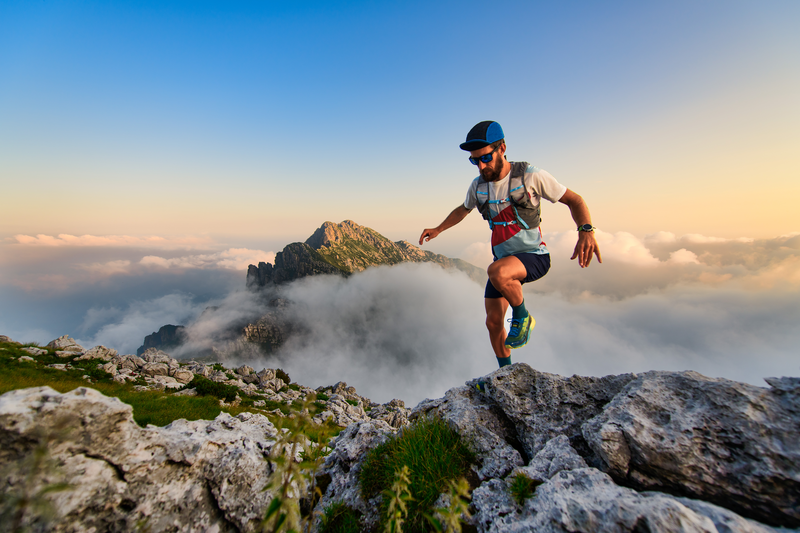
95% of researchers rate our articles as excellent or good
Learn more about the work of our research integrity team to safeguard the quality of each article we publish.
Find out more
MINI REVIEW article
Front. Immunol. , 26 May 2015
Sec. Antigen Presenting Cell Biology
Volume 6 - 2015 | https://doi.org/10.3389/fimmu.2015.00249
This article is part of the Research Topic Dendritic Cell and Macrophage nomenclature and classification View all 18 articles
As immune sentinels of the central nervous system (CNS), microglia not only respond rapidly to pathological conditions but also contribute to homeostasis in the healthy brain. In contrast to other populations of the myeloid lineage, adult microglia derive from primitive myeloid precursors that arise in the yolk sac early during embryonic development, after which they self-maintain locally and independently of blood-borne myeloid precursors. Under neuro-inflammatory conditions such as experimental autoimmune encephalomyelitis, circulating monocytes invade the CNS parenchyma where they further differentiate into macrophages or inflammatory dendritic cells. Often it is difficult to delineate resident microglia from infiltrating myeloid cells using currently known markers. Here, we will discuss the current means to reliably distinguish between these populations, and which recent advances have helped to make clear definitions between phenotypically similar, yet functionally diverse myeloid cell types.
Most tissues are populated by incredibly diverse and abundant myeloid cells. By contrast, the central nervous system (CNS) harbors comparatively few myeloid cell subsets. This is likely due to the immune privilege and relative isolation enjoyed by the CNS compared to other non-lymphoid tissues such as the gut or the lung, which are continually confronted with foreign entities. In the steady state, the CNS houses several populations of myeloid cells with distinct localizations including perivascular, choroid plexus, and meningeal macrophages/dendritic cells (DCs) and microglia, which are the most abundant (1). Microglia are considered the resident macrophages of the brain given that they are the only myeloid cells present in the CNS parenchyma. Microglia perform both homeostatic and immune-related functions and constitute about 5–20% of all cells in the CNS (2). They use their “ramified” morphology to act as immune sentinels, extending specialized processes, and sampling the local environment for foreign bodies (3, 4). Numerous recent reports have unmasked additional functions for microglia other than being simply the brain’s intrinsic immune system. For example, microglia are also critical for neuronal development, adult neurogenesis, learning-dependent synapse formation, and brain homeostasis (5–7). Microglia are classified as tissue resident macrophages but are clearly ontogenically distinct from other members of the mononuclear phagocyte system (MPS), which includes DCs, monocytes, and macrophages. Microglia originate from primitive macrophages that derive from erythro-myeloid precursors in the yolk sac (8–10). These primitive yolk sac macrophages colonize the developing brain in mice as early as embryonic day 9.5 (8). Throughout adult life microglia remain of embryonic origin in the healthy CNS and maintain themselves locally without any detectable contribution from circulating myeloid progenitors including monocytes. Yolk sac macrophages and microglia precursors in the developing brain express high levels of the fractalkine receptor (CX3CR1) and are positive for the integrin alpha M (Itgam, also know as CD11b; macrophage-1 antigen, Mac-1), F4/80, and the macrophage-colony stimulating factor receptor 1 (Csf-1R, CD115) similar to adult microglia as described below (8). Compared to adult microglia, however, microglia precursors are CD45hi. The development of microglia is dependent on Csf-1R (CD115), the transcription factors PU.1 and Irf8 but is independent of Myb, which is crucial for the development of hematopoietic stem cells (HSCs) (8, 9, 11, 12). In contrast to microglia, recent adoptive transfer and fate-mapping studies revealed that other macrophage populations are either embryonically derived from definitive hematopoiesis (e.g., alveolar or heart macrophages) or are constantly replaced by circulating monocytes (e.g., dermal or gut macrophages) (10, 13–18). Aside from the unique ontogeny of microglia within the MPS, a clear classification of microglia compared to other tissue macrophages in terms of phenotype and function has been difficult. Only recently, transcriptome and epigenetic analysis identified genes uniquely expressed and regulated by microglia but not by other macrophage populations (19–24). These studies might be useful to classify and distinguish microglia from other myeloid cells.
