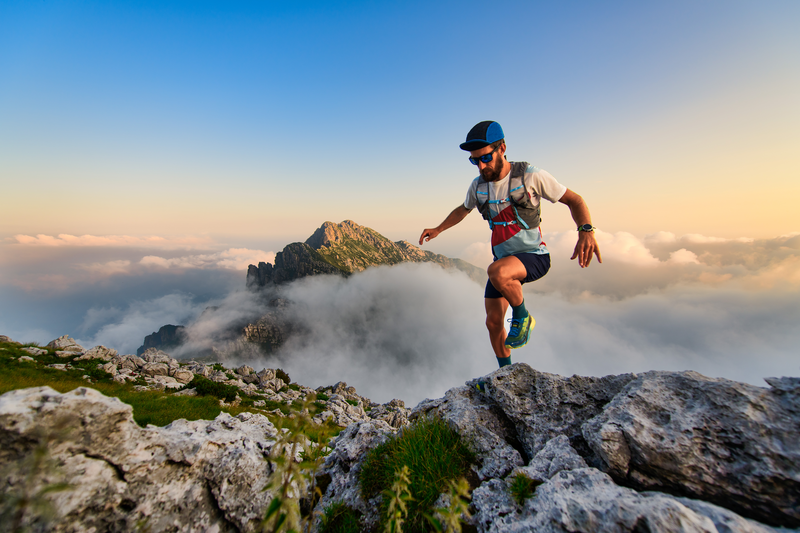
94% of researchers rate our articles as excellent or good
Learn more about the work of our research integrity team to safeguard the quality of each article we publish.
Find out more
ORIGINAL RESEARCH article
Front. Virol. , 08 January 2024
Sec. Viral Disease Investigation
Volume 3 - 2023 | https://doi.org/10.3389/fviro.2023.1343781
This article is part of the Research Topic Enterovirus Surveillance in Europe and Beyond View all 6 articles
Background: Enteroviruses have the potential to cause both high morbidity and mortality especially in children. High season in Norway is between August and November, but this seasonality was interrupted by the COVID-19 pandemic.
Methods: In this study, we describe the enterovirus surveillance in Norway before and during the COVID-19 pandemic including the years from the start of 2016 until the end of 2022. Screening of enterovirus was performed by both laboratory developed methods and FilmArray® ME Panel. Relevant samples were typed, mostly by VP1 sequencing.
Results: Seventy-four percent of all cases occurred in infants under five years of age. A significant reduction in positive cases was observed during the peak years of the COVID-19 pandemic compared to the years before. Pre-pandemic, a wide range of types from all four enterovirus species were detected. During the years with COVID-19 infection control measures, significantly fewer enterovirus types were found along with a substantial reduction in the detection rate.
Conclusion: Enterovirus surveillance discovered a large amount of different types mainly affecting infants. The positivity rate was markedly reduced during the pandemic in 2020-2022 and fewer types occurred.
Enterovirus infection, causing major disease burden especially in children, can present with a wide array of conditions, spanning from febrile illness to neurological disorders such as meningitis, encephalitis, or paralysis, and may also impact the heart and respiratory system (1–3).
Human enteroviruses, belonging to the Picornaviridae family, are grouped into four species: Enterovirus A to D, with polioviruses assigned to group C (4).
Enterovirus B types including echoviruses are known to cause meningitis (1–3). Coxsackievirus A 16 (CVA16) and CVA6 in group A often affects the skin or mucosa resulting in hand-foot-and-mouth disease (HFMD) along with enterovirus A71 (EVA71). Coxsackievirus B (CVB) can infect the myocardium in infants and lead to severe systemic infection and myocarditis also in young adults (1). Similar to other temperate regions, the enterovirus season in Norway typically peaks between late summer and mid-autumn (3). However, during the COVID-19 pandemic, this seasonal distribution was disrupted by the implication of strict hygiene and lockdown measures (5).
