- 1Department of Infectious Diseases and General Internal Medicine, University Hospital of Liège, Liège, Belgium
- 2Department of General Practice, University Hospital of Liège, Liège, Belgium
- 3Department of Animal Pathology, Fundamental and Applied Research for Animals and Health, University of Liège, Liège, Belgium
- 4Department of Clinical Microbiology, University Hospital of Liège, Liège, Belgium
- 5Functional and Evolutionary Entomology, Gembloux Agro-Bio Tech, University of Liège, Gembloux, Belgium
Climate change heavily threatens planetary and human health. Arboviral infections are best studied using the One Health concept, due to their reliance on complex interactions between environmental factors, arthropod vectors and vertebrate hosts. This review focuses on two arboviruses, namely West Nile Virus (WNV) and Usutu Virus (USUV), both causing emerging public health issues in northern Europe. They are both maintained in an enzootic cycle involving birds and Culex spp mosquitoes. WNV has demonstrated its sensitivity to the consequences of climate change and there is already evidence that global warming contributes to its expansion in Europe. Future WNV indigenous transmission in northern Europe is therefore plausible. Usutu is a lesser known arbovirosis, sharing similar vectors and hosts as WNV. USUV has a similar geographic expansion to WNV, exhibiting some level of co-circulation. It is therefore crucial to monitor these viruses in the hitherto relatively spared regions of northern Europe.
1 Introduction
The climate is changing (1). While it is still of crucial importance to mitigate these changes, it is also increasingly recognized that it cannot be avoided and have now to be taken into account (2).
Human health, in particular, is heavily threatened by future and current climate changes (2). One of the domains in which global change impacts human public health is related to infectious diseases (3). Among these, some are of particular interest in the OneHealth perspective: arboviral zoonoses. Arboviruses – arthropod-borne viruses – depend on the biology of arthropods, which are ectothermic animals, whose physiology is therefore largely driven by the abiotic parameters controlled by the local and global climate (4). Therefore, arboviruses are extremely influenced by the climate changes (4, 5). Most of arboviral diseases are zoonotic, and the implication of non-human hosts, often acting like reservoirs, increases the complexity of the epidemiology and the difficulty to control the associated diseases (6).
In this review, we focus on two arboviruses, namely the West Nile and Usutu viruses, to illustrate zoonose cases.
2 West Nile virus
2.1 Virus and cycle
West Nile Virus (WNV), a member of the Flaviviridae family, was first isolated in 1937 in Uganda (7). If the strains were originally grouped into two lineages, today 7 of them are recognized, among which the most common in Europe are lineage 1a and lineage 2 (8).
The main cycle (illustrated in Figure 1) of this WNV is sylvatic and involves birds, mainly passerines, and several species of mosquitoes, mostly belonging to the Culex genus (10). Mammals, especially humans and horses, can be infected, but usually constitute dead-end hosts, meaning they do not develop sufficient viremia to infect mosquitoes during a blood meal (11). Other uncommon hosts are reptiles and amphibians, with diverse levels of viraemia and altogether only an anecdotical contribution to the overall viral circulation and impact (12). West Nile Virus can also, even rarely, be transmitted via transfusion of infected blood products (13). Congenital infection has also, albeit uncommonly, been reported (14). Occupational exposure can occur among workers in close contact with animals or in a laboratory facility (15).
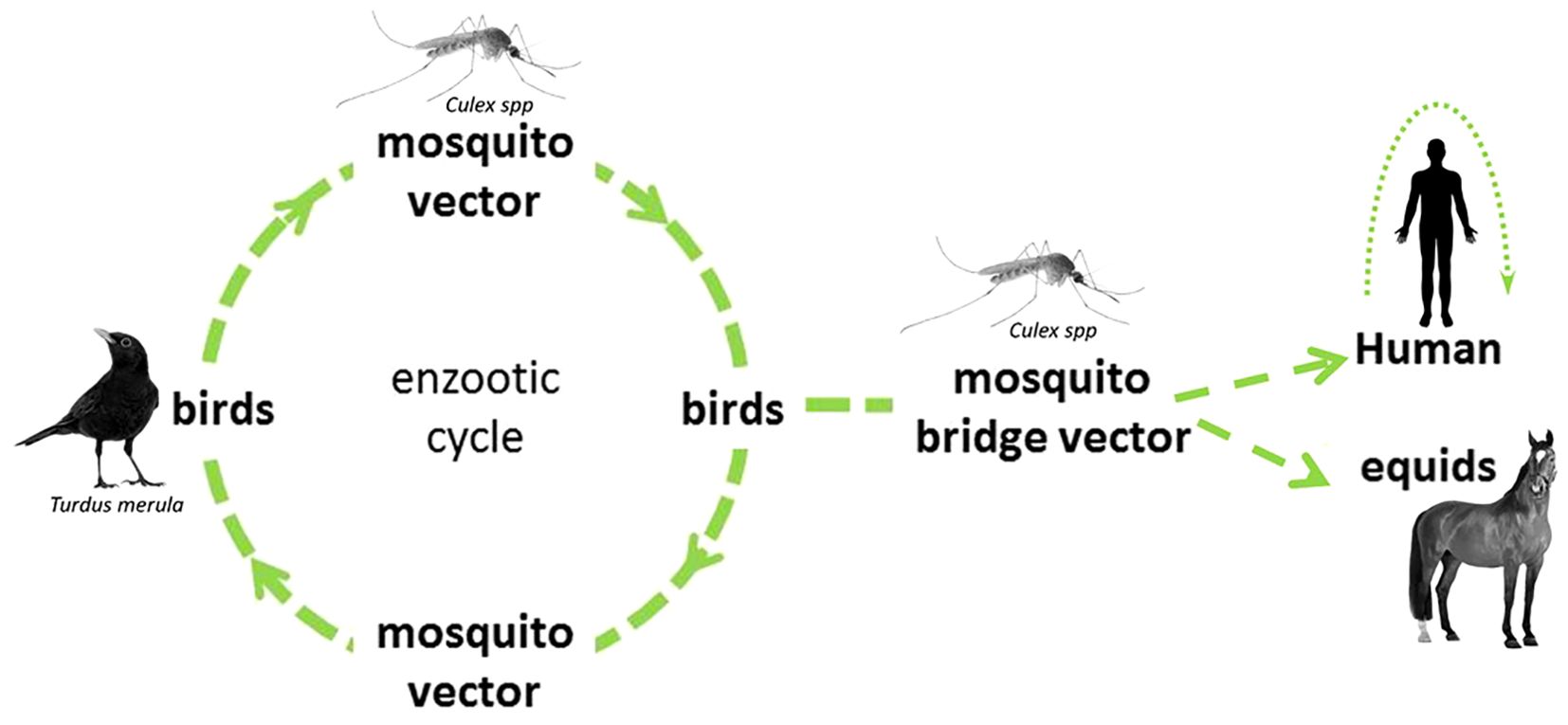
Figure 1. WNV cycle, with an illustration of most common hosts in Europe. Modified from ECDC (9).
In Europe, Cx pipiens and Cx modestus are the main proved competent vectors for the transmission of the WNV (16–18). The first is the most common, widely present in all Europe, with a well-known competence as an arboviral vector. Two distinct forms (or biotypes) are described. Culex pipiens f. pipiens is mostly ornithophilic, capable of diapause and found in open urban and peri-urban habitats (19, 20) while Cx pipiens f. molestus is mostly found in underground urban areas, do not enter diapause, mate in confined space and bite mammals (19, 20). A certain degree of hybridization is found to occur in zones of sympatry and the hybrids Cx pipiens pipiens x molestus feed on both avian and mammal species, constituting a potential bridge vector (20–22).