In steady state conditions, microglia express surface markers typically present on many other tissue macrophages and/or monocytes such as CD11b, F4/80, Fc-gamma receptor 1 (CD64), and CD115 (Csf-1R), ionized calcium-binding adapter molecule 1 (Iba-1) and proto-oncogene tyrosine-protein kinase MER (MerTK) (Figure 1) (19). In contrast to microglia, which are γ-irradiation resistant, perivascular myeloid cells are replaced by bone marrow (BM)-derived precursors after total-body irradiation and BM transplantation (25–28). However, the exact ontogeny of (non-microglia) myeloid cells associated with the CNS and whether they are also able to maintain themselves locally is, to date, not known (29). These perivascular cells are equipped to present antigen (varying levels of MHCII and CD11c). Whether they represent a homogeneous distinct population or a heterogeneous population of macrophages and/or DCs is not entirely resolved. In the past, a cell expressing F4/80 was deemed to be a macrophage, whereas a cell expressing CD11c was considered a DC. It is clear now that subsets of DCs can also express F4/80 and certain macrophage populations express CD11c. Upon Flt3L treatment, a CD11c+MHCII+ population in the meninges and choroid plexus expanded, which is indicative of the DC lineage whose development is dependent on Flt3L signaling (27, 28). In addition, a limited number of CD11c+ myeloid cells were also described to be in a juxtavascular location in the CNS parenchyma (30). These cells might, however, represent bona fide microglia expressing CD11c in certain regions of the brain. Further studies are required to dissect the ontogeny and characterize these elusive myeloid cells associated with the CNS in the steady state.
Figure 1. Central nervous system myeloid cells and their defining lineage markers. In the steady state and under inflammatory conditions, myeloid cells in the CNS express a diverse, yet overlapping set of markers commonly used to discriminate between MPS members.
As common to many other macrophage populations including microglia, most of the CNS-associated myeloid cells also express CD11b, CD115, Iba-1, and F4/80 (31). Therefore, apart from their location, the only available means to unequivocally distinguish microglia from other CNS-resident myeloid populations (CNS-associated macrophages/DCs) and circulating monocytes is by the reduced expression of the common leukocyte antigen CD45, which is readily detectable by flow cytometry. Adult microglia, unlike most other tissue macrophages, constitutively express high levels of the fractalkine receptor CX3CR1 (32). A major advance in the microglia field has been the generation of Cx3Cr1creER mice (32–34). This tamoxifen-inducible Cre-recombinase under the CX3CR1 promoter allows for microglia-specific gene targeting. Despite the fractalkine receptor being expressed by monocytes and myeloid precursors in the BM, microglia remain a self-contained population in the CNS and therefore remain targeted long after ceasing of tamoxifen administration, returning the short-lived, circulating myeloid cells to their wild-type origin.
Only recently, gene expression studies have identified surface markers and transcription factors specifically expressed by steady state microglia but not by other macrophage populations or monocytes. These include, for example, sialic acid-binding immunoglobulin-type lectin H (Siglec-H), Fc receptor-like S (Fcrls), and purinergic receptor P2Y G-protein coupled 12 (P2ry12) (20, 21). Furthermore, microglia seem to be the only hematopoietic cell population that specifically expresses Sal-like 1 (Sall1), a transcription factor that plays a crucial role in kidney development (35). While previous studies have reported expression of Sall1 only by stromal cells, in the adult CNS this factor is expressed exclusively by microglia. These aforementioned gene expression studies have compared the transcriptome of microglia to either macrophages derived from the spleen, the lung, the peritoneum, or to monocytes. Whether these microglia core signature markers are also expressed by CNS-associated macrophages/DCs remains to be shown.
In contrast to the healthy brain, during neuro-inflammation the picture becomes far more complicated. A hallmark of microglia is their rapid activation after a CNS insult, resulting in their migration toward injury, proliferation, and their change in morphology. They take on a more “amoeboid” shape with shorter and thicker processes, display increased immunoreactivity for Iba-1 and upregulate CD45. Experimental autoimmune encephalomyelitis (EAE), which is a mouse model for multiple sclerosis (MS), is characterized by infiltration of T cells, monocytes, and neutrophils. Monocytes and their progeny [macrophages/monocyte-derived DCs (moDCs)] are undoubtedly the prevailing cell type in the lesions (see below). However, activated microglia are also clearly detected in the vicinity of the inflammatory lesions. The downregulation of Ly6C by monocytes upon their differentiation adds complexity to the separation of these two distinct cell types based on the commonly used cell surface markers. Additionally, molecules involved in antigen presentation and T cell stimulation, which are barely detectable in steady state microglia, are expressed to some level by microglia already at disease onset and retain expression throughout disease progression. These markers include major histocompatibility complex class II (MHCII), CD11c (also known as integrin alpha X, Itgax), CD80 (B7-1), CD86 (B7-2), and CD40 (36–38). Under these conditions, it is considerably more difficult to distinguish these activated microglia from inflammatory monocyte-derived cells. Similar changes in microglia surface markers have been observed in mouse models of neurodegenerative disease such as Alzheimer’s disease (AD), Parkinson’s disease, and amyotrophic lateral sclerosis (ALS) (20, 31, 39).
