- Department of Pharmaceutical and Pharmacological Sciences, University of Padua, Padua, Italy
Oligodendrogenesis and oligodendrocyte precursor maturation are essential processes during the course of central nervous system development, and lead to the myelination of axons. Cells of the oligodendrocyte lineage are generated in the germinal zone from migratory bipolar oligodendrocyte precursor cells (OPCs), and acquire cell surface markers as they mature and respond specifically to factors which regulate proliferation, migration, differentiation, and survival. Loss of myelin underlies a wide range of neurological disorders, some of an autoimmune nature—multiple sclerosis probably being the most prominent. Current therapies are based on the use of immunomodulatory agents which are likely to promote myelin repair (remyelination) indirectly by subverting the inflammatory response, aspects of which impair the differentiation of OPCs. Cells of the oligodendrocyte lineage express and are capable of responding to a diverse array of ligand-receptor pairs, including neurotransmitters and nuclear receptors such as γ-aminobutyric acid, glutamate, adenosine triphosphate, serotonin, acetylcholine, nitric oxide, opioids, prostaglandins, prolactin, and cannabinoids. The intent of this review is to provide the reader with a synopsis of our present state of knowledge concerning the pharmacological properties of the oligodendrocyte lineage, with particular attention to these receptor-ligand (i.e., neurotransmitters and nuclear receptor) interactions that can influence oligodendrocyte migration, proliferation, differentiation, and myelination, and an appraisal of their therapeutic potential. For example, many promising mediators work through Ca2+ signaling, and the balance between Ca2+ influx and efflux can determine the temporal and spatial properties of oligodendrocytes (OLs). Moreover, Ca2+ signaling in OPCs can influence not only differentiation and myelination, but also process extension and migration, as well as cell death in mature mouse OLs. There is also evidence that oligodendroglia exhibit Ca2+ transients in response to electrical activity of axons for activity-dependent myelination. Cholinergic antagonists, as well as endocannabinoid-related lipid-signaling molecules target OLs. An understanding of such pharmacological pathways may thus lay the foundation to allow its leverage for therapeutic benefit in diseases of demyelination.
Introduction
The central nervous system (CNS) relies on a network of neuronal cells to transmit electrical impulses known as action potentials along the axon. A lipid-rich membrane, myelin (Virchow, 1854), insulates the axon and allows for rapid conduction of such impulses and proper delivery to the target cell (Hartline and Colman, 2007). Schwann cells supply myelin for the peripheral nervous system (PNS), whereas in the CNS, oligodendrocytes (OLs; Iglesias-Rozas and Garrosa, 2012) are responsible for myelin production, and are generated in the germinal zone from migratory bipolar oligodendrocyte precursor cells (OPCs; Grinspan, 2002; Brazel et al., 2003). Myelinating OLs not only provide trophic support for axons, but also release lactate through the monocarboxylate transporter 1 which is then utilized by axons for mitochondrial adenosine triphosphate (ATP) generation (Saab et al., 2013). The migration of OPCs is influenced by receptor-ligand adhesions with the extracellular matrix, such as integrins, and signaling molecules (Soliven, 2001) which may provide a critical link between neuronal cell activity and OPCs. Cells of the OL lineage acquire cell surface markers with maturation and respond specifically to factors which regulate proliferation, migration, differentiation, and survival (Figure 1).
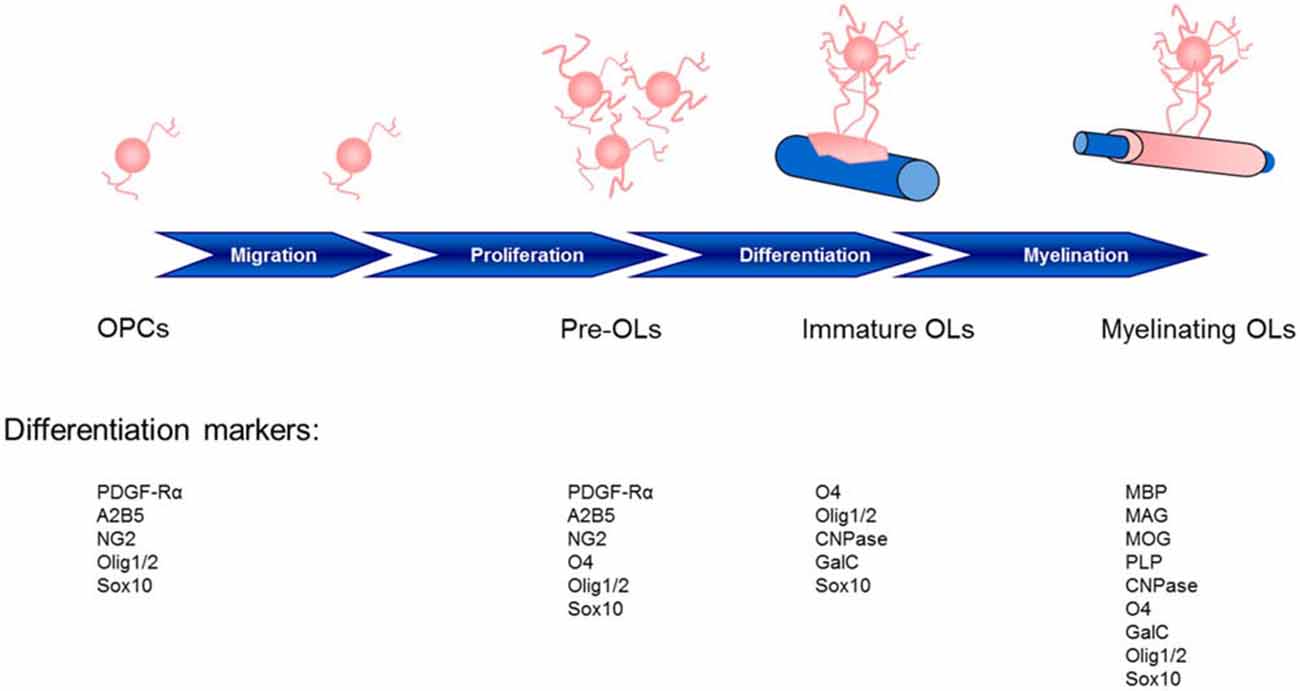
Figure 1. Stages of oligodendrocyte maturation toward the oligodendroglial lineage: oligodendrocyte precursor cells (OPCs), preoligodendrocytes (pre-OLs), immature oligodendrocytes (OLs) and myelinating mature OLs. These stages are identified by their increasingly complex morphology and expression pattern of well-defined markers. Two basic helix-loop-helix transcription factors, Olig1 and Olig2, play essential roles in determining the oligodendroglial lineage and in the generation and maturation of OLs (Zhou et al., 2000; Lu et al., 2001). The localization of Olig1/2 is observed in the nucleus of OPCs during development; however, although Olig2 remains in the nucleus of OPCs in the adult mouse (Arnett et al., 2004), Olig1 translocation into the cytosol highly correlates with the differentiation of OLs, the termination of the cell cycle and Olig1 phosphorylation (Niu et al., 2012). CNPase, 2′,3′-cyclic nucleotide 3′-phosphodiesterase; GalC, galactocerebroside C; MAG, myelin associated glycoprotein; MBP, myelin basic protein; MOG, myelin oligodendrocyte glycoprotein; PDGF-Rα, platelet-derived growth factor receptor; PLP, proteolipid protein.
Impairment of one or more of these processes can lead to myelin degeneration, dysfunction or loss in nerve signal conduction and ultimately, nerve deterioration (Felts et al., 1997; Mensch et al., 2015). Loss of myelin results in a wide range of neurological disorders, including reduced motor function, impaired cognitive abilities, and vision problems. Demyelinating diseases of the CNS include multiple sclerosis (MS), acute disseminated encephalomyelitis, neuromyelitis optica, transverse myelitis, central pontine myelinolysis and leukodystrophy, while those of the PNS include chronic inflammatory demyelinating polyneuropathy, Guillain-Barré syndrome and Charcot-Marie-Tooth disease (http://www.neurodegenerationresearch.eu/about/what). Perinatal white matter (WM) injury, or periventricular leukomalacia, is the most common cause of brain injury in premature infants and is the leading cause of cerebral palsy. Late OPCs exhibit selective vulnerability in this last pathology (Back et al., 2007). Contrary to what was once believed, we now know that the mammalian CNS can undergo neurogenesis and gliogenesis, re-establishing axon-glial interactions needed for remyelination (Compston, 2002). Importantly, cells of the OL lineage express and respond to a broad range of receptor-ligand pairs, in particular neurotransmitters and nuclear receptors (NRs) like glutamate (Glu), γ-aminobutyric acid (GABA), ATP, serotonin, acetylcholine (ACh), nitric oxide (NO), opioids, prostaglandins, prolactin (PRL), cannabinoids and the superfamilty of NRs (steroid hormones, sex hormones, oxysterols, vitamin D3, thyroid hormone (TH), retinoic acid, fatty acid amides). Clearly, knowledge about the OL lineage has increased greatly over the past few years, with attention shifting towards manipulation of that lineage for therapeutic purposes. This review is intended to provide an overview of current knowledge on the pharmacological properties of the OL lineage, with particular attention to these receptor-ligand interactions and how they may influence OL migration, proliferation, differentiation, and myelination, together with an appraisal of their therapeutic potential. In this context, a number of mediators work through Ca2+ signaling, with the balance between Ca2+ influx and efflux determining the temporal and spatial properties of OLs. Moreover, Ca2+ signaling in OPCs can influence not only differentiation and myelination, but also process extension and migration, as well as cell death in mature mouse OLs. Research until now has identified cholinergic antagonists, as well as endocannabinoid-related lipid-signaling molecules with the ability to target OLs. Understanding the above-mentioned relationships may help to delineate additional new therapeutic avenues for remyelination/repair. The main systems examined are briefly summarized on Figure 2.
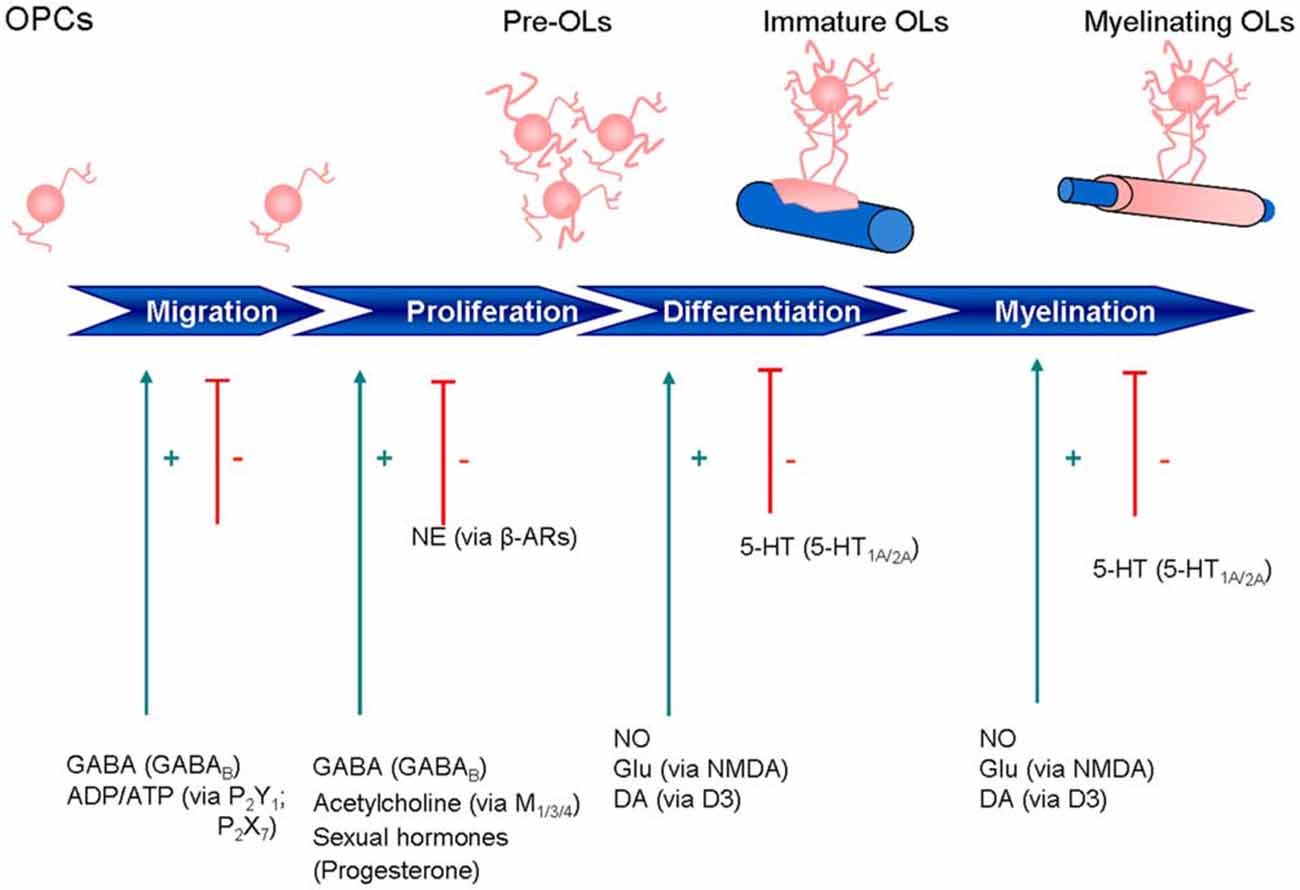
Figure 2. Expression of neurotransmitter and other signaling receptors during oligodendrocyte maturation. ADP, adenosine diphosphate; ATP, adenosine triphosphate; β-ARs, β-adrenoreceptors; DA, dopamine; GABA, γ-aminobutyric acid; GLU, glutamate; NE, norepinephrine; 5-HT, 5-hydroxytryptamine; NO, nitric oxide.
Materials and Methods
The literature search for this review was carried out according to the PRISMA (Preferred Reporting Items for Systematic Reviews and Meta-analyses) guidelines as they apply to systematic reviews (Moher et al., 2010). The search strategy is given after Conclusion and Perspectives. A total of 387 manuscripts were retrieved. Exclusion criteria are given in Figure 3. In all, 279 articles remained for this review.
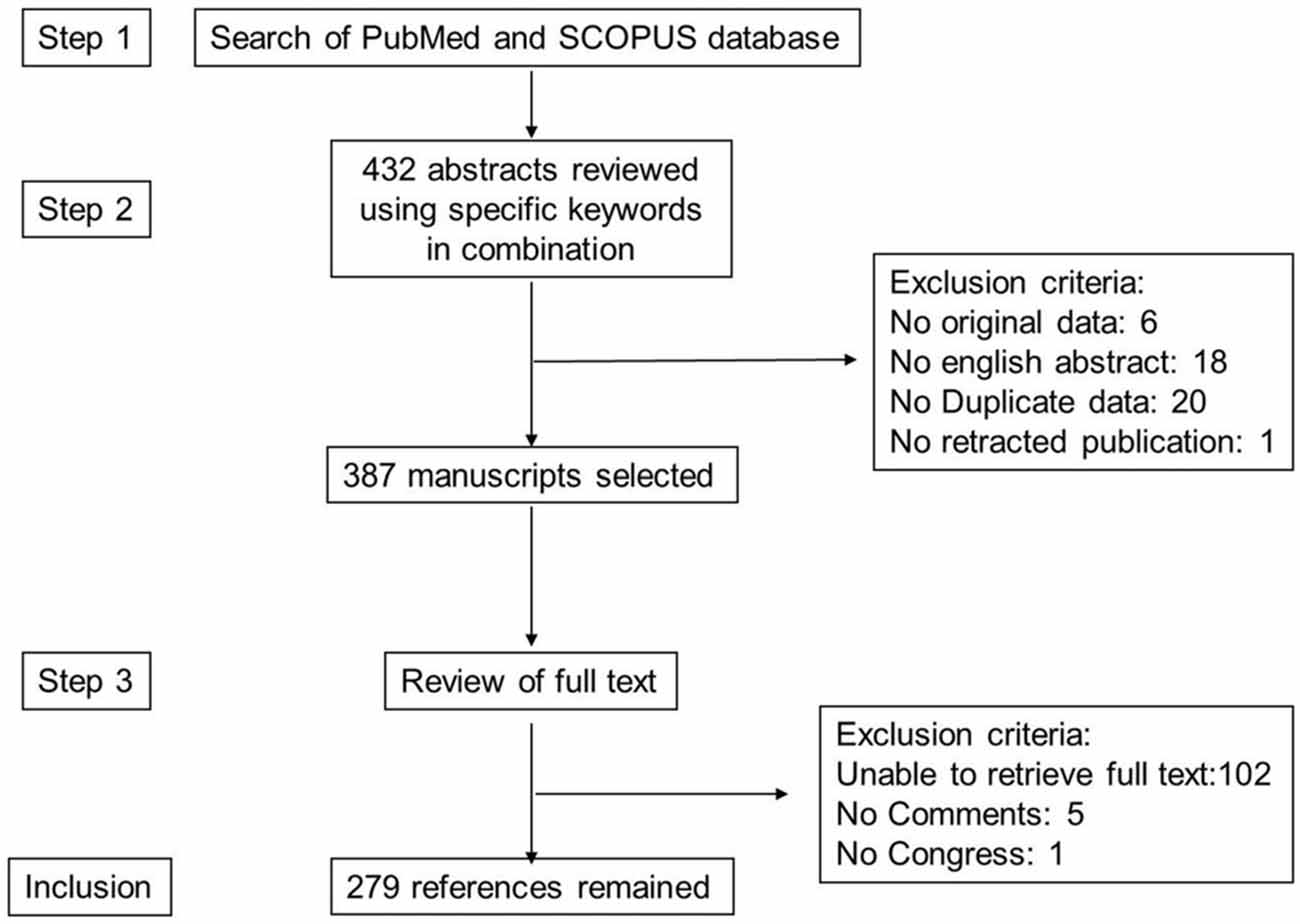
Figure 3. Study inclusion flowchart. This depicts the methodology for search and collection of relevant articles for this review, following PRISMA guidelines.