Culex modestus, firstly described in 1889 in Italy, is historically found in central and southern Europe, where it breeds in permanent water bodies in rural zones (23). In recent years, it has been found to be established in ever northern parts of the European continent (24), including in Denmark since 2011 (25), in United Kingdom since 2010 (26) and in Belgium since 2018 (27). The hypothesis of a recent introduction in United Kingdom (the alternative being an only recent correct identification of a long-established population) is supported by an analysis of genetic diversity in Europe (28). In addition to what seems to be an expansion of its geographical range, Cx modestus also shows an increase in abundance in its historical endemic zone in central and southern Europe (29, 30). Its competence as a WNV vector has been proven both in laboratory and in-field conditions (23, 31). Culex modestus is considered a bridge vector, as it feeds on avian population and on humans (23). Also, Ae. albopictus, the tiger mosquito, demonstrates its competence for the transmission of WNV under laboratory condition. Its role in the circulation of the virus is likely limited due to its feeding preference for mammals, which constitute dead-end hosts (16, 18, 32).
2.2 Clinical manifestations
Birds, as the main reservoir of WNV, are to variable degrees affected by the disease. Birds species more commonly affected by WNV are passerines species (33, 34). Strains belonging to the lineage 1 of WNV, and in particular NY, the strain introduced in North America in 1999, show higher virulence among avian species (34). Variants in non-structural proteins of the virus constitute some of the determinants of this higher virulence (35, 36). Mortality from WNV infection in birds is most often related to a multisystemic disease, with a particular tropism of the lesions for cerebral and cardiac tissues (37). Infected birds can show an alteration of general condition as well as diverse neurological signs (38).
Human WNV infection is most often asymptomatic. It is responsible for a mild flu-like disease, with fever and headache in 20% of cases (39). However, it also causes a potentially severe neuroinvasive disease (meningitis, encephalitis or flaccid paralysis) in less than 1% of the cases (39, 40). Risk factors for severe disease include older age, male gender and the presence of comorbidities (41, 42). The mortality rate of the neuroinvasive WNV disease is close to 10%, with high variability depending on the syndrome (flaccid paralysis being the most fatal) (43, 44). In 50% of cases, survivors present long-term after-effects (mainly motor and neuropsychological) (44).
The West Nile Virus also affects horses. The proportion of neurological manifestations is higher, reaching 20% and mortality rate is around 30% (45–47). Neurological manifestations in horses are diverse, with ataxia as the most common finding (48, 49). As in birds, NY99 is suspected to show a higher virulence towards equine species (49).
2.3 Current distribution
WNV is historically enzootic in the Old World, particularly in Africa and a southeastern part of Europe (50). Its human circulation in Europe remained limited to sporadic cases and limited outbreaks in the south of the territory until 1990s (51). In 1999, the virus was imported to the USA, where it colonized the territory with astonishing speed, until becoming endemic (32). In the 1990s, WNV was also caused its first major epidemics in Eastern Europe (50). The West Nile Virus spreads to new territories mainly occurs via infected birds (migratory or passively imported), leading to potential establishment if a suitable vector and a reservoir host are present (8). The WNV circulation can remain unnoticed, within an enzootic cycle, for months or even years before the occurrence of a human case (8). The virus is currently circulating in Europe mainly around the Mediterranean basin and in the southeastern part of the territory (notably in certain regions of France, Germany, Italy, Austria, Spain, Greece), where it is considered endemic (8, 52–54).
The 2018 year was an exceptional time in terms of cases number in Europe, with 2083 indigenous infections, which was more than the cumulative numbers of cases since 2010 (55). Even if the yearly number of human indigenous infections in Europe has dropped during the years after this exceptional season, the pattern of geographical expansion, especially towards northern European countries, persists (56). In 2023, human cases were reported in 11 European countries (57).
The Netherlands case exemplifies the way this geographical expansion can be observed, with first cases of birds (common whitethroat) and mosquitoes indigenous infection by WNV reported in August 2020, in the region of Utrecht, with a rapid subsequent information given to healthcare providers (58). In October of the same year, the first human indigenous case was diagnosed in the same region, leading to 5 more retrospective diagnoses among patients with unexplained encephalitis and their relatives (59). These successful early detections, contributing to the global knowledge of the underlying mechanisms of the geographical colonization of WNV, took place in the context of a national monitoring of arbovirosus with a structural One Health approach (60).
Before 2010, the main lineage identified during outbreaks in Europe was Lineage 1a. A shift has occurred and since 2013, Lineage 2 is the dominant lineage (61). This lineage shift is associated with the northward expansion and overwintering capacity of the virus (61).
2.4 Impact of climate change
Climate change involves an increase in average as well as extreme temperatures, and altered rainfall, leading to an increased risk of both flooding and drought episodes.
Life history traits of Cx. pipiens are heavily altered by increasing mean temperatures, with an increase in development rate (especially below 24°C) and an increase in mortality (especially above 28°C) (62). Models integrating these effects associated to field studies showed that the maximum biting adult population is reached for a mean temperature around 25°C (63). This optimal temperature could even be higher for southern populations of Cx. pipiens (64). Temporality of the exposure to higher temperature also plays a role, with high temperatures in spring resulting in an earlier onset of the breeding season and an increase in the total number of biting mosquitoes while high summer temperatures results in an increase of adult mortality (65).
Increase of temperature causes a shortening of the incubation period and an increase in infectivity for WNV in the mosquito (66, 67). Depending on the strain, the effect of the temperature could even be non-linear (68). The environmental factors that best predict a WNV outbreak include high summer and annual temperature, low precipitation and the presence of wetlands (69–73). A particularly mild winter could also be a factor involved (74).
Rainfall regimen has also a strong impact on mosquitoes’ population. An increase in (early) spring precipitation increases the summer population of Cx. pipiens (65). On the opposite, drought during the summer seems to maintain suitable larval habitats undisturbed during the breeding season and then to promote mosquitoes proliferation (75). Drought also increases the density of contacts between the vectors and the birds, favoring the WNV circulation (76, 77).
Another complex and less-known impact of climatic and global change on the distribution and outbreaks of WNV is thought to happen via their effect on the population of birds (71). Migratory routes are altered by climatic change (78), and migratory birds have been observed to reach their breeding locations earlier as an effect of rising temperatures (79, 80). This could have an effect on the timing, location and intensity of the introductions of WNV in Europe. Moreover, there is a concern that highly-vulnerable birds, in response to the loss and fragmentation of their habitat, thrive in a more urban habitat, closer to human populations (81). It is still difficult to assess precisely the part of endemicity and the part of annual reintroduction via migratory birds, stressing the importance of a deeper understanding of the complex interactions between the changing climate, the avian populations, the mosquito vectors and human health (82).
It is therefore not a surprise if the environmental factors that best predict a WNV human outbreak include mild winter, hot summer and warm annual temperature, low precipitation and the presence of wetlands (69–74, 83, 84). In northern Europe, epidemics could happen following an introduction of the virus during late Spring (85). They would be best predicted by high summer temperatures (86).
Aside from climate changes, other human-related global changes are proven to have a high impact on infectious, especially arboviral and zoonotic, diseases (87, 88). In particular, globalization and introduction of invasive species are well-known factors driving the colonization of already climatically-suitable habitats (88, 89). Reduction of biodiversity and wildlife could lead to a decrease in non-amplifying hosts, decreasing their dilution effect and increasing the circulation of the virus (90). Changes in agricultural methods towards deforestation, intensification and increased irrigation causes an increase of the interfaces between different species with an increased risk of spillover, a reduction of biodiversity and the creation of habitats suitable for the breeding of mosquitoes (91–93). Urbanization, human population growth and migration also contribute to the fragmentation of habitats, the reduction of biodiversity and to an increase of the human population at risk (87).
There is already evidence that global change contributes to the current increase of incidence and the geographical expansion of WNV in Europe (94–96). Projections predict an increase in WNV transmission in Europe, even in the northern regions, during the next decades (97, 98). In North America, an expansion of the range of the disease is also predicted at the continental scale by the end of the century (99). It therefore seems plausible that in the short-term autochthonous cases of WNV will be observed in Northern Europe.