A recent study, however, has used differentially expressed chemokine receptors on the surface of microglia and monocytes to distinguish those two myeloid populations and study their function during neuro-inflammation. Microglia were identified by their high expression of CX3CR1 whereas infiltrating monocytes, which subsequently differentiate into macrophages/DCs, were defined by their high expression of C–C chemokine receptor type 2 (CCR2), a receptor mediating monocyte recruitment to sites of inflammation. Gene expression profiles from macrophages versus “embryonically derived” microglia at different stages of EAE show that despite some similarities between these inflammatory cell types, microglia exhibit a distinct molecular signature (40). This genetic distinction reflects a different function of resident microglia and infiltrating monocytes under pathological circumstances. While monocyte-derived macrophages seemed to be the effector cell type causing CNS damage, microglia might have a regulatory function and could play a role in tissue repair and homeostasis (40). Another report also showed that monocytes recruited to the CNS in EAE do not acquire microglia-signature genes (21). These studies will unquestionably help attributing unique functions to microglia and CNS-invading myeloid cells in different pathological conditions in the brain.
Whether microglia-specific surface markers and transcription factors alter their expression between steady state and inflammation remains unclear. The microglia-specific ATP receptor P2ry12 was downregulated under inflammatory conditions such as lipopolysaccharide (LPS) systemic injection or in SOD1 mouse-model of ALS (41, 42). On the other hand, P2ry12 and Fcrls continue to be expressed by microglia in EAE but are not expressed by infiltrating monocytes (21). Therefore, further studies need to be undertaken in order to better characterize these “new” phenotypic microglia markers under pathological conditions.
Even though microglia homeostasis is maintained through local self-renewal, under certain conditions circulating precursors can give rise to microglia-like cells. For example, early studies using BM-chimeras showed that up to 10–20% of microglia were reconstituted by donor-derived cells 6-12 months after total-body irradiation and BM transplantation (43). However, this engraftment of BM-derived microglia can only be seen upon blood–brain-barrier (BBB) disruption (e.g. irradiation) and becomes minimal in models where the BBB is unperturbed (e.g. protection of the head during irradiation and parabiosis) (44, 45). This clearly indicates that under steady state conditions, monocytes or BM-derived myeloid progenitors do not infiltrate the CNS parenchyma and thus do not give rise to adult microglia. Similarly, as described above in experimental models of neuro-inflammation, monocytes infiltrate the CNS and differentiate into effector cells resembling phenotypically activated microglia. Despite these similarities, monocyte-derived cells do not persist in the CNS after inflammation has been resolved and thus do not contribute to long-lived microglia (46).
Finally, local administration of ganciclovir to transgenic mice expressing the thymidine kinase of herpes simplex virus under the CD11b promoter (CD11b-HSVTK) leads to a rapid depletion of microglia (47, 48). Subsequently, BM-derived cells enter the CNS and differentiate into long-lived microglia-like cells. Notably, while these cells form a network filling the niche for embryonically derived microglia, they do not obtain a complete microglia phenotype. Monocyte-derived “microglia” in this model show a less-ramified morphology and a higher expression of CD45 compared to yolk sac-derived microglia resembling more activated microglia (48). It has not yet been investigated whether these monocyte-derived “microglia” functionally resemble embryonically derived microglia or whether they acquire microglia-signature genes (Siglec-H, Fcrls, P2ry12) as described above.
Recent studies used a Csf-1R inhibitor to deplete microglia. Upon treatment stop, microglia were repopulated within 1 week (49, 50). These studies showed that new “microglia” were derived from CNS-resident nestin-positive precursors and resembled embryonically derived microglia in response to an inflammatory stimulus. Animals with newly repopulated “microglia” did not display any impairment in behavior, cognition, or motor function compared to control animals (50).
Brain inflammation or “encephalitis” invariably results in a reshaping of the myeloid cell populations inhabiting the CNS. An inflammatory response brought on by either infection or autoimmune manifestations results in a rapid increase in blood-derived cellularity to this otherwise dormant site. Despite EAE being fully dependent on T helper cells (51), the vast majority of the inflammatory infiltrate seen in EAE is of myeloid derivation. Two types of monocytes exist including the classical monocytes (Ly6ChiCCR2+CX3CR1lo) and the non-classical monocytes (Ly6CloCCR2−CX3CR1hi). Here, we will only discuss Ly6Chi monocytes given that during neuro-inflammation, this is the subset recruited to the brain. Engraftment of phagocytes derived from circulating CCR2+ monocytes has also been shown in an AD mouse model (39). Ly6Chi monocytes egress from the BM and cross the BBB in a CCR2-dependent manner (52, 53) followed by their differentiation into macrophages/moDCs and upregulation of a set of cell surface markers (e.g., MHCII, CD11c) expressed on a wide variety of MPS members. Likewise, microglia progressively alter their phenotype to resemble more classically activated macrophages during CNS inflammation, infection, and neuronal or myelin damage (54).