GABAergic Signals
GABA is the main inhibitory neurotransmitter in adult brain (Koós and Tepper, 1999; Markram et al., 2004; Takesian and Hensch, 2013), where it binds two main receptor types: fast hyperpolarizing ionotropic GABAA receptors and metabotropic GABAB receptors.
GABA participation in inhibitory neurotransmission is limited in adulthood, as in many brain regions during early development activation of GABAA receptors excites neurons through activation of Na-K-Cl cotransporter1 (NKCC1) symporter which increases extracellular K+ and a depolarizing efflux of Cl−, in turn triggering Ca2+ influx (Dzhala et al., 2005). Similarly OPCs, but not OLs, express NKCC1. Muscimol, a GABAA agonist, increases intracellular Ca2+ [Ca2+]i protecting cultured OLs following withdrawal of culture medium supplements (N1, biotin, platelet-derived growth factor, PDGF; Wang et al., 2003). Using the GABAA receptor antagonist pentylentetrazole, Mensch et al. (2015) showed a subtle increase in the number of OLs and a 40% increase in the number of myelin sheaths in the zebrafish ventral spinal cord. OLs may express also GABAB receptors, whose activation increases proliferation and migration of OPCs via a negatively coupled adenlyl cyclase signaling pathway. The GABAB receptor agonist baclofen significantly reduced cyclic adenosine monophosphate and adenlyl cyclase and led to an increase in OPC migration (Luyt et al., 2007).
Glutamatergic Signals
Glu, the principal excitatory neurotransmitter, acts on postsynaptic ionotropic and G-protein-coupled metabotropic (mGluR) receptors. The former comprise the ligand-gated ion channel N-methyl-D-aspartate (NMDA), alpha-amino-3-hydroxy-5-methylisoxazole-4-propionic acid (AMPA) and kainate receptor subtypes (Dingledine et al., 1999; Swanson et al., 2005). These receptors are expressed by glial cells in both gray matter (Jabs et al., 2005; Matute, 2006; Bakiri et al., 2009) and WM (Matute, 2010). Virtually all neurons expressing ionotropic Glu receptors are sensitive to excitotoxic injury (Choi, 1988; Lipton and Rosenberg, 1994). Glu is toxic also for astrocytes (Haas and Erdö, 1991) and OLs (Matute et al., 1997; McDonald et al., 1998).
Cells of the OL lineage express functional AMPA and kainate-type Glu receptors throughout their development and across species, including man. NMDA receptors on both immature and mature OLs can be activated during injury (Káradóttir et al., 2005; Salter and Fern, 2005; Micu et al., 2006). OLs express as well all three groups of metabotropic Glu receptors in a developmentally-regulated fashion (Deng et al., 2004; Spampinato et al., 2015). In addition to astrocytes, OLs also express glutamate transporters, mainly the Glu aspartate transporter (GLAST; EAAT1 in the modern nomenclature). The neuronal transporter, termed excitatory amino acid carrier 1 (EAAC1; EAAT3 in the modern nomenclature), is present in a subpopulation of adult OPCs (Domercq et al., 1999).
Primary cells of the OL lineage are highly vulnerable to a form of excitotoxic injury mediated by a transporter-related mechanism involving inhibition of cystine uptake, glutathione depletion and oxidative stress (Oka et al., 1993). Alterations in Glu homeostasis in WM may thus determine injury to OLs and myelin. Prolonged activation of GluRs is toxic to primary OLs in vitro and in vivo (Matute et al., 1997; McDonald et al., 1998; Li and Stys, 2000).
Activation of AMPA and kainate receptors on microglia leads to the release of tumour necrosis factor-α (TNF-α), which can potentiate Glu neurotoxicity and kill OLs, destroy myelin and damage axons (Merrill and Benveniste, 1996). Inflammatory cytokines like TNF-α and interleukin-1β released by reactive microglia can impair Glu uptake and trigger excitotoxic OL death (Takahashi et al., 2003). Indeed, inhibiting the expression and function of Glu transporters in axonal tracts is sufficient to induce OL loss and demyelination (Domercq et al., 2005). AMPA receptors on OLs lack GluR2 subunits, suggesting a higher Ca2+ permeability than for these cells in gray matter (Matute, 2006).
Myelin regeneration can occur spontaneously, even in pathological conditions such as MS. Using an in vivo remyelination model, Gautier et al. (2015) demonstrated that demyelinated axons are electrically active and generate de novo synapses with recruited OPCs which, early after lesion induction, sense neuronal activity by expressing AMPA/kainate receptors. Furthermore, blocking neuronal activity, axonal vesicular release or AMPA receptors in demyelinated lesions results in reduced remyelination. In the absence of neuronal activity there is a ~6-fold increase in OPC number within the lesions and a reduced proportion of differentiated OLs. These findings reveal that neuronal activity and release of glutamate instruct OPCs to differentiate into new myelinating OLs that recover lost function (Gautier et al., 2015).
Another mechanism of Glu action on OPC differentiation involves activation of specific NMDA receptor subunits, as NMDAR1 and NMDAR2A protein levels increase during differentiation whereas NMDAR2B and NMDAR3 levels decrease (Sawada et al., 1996; Cavaliere et al., 2012). These authors showed that activation of NMDA receptors during OLs differentiation elevated cytosolic Ca2+ levels and promoted myelination when co-cultured with neurons. NMDA receptors on multipotent stem cells promote maturation of OLs and favor myelination through production of reactive oxygen species; levels of the latter correlate with degree of differentiation, an effect negatively modulated by the NADPH inhibitor apocynin (Cavaliere et al., 2012). Interestingly, NMDA receptors are expressed in clusters on OL processes, whereas AMPA and kainate receptors are diffusely located on the cell somata (Káradóttir et al., 2005; Salter and Fern, 2005; Micu et al., 2006).
Activation of mGlu4 on astrocytes appears to be involved in sparing OLs from excitotoxic challenge (Spampinato et al., 2015), hinting that they may be a novel target to protect from demyelination. Other pharmacological approaches, such as ionotropic Glu receptor antagonists, increase OL survival but have no effect on neuroinflammation (Pitt et al., 2000). A close interplay between astrocytes and OLs is supported by the observation that kainate-induced toxicity is attenuated by stimulation of mGlu4 receptors only in a mixed culture of OLs and astrocytes; the mGlu4 receptor agonist L-AP4 does not act directly on OLs. Activation of mGluRs, including mGlu4 on astrocytes, is reported to be neuroprotective (Yao et al., 2005; Corti et al., 2007).
Soluble factors released by astrocytes might mediate L-AP4-increased OL viability. Transforming growth factor beta 1, which increases upon L-AP4 treatment, protects OLs from kainate-induced toxicity (Spampinato et al., 2015), an effect attenuated by a neutralizing anti-transforming growth factor beta 1 antibody. Factors that contribute to Glu homeostasis include glutaminase, which is present in OLs and microglia (Domercq et al., 1999; Werner et al., 2001). Non-toxic concentrations of Glu acting at kainate receptors can induce OL death also by sensitizing these cells to complement attack (Alberdi et al., 2006), an action mediated by proteolysis of complement protein C5 to generate the potent chemoattractant C5a which interacts with its G-protein-coupled receptor C5aR (CD88; Ward and Newman, 1969) on OLs and other CNS cells (Nataf et al., 2001).
In conclusion, factors that evoke an increase in [Ca2+]i can regulate cell proliferation, survival, growth and differentiation or induce a pathological condition, as a function of OL developmental stage.
Purinergic Signals
ATP is a co-transmitter released with other “classical” transmitters and activates ionotropic (P2X) and metabotropic (P2Y) receptors (Ralevic and Burnstock, 1998; North, 2002). In the CNS, ATP can be released by neurons and glia via membrane transporters, vesicles, and ATP-permeable channels (Fields and Stevens, 2000; Ballerini et al., 2002; Coco et al., 2003). OPCs express several ionotropic (P2X1,2,3,4,7) and metabotropic (P2Y1,2,4) receptors, with P2X7 and P2Y1 receptors being the main ionotropic and metabotropic P2 receptors, respectively (Agresti et al., 2005). ATP and adenosine diphosphate (ADP) may regulate OPC functions through P2Y1 receptors. For example, ATP and ADP (the P2Y1-specific agonist ADPbS) induced OPC migration. Moreover, ATP and ADP inhibited OPC proliferation induced by PDGF in purified cell cultures and in cerebellar tissue slices (Agresti et al., 2005). These effects of ATP and ADP on cell migration and proliferation were being blocked by a P2Y1 antagonist (MRS2179). P2Y1 receptors localize to NG2-labeled OPCs in the developing rat brain (Agresti et al., 2005).
OPC migration involves the interactive effects of growth factors, chemokines, integrins, neurotransmitters, and extracellular matrix molecules (Decker et al., 2000; Tsai and Miller, 2002). PDGF and basic fibroblast growth factor induce OPC migration in vitro, acting mainly as chemotactic stimuli (Armstrong et al., 1990; Milner et al., 1997), while ATP enhances the migration of OPCs by stimulating predominantly random motility and to a lesser extent, chemotaxis (Agresti et al., 2005). These authors propose that ATP, acting mainly at P2Y1 receptors, may be important in stimulating OPC migration along a chemotactic gradient towards specific sites. However, under pathological conditions the P2X7 receptor is involved in OPC migration (Feng et al., 2015). High concentrations of ATP or the P2X7 receptor agonist BzATP increased the number of migrating OPCs in vitro, while pre-treatment with the P2X7 receptor antagonist oxidized ATP decreased the promotive effect. This effect appeared to depend on Fyn, a member of the Src family of kinases (Feng et al., 2015).
Differentiated OLs in vitro express functional P2X and P2Y receptors, which can act as mediators of axono-oligodendroglial communication during myelination (Alberdi et al., 2005). P2X1 and P2X3 receptors produce rapidly desensitizing currents whereas P2X7 receptors, as well as P2X2 and P2X4 receptors, may undergo a conformational change which results in formation of a large pore upon prolonged exposure to ATP (Khakh et al., 1999). ATP signaling can directly trigger OL excitotoxicity via activation of Ca2+-permeable P2X7 receptors, leading to WM lesions reminiscent of MS plaques (Walz et al., 1993; Butt, 2006).
Serotoninergic Signals
Serotonin (5-hydroxytryptamine, 5-HT) plays critical roles in early neural development, being involved in neuronal cell differentiation and migration, axonal growth and pathfinding, dendritic arborization, synaptogenesis, circuit formation, and neuronal plasticity (Daubert and Condron, 2010). Mice with either 5-HT transporter (Esaki et al., 2005) or monoamine oxidase A gene deficiencies (Cases et al., 1996) show disrupted primary somatosensory cortical organization.
Depression is reported to be accompanied by myelin and OL abnormalities (Yamazaki et al., 2007), including a significant reduction in myelin basic protein (MBP) expression in multiple brain areas of post-mortem samples from depressed patients (Honer et al., 1999; Taylor et al., 2004; Regenold et al., 2007). Further, Aston et al. (2005) found in temporal cortex a down-regulation of both OL development-related and myelin formation-related genes.
Manipulation of 5-HT levels in postnatal day 6–8 rats with selective 5-HT reuptake inhibitors (SSRIs; Xu et al., 2004) or p-chlorophenylalanine-induced 5-HT depletion (Persico et al., 2000) leads to disorganized cortical barrel fields, abnormal social behavior, callosal myelin malformation, and OL pathology. 5-HT receptor subtypes 1A and 2A have been found in OL lineages and to co-localize with developmental lineage-selective markers (Fan et al., 2015). Exposure to 5-HT not only disturbed development of OLs with aberrant process outgrowth and reduced MBP and proteolipid protein (PLP) expression, but even caused a developmentally-dependent cell death at the highest doses via 5-HT2A. By means of a neuron-OL myelination co-culture model, Fan et al. (2015) demonstrated that 5-HT exposure alters the localization and regular space patterns of the paranodal contactin-associated protein (Caspr), but did not cause OL death or reduce OL density. These authors suggested that 5-HT exposure induces a diffuse and enlarged pattern affecting other axon-derived factors for myelination.
It is tempting to speculate that manipulation of 5-HT levels affects OL development and myelination, thereby contributing to the altered neural connectivity seen in SSRI-treated animals (Simpson et al., 2011). SSRIs (Loughhead et al., 2006) and serotonin/norepinephrine reuptake inhibitors (Wang et al., 2014), which are widely prescribed for major depression in pregnant women, are able to cross the placenta and reach fetal compartments. Animal data suggest that extracellular 5-HT levels in CNS could rise up to 20-fold upon SSRI exposure (Tao et al., 2000). Such high levels of extracellular 5-HT may adversely affect OL development and/or myelination, perhaps contributing to altered neural connectivity seen in depression and in autism spectrum disorders. Further, the late maturation of human myelin, especially in prefrontal cortex has potential implications in the treatment of humans with SSRIs in early adolescence or young adulthood.
Spinal cord injury (SCI) causes secondary damage involving OL cell death and axon demyelination. SSRIs like fluoxetine improve neurological outcome in animal models of SCI by inhibiting microglia activation which can cause the death of OLs. The underlying mechanism involves inhibition of p38 mitogen-activated protein kinase (p38-MAPK) and pro-nerve growth factor expression. In addition, fluoxetine can attenuate Ras homolog gene family member A activation, decrease the level of phosphorylated c-Jun and alleviate activation of caspase-3. Fluoxetine may thus be a novel therapeutic agent for acute SCI in humans (Lee et al., 2015).
Catecholaminergic Signals
The brain’s principal catecholamine neurotransmitters include 3,4-dihydroxyphenethylamine (dopamine, DA) and 4,5-β-trihydroxyphenethylamine (norepinephrine, NE). DA controls locomotor activity, cognition, emotion, positive reinforcement, food intake, and endocrine regulation (Missale et al., 1998). Neuropsychiatric and motor function disorders like schizophrenia and Parkinson’s disease (PD) are often linked to abnormal DA signaling (Civelli et al., 1993). DA receptors can be divided into two main groups: D1-like (D1 and D5; Sunahara et al., 1991; Tiberi et al., 1991) and D2-like (D2, D3, D4; Sokoloff et al., 1990; Van Tol et al., 1991; Sawada et al., 1998).
D2 and D3 receptors are expressed by differentiated rat cortical OLs (Rosin et al., 2005), although little is known about their role(s) in OL biology. Cortical OLs in vitro are vulnerable to receptor-independent Glu-induced cell death (Oka et al., 1993; Rosin et al., 2004) which involves oxidative stress, inhibition of cystine uptake and depletion of intracellular stores of reduced glutathione (Murphy et al., 1990). Glu-induced death of cortical OLs was protected by co-treatment with either D2 or D3 agonists (Avenell et al., 1999) and appeared to involve quinpirole-sensitive activation of MAPK/extracellular signal-regulated kinase and oxidative stress (Rosin et al., 2005). The protective effect of quinpirole was fully attenuated by the D2 receptor antagonist L-741,626 (Avenell et al., 1998). Intriguingly, oxygen/glucose deprivation up-regulated expression of D2 and D3 receptors over a much shorter time scale (2 h) compared to the time needed to induce injury (18 h; Rosin et al., 2005). Cellular responsiveness to oxygen/glucose deprivation injury may depend on the state of OL maturation (Deng et al., 2003). As D3 receptors are expressed in differentiating OLs before terminal maturation, it is possible that DA plays a role in OL lineage differentiation and/or the formation of myelin by mature OLs (Bongarzone et al., 1998).
NE is a hormone and neurotransmitter that affects brain areas involved in vigilant concentration. NE underlies the fight-or-flight response, directly increasing heart rate, triggering the release of glucose from energy stores, and increasing blood flow to skeletal muscle. OPCs express functional α- and β-adrenoreceptors (ARs). Response to NE is enhanced following OPC maturation into differentiated OLs: α1A-ARs induce phosphoinositide hydrolysis without effecting OPC proliferation, while β-ARs increase intracellular cyclic AMP and inhibit their proliferation (Cohen and Almazan, 1993; Ghiani et al., 1999). The effects of NE can be mirrored by molecules like phorbol esters, which mimic the action of second messengers, leading to inhibition of terminal differentiation of OLs and block of myelin-specific protein expression (Baron et al., 1998). Protein kinase C activators work at a later stage of differentiation to facilitate myelination (Vartanian et al., 1986; Asotra and Macklin, 1993; Yong et al., 1994).
Cholinergic Signals
ACh was the first identified neurotransmitter, and has important roles in neuron-glia signaling (Wessler et al., 2001). ACh can act on different tissues and cell types (Proskocil et al., 2004; Kawashima and Fuji, 2008) to modulate growth, survival, differentiation, and apoptosis (Eglen, 2005). While muscarinic cholinergic receptors can promote neuronal cell differentiation during neurogenesis (Lauder, 1993; Tata et al., 2003; Salani et al., 2009), nicotinic cholinergic receptors affect inflammation centrally by modulating microglial release of cytokines, and peripherally through immune system cells (Tracey, 2002; De Simone et al., 2005; Kawashima and Fuji, 2008). Several glial cell populations, including OLs express ACh receptors (Murphy et al., 1986; Van Der Zee et al., 1993; Rogers et al., 2001; Loreti et al., 2007).
Stimulation of muscarinic receptors on OLs (Larocca and Almazan, 1997; Molina-Holgado et al., 2003) activates MAPK and increases inositol trisphosphate levels and Ca2+ waves (Ritchie et al., 1987; Kastritsis and McCarthy, 1993; Cohen and Almazan, 1994; Larocca and Almazan, 1997). Rat OPCs express all muscarinic receptors with particular abundance of M3, while mature OLs have barely detectable levels of all subtypes except for M2, suggesting a dynamic regulation during OL maturation (De Angelis et al., 2011). Furthermore, specific physiological role(s) may be inferred from their diverse cellular distribution: M1 and M3 are localized in cell bodies and processes while M4 is found only in the cell body (De Angelis et al., 2011).