3 Usutu
3.1 Virus and cycle
Usutu virus (USUV) is an Orthoflavivirus, first isolated in 1958 in South Africa from a Culex neavei mosquito (100). It is closely related to WNV and undergoes a sylvatic cycle involving several species of birds (the predominant host being blackbirds) and mosquitoes (100–102). Mosquito vectors appear to belong mostly to the Culex genus, with Cx. pipiens as a proven competent vector in Europe (103, 104). It could also play a role in the virus winter persistence (in female overwintering mosquitoes and by vertical transmission) (105). Culex modestus could also support the spread of the virus and act as a bridge vector (106, 107). The competence of Ae. albopictus for Usutu transmission is still discussed, with contradictory results between experimental infections and in-field contaminations (104, 108). Interestingly, co-circulation of both viruses is widely demonstrated in Europe (109–112). Like for WNV, humans (and to a lesser extent, other mammals species such as horses) are accidental, dead-end hosts of Usutu (101, 113).
Until the beginning of the century, USUV infections had never been reported outside the African continent, where only two (mild) human cases have been observed (114). In 2001, a significant mortality excess among avian populations in Austria highlighted the USUV circulation on the European continent (100). Retrospectively, evidence of its presence was found already in 1996 in Tuscany, Italy (115). Phylogenetic studies have since concluded that USUV was introduced several times from Africa to Spain, Austria and Germany during the last century, but started to spread in the European continent after 2000 (116, 117). After 2001, the similarities with the spectacular spread of WNV in the USA, highlighted by a massive die-off of local birds, have attracted attention and a surveillance network was put in place. Circulation of the virus has since been observed in many European countries (118). Northwestern European countries are not spared, with human as well as bird cases reported in Germany, France and the Netherlands (54, 118–120) (see also Figure 2). In Belgium and Luxembourg, to date no human case has been reported, but the virus has been identified in birds (118, 121). Epizootics affecting several countries in Northern Europe were recorded in 2016 and 2018 (101, 122).
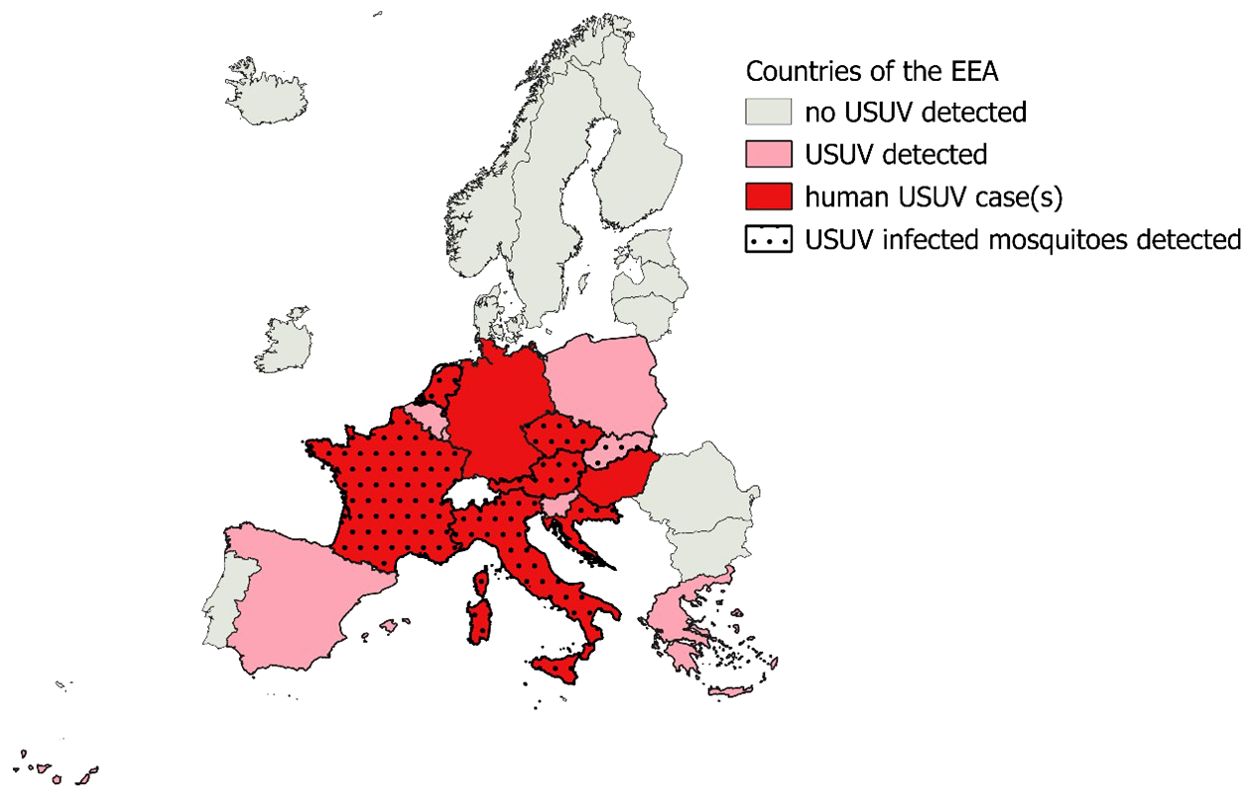
Figure 2. Detection in mosquitoes or vertebrate hosts of Usutu Virus in countries of the European Environment Agency 2012-2021. Data from the ECDC (118).
3.2 Clinical manifestations and detection
Usutu virus is a common cause of disease and death in reservoirs birds, mainly passerines and raptors. Clinical syndromes caused by the virus are still poorly understood but include neurological involvement (torticollis, seizures, opistothonos, inability to fly) as well as unspecific signs (apathy, anorexia) (122, 123)
The exact human pathogenicity of the Usutu virus remains unknown. In 2012, a seroprevalence study on blood from donors in Italy and Germany demonstrated the silent circulation of the virus in the human population (124, 125). Two first cases of neuroinvasive syndrome were demonstrated in 2009 in Italy, in two severely immunocompromised patients (126, 127). Since then, several neuroinvasive syndromes (mainly encephalitis and meningoencephalitis) have been reported in Europe, some in immunocompetent patients (111, 112, 128–131). Mild symptomatic flu-like cases have also been identified, but the proportion is difficult to assess in such a small sample (around 100 cases in Europe) (128, 131).
To date, no clinical cases of USUV infection have been described in equids, which can present a subclinical seroconversion and serve as a sentinel species to detect the circulation of the virus (132, 133).
The large co-circulation between USUV and the closely related flavivirus WNV adds another layer of complexity to the already difficult detection of this virus (134). The similarities of surface proteins of the two viruses causes a cross-reactivity of antibodies and makes it difficult to distinguish between the serological response against WNV and USUV (135). Even when using the virus neutralization test, a cross-neutralization occurs with a certain level of asymmetry at the expense of USUV detection (136).
3.3 Impact of climate change
Since the emergence of USUV in Europe, the impact of climate change on the intensification and spreading of the circulation of the virus has been questioned. There is a suspected association between climatic factors (abnormally high temperatures), the local abundance of the vector and significant circulation of the virus (120). A model developed in Austria in 2009, based on different climatic change scenarios showed a high risk of endemicity of USUV in Central Europe (137).
This climate change susceptibility, together with the current increase of the geographical range of the USUV and the probable underestimation of its current circulation suggest a current and future impact of this virus on human health in northern European countries and strongly advocate for a better surveillance of the circulation of this virus.
4 Conclusions
West Nile and Usutu viruses are closely related and ecologically similar Orthoflaviviruses, transmitted by Culex spp for which birds are amplifying hosts and humans are dead-end, accidental hosts. They both have a recent history of rapid expansion to ever more northern regions of Europe and of a dramatic global increase in the number of human cases. They show a large co-circulation and important antigenic similarities making their discrimination difficult.
