many regulatory proteins and multiple inflammatory mediators, which might obscure the true role of TRPV1 in the process. Furthermore, TRPV1 can be expressed on different cell subsets such as primary sensory nerves or immune cells and the interaction between them may shape the final outcome of TRPV1 activation.
TRPV1 and Implication in Cancer
TRPV1 and Cancer Development
It is widely known that chronic inflammation is associated with tumorigenesis and aberrant calcium signaling promotes proliferation, metastasis, and cancer cell survival. TRPV1 is linked with both the process of inflammation and calcium signaling, thus, its contribution to cancer progression gained more attention.
Functional expression of TRPV1 was demonstrated in several tumor types including human breast cancer cell lines (MCF-7 and BT-20), human papillary thyroid carcinoma BCPAP cells, prostate cancer (LNCaP and PC-3), urothelial cancer cells, and glioma (51–55).
Weber et al. (54) showed that TRPV1 expression was upregulated in the several native breast cancers comparing to healthy tissue. On the contrary, downregulation of TRPV1 expression was associated with progression of urothelial cancer and thus potentially could be used as a prognostic marker (56). Also, studies by Vinuesa et al. (57) showed that TRPV1-deficient mouse more often developed colitis-associated cancer in the distal colon. It is surprising, especially that TRPV1 was suspected to be involved in inflammatory bowel diseases that actually predispose to colon cancer development. However, the exact role of TRPV1 in tumorigenesis is not clear. TRPV1 is sensitized by inflammatory mediators and its activation can lead to the release of calcitonin gene-related peptide (CGRP) and substance P, known proinflammatory factors. On the other hand, activation of TRPV1 might also lead to secretion of neuropeptides with anti-inflammatory properties such as somatostatin (58).
Although it was suggested that TRPV1 antagonism might be associated with a risk of skin tumor development via EGFR/Akt signaling, more recent study have shown that two commercially available TRPV1 antagonists (AMG-9810 and SB-705498) do not affect human keratinocytes proliferation in vitro as well as do not have an impact on the skin carcinogenesis in mouse study (59, 60). This confirms the possibility of using TRPV1 antagonists in the treatment of skin conditions such as pruritus associated with atopic dermatitis. The study of Hwang et al. (61) also demonstrated that capsaicin acts as a co-carcinogen in 12-O-tetradecanoylphorbol-13-acetate (TPA)-promoted skin carcinogenesis through the activation of EGFR/Akt signaling but without the involvement of TRPV1 channel.
TRPV1 and Cancer Therapy
Several studies addressed the TRPV1 activation in anti-cancer therapy via harnessing the Ca2+ signaling. Activation of TRPV1 by capsaicin (150 μM), was shown to significantly reduce proliferation and induce apoptosis of aggressive triple-negative breast cancer cell line (SUM149PT) (54). On the other hand, studies by Pecze et al. (62) showed that despite the presence of the TRPV1 protein expression in the breast (MCF7, MDA-MB-231, BT-474) and prostate carcinoma (PC-3, Du 145, LNCaP) cell lines, capsaicin administration at 50 μM dose did not exhibit any cytotoxic effect. However, when cells were transiently transfected with cDNA coding human TRPV1, administration of lower doses of capsaicin (2 μM) caused significant Ca2+ accumulation in mitochondria, leading to apoptosis. Such discrepancies might result partially from complexity of cellular signaling pathways mediated and integrated by TRPV1 but also from other factors that influence TRPV1 functioning (Figure 2). Furthermore, capsaicin itself might act not only through TRPV1-dependent calcium signaling pathway. For instance, Hawng et al. (61) showed that the co-carcinogenic action of capsaicin in TPA-promoted skin carcinogenesis is associated with the activation of the EGFR/Akt pathway not Ca2+ signaling via TRPV1. Similarly, Bao et al. (63) demonstrated that in the osteosarcoma MG63 cells capsaicin treatment increases phosphorylation of AMPK and p53 in the TRPV1-independent manner. Also, Pramanik et al. (64) found that capsaicin inhibited β-catenin/TCF-1 signaling in the pancreatic cancer cells.
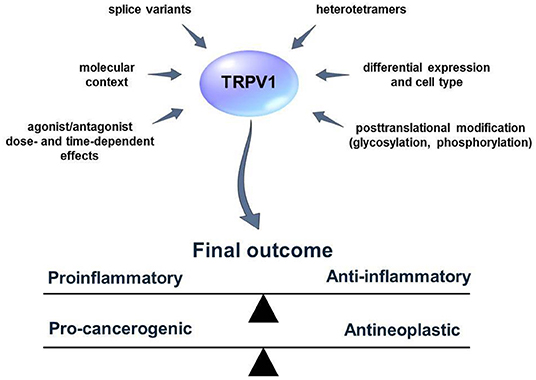
Figure 2. External and internal factors that influence TRPV1 activity and functions. Many aspects of the TRPV1 mode of action are not fully known. Several factors such as the existence of TRPV1 splice variants or heterotetramer formation can obscure the results of the studies concerning the role of TRPV1 in the process of inflammation and cancer. TRPV1 activators/blockers can exhibit synergistic/antagonistic or off-target effects with other elements of the living cell. Furthermore, TRPV1 might influence distinct cellular pathways and lead to cell-context depending effects. Defining those factors might help to predict the outcome of TRPV1 activation or blockage more accurately.
Also, little is known about functional expression of TRPV1 in cancer cells. To date, four splice variants of TRPV1 were described in the literature (65). The functional expression of TRPV1 splice variants in different cell types e.g., cancer or immune cells has to be addressed in future studies since it might have a great impact on the results. Pecze et al. (66), for instance, found expression of TRPV1 on both mRNA and protein levels in the human keratinocytes. However, they also show calcium overload associated cytotoxicity and subsequent keratinocytes death after CAP and RTX treatment at very high doses in a micromolar range (from 16 up to 300 μM) in contrast to nerve cells (nanomolar range). Interestingly, the authors found high mRNA expression of TRPV1b variant in keratinocytes but they were not able to show protein expression of both TRPV and TRPV1b. It might indicate no functional expression of the channel in the keratinocytes, however, it was not clearly stated whether the antibodies used in the study were able to recognize both splice variants of TRPV1. Furthermore, TRPV1b was documented to have a negative effect on the TRPV1 activation by capsaicin, heat, and pH as it was shown by Vos et al. (67) and thus in the cells with higher expression of TRPV1b over TRPV1, the effect of capsaicin treatment might not be visible when the low dose is applied. It is also speculated that different splice variants might undergo tetramerization, which is necessary for proper functioning of the TRPV1 ion channel. Also, it is suggested that TRPV1 forms heterotetramers with other TRP ion channels, for instance TRPA1 (68). This may produce unique properties in terms of activation and permeability of such heterotetramers. The effects of such a hetero-tetrameric assembly and its consequences in the progression of neoplastic diseases are yet to be determined.
Similarly, not only tetrameric assembly but also co-expression with other ion channels might have significant physiological effects. Weng et al. (69) demonstrated that regulation of association between TRPA1 and TRPV1 by transmembrane protein Tmem100 has a profound effect on pain perception. The authors found that close association between TRPA1 and TRPV1 leads to TRPV1-dependent inhibition of TRPA1 while in the presence of Tmem TRPA1-V1 association was weaker. Such results indicate that TRPV1 action in many cases cannot be considered separately from TRPA1.
Several studies have demonstrated that administration of chemotherapy along with TRPV1 activator—capsaicin, results in synergistic effect leading to increased apoptosis and suppression of tumor cell migration. Studies by Deveci et al. (70) showed that activation of TRPV1 is associated with significantly higher level of apoptosis in the MCF-7 human breast cancer cell line than in the cells treated with anticancer drug 5-Fluorouracil alone. Similarly, Nur et al. (71) revealed that the apoptotic effect of cisplatin was improved by activation of TRPV1 in the MCF-7 cell line. Furthermore, alteration of TRPV1 activity by capsaicin was shown to significantly reduce the migration and invasion of human papillary thyroid carcinoma BCPAP cells (55).
