- 1Molecular Medicine Group, Robarts Research Institute, Western University, London, ON, Canada
- 2Department of Clinical Neurological Sciences, Schulich School of Medicine & Dentistry, Western University, London, ON, Canada
Small GTPases participate in a broad range of cellular processes such as proliferation, differentiation, and migration. The exchange of GDP for GTP resulting in the activation of these GTPases is catalyzed by a group of enzymes called guanine nucleotide exchange factors (GEFs), of which two classes: Dbl-related exchange factors and the more recently described dedicator of cytokinesis proteins family exchange factors. Increasingly, deregulation of normal GEF activity or function has been associated with a broad range of disease states, including neurodegeneration and neurodevelopmental disorders. In this review, we examine this evidence with special emphasis on the novel role of Rho guanine nucleotide exchange factor (RGNEF/p190RhoGEF) in the pathogenesis of amyotrophic lateral sclerosis. RGNEF is the first neurodegeneration-linked GEF that regulates not only RhoA GTPase activation but also functions as an RNA binding protein that directly acts with low molecular weight neurofilament mRNA 3′ untranslated region to regulate its stability. This dual role for RGNEF, coupled with the increasing understanding of the key role for GEFs in modulating the GTPase function in cell survival suggests a prominent role for GEFs in mediating a critical balance between cytotoxicity and neuroprotection which, when disturbed, contributes to neuronal loss.
Introduction
Small GTPases are low-molecular-weight (approximately 21 kDa) guanine nucleotide-binding proteins that function as binary molecular switches by alternating between an active GTP-bound state and an inactive GDP-bound state and in doing so, regulate the activation of several effectors (Figure 1). The five subfamilies of small GTPases (Ras, Rho, Rab, Sar1/Arf, and Ran) have highly diverse cellular roles. For example, GTPases of the Rho family (including Rho proteins, Rac proteins, Cdc42, TC10, TCL, Wrch1, Chp/Wrch2, and Rnd proteins; Van Aelst and D’Souza-Schorey, 1997; Burridge and Wennerberg, 2004) work through several effectors to influence a broad range of cellular processes, including cell cycle progression, vesicular trafficking, cell migration, intracellular actin dynamics, cell–cell interactions, response to cellular injury, axonal development, and guidance and neurite formation (Govek et al., 2005; Thumkeo et al., 2013). GTPases of the Rab family, which includes at least 66 different proteins (Klopper et al., 2012), function as regulators of specific intracellular traffic pathways, coordinating consecutive stages of transport, such as vesicle formation, vesicle and organelle motility, and tethering of vesicles to their target compartment (Zerial and McBride, 2001). Rab GTPases have important roles in neurons such as participating in synaptic vesicles fusion and endocytosis, axonal and dendritic transport, and neurite formation (D’Adamo et al., 2014; Villarroel-Campos et al., 2014).
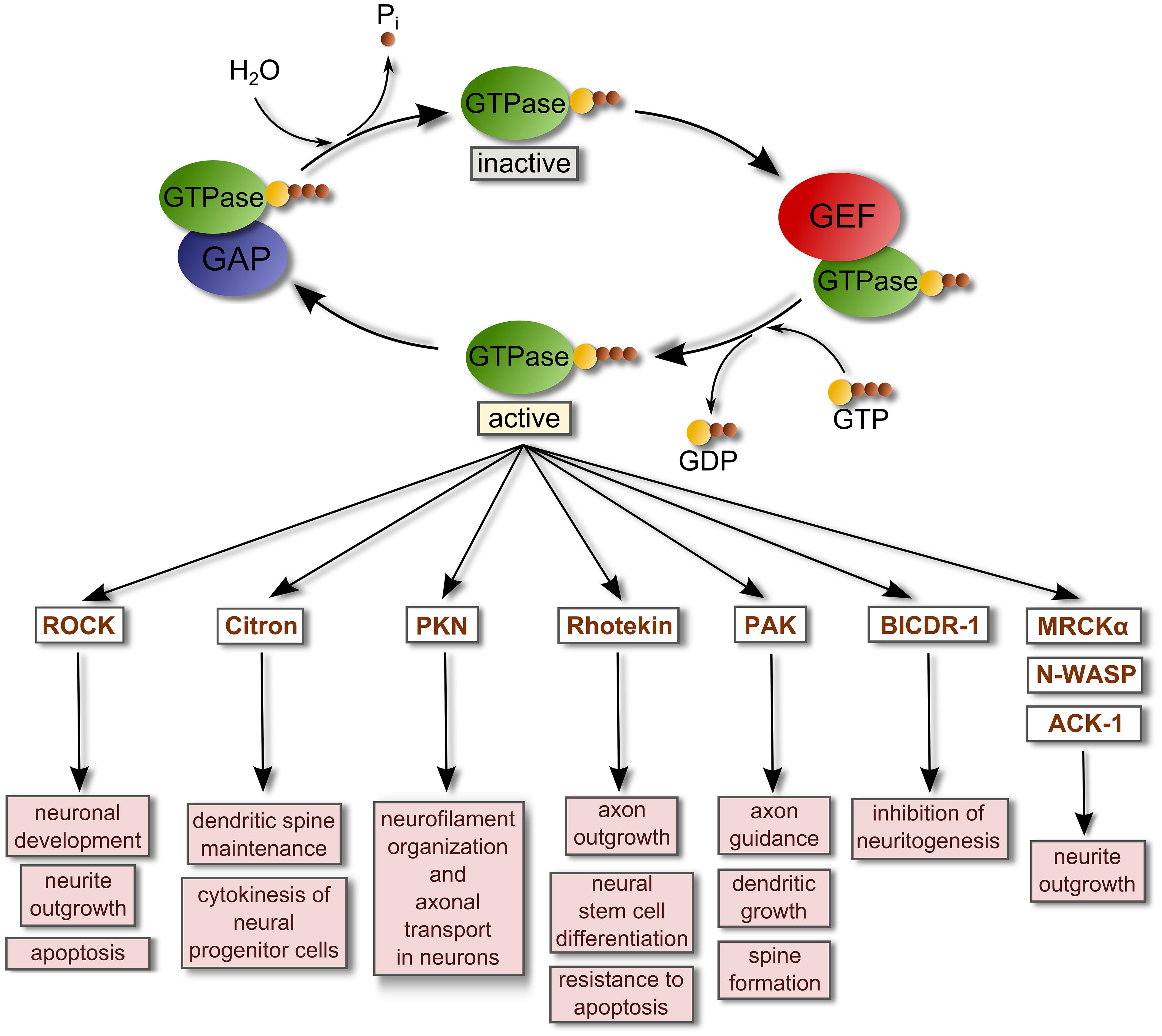
FIGURE 1. Regulation of small GTPase activity by GEFs and GAPs. Small GTPases are inactive when they are GDP-bound. GEFs bind and stabilize GDP-bound GTPases. However, due to the relatively high concentration of intracellular GTP these complexes rapidly dissociate into GTP-bound GTPases and free GEFs. When they are GTP-bound, GTPases regulate the activity of their binding targets or effectors to promote several cellular responses. GTPase-activating proteins (GAPs) stimulate the intrinsic hydrolytic capacity of Rho GTPases to promote GDP-bound forms, deactivating the GTPases and terminating the signaling. This reaction generates inorganic phosphate (Pi). A list of representative effectors of active small GTPases related with the regulation of the nervous system is shown (Govek et al., 2005; Thumkeo et al., 2013; Villarroel-Campos et al., 2014). Abbreviations used are: ACK-1, Activated Cdc42-associated kinase 1; BICDR-1, Bicaudal-D-related protein 1; MRCKα, Myotonic dystrophy kinase-related Cdc42-binding kinase alpha; N-WASP, Neural Wiskott-Aldrich syndrome protein; PAK, P21 protein (Cdc42/Rac)-activated kinase; PKN, Serine-threonine protein kinase N; ROCK, Rho-associated coiled-coil containing protein kinase.
The activation of small GTPases such as Rho or Rab proteins requires the participation of specialized enzymes called guanine nucleotide exchange factors (GEFs) which catalyze the exchange of GTP for GDP in the GTPase with the requirement of Mg2+. This event generates a GTP-bound active GTPase. Most GEFs belong to two broad families: the classical diffuse B-cell lymphoma (Dbl)-homology family in which the catalytic function is exerted by a tandem of two domains [a Dbl-homology domain (DH) and an adjacent pleckstrin-homology domain (PH) that together bind other proteins and phospholipids; Snyder et al., 2002; Rossman et al., 2005; Miller et al., 2014]; and, the dedicator of cytokinesis proteins (Dock) family of atypical GEFs that lack the Dbl domain. The highly conserved DH domain of the Dbl family of GEFs targets Dbl GEFs to the plasma membrane where binding to phospholipids is driven by the PH domain, while the DH domain interacts with inactive GTPase-GDP and catalyzes the exchange of GDP for GTP, activating the GTPase. Conversely, GTPase-activating proteins (GAPs) stimulate the intrinsic hydrolytic capacity of small GTPases to promote GDP-bound forms, deactivating the GTPases, and terminating signaling (Rossman et al., 2005; Lemmon, 2008; Figure 1).
Dbl GEFs are a highly complex family of about 80 proteins containing a variety of differing functional domains, including: (1) domains that modulate protein–protein interactions such as Proline rich domains (Ahn and Ye, 2005), PDZ binding domains (Garcia-Mata and Burridge, 2007), SH3 domains (van Rijssel and van Buul, 2012) and Fibronectin type-III domains (Rabiner et al., 2005); (2) modulators of GEF activity such as APC-binding region domains (Zhang et al., 2012) and Calponin homology domains (part of the actin binding domain superfamily; Yu et al., 2010); (3) modulators of plasma membrane interactions and localization such as FAK recognition domains (Zhai et al., 2003), CRAL-TRIO (SEC14) domains (van Rijssel and van Buul, 2012) and FERM domains (Kubo et al., 2002); and (4) modulators of protein structure/function such as Spectrin repeats (van Rijssel and van Buul, 2012), Zn binding domains (Gebbink et al., 1997), RGSL domains (Bielnicki et al., 2011), PDZ domains (Garcia-Mata and Burridge, 2007), and Leucine-rich domains (Gebbink et al., 1997). While perturbations in the Dbl GEF family have been best explored in cancer (Lazer and Katzav, 2011), they are also associated with a broad range of neurological disorders, including neurodegeneration.
The Dock proteins are atypical GEFs in that they lack the DH-PH module and instead contain a Dock homology region (DHR)1-DHR2 module (Figure 2). This module functions similarly to the DH-PH module in that DHR1 mediates phospholipid binding and membrane targeting of Docks while DHR2 catalyzes nucleotide exchange through a mechanism distinct from the Dbl domain. The Dock proteins are further differentiated from the classical GEFs in that they exhibit specificity for activation of Rac and/or Cdc42, but not RhoA or other members of the Rho family. Eleven Dock proteins have been described in mammals (Dock1–11; Table 1). They are divided into four subfamilies based on sequence homology: Dock-A (includes Dock1/180, 2 and 5); Dock-B (includes Dock3, 4); Dock-C (also known as the zizimin-related or zir family, includes Dock6, 7, 8), and Dock-D (also known as the zizimin family, includes Dock9, 10, 11). Dock-A and -B members contain both a Src homology 3 (SH3) domain in the N-terminal region that can bind to the adaptor proteins ELMO (Engulfment and Motility) 1, 2, and 3 in addition to a proline-rich domain in C-terminus portion that binds Crk proteins. Dock-D members contain an N-terminal PH domain. Conversely, Dock-C members lack other recognizable domains outside of the DHR1–DHR2 module (Figure 2; Cote and Vuori, 2002; Meller et al., 2005). There is increasing evidence to suggest that perturbations in the function of both classical and atypical GEFs are associated with a broad range of disease states, including neurodevelopmental and neuropsychiatric disorders and neurodegeneration. Although neurodegeneration is a broad term generally used to describe a heterogeneous group of pathologies sharing the progressive loss of neuronal function and the death of neurons, one of their core neuropathological features is the presence of intra and extracellular protein aggregates (DiFiglia et al., 1997; Spillantini et al., 1997; Sieradzan et al., 1999; Goedert, 2001; Tiraboschi et al., 2004; Strong et al., 2005; Bloom, 2014). Classical examples of such neurodegenerative disorders include Alzheimer’s disease (AD), Parkinson’s disease (PD), Huntington’s disease (HD), and amyotrophic lateral sclerosis (ALS). In many of these disorders, GEF proteins are increasingly recognized as determinants of both cell survival and neurodegeneration. In this review, we examine the evidence for both classical and atypical GEFs in neurodegeneration, with a specific focus on the recently identified role of Rho guanine nucleotide exchange factor (RGNEF/p190RhoGEF) in the pathogenesis of ALS.