Their reliance on an ectothermic vector is a key – but not unique – factor of the observed and predicted impact of climate change on the distribution of the diseases they cause. Aside for anthropogenic climate change, human actions have multiple direct and indirect impacts on their circulation. Modelization and observational data converge to identify Northern Europe as a territory at risk for future outbreaks and establishment of USUV and WNV.
The complexity and multiplicity of anthropogenic changes and their effects on per se complex cycles of transmission make it illusory to try to identify predictive factors for the transmission of the virus by focusing only on one aspect of its circulation. It is therefore a subject whose importance in terms of public and global health and whose multi-disciplinary nature - requiring the collaboration of specialists in climatology, virology, entomology, human and veterinary medicine - strongly advocate a One Health approach.
Author contributions
JL: Conceptualization, Writing – original draft, Writing – review & editing. HA: Writing – review & editing. PB: Writing – review & editing. DD: Writing – review & editing. FF: Writing – review & editing. M-MG: Writing – review & editing. M-PH: Writing – review & editing. AL: Writing – review & editing. GD: Methodology, Supervision, Writing – review & editing.
Funding
The author(s) declare financial support was received for the research, authorship, and/or publication of this article. GD is a postdoctoral clinical master specialist for the Belgian National Fund for Scientific Re-search.
Conflict of interest
The authors declare that the research was conducted in the absence of any commercial or financial relationships that could be construed as a potential conflict of interest.
The author(s) declared that they were an editorial board member of Frontiers, at the time of submission. This had no impact on the peer review process and the final decision.
Generative AI statement
The author(s) declare that no Generative AI was used in the creation of this manuscript.
Publisher’s note
All claims expressed in this article are solely those of the authors and do not necessarily represent those of their affiliated organizations, or those of the publisher, the editors and the reviewers. Any product that may be evaluated in this article, or claim that may be made by its manufacturer, is not guaranteed or endorsed by the publisher.
References
1. AR6 synthesis report: climate change (2023). Available online at: https://www.ipcc.ch/report/ar6/syr/ (Accessed August 7, 2024).
2. Romanello M, Di Napoli C, Drummond P, Green C, Kennard H, Lampard P, et al. The 2022 report of the Lancet Countdown on health and climate change: health at the mercy of fossil fuels. Lancet. (2022) 400:1619–54. doi: 10.1016/S0140-6736(22)01540-9
3. Jones KE, Patel NG, Levy MA, Storeygard A, Balk D, Gittleman JL, et al. Global trends in emerging infectious diseases. Nature. (2008) 451:990–3. doi: 10.1038/nature06536
4. Ma J, Guo Y, Gao J, Tang H, Xu K, Liu Q, et al. Climate change drives the transmission and spread of vector-borne diseases: an ecological perspective. Biol (Basel). (2022) 11:1628. doi: 10.3390/biology11111628
5. Jánová E. Emerging and threatening vector-borne zoonoses in the world and in Europe: a brief update. Pathog Glob Health. (2019) 113:49–57. doi: 10.1080/20477724.2019.1598127
6. Leal Filho W, Ternova L, Parasnis SA, Kovaleva M, Nagy GJ. Climate change and zoonoses: A review of concepts, definitions, and bibliometrics. Int J Environ Res Public Health. (2022) 19:893. doi: 10.3390/ijerph19020893
7. Smithburn KC, Hughes TP, Burke AW, Paul JH. A neurotropic virus isolated from the blood of a native of Uganda. Am J Trop Med Hygiene. (1940) s1-20:471–92. doi: 10.4269/ajtmh.1940.s1-20.471
8. Rizzoli A, Jiménez-Clavero MA, Barzon L, Cordioli P, Figuerola J, Koraka P, et al. The challenge of West Nile virus in Europe: knowledge gaps and research priorities. Eurosurveillance. (2015) 20:21135. doi: 10.2807/1560-7917.ES2015.20.20.21135
9. Factsheet about West Nile virus infection (2010). Available online at: https://www.ecdc.europa.eu/en/west-nile-fever/facts (Accessed September 2, 2024).
10. Petersen LR, Brault AC, Nasci RS. West nile virus: review of the literature. JAMA. (2013) 310:308–15. doi: 10.1001/jama.2013.8042
11. Kramer LD, Li J, Shi PY. West nile virus. Lancet Neurology. (2007) 6:171–81. doi: 10.1016/S1474-4422(07)70030-3
12. Zeller HG, Schuffenecker I. West nile virus: an overview of its spread in europe and the mediterranean basin in contrast to its spread in the americas. Eur J Clin Microbiol Infect Dis. (2004) 23:147–56. doi: 10.1007/s10096-003-1085-1
13. Pealer LN, Marfin AA, Petersen LR, Lanciotti RS, Page PL, Stramer SL, et al. Transmission of west nile virus through blood transfusion in the United States in 2002. N Engl J Med. (2003) 349:1236–45. doi: 10.1056/NEJMoa030969
14. Howard-Jones AR, Pham D, Sparks R, Maddocks S, Dwyer DE, Kok J, et al. Arthropod-borne flaviviruses in pregnancy. Microorganisms. (2023) 11:433. doi: 10.3390/microorganisms11020433
15. Odigie AE, Stufano A, Schino V, Zarea AAK, Ndiana LA, Mrenoshki D, et al. West nile virus infection in occupational settings-A systematic review. Pathogens. (2024) 13:157. doi: 10.3390/pathogens13020157
16. Vogels CB, Göertz GP, Pijlman GP, Koenraadt CJ. Vector competence of European mosquitoes for West Nile virus. Emerg Microbes Infect. (2017) 6:e96. doi: 10.1038/emi.2017.82
17. Martinet JP, Ferté H, Failloux AB, Schaffner F, Depaquit J. Mosquitoes of north-western europe as potential vectors of arboviruses: A review. Viruses. (2019) 11:1059. doi: 10.3390/v11111059
18. Martinet JP, Bohers C, Vazeille M, Ferté H, Mousson L, Mathieu B, et al. Assessing vector competence of mosquitoes from northeastern France to West Nile virus and Usutu virus. PloS Negl Trop Dis. (2023) 17:e0011144. doi: 10.1371/journal.pntd.0011144
19. Aardema ML, Olatunji SK, Fonseca DM. The enigmatic culex pipiens (Diptera: culicidae) species complex: phylogenetic challenges and opportunities from a notoriously tricky mosquito group. Ann Entomological Soc America. (2022) 115:95–104. doi: 10.1093/aesa/saab038
20. Osório HC, Zé-Zé L, Amaro F, Nunes A, Alves MJ. Sympatric occurrence of Culex pipiens (Diptera, Culicidae) biotypes pipiens, molestus and their hybrids in Portugal, Western Europe: feeding patterns and habitat determinants. Med Vet Entomol. (2014) 28:103–9. doi: 10.1111/mve.12020
21. Fonseca DM, Keyghobadi N, Malcolm CA, Mehmet C, Schaffner F, Mogi M, et al. Emerging vectors in the culex pipiens complex. Science. (2004) 303:1535–8. doi: 10.1126/science.1094247
22. Kent RJ, Harrington LC, Norris DE. Genetic differences between Culex pipiens f. molestus and Culex pipiens pipiens (Diptera: Culicidae) in New York. J Med Entomology. (2007) 44:50–9. doi: 10.1093/jmedent/41.5.50
23. Soto A, Delang L. Culex modestus: the overlooked mosquito vector. Parasit Vectors. (2023) 16:373. doi: 10.1186/s13071-023-05997-6
24. Culex modestus - current known distribution: October 2023 (2023). Available online at: https://www.ecdc.europa.eu/en/publications-data/culex-modestus-current-known-distribution-october-2023 (Accessed March 27, 2024).