The mechanism by which activation of TRPV1 channel improves chemotherapy outcomes is yet to be determined. It is speculated that activation of TRP channels and its subsequent opening allows for direct permeation of small cytotoxic drugs through the pore (72). It is in accordance with studies by Hofmann et al. (73) who demonstrated that TRPV1 is involved in the uptake of anandamide, the endogenous cannabinoid and agonist of TRPV1 implicated in tumor-angiogenesis, by human endothelial colony-forming cells (ECFCs), and a human endothelial-vein cell line (E.A.hy926). The authors suggest that TRPV1 might create a pore that allows for passage of small molecules. Ortega-Guerrero et al. (74) performed a simulation which shows a high probability of doxorubicin permeation through TRPV1 but only when the channel is dilated. Based on these results, the authors implied that the TRPV1 channel might serve as a pathway for cancer therapy drug delivery systems. Despite these promising results, we cannot exclude that there is other, unknown mechanism involved in the transport of chemotherapeutic agents and small molecules or cell sensitization.
In many studies, capsaicin was used as a potent TRPV1 activator. However, it is important to distinguish between the effect of TRPV1 activation and the effect of the capsaicin itself. Studies by Gonzalez et al. (75) revealed that capsaicin treatment decreased the viability of the oral squamous cell carcinoma (OSCC) cells but the mechanism was independent of TRPV1 activation. Using calcium imaging, authors proved that TRPV1 channels were not functional in the OSCC cells and the cytotoxic effect of capsaicin and CPZ, was due to the promotion of reactive oxygen species (ROS) production by vanilloids. Similarly, Zhang et al. (76) showed that administration of capsaicin along with sorafenib leads to significant inhibition of the hepatocellular carcinoma (HCC) cells proliferation. The authors revealed, however, that the expression of TRPV1 was not detected in the HCC cells and sensitivity to sorafenib was increased due to capsaicin-dependent phosphorylation of ERK. Also, Kim et al. (77) showed that longer exposure to high doses of capsaicin (100 μM, 24 h) indeed is able to induce apoptosis in the gastric cancer cells (AGS, MKN45, and Hs746T). However, in the same time, lower doses of capsaicin (~20 μM, ~3 h) caused significant impairment of NK cells performance including the reduction in cytotoxic activity and cytokine production important for augmenting NK cell-mediated antitumor reaction. Most importantly, capsaicin-mediated dysfunction of NK cell was mostly TRPV1-independent (77). Bao et al. (63) demonstrated that activation of TRPV1 by capsaicin was essential to induce cytotoxic effects on osteosarcoma MG63 cells. Nevertheless, authors also showed that capsaicin treatment induces activation of the AMPK-p53 pathway which promotes apoptosis. Importantly, blocking TRPV1 activation with CPZ did not inhibit the phosphorylation of AMPK and p53, suggesting a TRPV1-independent action of capsaicin. Importantly, several studies documented inhibition of transient potassium currents but also store-operated calcium entry (SOCE) caused by capsaicin itself, especially at high concentrations (78, 79). Furthermore, capsaicin was shown to halt the cell cycle at G2/M phase and disrupt mitochondrial membrane potential leading to mitochondria-mediated apoptosis (80). Thus, capsaicin may exert cytotoxic effects without TRPV1 channel involvement.
On the contrary to the antitumor effect of capsaicin, Caprodossi et al. (81) have demonstrated that capsaicin administration upregulated genes associated with angiogenesis, invasiveness and metastasis processes in the human urothelial cancer cell line (5,637) that was TRPV1-deficient. The same cell line transfected with TRPV1 and treated with capsaicin showed significant increase in intracellular Ca2+ levels and subsequent growth inhibition and the increase of apoptosis (81). The effects of capsaicin and TRPV1 blockers on cancer cells are summarized in Table 2.
The scientific data underlines the role of TRPV1 as a potential calcium signaling modulator and drug target against cancer.
TRPV1 and Immunity
TRPV1 was found recently to be expressed in the cells of both innate and adaptive immune system including macrophages, dendritic cells, T lymphocytes, NK cells (49, 77, 82, 83). TRPV1 appeared to be a putative modulator of the immune cell functioning since it is involved in both calcium signaling and transduction of external stimuli such as temperature or pH. However, the data about its exact role in the immune system is not well-determined and, in many cases, the results are contradictory. Furthermore, based on the studies by Cavanaugh et al. (84, 85) that incorporated two lines of knock-in mice expressing reporter genes under endogenous TRPV1 promotor (TRPV1Cre and TRPV1PLAP−nlacZ), TRPV1 mRNA expression was shown to be confined mostly to peptidergic sensory neurons but functional expression was also found in arteriolar smooth muscle cells. The results, therefore, do not support the hypothesis of TRPV1 expression in other cell types including immune cells. On the other hand, Xue et al. (86) drew attention to the fact that TRPV1 transcription might be regulated by a dual promotor system. Authors found that despite one of the promotors (P1) exhibit strong activity in sensory neurons, it was also active in the fibroblast cell line (3T3) in a lower but significant levels. For this reason, the authors did not rule out the possibility of TRPV1 expression in other cell types including PBMCs.
Calcium ions are well-known second messenger which in immune cell play a crucial role in activation, differentiation, proliferation, cytokine secretion, and effector functions (87, 88). Also, temperature and cationic milieu (including H+ concentration) were demonstrated to have a great impact on immune cells performance (89, 90). For instance, the elevated temperature was shown to enhance differentiation of T cell into effector cells while low pH is associated with impairment of T cell action (91, 92). Also, magnesium ions play important role in immunity and disruption of Mg2+ homeostasis was associated with immune system malfunctioning e.g., T cell-related immunodeficiency (93). Interestingly, studies by Cao et al. (94) showed that the presence of divalent cations such as magnesium alters TRPV1 activation threshold. Thus, TRPV1 seems to be a good candidate for immune cells modulation.
TRPV1 was reported to be involved in macrophages-associated defense mechanism. It was shown that treatment with capsazepine, an antagonist of TRPV1, significantly decreased the production of proinflammatory cytokines such as IL-6, IL-1β, and IL-18 and cyclooxygenase 2 (COX-2) in the LPS-stimulated murine macrophages (41). The data indicate that TRPV1 is involved in macrophages activity and that potentially TRPV1 antagonist can be used to suppress inflammatory responses and to treat sepsis. Also, TRPV1 activation by evodiamine (0.5 μM) or capsaicin (10 μM) in primary murine macrophages was linked with decrease in production of pro-inflammatory cytokines such as MCP-1 (Monocyte Chemoattractant Protein 1) and IL-6 induced by TNF-α while the treatment with TRPV1 antagonist, CPZ, abolish the anti-inflammatory effect (95). Deletion of TRPV1 in murine model caused impairment of proper macrophages-associated defense mechanism leading to systemic inflammation. Nevius et al. (96) showed that macrophages in the pancreatic lymph nodes (PLN) respond to orally administrated capsaicin and upregulate expression of anti-inflammatory cytokines such as IL-10. Interestingly, enhancement of macrophages population by capsaicin was linked with decrease in proliferation of autoreactive T cells. The authors demonstrated also that capsaicin did not inhibit proliferation of T cells from PLN in the TRPV1 KO mice which suggest that TRPV1 might be used as a pharmacological target against autoimmune diabetes (96).