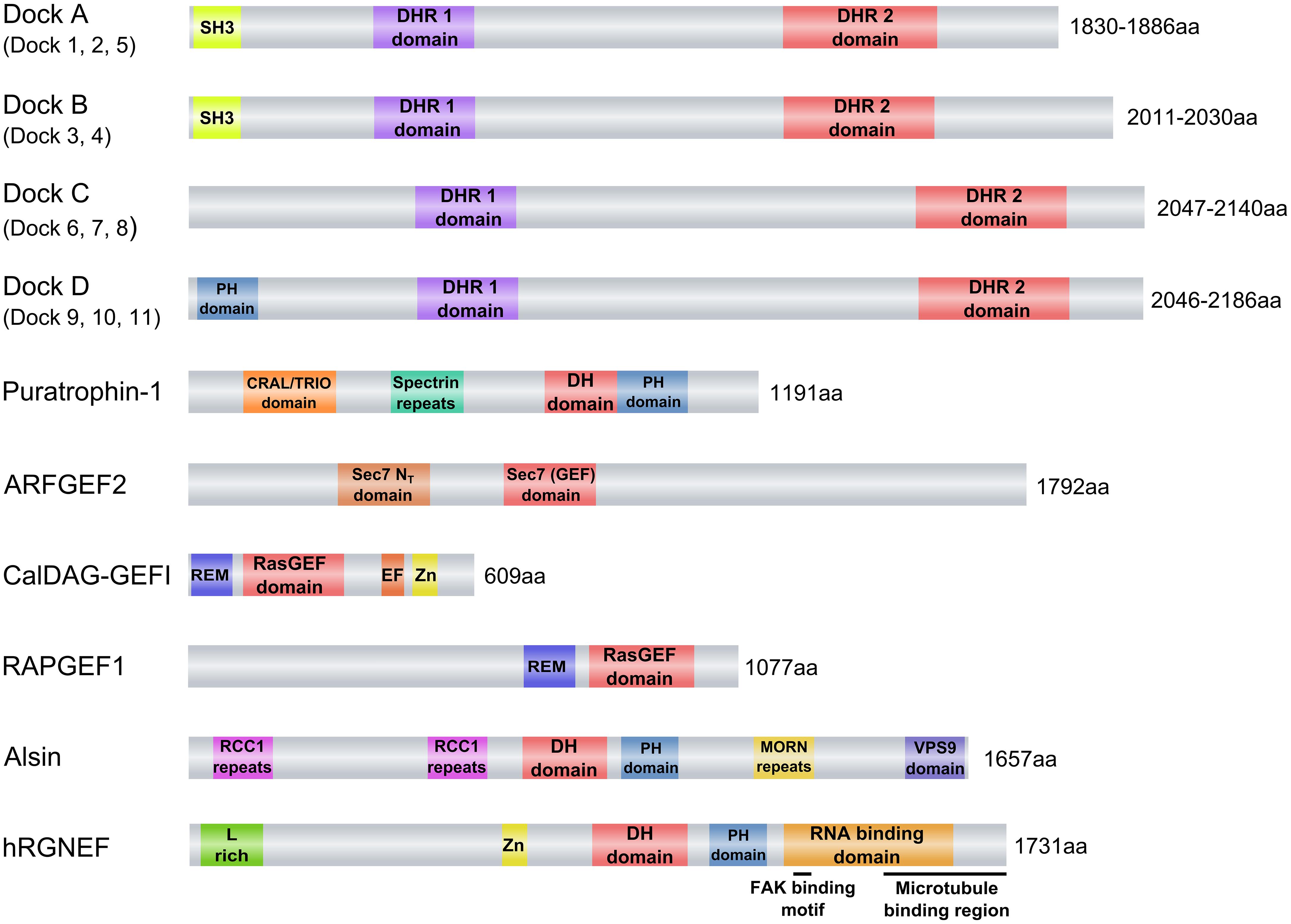
FIGURE 2. Guanine nucleotide exchange factors (GEFs) associated with neurodegenerative diseases. eIFB2 is not shown because its GEF catalytic activity is given by two subunits (epsilon and gamma) of a pentameric complex that forms the factor. Abbreviations used are: ARFGEF2, ADP-ribosylation factor GEF protein; C2 domain, C2 superfamily of C2 domain first identified in PKC; CalDAG-GEFI, calcium and diacylglycerol-regulated GEFI; CRAL/TRIO domain, SEC14 homology domain; DH domain, Dbl homology domain; DHR1, Dock Homology Region 1, a GEF domain; DHR2, Dock Homology Region 2, a GEF domain; Dock2, Dedicator of cytokinesis 2; Dock3, Dedicator of cytokinesis 3; EF, EF-hand motif (Calcium binding motif); hRGNEF, human Rho guanine nucleotide exchange factor; L-rich, Leucine-rich region; MORN repeats, Membrane Occupation and Recognition Nexus repeats; PH domain, Pleckstrin homology domain; RAPGEF1, Ras-related protein GEF1; RasGEF, Guanine nucleotide exchange factor for Ras-like small GTPases; RCC1 repeats, Regulator of chromosome condensation repeats; REM, Ras exchanger motif; Sec7, Domain named after the S. cerevisiae SEC7 gene product; Sec 7 NT, Amino-terminal Sec 7 domain; SH3, Src Homology 3 domain; Spectrin repeats, Spectrin-like repeats; Zn, cysteine-rich zinc binding domain. The schematics were based in the information obtained from the “conserved domain” search tool from NCBI (http://www.ncbi.nlm.nih.gov/Structure/cdd/wrpsb.cgi).
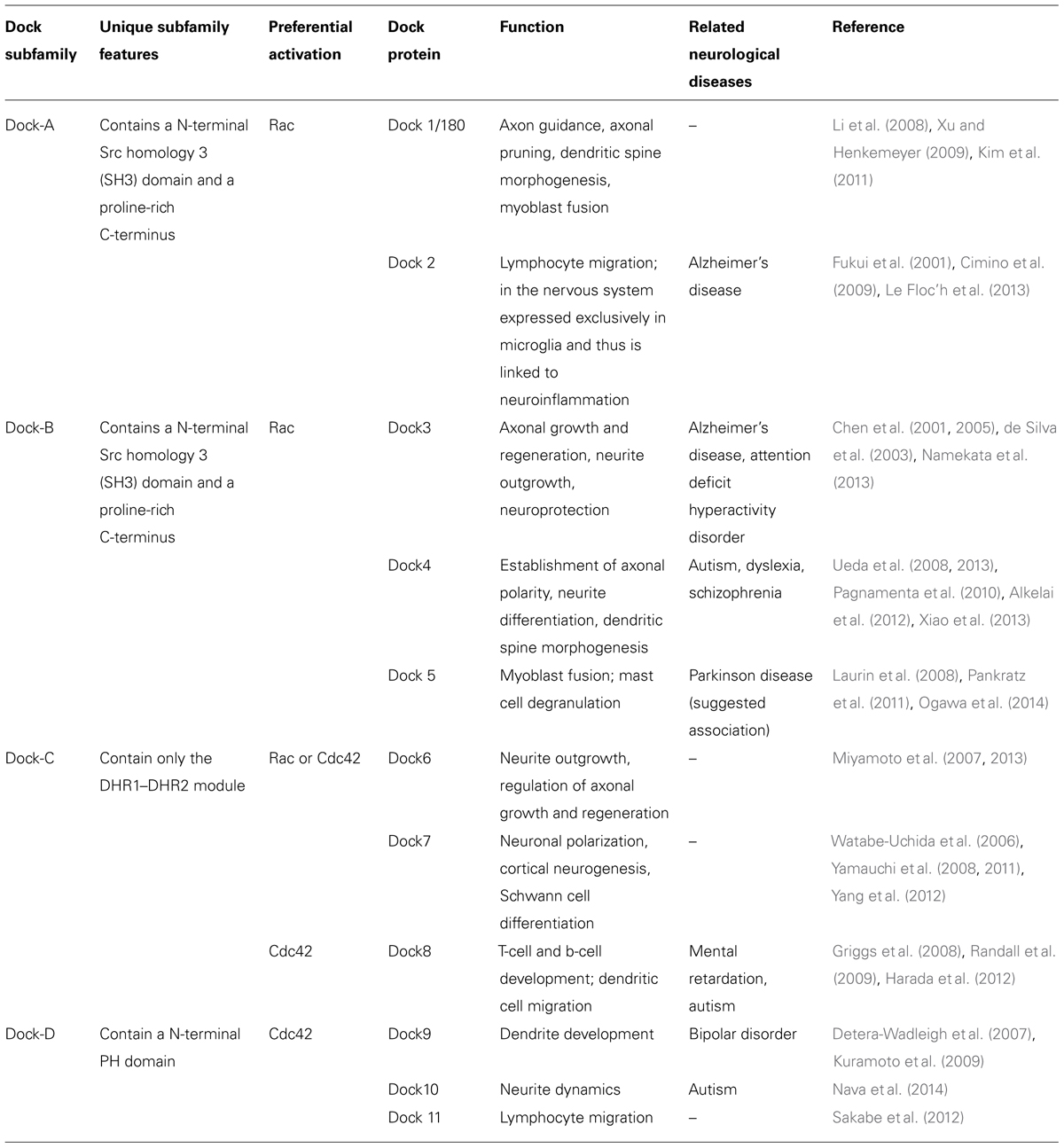
TABLE 1. Dock proteins, their role in the nervous system, and neurological disorders in which they have been implicated. Modified from Shi (2013) and Namekata et al. (2014).
Dock Protein Family
The highly conserved dedicator of cytokinesis proteins (Dock) family of proteins play a key role in multiple aspects of neuronal development, including both axonal and dendritic differentiation, in addition to involvement in both neuroinflammation and the differentiation of Schwann cells (Watabe-Uchida et al., 2006; Miyamoto et al., 2007; Li et al., 2008; Yamauchi et al., 2008, 2011; Xu and Henkemeyer, 2009; Miyamoto and Yamauchi, 2010; Kim et al., 2011; Shi, 2013; Xiao et al., 2013). Not surprisingly therefore, Dock proteins have been increasingly recognized to be associated with a variety of neurodegenerative and neuropsychiatric disorders (Table 1). In Alzheimer’s disease, there is an increase in the number of Dock2-expressing microglia, a finding of pathogenic significance given that Dock2 deficiency reduces the size of β-amyloid (Aβ) plaque in cerebral cortex and hippocampus of a mouse model of AD (Cimino et al., 2009, 2013). It is likely that this effect is mediated through Dock2 association with the prostaglandin E2 receptor (EP2), which in turn regulates neuroinflammation (Liang et al., 2005; Cimino et al., 2009). Dock4, while also expressed in the lung, is highly expressed in the central nervous system (CNS) where it is concentrated in dendritic spines within the hippocampus during development and in adulthood (Ueda et al., 2013). It is associated with autism, dyslexia, and schizophrenia (Table 1; Pagnamenta et al., 2010; Alkelai et al., 2012). Less is known with respect to Dock10 and Dock11, although a rare Dock10 gene deletion is associated with autism spectrum disorders (Nava et al., 2014). Although an association between Dock5 and Parkinson’s disease has been suggested (Pankratz et al., 2011), the authors themselves noted that the Dock5 copy number variants were more likely to be variable numbers of tandem repeats as opposed to a typical copy number variation. Thus, the reports of Dock5 association with PD remain to be validated.
It is however Dock3 that has the greatest biological and therapeutic implications for neurodegenerative disorders, in part due to the fact that it is the only Dock protein that demonstrates high tissue specificity for the CNS. Initially discovered through a yeast two-hybrid assay as a binding partner to presenilin 1 that shares 40% homology with Dock1/180 [and termed presenilin binding protein (PBP); Kashiwa et al., 2000], PBP was found to be highly enriched in the CNS and to be specifically associated with Alzheimer’s associated pathology including neurofibrillary tangles, dystrophic neurites, and neuropil threads (Chen et al., 2001). PBP was subsequently renamed MOCA (“modifier of cell adhesion”) based on its ability to modulate cell-substratum adhesion and amyloid-β secretion (Chen et al., 2002). Based on its high sequence homology with Dock1/180 (51% homology with the SH3 domain; 45% homology with the DHR1 domain, 42% homology with the DHR2 domain, and the inclusion of a Crk binding site), Dock3/MOCA was identified as a member of the Dock180 superfamily of proteins (Cote and Vuori, 2002). Dock3/MOCA was initially thought to be devoid of GEF activity; however, it was subsequently shown to induce RAC-GTP loading through the interaction of its SH3 domain with ELMO1 (engulfment and cell motility protein 1), a mechanism shared with Dock1/180 (Grimsley et al., 2004; Namekata et al., 2004, 2012). Although the exact mechanism remains uncertain, PBP stimulates phosphorylation of tau at Ser199 (Chen et al., 2001) suggesting a direct mechanism in leading to the pathological hyper-phosphorylation of tau in Alzheimer’s disease. The interaction of Dock3 with the γ-secretase presenilin 1 (De Strooper et al., 2012) suggests a role in the Aβ precursor protein (APP) processing. This is further supported by the observation that Dock3 over-expression suppresses Aβ protein secretion (Chen et al., 2002). Dock3 also acts downstream of the APP-mediated signaling pathway and in doing so, promotes neuronal cell death (Tachi et al., 2012). Distinct from this role in the pathogenesis of Alzheimer’s disease, Dock3 has recently also been suggested to attenuate NMDA-mediated neurotoxicity and in doing so, prevent excitotoxic cell death (Bai et al., 2013; Namekata et al., 2013).
There is thus significant evidence to implicate the Dock family of GEFs in both neurodegeneration (largely restricted to Alzheimer’s disease) and a range of both developmental and neuropsychiatric disorders. As suggested earlier, this is not entirely unexpected given the integral role of GEFs in neural development.
Dbl-Homology GEFs Associated with Neurodegeneration
While over 80 GEFs containing a Dbl-homology domain have been described, to date only a finite number have been associated with neurodegenerative disorders. The most lethal of these, presenting most often as a childhood disorder, is associated with mutations in any of the subunits of GEF eIF2B (eukaryotic initiation factor 2B). Conversely, alterations in the metabolism of the recently discovered Rho guanine nucleotide exchange factor (RGNEF or p190RhoGEF) are associated with the adult onset fatal disorder ALS. As is also typical of these proteins, the mechanisms by which these GEFs mediate neuronal degeneration is complicated by their pluripotential functions.
eIF2B
The eukaryotic initiation factors (eIFs) play an essential role in the initiation of translation in eukaryotes by mediating the formation of a complex between Met-tRNA (initiator methionyl-tRNA), the AUG start codon of the mRNA and the 80S ribosome. Key to this process is eIF2, a classical G-protein which recruits Met-tRNA to the 40S ribosome when in its GTP-bound (active) form. Inactive GDP-bound eIF2 is regenerated by the GEF eIF2B in a process that is the major point of regulation of protein synthesis in all eukaryote cells (Mohammad-Qureshi et al., 2007). eIF2B consists of five subunits (α–ε), of which eIF2B ε constitutes the catalytic subunit which in turn is stimulated by the γ subunit (Anthony et al., 2000; Mohammad-Qureshi et al., 2007). Given the critical role of eIF2 in the initiation of translation, it is not surprising that the activity of eIF2B would be subject to tight regulation in order to regulate protein synthesis. Indeed, environmental stress leads to phosphorylation of eIF2 which impedes the recycling of eIF2 to the active GTP-bound form by actively competing with eIF2B, which in turn reduces translation globally (Wek et al., 2006; Jennings et al., 2013). This inhibition of translation initiation can also be driven by phosphorylation of eIF2B in response to environmental stress conditions, mediated through a small number of kinases including casein kinases I and II, and glycogen synthase kinase-3 (Pavitt et al., 1998; Welsh et al., 1998; Quevedo et al., 2000; Wang et al., 2001; Singh et al., 2006).