Ly6C+ monocytes were shown to migrate into the CNS prior to disease onset and precede the development of paralysis and subsequent clinical manifestations of EAE, when “DC-like” cells are found in abundance in the inflamed tissue (55, 56). This corresponds well with a previous report showing CD205+ myeloid cells accumulating in the meninges, choroid plexus, and subpial space of the spinal cord and in perivascular cuffs in demyelinating lesions during acute disease (57). CD11b+ DCs within the inflamed CNS were demonstrated to be critical for the propagation of EAE (27, 58, 59). Further phenotypical characterization would be required to demarcate their lineage whether they resemble moDCs or are more similar to classical DCs. Indeed, monocyte-derived antigen presenting cells (APCs) have been shown to be required for optimal priming of T cells in models of infection (60). Current evidence suggests that phenotypically similar macrophages in the CNS can not only contribute to the generation of inflammatory lesions and perform a pathogenic role in the demyelination process but also contribute to regenerative repair mechanisms to resolve inflammation (61, 62). These studies emphasize that distinct functions are attributed to the different subsets of myeloid cells in the course of a CNS inflammation. As such, a complete understanding of cell types based on surface phenotype alone would be of great benefit both in preclinical models of CNS inflammation and also in human patients.
Even with the knowledge we now possess on myeloid cell diversity, it is still commonplace in the literature using animal models of CNS inflammation to use a simplistic CD45hiCD11bhi gating strategy to separate CNS infiltrating, blood-derived myeloid cells from CNS-resident, embryonically derived microglia (CD45low) (63). Efforts to sort cells using a broad CD45hiCD11bhi surface phenotype from within the inflamed CNS will inevitably result in analysis of multiple cell types, lacking any of the desired specificity. Indeed, without the removal of Ly6Ghi cells during sorting, a mixed population is inevitable and expression profiles subsequently attributed to moDCs are either confused with, or heavily influenced by, an abundant neutrophil contamination. Even if the effort is taken to remove neutrophils, moDCs at various stages of development will be incorporated. This distinction is increasingly important given that both neutrophils and moDCs have been shown to mediate BBB permeability and demyelination, and that different pathogenic mechanisms are likely active in the two populations during the same inflammation (40, 64).
We know that at least four clearly distinct cell types share this rather non-specific CD45hiCD11bhi surface phenotype in an inflamed CNS, namely neutrophils (CD11b+Ly6G+), monocytes (CD11b+Ly6ChiCX3CR1low), and their progeny such as moDCs and/or activated macrophages (Figure 1). The latter two cell types represent most likely the same population with just different names assigned by different studies. Ly6Chi monocytes that have migrated into the CNS can further be subdivided into numerous differentiation stages characterized by the upregulation of CD11c and MHCII, with the concomitant downregulation of Ly6C and CCR2. Upon differentiation and upregulation of MHCII, monocytes are then called moDCs/activated macrophages. Thus, moDCs in the CNS are characterized by the expression of CD11b+F4/80+MHCII+CD11cintLy6C+/−. These moDCs/activated macrophages also express CD64 and likely also MerTK, which both are universally expressed by tissue macrophages including microglia (19). Interestingly, it has been shown that monocytes recruited to the CNS during EAE do not express the newly identified microglia markers Fcrls and P2ry12 highlighting again the diverse ontogeny of these cell types and suggesting that the microglia-signature genes are indeed specific to microglia rather than location (CNS) specific (21). The FcεRIα (MAR-1) has been suggested to represent a moDC marker. Whether moDCs in the inflamed CNS express MAR-1 has so far not been analyzed (65). Perhaps a more functional distinction should be drawn on the level of relevance for the inflammatory process to persist. CNS-infiltrating myeloid cells with DC-like morphology express MHCII, CD40, and CD86, all of which have critical roles in multiple inflammatory models (66). The CD86/CD28 interaction between T cells and APCs is of critical importance for T cell activation. Furthermore, the CD40/CD40L interaction induces a maturation pathway within the inflamed CNS, resulting in further costimulatory capabilities and proinflammatory cytokine expression (67, 68). The levels of CD40 on monocyte-derived cells in the inflamed CNS are variable but generally not as high as on classical DCs.
After activation, inflammatory macrophages can not only express a wide range of inflammatory cytokines but also oxygen-based chemically reactive molecules involved in host defense. The route an activated macrophage takes depends largely on the T cell and/or NK cell-derived cytokines present during their activation. For example, activation in the presence of LPS and IFN-γ leads to a “classical” activation (often called “M1”), resulting in secretion of high levels of TNF-α, iNOS, IL-1, IL-6, and IL-12. Conversely, activation of the same cells in the presence of IL-4 and IL-10 will result in rapid upregulation of IL-10, production of Arginase-1, and upregulation of the mannose receptor CD206, generating a macrophage capable of suppressing T cell activity (called “M2”) (69). This intracellular divergence in phenotype illustrates that an apparently similar cell expressing F4/80, CD64, CD11b, MHCII, and CD11c on its surface may, in fact, differ greatly in its function. Indeed, markers identifying both M1 and M2 macrophage populations have been shown synergistically in CNS biopsies obtained from MS patients. CD40, CD64, CD86, and CD32, mannose receptor and CD163 were co-expressed in the large majority of foamy macrophages found in lesional CNS (70). Therefore, surface characterization of inflammatory macrophages would appear insufficient and may mask different macrophage populations in direct opposition to each other, depending on the type of inflammation taking place. Generally in the steady state, tissue macrophages display an “M2-like” phenotype and are critical for tissue homeostasis. Interestingly, in a model of spinal cord injury, it was shown that M2 macrophages (CD11b+F4/80+CX3CR1hiLy6Clo) are beneficial and promote recovery (62).