Dendritic cells (DCs), similarly to macrophages, are cells of the innate immune system responsible for antigen presentation, stimulation of T cells and shaping the adaptive immune system. TRPV1 was found to be expressed on the murine dendritic cells and the activation by capsaicin was shown to stimulate DC, their maturation and migration to the lymph nodes of the wild type mice. Dendritic cells derived from the bone marrow of TRPV1−/− mice did not undergo maturation after agonist treatment which indicated the pivotal role of TRPV1 in that process (97). On the other hand, studies by O'Connell et al. (98) have not confirmed the TRPV1 expression in the DCs. Authors were not able to elicit calcium influx on Ca2+-imaging experiment or change in membrane currents using electrophysiological techniques in DC treated with 1 μM capsaicin. The effect of capsaicin treatment was, however, pronounced in the sensory neurons and HEK293 cells expressing TRPV1. Also, the authors were not able to detect TRPV1 transcript in the DCs and thus they concluded that murine DCs do not express capsaicin receptor (98). It is worth to notice, that in the study by Basu and Srivastava (97) there was a dose-dependent effect of capsaicin on DCs maturation from WT mice with doses <10 μM having no significant effect. In the experiments performed by O'Connell et al. (98) 1 μM capsaicin was used. Discrepancies might arise from capsaicin doses, capsaicin treatment time or differences in the cell type (DC, neurons, transfected HEK cells). In DCs the expression of TRPV1 could be lower or they can express different splice variants of TRPV1 and thus they need a stronger signal to become fully activated. Also, we cannot exclude the TRPV1-independent effect of capsaicin on DCs, especially during prolonged exposures. Recent studies by Assas et al. (83) revealed the functional expression of TRPV1 in the murine splenic dendritic cells, macrophages and lymphocytes. Authors also proved that splenic DCs respond in a dose-dependent manner (12, 50, and 100 μM) to capsaicin by increase of calcium levels and subsequent release of CGRP (Calcitonin-Gene Related Peptide) and most importantly the reaction was TRPV1-dependent since the same effects were not observed in the cells isolated from the TRPV1−/− mice. TRPV1 activation in DCs might, therefore, be important for maintaining immune homeostasis.
Despite that, gene expression of TRPV1 was found in neutrophils, capsaicin and low pH did not alter calcium influx. It can indicate no functional expression of TRPV1 channel in neutrophils (99). On the contrary, Schepetkin et al. (100) however showed that phytochemicals from Ferula akitschkensis modulate responses of human neutrophils partially via TRPV1. Thus, the authors demonstrated that TRPV1 might be functionally active in human neutrophils. Furthermore, Kose and Nazıroǧlu (101) showed the evidence of calcium fluxes in neutrophils in response to capsaicin that were decreased by both administration of N-acetyl cysteine or TRPV1 blocker capsazepine. The authors concluded that TRPV1 in neutrophils from patients suffering from polycystic ovary syndrome (PCOS) takes part in calcium entry and might be associated with calcium-entry dependent activation of neutrophils and release of pro-inflammatory cytokines. Nevertheless, the knowledge about TRPV1 in neutrophils is very limited and thus it requires further refinement.
Most of the cells from the lymphoid lineage, namely T cells, B-cell and NK cells, express TRPV1 channel. Kim et al. (77) showed that both human NK cell line (NKL) and NK cells isolated from the human PBMCs express TRPV1 on both mRNA and protein level. Interestingly, authors demonstrated that protein expression of TRPV1 in the NK cell was comparable to PBMCs and U87 glioma cells, but was significantly lower from the expression level in the murine brain which confirms differences in the expression levels between different tissues. Importantly, TRPV1 expression in the human NK cells was proved to be functional as the treatment by 10 μM capsaicin-induced rise in intracellular Ca2+ concentration blocked by pretreatment with SB 366791 at the concentration of 10 μM or capsazepine at the concentration of 1 μM (77). The exact role of TRPV1 in the NK cells remains vague. It was shown that treatment with TRPV1 antagonist, capsazepine, caused the increase in the population of NK cells but also downregulated activation of NKT cells in the mice infected with Plasmodium (102). Nevertheless, it is hard to determine whether these effects were TRPV1-dependent.
Using flow cytometry, Assas et al. (103) demonstrated increase in the intracellular calcium concentration after capsaicin (100 μM) treatment. The murine splenic cells were gated on F480+, CD11c (macrophages and dendritic cells, respectively), CD19 which refers to B-cells and also on CD4+ and CD8+ standing for T helper and T cytotoxic cells. Also, it was confirmed that subpopulations of these cells express functional TRPV1 channel (103).
The data about TRPV1 expression in the B lymphocytes is very limited. To date, only studies by Soutar et al. (104) raised the issue related to TRPV1 and B lymphocytes. Authors showed that piperine-mediated inhibition of B cell proliferation, reduction of MHC II, CD40, and CD86 and decline in the production of IL-6, IL-10, and immunoglobulins. Nevertheless, piperine had the same effect on the B-cells isolated from TRPV1 KO mice and thus, the effect of piperine was TRPV1-independent. No data about TRPV1 expression in the B-cells and limited data leaves the room open for further investigation in this matter.
Unlike B cells, TRPV1 implication in T cells is a little bit more extensive, especially in the context of inflammatory and autoimmune disorders. TRPV1 was documented to be involved in the process of TCR signaling, T cell proliferation and differentiation and cytokine production (82, 97). Also, it was demonstrated that TRPV1 KO mice exhibit the reduction in the number of CD4+ and CD8+ cells in both blood and spleen when compared to wild type mice (105). The role of TRPV1 in the induction of pro- or anti-inflammatory properties of CD4+ cells is more elusive.
Studies by Bertin et al. (14) revealed high expression of TRPV1 in the murine splenic CD4+ cells on both mRNA and protein level, but also in human primary T cells and Jurkat cells. The authors also confirmed the functionality of the TRPV1 channel using patch-clamp recording along with cell stimulation with 16 μM of capsaicin. Most importantly, TRPV1 was shown to co-localize with CD4+ and Lck kinase and to be recruited into protein clusters upon TCR crosslinking with anti-CD3. This indicates that TRPV1 channel is involved in TCR signaling and in T cell activation. Also, TRPV1−/− CD4+ cells exhibited significantly lower calcium influx in comparison to wild type cells when TCR was stimulated with anti-CD3 (14).
Furthermore, the role of TRPV1 in the T helper cells was linked with proinflammatory properties. Samivel et al. (106) have demonstrated that TRPV1 knockdown caused decrease in production of IL-4, IL-5, IL-6, IL-17 by murine CD4+ cell after TCR stimulation. Also, Jurkat cells pretreated with TRPV1 inhibitor BCTC before CD3/CD28 stimulation showed downregulation of IL-10 production. Therefore, in the model of allergic rhinitis (AR), it was shown that TRPV1 plays an important role in the activation of proinflammatory properties of Th cells. It might be used as a potential therapeutic target against inflammatory and autoimmune diseases (106). Also, Bertin et al. (107) found that the expression of TRPV1 on CD4+ was implicated in T cells activation and more prone differentiation into Th1 effector subtype in the murine model of colitis. Genetic or pharmacological inhibition of TRPV1 on CD4+ cells was also associated with the reduction of airway inflammation in allergic asthma (16).
On the other hand, Motte et al. (108) found that oral pretreatment with capsaicin in the Lewis rat experimental autoimmune neuritis caused significant reduction of sciatic nerve inflammation, the decrease in the number of infiltrating T cell and macrophages and reduction in proinflammatory cytokines production (TNFα and ING-γ) (Table 3). Interestingly, capsaicin pretreatment also caused upregulation of TRPV1 expression in the T cells and macrophages infiltrating sciatic nerve. Whether the amelioration of inflammation was TRPV1-dependent or caused by capsaicin itself, needs to be further determined. Also, studies by Acharya et al. (109) demonstrated that TRPV1 is involved in maintaining immune homeostasis in the gut via endocannabinoid system. The authors revealed that capsaicin similarly to endogenous cannabinoid anandamide [AEA] increased the frequency of CXCR1hi MΦ known for their regulatory functions. Furthermore, co-culture of naïve splenic T cells with capsaicin-pretreated myeloid cells resulted in induction of the regulatory subset of CD4+ producing both IL-10 and INF-γ, namely Tr1 cells via stimulation of IL-27 production by MΦ. Interestingly, capsaicin treatment does not affect both the frequency and functions of FoxP3 T reg but caused the decrease in the frequency of Th17 cells (109). The study, thus emphasizes the role of TRPV1 agonist in the induction of anti-inflammatory properties of CD4+ cells in the gut. However, it is worth to mention that capsaicin was shown to decrease proliferation ratio also in T cells lacking TRPV1 and thus might indicate the TRPV1-independent inhibition of colitogenic T cells (115).