Muscarinic receptors can affect OPCs proliferation. For example, carbachol induces proliferation via both muscarinic and nicotinic cholinergic receptors (Peralta et al., 1987; Cohen et al., 1996). De Angelis et al. (2011) used selective muscarinic antagonists to discriminate receptor subtype involved in OPC proliferation. These authors showed that only M1, M3 and M4 antagonists were able to reduce proliferation. Indeed, gallamine did not affect proliferation via M2 antagonism. In contrast, M2 activation by arecaidine influenced OPC survival in a dose-dependent manner, showing toxic effects, indicating the involvement of M2 in OPC survival (De Angelis et al., 2011).
ACh receptors have been extensively studied in terms of expression levels of myelin proteins (i.e., MBP, PLP, and the myelin and lymphocyte protein). Muscarine treatment of cultured OPCs and mature OLs decreased expression of MBP (De Angelis et al., 2011), although the drug’s effect on expression of other myelin proteins was equivocal, suggesting a specific role of muscarinic activation in MBP production and terminal differentiation of OLs (De Angelis et al., 2011). However, it should be kept in mind that MBP is a marker for mature myelinating and non-myelinating OLs but not OPCs. Given their ability to control glial cell proliferation, M1, M3 and M4 muscarinic receptor antagonists (Felder et al., 2000; Wess, 2004; Langmead et al., 2008; McArthur et al., 2010) might be useful in the treatment of brain tumors such as gliomas and oligodendrogliomas (Guizzetti et al., 1996; Loreti et al., 2007; Tata and Calogero, 2010).
Muscarinic drugs could also find utility in stem cell therapy, based on their capability to regulate myelin repair in demyelinating diseases such as MS (Goldman et al., 2012). Abiraman et al. (2015), using OPCs isolated from human fetal forebrain showed that the potent, non-selective muscarinic receptor agonist oxotremorine-M reduces OL differentiation. In contrast, the selective M3 antagonist darifenacin (Moriya et al., 1999) failed to influence OL differentiation, suggesting that M3 receptors are not expressed by human OPCs and/or that darifenacin does not act as an inverse agonist. However, when human OPCs were cultured with fetal human neuronal re-aggregates, ACh release from ACh+ neurons delayed OPC differentiation in a darifenacin-sensitive manner, suggesting that M3 antagonism could promote human OL differentiation (Abiraman et al., 2015). Further, solifenacin, a blood-brain barrier-permeable analog of darifenacin, promoted differentiation and myelination in neonatal mice transplanted with human OPCs (Maruyama et al., 2008). Collectively, these findings encourage the view that such pharmacological treatments could one day serve to induce resident OPC differentiation and improve outcome in demyelinating diseases such as MS, where OL differentiation is impaired (Franklin and Kotter, 2008; Franklin and ffrench-Constant, 2008).
Nitric Oxide
NO is a gaseous, cell-penetrant neurotransmitter. Under physiological conditions NO is present in the picomolar-nanomolar range in the CNS (Hall and Garthwaite, 2009), where it is generated by neuronal NO synthase (nNOS) in response to NMDA receptor-triggered rises in [Ca2+]i (Hall and Garthwaite, 2009). NO stimulates specialized guanylyl cyclase-coupled receptors to generate cyclic GMP (Garthwaite, 2008; Hardingham et al., 2013). Neurons and astrocytes are the principal targets of NO, although neuronally-derived NO may also act on the microvasculature to regulate blood flow (Yang et al., 2003). NO, superoxide, and hydrogen peroxide regulate MBP phosphorylation which contributes to neuron–oligodendrocyte signaling by promoting stability of the myelin sheath (Atkins and Sweatt, 1999).
The cerebellum is an important area for motor control and one in which NO-cyclic GMP signaling contributes to synaptic plasticity and other phenomena (Garthwaite, 2008; Contestabile, 2012). Myelination in the rat cerebellum begins in the central WM at around postnatal day 6 (P6) and proceeds along the axial core of the lobules to appear in the internal granule cell layer 4–6 days later (Gianola et al., 2003). The development of OL NO-reactivity between P3 and P8 would be consistent with its role in this process. In cortical cell cultures, NO and cyclic GMP enhance arborization of OLs. Compounds that increase NO activity promote OL growth and maturation (Garthwaite et al., 2015). Moreover, NO can coordinate axon myelination with neuronal activity during development and contribute to adaptive changes in adult myelination (Garthwaite et al., 2015). However, in OLs high NO concentrations can inhibit mitochondrial respiration and contribute to oxidative/nitrosative stress through peroxynitrite formation that induces uncontrolled MBP phosphorylation (Atkins and Sweatt, 1999), which may render the myelin sheet less stable. Therefore, anti-oxidant and mitochondria protective therapeutic strategies may be beneficial in MS, in particular in early stages of the disease (Lassmann, 2011).
Opioids
Neural stem cells (NSCs) and cells at different stages along the oligodendrolial lineage express opioid receptors, whose modulation can induce both mitogenesis and differentiation (Knapp et al., 1998; Persson et al., 2006; Eschenroeder et al., 2012). These cells are also capable of synthesizing opioids in a developmentally regulated manner (Knapp et al., 2001). Cultured bipolar progenitors produce dynorphin, whose expression is lost upon differentiation into mature OLs. In contrast, proenkephalin-derived peptides are found in OPCs and differentiated OLs (Knapp et al., 2001). Activation of mu opioid receptor (MOR) in cultured OPC stimulates DNA synthesis, whereas kappa opioid receptor inhibition increases membrane extensions (Knapp et al., 1998). Although these observations suggest that interference with the endogenous opioid system affects OL maturation and myelination in the developing CNS, further studies will be needed to fully resolve this question.
The growing use/abuse of opioids among younger persons (Compton and Volkow, 2006; Calcaterra et al., 2013) poses an especially high risk to pregnant addicts (in whom drug effects could target early myelination in the fetus and newborn) and adolescents and young adults (in whom late myelination of cortical regions takes place). Prolonged administration of morphine to neonatal rats is associated with increased neuronal cell apoptosis in selective CNS areas (Bajic et al., 2013) and leads to increased Toll-like receptor-4 and microglial activation in adolescent rats (Schwarz and Bilbo, 2013). Heroin and morphine abusers can suffer from leukoencephalopathy and myelin loss (Chen et al., 2000; Nanan et al., 2000). Perinatal exposure to therapeutic doses of methadone and buprenorphine (used to treat opioid addiction) altered early myelination in the developing rat brain (Eschenroeder et al., 2012; Vestal-Laborde et al., 2014), with elevated brain levels of MBP, PLP, and myelin-oligodendrocyte glycoprotein. Not surprisingly, these animals showed an increased number of axons with a highly compacted myelin membrane. There was no parallel reduction in the number of axons with a still uncompacted myelin membrane, pointing to the existence of complex drug effects. Methadone exerts direct effects at specific stages of the OL lineage in vitro, stimulating the proliferation of OPCs while accelerating maturation of more differentiated but still immature preOLs (Vestal-Laborde et al., 2014). While the long-term effects of these observations remain unknown, accelerated or increased OL maturation and myelination could disrupt the complex sequence of synchronized events leading to normal connectivity in the developing brain. These findings further point to a crucial function of the endogenous opioid system in controlling OL development and the timing of myelination, as well as the potential for disrupting brain maturation at critical stages of myelin formation.
The partial MOR agonist and kappa opioid receptor antagonist buprenorphine merits special comment. While treatment of mothers with therapeutic doses of methadone results in accelerated and increased brain expression of mature markers of OLs in pups, supra-therapeutic doses delayed MBP expression and decreased the number of myelinated axons (Eschenroeder et al., 2012). Buprenorphine anti-nociceptive action also showed a biphasic bell-shaped curve, with low doses being analgesic and higher doses loosing efficacy (Dum and Herz, 1981; Lizasoain et al., 1991). Buprenorphine anti-nociceptive effects are mediated by MOR and counteracted by nociceptin (NOP) receptors (Lutfy et al., 2003). In the latter study J-113397, a specific NOP receptor inhibitor, not only enhanced the anti-nociceptive effect of buprenorphine but also eliminated its bell-shaped response. This biphasic response was not seen in NOP receptor knockout mice (Lutfy et al., 2003). In OL cultures J-113397 abolished the biphasic dose-dependent effect of buprenorphine on MBP expression (Eschenroeder et al., 2012). The latter findings point to a crucial role for interactive MOR and NOP receptor-mediated signaling in the timing of OL maturation. Perinatal exposure to buprenorphine increases the caliber of myelinated axons and with disproportionally thinner myelin sheaths, possibly indicative of alterations in axon-oligodendroglia/myelin interaction (Sanchez et al., 2008). Exposure of rat pups to the MOR agonist methadone did not cause these effects (Vestal-Laborde et al., 2014).
Prostaglandins
Cyclooxygenase (COX) catalyzes the rate-limiting step in the synthesis of prostanoids from arachidonic acid (Smith et al., 1996), and occurs as both constitutive (COX-1) and inducible (COX-2) forms (Hewett et al., 2000). COX-2 expression increases in CNS neurons in response to GluR activation (Nogawa et al., 1997; Carlson, 2003), and its inhibition by non-steroidal anti-inflammatory drugs and non-selective COX-1/COX-2 inhibitors protects neurons from GluR-mediated excitotoxic death in vitro (Hewett et al., 2000; Carlson, 2003) and in vivo (Nogawa et al., 1997). Transgenic mice over-expressing neuronal COX-2 are more susceptible to excitotoxicity (Kelley et al., 1999) and age-associated neuronal cell loss (Andreasson et al., 2001). In contrast, COX-2-deficient mice are more resistant to neuronal cell death caused by ischemia or NMDA receptor activation (Iadecola et al., 2001).
OLs and OPCs are more susceptible to excitotoxic death following GluR-dependent COX-2 induction (Carlson et al., 2010). COX-2 is expressed in dying OLs at the onset of demyelination in Theiler’s murine encephalomyelitis virus model of MS (Carlson et al., 2006) and in dying OLs in MS lesions (Carlson et al., 2010). In the former case COX-2 inhibitors reduced demyelination (Carlson et al., 2010). COX-2 contributes to OL vulnerability also in the cuprizone model of demyelination (Palumbo et al., 2012). COX-2 may thus have an important role in demyelinating diseases like MS.
Prostanoids can contribute to excitotoxic death in OPCs either via direct production by OPCs or activation of prostanoid receptors on OPCs. Prostaglandin E2 (PGE2) synthesized by OPCs in a GluR-dependent fashion engages EP3 subtype receptors and contributes to excitotoxic death of OPCs (Carlson, 2003). In contrast, the EP2 receptor is protective (Carlson, 2003). Although EP1 receptor activation does not affect an OPC excitotoxic challenge (Carlson et al., 2015), PGI2 can promote OPC migration and remyelination in damaged areas (Takahashi et al., 2013). During myelination in developing rat brain, EP3 and thromboxane A2 receptor are localized to the plasma membrane and perinuclear membrane of OPCs, and their expression levels—as well as thromboxane A2 synthesis—increase during OLs maturation in concert with enhanced MBP expression (Carlson et al., 2010).
Given the role of EP3 receptors in OPC excitotoxic death, this receptor may be a potential therapeutic target for promoting remyelination in demyelinating diseases such as MS.
Prolactin
PRL, originally described in the anterior pituitary gland plays a role not only in reproduction, but also controls a variety of behaviors and may participate in cell homeostasis, as well (Kelly et al., 1991; Freeman et al., 2000). Initially described in the brain in hypothalamic axon terminals (Fuxe et al., 1977), PRL immunoreactivity was later detected in telencephalon, cerebral cortex, hippocampus, amygdala, septum (Devito, 1988), caudate putamen (Harlan et al., 1989), brain stem (Devito, 1988; Harlan et al., 1989), cerebellum (Seroogy et al., 1988), spinal cord (Harlan et al., 1989; Siaud et al., 1989), choroid plexi, and circumventricular organs (Thompson, 1982). The PRL receptor belongs to class 1 of the cytokine receptor superfamily (Bazan, 1990a,b) whose gene shows widespread CNS expression.
PRL increases neurogenesis in the maternal mouse forebrain during the first week of pregnancy, along with an increase in OPC proliferation, OL generation, MBP expression and ultimately, increased numbers of myelinated axons (Gregg et al., 2007). PRL infused subcutaneously can mimic these effects (Gregg et al., 2007). These observations suggest a novel form of WM plasticity in the adult CNS and propose that PRL may have therapeutic potential in treating WM damage. At the same time, several reports suggest that PRL can exert also an inflammatory role. In an animal model of MS, the D2 agonists bromocriptine and dihydroergocryptine reduced the PRL level and disease severity (Riskind et al., 1991; Canonico et al., 1993; Zhornitsky et al., 2015). Although PRL or PRL receptor knockout mice develop a delayed onset experimental autoimmune encephalomyelitis but with full clinical severity (Costanza et al., 2013) a small clinical trial failed to demonstrate benefit of bromocriptine administration in MS patients (Bissay et al., 1994). Thus, the therapeutic utility of PRL in MS remains an open question; it would be interesting to perform a trial combining PRL with an immunomodulator (i.e., interferon-β commonly used in clinical practice; Zhornitsky et al., 2015).
Cannabinoids
Cannabidiol (CBD), the most abundant cannabinoid in Cannabis sativa, is devoid of psychoactive properties and exerts anti-inflammatory, antioxidant and neuroprotective effects (Izzo et al., 2009). CBD is used for the treatment of inflammation, pain and spasticity associated with MS (Sastre-Garriga et al., 2011). Its biological effects are mediated through cannabinoid receptors CB1 and CB2 (Howlett et al., 2002). The former is widely expressed in CNS neurons, where it modulates neurotransmitter release and synaptic plasticity regulated by Ca2+ and K+ channels (Chevaleyre et al., 2006; Basavarajappa, 2007). CB2 receptor expression is mainly, but not exclusively limited to immune cells (Walter and Stella, 2004). OPCs and mature OLs both expess CB1and CB2 receptors (Molina-Holgado et al., 2003; Benito et al., 2007).
In rat optic nerve OLs, the CB1 receptor agonist arachidonoyl-chloro-ethanolamide inhibited depolarization-induced Ca2+ influx (Mato et al., 2009). Similar effects were obtained with the endocannabinoids anandamide and 2-arachidonoylglycerol and the CB1 receptor agonist CP 55,940, but not the CB2 receptor agonist JWH133. In line with these findings CBD, which acts also as a low-affinity CB1/CB2 receptor antagonist raised [Ca2+]i concentration in OL primary cultures (Mato et al., 2009, 2010) and was cytotoxic (Mato et al., 2010). However, these [Ca2+]i changes appeared to be CB receptor-independent (Mato et al., 2010). Given its lipophilic properties, it is possible that CBD can directly affect [Ca2+]i stores. Not only are mitochondria the main source of Ca2+ released from CBD-stimulated OLs (Mato et al., 2010), but CB1 receptors have been identified on brain mitochondrial membranes (Hebert-Chatelain et al., 2014). In contrast, in neurons CBD reduced [Ca2+]i influx and prevented apoptosis (Ryan et al., 2009). The patho-physiological consequences of CBD action may thus depend on the cellular context in which it is expressed.
Nuclear Receptors
NRs are members of a large superfamily of evolutionarily related transcription factors that regulate a broad spectrum of physiological phenomena (Gronemeyer et al., 2004; Chambon, 2005; Evans, 2005). Each of the 48 NRs identified to date has crucial and non-redundant roles, notably in biologically important processes like growth, development, and homeostasis. NRs modulate transcription through mechanisms which include both activation and repression (Germain et al., 2006b). The NR superfamily includes receptors for hydrophobic molecules such as steroid hormones (e.g., estrogens, glucocorticoids (GCs), progesterone, mineralocorticoids, androgens, vitamin D3, ecdysone, oxysterols and bile acids), retinoic acids, TH, fatty acids, leukotrienes and prostaglandins (Laudet and Gronemeyer, 2002).
Steroid Hormone Receptors
GCs are steroid hormones with recognized anti-inflammatory properties. In the brain, two types of high-affinity receptors bind GCs: the type I mineralocorticoid receptor (MR) and the type II glucocorticoid receptor (GR; Vielkind et al., 1990). Each receptor dimerizes with a GC-responsive element leading to suppression of pro-inflammatory gene expression via block of nuclear factor-kB or activator protein-1 (Trousson et al., 2009). Both receptor types are widely expressed across neuronal cell populations and glia, although the latter finding awaits confirmation. Purified OLs and astrocytes from rat cerebrum and cerebellum were shown to express differential levels of GR by immunocytochemistry (Vielkind et al., 1990). At least in vitro, all classes of glial cells express GRs that can translocate to the nucleus in the presence of the cognate hormone ligand (Vielkind et al., 1990).
Dexamethasone (DEX) alters OLs differentiation and myelination in a developmental stage-specific manner. In rat forebrain cell cultures DEX stimulated the early stages of myelination but caused a markedly inhibition at later stages (Almazan et al., 1986). When given systemically to 3-day-old rats for 7 consecutive days DEX significantly decreased the relative abundance of MBP and PLP mRNAs at P20 and P30 in cerebrum, although in cerebellum MBP, PLP and glial fibrillary acidic protein mRNAs decreased at P10 (Tsuneishi et al., 1991). DEX thus appears to suppress expression of genes related to glial functions, especially myelination, when administered in the early postnatal period (Tsuneishi et al., 1991), notwithstanding differences in susceptibility across brain areas.
Sex Hormones
Sexual dimorphism in the brain vocal control area of the songbird was first demonstrated four decades ago (Nottebohm and Arnold, 1976). Magnetic resonance imaging shows morphological differences in human cortical gray matter volume (Gur et al., 1999) with females having larger volumes in areas associated with language functions (Schlaepfer et al., 1995; Harasty et al., 1997). In terms of WM differences between sexes, men have larger overall WM relative to cerebral size (Goldstein et al., 2001), while women appear to have larger WM volumes involved in interhemisphere connectivity (Nopoulos et al., 2000). Sexual dimorphism in rats was interpreted as a consequence of the action of different sex hormones on brain development (Cerghet et al., 2009).