Since Norway, along with other European countries, achieved polio-free status in 2002, enterovirus surveillance has been continued. Initially, the surveillance of acute flaccid paralysis (AFP) aimed to ensure Norway´s polio-free status by documenting the absence of poliovirus circulation (6). This was achieved by monitoring all AFP cases in children under fifteen years of age, involving the collection of two stool samples to detect potential poliovirus importation into the country. The last domestic polio case in Norway was reported in 1969, and between 1975 and 1992 only five cases were imported, mainly from Pakistan (6).
As enterovirus D68 (EV-D68) emerged in a large outbreak in 2014, causing respiratory infections and the polio-like illness acute flaccid myelitis (AFM) with varying degrees of paralysis (7), surveillance of this type of enterovirus was recommended (8, 9). In 2014, an additional nasopharyngeal specimen was included in the enterovirus surveillance conducted at the Norwegian Institute of Public Health (NIPH) to test for EV-D68, facilitating its detection in AFM cases (10–13).
In Norway, all AFP cases are examined and tested in hospitals by dedicated physicians, with notification sent to the NIPH, which then reports to the World Health Organization (14). Supplementary surveillance primarily targets cases with central nervous system (CNS) enterovirus infection (14). This surveillance relies on clinical diagnostics and routine testing at local microbiology laboratories. Viral CNS infections caused by enterovirus are notifiable according to the Norwegian Surveillance System for Communicable Diseases (MSIS). Positive samples from patients with confirmed CNS infections are sent to the reference laboratory for polio/enterovirus at NIPH for isolation, typing and further characterization. Additionally, samples from non-notifiable enterovirus infections, such as neonatal sepsis-like illness and HFMD, can be submitted for typing upon the clinical virologist’s request.
Oslo University Hospital (OUH) is a major contributor of EV-positive specimens due to its large catchment area. OUH comprise two large hospital sites, Ullevål and Rikshospitalet, that primarily serve the inhabitants of Oslo, the capital of Norway, with a population of approximately 709,000 people. Additionally, OUH provides specialized healthcare services in the densely populated South – East Health region.
This study aims to investigate the molecular epidemiology of enterovirus cases detected at OUH by comparing a four-years period before with the first years of the COVID-19 pandemic, spanning from 2016 to 2022.
This retrospective study encompassed all samples submitted for enterovirus infection testing at the Department of Microbiology at OUH in both Ullevål and Rikshospitalet locations. Respiratory specimens only tested using the in-house EV-D68 specific PCR were excluded. The study period spanned from January 1st, 2016, to December 31st, 2022. Each suspected case, based on clinical presentation, included at least one of the following specimen types: cerebrospinal fluid (CSF), plasma/serum, feces, vesicle/skin swab, or respiratory specimens.
Extraction of RNA from clinical specimens was performed using the MagNAPure Compact Nucleic Acid isolation Kit I (Roche Diagnostics, Basel, Switzerland), the EZ1 DSP Virus kit (Qiagen, Hilden, Germany), or the MagNAPure 24 Total Nucleic Acid Isolation Kit and Viral RNA Small Volume Kit (Roche Diagnostics, Basel, Switzerland) according to the manufacturer’s instructions.
Detection of enterovirus was done using the instruments Agilent Mx3005P qPCR System, Agilent AriaDx RealTime PCR Instrument or Roche Light Cycler 480 by two different laboratory developed methods for pan-EV PCR with 5’ untranslated region (UTR) as the PCR target. EV-PCR-1 and EV-PCR-2 are the methods used at the two laboratories at OUH; Ullevål and Rikshospitalet respectively. Primers and probes are listed in Table 1 (15–17).
Table 1 Primer and probes designed for detection of enterovirus RNA by the two methods at Oslo University Hospital Ullevål (EV-PCR-1) and Rikshospitalet (EV-PCR-2).
For the FilmArray Meningitis/Encephalitis Panel (FilmArray ME, BioFire Diagnostics®, Salt Lake City, USA), 200 µl of CSF was used following the manufacturer’s instructions. The BioFire® system automatically performs nucleic acid extraction, reverse transcription, and multiplex nested PCR. Although some samples were tested by both FilmArray ME and in-house PCR, the results were only counted once.