Mutations in the eIF2B ε-subunit were first described in patients with clinical and MRI characteristics of childhood ataxia with CNS hypomyelination (CACH)/leukoencephalopathy with vanishing white matter (VWM) neurodegenerative syndrome (Leegwater et al., 2001). Subsequently, mutations in the other four eIF2B subunits were identified in association with VWM disease (Leegwater et al., 2001; van der Knaap et al., 2002). This fatal inherited neurodegenerative disease is characterized mainly by progressive ataxia, spasticity, and variable optic atrophy, as well as seizures. While VWM disease is one of the most common childhood leukoencephalopathies, it can also be seen in later age groups (Ghezzi et al., 2012). Mutations in any of the eIF2B subunits can cause loss of GEF function of human and yeast eIF2B; some of them lead to almost complete loss-of-function (Fogli et al., 2004; Li et al., 2004; Richardson et al., 2004; Leng et al., 2011). Interestingly, the vast majority of the mutations found in eIF2B genes are missense mutations in the eIF2B ε-subunit (Fogli and Boespflug-Tanguy, 2006). Cell cultures from the brain of an individual with VWM carrying mutations in the eIF2B ε-subunit have shown that few GFAP-expressing astrocytes were present, induction of astrocytes was severely compromised and the few astrocytes generated showed abnormal morphologies and antigenic phenotypes. Consequently, it has been suggested that a deficiency in astrocyte function may contribute to the loss of white matter in VWM leukodystrophy (Dietrich et al., 2005).
Puratrophin-1
Puratrophin-1 (Purkinje cell atrophy associated protein-1) is associated with autosomal dominant spinocerebellar ataxia (ADCA), a cerebellar pathology that manifests primarily as lack of motor coordination. This protein contains spectrin repeats, a GEF for Rho GTPases containing the classical Dbl homologous domain (Ishikawa et al., 2005) and a CRAL/TRIO domain (Figure 2). In fact, puratrophin-1 is a bona fide GEF that facilitates activation of the small GTPases Rac1, Cdc42, and RhoA. The overexpression of this protein induces rearrangements of the actin cytoskeleton, specifically enhancing the formation of lamellipodia and filopodia (Gupta et al., 2013). Mutations in the 5′ untranslated region of the PLEKHG4 (puratrophin-1) gene have been found in patients with ADCA. Interestingly, puratrophin-1 is aggregated in Purkinje cells of ADCA brains (Ishikawa et al., 2005).
ARF GEF Proteins
The regulation of protein and lipid transfer within eukaryotic cells is largely mediated by vesicles that are formed in response to the need to transport between cellular compartments. The ADP-ribosylation factor (ARF) GEF family of proteins are important regulators of the initiation of vesicle formation in that they regulate the ARF GTPases through a GDP/GTP exchange (Donaldson and Jackson, 2011; Wright et al., 2014). The ARF GEF subfamily of proteins called the BIG/Sec7 ARF 1 GEFs activates ARF1. ARFGEF2 is one of two proteins in this family responsible for interior membrane trafficking in the trans-Golgi network and endosomes (Bui et al., 2009; Figure 2). ARFGEF2 has been postulated as a new potential biomarker of Huntington’s disease (HD) because of its significant up-regulation in blood samples of HD patients (Lovrecic et al., 2010).
CalDAG-GEFI
Another guanine nucleotide exchange factor (GEF) that has been described as being involved in HD is a calcium and diacylglycerol-regulated GEFI (CalDAG-GEFI), also called RasGRP2 (Figure 2). CalDAG-GEFI is highly enriched in striatum and targets the small G proteins, Rap1 and Rap2 (Kawasaki et al., 1998; Ohba et al., 2000; Crittenden et al., 2004) and has been described to participate in the signaling pathway of M(1) muscarinic acetylcholine receptor (Guo et al., 2001). One of the most significant transcriptional changes of HD patients and animal models is the down-regulation of CalDAG-GEFI (Morton et al., 2005; Desplats et al., 2006, 2008; Kuhn et al., 2007). In a brain-slice explant model of HD, knock-down of CalDAG-GEFI expression rescues striatal neurons from pathology induced by transfection of polyglutamine-expanded Huntingtin protein (Htt) exon 1. This observation suggests that the striking down-regulation of CalDAG-GEFI in HD could be a protective mechanism that mitigates Htt-induced degeneration (Crittenden et al., 2010).
RAPGEF1
The neuronal ceroid lipofuscinoses (NCLs) are the most common inherited neurodegenerative disorders mainly affecting children. That associated with mutations in the CLN3 gene gives rise to CLN3 (ceroid-lipofuscinosis, neuronal 3) which manifests with a progressive retinopathy leading to blindness, dementia, epilepsy, and motor dysfunction. Microarray analyses have identified a GEF protein for small GTPase of the Ras family, Ras-Related Protein GEF1 (RAPGEF1 or C3G; Figure 2), which is dysregulated regardless of the clinical course of CLN3 disease, suggesting that this protein could be a potential biomarker (Lebrun et al., 2011). This is not surprising, considering the critical role of RAPGEF1 in multiple signal transduction pathways that regulate growth, differentiation, migration, and survival in neuronal cells (D’Arcangelo et al., 1995; Voss et al., 2006, 2008; Radha et al., 2008).
Dbl-Homology GEFs in the Pathogenesis of ALS
Amyotrophic lateral sclerosis (ALS) is a neurodegenerative disorder characterized by the progressive loss of motor neurons leading to death within three to five years of symptom onset. While currently 1:350 men and 1:450 women are affected, increased incidence rates are predicted as the population ages (Strong, 2004; Murphy et al., 2008). Most cases are clinically sporadic ALS (sALS) with approximately 5% being familial (fALS; Byrne et al., 2011). The lack of treatments that fundamentally alter the disease course is in part related to the confounding array of disturbed cellular processes in ALS. However, there is increasing consensus that perturbations in RNA metabolism are critical to the disease process (Strong, 2010; Droppelmann et al., 2014).
One of the pathological hallmarks of ALS is the presence of neuronal cytoplasmic inclusions (NCIs) including RNA binding proteins such as mutant superoxide dismutase 1 (mtSOD1), TAR DNA binding protein (TDP-43), fused in sarcoma/translocated in liposarcoma (FUS/TLS), TATA-binding protein-associated factor 15 (TAF15), Ewing sarcoma breakpoint region 1 protein (EWS), and RNA binding motif protein 45 (RBM45; Stieber et al., 2000; Ge et al., 2005; Arai et al., 2006; Neumann et al., 2006; Kwiatkowski et al., 2009; Vance et al., 2009; Couthouis et al., 2011, 2012; Collins et al., 2012) in addition to NCIs consisting of intermediate filament cytoskeletal proteins such as neurofilament (NF; Kondo et al., 1986) and peripherin (He and Hays, 2004). The NF inclusions have been shown to arise in response to an alteration in the expression ratio of the three NF subunits (low, middle, and high molecular weight NFs; NFL, NFM, and NFH respectively, encoded by NEFL, NEFM, NEFH, respectively). The traditional view of NF function is that they are integral to the formation and maintenance of the cytoskeleton, including axon diameter and integrity. The contemporary view includes a critical role for NF as a protective sink for reactive nitrating species (Strong et al., 2003; Szaro and Strong, 2010), in cell signaling and transcriptional regulation (Eriksson et al., 2009), and in mitochondrial morphology, motility, and fusion (Gentil et al., 2012).
Notably, in ALS there is a selective loss of NEFL mRNA in spinal motor neurons while the levels of NEFM and NEFH mRNA are unchanged (Bergeron et al., 1994; Wong et al., 2000; Menzies et al., 2002). The biological importance of perturbing the expression ratio of NF has been repeatedly demonstrated both in vivo through the induction of selective motor neuron degeneration (Eyer and Peterson, 1994; Tu et al., 1997; McLean et al., 2005; Strong et al., 2005; Li et al., 2006) and in vitro (Lin et al., 2003, 2004, 2005; Strong et al., 2003). This evidence implies that alterations of NEFL mRNA levels on motor neurons could be associated with motor neuron death and consequently with ALS pathogenesis.
Alsin/ALS2
ALS2 gene encodes for a protein called ALS2 or alsin. Alsin is a GEF protein predominantly expressed in central nervous system (Devon et al., 2005) that exhibits selective GEF activity on the members of small GTPase Rab5 (Rab5A, Rab5B, and Rab5C; Otomo et al., 2003; Topp et al., 2004). This protein has been involved in receptor trafficking, macropinocytic endocytosis, autophagosome-endolysosomal trafficking and axonal outgrowth (Devon et al., 2006; Hadano et al., 2006, 2010; Jacquier et al., 2006; Kunita et al., 2007; Otomo et al., 2008).
A comparison of alsin with other proteins reveals the presence of several interesting motifs. In its N-terminus domain, alsin contains a GEF domain for Ran GTPase, called RCC1. Alsin has been reported to have 5 RCC1 repeats that form a β-propeller similar to RCC1 protein in which this domain was first described (Dasso, 2001; Topp et al., 2004). The middle portion of alsin contains a tandem organization of diffuse B cell lymphoma Dbl homology (DH) and pleckstrin homology (PH) domains, the hallmark of GEFs for Rho-type GTPases (Rossman et al., 2005). The C-terminal region contains the vacuolar protein sorting 9 (VPS9) domain, which has been found in Rab5 GEFs (Zerial and McBride, 2001; Carney et al., 2006) and a tandem of eight membrane occupation and recognition nexus (MORN) motifs, which is implicated in the targeting and binding to the plasma membrane (Takeshima et al., 2000; Ma et al., 2006; Figure 2).
ALS2 is a causative gene for the juvenile autosomal recessive form of multiple motor neuron diseases, including type 2 ALS (Hadano et al., 2001; Yang et al., 2001), primary lateral sclerosis (Yang et al., 2001) and infantile-onset ascending hereditary spastic paralysis (Eymard-Pierre et al., 2002). Several mutations distributed widely across the entire coding sequence of ALS2 have been reported. They are predicted to result in either premature termination of translation or substitution of an evolutionarily conserved amino acid, leading to loss of its function (Hadano et al., 2001; Yang et al., 2001; Eymard-Pierre et al., 2002; Sztriha et al., 2008; Verschuuren-Bemelmans et al., 2008; Herzfeld et al., 2009; Mintchev et al., 2009; Shirakawa et al., 2009; Luigetti et al., 2013; Kocak Eker et al., 2014; Wakil et al., 2014). Despite all this evidence, studies using ALS2 KO mice have demonstrated that the absence of alsin does not produce a severe phenotype. However, it has been reported that ALS2 KO mice develop age-dependent deficits in motor coordination (Cai et al., 2005), an age-dependent and slow progressive loss of cerebellar Purkinje cells, a reduction in ventral motor axons during aging, astrogliosis, and evidence of deficits in endosome trafficking (Hadano et al., 2006), degeneration of corticospinal axons and axonal transport defects (Gros-Louis et al., 2008). In addition, primary cultured motor neurons lacking ALS2 have been found to be more susceptible to oxidative stress (Cai et al., 2005).
RGNEF/p190RhoGEF
RGNEF protein (Rho guanine nucleotide exchange factor) is a Rho-specific nucleotide exchange factor (GEF) that is part of the Dbl family of GEFs encoded by the ARHGEF28 gene. This protein was first cloned from a mouse brain cDNA library and was named p190RhoGEF (Gebbink et al., 1997). Mouse RGNEF (mRGNEF) is ubiquitously expressed and it is able to specifically activate RhoA both in vitro and in vivo and binds to and co-localizes with microtubules (van Horck et al., 2001). mRGNEF can interact with c-Jun amino-terminal kinase (JNK) interacting protein-1 (JIP-1; Meyer et al., 1999), the 14-3-3 adapter protein (Zhai et al., 2001) and the Focal adhesion kinase (FAK; Zhai et al., 2003). The interaction with FAK is integral to the progression of colon carcinoma tumors (Yu et al., 2011).
Structurally, RGNEF has five important domains: an L-rich region and a cysteine-rich Zn binding domain in the amino terminus half of the protein; a Dbl homology domain (DH), a Pleckstrin homology domain (PH), and an RNA binding domain in the carboxyl terminus half of the protein (Gebbink et al., 1997; Canete-Soler et al., 2001; van Horck et al., 2001; Volkening et al., 2010; Droppelmann et al., 2013a; Figure 2). It is this latter aspect that renders RGNEF as being unique amongst the remaining members of the Dbl-homology GEF family of proteins in that it is the only member that combines RNA binding activity with GEF activity.
Mouse RGNEF can bind to a destabilizing element in the 3′ untranslated region (3′UTR) of the murine NEFL mRNA, consequently stabilizing the transcript (Canete-Soler et al., 1998, 2001). This interaction can be modulated by BC1 RNA (Ge et al., 2002), an untranslated 152-nucleotide polymerase III transcript that is highly expressed in large neurons of rat brain and spinal cord (DeChiara and Brosius, 1987). Interestingly, mRGNEF is involved in the NF protein aggregation formation observed in an RNA-triggered transgenic model of motor neuron disease (Nie et al., 2002; Lin et al., 2005) in which mRGNEF forms a protein complex with aldolase A and C which participates in the regulation of NEFL mRNA stability (Canete-Soler et al., 2005; Lin et al., 2005).
Given the evidence suggesting an important role for RGNEF in the regulation of RNA metabolism in motor neurons, we postulated that human RGNEF (hRGNEF) would play a key role in the pathogenesis of ALS. Our group determined that hRGNEF, similar to mRGNEF, is an RNA binding protein that regulates the stability of NEFL mRNA (Volkening et al., 2010; Droppelmann et al., 2013a). We observed that the RNA binding domain of hRGNEF could interact with NEFL mRNA in whole cell lysates (including proteins and RNA) from spinal cord in ALS but not control cases (Volkening et al., 2010). This observation was critical in that, in contrast to the effect of mRGNEF in stabilizing murine NEFL mRNA, we found that the effect of full length hRGNEF over human NEFL mRNA stability was the opposite. hRGNEF destabilizes NEFL mRNA and decreases the levels of NFL protein in vitro (Droppelmann et al., 2013a).
We also observed that hRGNEF immunoreactive NCIs in spinal motor neurons in ALS (Figure 3) co-localized with other RNA binding proteins, suggesting a common pathway of NCI formation in which multiple RNA binding proteins co-aggregate (Keller et al., 2012; Droppelmann et al., 2013a). Moreover, we and others found a heterozygous frameshift mutation in ARHGEF28 in both fALS and sALS patients that predicts the expression of a truncated protein by the mutated allele (Droppelmann et al., 2013b; Ma et al., 2014).