We know relatively little concerning sexual dimorphism of OLs and central myelin. WM volume (Gur et al., 1999), especially in the corpus callosum (Fitch et al., 1990) is increased in males, with females having more unmyelinated fibers. Yang et al. (2008) later confirmed that WM volume, myelinated fiber volume and volume of myelin sheaths in young rats are significantly larger in males, although this ratio was reversed in middle-aged animals. Differences in steroid hormone levels in developing animals could account for sex differences in myelination of axons through hormone action on myelin protein expression (Jung-Testas and Baulieu, 1998; Nuñez et al., 2000). It is also possible that sex steroids could exert a protective effect on myelin in developing, as well as injured WM (De Nicola et al., 2006; Gerstner et al., 2007; Garay et al., 2008).
Sexual differences in rodents are also manifested in terms of OL myelin protein expression (Cerghet et al., 2009). Female rodent OLs showed increased proliferation and death rate, suggesting that their OLs have a shorter lifespan and more rapid turnover. In male mice, castration increased glial cell proliferation and decreased OL density (Cerghet et al., 2009). Sex hormones, in particular testosterone, influence proliferation and are potent inducers of remyelination (Hussain et al., 2013). In enriched mouse OLs primary cultures, progesterone (P2), estrogen (E2) and dihydrotestosterone (DHT) influenced total cell number, proliferation and cell death independent of sex. In particular, P2 significantly increased OL numbers (more so in cultures from female mice), while E2 had a minor effect and DHT reduced OL numbers in both sexes (Cerghet et al., 2009).
Protein kinase B (Akt), MAPK and mammalian target of rapamycin (mTOR) pathways are highly expressed in OLs (Flores et al., 2000; Horiuchi et al., 2006) and represent, in relation to cell survival/proliferation, possible biochemical targets for these hormones (Hay, 2005; Chong et al., 2007). P2 caused a more robust Akt up-regulation in OLs cultured from female mice in comparison to male-derived cells, in line with effects of P2 on OL numbers. Akt influences cell survival and proliferation through downstream mTOR activation via transcriptional regulation (Hay, 2005; Chong et al., 2007). P2 markedly increased mTOR activity in an Akt-dependent manner, indicating a role for this pathway in OL survival. As expected from its effects on OL numbers, DHT reduced Akt activity in both sexes (Cerghet et al., 2009). Interestingly, MAPK p42/p44 activation is involved in excitotoxic cell death, mediated by Glu and pro-inflammatory processes and leading to neurodegenerative diseases (Rosin et al., 2004; Nikodemova et al., 2006). It is tempting to speculate that by influencing proliferation and death of Ols, sex hormones may contribute to differences in disease phenotype between male and female MS patients.
Oxysterols and Liver X Receptors
The liver X receptor (LXR) is a member of the NR family of transcription factors (Janowski et al., 1996) and is closely related to NRs such as the peroxisome proliferator-activated receptor (PPAR), farnesoid X receptor and retinoid X receptor (RXR). Both LXR isoforms (α and β) are activated by oxysterols originating from cholesterol oxidation (Freemantle et al., 2013). OLs express LXR α and β which are crucial for cholesterol homeostasis, a major lipid constituent of myelin sheaths. LXRs are involved in myelination and remyelination processes. LXRα/β double knockout mice exhibit altered motor coordination and spatial learning, thinner myelin sheaths, and reduced myelin gene expression in cerebellum. Conversely, activation of LXRs by either 25-hydroxycholesterol or the agonist TO901317 stimulates myelin gene expression at the promoter, mRNA, and protein (PLP and MBP) levels. LXR activation also promotes OLs cell maturation and remyelination after lysolecithin-induced demyelination in organotypic cerebellar slice cultures. These data strongly support a new role for LXRs as positive modulators in central (re)myelination processes (Meffre et al., 2015).
1,25-Dihydroxyvitamin D3
1,25-Dihydroxyvitamin D3 (1,25(OH)2D3) was initially described to suppress experimental autoimmune encephalomyelitis (Lemire and Archer, 1991; Spach et al., 2006) through an anti-inflammatory mechanism. New data show that 1,25(OH)2D3 can directly promote neural stem cell (NSC) proliferation, survival and differentiation along the neuron and OL, but not astrocyte, lineages. NSCs constitutively express vitamin D receptor, which can be up-regulated by 1,25(OH)2D3. NSCs treated with 1,25(OH)2D3 showed increased expression of neurotrophic factors subserving neuronal cell survival and differentiation (Shirazi et al., 2015).
Retinoic Acid and Retinoid X Receptor
The RXR (Prineas and Connell, 1979) is activated by 9-cis retinoic acid (Patani et al., 2007), as well as 9-cis-13,14-dihydro-retinoic acid (Raine and Wu, 1993) and comprises three isoforms: RXR-α, -β and -γ. RXRs work through heterodimeric association with other NRs, such as retinoic acid receptors, TH receptors, vitamin D receptors, PPARs and LXRs to regulate cell proliferation, differentiation and apoptosis (Germain et al., 2006a). RXR-γ is generally expressed at low levels in all glial cell populations (Moreno et al., 2004) but is up-regulated in activated microglia and macrophages, reactive astrocytes and OLs after CNS injury (Schrage et al., 2006). RXR signaling is associated with the CNS regenerative response and is involved in the remyelination transcriptome (Huang et al., 2011). The remyelination environment is composed of demyelinated axons, activated adult OPCs, regenerated OLs, microglia and/or macrophages and reactive astrocytes (Franklin and ffrench-Constant, 2008). Functional analyses in cultured OPCs using RNA interference to knock down RXR-γ gene expression, RXR-specific antagonists, as well as RXR-γ null mice showed inefficient OL differentiation, indicating that RXR-γ is an important regulator of remyelination (Huang et al., 2011). The ability of RXR to form permissive or non-permissive heterodimers with other NRs suggests that RXR can modulate the expression of different genes, in a receptor- and context-specific fashion (Altucci et al., 2007). Identification of which nuclear receptor(s) heterodimerize with RXR-γ in OL lineage cells after demyelination, and what genes are transcribed in response to RXR-γ activation to promote the differentiation of OPCs have remained open questions until now. However, a new study by de la Fuente et al. (2015) provides data indicating that RXR-γ binds to several NRs, including the vitamin D receptor, in OPCs and mature OLs. These authors showed that inhibition of vitamin D receptor signaling impaired OPC differentiation and reduced the cells’ ability to remyelinate axons ex vivo. Vitamin D, in contrast, boosted OPC differentiation (de la Fuente et al., 2015).
Thyroid Hormone
TH actions are mediated mainly by T3 (Yen, 2001) which derives by conversion of T4 to T3 via deiodinases. THs activate two nuclear hormone receptor genes. The THR-α gene encodes two isoforms (α1 and α2) while four additional isoforms are encoded by the β gene (β1–4; Williams, 2000; Yen, 2001; Tagami et al., 2010). THs play a critical role in oligodendrogenesis and myelination. Acting through their cognate receptors, THs promote expression of OL-specific genes including MBP, myelin-associated glycoprotein (MAG), PLP, and 2′,3′-cyclic nucleotide 3′-phosphodiesterase (CNPase) (Farsetti et al., 1991; Tosic et al., 1992; Rodriguez-Pena et al., 1993). Studies using hypo- and hyperthyroid animals as well as genetically modified rodents clearly show that THs regulate OL differentiation and maturation (Bernal, 2002; O’Shea and Williams, 2002; Zoeller and Rovet, 2004; Dugas et al., 2012). In vitro, OPCs express both THR-α and THR-β1; expression of the latter increases with OL maturation (Barres et al., 1994; Gao et al., 1998). GC-1, a thyromimetic with selective THR-β action (Chiellini et al., 1998), promotes differentiation of both rodent and human OPCs in vitro (Trost et al., 2000; Manzano et al., 2003). Furthermore, GC-1 induces OL maturation during development with increased production of MBP, CNPase and MAG (Baxi et al., 2014).
OL differentiation can follow several distinct pathways, including mitogen withdrawal and exposure to T3 (Barres et al., 1993; Dugas et al., 2010), whereas OPC proliferation is induced by PDGF (Barres et al., 1992). When exposed to T3 and PDGF, OPCs continue to proliferate, differentiating only after multiple rounds of cell division (Temple and Raff, 1986; Barres et al., 1994; Durand and Raff, 2000). Like T3, GC-1 does not significantly affect PDGF-induced OPC proliferation. Inappropriate OL differentiation could thus deplete OPC numbers and hinder myelination (Baxi et al., 2014).
PPAR-γ and 15-deoxy 12,14 Prostaglandin J2
The three known PPARs (PPAR-α, PPAR-β/δ and PPAR-γ) belong to the superfamily of NRs. While ubiquitously expressed, they also exhibit specific patterns of distribution. PPAR-γ agonists exert anti-inflammatory, insulin-sensitizing and neuroprotective effects, as well as promote the protection and differentiation of OLs (Bernardo et al., 2009). In addition, PPAR-γ agonists increase OL mitochondrial respiratory chain activity and the cell’s ability to respond to environmental signals with oscillatory Ca2+ waves. Both OL maturation and oscillatory Ca2+ waves are prevented by the mitochondrial inhibitor rotenone and restored by PPAR-γ agonists, suggesting that PPAR-γ promotes myelination through mechanisms involving mitochondria (Bernardo et al., 2009, 2013). Calcium signaling plays an important role in OPC differentiation and myelination (Soliven, 2001), process extension and migration (Simpson and Armstrong, 1999; Yoo et al., 1999), and in retraction of membrane sheets and cell death in mature mouse OLs (Benjamins and Nedelkoska, 1996).
Conclusion and Perspectives
The main functions of OLs in CNS are to provide support and insulation to axons of some vertebrates, forming functional units that allow the propagation of electrical signals. On the other hand, neuronal activity regulates myelinating cell development through signals that activate Ca2+-dependent pathways (Butt, 2006). As discussed in this review, activation is evoked by a wide range of membrane/intracellular receptors or ion channels (for the latter, see Cheli et al., 2015) that can induce an influx or release from intracellular stores, increasing [Ca2+]i. To restore homeostasis, [Ca2+]i can be sequestered into endoplasmic reticulum or extruded (Butt, 2006). The balance between Ca2+ influx and efflux can determine the temporal and special properties of OLs. Moreover, Ca2+ signaling in OPCs can influence not only differentiation and myelination (Soliven, 2001), but also process extension and migration (Simpson and Armstrong, 1999; Yoo et al., 1999), and cell death in mature mouse OLs (Benjamins and Nedelkoska, 1996). There is also evidence that oligodendroglia exhibit Ca2+ transients in response to electrical activity of axons for activity-dependent myelination (Wake et al., 2015).
Failure of myelin development and oligodendrocyte loss results in serious human disorders, including MS. Understanding how different neurotransmitters and hormones can regulate [Ca2+]i, driving important effects on different stages of OPCs development and specific molecular events could provide the framework to facilitate the development of effective new approaches in the therapeutic repair of myelin disorders that complement established immunosuppressive approaches. In this regard the landscape is beginning to show the fruits of these investigations (Mullard, 2014; Ransohoff et al., 2015). For example, several U.S. Food and Drug Administration-approved drugs have shown promise in this direction, although most are anticholinergics. Whether or not the other possible pharmacological pathways are ineffective or have not been tried in not known. The antidyskinetic drug benztropine, a selective M1 muscarinic acetylcholine receptor antagonist, induced MBP expression in primary rat optic-nerve-derived OPCs and decreased clinical severity in an experimental autoimmune encephalomyelitis model of relapsing-remitting MS when administered alone or in combination with the approved immunosuppressive MS drugs FTY720 or interferon-β (Deshmukh et al., 2013). However, potential dose-dependent side-effects are associated with benztropine treatment in man (Modell et al., 1989). Systemic treatment with the selective M3 antagonist solifenacin increased oligodendrocyte differentiation of transplanted human OPCs in hypomyelinated shiverer/rag2 brain and enhanced functional repair (Abiraman et al., 2015). Donepezil, an acetylcholinesterase inhibitor developed for the treatment of Alzheimer’s disease, can stimulate oligodendrocyte differentiation and maturation of NSC-derived OPCs (Imamura et al., 2015). Importantly, neural precursor cells have recently been demonstrated to make a major contribution to remyelination in the cuprizone model of CNS demyelination (Xing et al., 2014). Although some of the reviewed pathways are involved in apoptosis (e.g., opioids, acetylcholine), the PPAR-α agonist palmitoylethanolamide (a fatty acid amide signaling molecule structurally related to the endocannabinoid anandamide), presented as a co-ultramicronized composite with the flavonoid luteolin is also reported to promote OPC maturation (Barbierato et al., 2015). Neither palmitoylethanolamide (Skaper et al., 2014) not luteolin (Theoharides et al., 2012), at pharmacologically relevant doses, has been reported to show adverse effects in man. While we have focused on MS as an emblematic pathological condition that could eventually benefit from OL-based repair therapies for myelin loss, preventive treatment of premature infants with drugs that accelerate the proliferation and/or maturation of OPCs could reveal itself as the key to prevent an important cause of mental retardation. No doubt the initial results discussed in this review will encourage further progress in the years ahead.
Search Strategy
A search for original articles focusing on OLs and neurotransmitters and signaling was performed in PubMed and SCOPUS. The search terms used were “oligodendrocyte”, “myelin”, “myelination”, “oligodendrocyte precursor”, “nuclear receptor”, “opioid”, “glutamate”, “GABA”, “serotonin”, “cholinergic”, “muscarinic”, “cannabinoid”, “demyelination”, “autoimmune”, alone and in combination (other terms?). All articles identified were English language (with the exception of Virchow, R. 1854, originally written in German), full-text articles. We also searched the reference lists of identified articles for additional relevant articles.
Author Contributions
PG and CM conceived the study, and PG, CM, TB, MZ and SDS participated in drafting the manuscript. All authors critically revised and approved the final manuscript.
Conflict of Interest Statement
The authors declare that the research was conducted in the absence of any commercial or financial relationships that could be construed as a potential conflict of interest.
References
Abiraman, K., Pol, S. U., O’Bara, M. A., Chen, G. D., Khaku, Z. M., Wang, J., et al. (2015). Anti-muscarinic adjunct therapy accelerates functional human oligodendrocyte repair. J. Neurosci. 35, 3676–3688. doi: 10.1523/JNEUROSCI.3510-14.2015
Agresti, C., Meomartini, M. E., Amadio, S., Ambrosini, E., Volonté, C., Aloisi, F., et al. (2005). Metabotropic P2 receptor activation regulates oligodendrocyte progenitor migration and development. Glia 50, 132–144. doi: 10.1002/glia.20160
Alberdi, E., Sánchez-Gómez, M. V., and Matute, C. (2005). Calcium and glial cell death. Cell Calcium 38, 417–425. doi: 10.1016/j.ceca.2005.06.020
Alberdi, E., Sánchez-Gómez, M. V., Torre, I., Domercq, M., Pérez-Samartín, A., Pérez-Cerdá, F., et al. (2006). Activation of kainate receptors sensitizes oligodendrocytes to complement attack. J. Neurosci. 26, 3220–3228. doi: 10.1523/jneurosci.3780-05.2006
Almazan, G., Honegger, P., Du Pasquier, P., and Matthieu, J. M. (1986). Dexamethasone stimulates the biochemical differentiation of fetal forebrain cells in reaggregating cultures. Dev. Neurosci. 8, 14–23. doi: 10.1159/000112237
Altucci, L., Leibowitz, M. D., Ogilvie, K. M., de Lera, A. R., and Gronemeyer, H. (2007). RAR and RXR modulation in cancer and metabolic disease. Nat. Rev. Drug Discov. 6, 793–810. doi: 10.1038/nrd2397
Andreasson, K. I., Savonenko, A., Vidensky, S., Goellner, J. J., Zhang, Y., and Shaffer, A. (2001). Age dependent cognitive deficits and neuronal apoptosis in cyclooxygenase-2 transgenic mice. J. Neurosci. 21, 8198–8209.
Armstrong, R. C., Harvath, L., and Dubois-Dalcq, M. E. (1990). Type 1 astrocytes and oligodendrocyte-type2 astrocyte glial progenitors migrate toward distinct molecole. J. Neurosci. Res. 27, 400–407. doi: 10.1002/jnr.490270319
Arnett, H. A., Fancy, S. P., Alberta, J. A., Zhao, C., Plant, S. R., Kaing, S., et al. (2004). bHLH transcription factor Olig1 is required to repair demyelinated lesions in the CNS. Science 306, 2111–2115. doi: 10.1126/science.1103709
Asotra, K., and Macklin, W. B. (1993). Protein kinase C activity modulates myelin gene expression in enriched oligodendrocytes. J. Neurosci. Res. 34, 571–588. doi: 10.1002/jnr.490340509
Aston, C., Jiang, L., and Sokolov, B. P. (2005). Transcriptional profiling reveals evidence for signaling and oligodendroglial abnormalities in the temporal cortex from patients with major depressive disorder. Mol. Psychiatry 10, 309–322. doi: 10.1038/sj.mp.4001565
Atkins, C. M., and Sweatt, J. D. (1999). Reactive oxygen species mediate activity- dependent neuron-glia signaling in output fibers of the hippocampus. J. Neurosci. 19, 7241–7248.