An important aspect to consider is the capsaicin dose used in the studies. It is widely known that capsaicin triggers calcium influx into nerve cells or transfected with TRPV1 HEK293 cells at relatively low doses (1 μM or less). The same effect was not observed, however, in most of the studies on the immune cells at the same doses. The use of higher doses of capsaicin raises the question about receptor-dependent signaling. In contrast to neurons, TRPV1 expression is lower in other cell types such as immune cells or keratinocytes (31, 66). Correlation between TRPV1 expression level and receptor-dependent calcium influx caused by capsaicin in other cell types, including immune cells, should be carefully addressed in further studies.
Another important aspect that should not be overlooked is an indirect impact of TRPV1 activation on the immune cell functioning. Currently, it is known that nociceptors with high expression of TRPV1 channel and immune cells act together especially at lymphoid tissues (lymph nodes) and barrier tissues such as skin, mucosa or gut (111, 116, 117). Activation of TRPV1+ neurons leads to the release of neuropeptides such as CGRP which affects the cytokine production, recruitment or polarization of the immune cells (118).
Cohen et al. (111) found that cutaneous TRPV1+ neurons are located close to dermal DCs. More importantly, the authors revealed that activation of neuronal TRPV1 channel was associated with the production of IL-23 by dermal DCs and further production of IL-17A by γδ T cells that elicit host defense against pathogens such as Candida albicans and Staphylococcus aureus. Similarly, Kashem et al. (112) demonstrated that the ablation of TRPV1+ sensory neurons led to a decrease in IL-23 production, γδ T cells number and subsequent increase in fungal burden. Nevertheless, several studies reported that the ablation of TRPV1+ neurons might be beneficial in the treatment of inflammatory disorders and some infection. Riol–Blanco et al. (113) found that the pharmacological or genetic ablation of TRPV1+ fibers is followed by a decrease in IL-23 production and recruitment of inflammatory cells in the murine model of psoriasiform skin inflammation. The authors confirmed that TRPV1+ neurons are a crucial element of the IL-23/IL-17 pathway and thus, might be used as a target to modulate the local immune response. Pinho–Riberio et al. (110) demonstrated that the induction of severe hyperalgesia, associated with infection with Streptococcus pyogenes, was mediated by direct activation of nociceptors by bacterial toxin SLS. Activation of TRPV+ neurons by SLS results in CGRP release which in turn suppresses the neutrophils defense against the pathogen. Authors, also found that silencing the nociceptors by botulinum neurotoxin A or use of CGPR antagonist stopped the progression of infection. Also, Baral et al. (114) found that activation of TRPV1+ nociceptors is associated with suppression of the host defense in the course of Staphylococcus aureus pneumonia. The authors showed that the ablation of TRPV1+ nociceptors increased survival rate and decreased bacterial burden in a murine pneumonia model.
The neuro-immune crosstalk in many aspects remains elusive. Nevertheless, communication between the nervous and immune system is a promising field for future research and is of great importance for medical purposes.
Conclusions and Perspectives
TRPV1 ion channel is a polymodal cellular receptor that can perceive different stimuli, integrate them and translate to the language of calcium-based signals. Therefore, it constitutes an important link between the extracellular environment and cellular response. Recent studies are increasingly turning attention to TRPV1 as a potential target to treat different disorders including inflammation, autoimmune diseases, and cancer. There are no doubts that TRPV1-based signaling might take part in the regulation of cellular functions in both health and disease. However, TRPV1 display a promiscuous nature with regard to its expression and activation. In many cases, the results regarding its role in inflammation, cancer, and immunity are contradictory. The experimental design, as well as the results analysis, requires thus greater cautions. It is important to take into consideration differences that result from study type (in vitro vs. in vivo), cell type and subsequent expression level, possible splice variants, agonist dose and time interactions, synergistic or antagonistic influence of endogenous agonists, or point mutations that can modulate channel activity. More efforts need to be put to distinguish the effect of TRPV1 activation from capsaicin action, especially with particular emphasizes on doses and treatment duration in both in vitro and in vivo studies. Furthermore, the dynamics of TRPV1 expression has to be more precisely determined along with the phenomenon called desensitization and internalization which might influence the final outcome. Moreover, the exact molecular pathways that TRPV1 is involved in are not well-understood and thus special precautions need to be taken. Lastly, more specific activators and blockers of TRPV1 ion channel should be used in order to assess its function more precisely and avoid off-target effects.
Studies on the role of TRPV1 channel are complex and demanding, however, they also offer an attractive treatment possibility for several diseases by modulation of cell functions. Deepening the knowledge about TRPV1 functioning contribute toward better understanding of cellular processes important not only in the inflammation or malignant transformation. Recent attempts showed that TRPV1 can be used in the remote regulation of gene expression and modulation of insulin secretion (119). Also, TRPV1 KO mice exhibit youth-metabolism and are long living which implicates TRPV1 in studies related to obesity, metabolic dysfunctions, and aging. Therefore, further studies addressing the role of TRPV1 might be beneficial also for other fields of biomedical sciences.
Author Contributions
JB and DK: manuscript writing. JB, DK, and IS: table and figures preparation. PB and KM: critical review, help with conception, and expertise.
Funding
This work was supported in part, by National Science Center Poland 2016/21/N/NZ6/02137 grant (to JB) and programme First TEAM, Grant No. POIR.04.04.00-00-3EE9/17-00 of Foundation for Polish Science financed by the European Union under the European Regional Development Fund (to KM).
Conflict of Interest
The authors declare that the research was conducted in the absence of any commercial or financial relationships that could be construed as a potential conflict of interest.
References
1. Binnewies M, Roberts EW, Kersten K, Chan V, Fearon DF, Merad M, et al. Understanding the tumor immune microenvironment (TIME) for effective therapy. Nat Med. (2018) 24:541–50. doi: 10.1038/s41591-018-0014-x