25. Bødker R, Klitgård K, Byriel DB, Kristensen B. Establishment of the West Nile virus vector, Culex modestus, in a residential area in Denmark. J Vector Ecology. (2014) 39:1–3. doi: 10.1111/jvec.12121
26. Golding N, Nunn MA, Medlock JM, Purse BV, Vaux AGC, Schäfer SM. West Nile virus vector Culex modestus established in southern England. Parasit Vectors. (2012) 5:32. doi: 10.1186/1756-3305-5-32
27. Wolf K DE, Vanderheyden A, Deblauwe I, Smitz N, Gombeer S, Vanslembrouck A, et al. First record of the West Nile virus bridge vector Culex modestus Ficalbi (Diptera: Culicidae) in Belgium, validated by DNA barcoding. Zootaxa. (2021) 4920:zootaxa.4920.1.7. doi: 10.11646/zootaxa.4920.1.7
28. Hernández-Triana LM, Brugman VA, Pramual P, Barrero E, Nikolova NI, Ruiz-Arrondo I, et al. Genetic diversity and population structure of Culex modestus across Europe: does recent appearance in the United Kingdom reveal a tendency for geographical spread? Med Vet Entomol. (2020) 34:86–96. doi: 10.1111/mve.12412
29. Balenghien T, Fouque F, Sabatier P, Bicout DJ. Horse-, bird-, and human-seeking behavior and seasonal abundance of mosquitoes in a west nile virus focus of southern France. J OF Med ENTOMOLOGY. (2006) 43:936–46. doi: 10.1093/jmedent/43.5.936
30. Ponçon N, Balenghien T, Toty C, Baptiste Ferré J, Thomas C, Dervieux A, et al. Effects of local anthropogenic changes on potential malaria vector Anopheles hyrcanus and West Nile virus vector Culex modestus, Camargue, France. Emerg Infect Dis. (2007) 13:1810–5. doi: 10.3201/eid1312.070730
31. Balenghien T, Vazeille M, Reiter P, Schaffner F, Zeller H, Bicout DJ. Evidence of laboratory vector competence of Culex modestus for West Nile virus. J Am Mosq Control Assoc. (2007) 23:233–6. doi: 10.2987/8756-971X(2007)23[233:EOLVCO]2.0.CO;2
32. Fortuna C, Remoli ME, Severini F, Di Luca M, Toma L, Fois F, et al. Evaluation of vector competence for West Nile virus in Italian Stegomyia albopicta (=Aedes albopictus) mosquitoes. Med Veterinary Entomology. (2015) 29:430–3. doi: 10.1111/mve.2015.29.issue-4
33. Ziegler U, Bergmann F, Fischer D, Müller K, Holicki CM, Sadeghi B, et al. Spread of west nile virus and usutu virus in the german bird population, 2019-2020. Microorganisms. (2022) 10:807. doi: 10.3390/microorganisms10040807
34. McLean RG. West nile virus in north american birds. Ornithological Monographs. (2006) 60):44–64. doi: 10.1642/0078-6594(2006)60[44:WNVINA]2.0.CO;2
35. Audsley M, Edmonds J, Liu W, Mokhonov V, Mokhonova E, Melian EB, et al. Virulence determinants between New York 99 and Kunjin strains of West Nile virus. Virology. (2011) 414:63–73. doi: 10.1016/j.virol.2011.03.008
36. Dietrich EA, Langevin SA, Huang CYH, Maharaj PD, Delorey MJ, Bowen RA, et al. West nile virus temperature sensitivity and avian virulence are modulated by NS1-2B polymorphisms. PloS Negl Trop Dis. (2016) 10:e0004938. doi: 10.1371/journal.pntd.0004938
37. Steele KE, Linn MJ, Schoepp RJ, Komar N, Geisbert TW, Manduca RM, et al. Pathology of fatal West Nile virus infections in native and exotic birds during the 1999 outbreak in New York City, New York. Vet Pathol. (2000) 37:208–24. doi: 10.1354/vp.37-3-208
38. Feyer S, Bartenschlager F, Bertram CA, Ziegler U, Fast C, Klopfleisch R, et al. Clinical, pathological and virological aspects of fatal West Nile virus infections in ten free-ranging goshawks (Accipiter gentilis) in Germany. Transbound Emerg Dis. (2021) 68:907–19. doi: 10.1111/tbed.13759
39. Carson PJ, Borchardt SM, Custer B, Prince HE, Dunn-Williams J, Winkelman V, et al. Neuroinvasive disease and west nile virus infection, north dakota, USA, 1999–2008. Emerg Infect Dis. (2012) 18:684–6. doi: 10.3201/eid1804.111313
40. Mostashari F, Bunning ML, Kitsutani PT, Singer DA, Nash D, Cooper MJ, et al. Epidemic West Nile encephalitis, New York, 1999: results of a household-based seroepidemiological survey. Lancet. (2001) 358:261–4. doi: 10.1016/S0140-6736(01)05480-0
41. Murray K, Baraniuk S, Resnick M, Arafat R, Kilborn C, Cain K, et al. Risk factors for encephalitis and death from West Nile virus infection. Epidemiol Infect. (2006) 134:1325–32. doi: 10.1017/S0950268806006339
42. Yeung MW, Shing E, Nelder M, Sander B. Epidemiologic and clinical parameters of West Nile virus infections in humans: a scoping review. BMC Infect Dis. (2017) 17:609. doi: 10.1186/s12879-017-2637-9
43. Gyure KA. West nile virus infections. J Neuropathol Exp Neurol. (2009) 68:1053–60. doi: 10.1097/NEN.0b013e3181b88114
44. Sejvar JJ, Haddad MB, Tierney BC, Campbell GL, Marfin AA, Van Gerpen JA, et al. Neurologic manifestations and outcome of West Nile virus infection. JAMA. (2003) 290:511–5. doi: 10.1001/jama.290.4.511
45. McLean RG, Ubico SR, Bourne D, Komar N. West nile virus in livestock and wildlife. In: Mackenzie JS, Barrett ADT, Deubel V, editors. Japanese encephalitis and west nile viruses. Springer, Berlin, Heidelberg (2002). p. 271–308. doi: 10.1007/978-3-642-59403-8_14
46. Castillo-Olivares J, Wood J. West Nile virus infection of horses. Vet Res. (2004) 35:467–83. doi: 10.1051/vetres:2004022
47. Schwarz ER, Long MT. Comparison of west nile virus disease in humans and horses: exploiting similarities for enhancing syndromic surveillance. Viruses. (2023) 15:1230. doi: 10.3390/v15061230
48. Ostlund EN, Andresen JE, Andresen M. West nile encephalitis. Veterinary Clinics North America: Equine Practice. (2000) 16:427–41. doi: 10.1016/S0749-0739(17)30087-1
49. Read A, Finlaison D, Gu X, Hick P, Moloney B, Wright T, et al. Clinical and epidemiological features of West Nile virus equine encephalitis in New South Wales, Australia, 2011. Aust Veterinary J. (2019) 97:133–43. doi: 10.1111/avj.2019.97.issue-5
50. Reiter P. West Nile virus in Europe: understanding the present to gauge the future. Eurosurveillance. (2010) 15:19508. doi: 10.2807/ese.15.10.19508-en
51. Murgue B, Murri S, Triki H, Deubel V, Zeller HG. West nile in the mediterranean basin: 1950-2000. Ann New York Acad Sci. (2001) 951:117–26. doi: 10.1111/j.1749-6632.2001.tb02690.x
52. Gossner CM, Marrama L, Carson M, Allerberger F, Calistri P, Dilaveris D, et al. West Nile virus surveillance in Europe: moving towards an integrated animal-human-vector approach. Eurosurveillance. (2017) 22. doi: 10.2807/1560-7917.ES.2017.22.18.30526
53. Papa A. Emerging arboviral human diseases in Southern Europe. J Med Virology. (2017) 89:1315–22. doi: 10.1002/jmv.24803
54. Schopf F, Sadeghi B, Bergmann F, Fischer D, Rahner R, Müller K, et al. Circulation of West Nile virus and Usutu virus in birds in Germany, 2021 and 2022. Infect Dis (Lond). (2024) 0:1–22. doi: 10.1080/23744235.2024.2419859
55. Epidemiological update: West Nile virus transmission season in Europe, 2018 (2018). Available online at: https://www.ecdc.europa.eu/en/news-events/epidemiological-update-west-nile-virus-transmission-season-europe-2018 (Accessed June 5, 2023).