Under steady state conditions, site specific and phenotypic characteristics exist to distinguish between microglia and other CNS-associated macrophages. As with almost all innate and adaptive immune cell types, consensus with respect to nomenclature in CNS-resident versus CNS-infiltrating myeloid cells has not been effectively reached under inflammatory conditions. The advent of microarray technology and next generation sequencing will serve to provide more useful ways to distinguish between these two apparently similar, yet ever more functionally diverse cell types. An ever-increasing variety of previously unappreciated, and non-immune homeostatic functions performed by macrophages are now beginning to emerge, making a more detailed separation of these cell types highly desirable (71). Ultimately, better characterization and dissection of the various myeloid cells in an inflamed brain will help deciphering the specialized functions of the different members of the MPS in pathological conditions.
The authors declare that the research was conducted in the absence of any commercial or financial relationships that could be construed as a potential conflict of interest.
1. Katsumoto A, Lu H, Miranda AS, Ransohoff RM. Ontogeny and functions of central nervous system macrophages. J Immunol (2014) 193:2615–21. doi: 10.4049/jimmunol.1400716
2. Lawson LJ, Perry VH, Dri P, Gordon S. Heterogeneity in the distribution and morphology of microglia in the normal adult mouse brain. Neuroscience (1990) 39:151–70. doi:10.1016/0306-4522(90)90229-W
3. Davalos D, Grutzendler J, Yang G, Kim JV, Zuo Y, Jung S, et al. ATP mediates rapid microglial response to local brain injury in vivo. Nat Neurosci (2005) 8:752–8. doi:10.1038/nn1472
4. Nimmerjahn A, Kirchhoff F, Helmchen F. Resting microglial cells are highly dynamic surveillants of brain parenchyma in vivo. Science (2005) 308:1314–8. doi:10.1126/science.1110647
5. Paolicelli RC, Bolasco G, Pagani F, Maggi L, Scianni M, Panzanelli P, et al. Synaptic pruning by microglia is necessary for normal brain development. Science (2011) 333:1456–8. doi:10.1126/science.1202529
6. Schafer DP, Lehrman EK, Kautzman AG, Koyama R, Mardinly AR, Yamasaki R, et al. Microglia sculpt postnatal neural circuits in an activity and complement-dependent manner. Neuron (2012) 74:691–705. doi:10.1016/j.neuron.2012.03.026
7. Zhan Y, Paolicelli RC, Sforazzini F, Weinhard L, Bolasco G, Pagani F, et al. Deficient neuron-microglia signaling results in impaired functional brain connectivity and social behavior. Nat Neurosci (2014) 17:400–6. doi:10.1038/nn.3641
8. Ginhoux F, Greter M, Leboeuf M, Nandi S, See P, Gokhan S, et al. Fate mapping analysis reveals that adult microglia derive from primitive macrophages. Science (2010) 330:841–5. doi:10.1126/science.1194637
9. Kierdorf K, Erny D, Goldmann T, Sander V, Schulz C, Perdiguero EG, et al. Microglia emerge from erythromyeloid precursors via Pu.1- and Irf8-dependent pathways. Nat Neurosci (2013) 16:273–80. doi:10.1038/nn.3318
10. Perdiguero EG, Klapproth K, Schulz C, Busch K, Azzoni E, Crozet L, et al. Tissue-resident macrophages originate from yolk-sac-derived erythro-myeloid progenitors. Nature (2014) 518(7540):547–51. doi:10.1038/nature13989
11. Schulz C, Gomez Perdiguero E, Chorro L, Szabo-Rogers H, Cagnard N, Kierdorf K, et al. A lineage of myeloid cells independent of Myb and hematopoietic stem cells. Science (2012) 336:86–90. doi:10.1126/science.1219179
12. Shiau CE, Kaufman Z, Meireles AM, Talbot WS. Differential requirement for irf8 in formation of embryonic and adult macrophages in zebrafish. PLoS One (2015) 10:e0117513. doi:10.1371/journal.pone.0117513
13. Varol C, Landsman L, Fogg DK, Greenshtein L, Gildor B, Margalit R, et al. Monocytes give rise to mucosal, but not splenic, conventional dendritic cells. J Exp Med (2007) 204:171–80. doi:10.1084/jem.20061011
14. Bogunovic M, Ginhoux F, Helft J, Shang L, Hashimoto D, Greter M, et al. Origin of the lamina propria dendritic cell network. Immunity (2009) 31:513–25. doi:10.1016/j.immuni.2009.08.010
15. Guilliams M, De Kleer I, Henri S, Post S, Vanhoutte L, De Prijck S, et al. Alveolar macrophages develop from fetal monocytes that differentiate into long-lived cells in the first week of life via GM-CSF. J Exp Med (2013) 210:1977–92. doi:10.1084/jem.20131199
16. Bain CC, Bravo-Blas A, Scott CL, Gomez Perdiguero E, Geissmann F, Henri S, et al. Constant replenishment from circulating monocytes maintains the macrophage pool in the intestine of adult mice. Nat Immunol (2014) 15:929–37. doi:10.1038/ni.2967