Avenell, K. Y., Boyfield, I., Coldwell, M. C., Hadley, M. S., Healy, M. A., Jeffrey, P. M., et al. (1998). Fused aminotetralins: novel antagonists with high selectivity for the dopamine D3 receptor. Bioorg. Med. Chem. Lett. 8, 2859–2864. doi: 10.1016/s0960-894x(98)00512-5
Avenell, K. Y., Boyfield, I., Hadley, M. S., Johnson, C. N., Nash, D. J., Riley, G. J., et al. (1999). Heterocyclic analogues of 2-aminotetralins with high affinity and selectivity for the dopamine D3 receptor. Bioorg. Med. Chem. Lett. 9, 2715–2720. doi: 10.1016/s0960-894x(99)00454-0
Back, S. A., Riddle, A., and McClure, M. M. (2007). Maturation-dependent vulnerability of perinatal white matter in premature birth. Stroke 38, 724–730. doi: 10.1161/01.str.0000254729.27386.05
Bajic, D., Commons, K. G., and Soriano, S. G. (2013). Morphine-enhanced apoptosis in selective brain regions of neonatal rats. Int. J. Dev. Neurosci. 31, 258–266. doi: 10.1016/j.ijdevneu.2013.02.009
Bakiri, Y., Burzomato, V., and Frugier, G. (2009). Glutamatergic signaling in the brain’s white matter. Neuroscience 158, 266–274. doi: 10.1016/j.neuroscience.2008.01.015
Ballerini, P., Di Iorio, P., Ciccarelli, R., Nargi, E., D’Alimonte, I., Traversa, U., et al. (2002). Glial cells express multiple ATP binding cassette proteins which are involved in ATP release. Neuroreport 13, 1789–1792. doi: 10.1097/00001756-200210070-00019
Barbierato, M., Facci, L., Marinelli, C., Zusso, M., Argentini, C., Skaper, S. D., et al. (2015). Co-ultramicronized palmitoylethanolamide/luteolin promotes the maturation of oligodendrocyte precursor cells. Sci. Rep. 5:16676. doi: 10.1038/srep16676
Baron, W., de Jonge, J. C., de Vries, H., and Hoekstra, D. (1998). Regulation of oligodendrocyte differentiation: protein kinase C activation prevents differentiation of O2A progenitor cells toward oligodendrocytes. Glia 22, 121–129. doi: 10.1002/(sici)1098-1136(199802)22:2<121::aid-glia3>3.0.co;2-a
Barres, B. A., Hart, I. K., Coles, H. S., Burne, J. F., Voyvodic, J. T., Richardson, W. D., et al. (1992). Cell death and control of cell survival in the OL lineage. Cell 70, 31–46. doi: 10.1016/0092-8674(92)90531-g
Barres, B. A., Lazar, M. A., and Raff, M. C. (1994). A novel role for thyroid hormone, glucocorticoids and retinoic acid in timing oligodendrocyte development. Development 120, 1097–1108.
Barres, B. A., Schmid, R., Sendnter, M., and Raff, M. C. (1993). Multiple extracellular signals are required for long-term oligodendrocyte survival. Development 118, 283–295.
Basavarajappa, B. S. (2007). Neuropharmacology of the endocannabinoid signaling system-molecular mechanisms, biological actions and synaptic plasticity. Curr. Neuropharmacol. 5, 81–97. doi: 10.2174/157015907780866910
Baxi, E. G., Schott, J. T., Fairchild, A. N., Kirby, L. A., Karani, R., Uapinyoying, P., et al. (2014). A selective thyroid hormone β receptor agonist enhances human and rodent oligodendrocyte differentiation. Glia 62, 1513–1529. doi: 10.1002/glia.22697
Bazan, J. F. (1990a). Haemopoietic receptors and helical cytokines. Immunol. Today 11, 350–354. doi: 10.1016/0167-5699(90)90139-z
Bazan, J. F. (1990b). Structural design and molecular evolution of a cytokine receptor superfamily. Proc. Natl. Acad. Sci. U S A 87, 6934–6938. doi: 10.1073/pnas.87.18.6934
Benito, C., Romero, J. P., Tolón, R. M., Clemente, D., Docagne, F., Hillard, C. J., et al. (2007). Cannabinoid CB1 and CB2 receptors and fatty acid amide hydrolase are specific markers of plaque cell subtypes in human multiple sclerosis. J. Neurosci. 27, 2396–2402. doi: 10.1523/jneurosci.4814-06.2007
Benjamins, J. A., and Nedelkoska, L. (1996). Release of intracellular calcium stores leads to retraction of membrane sheets and cell death in mature mouse oligodendrocytes. Neurochem. Res. 21, 471–479. doi: 10.1007/bf02527712
Bernal, J. (2002). Action of thyroid hormone in brain. J. Endocrinol. Invest. 25, 268–288. doi: 10.1007/BF03344003
Bernardo, A., Bianchi, D., Magnaghi, V., and Minghetti, L. (2009). Peroxisome proliferator-activated receptor-gamma agonists promote differentiation and antioxidant defenses of oligodendrocyte progenitor cells. J. Neuropathol. Exp. Neurol. 68, 797–808. doi: 10.1097/NEN.0b013e3181aba2c1
Bernardo, A., De Simone, R., De Nuccio, C., Visentin, S., and Minghetti, L. (2013). The nuclear receptor peroxisome proliferator-activated receptor-γ promotes OL differentiation through mechanisms involving mitochondria and oscillatory Ca2+ waves. Biol. Chem. 394, 1607–1614. doi: 10.1515/hsz-2013-0152
Bissay, V., De Klippel, N., Herroelen, L., Schmedding, E., Buisseret, T., Ebinger, G., et al. (1994). Bromocriptine therapy in multiple sclerosis: an open label pilot study. Clin. Neuropharmacol. 17, 473–476. doi: 10.1097/00002826-199410000-00011
Bongarzone, E. R., Howard, S. G., Schonmann, V., and Campagnoni, A. T. (1998). Identification of the dopamine D3 receptor in oligodendrocyte precursors: potential role in regulating differentiation and myelin formation. J. Neurosci. 18, 5344–5353.
Brazel, C. Y., Romanko, M. J., Rothstein, R. P., and Levison, S. W. (2003). Roles of the mammalian subventricular zone in brain development. Prog. Neurobiol. 69, 49–69. doi: 10.1016/s0301-0082(03)00002-9
Butt, A. M. (2006). Neurotransmitter-mediated calcium signalling in oligodendrocyte physiology and pathology. Glia 54, 666–675. doi: 10.1002/glia.20424
Calcaterra, S., Glanz, J., and Binswanger, I. A. (2013). National trends in pharmaceutical opioid related overdose deaths compared to other substance related overdose deaths: 1999–2009. Drug Alcohol Depend. 131, 263–270. doi: 10.1016/j.drugalcdep.2012.11.018
Canonico, P. L., Sortino, M. A., Favit, A., Aleppo, G., and Scapagnini, U. (1993). Dihydroergocryptine protects from acute experimental allergic encephalomyelitis in the rat. Funct. Neurol. 8, 183–188.
Carlson, N. G. (2003). Neuroprotection of cultured cortical neurons mediated by the cyclooxygenase-2 inhibitor APHS can be reversed by a prostanoid. J. Neurosci. Res. 71, 79–88. doi: 10.1002/jnr.10465
Carlson, N. G., Bellamkonda, S., Schmidt, L., Redd, J., Huecksteadt, T., Weber, L. M., et al. (2015). The role of the prostaglandin E2 receptors in vulnerability of OL precursor cells to death. J. Neuroinflammation 12:101. doi: 10.1186/s12974-015-0323-7
Carlson, N. G., Hill, K. E., Tsunoda, I., Fujinami, R. S., and Rose, J. W. (2006). The pathologic role for COX-2 in apoptotic oligodendrocytes in virus induced demyelinating disease: implications for multiple sclerosis. J. Neuroimmunol. 174, 21–31. doi: 10.1016/j.jneuroim.2006.01.008
Carlson, N. G., Rojas, M. A., Redd, J. W., Tang, P., Wood, B., and Hill, K. E. (2010). Cyclooxygenase-2 expression in oligodendrocytes increases sensitivity to excitotoxic death. J. Neuroinflammation 7:25. doi: 10.1186/1742-2094-7-25
Cases, O., Vitalis, T., Seif, I., De Maeyer, E., Sotelo, C., and Gaspar, P. (1996). Lack of barrels in the somatosensory cortex of monoamine oxidase A-deficient mice: role of a serotonin excess during the critical period. Neuron 16, 297–307. doi: 10.1016/s0896-6273(00)80048-3
Cavaliere, F., Urra, O., Alberdi, E., and Matute, C. (2012). Oligodendrocyte differentiation from adult multipotent stem cells is modulated by glutamate. Cell Death Dis. 3:e268. doi: 10.1038/cddis.2011.144
Cerghet, M., Skoff, R. P., Swamydas, M., and Bessert, D. (2009). Sexual dimorphism in the white matter of rodents. J. Neurol. Sci. 286, 76–80. doi: 10.1016/j.jns.2009.06.039
Chambon, P. (2005). The nuclear receptor superfamily: a personal retrospect on the first two decades. Mol. Endocrinol. 19, 1418–1428. doi: 10.1210/me.2005-0125
Cheli, V. T., Santiago González, D. A., Spreuer, V., and Paez, P. M. (2015). Voltage-gated Ca2+ entry promotes oligodendrocyte progenitor cell maturation and myelination in vitro. Exp. Neurol. 265, 69–83. doi: 10.1016/j.expneurol.2014.12.012
Chen, C. Y., Lee, K. W., Lee, C. C., Chin, S. C., Chung, H. W., and Zimmerman, R. A. (2000). Heroin-induced spongiform leukoencephalopathy: value of diffusion MR imaging. J. Comput. Assist. Tomogr. 24, 735–737. doi: 10.1097/00004728-200009000-00013
Chevaleyre, V., Takahashi, K. A., and Castillo, P. E. (2006). Endocannabinoid-mediated synaptic plasticity in the CNS. Annu. Rev. Neurosci. 29, 37–76. doi: 10.1146/annurev.neuro.29.051605.112834
Chiellini, G., Apriletti, J. W., Yoshihara, H. A., Baxter, J. D., Ribeiro, R. C., and Scanlan, T. S. (1998). A high-affinity subtype-selective agonist ligand for the thyroid hormone receptor. Chem. Biol. 5, 299–306. doi: 10.1016/s1074-5521(98)90168-5
Choi, D. W. (1988). Glutamate neurotoxicity and diseases of the nervous system. Neuron 1, 623–634. doi: 10.1016/0896-6273(88)90162-6
Chong, Z. Z., Li, F., and Maiese, K. (2007). The pro-survival pathways of mTOR and protein kinase B target glucogen synthase kinase-3beta and nuclear factor-kappaB to foster endogenous microglial cell protection. Int. J. Mol. Med. 19, 263–272. doi: 10.3892/ijmm.19.2.263
Civelli, O., Bunzow, J. R., and Grandy, D. K. (1993). Molecular diversity of the dopamine receptors. Annu. Rev. Pharmacol. Toxicol. 33, 281–307. doi: 10.1146/annurev.pharmtox.33.1.281
Coco, S., Calegari, F., Pravettoni, E., Pozzi, D., Taverna, E., Rosa, P., et al. (2003). Storage and release of ATP from astrocytes in culture. J. Biol. Chem. 278, 1354–1362. doi: 10.1074/jbc.m209454200
Cohen, R. I., and Almazan, G. (1993). Norepinephrine-stimulated PI hydrolysis in oligodendrocytes is mediated by alpha 1A-adrenoceptors. Neuroreport 4, 1115–1158.
Cohen, R. I., and Almazan, G. (1994). Rat oligodendrocytes express muscarinic receptors coupled to phosphoinositide hydrolysis and adenylyl ciclase. Eur. J. Neurosci. 6, 1213–1224. doi: 10.1111/j.1460-9568.1994.tb00620.x
Cohen, R. I., Molina-Holgado, E., and Almazan, G. (1996). Carbachol stimulates c-fos expression and proliferation in oligodendrocyte progenitors. Brain Res. Mol. Brain Res. 43, 193–201. doi: 10.1016/s0169-328x(96)00176-3
Compston, A. (2002). “Myelination, demyelination and remyelination,” in Diseases of the Nervous System: Clinical Neuroscience and Therapeutic Principles, 3rd Edn. eds A. K. Asbury, G. M. McKhann, W. I. McDonald, P. J. Goadsby, and J. C. McArthur (Cambridge: Cambridge University Press), 1593–1605.
Compton, W. M., and Volkow, N. D. (2006). Major increases in opioid analgesic abuse in the United States: concerns and strategies. Drug Alcohol Depend. 81, 103–107. doi: 10.1016/j.drugalcdep.2005.05.009
Contestabile, A. (2012). Role of nitric oxide in cerebellar development and function: focus on granule neurons. Cerebellum 11, 50–61. doi: 10.1007/s12311-010-0234-1
Corti, C., Battaglia, G., Molinaro, G., Riozzi, B., Pittaluga, A., and Corsi, M. (2007). The use of knock-out mice unravels distinct roles for mGlu2 and mGlu3 metabotropic glutamate receptors in mechanisms of neurodegeneration/neuroprotection. J. Neurosci. 27, 8297–8308. doi: 10.1523/JNEUROSCI.1889-07.2007
Costanza, M., Musio, S., Abou-Hamdan, M., Binart, N., and Pedotti, R. (2013). Prolactin is not required for the development of severe chronic experimental autoimmune encephalomyelitis. J. Immunol. 191, 2082–2088. doi: 10.4049/jimmunol.1301128
Daubert, E. A., and Condron, B. G. (2010). Serotonin: a regulator of neuronal morphology and circuitry. Trends Neurosci. 33, 424–434. doi: 10.1016/j.tins.2010.05.005
De Angelis, F., Bernardo, A., Magnaghi, V., Minghetti, L., and Tata, A. M. (2011). Muscarinic receptor subtypes as potential targets to modulate oligodendrocyte progenitor survival, proliferation, and differentiation. Dev. Neurobiol. 72, 713–728. doi: 10.1002/dneu.20976
Decker, L., Avellana-Adalid, V., Nait-Oumesmar, B., Durbec, P., and Baron-VanEvercooren, A. (2000). Oligodendrocyte precursor migration and differentiation: combined effects of PSA residues, growth factors and substrates. Mol. Cell. Neurosci. 16, 422–439. doi: 10.1006/mcne.2000.0885
de la Fuente, A. G., Errea, O., van Wijngaarden, P., Gonzalez, G. A., Kerninon, C., Jarjour, A. A., et al. (2015). Vitamin D receptor-retinoid X receptor heterodimer signaling regulates oligodendrocyte progenitor cell differentiation. J. Cell Biol. 211, 975–985. doi: 10.1083/jcb.201505119
Deng, W., Rosenberg, P. A., Volpe, J. J., and Jensen, F. E. (2003). Calcium-permeable AMPA/kainate receptors mediate toxicity and preconditioning by oxygen-glucose deprivation in oligodendrocyte precursors. Proc. Natl. Acad. Sci. U S A 100, 6801–6806. doi: 10.1073/pnas.1136624100
Deng, W., Wang, H., Rosenberg, P. A., Volpe, J. J., and Jensen, F. E. (2004). Role of metabotropic glutamate receptors in oligodendrocyte excitotoxicity and oxidative stress. Proc. Natl. Acad. Sci. U S A 101, 7751–7756. doi: 10.1073/pnas.0307850101
De Nicola, A. F., Gonzalez, S. L., Labombarda, F., González Deniselle, M. C., Garay, L., Guennoun, R., et al. (2006). Progesterone treatment of spinal cord injury: effects on receptors, neurotrophins and myelination. J. Mol. Neurosci. 28, 3–15. doi: 10.1385/jmn:28:1:3
Deshmukh, V. A., Tardif, V., Lyssiotis, C. A., Green, C. C., Kerman, B., Kim, H. J., et al. (2013). A regenerative approach to the treatment of multiple sclerosis. Nature 502, 327–332. doi: 10.1038/nature12647
De Simone, R., Ajmone-Cat, M. A., Carnevale, D., and Minghetti, L. (2005). Activation of alpha7 nicotinic acetylcholine receptor by nicotine selectively up-regulates cyclooxygenase-2 and prostaglandin E2 in rat microglial cultures. J. Neuroinflammation 2:4. doi: 10.1186/1742-2094-2-4
Devito, W. J. (1988). Distribution of immunoreactive prolactin in the male and female rat brain: effects of hypophysectomy and intraventricular administration of colchicine. Neuroendocrinology 47, 284–289. doi: 10.1159/000124926
Dingledine, R., Borges, K., Bowie, D., and Traynelis, S. F. (1999). The glutamate receptor ion channels. Pharmacol. Rev. 51, 7–61.
Domercq, M., Etxebarria, E., Pérez-Samartín, A., and Matute, C. (2005). Excitotoxic oligodendrocyte death and axonal damage induced by glutamate transporter inhibition. Glia 52, 36–46. doi: 10.1002/glia.20221
Domercq, M., Sánchez-Gómez, M. V., Areso, P., and Matute, C. (1999). Expression of glutamate transporters in rat optic nerve oligodendrocytes. Eur. J. Neurosci. 11, 2226–2236. doi: 10.1046/j.1460-9568.1999.00639.x
Dugas, J. C., Cuellar, T. L., Scholze, A., Ason, B., Ibrahim, A., Emery, B., et al. (2010). Dicer1 and miR-219 are required for normal oligodendrocyte differentiation and myelination. Neuron 65, 597–611. doi: 10.1016/j.neuron.2010.01.027
Dugas, J. C., Ibrahim, A., and Barres, B. A. (2012). The T3-induced gene KLF9 regulates oligodendrocyte differentiation and myelin regeneration. Mol. Cell. Neurosci. 50, 45–57. doi: 10.1016/j.mcn.2012.03.007
Dum, J. E., and Herz, A. (1981). In vivo receptor binding of the opiate partial agonist, buprenorphine, correlated with its agonistic and antagonistic actions. Br. J. Pharmacol. 74, 627–633. doi: 10.1111/j.1476-5381.1981.tb10473.x
Durand, B., and Raff, M. (2000). A cell-intrinsic timer that operates during oligodendrocyte development. Bioessays 22, 64–71. doi: 10.1002/(sici)1521-1878(200001)22:1<64::aid-bies11>3.0.co;2-q
Dzhala, V. I., Talos, D. M., Sdrulla, D. A., Brumback, A. C., Mathews, G. C., Benke, T. A., et al. (2005). NKCC1 transporter facilitates seizures in the developing brain. Nat. Med. 11, 1205–1213. doi: 10.1038/nm1301
Eglen, R. M. (2005). Muscarinic receptor subtypes in neuronal and non-neuronal cholinergic function. Autonom. Autocoid Pharmacol. 26, 219–233. doi: 10.1111/j.1474-8673.2006.00368.x
Esaki, T., Cook, M., Shimoji, K., Murphy, D. L., Sokoloff, L., and Holmes, A. (2005). Developmental disruption of serotonin transporter function impairs cerebral responses to whisker stimulation in mice. Proc. Natl. Acad. Sci. U S A 102, 5582–5587. doi: 10.1073/pnas.0501509102
Eschenroeder, A. C., Vestal-Laborde, A. A., Sanchez, E. S., Robinson, S. E., and Sato-Bigbee, C. (2012). Oligodendrocyte responses to buprenorphine uncover novel and opposing roles of μ-opioid- and nociceptin/orphanin FQ receptors in cell development: implications for drug addiction treatment during pregnancy. Glia 60, 125–136. doi: 10.1002/glia.21253
Evans, R. M. (2005). The nuclear receptor superfamily: a Rosetta stone for physiology. Mol. Endocrinol. 19, 1429–1438. doi: 10.1210/me.2005-0046
Fan, L. W., Bhatt, A., Tien, L. T., Zheng, B., Simpson, K. L., Lin, R. C., et al. (2015). Exposure to serotonin adversely affects oligodendrocyte development and myelination in vitro. J. Neurochem. 133, 532–543. doi: 10.1111/jnc.12988
Farsetti, A., Mitsuhashi, T., Desvergne, B., Robbins, J., and Nikodem, V. M. (1991). Molecular basis of thyroid hormone regulation of myelin basic protein gene expression in rodent brain. J. Biol. Chem. 266, 23226–23232.