56. Bakonyi T, Haussig JM. West Nile virus keeps on moving up in Europe. Euro Surveill. (2020) 25:2001938. doi: 10.2807/1560-7917.ES.2020.25.46.2001938
57. Epidemiological update: West Nile virus transmission season in Europe, 2021 (2022). Available online at: https://www.ecdc.europa.eu/en/news-events/epidemiological-update-west-nile-virus-transmission-season-europe-2021 (Accessed June 5, 2023).
58. Sikkema RS, Schrama M, van den BT, Morren J, Munger E, Krol L, et al. Detection of West Nile virus in a common whitethroat (Curruca communis) and Culex mosquitoes in the Netherlands, 2020. Eurosurveillance. (2020) 25:2001704. doi: 10.2807/1560-7917.ES.2020.25.40.2001704
59. Vlaskamp DR, Thijsen SF, Reimerink J, Hilkens P, Bouvy WH, Bantjes SE, et al. First autochthonous human West Nile virus infections in the Netherlands, July to August 2020. Eurosurveillance. (2020) 25:2001904. doi: 10.2807/1560-7917.ES.2020.25.46.2001904
60. Sikkema RS, Schrama M, Rockx B, Koopmans M. A One Health approach to predict, monitor and prevent arbovirus infections, an extensive case study from the Netherlands - Editorial. One Health. (2023) 17:100635. doi: 10.1016/j.onehlt.2023.100635
61. Koch RT, Erazo D, Folly AJ, Johnson N, Dellicour S, Grubaugh ND, et al. Genomic epidemiology of West Nile virus in Europe. One Health. (2023) 18:100664. doi: 10.1016/j.onehlt.2023.100664
62. Ciota AT, Matacchiero AC, Kilpatrick AM, Kramer LD. The effect of temperature on life history traits of culex mosquitoes. J Med Entomology. (2014) 51:55–62. doi: 10.1603/ME13003
63. Yu D, Madras N, Zhu H. Temperature-driven population abundance model for Culex pipiens and Culex restuans (Diptera: Culicidae). J Theor Biol. (2018) 443:28–38. doi: 10.1016/j.jtbi.2018.01.024
64. Ruybal JE, Kramer LD, Kilpatrick AM. Geographic variation in the response of Culex pipiens life history traits to temperature. Parasit Vectors. (2016) 9:116. doi: 10.1186/s13071-016-1402-z
65. Marini G, Poletti P, Giacobini M, Pugliese A, Merler S, Rosà R. The role of climatic and density dependent factors in shaping mosquito population dynamics: the case of culex pipiens in northwestern Italy. PloS One. (2016) 11:e0154018. doi: 10.1371/journal.pone.0154018
66. Reisen WK, Fang Y, Martinez VM. Effects of temperature on the transmission of west nile virus by culex tarsalis (Diptera: culicidae). J Med Entomology. (2006) 43:309–17. doi: 10.1093/jmedent/43.2.309
67. Dohm DJ, O’Guinn ML, Turell MJ. Effect of environmental temperature on the ability of Culex pipiens (Diptera: Culicidae) to transmit West Nile virus. J Med Entomol. (2002) 39:221–5. doi: 10.1603/0022-2585-39.1.221
68. Kilpatrick AM, Meola MA, Moudy RM, Kramer LD. Temperature, viral genetics, and the transmission of west nile virus by culex pipiens mosquitoes. PLoS Pathog. (2008) 4:e1000092.
69. Tran A, Sudre B, Paz S, Rossi M, Desbrosse A, Chevalier V, et al. Environmental predictors of West Nile fever risk in Europe. Int J Health Geogr. (2014) 13:26. doi: 10.1186/1476-072X-13-26
70. Soverow JE, Wellenius GA, Fisman DN, Mittleman MA. Infectious disease in a warming world: how weather influenced west nile virus in the United States (2001–2005). Environ Health Perspect. (2009) 117:1049–52. doi: 10.1289/ehp.0800487
71. Giesen C, Herrador Z, Fernandez-Martinez B, Figuerola J, Gangoso L, Vazquez A, et al. A systematic review of environmental factors related to WNV circulation in European and Mediterranean countries. One Health. (2023) 16:100478. doi: 10.1016/j.onehlt.2022.100478
72. García-Carrasco JM, Muñoz AR, Olivero J, Segura M, Real R. Predicting the spatio-temporal spread of West Nile virus in Europe. PloS Negl Trop Dis. (2021) 15:e0009022. doi: 10.1371/journal.pntd.0009022
73. Roiz D, Boussès P, Simard F, Paupy C, Fontenille D. Autochthonous chikungunya transmission and extreme climate events in southern France. PloS Negl Trop Diseases. (2015) 9:e0003854. doi: 10.1371/journal.pntd.0003854
74. Platonov AE, Fedorova MV, Karan LS, Shopenskaya TA, Platonova OV, Zhuravlev VI. Epidemiology of West Nile infection in Volgograd, Russia, in relation to climate change and mosquito (Diptera: Culicidae) bionomics. Parasitol Res. (2008) 103:45–53. doi: 10.1007/s00436-008-1050-0
75. Deichmeister JM, Telang A. Abundance of West Nile virus mosquito vectors in relation to climate and landscape variables. J Vector Ecology. (2011) 36:75–85. doi: 10.1111/j.1948-7134.2011.00143.x
76. Shaman J, Day JF, Stieglitz M. Drought-induced amplification and epidemic transmission of west nile virus in southern florida. J Med Entomology. (2005) 42:134–41. doi: 10.1093/jmedent/42.2.134
77. Wang G, Minnis RB, Belant JL, Wax CL. Dry weather induces outbreaks of human West Nile virus infections. BMC Infect Diseases. (2010) 10:38. doi: 10.1186/1471-2334-10-38
78. Lisovski S, Hoye BJ, Conklin JR, Battley PF, Fuller RA, Gosbell KB, et al. Predicting resilience of migratory birds to environmental change. Proc Natl Acad Sci U S A. (2024) 121:e2311146121. doi: 10.1073/pnas.2311146121
79. Marra PP, Francis CM, Mulvihill RS, Moore FR. The influence of climate on the timing and rate of spring bird migration. Oecologia. (2005) 142:307–15. doi: 10.1007/s00442-004-1725-x
80. Cotton PA. Avian migration phenology and global climate change. Proc Natl Acad Sci. (2003) 100:12219–22. doi: 10.1073/pnas.1930548100
81. Vidaña B, Busquets N, Napp S, Pérez-Ramírez E, Jiménez-Clavero MÁ, Johnson N. The role of birds of prey in west nile virus epidemiology. Vaccines (Basel). (2020) 8:550. doi: 10.3390/vaccines8030550
82. Simonin Y. Circulation of west nile virus and usutu virus in europe: overview and challenges. Viruses. (2024) 16:599. doi: 10.3390/v16040599
83. Johnson BJ, Sukhdeo MVK. Drought-induced amplification of local and regional West Nile virus infection rates in New Jersey. J Med Entomol. (2013) 50:195–204. doi: 10.1603/ME12035
84. Magallanes S, Llorente F, Ruiz-López MJ, de la PJM, Ferraguti M, Gutiérrez-López R, et al. Warm winters are associated to more intense West Nile virus circulation in southern Spain. Emerg Microbes Infect. (2024) 13:2348510. doi: 10.1080/22221751.2024.2348510
85. de Freitas Costa E, Streng K, Avelino de Souza Santos M, Counotte MJ. The effect of temperature on the boundary conditions of West Nile virus circulation in Europe. PloS Negl Trop Dis. (2024) 18:e0012162. doi: 10.1371/journal.pntd.0012162
86. Paz S, Malkinson D, Green MS, Tsioni G, Papa A, Danis K, et al. Permissive summer temperatures of the 2010 european west nile fever upsurge. PloS One. (2013) 8:e56398. doi: 10.1371/journal.pone.0056398
87. Lindahl JF, Grace D. The consequences of human actions on risks for infectious diseases: a review. Infect Ecol Epidemiol. (2015) 5:30048. doi: 10.3402/iee.v5.30048
88. Mahon MB, Sack A, Aleuy OA, Barbera C, Brown E, Buelow H, et al. A meta-analysis on global change drivers and the risk of infectious disease. Nature. (2024) 629:830–6. doi: 10.1038/s41586-024-07380-6