17. Epelman S, Lavine KJ, Beaudin AE, Sojka DK, Carrero JA, Calderon B, et al. Embryonic and adult-derived resident cardiac macrophages are maintained through distinct mechanisms at steady state and during inflammation. Immunity (2014) 40:91–104. doi:10.1016/j.immuni.2013.11.019
18. Hoeffel G, Chen J, Lavin Y, Low D, Almeida FF, See P, et al. C-myb(+) erythro-myeloid progenitor-derived fetal monocytes give rise to adult tissue-resident macrophages. Immunity (2015) 42:665–78. doi:10.1016/j.immuni.2015.03.011
19. Gautier EL, Shay T, Miller J, Greter M, Jakubzick C, Ivanov S, et al. Gene-expression profiles and transcriptional regulatory pathways that underlie the identity and diversity of mouse tissue macrophages. Nat Immunol (2012) 13:1118–28. doi:10.1038/ni.2419
20. Chiu IM, Morimoto ET, Goodarzi H, Liao JT, O’Keeffe S, Phatnani HP, et al. A neurodegeneration-specific gene-expression signature of acutely isolated microglia from an amyotrophic lateral sclerosis mouse model. Cell Rep (2013) 4:385–401. doi:10.1016/j.celrep.2013.06.018
21. Butovsky O, Jedrychowski MP, Moore CS, Cialic R, Lanser AJ, Gabriely G, et al. Identification of a unique TGF-beta-dependent molecular and functional signature in microglia. Nat Neurosci (2014) 17:131–43. doi:10.1038/nn.3599
22. Gosselin D, Link VM, Romanoski CE, Fonseca GJ, Eichenfield DZ, Spann NJ, et al. Environment drives selection and function of enhancers controlling tissue-specific macrophage identities. Cell (2014) 159:1327–40. doi:10.1016/j.cell.2014.11.023
23. Lavin Y, Winter D, Blecher-Gonen R, David E, Keren-Shaul H, Merad M, et al. Tissue-resident macrophage enhancer landscapes are shaped by the local microenvironment. Cell (2014) 159:1312–26. doi:10.1016/j.cell.2014.11.018
24. Zeisel A, Munoz-Manchado AB, Codeluppi S, Lonnerberg P, La Manno G, Jureus A, et al. Brain structure. Cell types in the mouse cortex and hippocampus revealed by single-cell RNA-seq. Science (2015) 347:1138–42. doi:10.1126/science.aaa1934
25. Hickey WF, Kimura H. Perivascular microglial cells of the CNS are bone marrow-derived and present antigen in vivo. Science (1988) 239:290–2. doi:10.1126/science.3276004
26. Lassmann H, Hickey WF. Radiation bone marrow chimeras as a tool to study microglia turnover in normal brain and inflammation. Clin Neuropathol (1993) 12:284–5.
27. Greter M, Heppner FL, Lemos MP, Odermatt BM, Goebels N, Laufer T, et al. Dendritic cells permit immune invasion of the CNS in an animal model of multiple sclerosis. Nat Med (2005) 11:328–34. doi:10.1038/nm1197
28. Anandasabapathy N, Victora GD, Meredith M, Feder R, Dong B, Kluger C, et al. Flt3L controls the development of radiosensitive dendritic cells in the meninges and choroid plexus of the steady-state mouse brain. J Exp Med (2011) 208:1695–705. doi:10.1084/jem.20102657
29. Hashimoto D, Chow A, Noizat C, Teo P, Beasley MB, Leboeuf M, et al. Tissue-resident macrophages self-maintain locally throughout adult life with minimal contribution from circulating monocytes. Immunity (2013) 38:792–804. doi:10.1016/j.immuni.2013.04.004
30. Prodinger C, Bunse J, Kruger M, Schiefenhovel F, Brandt C, Laman JD, et al. CD11c-expressing cells reside in the juxtavascular parenchyma and extend processes into the glia limitans of the mouse nervous system. Acta Neuropathol (2011) 121:445–58. doi:10.1007/s00401-010-0774-y
31. Prinz M, Priller J, Sisodia SS, Ransohoff RM. Heterogeneity of CNS myeloid cells and their roles in neurodegeneration. Nat Neurosci (2011) 14:1227–35. doi:10.1038/nn.2923
32. Yona S, Kim KW, Wolf Y, Mildner A, Varol D, Breker M, et al. Fate mapping reveals origins and dynamics of monocytes and tissue macrophages under homeostasis. Immunity (2013) 38:79–91. doi:10.1016/j.immuni.2012.12.001
33. Goldmann T, Wieghofer P, Muller PF, Wolf Y, Varol D, Yona S, et al. A new type of microglia gene targeting shows TAK1 to be pivotal in CNS autoimmune inflammation. Nat Neurosci (2013) 16:1618–26. doi:10.1038/nn.3531
34. Parkhurst CN, Yang G, Ninan I, Savas JN, Yates JR III, Lafaille JJ, et al. Microglia promote learning-dependent synapse formation through brain-derived neurotrophic factor. Cell (2013) 155:1596–609. doi:10.1016/j.cell.2013.11.030
35. Nishinakamura R, Matsumoto Y, Nakao K, Nakamura K, Sato A, Copeland NG, et al. Murine homolog of SALL1 is essential for ureteric bud invasion in kidney development. Development (2001) 128:3105–15.