Felder, C. C., Bymaster, F. P., Ward, J., and DeLapp, N. (2000). Therapeutic opportunities for muscarinic receptors in central nervous system. J. Med. Chem. 43, 4333–4353. doi: 10.1021/jm990607u
Felts, P. A., Baker, T. A., and Smith, K. J. (1997). Conduction in segmentally demyelinated mammalian central axons. J. Neurosci. 17, 7267–7277.
Feng, J. F., Gao, X. F., Pu, Y. Y., Burnstock, G., Xiang, Z., and He, C. (2015). P2X7 receptors and Fyn kinase mediate ATP-induced oligodendrocyte progenitor cell migration. Purinergic Signal. 11, 361–369. doi: 10.1007/s11302-015-9458-3
Fields, R. D., and Stevens, B. (2000). ATP: an extracellular signaling molecule between neurons and glia. Trends Neurosci. 23, 625–633. doi: 10.1016/s0166-2236(00)01674-x
Fitch, R. H., Berrebi, A. S., Cowell, P. E., Schrott, L. M., and Denenberg, V. H. (1990). Corpus callosum: effects of neonatal hormones on sexual dimorphism in the rat. Brain Res. 51, 111–116. doi: 10.1016/0006-8993(90)90584-x
Flores, A. I., Mallon, B. S., Matsui, T., Ogawa, W., Rosenzweig, A., Okamoto, T., et al. (2000). Akt-mediated survival of oligodendrocytes induced by neuregulins. J. Neurosci. 20, 7622–7630. doi: 10.1074/jbc.m910419199
Franklin, R. J. M., and ffrench-Constant, C. (2008). Remyelination in the CNS: from biology to therapy. Nat. Rev. Neurosci. 9, 839–855. doi: 10.1038/nrn2480
Franklin, R. J., and Kotter, M. R. (2008). The biology of CNS remyelination: the key to therapeutic advances. J. Neurol. 255, 19–25. doi: 10.1007/s00415-008-1004-6
Freeman, M. E., Kanyicska, B., Lerant, A., and Nagy, G. (2000). Prolactin: structure, function and regulation of secretion. Physiol. Rev. 80, 1523–1631.
Freemantle, E., Chen, G. G., Cruceanu, C., Mechawar, N., and Turecki, G. (2013). Analysis of oxysterols and cholesterol in prefrontal cortex of suicides. Int. J. Neuropsychopharmacol. 16, 1241–1249. doi: 10.1017/s1461145712001587
Fuxe, K., Hökfelt, T., Eneroth, P., Gustafsson, J. A., and Skett, P. (1977). Prolactin-like immunoreactivity: localization in nerve terminals of rat hypothalamus. Science 196, 899–900. doi: 10.1126/science.323973
Gao, F. B., Apperly, J., and Raff, M. (1998). Cell-intrinsic timers and thyroid hormone regulate the probability of cell-cycle withdrawal and differentiation of oligodendrocyte precursor cells. Dev. Biol. 197, 54–66. doi: 10.1006/dbio.1998.8877
Garay, L., Gonzalez Deniselle, M. C., Gierman, L., Meyer, M., Lima, A., Roig, P., et al. (2008). Steroid protection in the experimental autoimmune encephalomyelitis model of multiple sclerosis. Neuroimmunomodulation 15, 76–83. doi: 10.1159/000135627
Garthwaite, J. (2008). Concepts of neural nitric oxide-mediated transmission. Eur. J. Neurosci. 27, 2783–2802. doi: 10.1111/j.1460-9568.2008.06285.x
Garthwaite, G., Hampden-Smith, K., Wilson, G. W., Goodwin, D. A., and Garthwaite, J. (2015). Nitric oxide targets oligodendrocytes and promotes their morphological differentiation. Glia 63, 383–399. doi: 10.1002/glia.22759
Gautier, H. O., Evans, K. A., Volbracht, K., James, R., Sitnikov, S., Lundgaard, I., et al. (2015). Neuronal activity regulates remyelination via glutamate signalling to oligodendrocyte progenitors. Nat. Commun. 6:8518. doi: 10.1038/ncomms9518
Germain, P., Chambon, P., Eichele, G., Evans, R. M., Lazar, M. A., Leid, M., et al. (2006a). International union of pharmacology. LXIII. Retinoid X receptors. Pharmacol. Rev. 58, 760–772. doi: 10.1124/pr.58.4.7
Germain, P., Staels, B., Dacquet, C., Spedding, M., and Laudet, V. (2006b). Overview of nomenclature of nuclear receptors. Pharmacol. Rev. 58, 685–704. doi: 10.1124/pr.58.4.2
Gerstner, B., Sifringe, M., Dzietko, M., Schüller, A., Lee, J., Simons, S., et al. (2007). Estradiol attenuates-induced cell death in the developing white matter. Ann. Neurol. 61, 562–573. doi: 10.1002/ana.21118
Ghiani, C. A., Eisen, A. M., Yuan, X., DePinho, R. A., McBain, C. J., and Gallo, V. (1999). Neurotransmitter receptor activation triggers p27(Kip1 )and p21(CIP1) accumulation and G1 cell cycle arrest in oligodendrocyte progenitors. Development 12, 1077–1090.
Gianola, S., Savio, T., Schwab, M. E., and Rossi, F. (2003). Cell-autonomous mechanisms and myelin-associated factors contribute to the development of Purkinje axon intracortical plexus in the rat cerebellum. J. Neurosci. 23, 4613–4624.
Goldman, S. A., Nedergaard, M., and Windrem, M. S. (2012). Glial progenitor cell-based treatment and modeling of neurological disease. Science 338, 491–495. doi: 10.1126/science.1218071
Goldstein, J. M., Seidman, L. J., Horton, N. J., Makris, N., Kennedy, D. N., Caviness Jr, V. S., et al. (2001). Normal sexual dimorphism of the adult brain assessed by in vivo magnetic resonance imaging. Cereb. Cortex 11, 490–497. doi: 10.1093/cercor/11.6.490
Gregg, C., Shikar, V., Larsen, P., Mak, G., Chojnacki, A., Yong, V. W., et al. (2007). White matter plasticity and enhanced remyelination in the maternal CNS. J. Neurosci. 27, 1812–1823. doi: 10.1523/jneurosci.4441-06.2007
Grinspan, J. (2002). Cells and signaling in oligodendrocyte development. J. Neuropathol. Exp. Neurol. 61, 297–306. doi: 10.1093/jnen/61.4.297
Gronemeyer, H., Gustafsson, J. A., and Laudet, V. (2004). Principles for modulation of the nuclear receptor superfamily. Nat. Rev. Drug Discov. 3, 950–964. doi: 10.1038/nrd1551
Guizzetti, M., Costa, P., Peters, J., and Costa, L. G. (1996). Acetylcholine as a mitogen: muscarinic receptor-mediated proliferation of rat astrocytes and human astrocytoma cells. Eur. J. Pharmacol. 297, 265–273. doi: 10.1016/0014-2999(95)00746-6
Gur, R. C., Turetsky, B. I., Matsui, M., Yan, M., Bilker, W., Hughett, P., et al. (1999). Sex differences in brain gray and white matter in healthy young adults: correlations with cognitive performance. J. Neurosci. 19, 4065–4072.
Haas, J., and Erdö, S. L. (1991). Quisqualate-induced excitotoxic death of glial cells: transient vulnerability of cultured astrocytes. Glia 4, 111–114. doi: 10.1002/glia.440040113
Hall, C. N., and Garthwaite, J. (2009). What is the real physiological NO concentration in vivo? Nitric Oxide 21, 92–103. doi: 10.1016/j.niox.2009.07.002
Harasty, J., Double, K. L., Halliday, G. M., Kril, J. J., and McRitchie, D. A. (1997). Language-associated cortical regions are proportionally larger in the female brain. Arch. Neurol. 54, 171–176. doi: 10.1001/archneur.1997.00550140045011
Hardingham, N., Dachtler, J., and Fox, K. (2013). The role of nitric oxide in pre-synaptic plasticity and homeostasis. Front. Cell. Neurosci. 7:190. doi: 10.3389/fncel.2013.00190
Harlan, R. E., Shivers, B. D., Fox, S. R., Kaplove, K. A., Schachter, B. S., and Pfaff, S. W. (1989). Distribution and partial characterization of immunoreactive prolactin in the rat brain. Neuroendocrinology 49, 7–22. doi: 10.1159/000125085
Hartline, D. K., and Colman, D. R. (2007). Rapid conduction and the evolution of giant axons and myelinated fibers. Curr. Biol. 17, R29–R35. doi: 10.1016/j.cub.2006.11.042
Hay, N. (2005). The Akt-mTOR tango and its relevance to cancer. Cancer Cell 8, 179–183. doi: 10.1016/j.ccr.2005.08.008
Hebert-Chatelain, E., Reguero, L., Puente, N., Lutz, B., Chaouloff, F., Rossignol, R., et al. (2014). Cannabinoid control of brain bioenergetics: exploring the subcellular localization of the CB1 receptor. Mol. Metab. 3, 495–504. doi: 10.1016/j.molmet.2014.03.007
Hewett, S. J., Uliasz, T. F., Vidwans, A. S., and Hewett, J. A. (2000). Cyclooxygenase-2 contributes to N-methyl-D-aspartate-mediated neuronal cell death in primary cortical cell culture. J. Pharmacol. Exp. Ther. 293, 417–425.
Honer, W. G., Falkal, P., Chen, C., Arango, V., Mann, J. J., and Dwork, A. J. (1999). Synaptic and plasticity-associated proteins in anterior frontal cortex in severe mental illness. Neuroscience 91, 1247–1255. doi: 10.1016/s0306-4522(98)00679-4
Horiuchi, M., Itoh, A., Pleasure, D., and Itoh, T. (2006). MEK-ERK signaling is involved in interferon-gamma-induced death of oligodendroglial progenitor cells. J. Biol. Chem. 281, 20095–20106. doi: 10.1074/jbc.m603179200
Howlett, A. C., Barth, F., Bonner, T. I., Cabral, G., Casellas, P., Devane, W. A., et al. (2002). International union of pharmacology. XXVII. Classification of cannabinoid receptors. Pharmacol. Rev. 54, 161–202. doi: 10.1124/pr.54.2.161
Huang, J. K., Jarjour, A. A., Nait Oumesmar, B., Kerninon, C., Williams, A., Krezel, W., et al. (2011). Retinoid X receptor gamma signaling accelerates CNS remyelination. Nat. Neurosci. 14, 45–53. doi: 10.1038/nn.2702
Hussain, R., Ghoumari, A. M., Bielecki, B., Steibel, J., Boehm, N., Liere, P., et al. (2013). The neural androgen receptor: a therapeutic target for myelin repair in chronic demyelination. Brain 1, 132–146. doi: 10.1093/brain/aws284
Iadecola, C., Niwa, K., Nogawa, S., Zhao, X., Nagayama, M., and Araki, E. (2001). Reduced susceptibility to ischemic brain injury and N-methyl-D-aspartate-mediated neurotoxicity in cyclooxygenase-2-deficient mice. Proc. Natl. Acad. Sci. U S A 98, 1294–1299. doi: 10.1073/pnas.98.3.1294
Iglesias-Rozas, J. R., and Garrosa, M. (2012). The discovery of oligodendroglia cells by Rio-Hortega: his original articles. 1921. Clin. Neuropathol. 31, 437–439. doi: 10.5414/np301001
Imamura, O., Arai, M., Dateki, M., Ogata, T., Uchida, R., Tomoda, H., et al. (2015). Nicotinic acetylcholine receptors mediate donepezil-induced oligodendrocyte differentiation. J. Neurochem. 135, 1086–1098. doi: 10.1111/jnc.13294
Izzo, A. A., Borrelli, F., Capasso, R., Di Marzo, V., and Mechoulam, R. (2009). Non-psychotropic plant cannabinoids: new therapeutic opportunities from an ancient herb. Trends Pharmacol. Sci. 30, 515–527. doi: 10.1016/j.tips.2009.07.006
Jabs, R., Pivneva, T., Hüttmann, K., Wyczynski, A., Nolte, C., Kettenmann, H., et al. (2005). Synaptic transmission onto hippocampal glial cells with hGFAP promoter activity. J. Cell Sci. 118, 3791–3803. doi: 10.1242/jcs.02515
Janowski, B. A., Willy, P. J., Devi, T. R., Falck, J. R., and Mangelsdorf, D. J. (1996). An oxysterol signalling pathway mediated by the nuclear receptor LXR alpha. Nature 383, 728–731. doi: 10.1038/383728a0
Jung-Testas, I., and Baulieu, E. E. (1998). Steroid hormone receptors and steroid action in rat glial cells of the central and peripheral nervous system. J. Steroid Biochem. Mol. Biol. 65, 243–251. doi: 10.1016/s0960-0760(97)00191-x
Káradóttir, R., Cavelier, P., Bergersen, L. H., and Attwell, D. (2005). NMDA receptors are expressed in oligodendrocytes and activated in ischaemia. Nature 438, 1162–1166. doi: 10.1038/nature04302
Kastritsis, C. H., and McCarthy, K. D. (1993). Oligodendroglial lineage cells express neuroligand receptors. Glia 8, 106–113. doi: 10.1002/glia.440080206
Kawashima, K., and Fuji, T. (2008). Basic and clinical aspects of non-neuronal acetylcholine: overview of non-neuronal cholinergic systems and their biological significance. J. Pharmacol. Sci. 106, 167–173. doi: 10.1254/jphs.fm0070073
Kelley, K. A., Ho, L., Winger, D., Freire-Moar, J., Borelli, C. B., Aisen, P. S., et al. (1999). Potentiation of excitotoxicity in transgenic mice overexpressing neuronal cyclooxygenase-2. Am. J. Pathol. 155, 995–1004. doi: 10.1016/s0002-9440(10)65199-1
Kelly, P. A., Djiane, J., Postel-Vinay, M. C., and Edery, M. (1991). The prolactin/growth hormone receptor family. Endocr. Rev. 12, 235–251. doi: 10.1210/edrv-12-3-235
Khakh, B. S., Bao, X. R., Labarca, C., and Lester, H. A. (1999). Neuronal P2X transmitter-gated cation channels change their ion selectivity in seconds. Nat. Neurosci. 2, 322–330. doi: 10.1038/7233
Knapp, P. E., Itkis, O. S., Zhang, L., Spruce, B. A., Bakalkin, G., and Hauser, K. F. (2001). Endogenous opioids and oligodendroglial function: possible autocrine/paracrine effects on cell survival and development. Glia 35, 156–165. doi: 10.1002/glia.1080
Knapp, P. E., Maderspach, K., and Hauser, K. F. (1998). Endogenous opioid system in developing normal and jimpy oligodendrocytes: mu and kappa opioid receptors mediate differential mitogenic and growth responses. Glia 22, 189–201. doi: 10.1002/(sici)1098-1136(199802)22:2<189::aid-glia10>3.0.co;2-u
Koós, T., and Tepper, J. M. (1999). Inhibitory control of neostriatal projection neurons by GABAergic interneurons. Nat. Neurosci. 2, 467–472. doi: 10.1038/8138
Langmead, C. J., Watson, J., and Reavill, C. (2008). Muscarinic acetylcholine receptors as CNS drug targets. Pharmacol. Ther. 117, 232–243. doi: 10.1016/j.pharmthera.2007.09.009
Larocca, J. N., and Almazan, G. (1997). Acetylcholine agonists stimulate mitogen-activated protein-kinase in oligodendrocyte progenitors by muscarinic receptors. J. Neurosci. Res. 50, 743–754. doi: 10.1002/(sici)1097-4547(19971201)50:5<743::aid-jnr11>3.3.co;2-y
Lassmann, H. (2011). Mechanisms of neurodegeneration shared between multiple sclerosis and Alzheimer’s disease. J. Neural Transm. (Vienna) 118, 747–752. doi: 10.1007/s00702-011-0607-8
Lauder, J. M. (1993). Neurotransmitters as growth regulatory signal: role of receptors and second messangers. Trends Neurosci. 16, 233–239. doi: 10.1016/0166-2236(93)90162-f
Laudet, V., and Gronemeyer, H. (2002). The Nuclear Receptor Facts Book. San Diego, CA: Academic Press.
Lee, J. Y., Kang So, R., and Yune, T. Y. (2015). Fluoxetine prevents oligodendrocyte cell death by inhibiting microglia activation after spinal cord injury. J. Neurotrauma 32, 633–644. doi: 10.1089/neu.2014.3527
Lemire, J. M., and Archer, D. C. (1991). 1,25-Dihydroxyvitamin D3 prevents the in vivo induction of murine experimental autoimmune encephalomyelitis. J. Clin. Invest. 87, 1103–1107. doi: 10.1172/jci115072
Li, S., and Stys, P. K. (2000). Mechanisms of ionotropic glutamate receptor-mediated excitotoxicity in isolated spinal cord white matter. J. Neurosci. 20, 1190–1198.