2. Lin Q, Jin S, Han M, Zheng W, Liu J, Wei X. Inflammation in the tumor microenvironment. J Immunol Res. (2018) 2018:1965847. doi: 10.1155/2018/1965847
3. Frankenreiter S, Bednarczyk P, Kniess A, Bork NI, Straubinger J, Koprowski P, et al. cGMP-elevating compounds and ischemic conditioning provide cardioprotection against ischemia and reperfusion injury via cardiomyocyte-specific BK channels. Circulation. (2017) 136:2337–55. doi: 10.1161/CIRCULATIONAHA.117.028723
4. Leanza L, Managò A, Zoratti M, Gulbins E, Szabo I. Pharmacological targeting of ion channels for cancer therapy: in vivo evidences. Biochim Biophys Acta. (2016) 1863:1385–97. doi: 10.1016/j.bbamcr.2015.11.032
5. Prevarskaya N, Skryma R, Shuba Y. Ion channels in cancer: are cancer hallmarks oncochannelopathies? Physiol Rev. (2018) 98:559–621. doi: 10.1152/physrev.00044.2016
6. Nilius B, Owsianik G. The transient receptor potential family of ion channels. Genome Biol. (2011) 12:218. doi: 10.1186/gb-2011-12-3-218
7. Wang H, Siemens J. TRP ion channels in thermosensation, thermoregulation and metabolism. Temperature. (2015) 2:178–87. doi: 10.1080/23328940.2015.1040604
8. Caterina MJ, Schumacher MA, Tominaga M, Rosen TA, Levine JD, David J. The capsaicin receptor: a heat-activated ion channel in the pain pathway. In Nature. (1997) 816–24. doi: 10.1038/39807
9. Aneiros E, Cao L, Papakosta M, Stevens EB, Phillips S, Grimm C. The biophysical and molecular basis of TRPV1 proton gating. EMBO J. (2011) 30:994–1002. doi: 10.1038/emboj.2011.19
10. Boonen B, Alpizar YA, Sanchez A, López-Requena A, Voets T, Talavera K. Differential effects of lipopolysaccharide on mouse sensory TRP channels. Cell Calcium. (2018) 73:72–81. doi: 10.1016/j.ceca.2018.04.004
11. Yang F, Ma L, Cao X, Wang K, Zheng J. Divalent cations activate TRPV1 through promoting conformational change of the extracellular region. J Gen Physiol. (2014) 143:91–103. doi: 10.1085/jgp.201311024
12. Birder LA, Nakamura Y, Kiss S, Nealen ML, Barrick S, Kanai AJ, et al. Altered urinary bladder function in mice lacking the vanilloid receptor TRPV1. Nat Neurosci. (2002) 5:856–60. doi: 10.1038/nn902
13. Rehman R, Bhat YA, Panda L, Mabalirajan U. TRPV1 inhibition attenuates IL-13 mediated asthma features in mice by reducing airway epithelial injury. Int Immunopharmacol. (2013) 15:597–605. doi: 10.1016/j.intimp.2013.02.010
14. Bertin S, Aoki-Nonaka Y, de Jong PR, Nohara LL, Xu H, Stanwood SR, et al. The ion channel TRPV1 regulates the activation and proinflammatory properties of CD4+T cells. Nat Immunol. (2014) 15:1055–63. doi: 10.1038/ni.3009
15. Diaz-Garcia CM, Morales-Lázaro SL, Sánchez-Soto C, Velasco M, Rosenbaum T, Hiriart M. Role for the TRPV1 channel in insulin secretion from pancreatic beta cells. J Membr Biol. (2014) 247:479–91. doi: 10.1007/s00232-014-9658-8
16. Baker K, Raemdonck K, Dekkak B, Snelgrove RJ, Ford J, Shala F, et al. Role of the ion channel, transient receptor potential cation channel subfamily V member 1 (TRPV1) in allergic asthma. Respir Res. (2016) 17:67. doi: 10.1186/s12931-016-0384-x
17. Han SB, Kim H, Cho SH, Lee JD, Chung JH, Kim HS. Transient receptor potential vanilloid-1 in epidermal keratinocytes may contribute to acute pain in herpes zoster. Acta Derm Venereol. (2016) 96:319–22. doi: 10.2340/00015555-2247
18. Yu X, Yu M, Liu Y, Yu S. TRP channel functions in the gastrointestinal tract. Semin Immunopathol. (2016) 38:385–96. doi: 10.1007/s00281-015-0528-y
19. Malenczyk K, Girach F, Szodorai E, Storm P, Segerstolpe Å, Tortoriello G, et al. A TRPV1-to-secretagogin regulatory axis controls pancreatic β-cell survival by modulating protein turnover. EMBO J. (2017) 36:2107–25. doi: 10.15252/embj.201695347
20. Medzhitov R. Origin and physiological roles of inflammation. Nature. (2008) 454:428–35. doi: 10.1038/nature07201
21. Sharma P. Inflammation and the metabolic syndrome. Indian J Clin Biochem. (2011) 26:317–8. doi: 10.1007/s12291-011-0175-6
22. Pohl D, Benseler S. Systemic inflammatory and autoimmune disorders. Handb Clin Neurol. (2013) 112:1243–52. doi: 10.1016/B978-0-444-52910-7.00047-7
23. Chen WW, Zhang X, Huang WJ. Role of neuroinflammation in neurodegenerative diseases (Review). Mol Med Rep. (2016) 13:3391–6. doi: 10.3892/mmr.2016.4948
24. Murata M. Inflammation and cancer. Environ Health Prev Med. (2018) 23:50. doi: 10.1186/s12199-018-0740-1
25. Tsuji F, Aono H. Role of transient receptor potential vanilloid 1 in inflammation and autoimmune diseases. Pharmaceuticals. (2012) 5:837–52. doi: 10.3390/ph5080837
26. Hsieh WS, Kung CC, Huang SL, Lin SC, Sun WH. TDAG8, TRPV1, and ASIC3 involved in establishing hyperalgesic priming in experimental rheumatoid arthritis. Sci Rep. (2017) 7:8870. doi: 10.1038/s41598-017-09200-6
27. Kim JH. The emerging role of TRPV1 in airway inflammation. Allergy Asthma Immunol Res. (2018) 10:187–8. doi: 10.4168/aair.2018.10.3.187
28. Csekő K, Beckers B, Keszthelyi D, Helyes Z. Role of TRPV1 and TRPA1 ion channels in inflammatory bowel diseases: potential therapeutic targets? Pharmaceuticals. (2019) 12:E48. doi: 10.3390/ph12020048
29. Song J, Kang J, Lin B, Li J, Zhu Y, Du J, et al. Mediating role of TRPV1 ion channels in the co-exposure to PM2.5 and formaldehyde of Balb/c mice asthma model. Sci Rep. (2017) 7:11926. doi: 10.1038/s41598-017-11833-6
30. Choi JY, Lee HY, Hur J, Kim KH, Kang JY, Rhee CK, et al. TRPV1 blocking alleviates airway inflammation and remodeling in a chronic asthma murine model. Allergy Asthma Immunol Res. (2018) 10:216–24. doi: 10.4168/aair.2018.10.3.216
31. Engler A, Aeschlimann A, Simmen BR, Michel BA, Gay RE, Gay S, et al. Expression of transient receptor potential vanilloid 1 (TRPV1) in synovial fibroblasts from patients with osteoarthritis and rheumatoid arthritis. Biochem Biophys Res Commun. (2007) 359:884–8. doi: 10.1016/j.bbrc.2007.05.178
32. Guarino MP, Cheng L, Ma J, Harnett K, Biancani P, Altomare A, et al. Increased TRPV1 gene expression in esophageal mucosa of patients with non-erosive and erosive reflux disease. Neurogastroenterol Motil. (2010) 22:746–51 e219. doi: 10.1111/j.1365-2982.2010.01514.x
33. Silva RO, Bingana RD, Sales TMAL, Moreira RLR, Costa DVS, Sales KMO, et al. Role of TRPV1 receptor in inflammation and impairment of esophageal mucosal integrity in a murine model of nonerosive reflux disease. Neurogastroenterol Motil. (2018) 23:e13340. doi: 10.1111/nmo.13340
34. Ma J, Altomare A, Guarino M, Cicala M, Rieder F, Fiocchi C, et al. HCl-induced and ATP-dependent upregulation of TRPV1 receptor expression and cytokine production by human esophageal epithelial cells. Am J Physiol Gastrointest Liver Physiol. (2012) 303:G635–45. doi: 10.1152/ajpgi.00097.2012
35. Kistner K, Siklosi N, Babes A, Khalil M, Selescu T, Zimmermann K, et al. Systemic desensitization through TRPA1 channels by capsazepine and mustard oil - a novel strategy against inflammation and pain. Sci Rep. (2016) 6:28621. doi: 10.1038/srep28621