Stool specimens from AFP cases or CNS cases sent for typing were inoculated in relevant cell lines like RD, BGM and L20B at the polio/enterovirus reference laboratory at NIPH. All cell culture supernatants were passaged onto fresh cells, and typical cytopathic effects documented. Virus isolates were typed by neutralization assay using RIVM antibody pools, type-specific antibodies or VP1 sequencing as described by Nix et al. (18). VP1 sequencing was employed for typing of all other specimen types.
Chi-square tests were used to assess the statistical significance in differences during the study period. Only p-values < 0.05 were considered significant. All analyses were performed using SPSS version 19 (IBM Statistics, USA).
Throughout the entire study period, a total of 13,938 samples underwent enterovirus testing, identifying 1,236 (8.8%) positive samples. FilmArray ME was performed on 1515 CSF samples, resulting in 62 (4%) positives, of these 48 were detected by this method only. Of the samples tested by FilmArray ME, 348 also underwent in-house PCR testing. There was agreement between FilmArray ME and in-house PCR results in all but two cases, both with Ct values of 38 by in-house PCR although negative in the FilmArray ME.
The highest number of cases and highest positivity rates were observed in 2018 and 2019. However, during the COVID-19 pandemic, from 2020 until 2021, significantly fewer enterovirus cases were detected, with rates of 2.9% and 4.5%, respectively. Detailed information on the distribution of specimen types and positivity rates by year and age is presented in Table 2.
Table 2 Number of tested patients per year and EV cases detected according to gender, age groups and sample types at Oslo University Hospital from 2016 to 2022.
Most enterovirus infections were diagnosed through skin swabs, feces, blood, or CSF, comprising 30.1%, 24.7%, 23.2% and 14.2% respectively. Overall, there was a predominance of males (58%), except for the year 2022, which saw a slight predominance of female cases. The majority of enterovirus patients (74%) fell within the age range from newborns to five years. Notably, most enterovirus infections occurred in children under the age of 12 months (59.3%), with the second-highest incidence observed in children aged one to five (14.7%). The highest number of infections among adults was seen in the age group between 30-39.
Out of the positive samples, 450 (36% of enterovirus positive samples) were successfully typed. Enterovirus typing revealed that a wide range of types were detected throughout the study period, except for 2020 and 2021 when fewer types were found (Figure 1). Analysis of the 450 characterized enteroviruses, showed that EV-A was the most prevalent species overall, peaking in 2019 (Table 3). The total number of typed samples decreased from 221 in 2018/2019 to 71 in 2020/2021, a 68% reduction. The largest decline was a 100% decrease in enterovirus types with a likely dominant respiratory transmission pathway (EV-A71, EV-A76, and EV-D68). Echoviruses decreased by 86%, and a 54% reduction was observed for the Coxsackieviruses. Overall, the most prevalent type was CVA6 with 133 cases, followed by various echoviruses accounting for 122 cases. The prevalence of various enterovirus types during the pre-pandemic period and the years following is visualized in Figure 1. Figure 2 shows the distribution of enterovirus types according to age of the patients. The widest range of types were found in infants under the age of one year, while adults were infected with mostly echoviruses.
Figure 1 The number of enterovirus types in 450 samples during the years from 2016 – 2022 at Oslo University Hospital.
Figure 2 The number of enterovirus types according to age in 450 samples during the years from 2016 – 2022 at Oslo University Hospital.
Table 4 presents the enterovirus species and types categorized by the type of specimen, showing that CVB5 and CVA6 peaked in 2019. The widest range of enterovirus types was identified in feces, where all types except for E15 could be detected. In CSF, the most prevalent types were CVB5, E6 and E30, while vesicle fluid or skin swabs predominantly featured CVA6.
Table 4 Distribution of enterovirus species according to sample types at Oslo University Hospital during the period from 2016 to 2022.