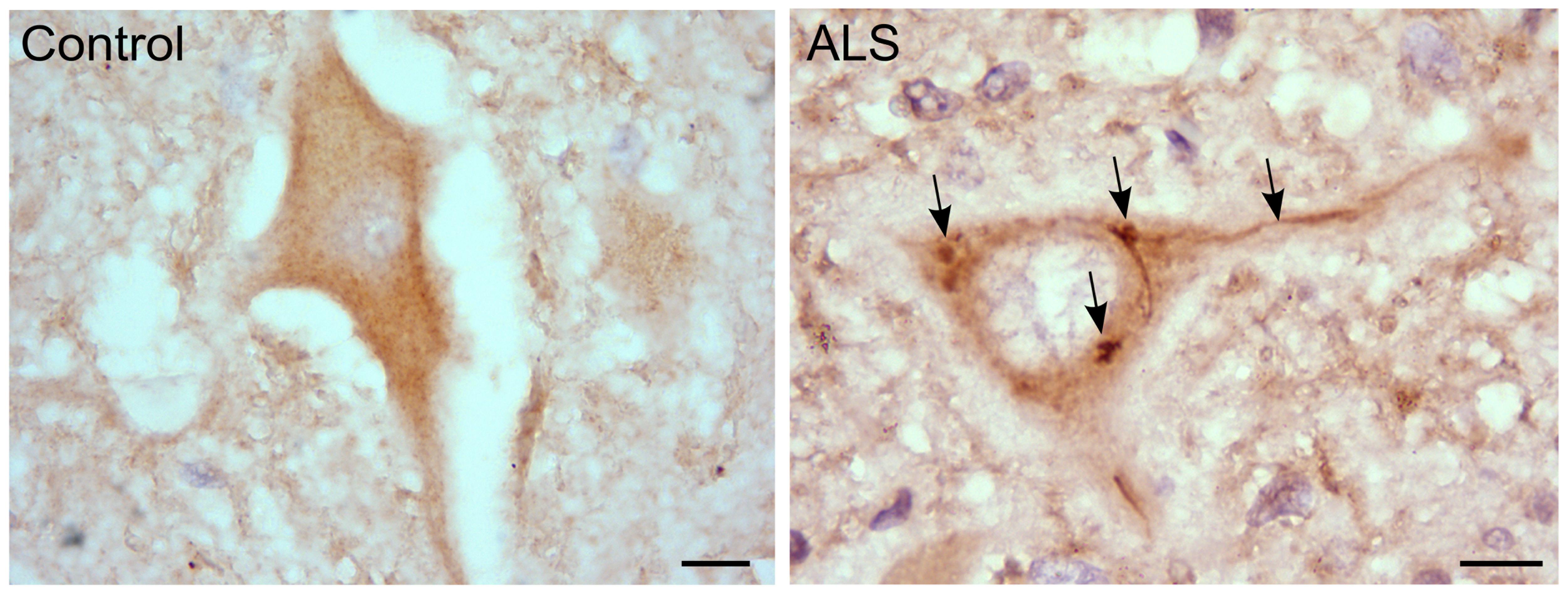
FIGURE 3. RGNEF pathology in ALS motor neurons. Representative image of RGNEF cytoplasmic inclusions observed in motor neurons of ALS patients (indicated by arrows) compared with a control case. Immunohisto chemistry was performed as described previously (Keller et al., 2012; Droppelmann et al., 2013a) using goat polyclonal anti-RGNEF (MediMabs; 1:500 dilution; Scale bar = 10 μm).
It is interesting to note that mRGNEF has been described as an anti-apoptotic factor in neuronal cells (Wu et al., 2003). It was observed that mRGNEF confers protection against stress-induced apoptosis in neuronal cells which could be associated with the interaction of mRGNEF with JIP-1 and 14-3-3 proteins. This observation suggests that the sequestration of RGNEF into aggregates in ALS may contribute to neuronal death.
C9ORF72
C9ORF72 is another gene strongly involved in neurodegeneration. An expanded GGGGCC hexanucleotide repeat in the first intron located between the 1st and 2nd non-coding exons of C9ORF72 is the most frequent genetic cause of frontotemporal dementia and ALS (DeJesus-Hernandez et al., 2011; Renton et al., 2011). C9ORF72 encodes a protein with largely unknown function. However, sensitive homology searches have shown that C9ORF72 is a full-length distant homolog of proteins related to Differentially Expressed in Normal and Neoplasia (DENN), a GEF that activates Rab-GTPases. These results suggest that C9ORF72 is likely to regulate membrane traffic in conjunction with Rab-GTPase switches (Levine et al., 2013).
Concluding Remarks
There is compelling evidence for the emerging role of GEFs in neurodegeneration. Given the key role of many of these proteins in neuronal development and differentiation as well as cellular vesicular transport through the regulation of small GTPases, this is perhaps not unexpected. In the examples provided, the associated diseases range from lethal childhood onset disorders such a VWM disease, to developmental disorders such as the autism spectrum disorders, through to the fatal adult onset disorder ALS.
In this context, the example of RGNEF and its association with ALS is notable as this is the only GEF that combines RNA binding activity with the capacity to activate RhoA GTPase (Figure 4). The observation that RGNEF is able to immunoprecipitate NEFL mRNA from ALS spinal cord homogenates and not from controls suggests a preferential interaction in ALS that would be predicted to suppress NEFL mRNA levels, consistent with the loss of NEFL mRNA detected by in situ hybridization in ALS motor neurons (Wong et al., 2000). This may be further augmented through the sequestration of multiple RNA binding proteins within pathological NCIs in ALS spinal motor neurons with the net effect being a loss of the regulation of NEFL and other mRNAs stability. In a similar vein, the sequestration of RGNEF within NCIs in ALS would also be predicted to render it unavailable to participate in the modulation of RhoA GTPase activation and potentially lead to neuronal death. Understanding this intricate relationship is currently the focus of ongoing studies.
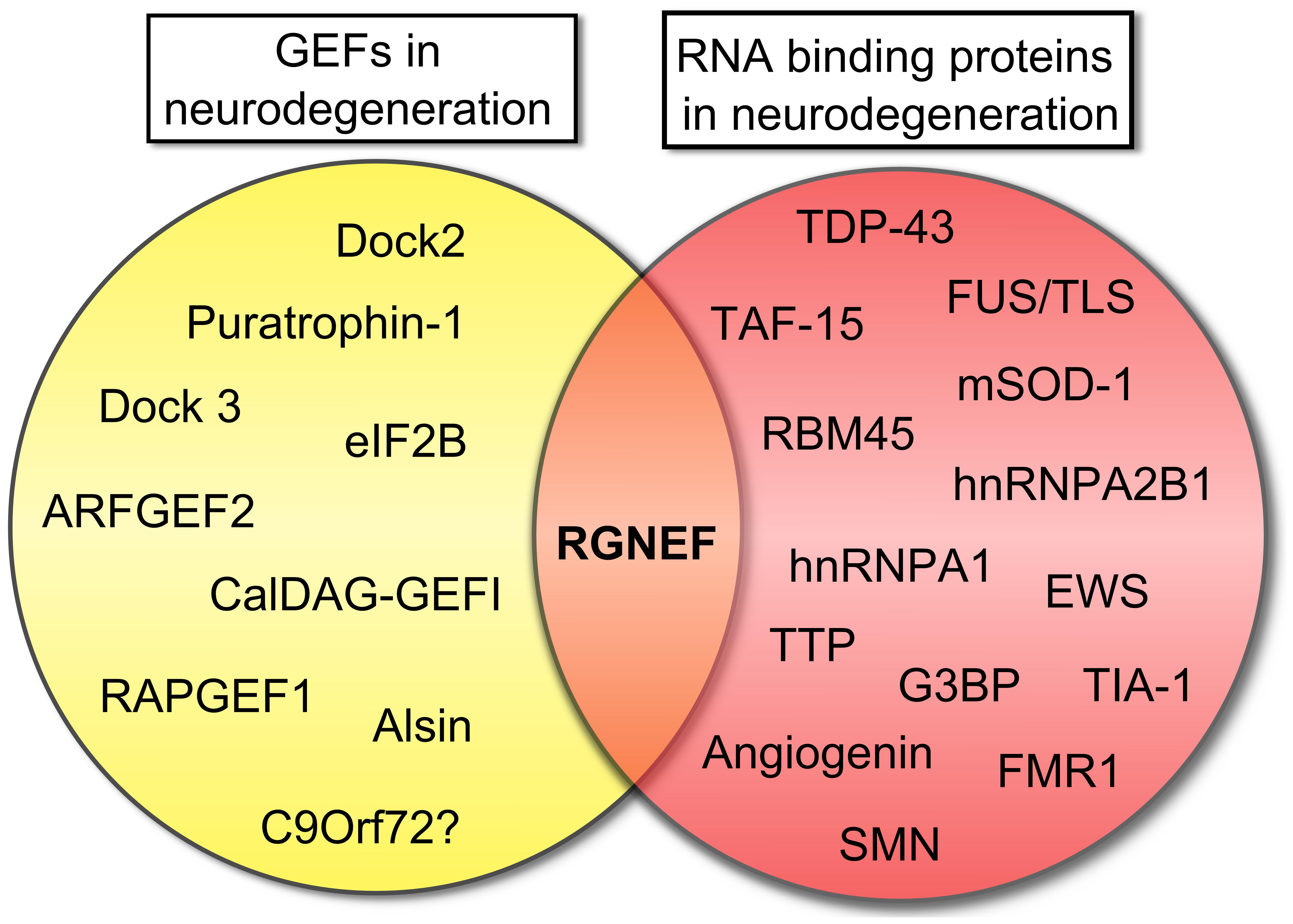
FIGURE 4. Rho guanine nucleotide exchange factor (RGNEF) is the only GEF involved in neurodegeneration that also contains a RNA binding domain. Scheme showing the participation of several GEFs in neurodegeneration as was described in this review and RNA binding proteins that participate in neurodegeneration (Ge et al., 2005; Vanderweyde et al., 2013). RGNEF is the only protein involved in neurodegeneration that can be grouped in both categories. Abbreviations are: ARFGEF2, ADP-ribosylation factor GEF protein; CalDAG-GEFI, calcium and diacylglycerol-regulated GEFI; Dock2, Dedicator of cytokinesis 2; Dock3, Dedicator of cytokinesis 3; eIF2B, eukaryotic initiation factor 2B; EWS, Ewing sarcoma breakpoint region 1 protein; FMR1, Fragile X mental retardation protein 1; FUS/TLS, Fused in sarcoma/Translocated in liposarcoma; G3BP, Ras-GTPase-activating protein SH3-domain-binding protein; hnRNPA1, heterogeneous nuclear ribonucleoprotein A1; hnRNPA2B1, heterogeneous nuclear ribonucleoprotein A2/B1; mSOD1, mutant superoxide dismutase 1; RAPGEF1, Ras-related protein GEF1; RBM45, RNA binding motif protein 45; RGNEF, Rho guanine nucleotide exchange factor; SMN, Survival motor neuron protein; TAF15, TATA-binding protein-associated Factor 15; TDP-43, TAR DNA binding protein; TIA-1, T-cell intracellular antigen 1; TTP, Tristetraprolin.
It is also interesting to postulate that alterations in GEF activity, while not directly inducing a disease state, could function as a critical “second insult” through the loss of one or more effector pathways. For instance, while the loss of NEFL mRNA stability is critical to the formation of pathological NCIs in spinal motor neurons, the concomitant loss of GEF activity due to RGNEF sequestration may be sufficient to render the cell incapable of responding to the subsequent cellular stressors. Such a conceptualization is consistent with the increasing evidence for oligogenic inheritance in ALS where a second gene might participate as a critical modulator of the pathological phenotype of the disease (van Blitterswijk et al., 2012; Droppelmann et al., 2013b). In this context, perturbations in the function of GEF proteins might well be considered as key candidates for this “second hit” because of their direct or indirect roles on cell survival (Chen et al., 2002, 2009; Wu et al., 2003; Cai et al., 2005; Dietrich et al., 2005; Crittenden et al., 2010; Bai et al., 2013; Namekata et al., 2013).
Conflict of Interest Statement
The authors declare that the research was conducted in the absence of any commercial or financial relationships that could be construed as a potential conflict of interest.
Acknowledgments
We are grateful to Mr. Michael Tavolieri for his critical reading of the manuscript. Michael J. Strong research is supported by the Canadian Institutes for Health Research (CIHR), the ALS Society of Canada and the Michael Halls Endowment.