36. Behrendt HJ, Germann T, Gillen C, Hatt H, Jostock R. Characterization of the mouse cold-menthol receptor TRPM8 and vanilloid receptor type-1 VR1 using a fluorometric imaging plate reader (FLIPR) assay. Br J Pharmacol. (2004) 141:737–45. doi: 10.1038/sj.bjp.0705652
37. McNamara CR, Mandel-Brehm J, Bautista DM, Siemens J, Deranian KL, Zhao M, et al. TRPA1 mediates formalin-induced pain. Proc Natl Acad Sci USA. (2007) 104:13525–30. doi: 10.1073/pnas.0705924104
38. Hoffmeister C, Silva MA, Rossato MF, Trevisan G, Oliveira SM, Guerra GP, et al. Participation of the TRPV1 receptor in the development of acute gout attacks. Rheumatology. (2014) 53:240–9. doi: 10.1093/rheumatology/ket352
39. Lee JH, Choi CS, Bae IH, Choi JK, Park YH, Park M. A novel, topical, nonsteroidal, TRPV1 antagonist, PAC-14028 cream improves skin barrier function and exerts anti-inflammatory action through modulating epidermal differentiation markers and suppressing Th2 cytokines in atopic dermatitis. J Dermatol Sci. (2018) 91:184–94. doi: 10.1016/j.jdermsci.2018.04.017
40. Bryant P, Shumate M, Yumet G, Lang CH, Vary TC, Cooney RN. Capsaicin-sensitive nerves regulate the metabolic response to abdominal sepsis. J Surg Res. (2003) 112:152–61. doi: 10.1016/S0022-4804(03)00154-9
41. Ninomiya Y, Tanuma SI, Tsukimoto M. Differences in the effects of four TRPV1 channel antagonists on lipopolysaccharide-induced cytokine production and COX-2 expression in murine macrophages. Biochem Biophys Res Commun. (2017) 484:668–74. doi: 10.1016/j.bbrc.2017.01.173
42. Cabral LD, Giusti-Paiva A. The transient receptor potential vanilloid 1 antagonist capsazepine improves the impaired lung mechanics during endotoxemia. Basic Clin Pharmacol Toxicol. (2016) 119:421–7. doi: 10.1111/bcpt.12605
43. Kobayashi M, Watanabe K, Yokoyama S, Matsumoto C, Hirata M, Tominari T, et al. Capsaicin, a TRPV1 ligand, suppresses bone resorption by inhibiting the prostaglandin E production of osteoblasts, and attenuates the inflammatory bone loss induced by lipopolysaccharide. ISRN Pharmacol. (2012) 2012:439860. doi: 10.5402/2012/439860
44. Wang Y, Cui L, Xu H, Liu S, Zhu F, Yan F, et al. TRPV1 agonism inhibits endothelial cell inflammation via activation of eNOS/NO pathway. Atherosclerosis. (2017) 260:13–9. doi: 10.1016/j.atherosclerosis.2017.03.016
45. Wang Y, Novotny M, Quaiserová-Mocko V, Swain GM, Wang DH. TRPV1-mediated protection against endotoxin-induced hypotension and mortality in rats. Am J Physiol Regul Integr Comp Physiol. (2008) 294:R1517–23. doi: 10.1152/ajpregu.00005.2008
46. Chen JA, Hamers JP, Finsterbusch M, Massimo G, Zafar M, Corder R, et al. Endogenously generated arachidonate-derived ligands for TRPV1 induce cardiac protection in sepsis. In FASEB J. (2018) 32:3816–3831. doi: 10.1096/fj.201701303R
47. Feng J, Yang P, Mack MR, Dryn D, Luo J, Gong X, et al. Sensory TRP channels contribute differentially to skin inflammation and persistent itch. Nat Commun. (2017) 8:980. doi: 10.1038/s41467-017-01056-8
48. Wang Y, Wang DH. TRPV1 ablation aggravates inflammatory responses and organ damage during endotoxic shock. Clin Vaccine Immunol. (2013) 20:1008–15. doi: 10.1128/CVI.00674-12
49. Fernandes ES, Liang L, Smillie SJ, Kaiser F, Purcell R, Rivett DW, et al. TRPV1 deletion enhances local inflammation and accelerates the onset of systemic inflammatory response syndrome. J Immunol. (2012) 188:5741–51. doi: 10.4049/jimmunol.1102147
50. Clark N, Keeble J, Fernandes ES, Starr A, Liang L, Sugden D, et al. The transient receptor potential vanilloid 1 (TRPV1) receptor protects against the onset of sepsis after endotoxin. FASEB J. (2007) 21:3747–55. doi: 10.1096/fj.06-7460com
51. Sanchez MG, Sanchez AM, Collado B, Malagarie-Cazenave S, Olea N, Carmena MJ, et al. Expression of the transient receptor potential vanilloid 1 (TRPV1) in LNCaP and PC-3 prostate cancer cells and in human prostate tissue. Eur J Pharmacol. (2005) 515:20–7. doi: 10.1016/j.ejphar.2005.04.010
52. Amantini C, Mosca M, Nabissi M, Lucciarini R, Caprodossi S, Arcella A, et al. Capsaicin-induced apoptosis of glioma cells is mediated by TRPV1 vanilloid receptor and requires p38 MAPK activation. J Neurochem. (2007) 102:977–90. doi: 10.1111/j.1471-4159.2007.04582.x
53. Amantini C, Ballarini P, Caprodossi S, Nabissi M, Morelli MB, Lucciarini R, et al. Triggering of transient receptor potential vanilloid type 1 (TRPV1) by capsaicin induces Fas/CD95-mediated apoptosis of urothelial cancer cells in an ATM-dependent manner. Carcinogenesis. (2009) 30:1320–9. doi: 10.1093/carcin/bgp138
54. Weber LV, Al-Refae K, Wölk G, Bonatz G, Altmüller J, Becker C, et al. Expression and functionality of TRPV1 in breast cancer cells. Breast Cancer. (2016) 8:243–52. doi: 10.2147/BCTT.S121610
55. Xu S, Zhang L, Cheng X, Yu H, Bao J, Lu R. Capsaicin inhibits the metastasis of human papillary thyroid carcinoma BCPAP cells through the modulation of the TRPV1 channel. Food Funct. (2018) 9:344–54. doi: 10.1039/C7FO01295K
56. Kalogris C, Caprodossi S, Amantini C, Lambertucci F, Nabissi M, Morelli MB, et al. Expression of transient receptor potential vanilloid-1 (TRPV1) in urothelial cancers of human bladder: relation to clinicopathological and molecular parameters. Histopathology. (2010) 57:744–52. doi: 10.1111/j.1365-2559.2010.03683.x
57. Vinuesa AG, Sancho R, García-Limones C, Behrens A, Ten Dijke P, Calzado MA, et al. Vanilloid receptor-1 regulates neurogenic inflammation in colon and protects mice from colon cancer. Cancer Res. (2012) 72:1705–1716. doi: 10.1158/0008-5472.CAN-11-3693
58. Szolcsányi J, Pintér E, Helyes Z, Petho G. Inhibition of the function of TRPV1-expressing nociceptive sensory neurons by somatostatin 4 receptor agonism: mechanism and therapeutical implications. Curr Top Med Chem. (2011) 11:2253–63. doi: 10.2174/156802611796904852
59. Li S, Bode AM, Zhu F, Liu K, Zhang J, Kim MO, et al. TRPV1-antagonist AMG9810 promotes mouse skin tumorigenesis through EGFR/Akt signaling. Carcinogenesis. (2011) 32:779–85. doi: 10.1093/carcin/bgr037
60. Park M, Naidoo AA, Burns A, Choi JK, Gatfield KM, Vidgeon-Hart M, et al. Do TRPV1 antagonists increase the risk for skin tumourigenesis? A collaborative in vitro and in vivo assessment. Cell Biol Toxicol. (2018) 34:143–62. doi: 10.1007/s10565-017-9407-8
61. Hwang MK, Bode AM, Byun S, Song NR, Lee HJ, Lee KW, et al. Cocarcinogenic effect of capsaicin involves activation of EGFR signaling but not TRPV1. Cancer Res. (2010) 70:6859–69. doi: 10.1158/0008-5472.CAN-09-4393
62. Pecze L, Jósvay K, Blum W, Petrovics G, Vizler C, Oláh Z, et al. Activation of endogenous TRPV1 fails to induce overstimulation-based cytotoxicity in breast and prostate cancer cells but not in pain-sensing neurons. Biochim Biophys Acta. (2016) 1863:2054–64. doi: 10.1016/j.bbamcr.2016.05.007