Our study demonstrated a notable reduction in the enterovirus positivity rate during the COVID-19 pandemic period 2020-2021 compared to the average rate in the pre-pandemic years. This is in line with a previous study that reported fewer enterovirus cases, as well as a marked decrease in the detection of respiratory viruses such as influenza, coinciding with the implementation of strict infection control measures (5). Additionally, our study observed a lower diversity of detected enterovirus types during 2020 and 2021, and a total absence of EV types with a likely respiratory transmission pathway in this period.
Sequencing revealed a wide range of enterovirus types in the pre-pandemic period, with the majority belonging to the subtypes Enterovirus A and B, which are common worldwide (1, 19). Notably, Coxsackievirus A and B were frequently detected in infants, representing the highest number of cases overall in our study, consistent with findings in studies from other countries (1, 20). Throughout the entire study period, CVA6 emerged as the most predominant type among the Enterovirus A, a trend also reported by others as a common species A type (1). CVA6 was particularly dominant in skin swabs and vesicle fluid samples, followed by CVA16. Both CVA6 and CVA16 are frequently found in HFMD, especially affecting children aged over 3 months (20). CVA6 remained the dominant type of enterovirus also during COVID-19, when all other types of enteroviruses became rare.
Among the Enterovirus B subtypes, various echoviruses predominated, with E30 being the most prevalent. A notable increase in E30 cases was observed in 2018, coinciding with a surge reported in Europe, affecting both young children and young adults, primarily associated with CNS illness (21). Additionally, CVB5 and E6 were frequently identified in CSF samples in our study. CVB5, E30, E6, and other echoviruses are common causes of viral meningitis (22–24). It is worth noting that the types of enterovirus detected in meningitis cases can vary over time due to local outbreak situations, as these viruses tend to peak with years in-between. Additionally, population susceptibility may also play a role, putting naïve infants with no prior immunity and adults with waning immunity at increased risk of infection.
One limitation of our study is that the number of samples sent for typing depended on the sequencing capacity at NIPH. Furthermore, weak positive samples (Ct values >35) were rarely subjected to typing. In the latter part of the study period, fewer vesicle fluid samples were submitted for typing, leading to a reduced frequency of certain enterovirus types typically associated with HFMD. Sample types such as feces and cerebrospinal fluid were prioritized for typing. In some cases, stool specimens were chosen over CSF from meningitis patients with enterovirus because they contained a larger quantity of virus, increasing the likelihood of successful sequencing. As a result, many enterovirus types found in stool and CSF were associated with meningitis.
Another limitation of this study is the exclusion of EV-D68 positive respiratory samples since they were not part of the enterovirus surveillance. A separate study of EV-D68 circulation pattern at OUH during the years 2012-2022, showed a significant reduction during 2020-2021 seasons (manuscript by Landaas, Frontiers in Virology, Enterovirus Surveillance in Europe and beyond). Finally, we did not conduct whole genome sequencing, which would have provided a more in-depth characterization of the different enterovirus types, potentially revealing different strains before and during the pandemic.
In conclusion, the positivity rate and diversity of enteroviruses were substantially reduced in all age groups during the years with COVID-19 restriction measures. Our study unveiled that enteroviruses caused considerable disease burden in the patients attending our hospital, especially affecting young children and adults in their thirties. Our findings highlight the wide range of enterovirus types affecting infants, and the vast majority of cases occurred in this group. Given enteroviruses’ potential to cause high disease burden and mortality in infants, enterovirus surveillance should be continued in post-polio era to focus on non-polio enteroviruses, including those associated with AFP.
The original contributions presented in the study are included in the article/supplementary material. Further inquiries can be directed to the corresponding author.
The requirement of ethical approval was waived by T. Martinsen, data protection officer, Oslo University Hospital for the studies involving humans because of anonymous aggregated laboratory data only. The studies were conducted in accordance with the local legislation and institutional requirements. The ethics committee/institutional review board also waived the requirement of written informed consent for participation from the participants or the participants’ legal guardians/next of kin because this study only involved aggregated anonymous laboratory data with no personal identification.