36. Juedes AE, Ruddle NH. Resident and infiltrating central nervous system APCs regulate the emergence and resolution of experimental autoimmune encephalomyelitis. J Immunol (2001) 166:5168–75. doi:10.4049/jimmunol.166.8.5168
37. Ponomarev ED, Shriver LP, Maresz K, Dittel BN. Microglial cell activation and proliferation precedes the onset of CNS autoimmunity. J Neurosci Res (2005) 81:374–89. doi:10.1002/jnr.20488
38. Almolda B, Gonzalez B, Castellano B. Antigen presentation in EAE: role of microglia, macrophages and dendritic cells. Front Biosci (2011) 16:1157–71. doi:10.2741/3781
39. Mildner A, Schlevogt B, Kierdorf K, Bottcher C, Erny D, Kummer MP, et al. Distinct and non-redundant roles of microglia and myeloid subsets in mouse models of Alzheimer’s disease. J Neurosci (2011) 31:11159–71. doi:10.1523/JNEUROSCI.6209-10.2011
40. Yamasaki R, Lu H, Butovsky O, Ohno N, Rietsch AM, Cialic R, et al. Differential roles of microglia and monocytes in the inflamed central nervous system. J Exp Med (2014) 211:1533–49. doi:10.1084/jem.20132477
41. Haynes SE, Hollopeter G, Yang G, Kurpius D, Dailey ME, Gan WB, et al. The P2Y12 receptor regulates microglial activation by extracellular nucleotides. Nat Neurosci (2006) 9:1512–9. doi:10.1038/nn1805
42. Butovsky O, Jedrychowski MP, Cialic R, Krasemann S, Murugaiyan G, Fanek Z, et al. Targeting miR-155 restores abnormal microglia and attenuates disease in SOD1 mice. Ann Neurol (2015) 77:75–99. doi:10.1002/ana.24304
43. Kennedy DW, Abkowitz JL. Kinetics of central nervous system microglial and macrophage engraftment: analysis using a transgenic bone marrow transplantation model. Blood (1997) 90(3):986–93.
44. Ajami B, Bennett JL, Krieger C, Tetzlaff W, Rossi FM. Local self-renewal can sustain CNS microglia maintenance and function throughout adult life. Nat Neurosci (2007) 10:1538–43. doi:10.1038/nn2014
45. Mildner A, Schmidt H, Nitsche M, Merkler D, Hanisch UK, Mack M, et al. Microglia in the adult brain arise from Ly-6ChiCCR2+ monocytes only under defined host conditions. Nat Neurosci (2007) 10:1544–53. doi:10.1038/nn2015
46. Ajami B, Bennett JL, Krieger C, McNagny KM, Rossi FM. Infiltrating monocytes trigger EAE progression, but do not contribute to the resident microglia pool. Nat Neurosci (2011) 14:1142–9. doi:10.1038/nn.2887
47. Heppner FL, Greter M, Marino D, Falsig J, Raivich G, Hovelmeyer N, et al. Experimental autoimmune encephalomyelitis repressed by microglial paralysis. Nat Med (2005) 11:146–52. doi:10.1038/nm0405-455
48. Varvel NH, Grathwohl SA, Baumann F, Liebig C, Bosch A, Brawek B, et al. Microglial repopulation model reveals a robust homeostatic process for replacing CNS myeloid cells. Proc Natl Acad Sci U S A (2012) 109:18150–5. doi:10.1073/pnas.1210150109
49. Elmore MR, Najafi AR, Koike MA, Dagher NN, Spangenberg EE, Rice RA, et al. Colony-stimulating factor 1 receptor signaling is necessary for microglia viability, unmasking a microglia progenitor cell in the adult brain. Neuron (2014) 82:380–97. doi:10.1016/j.neuron.2014.02.040
50. Elmore MR, Lee RJ, West BL, Green KN. Characterizing newly repopulated microglia in the adult mouse: impacts on animal behavior, cell morphology, and neuroinflammation. PLoS One (2015) 10:e0122912. doi:10.1371/journal.pone.0122912
51. Schreiner B, Heppner FL, Becher B. Modeling multiple sclerosis in laboratory animals. Semin Immunopathol (2009) 31:479–95. doi:10.1007/s00281-009-0181-4
52. Fife BT, Huffnagle GB, Kuziel WA, Karpus WJ. CC chemokine receptor 2 is critical for induction of experimental autoimmune encephalomyelitis. J Exp Med (2000) 192:899–905. doi:10.1084/jem.192.6.899
53. Gaupp S, Pitt D, Kuziel WA, Cannella B, Raine CS. Experimental autoimmune encephalomyelitis (EAE) in CCR2(-/-) mice: susceptibility in multiple strains. Am J Pathol (2003) 162:139–50. doi:10.1016/S0002-9440(10)63805-9
54. Kreutzberg GW. Microglia: a sensor for pathological events in the CNS. Trends Neurosci (1996) 19:312–8. doi:10.1016/0166-2236(96)10049-7
55. King IL, Dickendesher TL, Segal BM. Circulating Ly-6C+ myeloid precursors migrate to the CNS and play a pathogenic role during autoimmune demyelinating disease. Blood (2009) 113:3190–7. doi:10.1182/blood-2008-07-168575
56. Mildner A, Mack M, Schmidt H, Bruck W, Djukic M, Zabel MD, et al. CCR2+Ly-6Chi monocytes are crucial for the effector phase of autoimmunity in the central nervous system. Brain (2009) 132:2487–500. doi:10.1093/brain/awp144
57. Serafini B, Columba-Cabezas S, Di Rosa F, Aloisi F. Intracerebral recruitment and maturation of dendritic cells in the onset and progression of experimental autoimmune encephalomyelitis. Am J Pathol (2000) 157:1991–2002. doi:10.1016/S0002-9440(10)64838-9