References
Ahn, J. Y., and Ye, K. (2005). PIKE GTPase signaling and function. Int. J. Biol. Sci. 1, 44–50. doi: 10.7150/ijbs.1.44
Alkelai, A., Lupoli, S., Greenbaum, L., Kohn, Y., Kanyas-Sarner, K., Ben-Asher, E.,et al. (2012). DOCK4 and CEACAM21 as novel schizophrenia candidate genes in the Jewish population. Int. J. Neuropsychopharmacol. 15, 459–469. doi: 10.1017/S1461145711000903
Anthony, T. G., Fabian, J. R., Kimball, S. R., and Jefferson, L. S. (2000). Identification of domains within the epsilon-subunit of the translation initiation factor eIF2B that are necessary for guanine nucleotide exchange activity and eIF2B holoprotein formation. Biochim. Biophys. Acta 1492, 56–62. doi: 10.1016/S0167-4781(00)00062-2
Arai, T., Hasegawa, M., Akiyama, H., Ikeda, K., Nonaka, T., Mori, H.,et al. (2006). TDP-43 is a component of ubiquitin-positive tau-negative inclusions in frontotemporal lobar degeneration and amyotrophic lateral sclerosis. Biochem. Biophys. Res. Commun. 351, 602–611. doi: 10.1016/j.bbrc.2006.10.093
Bai, N., Hayashi, H., Aida, T., Namekata, K., Harada, T., Mishina, M.,et al. (2013). Dock3 interaction with a glutamate-receptor NR2D subunit protects neurons from excitotoxicity. Mol. Brain 6, 22. doi: 10.1186/1756-6606-6-22
Bergeron, C., Beric-Maskarel, K., Muntasser, S., Weyer, L., Somerville, M. J., and Percy, M. E. (1994). Neurofilament light and polyadenylated mRNA levels are decreased in amyotrophic lateral sclerosis motor neurons. J. Neuropathol. Exp. Neurol. 53, 221–230. doi: 10.1097/00005072-199405000-00002
Bielnicki, J. A., Shkumatov, A. V., Derewenda, U., Somlyo, A. V., Svergun, D. I., and Derewenda, Z. S. (2011). Insights into the molecular activation mechanism of the RhoA-specific guanine nucleotide exchange factor, PDZRhoGEF. J. Biol. Chem. 286, 35163–35175. doi: 10.1074/jbc.M111.270918
Bloom, G. S. (2014). Amyloid-beta and tau: the trigger and bullet in Alzheimer disease pathogenesis. J. Am. Med. Assoc. Neurol. 71, 505–508. doi: 10.1001/jamaneurol.2013.5847
Bui, Q. T., Golinelli-Cohen, M. P., and Jackson, C. L. (2009). Large Arf1 guanine nucleotide exchange factors: evolution, domain structure, and roles in membrane trafficking and human disease. Mol. Genet. Genomics 282, 329–350. doi: 10.1007/s00438-009-0473-3
Burridge, K., and Wennerberg, K. (2004). Rho and Rac take center stage. Cell 116, 167–179. doi: 10.1016/S0092-8674(04)00003-0
Byrne, S., Walsh, C., Lynch, C., Bede, P., Elamin, M., Kenna, K.,et al. (2011). Rate of familial amyotrophic lateral sclerosis: a systematic review and meta-analysis. J. Neurol. Neurosurg. Psychiatry 82, 623–627. doi: 10.1136/jnnp.2010.224501
Cai, H., Lin, X., Xie, C., Laird, F. M., Lai, C., Wen, H.,et al. (2005). Loss of ALS2 function is insufficient to trigger motor neuron degeneration in knock-out mice but predisposes neurons to oxidative stress. J. Neurosci. 25, 7567–7574. doi: 10.1523/JNEUROSCI.1645-05.2005
Canete-Soler, R., Reddy, K. S., Tolan, D. R., and Zhai, J. (2005). Aldolases A and C are ribonucleolytic components of a neuronal complex that regulates the stability of the light-neurofilament mRNA. J. Neurosci. 25, 4353–4364. doi: 10.1523/JNEUROSCI.0885-05.2005
Canete-Soler, R., Schwartz, M. L., Hua, Y., and Schlaepfer, W. W. (1998). Stability determinants are localized to the 3′-untranslated region and 3′-coding region of the neurofilament light subunit mRNA using a tetracycline-inducible promoter. J. Biol. Chem. 273, 12650–12654. doi: 10.1074/jbc.273.20.12650
Canete-Soler, R., Wu, J., Zhai, J., Shamim, M., and Schlaepfer, W. W. (2001). p190RhoGEF binds to a destabilizing element in the 3′ untranslated region of light neurofilament subunit mRNA and alters the stability of the transcript. J. Biol. Chem. 276, 32046–32050. doi: 10.1074/jbc.M104104200
Carney, D. S., Davies, B. A., and Horazdovsky, B. F. (2006). Vps9 domain-containing proteins: activators of Rab5 GTPases from yeast to neurons. Trends Cell Biol. 16, 27–35. doi: 10.1016/j.tcb.2005.11.001
Chen, Q., Chen, T. J., Letourneau, P. C., Costa Lda, F., and Schubert, D. (2005). Modifier of cell adhesion regulates N-cadherin-mediated cell-cell adhesion and neurite outgrowth. J. Neurosci. 25, 281–290. doi: 10.1523/JNEUROSCI.3692-04.2005
Chen, Q., Kimura, H., and Schubert, D. (2002). A novel mechanism for the regulation of amyloid precursor protein metabolism. J. Cell Biol. 158, 79–89. doi: 10.1083/jcb.200110151
Chen, Q., Peto, C. A., Shelton, G. D., Mizisin, A., Sawchenko, P. E., and Schubert, D. (2009). Loss of modifier of cell adhesion reveals a pathway leading to axonal degeneration. J. Neurosci. 29, 118–130. doi: 10.1523/JNEUROSCI.3985-08.2009
Chen, Q., Yoshida, H., Schubert, D., Maher, P., Mallory, M., and Masliah, E. (2001). Presenilin binding protein is associated with neurofibrillary alterations in Alzheimer’s disease and stimulates tau phosphorylation. Am. J. Pathol. 159, 1597–1602. doi: 10.1016/S0002-9440(10)63005-2
Cimino, P. J., Sokal, I., Leverenz, J., Fukui, Y., and Montine, T. J. (2009). DOCK2 is a microglial specific regulator of central nervous system innate immunity found in normal and Alzheimer’s disease brain. Am. J. Pathol. 175, 1622–1630. doi: 10.2353/ajpath.2009.090443
Cimino, P. J., Yang, Y., Li, X., Hemingway, J. F., Cherne, M. K., Khademi, S. B.,et al. (2013). Ablation of the microglial protein DOCK2 reduces amyloid burden in a mouse model of Alzheimer’s disease. Exp. Mol. Pathol. 94, 366–371. doi: 10.1016/j.yexmp.2013.01.002
Collins, M., Riascos, D., Kovalik, T., An, J., Krupa, K., Hood, B. L.,et al. (2012). The RNA-binding motif 45 (RBM45) protein accumulates in inclusion bodies in amyotrophic lateral sclerosis (ALS) and frontotemporal lobar degeneration with TDP-43 inclusions (FTLD-TDP) patients. Acta Neuropathol. 124, 717–732. doi: 10.1007/s00401-012-1045-x
Cote, J. F., and Vuori, K. (2002). Identification of an evolutionarily conserved superfamily of DOCK180-related proteins with guanine nucleotide exchange activity. J. Cell Sci. 115, 4901–4913. doi: 10.1242/jcs.00219
Couthouis, J., Hart, M. P., Erion, R., King, O. D., Diaz, Z., Nakaya, T.,et al. (2012). Evaluating the role of the FUS/TLS-related gene EWSR1 in amyotrophic lateral sclerosis. Hum. Mol. Genet. 21, 2899–2911. doi: 10.1093/hmg/dds116
Couthouis, J., Hart, M. P., Shorter, J., Dejesus-Hernandez, M., Erion, R., Oristano, R.,et al. (2011). A yeast functional screen predicts new candidate ALS disease genes. Proc. Natl. Acad. Sci. U.S.A. 108, 20881–20890. doi: 10.1073/pnas.1109434108
Crittenden, J. R., Bergmeier, W., Zhang, Y., Piffath, C. L., Liang, Y., Wagner, D. D.,et al. (2004). CalDAG-GEFI integrates signaling for platelet aggregation and thrombus formation. Nat. Med. 10, 982–986. doi: 10.1038/nm1098
Crittenden, J. R., Dunn, D. E., Merali, F. I., Woodman, B., Yim, M., Borkowska, A. E.,et al. (2010). CalDAG-GEFI down-regulation in the striatum as a neuroprotective change in Huntington’s disease. Hum. Mol. Genet. 19, 1756–1765. doi: 10.1093/hmg/ddq055
D’Adamo, P., Masetti, M., Bianchi, V., More, L., Mignogna, M. L., Giannandrea, M.,et al. (2014). RAB GTPases and RAB-interacting proteins and their role in the control of cognitive functions. Neurosci. Biobehav. Rev. doi: 10.1016/j.neubiorev.2013.12.009 [Epub ahead of print].
D’Arcangelo, G., Miao, G. G., Chen, S. C., Soares, H. D., Morgan, J. I., and Curran, T. (1995). A protein related to extracellular matrix proteins deleted in the mouse mutant reeler. Nature 374, 719–723. doi: 10.1038/374719a0
Dasso, M. (2001). Running on Ran: nuclear transport and the mitotic spindle. Cell 104, 321–324. doi: 10.1016/S0092-8674(01)00218-5
DeChiara, T. M., and Brosius, J. (1987). Neural BC1 RNA: cDNA clones reveal nonrepetitive sequence content. Proc. Natl. Acad. Sci. U.S.A. 84, 2624–2628. doi: 10.1073/pnas.84.9.2624
DeJesus-Hernandez, M., Mackenzie, I. R., Boeve, B. F., Boxer, A. L., Baker, M., Rutherford, N. J.,et al. (2011). Expanded GGGGCC hexanucleotide repeat in noncoding region of C9ORF72 causes chromosome 9p-linked FTD and ALS. Neuron 72, 245–256. doi: 10.1016/j.neuron.2011.09.011
de Silva, M. G., Elliott, K., Dahl, H. H., Fitzpatrick, E., Wilcox, S., Delatycki, M.,et al. (2003). Disruption of a novel member of a sodium/hydrogen exchanger family and DOCK3 is associated with an attention deficit hyperactivity disorder-like phenotype. J. Med. Genet. 40, 733–740. doi: 10.1136/jmg.40.10.733
De Strooper, B., Iwatsubo, T., and Wolfe, M. S. (2012). Presenilins and gamma-secretase: structure, function, and role in Alzheimer Disease. Cold Spring Harb. Perspect. Med. 2:a006304. doi: 10.1101/cshperspect.a006304
Desplats, P. A., Kass, K. E., Gilmartin, T., Stanwood, G. D., Woodward, E. L., Head, S. R.,et al. (2006). Selective deficits in the expression of striatal-enriched mRNAs in Huntington’s disease. J. Neurochem. 96, 743–757. doi: 10.1111/j.1471-4159.2005.03588.x
Desplats, P. A., Lambert, J. R., and Thomas, E. A. (2008). Functional roles for the striatal-enriched transcription factor, Bcl11b, in the control of striatal gene expression and transcriptional dysregulation in Huntington’s disease. Neurobiol. Dis. 31, 298–308. doi: 10.1016/j.nbd.2008.05.005
Detera-Wadleigh, S. D., Liu, C. Y., Maheshwari, M., Cardona, I., Corona, W., Akula, N.,et al. (2007). Sequence variation in DOCK9 and heterogeneity in bipolar disorder. Psychiatr. Genet. 17, 274–286. doi: 10.1097/YPG.0b013e328133f352
Devon, R. S., Orban, P. C., Gerrow, K., Barbieri, M. A., Schwab, C., Cao, L. P.,et al. (2006). Als2-deficient mice exhibit disturbances in endosome trafficking associated with motor behavioral abnormalities. Proc. Natl. Acad. Sci. U.S.A. 103, 9595–9600. doi: 10.1073/pnas.0510197103
Devon, R. S., Schwab, C., Topp, J. D., Orban, P. C., Yang, Y. Z., Pape, T. D.,et al. (2005). Cross-species characterization of the ALS2 gene and analysis of its pattern of expression in development and adulthood. Neurobiol. Dis. 18, 243–257. doi: 10.1016/j.nbd.2004.10.002
Dietrich, J., Lacagnina, M., Gass, D., Richfield, E., Mayer-Proschel, M., Noble, M.,et al. (2005). EIF2B5 mutations compromise GFAP+ astrocyte generation in vanishing white matter leukodystrophy. Nat. Med. 11, 277–283. doi: 10.1038/nm1195
DiFiglia, M., Sapp, E., Chase, K. O., Davies, S. W., Bates, G. P., Vonsattel, J. P.,et al. (1997). Aggregation of huntingtin in neuronal intranuclear inclusions and dystrophic neurites in brain. Science 277, 1990–1993. doi: 10.1126/science.277.5334.1990
Donaldson, J. G., and Jackson, C. L. (2011). ARF family G proteins and their regulators: roles in membrane transport, development and disease. Nat. Rev. Mol. Cell Biol. 12, 362–375. doi: 10.1038/nrm3117
Droppelmann, C. A., Campos-Melo, D., Ishtiaq, M., Volkening, K., and Strong, M. J. (2014). RNA metabolism in ALS: when normal processes become pathological. Amyotroph. Lateral Scler. Frontotemporal Degener. 15, 321–336. doi: 10.3109/21678421.2014.881377
Droppelmann, C. A., Keller, B. A., Campos-Melo, D., Volkening, K., and Strong, M. J. (2013a). Rho guanine nucleotide exchange factor is an NFL mRNA destabilizing factor that forms cytoplasmic inclusions in amyotrophic lateral sclerosis. Neurobiol. Aging 34, 248–262. doi: 10.1016/j.neurobiolaging.2012.06.021
Droppelmann, C. A., Wang, J., Campos-Melo, D., Keller, B., Volkening, K., Hegele, R. A.,et al. (2013b). Detection of a novel frameshift mutation and regions with homozygosis within ARHGEF28 gene in familial amyotrophic lateral sclerosis. Amyotroph. Lateral Scler. Frontotemporal Degener. 14, 444–451. doi: 10.3109/21678421.2012.758288
Eriksson, J. E., Dechat, T., Grin, B., Helfand, B., Mendez, M., Pallari, H. M.,et al. (2009). Introducing intermediate filaments: from discovery to disease. J. Clin. Invest. 119, 1763–1771. doi: 10.1172/JCI38339
Eyer, J., and Peterson, A. (1994). Neurofilament-deficient axons and perikaryal aggregates in viable transgenic mice expressing a neurofilament-beta-galactosidase fusion protein. Neuron 12, 389–405. doi: 10.1016/0896-6273(94)90280-1
Eymard-Pierre, E., Lesca, G., Dollet, S., Santorelli, F. M., Di Capua, M., Bertini, E.,et al. (2002). Infantile-onset ascending hereditary spastic paralysis is associated with mutations in the alsin gene. Am. J. Hum. Genet. 71, 518–527. doi: 10.1086/342359
Fogli, A., and Boespflug-Tanguy, O. (2006). The large spectrum of eIF2B-related diseases. Biochem. Soc. Trans. 34, 22–29. doi: 10.1042/BST20060022