89. Kraemer MUG, Reiner RC, Brady OJ, Messina JP, Gilbert M, Pigott DM, et al. Past and future spread of the arbovirus vectors Aedes aEgypti and Aedes albopictus. Nat Microbiol. (2019) 4:854–63. doi: 10.1038/s41564-019-0376-y
90. Keesing F, Belden LK, Daszak P, Dobson A, Harvell CD, Holt RD, et al. Impacts of biodiversity on the emergence and transmission of infectious diseases. Nature. (2010) 468:647–52. doi: 10.1038/nature09575
91. Greger M. The human/animal interface: emergence and resurgence of zoonotic infectious diseases. Crit Rev Microbiol. (2007) 33:243–99. doi: 10.1080/10408410701647594
92. Patz JA, Graczyk TK, Geller N, Vittor AY. Effects of environmental change on emerging parasitic diseases. Int J Parasitol. (2000) 30:1395–405. doi: 10.1016/S0020-7519(00)00141-7
93. Jones BA, Grace D, Kock R, Alonso S, Rushton J, Said MY, et al. Zoonosis emergence linked to agricultural intensification and environmental change. Proc Natl Acad Sci U S A. (2013) 110:8399–404. doi: 10.1073/pnas.1208059110
94. D’Amore C, Grimaldi P, Ascione T, Conti V, Sellitto C, Franci G, et al. West Nile Virus diffusion in temperate regions and climate change. A systematic review. Infez Med. (2023) 31:20–30. doi: 10.53854/liim-3101-4
95. Erazo D, Grant L, Ghisbain G, Marini G, Colón-González FJ, Wint W, et al. Contribution of climate change to the spatial expansion of West Nile virus in Europe. Nat Commun. (2024) 15:1196. doi: 10.1038/s41467-024-45290-3
96. Lu L, Zhang F, Munnink BBO, Munger E, Sikkema RS, Pappa S, et al. West Nile virus spread in Europe: Phylogeographic pattern analysis and key drivers. PloS Pathogens. (2024) 20:e1011880. doi: 10.1371/journal.ppat.1011880
97. Mihailović DT, Petrić D, Petrović T, Hrnjaković-Cvjetković I, Djurdjevic V, Nikolić-Đorić E, et al. Assessment of climate change impact on the malaria vector Anopheles hyrcanus, West Nile disease, and incidence of melanoma in the Vojvodina Province (Serbia) using data from a regional climate model. PloS One. (2020) 15:e0227679. doi: 10.1371/journal.pone.0227679
98. Ewing DA, Purse BV, Cobbold CA, White SM. A novel approach for predicting risk of vector-borne disease establishment in marginal temperate environments under climate change: West Nile virus in the UK. J R Soc Interface. (2021) 18:20210049. doi: 10.1098/rsif.2021.0049
99. Harrigan RJ, Thomassen HA, Buermann W, Smith TB. A continental risk assessment of West Nile virus under climate change. Glob Chang Biol. (2014) 20:2417–25. doi: 10.1111/gcb.2014.20.issue-8
100. Weissenböck H, Kolodziejek J, Url A, Lussy H, Rebel-Bauder B, Nowotny N. Emergence of usutu virus, an african mosquito-borne flavivirus of the Japanese encephalitis virus group, central europe. Emerg Infect Dis. (2002) 8:652–6. doi: 10.3201/eid0807.020094
101. Clé M, Beck C, Salinas S, Lecollinet S, Gutierrez S, Van de Perre P, et al. Usutu virus: A new threat? Epidemiol Infect. (2019) 147:e232. doi: 10.1017/S0950268819001213
102. Ashraf U, Ye J, Ruan X, Wan S, Zhu B, Cao S. Usutu virus: an emerging flavivirus in europe. Viruses. (2015) 7:219–38. doi: 10.3390/v7010219
103. Fros JJ, Miesen P, Vogels CB, Gaibani P, Sambri V, Martina BE, et al. Comparative Usutu and West Nile virus transmission potential by local Culex pipiens mosquitoes in north-western Europe. One Health. (2015) 1:31–6. doi: 10.1016/j.onehlt.2015.08.002
104. Mancini G, Montarsi F, Calzolari M, Capelli G, Dottori M, Ravagnan S, et al. Mosquito species involved in the circulation of West Nile and Usutu viruses in Italy. Vet Ital. (2017) 53:97–110. doi: 10.12834/vetit.114.933.4764.2
105. Vázquez A, Jiménez-Clavero MA, Franco L, Donoso-Mantke O, Sambri V, Niedrig M, et al. Usutu virus – potential risk of human disease in Europe. Eurosurveillance. (2011) 16. doi: 10.2807/ese.16.31.19935-en
106. Soto A, De Coninck L, Devlies AS, Van De Wiele C, Rosales Rosas AL, Wang L, et al. Belgian Culex pipiens pipiens are competent vectors for West Nile virus while Culex modestus are competent vectors for Usutu virus. PloS Negl Trop Dis. (2023) 17:e0011649. doi: 10.1371/journal.pntd.0011649
107. Soto A, Wauters L, Delang L. Is Culex modestus a New Usutu virus vector? Parasit Vectors. (2024) 17:285. doi: 10.1186/s13071-024-06360-z
108. Puggioli A, Bonilauri P, Calzolari M, Lelli D, Carrieri M, Urbanelli S, et al. Does Aedes albopictus (Diptera: Culicidae) play any role in Usutu virus transmission in Northern Italy? Experimental oral infection and field evidences. Acta Tropica. (2017) 172:192–6. doi: 10.1016/j.actatropica.2017.05.006
109. Vázquez A, Ruiz S, Herrero L, Moreno J, Molero F, Magallanes A, et al. West nile and usutu viruses in mosquitoes in Spain, 2008–2009. Am J Trop Med Hygiene. (2011) 85:178–81. doi: 10.4269/ajtmh.2011.11-0042
110. Calzolari M, Bonilauri P, Bellini R, Albieri A, Defilippo F, Maioli G, et al. Evidence of simultaneous circulation of West Nile and Usutu viruses in mosquitoes sampled in Emilia-Romagna region (Italy) in 2009. PloS One. (2010) 5:e14324. doi: 10.1371/journal.pone.0014324
111. Vilibic-Cavlek T, Kaic B, Barbic L, Pem-Novosel I, Slavic-Vrzic V, Lesnikar V, et al. First evidence of simultaneous occurrence of West Nile virus and Usutu virus neuroinvasive disease in humans in Croatia during the 2013 outbreak. Infection. (2014) 42:689–95. doi: 10.1007/s15010-014-0625-1
112. Zelená H, Kleinerová J, Šikutová S, Straková P, Kocourková H, Stebel R, et al. First autochthonous west nile lineage 2 and usutu virus infections in humans, july to october 2018, Czech Republic. Pathogens. (2021) 10:651. doi: 10.3390/pathogens10060651
113. Constant O, Gil P, Barthelemy J, Bolloré K, Foulongne V, Desmetz C, et al. One Health surveillance of West Nile and Usutu viruses: a repeated cross-sectional study exploring seroprevalence and endemicity in Southern France, 2016 to 2020. Euro Surveill. (2022) 27:2200068. doi: 10.2807/1560-7917.ES.2022.27.25.2200068
114. Nikolay B, Diallo M, Boye CSB, Sall AA. Usutu virus in africa. Vector-Borne Zoonotic Diseases. (2011) 11:1417–23. doi: 10.1089/vbz.2011.0631
115. Weissenböck H, Bakonyi T, Rossi G, Mani P, Nowotny N. Usutu virus, Italy, 1996. Emerg Infect Dis. (2013) 19:274–7. doi: 10.3201/eid1902.121191
116. Siljic M, Sehovic R, Jankovic M, Stamenkovic G, Loncar A, Todorovic M, et al. Evolutionary dynamics of Usutu virus: Worldwide dispersal patterns and transmission dynamics in Europe. Front Microbiol. (2023) 14:1145981. doi: 10.3389/fmicb.2023.1145981
117. Engel D, Jöst H, Wink M, Börstler J, Bosch S, Garigliany MM, et al. Reconstruction of the evolutionary history and dispersal of usutu virus, a neglected emerging arbovirus in europe and africa. mBio. (2016) 7:e01938–15. doi: 10.1128/mBio.01938-15
118. Angeloni G, Bertola M, Lazzaro E, Morini M, Masi G, Sinigaglia A, et al. Epidemiology, surveillance and diagnosis of Usutu virus infection in the EU/EEA, 2012 to 2021. Euro Surveill. (2023) 28:2200929. doi: 10.2807/1560-7917.ES.2023.28.33.2200929
119. Becker N, Jöst H, Ziegler U, Eiden M, Höper D, Emmerich P, et al. Epizootic emergence of usutu virus in wild and captive birds in Germany. PLoS ONE. (2012) e32604.