63. Bao Z, Dai X, Wang P, Tao Y, Chai D. Capsaicin induces cytotoxicity in human osteosarcoma MG63 cells through TRPV1-dependent and -independent pathways. Cell Cycle. (2019) 18:1379–92. doi: 10.1080/15384101.2019.1618119
64. Pramanik KC, Fofaria NM, Gupta P, Ranjan A, Kim SH, Srivastava SK. Inhibition of β-catenin signaling suppresses pancreatic tumor growth by disrupting nuclear β-catenin/TCF-1 complex: critical role of STAT-3. Oncotarget. (2015) 6:11561–74. doi: 10.18632/oncotarget.3427
65. Schumacher MA, Eilers H. TRPV1 splice variants: structure and function. Front Biosci. (2010) 15:872–82. doi: 10.2741/3651
66. Pecze L, Szabó K, Széll M, Jósvay K, Kaszás K, Kúsz E, et al. Human keratinocytes are vanilloid resistant. PLoS ONE. (2008) 3:e3419. doi: 10.1371/journal.pone.0003419
67. Vos MH, Neelands TR, McDonald HA, Choi W, Kroeger PE, Puttfarcken PS, et al. TRPV1b overexpression negatively regulates TRPV1 responsiveness to capsaicin, heat and low pH in HEK293 cells. J Neurochem. (2006) 99:1088–102. doi: 10.1111/j.1471-4159.2006.04145.x
68. Staruschenko A, Jeske NA, Akopian AN. Contribution of TRPV1-TRPA1 interaction to the single channel properties of the TRPA1 channel. J Biol Chem. (2010) 285:15167–77. doi: 10.1074/jbc.M110.106153
69. Weng HJ, Patel KN, Jeske NA, Bierbower SM, Zou W, Tiwari V, et al. Tmem100 is a regulator of TRPA1-TRPV1 complex and contributes to persistent pain. Neuron. (2015) 85:833–46. doi: 10.1016/j.neuron.2014.12.065
70. Deveci HA, Nazıroǧlu M, Nur G. 5-Fluorouracil-induced mitochondrial oxidative cytotoxicity and apoptosis are increased in MCF-7 human breast cancer cells by TRPV1 channel activation but not Hypericum perforatum treatment. Mol Cell Biochem. (2018) 439:189–98. doi: 10.1007/s11010-017-3147-1
71. Nur G, Nazıroǧlu M, Deveci HA. Synergic prooxidant, apoptotic and TRPV1 channel activator effects of alpha-lipoic acid and cisplatin in MCF-7 breast cancer cells. J Recept Signal Transduct Res. (2017) 37:569–77. doi: 10.1080/10799893.2017.1369121
72. Nabissi M, Morelli MB, Santoni M, Santoni G. Triggering of the TRPV2 channel by cannabidiol sensitizes glioblastoma cells to cytotoxic chemotherapeutic agents. Carcinogenesis. (2013) 34:48–57. doi: 10.1093/carcin/bgs328
73. Hofmann NA, Barth S, Waldeck-Weiermair M, Klec C, Strunk D, Malli R, et al. TRPV1 mediates cellular uptake of anandamide and thus promotes endothelial cell proliferation and network-formation. Biol Open. (2014) 3:1164–72. doi: 10.1242/bio.20149571
74. Ortega-Guerrero A, Espinosa-Duran JM, Velasco-Medina J. TRPV1 channel as a target for cancer therapy using CNT-based drug delivery systems. Eur Biophys J. (2016) 45:423–33. doi: 10.1007/s00249-016-1111-8
75. Gonzalez CB, Kirma NB, De La Chapa JJ, Chen R, Henry MA, Luo S, et al. Vanilloids induce oral cancer apoptosis independent of TRPV1. Oral Oncol.(2014) 50:437–47. doi: 10.1016/j.oraloncology.2013.12.023
76. Zhang SS, Ni YH, Zhao CR, Qiao Z, Yu HX, Wang LY, et al. Capsaicin enhances the antitumor activity of sorafenib in hepatocellular carcinoma cells and mouse xenograft tumors through increased ERK signaling. Acta Pharmacol Sin. (2018) 39:438–48. doi: 10.1038/aps.2017.156
77. Kim HS, Kwon HJ, Kim GE, Cho MH, Yoon SY, Davies AJ, et al. Attenuation of natural killer cell functions by capsaicin through a direct and TRPV1-independent mechanism. Carcinogenesis. (2014) 35:1652–60. doi: 10.1093/carcin/bgu091
78. Wang JP, Tseng CS, Sun SP, Chen YS, Tsai CR, Hsu MF. Capsaicin stimulates the non-store-operated Ca2+ entry but inhibits the store-operated Ca2+ entry in neutrophils. Toxicol Appl Pharmacol. (2005) 209:134–44. doi: 10.1016/j.taap.2005.04.002
79. Yang R, Xiong Z, Liu C, Liu L. Inhibitory effects of capsaicin on voltage-gated potassium channels by TRPV1-independent pathway. Cell Mol Neurobiol. (2014) 34:565–76. doi: 10.1007/s10571-014-0041-1
80. Lin CH, Lu WC, Wang CW, Chan YC, Chen MK. Capsaicin induces cell cycle arrest and apoptosis in human KB cancer cells. BMC Complement Altern Med. (2013) 13:46. doi: 10.1186/1472-6882-13-46
81. Caprodossi S, Amantini C, Nabissi M, Morelli MB, Farfariello V, Santoni M, et al. Capsaicin promotes a more aggressive gene expression phenotype and invasiveness in null-TRPV1 urothelial cancer cells. Carcinogenesis. (2011) 32:686–94. doi: 10.1093/carcin/bgr025
82. Majhi RK, Sahoo SS, Yadav M, Pratheek BM, Chattopadhyay S, Goswami C. Functional expression of TRPV channels in T cells and their implications in immune regulation. FEBS J. (2015) 282:2661–81. doi: 10.1111/febs.13306
83. Assas BM, Wakid MH, Zakai HA, Miyan JA, Pennock JL. Transient receptor potential vanilloid 1 expression and function in splenic dendritic cells: a potential role in immune homeostasis. Immunology. (2016) 147:292–304. doi: 10.1111/imm.12562
84. Cavanaugh DJ, Chesler AT, Bráz JM, Shah NM, Julius D, Basbaum AI. Restriction of transient receptor potential vanilloid-1 to the peptidergic subset of primary afferent neurons follows its developmental downregulation in nonpeptidergic neurons. J Neurosci. (2011) 31:10119–27. doi: 10.1523/JNEUROSCI.1299-11.2011
85. Cavanaugh DJ, Chesler AT, Jackson AC, Sigal YM, Yamanaka H, Grant R, et al. Trpv1 reporter mice reveal highly restricted brain distribution and functional expression in arteriolar smooth muscle cells. J Neurosci. (2011) 31:5067–77. doi: 10.1523/JNEUROSCI.6451-10.2011
86. Xue Q, Jong B, Chen T, Schumacher MA. Transcription of rat TRPV1 utilizes a dual promoter system that is positively regulated by nerve growth factor. J Neurochem. (2007) 101:212–22. doi: 10.1111/j.1471-4159.2006.04363.x
87. Toldi G. The regulation of calcium homeostasis in T lymphocytes. Front Immunol. (2013) 4:432. doi: 10.3389/fimmu.2013.00432
88. Feske S, Wulff H, Skolnik EY. Ion channels in innate and adaptive immunity. Annu Rev Immunol. (2015) 33:291–353. doi: 10.1146/annurev-immunol-032414-112212
89. Bellone M, Calcinotto A, Filipazzi P, De Milito A, Fais S, Rivoltini L. The acidity of the tumor microenvironment is a mechanism of immune escape that can be overcome by proton pump inhibitors. Oncoimmunology. (2013) 2:e22058. doi: 10.4161/onci.22058
90. Eil R, Vodnala SK, Clever D, Klebanoff CA, Sukumar M, Pan JH, et al. Ionic immune suppression within the tumour microenvironment limits T cell effector function. Nature. (2016) 537:539–43. doi: 10.1038/nature19364
91. Mace TA, Zhong L, Kilpatrick C, Zynda E, Lee CT, Capitano M, et al. Differentiation of CD8+ T cells into effector cells is enhanced by physiological range hyperthermia. J Leukoc Biol. (2011) 90:951–62. doi: 10.1189/jlb.0511229
92. Nakagawa Y, Negishi Y, Shimizu M, Takahashi M, Ichikawa M, Takahashi H. Effects of extracellular pH and hypoxia on the function and development of antigen-specific cytotoxic T lymphocytes. Immunol Lett. (2015) 167:72–86. doi: 10.1016/j.imlet.2015.07.003
93. Li FY, Chaigne-Delalande B, Kanellopoulou C, Davis JC, Matthews HF, Douek DC, et al. Second messenger role for Mg2+ revealed by human T-cell immunodeficiency. Nature. (2011) 475:471–6. doi: 10.1038/nature10246