Fogli, A., Schiffmann, R., Hugendubler, L., Combes, P., Bertini, E., Rodriguez, D.,et al. (2004). Decreased guanine nucleotide exchange factor activity in eIF2B-mutated patients. Eur. J. Hum. Genet. 12, 561–566. doi: 10.1038/sj.ejhg.5201189
Fukui, Y., Hashimoto, O., Sanui, T., Oono, T., Koga, H., Abe, M.,et al. (2001). Haematopoietic cell-specific CDM family protein DOCK2 is essential for lymphocyte migration. Nature 412, 826–831. doi: 10.1038/35090591
Garcia-Mata, R., and Burridge, K. (2007). Catching a GEF by its tail. Trends Cell Biol. 17, 36–43. doi: 10.1016/j.tcb.2006.11.004
Ge, W., Wu, J., Zhai, J., Nie, Z., Lin, H., Schlaepfer, W. W.,et al. (2002). Binding of p190RhoGEF to a destabilizing element on the light neurofilament mRNA is competed by BC1 RNA. J. Biol. Chem. 277, 42701–42705. doi: 10.1074/jbc.M206635200
Ge, W. W., Wen, W., Strong, W., Leystra-Lantz, C., and Strong, M. J. (2005). Mutant copper-zinc superoxide dismutase binds to and destabilizes human low molecular weight neurofilament mRNA. J. Biol. Chem. 280, 118–124. doi: 10.1074/jbc.M405065200
Gebbink, M. F., Kranenburg, O., Poland, M., Van Horck, F. P., Houssa, B., and Moolenaar, W. H. (1997). Identification of a novel, putative Rho-specific GDP/GTP exchange factor and a RhoA-binding protein: control of neuronal morphology. J. Cell Biol. 137, 1603–1613. doi: 10.1083/jcb.137.7.1603
Gentil, B. J., Minotti, S., Beange, M., Baloh, R. H., Julien, J. P., and Durham, H. D. (2012). Normal role of the low-molecular-weight neurofilament protein in mitochondrial dynamics and disruption in Charcot-Marie-Tooth disease. FASEB J. 26, 1194–1203. doi: 10.1096/fj.11–196345
Ghezzi, L., Scarpini, E., Rango, M., Arighi, A., Bassi, M. T., Tenderini, E.,et al. (2012). A 66-year-old patient with vanishing white matter disease due to the p.Ala87Val EIF2B3 mutation. Neurology 79, 2077–2078. doi: 10.1212/WNL.0b013e3182749edc
Goedert, M. (2001). Alpha-synuclein and neurodegenerative diseases. Nat. Rev. Neurosci. 2, 492–501. doi: 10.1038/35081564
Govek, E. E., Newey, S. E., and Van Aelst, L. (2005). The role of the Rho GTPases in neuronal development. Genes Dev. 19, 1–49. doi: 10.1101/gad.1256405
Griggs, B. L., Ladd, S., Saul, R. A., Dupont, B. R., and Srivastava, A. K. (2008). Dedicator of cytokinesis 8 is disrupted in two patients with mental retardation and developmental disabilities. Genomics 91, 195–202. doi: 10.1016/j.ygeno.2007.10.011
Grimsley, C. M., Kinchen, J. M., Tosello-Trampont, A. C., Brugnera, E., Haney, L. B., Lu, M.,et al. (2004). Dock180 and ELMO1 proteins cooperate to promote evolutionarily conserved Rac-dependent cell migration. J. Biol. Chem. 279, 6087–6097. doi: 10.1074/jbc.M307087200
Gros-Louis, F., Kriz, J., Kabashi, E., Mcdearmid, J., Millecamps, S., Urushitani, M.,et al. (2008). Als2 mRNA splicing variants detected in KO mice rescue severe motor dysfunction phenotype in Als2 knock-down zebrafish. Hum. Mol. Genet. 17, 2691–2702. doi: 10.1093/hmg/ddn171
Guo, F. F., Kumahara, E., and Saffen, D. (2001). A CalDAG-GEFI/Rap1/B-Raf cassette couples M(1) muscarinic acetylcholine receptors to the activation of ERK1/2. J. Biol. Chem. 276, 25568–25581. doi: 10.1074/jbc.M101277200
Gupta, M., Kamynina, E., Morley, S., Chung, S., Muakkassa, N., Wang, H.,et al. (2013). Plekhg4 is a novel Dbl family guanine nucleotide exchange factor protein for rho family GTPases. J. Biol. Chem. 288, 14522–14530. doi: 10.1074/jbc.M112.430371
Hadano, S., Benn, S. C., Kakuta, S., Otomo, A., Sudo, K., Kunita, R.,et al. (2006). Mice deficient in the Rab5 guanine nucleotide exchange factor ALS2/alsin exhibit age-dependent neurological deficits and altered endosome trafficking. Hum. Mol. Genet. 15, 233–250. doi: 10.1093/hmg/ddi440
Hadano, S., Hand, C. K., Osuga, H., Yanagisawa, Y., Otomo, A., Devon, R. S.,et al. (2001). A gene encoding a putative GTPase regulator is mutated in familial amyotrophic lateral sclerosis 2. Nat. Genet. 29, 166–173. doi: 10.1038/ng1001-166
Hadano, S., Otomo, A., Kunita, R., Suzuki-Utsunomiya, K., Akatsuka, A., Koike, M.,et al. (2010). Loss of ALS2/Alsin exacerbates motor dysfunction in a SOD1-expressing mouse ALS model by disturbing endolysosomal trafficking. PLoS ONE 5:e9805. doi: 10.1371/journal.pone.0009805
Harada, Y., Tanaka, Y., Terasawa, M., Pieczyk, M., Habiro, K., Katakai, T.,et al. (2012). DOCK8 is a Cdc42 activator critical for interstitial dendritic cell migration during immune responses. Blood 119, 4451–4461. doi: 10.1182/blood-2012-01-407098
He, C. Z., and Hays, A. P. (2004). Expression of peripherin in ubiquinated inclusions of amyotrophic lateral sclerosis. J. Neurol. Sci. 217, 47–54. doi: 10.1016/j.jns.2003.08.016
Herzfeld, T., Wolf, N., Winter, P., Hackstein, H., Vater, D., and Muller, U. (2009). Maternal uniparental heterodisomy with partial isodisomy of a chromosome 2 carrying a splice acceptor site mutation (IVS9-2A>T) in ALS2 causes infantile-onset ascending spastic paralysis (IAHSP). Neurogenetics 10, 59–64. doi: 10.1007/s10048-008-0148-y
Ishikawa, K., Toru, S., Tsunemi, T., Li, M., Kobayashi, K., Yokota, T.,et al. (2005). An autosomal dominant cerebellar ataxia linked to chromosome 16q22.1 is associated with a single-nucleotide substitution in the 5′ untranslated region of the gene encoding a protein with spectrin repeat and Rho guanine-nucleotide exchange-factor domains. Am. J. Hum. Genet. 77, 280–296. doi: 10.1086/432518
Jacquier, A., Buhler, E., Schafer, M. K., Bohl, D., Blanchard, S., Beclin, C.,et al. (2006). Alsin/Rac1 signaling controls survival and growth of spinal motoneurons. Ann. Neurol. 60, 105–117. doi: 10.1002/ana.20886
Jennings, M. D., Zhou, Y., Mohammad-Qureshi, S. S., Bennett, D., and Pavitt, G. D. (2013). eIF2B promotes eIF5 dissociation from eIF2*GDP to facilitate guanine nucleotide exchange for translation initiation. Genes Dev. 27, 2696–2707. doi: 10.1101/gad.231514.113
Kashiwa, A., Yoshida, H., Lee, S., Paladino, T., Liu, Y., Chen, Q.,et al. (2000). Isolation and characterization of novel presenilin binding protein. J. Neurochem. 75, 109–116. doi: 10.1046/j.1471-4159.2000.0750109.x
Kawasaki, H., Springett, G. M., Toki, S., Canales, J. J., Harlan, P., Blumenstiel, J. P.,et al. (1998). A Rap guanine nucleotide exchange factor enriched highly in the basal ganglia. Proc. Natl. Acad. Sci. U.S.A. 95, 13278–13283. doi: 10.1073/pnas.95.22.13278
Keller, B. A., Volkening, K., Droppelmann, C. A., Ang, L. C., Rademakers, R., and Strong, M. J. (2012). Co-aggregation of RNA binding proteins in ALS spinal motor neurons: evidence of a common pathogenic mechanism. Acta Neuropathol. 124, 733–747. doi: 10.1007/s00401-012-1035-z
Kim, J. Y., Oh, M. H., Bernard, L. P., Macara, I. G., and Zhang, H. (2011). The RhoG/ELMO1/Dock180 signaling module is required for spine morphogenesis in hippocampal neurons. J. Biol. Chem. 286, 37615–37624. doi: 10.1074/jbc.M111.268029
Klopper, T. H., Kienle, N., Fasshauer, D., and Munro, S. (2012). Untangling the evolution of Rab G proteins: implications of a comprehensive genomic analysis. BMC Biol. 10:71. doi: 10.1186/1741-7007-10-71
Kocak Eker, H., Unlu, S. E., Al-Salmi, F., and Crosby, A. H. (2014). A novel homozygous mutation in ALS2 gene in four siblings with infantile-onset ascending hereditary spastic paralysis. Eur. J. Med. Genet. 57, 275–278. doi: 10.1016/j.ejmg.2014.03.006
Kondo, A., Iwaki, T., Tateishi, J., Kirimoto, K., Morimoto, T., and Oomura, I. (1986). Accumulation of neurofilaments in a sporadic case of amyotrophic lateral sclerosis. Jpn. J. Psychiatry Neurol. 40, 677–684.
Kubo, T., Yamashita, T., Yamaguchi, A., Sumimoto, H., Hosokawa, K., and Tohyama, M. (2002). A novel FERM domain including guanine nucleotide exchange factor is involved in Rac signaling and regulates neurite remodeling. J. Neurosci. 22, 8504–8513.
Kuhn, A., Goldstein, D. R., Hodges, A., Strand, A. D., Sengstag, T., Kooperberg, C.,et al. (2007). Mutant huntingtin’s effects on striatal gene expression in mice recapitulate changes observed in human Huntington’s disease brain and do not differ with mutant huntingtin length or wild-type huntingtin dosage. Hum. Mol. Genet. 16, 1845–1861. doi: 10.1093/hmg/ddm133
Kunita, R., Otomo, A., Mizumura, H., Suzuki-Utsunomiya, K., Hadano, S., and Ikeda, J. E. (2007). The Rab5 activator ALS2/alsin acts as a novel Rac1 effector through Rac1-activated endocytosis. J. Biol. Chem. 282, 16599–16611. doi: 10.1074/jbc.M610682200
Kuramoto, K., Negishi, M., and Katoh, H. (2009). Regulation of dendrite growth by the Cdc42 activator Zizimin1/Dock9 in hippocampal neurons. J. Neurosci. Res. 87, 1794–1805. doi: 10.1002/jnr.21997
Kwiatkowski, T. J. Jr., Bosco, D. A., Leclerc, A. L., Tamrazian, E., Vanderburg, C. R., Russ, C.,et al. (2009). Mutations in the FUS/TLS gene on chromosome 16 cause familial amyotrophic lateral sclerosis. Science 323, 1205–1208. doi: 10.1126/science.1166066
Laurin, M., Fradet, N., Blangy, A., Hall, A., Vuori, K., and Cote, J. F. (2008). The atypical Rac activator Dock180 (Dock1) regulates myoblast fusion in vivo. Proc. Natl. Acad. Sci. U.S.A. 105, 15446–15451. doi: 10.1073/pnas.0805546105
Lazer, G., and Katzav, S. (2011). Guanine nucleotide exchange factors for RhoGTPases: good therapeutic targets for cancer therapy? Cell. Signal. 23, 969–979. doi: 10.1016/j.cellsig.2010.10.022
Le Floc’h, A., Tanaka, Y., Bantilan, N. S., Voisinne, G., Altan-Bonnet, G., Fukui, Y.,et al. (2013). Annular PIP3 accumulation controls actin architecture and modulates cytotoxicity at the immunological synapse. J. Exp. Med. 210, 2721–2737. doi: 10.1084/jem.20131324
Lebrun, A. H., Moll-Khosrawi, P., Pohl, S., Makrypidi, G., Storch, S., Kilian, D.,et al. (2011). Analysis of potential biomarkers and modifier genes affecting the clinical course of CLN3 disease. Mol. Med. 17, 1253–1261. doi: 10.2119/molmed.2010.00241
Leegwater, P. A., Vermeulen, G., Konst, A. A., Naidu, S., Mulders, J., Visser, A.,et al. (2001). Subunits of the translation initiation factor eIF2B are mutant in leukoencephalopathy with vanishing white matter. Nat. Genet. 29, 383–388. doi: 10.1038/ng764
Lemmon, M. A. (2008). Membrane recognition by phospholipid-binding domains. Nat. Rev. Mol. Cell Biol. 9, 99–111. doi: 10.1038/nrm2328
Leng, X., Wu, Y., Wang, X., Pan, Y., Wang, J., Li, J.,et al. (2011). Functional analysis of recently identified mutations in eukaryotic translation initiation factor 2Bvarepsilon (eIF2Bvarepsilon) identified in Chinese patients with vanishing white matter disease. J. Hum. Genet. 56, 300–305. doi: 10.1038/jhg.2011.9
Levine, T. P., Daniels, R. D., Gatta, A. T., Wong, L. H., and Hayes, M. J. (2013). The product of C9orf72, a gene strongly implicated in neurodegeneration, is structurally related to DENN Rab-GEFs. Bioinformatics 29, 499–503. doi: 10.1093/bioinformatics/bts725
Li, W., Wang, X., Van Der Knaap, M. S., and Proud, C. G. (2004). Mutations linked to leukoencephalopathy with vanishing white matter impair the function of the eukaryotic initiation factor 2B complex in diverse ways. Mol. Cell. Biol. 24, 3295–3306. doi: 10.1128/MCB.24.8.3295-3306.2004
Li, X., Gao, X., Liu, G., Xiong, W., Wu, J., and Rao, Y. (2008). Netrin signal transduction and the guanine nucleotide exchange factor DOCK180 in attractive signaling. Nat. Neurosci. 11, 28–35. doi: 10.1038/nn2022
Li, Z. H., Lu, J., Tay, S. S., Wu, Y. J., Strong, M. J., and He, B. P. (2006). Mice with targeted disruption of neurofilament light subunit display formation of protein aggregation in motoneurons and downregulation of complement receptor type 3 alpha subunit in microglia in the spinal cord at their earlier age: a possible feature in pre-clinical development of neurodegenerative diseases. Brain Res. 1113, 200–209. doi: 10.1016/j.brainres.2006.07.041
Liang, X., Wang, Q., Hand, T., Wu, L., Breyer, R. M., Montine, T. J.,et al. (2005). Deletion of the prostaglandin E2 EP2 receptor reduces oxidative damage and amyloid burden in a model of Alzheimer’s disease. J. Neurosci. 25, 10180–10187. doi: 10.1523/JNEUROSCI.3591-05.2005
Lin, H., Zhai, J., Canete-Soler, R., and Schlaepfer, W. W. (2004). 3′ untranslated region in a light neurofilament (NF-L) mRNA triggers aggregation of NF-L and mutant superoxide dismutase 1 proteins in neuronal cells. J. Neurosci. 24, 2716–2726. doi: 10.1523/JNEUROSCI.5689-03.2004
Lin, H., Zhai, J., Nie, Z., Wu, J., Meinkoth, J. L., Schlaepfer, W. W.,et al. (2003). Neurofilament RNA causes neurodegeneration with accumulation of ubiquitinated aggregates in cultured motor neurons. J. Neuropathol. Exp. Neurol. 62, 936–950.