Lipton, S. A., and Rosenberg, P. A. (1994). Excitatory amino acids as a final common pathway for neurologic disorders. N. Engl. J. Med. 330, 613–622. doi: 10.1056/nejm199403033300907
Lizasoain, I., Leza, J. C., and Lorenzo, P. (1991). Buprenorphine: bell-shaped dose-response curve for its antagonist effects. Gen. Pharmacol. 22, 297–300. doi: 10.1016/0306-3623(91)90452-c
Loreti, S., Ricordy, R., De Stefano, M. E., Augusti-Tocco, G., and Tata, A. M. (2007). Acetylcholine inhibits cell cycle progression in rat Schwann cells by activation of the M2 receptor subtype. Neuron Glia Biol. 4, 269–279. doi: 10.1017/s1740925x08000045
Loughhead, A. M., Stowe, Z. N., Newport, D. J., Ritchie, J. C., DeVane, C. L., and Owens, M. J. (2006). Placental passage of tricyclic antidepressants. Biol. Psychiatry 59, 287–290. doi: 10.1016/j.biopsych.2005.06.040
Lu, Q. R., Cai, L., Rowitch, D., Cepko, C. L., and Stiles, C. D. (2001). Ectopic expression of Olig1 promotes oligodendrocyte formation and reduces neuronal survival in developing mouse cortex. Nat. Neurosci. 4, 973–974. doi: 10.1038/nn718
Lutfy, K., Eitan, S., Bryant, C. D., Yang, Y. C., Saliminejad, N., Walwyn, W., et al. (2003). Buprenorphine-induced antinociception is mediated by mu-opioid receptors and compromised by concomitant activation of opioid receptor-like receptors. J. Neurosci. 23, 10331–10337.
Luyt, K., Slade, T. P., Dorward, J. J., Durant, C. F., Yue, W., Shigemoto, R., et al. (2007). Developing oligodendrocytes express functional GABAB receptors that stimulate cell proliferation and migration. J. Neurochem. 100, 822–840. doi: 10.1111/j.1471-4159.2006.04255.x
Manzano, J., Morte, B., Scanlan, T. S., and Bernal, J. (2003). Differential effects of triiodothyronine and the thyroid hormone receptor beta-specific agonist GC-1 on thyroid hormone target genes in the brain. Endocrinology 144, 5480–5487. doi: 10.1210/en.2003-0633
Markram, H., Toledo-Rodriguez, M., Wang, Y., Gupta, A., Silberberg, G., and Wu, C. (2004). Interneurons of the neocortical inhibitory system. Nat. Rev. Neurosci. 5, 793–807. doi: 10.1038/nrn1519
Maruyama, S., Tsukada, H., Nishiyama, S., Kakiuchi, T., Fukumoto, D., Oku, N., et al. (2008). In vivo quantitative autoradiographic analysis of brain muscarinic receptor occupancy by antimuscarinic agents for overactive bladder treatment. J. Pharmacol. Exp. Ther. 325, 774–781. doi: 10.1124/jpet.108.136390
Mato, S., Alberdi, E., Ledent, C., Watanabe, M., and Matute, C. (2009). CB1 cannabinoid receptor-dependent and -independent inhibition of depolarization-induced calcium influx in oligodendrocytes. Glia 57, 295–306. doi: 10.1002/glia.20757
Mato, S., Victoria Sánchez-Gómez, M., and Matute, C. (2010). Cannabidiol induces intracellular calcium elevation and cytotoxicity in oligodendrocytes. Glia 58, 1739–1747. doi: 10.1002/glia.21044
Matute, C. (2006). Oligodendrocyte NMDA receptors: a novel therapeutic target. Trends Mol. Med. 12, 289–292. doi: 10.1016/j.molmed.2006.05.004
Matute, C. (2010). Calcium dyshomeostasis in white matter pathology. Cell Calcium 47, 150–157. doi: 10.1016/j.ceca.2009.12.004
Matute, C., Sánchez-Gómez, M. V., Martínez-Millán, L., and Miledi, R. (1997). Glutamate receptor-mediated toxicity in optic nerve oligodendrocytes. Proc. Natl. Acad. Sci. U S A 94, 8830–8835. doi: 10.1073/pnas.94.16.8830
McArthur, R. A., Gray, J., and Schreiber, R. (2010). Cognitive effects of muscarinic M1 functional agonists in non-human primates and clinical trials. Curr. Opin. Investig. Drugs 11, 740–760.
McDonald, J. W., Althomsons, S. P., Hyrc, K. L., Choi, D. W., and Goldberg, M. P. (1998). Oligodendrocytes from forebrain are highly vulnerable to AMPA/kainate receptor-mediated excitotoxicity. Nat. Med. 4, 291–297. doi: 10.1038/nm0398-291
Meffre, D., Shackleford, G., Hichor, M., Gorgievski, V., Tzavara, E. T., Trousson, A., et al. (2015). Liver X receptors alpha and beta promote myelination and remyelination in the cerebellum. Proc. Natl. Acad. Sci. U S A 112, 7587–7592. doi: 10.1073/pnas.1424951112
Mensch, S., Baraban, M., Almeida, R., Czopka, T., Ausborn, J., El Manira, A., et al. (2015). Synaptic vesicle release regulates myelin sheath number of individual oligodendrocytes in vivo. Nat. Neurosci. 18, 628–630. doi: 10.1038/nn.3991
Merrill, J. E., and Benveniste, E. N. (1996). Cytokines in inflammatory brain lesions: helpful and harmful. Trends Neurosci. 19, 331–338. doi: 10.1016/0166-2236(96)10047-3
Micu, I., Jiang, Q., Coderre, E., Ridsdale, A., Zhang, L., Woulfe, J., et al. (2006). NMDA receptors mediate calcium accumulation in myelin during chemical ischaemia. Nature 439, 988–992. doi: 10.1038/nature04474
Milner, R., Anderson, H. J., Rippon, R. F., McKay, J. S., Franklin, R. J., Marchionni, M. A., et al. (1997). Contrasting effects of mitogenic growth factors on oligodendrocyte precursor cell migration. Glia 19, 85–90. doi: 10.1002/(sici)1098-1136(199701)19:1<85::aid-glia9>3.0.co;2-9
Missale, C., Nash, S. R., Robinson, S. W., Jaber, M., and Caron, M. G. (1998). Dopamine receptors: from structure to function. Physiol. Rev. 78, 189–225.
Modell, J. G., Tandon, R., and Beresford, T. P. (1989). Dopaminergic activity of the antimuscarinic antiparkinsonian agents. J. Clin. Psychopharmacol. 9, 347–351. doi: 10.1097/00004714-198910000-00006
Moher, D., Liberati, A., Tetzlaff, J., Altman, D. G., and PRISMA Group. (2010). Preferred reporting items for systematic reviews and meta-analyses: the PRISMA statement. Int. J. Surg. 8, 336–341. doi: 10.1016/j.ijsu.2010.02.007
Molina-Holgado, E., Khorchid, A., Liu, H. N., and Almazan, G. (2003). Regulation of muscarinic receptor function in developing oligodendrocytes by agonistic exposure. Br. J. Pharmacol. 138, 47–56. doi: 10.1038/sj.bjp.0705002
Moreno, S., Farioli-Vecchioli, S., and Cerù, M. P. (2004). Immunolocalization of peroxisome proliferator-activated receptors and retinoid X receptors in the adult rat CNS. Neuroscience 123, 131–145. doi: 10.1016/j.neuroscience.2003.08.064
Moriya, H., Takagi, Y., Nakanishi, T., Hayashi, M., Tani, T., and Hirotsu, I. (1999). Affinity profiles of various muscarinic antagonists for cloned human muscarinic acetylcholine receptor (mAChR) subtypes and mAChRs in rat heart and submandibular gland. Life Sci. 64, 2351–2358. doi: 10.1016/s0024-3205(99)00188-5
Murphy, S., Pearce, B., and Morrow, C. (1986). Astrocytes have both M1 and M2 muscarinic receptor subtypes. Brain Res. 29, 177–180. doi: 10.1016/0006-8993(86)91000-0
Murphy, T. H., Schnaar, R. L., and Coyle, J. T. (1990). Immature cortical neurons are uniquely sensitive to glutamate toxicity by inhibition of cystine uptake. FASEB J. 4, 1624–1633.
Nanan, R., von Stockhausen, H. B., Petersen, B., Solymosi, L., and Warmuth-Metz, M. (2000). Unusual pattern of leukoencephalopathy after morphine sulphate intoxication. Neuroradiology 42, 845–848. doi: 10.1007/s002340000442
Nataf, S., Levison, S. W., and Barnum, S. R. (2001). Expression of the anaphylatoxin C5a receptor in the oligodendrocyte lineage. Brain Res. 894, 321–326. doi: 10.1016/s0006-8993(01)02003-0
Nikodemova, M., Duncan, I. D., and Watters, J. J. (2006). Minocycline exerts inhibitory effects on multiple mitogen-activated protein kinase and IκBα degradation in a stimulus-specific manner in microglia. J. Neurochem. 96, 314–323. doi: 10.1111/j.1471-4159.2005.03520.x
Niu, J., Mei, F., Wang, L., Liu, S., Tian, Y., Mo, W., et al. (2012). Phosphorylated olig1 localizes to the cytosol of oligodendrocytes and promotes membrane expansion and maturation. Glia 60, 1427–1436. doi: 10.1002/glia.22364
Nogawa, S., Zhang, F., Ross, M. E., and Iadecola, C. (1997). Cyclooxygenase-2 gene expression in neurons contributes to ischemic brain damage. J. Neurosci. 17, 2746–2755.
Nopoulos, P., Flaum, M., O’Leary, D., and Anderson, N. C. (2000). Sexual dimorphism in the human brain: evaluation of tissue volume, tissue composition and surface anatomy using magnetic resonance imaging. Psychiatry Res. 98, 1–13. doi: 10.1016/s0925-4927(99)00044-x
North, R. A. (2002). Molecular physiology of P2X receptors. Physiol. Rev. 82, 1013–1067. doi: 10.1152/physrev.00015.2002
Nottebohm, F., and Arnold, A. P. (1976). Sexual dimorphism in vocal control areas of the songbird brain. Science 194, 211–213. doi: 10.1126/science.959852
Nuñez, J. L., Nelson, J., Pych, J. C., Kim, J. H. Y., and Juraska, J. M. (2000). Myelination in the splenium of the corpus callosum in adult male and female rats. Brain Res. Dev. Brain Res. 120, 87–90. doi: 10.1016/s0165-3806(99)00193-5
Oka, A., Belliveau, M. J., Rosenberg, P. A., and Volpe, J. J. (1993). Vulnerability of oligodendroglia to glutamate: pharmacology, mechanisms and prevention. J. Neurosci. 13, 1441–1453.
O’Shea, P. J., and Williams, G. R. (2002). Insight into the physiological actions of thyroid hormone receptors from genetically modified mice. J. Endocrinol. 175, 553–570. doi: 10.1677/joe.0.1750553
Palumbo, S., Toscano, C. D., Parente, L., Weigert, R., and Bosetti, F. (2012). The cyclooxygenase-2 pathway via the PGE2 EP2 receptor contributes to oligodendrocytes apoptosis in cuprizone-induced demyelination. J. Neurochem. 121, 418–427. doi: 10.1111/j.1471-4159.2011.07363.x
Patani, R., Balaratnam, M., Vora, A., and Reynolds, R. (2007). Remyelination can be extensive in multiple sclerosis despite a long disease course. Neuropathol. Appl. Neurobiol. 33, 277–287. doi: 10.1111/j.1365-2990.2007.00805.x
Peralta, E. G., Ashkenazi, A., Winslow, J. W., Smith, D. H., Ramachandran, J., and Capon, D. J. (1987). Distinct primary structures, ligand-binding properties and tissue-specific expression of four human muscarinic acetylcholine receptors. EMBO J. 6, 3923–3929.
Persico, A. M., Altamura, C., Calia, E., Puglisi-Allegra, S., Ventura, R., Lucchese, F., et al. (2000). Serotonin depletion and barrel cortex development: impact of growth impairment vs. serotonin effects on thalamocortical endings. Cereb. Cortex 10, 181–191. doi: 10.1093/cercor/10.2.181
Persson, A. I., Bull, C., and Eriksson, P. S. (2006). Requirement for Id1 in opioid-induced oligodendrogenesis in cultured adult rat hippocampal progenitors. Eur. J. Neurosci. 23, 2277–2288. doi: 10.1111/j.1460-9568.2006.04764.x
Pitt, D., Werner, P., and Raine, C. S. (2000). Glutamate excitotoxicity in a model of multiple sclerosis. Nat. Med. 6, 67–70. doi: 10.1038/71555
Prineas, J. W., and Connell, F. (1979). Remyelination in multiple sclerosis. Ann. Neurol. 5, 22–31. doi: 10.1002/ana.410050105
Proskocil, B. J., Sekhon, H. S., Jia, Y., Savchenk, V., Blakely, R. D., Lindstrom, J., et al. (2004). Acetylcholine is an autocrine or paracrine hormone synthesized and secreted by airway bronchial epithelial cells. Endocrinology 145, 2498–2506. doi: 10.1210/en.2003-1728
Raine, C. S., and Wu, E. (1993). Multiple sclerosis: remyelination in acute lesions. J. Neuropathol. Exp. Neurol. 52, 199–204. doi: 10.1097/00005072-199305000-00003
Ralevic, V., and Burnstock, G. (1998). Receptors for purines and pyrimidines. Pharmacol. Rev. 50, 413–492.