94. Cao X, Ma L, Yang F, Wang K, Zheng J. Divalent cations potentiate TRPV1 channel by lowering the heat activation threshold. J Gen Physiol. (2014) 143:75–90. doi: 10.1085/jgp.201311025
95. Zhao JF, Ching LC, Kou YR, Lin SJ, Wei J, Shyue SK, et al. Activation of TRPV1 prevents OxLDL-induced lipid accumulation and TNF-α-induced inflammation in macrophages: role of liver X receptor α. Mediators Inflamm. (2013) 2013:925171. doi: 10.1155/2013/925171
96. Nevius E, Srivastava PK, Basu S. Oral ingestion of Capsaicin, the pungent component of chili pepper, enhances a discreet population of macrophages and confers protection from autoimmune diabetes. Mucosal Immunol. (2012) 5:76–86. doi: 10.1038/mi.2011.50
97. Basu S, Srivastava P. Immunological role of neuronal receptor vanilloid receptor 1 expressed on dendritic cells. Proc Natl Acad Sci USA. (2005) 102:5120–5. doi: 10.1073/pnas.0407780102
98. O'Connell PJ, Pingle SC, Ahern GP. Dendritic cells do not transduce inflammatory stimuli via the capsaicin receptor TRPV1. FEBS Lett. (2005) 579:5135–9. doi: 10.1016/j.febslet.2005.08.023
99. Heiner I, Eisfeld J, Lückhoff A. Role and regulation of TRP channels in neutrophil granulocytes. Cell Calcium. (2003) 33:533–40. doi: 10.1016/S0143-4160(03)00058-7
100. Schepetkin IA, Kushnarenko SV, Özek G, Kirpotina LN, Sinharoy P, Utegenova GA, et al. Modulation of human neutrophil responses by the essential oils from ferula akitschkensis and their constituents. J Agric Food Chem. (2016) 64:7156–70. doi: 10.1021/acs.jafc.6b03205
101. Köse SA, Nazıroǧlu M. N-acetyl cysteine reduces oxidative toxicity, apoptosis, and calcium entry through TRPV1 channels in the neutrophils of patients with polycystic ovary syndrome. Free Radic Res. (2015) 49:338–46. doi: 10.3109/10715762.2015.1006214
102. Fernandes ES, Brito CX, Teixeira SA, Barboza R, dos Reis AS, Azevedo-Santos AP, et al. TRPV1 antagonism by capsazepine modulates innate immune response in mice infected with Plasmodium berghei ANKA. Mediators Inflamm. (2014) 2014:506450. doi: 10.1155/2014/506450
103. Assas BM, Abdulaal WH, Wakid MH, Zakai HA, Miyan J, Pennock JL. The use of flow cytometry to examine calcium signalling by TRPV1 in mixed cell populations. Anal Biochem. (2017) 527:13–9. doi: 10.1016/j.ab.2017.03.025
104. Soutar DA, Doucette CD, Liwski RS, Hoskin DW. Piperine, a pungent alkaloid from black pepper, inhibits B lymphocyte activation and effector functions. Phytother Res. (2017) 31:466–74. doi: 10.1002/ptr.5772
105. Amantini C, Farfariello V, Cardinali C, Morelli MB, Marinelli O, Nabissi M, et al. The TRPV1 ion channel regulates thymocyte differentiation by modulating autophagy and proteasome activity. Oncotarget. (2017) 8:90766–80. doi: 10.18632/oncotarget.21798
106. Samivel R, Kim DW, Son HR, Rhee YH, Kim EH, Kim JH, et al. The role of TRPV1 in the CD4+ T cell-mediated inflammatory response of allergic rhinitis. Oncotarget. (2016) 7:148–60. doi: 10.18632/oncotarget.6653
107. Bertin S, Aoki-Nonaka Y, Lee J, de Jong PR, Kim P, Han T, et al. The TRPA1 ion channel is expressed in CD4+ T cells and restrains T-cell-mediated colitis through inhibition of TRPV1. Gut. (2017) 66:1584–96. doi: 10.1136/gutjnl-2015-310710
108. Motte J, Ambrosius B, Grüter T, Bachir H, Sgodzai M, Pedreiturria X, et al. Capsaicin-enriched diet ameliorates autoimmune neuritis in rats. J Neuroinflammation. (2018) 15:122. doi: 10.1186/s12974-018-1165-x
109. Acharya N, Penukonda S, Shcheglova T, Hagymasi AT, Basu S, Srivastava PK. Endocannabinoid system acts as a regulator of immune homeostasis in the gut. Proc Natl Acad Sci USA. (2017) 114:5005–10. doi: 10.1073/pnas.1612177114
110. Pinho-Ribeiro FA, Baddal B, Haarsma R, O'Seaghdha M, Yang NJ, Blake KJ, et al. Blocking neuronal signaling to immune cells treats streptococcal invasive infection. Cell. (2018) 173:1083–97.e1022. doi: 10.1016/j.cell.2018.04.006
111. Cohen JA, Edwards TN, Liu AW, Hirai T, Jones MR, Wu J, et al. Cutaneous TRPV1 + neurons trigger protective innate type 17 anticipatory immunity. Cell. (2019) 178:919–932.e914. doi: 10.1016/j.cell.2019.06.022
112. Kashem SW, Riedl MS, Yao C, Honda CN, Vulchanova L, Kaplan DH. Nociceptive sensory fibers drive interleukin-23 production from CD301b+ dermal dendritic cells and drive protective cutaneous Immunity. Immunity. (2015) 43:515–26. doi: 10.1016/j.immuni.2015.08.016
113. Riol-Blanco L, Ordovas-Montanes J, Perro M, Naval E, Thiriot A, Alvarez D, et al. Nociceptive sensory neurons drive interleukin-23-mediated psoriasiform skin inflammation. Nature. (2014) 510:157–61. doi: 10.1038/nature13199
114. Baral P, Umans BD, Li L, Wallrapp A, Bist M, Kirschbaum T, et al. Nociceptor sensory neurons suppress neutrophil and γδ T cell responses in bacterial lung infections and lethal pneumonia. Nat Med. (2018) 24:417–26. doi: 10.1038/nm.4501
115. Belmaáti MS, Diemer S, Hvarness T, Baumann K, Pedersen AE, Christensen RE, et al. Antiproliferative effects of TRPV1 ligands on non-specific and enteroantigen-specific T cells from wild-type and Trpv1 KO mice. Inflamm Bowel Dis. (2014) 20:1004–14. doi: 10.1097/MIB.0000000000000039
116. Wülfing C, Günther HS. Dendritic cells and macrophages neurally hard-wired in the lymph node. Sci Rep. (2015) 5:16866. doi: 10.1038/srep16866
117. Verheijden S, Boeckxstaens GE. Neuroimmune interaction and the regulation of intestinal immune homeostasis. Am J Physiol Gastrointest Liver Physiol. (2018) 314:G75–80. doi: 10.1152/ajpgi.00425.2016
118. Dantzer R. Neuroimmune interactions: from the brain to the immune system and vice versa. Physiol Rev. (2018) 98:477–504. doi: 10.1152/physrev.00039.2016
Keywords: TRPV1, capsaicin, immune cells, LPS, capsazepine, ion channels
Citation: Bujak JK, Kosmala D, Szopa IM, Majchrzak K and Bednarczyk P (2019) Inflammation, Cancer and Immunity—Implication of TRPV1 Channel. Front. Oncol. 9:1087. doi: 10.3389/fonc.2019.01087
Received: 17 July 2019; Accepted: 02 October 2019;
Published: 16 October 2019.
Edited by:
Benjamin Frey, University of Erlangen Nuremberg, GermanyReviewed by:
Avi Priel, Hebrew University of Jerusalem, IsraelHongzhen Hu, Washington University in St. Louis, United States
Peter W. Reeh, University of Erlangen Nuremberg, Germany
Copyright © 2019 Bujak, Kosmala, Szopa, Majchrzak and Bednarczyk. This is an open-access article distributed under the terms of the Creative Commons Attribution License (CC BY). The use, distribution or reproduction in other forums is permitted, provided the original author(s) and the copyright owner(s) are credited and that the original publication in this journal is cited, in accordance with accepted academic practice. No use, distribution or reproduction is permitted which does not comply with these terms.
*Correspondence: Joanna Katarzyna Bujak, am9hbm5hX2J1amFrQHNnZ3cucGw=