Lin, H., Zhai, J., and Schlaepfer, W. W. (2005). RNA-binding protein is involved in aggregation of light neurofilament protein and is implicated in the pathogenesis of motor neuron degeneration. Hum. Mol. Genet. 14, 3643–3659. doi: 10.1093/hmg/ddi392
Lovrecic, L., Slavkov, I., Dzeroski, S., and Peterlin, B. (2010). ADP-ribosylation factor guanine nucleotide-exchange factor 2 (ARFGEF2): a new potential biomarker in Huntington’s disease. J. Int. Med. Res. 38, 1653–1662. doi: 10.1177/147323001003800510
Luigetti, M., Lattante, S., Conte, A., Romano, A., Zollino, M., Marangi, G.,et al. (2013). A novel compound heterozygous ALS2 mutation in two Italian siblings with juvenile amyotrophic lateral sclerosis. Amyotroph. Lateral Scler. Frontotemporal Degener. 14, 470–472. doi: 10.3109/21678421.2012.756036
Ma, H., Lou, Y., Lin, W. H., and Xue, H. W. (2006). MORN motifs in plant PIPKs are involved in the regulation of subcellular localization and phospholipid binding. Cell Res. 16, 466–478. doi: 10.1038/sj.cr.7310058
Ma, Y., Tang, L., Chen, L., Zhang, B., Deng, P., Wang, J.,et al. (2014). ARHGEF28 gene exon 6/intron 6 junction mutations in Chinese amyotrophic lateral sclerosis cohort. Amyotroph. Lateral Scler. Frontotemporal Degener. 15, 309–311. doi: 10.3109/21678421.2014.896926
McLean, J. R., Sanelli, T. R., Leystra-Lantz, C., He, B. P., and Strong, M. J. (2005). Temporal profiles of neuronal degeneration, glial proliferation, and cell death in hNFL(+/+) and NFL(–/–) mice. Glia 52, 59–69. doi: 10.1002/glia.20218
Meller, N., Merlot, S., and Guda, C. (2005). CZH proteins: a new family of Rho-GEFs. J. Cell Sci. 118, 4937–4946. doi: 10.1242/jcs.02671
Menzies, F. M., Grierson, A. J., Cookson, M. R., Heath, P. R., Tomkins, J., Figlewicz, D. A.,et al. (2002). Selective loss of neurofilament expression in Cu/Zn superoxide dismutase (SOD1) linked amyotrophic lateral sclerosis. J. Neurochem. 82, 1118–1128. doi: 10.1046/j.1471-4159.2002.01045.x
Meyer, D., Liu, A., and Margolis, B. (1999). Interaction of c-Jun amino-terminal kinase interacting protein-1 with p190 rhoGEF and its localization in differentiated neurons. J. Biol. Chem. 274, 35113–35118. doi: 10.1074/jbc.274.49.35113
Miller, N. L., Kleinschmidt, E. G., and Schlaepfer, D. D. (2014). RhoGEFs in cell motility: novel links between Rgnef and focal adhesion kinase. Curr. Mol. Med. 14, 221–234. doi: 10.2174/1566524014666140128110339
Mintchev, N., Zamba-Papanicolaou, E., Kleopa, K. A., and Christodoulou, K. (2009). A novel ALS2 splice-site mutation in a Cypriot juvenile-onset primary lateral sclerosis family. Neurology 72, 28–32. doi: 10.1212/01.wnl.0000338530.77394.60
Miyamoto, Y., Torii, T., Yamamori, N., Ogata, T., Tanoue, A., and Yamauchi, J. (2013). Akt and PP2A reciprocally regulate the guanine nucleotide exchange factor Dock6 to control axon growth of sensory neurons. Sci. Signal. 6:ra15. doi: 10.1126/scisignal.2003661
Miyamoto, Y., and Yamauchi, J. (2010). Cellular signaling of Dock family proteins in neural function. Cell. Signal. 22, 175–182. doi: 10.1016/j.cellsig.2009.09.036
Miyamoto, Y., Yamauchi, J., Sanbe, A., and Tanoue, A. (2007). Dock6, a Dock-C subfamily guanine nucleotide exchanger, has the dual specificity for Rac1 and Cdc42 and regulates neurite outgrowth. Exp. Cell Res. 313, 791–804. doi: 10.1016/j.yexcr.2006.11.017
Mohammad-Qureshi, S. S., Haddad, R., Hemingway, E. J., Richardson, J. P., and Pavitt, G. D. (2007). Critical contacts between the eukaryotic initiation factor 2B (eIF2B) catalytic domain and both eIF2beta and -2gamma mediate guanine nucleotide exchange. Mol. Cell. Biol. 27, 5225–5234. doi: 10.1128/MCB.00495-07
Morton, A. J., Hunt, M. J., Hodges, A. K., Lewis, P. D., Redfern, A. J., Dunnett, S. B.,et al. (2005). A combination drug therapy improves cognition and reverses gene expression changes in a mouse model of Huntington’s disease. Eur. J. Neurosci. 21, 855–870. doi: 10.1111/j.1460-9568.2005.03895.x
Murphy, M., Quinn, S., Young, J., Parkin, P., and Taylor, B. (2008). Increasing incidence of ALS in Canterbury, New Zealand: a 22-year study. Neurology 71, 1889–1895. doi: 10.1212/01.wnl.0000336653.65605.ac
Namekata, K., Enokido, Y., Iwasawa, K., and Kimura, H. (2004). MOCA induces membrane spreading by activating Rac1. J. Biol. Chem. 279, 14331–14337. doi: 10.1074/jbc.M311275200
Namekata, K., Kimura, A., Kawamura, K., Guo, X., Harada, C., Tanaka, K.,et al. (2013). Dock3 attenuates neural cell death due to NMDA neurotoxicity and oxidative stress in a mouse model of normal tension glaucoma. Cell Death Differ. 20, 1250–1256. doi: 10.1038/cdd.2013.91
Namekata, K., Kimura, A., Kawamura, K., Harada, C., and Harada, T. (2014). Dock GEFs and their therapeutic potential: neuroprotection and axon regeneration. Prog. Retin. Eye Res. doi: 10.1016/j.preteyeres.2014.06.005 [Epub ahead of print].
Namekata, K., Watanabe, H., Guo, X., Kittaka, D., Kawamura, K., Kimura, A.,et al. (2012). Dock3 regulates BDNF-TrkB signaling for neurite outgrowth by forming a ternary complex with Elmo and RhoG. Genes Cells 17, 688–697. doi: 10.1111/j.1365-2443.2012.01616.x
Nava, C., Keren, B., Mignot, C., Rastetter, A., Chantot-Bastaraud, S., Faudet, A.,et al. (2014). Prospective diagnostic analysis of copy number variants using SNP microarrays in individuals with autism spectrum disorders. Eur. J. Hum. Genet. 22, 71–78. doi: 10.1038/ejhg.2013.88
Neumann, M., Sampathu, D. M., Kwong, L. K., Truax, A. C., Micsenyi, M. C., Chou, T. T.,et al. (2006). Ubiquitinated TDP-43 in frontotemporal lobar degeneration and amyotrophic lateral sclerosis. Science 314, 130–133. doi: 10.1126/science.1134108
Nie, Z., Wu, J., Zhai, J., Lin, H., Ge, W., Schlaepfer, W. W.,et al. (2002). Untranslated element in neurofilament mRNA has neuropathic effect on motor neurons of transgenic mice. J. Neurosci. 22, 7662–7670.
Ogawa, K., Tanaka, Y., Uruno, T., Duan, X., Harada, Y., Sanematsu, F.,et al. (2014). DOCK5 functions as a key signaling adaptor that links FcepsilonRI signals to microtubule dynamics during mast cell degranulation. J. Exp. Med. 211, 1407–1419. doi: 10.1084/jem.20131926
Ohba, Y., Mochizuki, N., Matsuo, K., Yamashita, S., Nakaya, M., Hashimoto, Y.,et al. (2000). Rap2 as a slowly responding molecular switch in the Rap1 signaling cascade. Mol. Cell. Biol. 20, 6074–6083. doi: 10.1128/MCB.20.16.6074-6083.2000
Otomo, A., Hadano, S., Okada, T., Mizumura, H., Kunita, R., Nishijima, H.,et al. (2003). ALS2, a novel guanine nucleotide exchange factor for the small GTPase Rab5, is implicated in endosomal dynamics. Hum. Mol. Genet. 12, 1671–1687. doi: 10.1093/hmg/ddg184
Otomo, A., Kunita, R., Suzuki-Utsunomiya, K., Mizumura, H., Onoe, K., Osuga, H.,et al. (2008). ALS2/alsin deficiency in neurons leads to mild defects in macropinocytosis and axonal growth. Biochem. Biophys. Res. Commun. 370, 87–92. doi: 10.1016/j.bbrc.2008.01.177
Pagnamenta, A. T., Bacchelli, E., De Jonge, M. V., Mirza, G., Scerri, T. S., Minopoli, F.,et al. (2010). Characterization of a family with rare deletions in CNTNAP5 and DOCK4 suggests novel risk loci for autism and dyslexia. Biol. Psychiatry 68, 320–328. doi: 10.1016/j.biopsych.2010.02.002
Pankratz, N., Dumitriu, A., Hetrick, K. N., Sun, M., Latourelle, J. C., Wilk, J. B.,et al. (2011). Copy number variation in familial Parkinson disease. PLoS ONE 6:e20988. doi: 10.1371/journal.pone.0020988
Pavitt, G. D., Ramaiah, K. V., Kimball, S. R., and Hinnebusch, A. G. (1998). eIF2 independently binds two distinct eIF2B subcomplexes that catalyze and regulate guanine-nucleotide exchange. Genes Dev. 12, 514–526. doi: 10.1101/gad.12.4.514
Quevedo, C., Alcazar, A., and Salinas, M. (2000). Two different signal transduction pathways are implicated in the regulation of initiation factor 2B activity in insulin-like growth factor-1-stimulated neuronal cells. J. Biol. Chem. 275, 19192–19197. doi: 10.1074/jbc.M000238200
Rabiner, C. A., Mains, R. E., and Eipper, B. A. (2005). Kalirin: a dual Rho guanine nucleotide exchange factor that is so much more than the sum of its many parts. Neuroscientist 11, 148–160. doi: 10.1177/1073858404271250
Radha, V., Rajanna, A., Gupta, R. K., Dayma, K., and Raman, T. (2008). The guanine nucleotide exchange factor, C3G regulates differentiation and survival of human neuroblastoma cells. J. Neurochem. 107, 1424–1435. doi: 10.1111/j.1471-4159.2008.05710.x
Randall, K. L., Lambe, T., Johnson, A. L., Treanor, B., Kucharska, E., Domaschenz, H.,et al. (2009). Dock8 mutations cripple B cell immunological synapses, germinal centers and long-lived antibody production. Nat. Immunol. 10, 1283–1291. doi: 10.1038/ni.1820
Renton, A. E., Majounie, E., Waite, A., Simon-Sanchez, J., Rollinson, S., Gibbs, J. R.,et al. (2011). A hexanucleotide repeat expansion in C9ORF72 is the cause of chromosome 9p21-linked ALS-FTD. Neuron 72, 257–268. doi: 10.1016/j.neuron.2011.09.010
Richardson, J. P., Mohammad, S. S., and Pavitt, G. D. (2004). Mutations causing childhood ataxia with central nervous system hypomyelination reduce eukaryotic initiation factor 2B complex formation and activity. Mol. Cell. Biol. 24, 2352–2363. doi: 10.1128/MCB.24.6.2352-2363.2004
Rossman, K. L., Der, C. J., and Sondek, J. (2005). GEF means go: turning on RHO GTPases with guanine nucleotide-exchange factors. Nat. Rev. Mol. Cell Biol. 6, 167–180. doi: 10.1038/nrm1587
Sakabe, I., Asai, A., Iijima, J., and Maruyama, M. (2012). Age-related guanine nucleotide exchange factor, mouse Zizimin2, induces filopodia in bone marrow-derived dendritic cells. Immun. Ageing 9:2. doi: 10.1186/1742-4933-9-2
Shi, L. (2013). Dock protein family in brain development and neurological disease. Commun. Integr. Biol. 6:e26839. doi: 10.4161/cib.26839
Shirakawa, K., Suzuki, H., Ito, M., Kono, S., Uchiyama, T., Ohashi, T.,et al. (2009). Novel compound heterozygous ALS2 mutations cause juvenile amyotrophic lateral sclerosis in Japan. Neurology 73, 2124–2126. doi: 10.1212/WNL.0b013e3181c67be0
Sieradzan, K. A., Mechan, A. O., Jones, L., Wanker, E. E., Nukina, N., and Mann, D. M. (1999). Huntington’s disease intranuclear inclusions contain truncated, ubiquitinated huntingtin protein. Exp. Neurol. 156, 92–99. doi: 10.1006/exnr.1998.7005
Singh, C. R., Lee, B., Udagawa, T., Mohammad-Qureshi, S. S., Yamamoto, Y., Pavitt, G. D.,et al. (2006). An eIF5/eIF2 complex antagonizes guanine nucleotide exchange by eIF2B during translation initiation. EMBO J. 25, 4537–4546. doi: 10.1038/sj.emboj.7601339
Snyder, J. T., Worthylake, D. K., Rossman, K. L., Betts, L., Pruitt, W. M., Siderovski, D. P.,et al. (2002). Structural basis for the selective activation of Rho GTPases by Dbl exchange factors. Nat. Struct. Biol. 9, 468–475. doi: 10.1038/nsb796
Spillantini, M. G., Schmidt, M. L., Lee, V. M., Trojanowski, J. Q., Jakes, R., and Goedert, M. (1997). Alpha-synuclein in Lewy bodies. Nature 388, 839–840. doi: 10.1038/42166
Stieber, A., Gonatas, J. O., and Gonatas, N. K. (2000). Aggregates of mutant protein appear progressively in dendrites, in periaxonal processes of oligodendrocytes, and in neuronal and astrocytic perikarya of mice expressing the SOD1(G93A) mutation of familial amyotrophic lateral sclerosis. J. Neurol. Sci. 177, 114–123. doi: 10.1016/S0022-510X(00)00351-8
Strong, M., Sopper, M., and He, B. P. (2003). In vitro reactive nitrating species toxicity in dissociated spinal motor neurons from NFL (–/–) and hNFL (+/+) transgenic mice. Amyotroph. Lateral Scler. Other Motor Neuron Disord. 4, 81–89. doi: 10.1080/14660820310012727
Strong, M. J. (2004). Amyotrophic lateral sclerosis: contemporary concepts in etiopathogenesis and pharmacotherapy. Expert Opin. Investig. Drugs 13, 1593–1614. doi: 10.1517/13543784.13.12.1593
Strong, M. J. (2010). The evidence for altered RNA metabolism in amyotrophic lateral sclerosis (ALS). J. Neurol. Sci. 288, 1–12. doi: 10.1016/j.jns.2009.09.029
Strong, M. J., Kesavapany, S., and Pant, H. C. (2005). The pathobiology of amyotrophic lateral sclerosis: a proteinopathy? J. Neuropathol. Exp. Neurol. 64, 649–664. doi: 10.1097/01.jnen.0000173889.71434.ea
Szaro, B. G., and Strong, M. J. (2010). Post-transcriptional control of neurofilaments: new roles in development, regeneration and neurodegenerative disease. Trends Neurosci. 33, 27–37. doi: 10.1016/j.tins.2009.10.002
Sztriha, L., Panzeri, C., Kalmanchey, R., Szabo, N., Endreffy, E., Turi, S.,et al. (2008). First case of compound heterozygosity in ALS2 gene in infantile-onset ascending spastic paralysis with bulbar involvement. Clin. Genet. 73, 591–593. doi: 10.1111/j.1399-0004.2008.00993.x
Tachi, N., Hashimoto, Y., and Matsuoka, M. (2012). MOCA is an integrator of the neuronal death signals that are activated by familial Alzheimer’s disease-related mutants of amyloid beta precursor protein and presenilins. Biochem. J. 442, 413–422. doi: 10.1042/BJ20100993
Takeshima, H., Komazaki, S., Nishi, M., Iino, M., and Kangawa, K. (2000). Junctophilins: a novel family of junctional membrane complex proteins. Mol. Cell. 6, 11–22.