Ransohoff, R. M., Hafler, D. A., and Lucchinetti, C. F. (2015). Multiple sclerosis - a quiet revolution. Nat. Rev. Neurol. 11, 134–142. doi: 10.1038/nrneurol.2015.14
Regenold, W. T., Phatak, P., Marano, C. M., Gearhart, L., Viens, C. H., and Hisley, K. C. (2007). Myelin staining of deep white matter in the dorsolateral prefrontal cortex in schizophrenia, bipolar disorder and unipolar major depression. Psychiatry Res. 151, 179–188. doi: 10.1016/j.psychres.2006.12.019
Riskind, P. N., Massacesi, L., Doolittle, T. H., and Hauser, S. L. (1991). The role of prolactin in autoimmune demyelination: suppression of experimental allergic encephalomyelitis by bromocriptine. Ann. Neurol. 29, 542–547. doi: 10.1002/ana.410290514
Ritchie, T., Cole, R., Kim, H. S., de Vellis, J., and Noble, E. P. (1987). Inositol phospholipids hydrolysis in cultured astrocytes and oligodendrocytes. Life Sci. 41, 31–39. doi: 10.1016/0024-3205(87)90553-4
Rodriguez-Pena, A., Ibarrola, N., Iñiguez, M. A., Muñoz, A., and Bernal, J. (1993). Neonatal hypothyroidism affects the timely expression of myelin-associated glycoprotein in the rat brain. J. Clin. Invest. 91, 812–818. doi: 10.1172/jci116301
Rogers, S. W., Gregori, N. Z., Carlson, N., Gahring, L. C., and Noble, M. (2001). Neuronal nicotinic acetylcholine receptor expression by O2A/oligodendrocytes progenitor cells. Glia 33, 306–313. doi: 10.1002/1098-1136(20010315)33:4<306::aid-glia1029>3.3.co;2-n
Rosin, C., Bates, T. E., and Skaper, S. D. (2004). Excitatory amino-acid induced oligodendrocyte cell death in vitro: receptor-dependent and independent mechanisms. J. Neurochem. 90, 1173–1185. doi: 10.1111/j.1471-4159.2004.02584.x
Rosin, C., Colombo, S., Calver, A., Bates, T. E., and Skaper, S. D. (2005). Dopamine D2 and D3 receptor agonists limit oligodendrocyte injury caused by glutamate oxidative stress and oxygen/glucose deprivation. Glia 52, 336–343. doi: 10.1002/glia.20250
Ryan, D., Drysdale, A. J., Lafourcade, C., Pertwee, R. G., and Platt, B. (2009). Cannabidiol targets mitochondria to regulate intracellular Ca2+ levels. J. Neurosci. 29, 2053–2063. doi: 10.1523/JNEUROSCI.4212-08.2009
Saab, A. S., Tzvetanova, I. D., and Nave, K. A. (2013). The role of myelin and oligodendrocytes in axonal energy metabolism. Curr. Opin. Neurobiol. 23, 1065–1072. doi: 10.1016/j.conb.2013.09.008
Salani, M., Anelli, T., Tocco, G. A., Lucarini, E., Mozzetta, C., Poiana, G., et al. (2009). Acetylcholine induced neuronal differentiation: muscarinic receptor activations regulates EGR-1 and REST expression in neuroblastoma cells. J. Neurochem. 108, 821–834. doi: 10.1111/j.1471-4159.2008.05829.x
Salter, M. G., and Fern, R. (2005). NMDA receptors are expressed in developing oligodendrocyte processes and mediate injury. Nature 438, 1167–1171. doi: 10.1038/nature04301
Sanchez, E. S., Bigbee, J. W., Fobbs, W., Robinson, S. E., and Sato-Bigbee, C. (2008). Opioid addiction and pregnancy: perinatal exposure to buprenorphine affects myelination in the developing brain. Glia 56, 1017–1027. doi: 10.1002/glia.20675
Sastre-Garriga, J., Vila, C., Clissold, S., and Montalban, X. (2011). THC and CBD oromucosal spray (Sativex®) in the management of spasticity associated with multiple sclerosis. Expert Rev. Neurother. 11, 627–637. doi: 10.1586/ern.11.47
Sawada, H., Ibi, M., Kihara, T., Urushitani, M., Akaike, A., Kimura, J., et al. (1998). Dopamine D2-type agonists protect mesencephalic neurons from glutamate neurotoxicity: mechanisms of neuroprotective treatment against oxidative stress. Ann. Neurol. 44, 110–119. doi: 10.1002/ana.410440117
Sawada, H., Shimohama, S., Tamura, Y., Kawamura, T., Akaike, A., and Kimura, J. (1996). Methylphenylpyridium ion (MPP+) enhances glutamate-induced cytotoxicity against dopaminergic neurons in cultured rat mesencephalon. J. Neurosci. Res. 43, 55–62. doi: 10.1002/jnr.490430107
Schlaepfer, T. E., Harris, G. J., Tien, A. Y., Peng, L., Lee, S., and Pearlson, G. D. (1995). Structural differences in the cerebral cortex of healthy female and male subjects: a magnetic resonance imaging study. Psychiatry Res. 61, 129–135. doi: 10.1016/0925-4927(95)02634-a
Schrage, K., Koopmans, G., Joosten, E. A. J., and Mey, J. (2006). Macrophages and neurons are targets of retinoic acid signaling after spinal cord contusion injury. Eur. J. Neurosci. 23, 285–295. doi: 10.1111/j.1460-9568.2005.04534.x
Schwarz, J. M., and Bilbo, S. D. (2013). Adolescent morphine exposure affects long-term microglial function and later-life relapse liability in a model of addiction. J. Neurosci. 33, 961–971. doi: 10.1523/JNEUROSCI.2516-12.2013
Seroogy, K., Tsuruo, Y., Hökfelt, T., Walsh, J., Fahrenkrug, J., Emson, P. C., et al. (1988). Further analysis of presence of peptides in dopamine neurons: cholecystokinin, peptide histidine-isoleucine/vasoactive intestinal polypeptide and substance P in rat supramammillary region and mesencephalon. Exp. Brain Res. 72, 523–534. doi: 10.1007/bf00250598
Shirazi, H. A., Rasouli, J., Ciric, B., Rostami, A., and Zhang, G. X. (2015). 1,25-Dihydroxyvitamin D3 enhances neural stem cell proliferation and oligodendrocyte differentiation. Exp. Mol. Pathol. 98, 240–245. doi: 10.1016/j.yexmp.2015.02.004
Siaud, P., Manzoni, O., Balmefrezol, M., Barbanel, G., Assenmacher, I., and Alonso, G. (1989). The organization of prolactin-like-immunoreactive neurons in the rat central nervous system. Light- and electron-microscopic immunocytochemical studies. Cell Tissue Res. 255, 107–115. doi: 10.1007/BF00229071
Simpson, P. B., and Armstrong, R. C. (1999). Intracellular signals and cytoskeletal elements involved in oligodendrocyte progenitor migration. Glia 26, 22–35. doi: 10.1002/(sici)1098-1136(199903)26:1<22::aid-glia3>3.3.co;2-d
Simpson, K. L., Weaver, K. J., de Villers-Sidani, E., Lu, J. Y., Cai, Z., Pang, Y., et al. (2011). Perinatal antidepressant exposure alters cortical network function in rodents. Proc. Natl. Acad. Sci. U S A 108, 18465–18470. doi: 10.1073/pnas.1109353108
Skaper, S. D., Facci, L., Fusco, M., Della Valle, M. F., Zusso, M., Costa, B., et al. (2014). Palmitoylethanolamide, a naturally occurring disease-modifying agent in neuropathic pain. Inflammopharmacology 22, 79–94. doi: 10.1007/s10787-013-0191-7
Smith, W. L., Garavito, R. M., and DeWitt, D. L. (1996). Prostaglandin endoperoxide H synthases (cyclooxygenases)−1 and −2. J. Biol. Chem. 271, 33157–33160. doi: 10.1074/jbc.271.52.33157
Sokoloff, P., Giros, B., Martres, M. P., Bouthenet, M. L., and Schwartz, J. C. (1990). Molecular cloning and characterization of a novel dopamine receptor (D3) as a target for neuroleptics. Nature 347, 146–151. doi: 10.1038/347146a0
Soliven, B. (2001). Calcium signalling in cells of oligodendroglial lineage. Microsc. Res. Tech. 52, 672–679. doi: 10.1002/jemt.1051
Spach, K. M., Nashold, F. E., Dittel, B. N., and Hayes, C. E. (2006). IL-10 signaling is essential for 1,25-dihydroxyvitamin D3-mediated inhibition of experimental autoimmune encephalomyelitis. J. Immunol. 177, 6030–6037. doi: 10.4049/jimmunol.177.9.6030
Spampinato, S. F., Merlo, S., Chisari, M., Nicoletti, F., and Sortino, M. A. (2015). Glial metabotropic glutamate receptor-4 increases maturation and survival of oligodendrocytes. Front. Cell. Neurosci. 8:462. doi: 10.3389/fncel.2014.00462
Sunahara, R. K., Guan, H. C., O’Dowd, B. F., Seeman, P., Laurier, L. G., Ng, G., et al. (1991). Cloning of the gene for a human dopamine D5 receptor with higher affinity for dopamine than D1. Nature 350, 614–619. doi: 10.1038/350614a0
Swanson, C. J., Bures, M., Johnson, M. P., Linden, A. M., Monn, J. A., and Schoepp, D. D. (2005). Metabotropic glutamate receptors as novel targets for anxiety and stress disorders. Nat. Rev. Drug Discov. 4, 131–144. doi: 10.1038/nrd1630
Tagami, T., Yamamoto, H., Moriyama, K., Sawai, K., Usui, T., Shimatsu, A., et al. (2010). Identification of a novel human thyroid hormone receptor beta isoform as a transcriptional modulator. Biochem. Biophys. Res. Commun. 396, 983–988. doi: 10.1016/j.bbrc.2010.05.038
Takahashi, J. L., Giuliani, F., Power, C., Imai, Y., and Yong, V. W. (2003). Interleukin-1beta promotes oligodendrocyte death through glutamate excitotoxicity. Ann. Neurol. 53, 588–595. doi: 10.1002/ana.10519
Takahashi, C., Muramatsu, R., Fujimura, H., Mochizuki, H., and Yamashita, T. (2013). Prostacyclin promotes oligodendrocyte precursor recruitment and remyelination after spinal cord demyelination. Cell Death Dis. 4:e795. doi: 10.1038/cddis.2013.335
Takesian, A. E., and Hensch, T. K. (2013). Balancing plasticity/stability across brain development. Prog. Brain Res. 207, 3–34. doi: 10.1016/b978-0-444-63327-9.00001-1
Tao, R., Ma, Z., and Auerbach, S. B. (2000). Differential effect of local infusion of serotonin reuptake inhibitors in the raphe versus forebrain and the role of depolarization-induced release in increased extracellular serotonin. J. Pharmacol. Exp. Ther. 294, 571–579.
Tata, A. M., and Calogero, A. (2010). Muscarinic receptors and cancer: possible implications in tumors of the nervous system. Curr. Trends Neurol. 4, 23–31.
Tata, A. M., Cursi, S., Biagioni, S., and Augusti-Tocco, G. (2003). Cholinergic modulation of neurofilament expression and neurite outgrowth in chick sensory neurons. J. Neurosci. Res. 73, 227–234. doi: 10.1002/jnr.10650
Taylor, W. D., MacFall, J. R., Payne, M. E., McQuoid, D. R., Provenzale, J. M., Steffens, D. C., et al. (2004). Late-life depression and microstructural abnormalities in dorsolateral prefrontal cortex white matter. Am. J. Psychiatry 161, 1293–1296. doi: 10.1176/appi.ajp.161.7.1293
Temple, S., and Raff, M. C. (1986). Clonal analysis of oligodendrocyte development in culture: evidence for a developmental clock that counts cell divisions. Cell 44, 773–779. doi: 10.1016/0092-8674(86)90843-3
Theoharides, T. C., Asadi, S., and Panagiotidou, S. (2012). A case series of a luteolin formulation (NeuroProtek®) in children with autism spectrum disorders. Int. J. Immunopathol. Pharmacol. 25, 317–323.
Thompson, S. A. (1982). Localization of immunoreactive prolactin in ependyma and circumventricular organs of rat brain. Cell Tissue Res. 225, 79–93. doi: 10.1007/bf00216220
Tiberi, M., Jarvie, K. R., Silvia, C., Falardeau, P., Gingrich, J. A., Godinot, N., et al. (1991). Cloning, molecular characterization, and chromosomal assignment of a gene encoding a second D1 dopamine receptor subtype: differential expression pattern in rat brain compared with the D1A receptor. Proc. Natl. Acad. Sci. U S A 88, 7491–7495. doi: 10.1073/pnas.88.17.7491
Tosic, M., Torch, S., Comte, V., Dolivo, M., Honegger, P., and Matthieu, J. M. (1992). Triiodothyronine has diverse and multiple stimulating effects on expression of the major myelin protein genes. J. Neurochem. 59, 1770–1777. doi: 10.1111/j.1471-4159.1992.tb11009.x
Trost, S. U., Swanson, E., Gloss, B., Wang-Iverson, D. B., Zhang, H., Volodarsky, T., et al. (2000). The thyroid hormone receptor-beta-selective agonist GC-1 differentially affects plasma lipids and cardiac activity. Endocrinology 141, 3057–3064. doi: 10.1210/en.141.9.3057
Trousson, A., Makoukji, J., Petit, P. X., Bernard, S., Slomianny, C., Schumacher, M., et al. (2009). Cross-talk between oxysterols and glucocorticoids: differential regulation of secreted phopholipase A2 and impact on oligodendrocyte death. PLoS One 4:e8080. doi: 10.1371/journal.pone.0008080
Tsai, H. H., and Miller, R. H. (2002). Glial cell migration directed by axon guidance cues. Trends Neurosci. 25, 173–175. doi: 10.1016/s0166-2236(00)02096-8
Tsuneishi, S., Takada, S., Motoike, T., Ohashi, T., Sano, K., and Nakamura, H. (1991). Effects of dexamethasone on the expression of myelin basic protein, proteolipid protein, and glial fibrillary acidic protein genes in developing rat brain. Brain Res. Dev. Brain Res. 61, 117–123. doi: 10.1016/0165-3806(91)90121-x
Van Der Zee, E. A., De Jong, G. I., Strosberg, A. D., and Luiten, P. G. M. (1993). Muscarinic acetylcholine receptor-expression in astrocytes in the cortex of young and aged rats. Glia 8, 42–50. doi: 10.1002/glia.440080106
Van Tol, H. H., Bunzow, J. R., Guan, H. C., Sunahara, R. K., Seeman, P., Niznik, H. B., et al. (1991). Cloning of the gene for a human dopamine D4 receptor with high affinity for the antipsychotic clozapine. Nature 350, 610–614. doi: 10.1038/350610a0
Vartanian, T., Szuchet, S., Dawson, G., and Campagnoni, A. T. (1986). Oligodendrocyte adhesion activates protein kinase C-mediated phosphorylation of myelin basic protein. Science 234, 1395–1398. doi: 10.1126/science.2431483
Vestal-Laborde, A. A., Eschenroeder, A. C., Bigbee, J. W., Robinson, S. E., and Sato-Bigbee, C. (2014). The opioid system and brain development: effects of methadone on the OL lineage and the early stages of myelination. Dev. Neurosci. 36, 409–421. doi: 10.1159/000365074
Vielkind, U., Walencewicz, A., Levine, J. M., and Bohn, M. C. (1990). Type II glucocorticoid receptors are expressed in oligodendrocytes and astrocytes. J. Neurosci. Res. 27, 360–373. doi: 10.1002/jnr.490270315
Virchow, R. (1854). “Über das ausgebreitete vorkommen einer dem nervenmark analogen substanz in den tierischen Geweben”. Archiv für pathologische Anatomie und Physiologie und für klinische Medizin (in German) 6, 562–572.
Wake, H., Ortiz, F. C., Woo, D. H., Lee, P. R., Angulo, M. C., and Fields, R. D. (2015). Nonsynaptic junctions on myelinating glia promote preferential myelination of electrically active axons. Nat. Commun. 6:7844. doi: 10.1038/ncomms8844
Walter, L., and Stella, N. (2004). Cannabinoids and neuroinflammation. Br. J. Pharmacol. 141, 775–785. doi: 10.1038/sj.bjp.0705667
Walz, W., Ilschner, S., Ohlemeyer, C., Banati, R., and Kettenmann, H. (1993). Extracellular ATP activates a cation conductance and a K+ conductance in cultured microglial cells from mouse brain. J. Neurosci. 13, 4403–4411.
Wang, J., Qiao, J., Zhang, Y., Wang, H., Zhu, S., Zhang, H., et al. (2014). Desvenlafaxine prevents white matter injury and improves the decreased phosphorylation of the rate-limiting enzyme of cholesterol synthesis in a chronic mouse model of depression. J. Neurochem. 131, 229–238. doi: 10.1111/jnc.12792
Wang, H., Yan, Y., Kintner, D. B., Lytle, C., and Sun, D. (2003). GABA-mediated trophic effect on oligodendrocytes requires Na-K-2Cl cotransport activity. J. Neurophysiol. 90, 1257–1265. doi: 10.1152/jn.01174.2002
Ward, P. A., and Newman, L. J. (1969). A neutrophil chemotactic factor from human C’5. J. Immunol. 102, 93–99.
Werner, P., Pitt, D., and Raine, C. S. (2001). Multiple sclerosis: altered glutamate homeostasis in lesions correlates with oligodendrocyte and axonal damage. Ann. Neurol. 50, 169–180. doi: 10.1002/ana.1077
Wess, J. (2004). Muscarinic acetylcholine receptor knockout mice: novel phenotypes and clinical implications. Annu. Rev. Pharmacol. Toxicol. 44, 423–450. doi: 10.1146/annurev.pharmtox.44.101802.121622
Wessler, I., Kilbinger, H., Bittinger, F., Unger, R., and Kirkpatrick, C. J. (2001). The biological role of non-neuronal acetylcholine in plants and humans. Jpn. J. Pharmacol. 85, 2–10. doi: 10.1254/jjp.85.2
Williams, G. R. (2000). Cloning and characterization of two novel thyroid hormone receptor beta isoforms. Mol. Cell. Biol. 20, 8329–8342. doi: 10.1128/mcb.20.22.8329-8342.2000
Xing, Y. L., Röth, P. T., Stratton, J. A., Chuang, B. H., Danne, J., Ellis, S. L., et al. (2014). Adult neural precursor cells from the subventricular zone contribute significantly to oligodendrocyte regeneration and remyelination. J. Neurosci. 34, 14128–14146. doi: 10.1523/jneurosci.3491-13.2014
Xu, Y., Sari, Y., and Zhou, F. C. (2004). Selective serotonin reuptake inhibitor disrupts organization of thalamocortical somatosensory barrels during development. Brain Res. Dev. Brain Res. 150, 151–161. doi: 10.1016/j.devbrainres.2003.02.001
Yamazaki, Y., Hozumi, Y., Kaneko, K., Sugihara, T., Fujii, S., Goto, K., et al. (2007). Modulatory effects of oligodendrocytes on the conduction velocity of velocity of action potentials along axons in the alveus of rat CA1 hippocampal region. Neuron Glia Biol. 3, 325–334. doi: 10.1017/S1740925x08000070
Yang, S., Li, C., Zhang, W., Wang, W., and Tang, Y. (2008). Sex differences in the white matter and myelinated nerve fibers of Long-Evans rats. Brain Res. 1216, 16–23. doi: 10.1016/j.brainres.2008.03.052
Yang, G., Zhang, Y., Ross, M. E., and Iadecola, C. (2003). Attenuation of activity-induced increases in cerebellar blood flow in mice lacking neuronal nitric oxide synthase. Am. J. Physiol. Heart Circ. Physiol. 285, H298–H304. doi: 10.1152/ajpheart.00043.2003
Yao, H. H., Ding, J. H., Zhou, F., Wang, F., Hu, L. F., Sun, T., et al. (2005). Enhancement of glutamate uptake mediates the neuroprotection exerted by activating group II or III metabotropic glutamate receptors on astrocytes. J. Neurochem. 92, 948–961. doi: 10.1111/j.1471-4159.2004.02937.x
Yen, P. M. (2001). Physiological and molecular basis of thyroid hormone action. Physiol. Rev. 81, 1097–1142.
Yong, V. W., Dooley, N. P., and Noble, P. G. (1994). Protein kinase C in cultured adult human oligodendrocytes: a potential role for isoform alpha as a mediator of process outgrowth. J. Neurosci. Res. 39, 83–96. doi: 10.1002/jnr.490390111
Yoo, A. S., Krieger, C., and Kim, S. U. (1999). Process extension and intracellular Ca2+ in cultured murine oligodendrocytes. Brain Res. 827, 19–27. doi: 10.1016/s0006-8993(99)01282-2
Zhornitsky, S., Johnson, T. A., Metz, L. M., Weiss, S., and Yong, V. W. (2015). Prolactin in combination with interferon-β reduces disease severity in an animal model of multiple sclerosis. J. Neuroinflammation 12:55. doi: 10.1186/s12974-015-0278-8
Zhou, Q., Wang, S., and Anderson, D. J. (2000). Identification of a novel family of oligodendrocyte lineage-specific basic helix-loop-helix transcription factors. Neuron 25, 331–343. doi: 10.1016/s0896-6273(00)80898-3
Keywords: oligodendrocyte, GABA, glutamatergic, cholinergic, muscarinergic, opioids, nuclear receptor, regeneration
Citation: Marinelli C, Bertalot T, Zusso M, Skaper SD and Giusti P (2016) Systematic Review of Pharmacological Properties of the Oligodendrocyte Lineage. Front. Cell. Neurosci. 10:27. doi: 10.3389/fncel.2016.00027
Received: 30 November 2015; Accepted: 25 January 2016;
Published: 12 February 2016.
Edited by:
Tycho M. Hoogland, Erasmus MC, NetherlandsReviewed by:
Gonzalo Alvarez-Bolado, University of Heidelberg, GermanyTobias David Merson, Florey Institute of Neuroscience and Mental Health, Australia
Copyright © 2016 Marinelli, Bertalot, Zusso, Skaper and Giusti. This is an open-access article distributed under the terms of the Creative Commons Attribution License (CC BY). The use, distribution and reproduction in other forums is permitted, provided the original author(s) or licensor are credited and that the original publication in this journal is cited, in accordance with accepted academic practice. No use, distribution or reproduction is permitted which does not comply with these terms.
*Correspondence: Pietro Giusti, pietro.giusti@unipd.it