120. Rijks J, Kik M, Slaterus R, Foppen R, Stroo A, IJzer J, et al. Widespread Usutu virus outbreak in birds in the Netherlands, 2016. Euro Surveill. (2016) 21:30391. doi: 10.2807/1560-7917.ES.2016.21.45.30391
121. Garigliany MM, Marlier D, Tenner-Racz K, Eiden M, Cassart D, Gandar F, et al. Detection of Usutu virus in a bullfinch (Pyrrhula pyrrhula) and a great spotted woodpecker (Dendrocopos major) in north-west Europe. Veterinary J. (2014) 199:191–3. doi: 10.1016/j.tvjl.2013.10.017
122. Garigliany M, Linden A, Gilliau G, Levy E, Sarlet M, Franssen M, et al. Usutu virus, Belgium, 2016. Infection Genet Evolution. (2017) 48:116–9. doi: 10.1016/j.meegid.2016.12.023
123. Giglia G, Agliani G, Munnink BBO, Sikkema RS, Mandara MT, Lepri E, et al. Pathology and pathogenesis of eurasian blackbirds (Turdus merula) naturally infected with usutu virus. Viruses. (2021) 13:1481. doi: 10.3390/v13081481
124. Gaibani P, Pierro A, Alicino R, Rossini G, Cavrini F, Landini MP, et al. Detection of usutu-virus-specific igG in blood donors from northern Italy. Vector-Borne Zoonotic Diseases. (2012) 12:431–3. doi: 10.1089/vbz.2011.0813
125. Allering L, Jöst H, Emmerich P, Günther S, Lattwein E, Schmidt M, et al. Detection of Usutu virus infection in a healthy blood donor from south-west Germany, 2012. Eurosurveillance. (2012) 17:20341. doi: 10.2807/ese.17.50.20341-en
126. Pecorari M, Longo G, Gennari W, Grottola A, Sabbatini AM, Tagliazucchi S, et al. First human case of Usutu virus neuroinvasive infection, Italy, August-September 2009. Eurosurveillance. (2009) 14:19446. doi: 10.2807/ese.14.50.19446-en
127. Cavrini F, Gaibani P, Longo G, Pierro AM, Rossini G, Bonilauri P, et al. Usutu virus infection in a patient who underwent orthotropic liver transplantation, Italy, August-September 2009. Eurosurveillance. (2009) 14:19448. doi: 10.2807/ese.14.50.19448-en
128. Cadar D, Simonin Y. Human usutu virus infections in europe: A new risk on horizon? Viruses. (2022) 15:77. doi: 10.3390/v15010077
129. Simonin Y, Sillam O, Carles MJ, Gutierrez S, Gil P, Constant O, et al. Human usutu virus infection with atypical neurologic presentation, montpellier, France, 2016. Emerging Infect Dis J - CDC. (2018) 24:875–8. https://wwwnc.cdc.gov/eid/article/24/5/17-1122_article (Accessed June 6, 2024).
130. Nagy A, Mezei E, Nagy O, Bakonyi T, Csonka N, Kaposi M, et al. Extraordinary increase in West Nile virus cases and first confirmed human Usutu virus infection in Hungary, 2018. Eurosurveillance. (2019) 24:1900038. doi: 10.2807/1560-7917.ES.2019.24.28.1900038
131. Pacenti M, Sinigaglia A, Martello T, Rui MED, Franchin E, Pagni S, et al. Clinical and virological findings in patients with Usutu virus infection, northern Italy, 2018. Eurosurveillance. (2019) 24:1900180. doi: 10.2807/1560-7917.ES.2019.24.47.1900180
132. Savini G, Monaco F, Terregino C, Di Gennaro A, Bano L, Pinoni C, et al. Usutu virus in ITALY: An emergence or a silent infection? Veterinary Microbiology. Veterinary Microbiol. (2011) 151:264–74. doi: 10.1016/j.vetmic.2011.03.036
133. Bażanów B, Jansen van Vuren P, Szymański P, Stygar D, Frącka A, Twardoń J, et al. A survey on west nile and usutu viruses in horses and birds in Poland. Viruses. (2018) 10:87. doi: 10.3390/v10020087
134. Nikolay B. A review of West Nile and Usutu virus co-circulation in Europe: how much do transmission cycles overlap? Trans R Soc Trop Med Hyg. (2015) 109:609–18. doi: 10.1093/trstmh/trv066
135. Nikolay B, Fall G, Boye CSB, Sall AA, Skern T. Validation of a structural comparison of the antigenic characteristics of Usutu virus and West Nile virus envelope proteins. Virus Res. (2014) 189:87–91. doi: 10.1016/j.virusres.2014.05.018
136. Llorente F, García-Irazábal A, Pérez-Ramírez E, Cano-Gómez C, Sarasa M, Vázquez A, et al. Influence of flavivirus co-circulation in serological diagnostics and surveillance: A model of study using West Nile, Usutu and Bagaza viruses. Transboundary Emerging Diseases. (2019) 66:2100–6. doi: 10.1111/tbed.13262
Keywords: arbovirosis, West Nile virus, Usutu, zoonosis, One Health, climate change, Europe
Citation: Laverdeur J, Amory H, Beckers P, Desmecht D, Francis F, Garigliany M-M, Hayette M-P, Linden A and Darcis G (2025) West Nile and Usutu viruses: current spreading and future threats in a warming northern Europe. Front. Virol. 5:1544884. doi: 10.3389/fviro.2025.1544884
Received: 17 December 2024; Accepted: 07 January 2025;
Published: 28 January 2025.
Edited by:
Xiaoyu Zhao, Fudan University, ChinaReviewed by:
Jolanta Morozińska-Gogol, Pomeranian University of Slupsk, PolandCopyright © 2025 Laverdeur, Amory, Beckers, Desmecht, Francis, Garigliany, Hayette, Linden and Darcis. This is an open-access article distributed under the terms of the Creative Commons Attribution License (CC BY). The use, distribution or reproduction in other forums is permitted, provided the original author(s) and the copyright owner(s) are credited and that the original publication in this journal is cited, in accordance with accepted academic practice. No use, distribution or reproduction is permitted which does not comply with these terms.
*Correspondence: Justine Laverdeur, amxhdmVyZGV1ckB1bGllZ2UuYmU=