Thumkeo, D., Watanabe, S., and Narumiya, S. (2013). Physiological roles of Rho and Rho effectors in mammals. Eur. J. Cell Biol. 92, 303–315. doi: 10.1016/j.ejcb.2013.09.002
Tiraboschi, P., Hansen, L. A., Thal, L. J., and Corey-Bloom, J. (2004). The importance of neuritic plaques and tangles to the development and evolution of AD. Neurology 62, 1984–1989. doi: 10.1212/01.WNL.0000129697.01779.0A
Topp, J. D., Gray, N. W., Gerard, R. D., and Horazdovsky, B. F. (2004). Alsin is a Rab5 and Rac1 guanine nucleotide exchange factor. J. Biol. Chem. 279, 24612–24623. doi: 10.1074/jbc.M313504200
Tu, P. H., Gurney, M. E., Julien, J. P., Lee, V. M., and Trojanowski, J. Q. (1997). Oxidative stress, mutant SOD1, and neurofilament pathology in transgenic mouse models of human motor neuron disease. Lab. Invest. 76, 441–456.
Ueda, S., Fujimoto, S., Hiramoto, K., Negishi, M., and Katoh, H. (2008). Dock4 regulates dendritic development in hippocampal neurons. J. Neurosci. Res. 86, 3052–3061. doi: 10.1002/jnr.21763
Ueda, S., Negishi, M., and Katoh, H. (2013). Rac GEF Dock4 interacts with cortactin to regulate dendritic spine formation. Mol. Biol. Cell 24, 1602–1613. doi: 10.1091/mbc.E12-11–0782
Van Aelst, L., and D’Souza-Schorey, C. (1997). Rho GTPases and signaling networks. Genes Dev. 11, 2295–2322. doi: 10.1101/gad.11.18.2295
van Blitterswijk, M., Van Es, M. A., Hennekam, E. A., Dooijes, D., Van Rheenen, W., Medic, J.,et al. (2012). Evidence for an oligogenic basis of amyotrophic lateral sclerosis. Hum. Mol. Genet. 21, 3776–3784. doi: 10.1093/hmg/dds199
Vance, C., Rogelj, B., Hortobagyi, T., De Vos, K. J., Nishimura, A. L., Sreedharan, J.,et al. (2009). Mutations in FUS, an RNA processing protein, cause familial amyotrophic lateral sclerosis type 6. Science 323, 1208–1211. doi: 10.1126/science.1165942
van der Knaap, M. S., Leegwater, P. A., Konst, A. A., Visser, A., Naidu, S., Oudejans, C. B.,et al. (2002). Mutations in each of the five subunits of translation initiation factor eIF2B can cause leukoencephalopathy with vanishing white matter. Ann. Neurol. 51, 264–270. doi: 10.1002/ana.10112
van Horck, F. P., Ahmadian, M. R., Haeusler, L. C., Moolenaar, W. H., and Kranenburg, O. (2001). Characterization of p190RhoGEF, a RhoA-specific guanine nucleotide exchange factor that interacts with microtubules. J. Biol. Chem. 276, 4948–4956. doi: 10.1074/jbc.M003839200
van Rijssel, J., and van Buul, J. D. (2012). The many faces of the guanine-nucleotide exchange factor trio. Cell Adh. Migr. 6, 482–487. doi: 10.4161/cam.21418
Vanderweyde, T., Youmans, K., Liu-Yesucevitz, L., and Wolozin, B. (2013). Role of stress granules and RNA-binding proteins in neurodegeneration: a mini-review. Gerontology 59, 524–533. doi: 10.1159/000354170
Verschuuren-Bemelmans, C. C., Winter, P., Sival, D. A., Elting, J. W., Brouwer, O. F., and Muller, U. (2008). Novel homozygous ALS2 nonsense mutation (p.Gln715X) in sibs with infantile-onset ascending spastic paralysis: the first cases from northwestern Europe. Eur. J. Hum. Genet. 16, 1407–1411. doi: 10.1038/ejhg.2008.108
Villarroel-Campos, D., Gastaldi, L., Conde, C., Caceres, A., and Gonzalez-Billault, C. (2014). Rab-mediated trafficking role in neurite formation. J. Neurochem. 129, 240–248. doi: 10.1111/jnc.12676
Volkening, K., Leystra-Lantz, C., and Strong, M. J. (2010). Human low molecular weight neurofilament (NFL) mRNA interacts with a predicted p190RhoGEF homologue (RGNEF) in humans. Amyotroph. Lateral Scler. 11, 97–103. doi: 10.3109/17482960902995584
Voss, A. K., Britto, J. M., Dixon, M. P., Sheikh, B. N., Collin, C., Tan, S. S.,et al. (2008). C3G regulates cortical neuron migration, preplate splitting and radial glial cell attachment. Development 135, 2139–2149. doi: 10.1242/dev.016725
Voss, A. K., Krebs, D. L., and Thomas, T. (2006). C3G regulates the size of the cerebral cortex neural precursor population. EMBO J. 25, 3652–3663. doi: 10.1038/sj.emboj.7601234
Wakil, S. M., Ramzan, K., Abuthuraya, R., Hagos, S., Al-Dossari, H., Al-Omar, R.,et al. (2014). Infantile-onset ascending hereditary spastic paraplegia with bulbar involvement due to the novel ALS2 mutation c.2761C>T. Gene 536, 217–220. doi: 10.1016/j.gene.2013.11.043
Wang, X., Paulin, F. E., Campbell, L. E., Gomez, E., O’brien, K., Morrice, N.,et al. (2001). Eukaryotic initiation factor 2B: identification of multiple phosphorylation sites in the epsilon-subunit and their functions in vivo. EMBO J. 20, 4349–4359. doi: 10.1093/emboj/20.16.4349
Watabe-Uchida, M., John, K. A., Janas, J. A., Newey, S. E., and Van Aelst, L. (2006). The Rac activator DOCK7 regulates neuronal polarity through local phosphorylation of stathmin/Op18. Neuron 51, 727–739. doi: 10.1016/j.neuron.2006.07.020
Wek, R. C., Jiang, H. Y., and Anthony, T. G. (2006). Coping with stress: eIF2 kinases and translational control. Biochem. Soc. Trans. 34, 7–11. doi: 10.1042/BST20060007
Welsh, G. I., Miller, C. M., Loughlin, A. J., Price, N. T., and Proud, C. G. (1998). Regulation of eukaryotic initiation factor eIF2B: glycogen synthase kinase-3 phosphorylates a conserved serine which undergoes dephosphorylation in response to insulin. FEBS Lett. 421, 125–130. doi: 10.1016/S0014-5793(97)01548-2
Wong, N. K., He, B. P., and Strong, M. J. (2000). Characterization of neuronal intermediate filament protein expression in cervical spinal motor neurons in sporadic amyotrophic lateral sclerosis (ALS). J. Neuropathol. Exp. Neurol. 59, 972–982.
Wright, J., Kahn, R. A., and Sztul, E. (2014). Regulating the large Sec7 ARF guanine nucleotide exchange factors: the when, where and how of activation. Cell. Mol. Life Sci. 71, 3419–3438. doi: 10.1007/s00018-014-1602-7
Wu, J., Zhai, J., Lin, H., Nie, Z., Ge, W. W., Garcia-Bermejo, L.,et al. (2003). Cytoplasmic retention sites in p190RhoGEF confer anti-apoptotic activity to an EGFP-tagged protein. Brain Res. Mol. Brain Res. 117, 27–38. doi: 10.1016/S0169-328X(03)00263-8
Xiao, Y., Peng, Y., Wan, J., Tang, G., Chen, Y., Tang, J.,et al. (2013). The atypical guanine nucleotide exchange factor Dock4 regulates neurite differentiation through modulation of Rac1 GTPase and actin dynamics. J. Biol. Chem. 288, 20034–20045. doi: 10.1074/jbc.M113.458612
Xu, N. J., and Henkemeyer, M. (2009). Ephrin-B3 reverse signaling through Grb4 and cytoskeletal regulators mediates axon pruning. Nat. Neurosci. 12, 268–276. doi: 10.1038/nn.2254
Yamauchi, J., Miyamoto, Y., Chan, J. R., and Tanoue, A. (2008). ErbB2 directly activates the exchange factor Dock7 to promote Schwann cell migration. J. Cell Biol. 181, 351–365. doi: 10.1083/jcb.200709033
Yamauchi, J., Miyamoto, Y., Hamasaki, H., Sanbe, A., Kusakawa, S., Nakamura, A.,et al. (2011). The atypical Guanine-nucleotide exchange factor, dock7, negatively regulates schwann cell differentiation and myelination. J. Neurosci. 31, 12579–12592. doi: 10.1523/JNEUROSCI.2738-11.2011
Yang, Y., Hentati, A., Deng, H. X., Dabbagh, O., Sasaki, T., Hirano, M.,et al. (2001). The gene encoding alsin, a protein with three guanine-nucleotide exchange factor domains, is mutated in a form of recessive amyotrophic lateral sclerosis. Nat. Genet. 29, 160–165. doi: 10.1038/ng1001-160
Yang, Y. T., Wang, C. L., and Van Aelst, L. (2012). DOCK7 interacts with TACC3 to regulate interkinetic nuclear migration and cortical neurogenesis. Nat. Neurosci. 15, 1201–1210. doi: 10.1038/nn.3171
Yu, B., Martins, I. R., Li, P., Amarasinghe, G. K., Umetani, J., Fernandez-Zapico, M. E.,et al. (2010). Structural and energetic mechanisms of cooperative autoinhibition and activation of Vav1. Cell 140, 246–256. doi: 10.1016/j.cell.2009.12.033
Yu, H. G., Nam, J. O., Miller, N. L., Tanjoni, I., Walsh, C., Shi, L.,et al. (2011). p190RhoGEF (Rgnef) promotes colon carcinoma tumor progression via interaction with focal adhesion kinase. Cancer Res. 71, 360–370. doi: 10.1158/0008-5472.CAN-10-2894
Zerial, M., and McBride, H. (2001). Rab proteins as membrane organizers. Nat. Rev. Mol. Cell Biol. 2, 107–117. doi: 10.1038/35052055
Zhai, J., Lin, H., Nie, Z., Wu, J., Canete-Soler, R., Schlaepfer, W. W.,et al. (2003). Direct interaction of focal adhesion kinase with p190RhoGEF. J. Biol. Chem. 278, 24865–24873. doi: 10.1074/jbc.M302381200
Zhai, J., Lin, H., Shamim, M., Schlaepfer, W. W., and Canete-Soler, R. (2001). Identification of a novel interaction of 14-3-3 with p190RhoGEF. J. Biol. Chem. 276, 41318–41324. doi: 10.1074/jbc.M107709200
Keywords: neurofilament, RNA binding proteins, GEF, neurodegeneration, motor neuron disease
Citation: Droppelmann CA, Campos-Melo D, Volkening K and Strong MJ (2014) The emerging role of guanine nucleotide exchange factors in ALS and other neurodegenerative diseases. Front. Cell. Neurosci. 8:282. doi: 10.3389/fncel.2014.00282
Received: 11 July 2014; Paper pending published: 08 August 2014;
Accepted: 25 August 2014; Published online: 10 September 2014.
Edited by:
Trisha Stankiewicz, University of Denver, USAReviewed by:
Mario Buffelli, University of Verona, ItalyGunnar P. H. Dietz, Schwabe Pharma Deutschland, Germany
Copyright © 2014 Droppelmann, Campos-Melo, Volkening and Strong. This is an open-access article distributed under the terms of the Creative Commons Attribution License (CC BY). The use, distribution or reproduction in other forums is permitted, provided the original author(s) or licensor are credited and that the original publication in this journal is cited, in accordance with accepted academic practice. No use, distribution or reproduction is permitted which does not comply with these terms.
*Correspondence: Michael J. Strong, Department of Clinical Neurological Sciences, Schulich School of Medicine & Dentistry, Western University, Room C7-120, University Hospital, LHSC, 339 Windermere Road, London, ON N6A 5A5, Canada e-mail: michael.strong@schulich.uwo.ca