58. McMahon EJ, Bailey SL, Castenada CV, Waldner H, Miller SD. Epitope spreading initiates in the CNS in two mouse models of multiple sclerosis. Nat Med (2005) 11:335–9. doi:10.1038/nm1202
59. Bailey SL, Schreiner B, McMahon EJ, Miller SD. CNS myeloid DCs presenting endogenous myelin peptides ‘preferentially’ polarize CD4+ T(H)-17 cells in relapsing EAE. Nat Immunol (2007) 8:172–80. doi:10.1038/ni1430
60. Schreiber HA, Loschko J, Karssemeijer RA, Escolano A, Meredith MM, Mucida D, et al. Intestinal monocytes and macrophages are required for T cell polarization in response to Citrobacter rodentium. J Exp Med (2013) 210:2025–39. doi:10.1084/jem.20130903
61. Kigerl KA, Gensel JC, Ankeny DP, Alexander JK, Donnelly DJ, Popovich PG. Identification of two distinct macrophage subsets with divergent effects causing either neurotoxicity or regeneration in the injured mouse spinal cord. J Neurosci (2009) 29:13435–44. doi:10.1523/JNEUROSCI.3257-09.2009
62. Shechter R, Miller O, Yovel G, Rosenzweig N, London A, Ruckh J, et al. Recruitment of beneficial M2 macrophages to injured spinal cord is orchestrated by remote brain choroid plexus. Immunity (2013) 38:555–69. doi:10.1016/j.immuni.2013.02.012
63. Vainchtein ID, Vinet J, Brouwer N, Brendecke S, Biagini G, Biber K, et al. In acute experimental autoimmune encephalomyelitis, infiltrating macrophages are immune activated, whereas microglia remain immune suppressed. Glia (2014) 62:1724–35. doi:10.1002/glia.22711
64. Aube B, Levesque SA, Pare A, Chamma E, Kebir H, Gorina R, et al. Neutrophils mediate blood-spinal cord barrier disruption in demyelinating neuroinflammatory diseases. J Immunol (2014) 193:2438–54. doi:10.4049/jimmunol.1400401
65. Plantinga M, Guilliams M, Vanheerswynghels M, Deswarte K, Branco-Madeira F, Toussaint W, et al. Conventional and monocyte-derived CD11b(+) dendritic cells initiate and maintain T helper 2 cell-mediated immunity to house dust mite allergen. Immunity (2013) 38:322–35. doi:10.1016/j.immuni.2012.10.016
66. Banchereau J, Steinman RM. Dendritic cells and the control of immunity. Nature (1998) 392:245–52. doi:10.1038/32588
67. Stout RD, Suttles J, Xu J, Grewal IS, Flavell RA. Impaired T cell-mediated macrophage activation in CD40 ligand-deficient mice. J Immunol (1996) 156:8–11.
68. Becher B, Durell BG, Miga AV, Hickey WF, Noelle RJ. The clinical course of experimental autoimmune encephalomyelitis and inflammation is controlled by the expression of CD40 within the central nervous system. J Exp Med (2001) 193:967–74. doi:10.1084/jem.193.8.967
69. Mosser DM, Edwards JP. Exploring the full spectrum of macrophage activation. Nat Rev Immunol (2008) 8:958–69. doi:10.1038/nri2448
70. Vogel DY, Vereyken EJ, Glim JE, Heijnen PD, Moeton M, Van Der Valk P, et al. Macrophages in inflammatory multiple sclerosis lesions have an intermediate activation status. J Neuroinflammation (2013) 10:35. doi:10.1186/1742-2094-10-35
Keywords: microglia, macrophage, monocyte, dendritic cell, CNS inflammation
Citation: Greter M, Lelios I and Croxford AL (2015) Microglia versus myeloid cell nomenclature during brain inflammation. Front. Immunol. 6:249. doi: 10.3389/fimmu.2015.00249
Received: 26 February 2015; Accepted: 07 May 2015;
Published: 26 May 2015
Edited by:
Martin Guilliams, Ghent University – VIB, BelgiumReviewed by:
Steffen Jung, Weizmann Institute of Science, IsraelCopyright: © 2015 Greter, Lelios and Croxford. This is an open-access article distributed under the terms of the Creative Commons Attribution License (CC BY). The use, distribution or reproduction in other forums is permitted, provided the original author(s) or licensor are credited and that the original publication in this journal is cited, in accordance with accepted academic practice. No use, distribution or reproduction is permitted which does not comply with these terms.
*Correspondence: Melanie Greter, Institute of Experimental Immunology, University of Zurich, Winterthurerstrasse 190, Zurich 8057, Switzerland,Z3JldGVyQGltbXVub2xvZ3kudXpoLmNo
Disclaimer: All claims expressed in this article are solely those of the authors and do not necessarily represent those of their affiliated organizations, or those of the publisher, the editors and the reviewers. Any product that may be evaluated in this article or claim that may be made by its manufacturer is not guaranteed or endorsed by the publisher.
Research integrity at Frontiers
Learn more about the work of our research integrity team to safeguard the quality of each article we publish.