SD: Conceptualization, Data curation, Formal analysis, Investigation, Methodology, Project administration, Software, Validation, Writing – original draft, Writing – review & editing. IK: Data curation, Formal analysis, Investigation, Methodology, Software, Validation, Writing – original draft, Writing – review & editing. JØ: Data curation, Formal analysis, Investigation, Validation, Writing – original draft, Writing – review & editing. SN: Data curation, Formal analysis, Methodology, Validation, Writing – original draft, Writing – review & editing. MN: Formal analysis, Methodology, Validation, Writing – original draft, Writing – review & editing. AL: Formal analysis, Methodology, Writing – original draft, Writing – review & editing. MH-P: Formal analysis, Writing – original draft, Writing – review & editing, Data curation, Methodology, Validation. EL: Conceptualization, Data curation, Formal analysis, Project administration, Validation, Writing – original draft, Writing – review & editing.
The author(s) declare that no financial support was received for the research, authorship, and/or publication of this article.
OUH staff for collecting samples. Staff at OUH laboratories for testing the samples. Zeidad Fernandez at OUH Rikshospitalet for technical support during testing, as well as Grete Birkeland Kro and Regine Barlinn for clinical validation. Huda Al-Baldawi for data collection.
The authors declare that the research was conducted in the absence of any commercial or financial relationships that could be construed as a potential conflict of interest.
All claims expressed in this article are solely those of the authors and do not necessarily represent those of their affiliated organizations, or those of the publisher, the editors and the reviewers. Any product that may be evaluated in this article, or claim that may be made by its manufacturer, is not guaranteed or endorsed by the publisher.
1. Coyne CB, Oberste MS, Pallansch MA. Howley P.M. Knipe D.M. Fields virology: emerging viruses. 7th Edition. Philadelphia, USA: Wolters Kluwer Health. (2020) 20200211.
2. Brouwer L, Moreni G, Wolthers KC, Pajkrt D. World-wide prevalence and genotype distribution of enteroviruses. Viruses (2021) 13:434. doi: 10.3390/v13030434
3. Bubba L, Broberg EK, Jasir A, Simmonds P, Harvala H, Enterovirus study collaborators. Circulation of non-polio enteroviruses in 24 EU and EEA countries between 2015 and 2017: a retrospective surveillance study. Lancet Infect Dis (2020) 20(3):350–61. doi: 10.1016/S1473-3099(19)30566-3
4. Simmonds P, Gorbalenya AE, Harvala H, Hovi T, Knowles NJ, Lindberg AM, et al. Recommendations for the nomenclature of enteroviruses and rhinoviruses. Arch Virol (2020) 165(3):793–7. doi: 10.1007/s00705-019-04520-6
5. Knudsen PK, Lind A, Klundby I, Dudman S. The incidence of infectious diseases and viruses other than SARS-CoV-2 amongst hospitalised children in Oslo, Norway during the Covid-19 pandemic 2020-2021. J Clin Virol Plus (2021) 2(1):100060. doi: 10.1016/j.jcvp.2021.100060
6. National plan of action to sustain a poliomyelitis-free status. National polio outbreak preparedness and response plan. Norway. Updated on 19. September 2018. Report 2018. Oslo: Norwegian Institute of Public Health (2018).
7. Greninger AL, Naccache SN, Messacar K, Clayton A, Yu G, Somasekar S, et al. A novel outbreak enterovirus D68 strain associated with acute flaccid myelitis cases in the USA (2012–14): a retrospective cohort study. Lancet Infect Dis (2015) 15:671–82. doi: 10.1016/S1473-3099(15)70093-9
8. Fischer TK, Simmonds P, Harvala H. The importance of enterovirus surveillance in a post-polio world. Lancet Infect Dis (2022) 22(1):e35–40. doi: 10.1016/S1473-3099(20)30852-5
9. Harvala H, Benschop KSM, Berginc N, Midgley S, Wolthers K, Simmonds P, et al. European non-polio enterovirus network: introduction of hospital-based surveillance network to understand the true disease burden of non-polio enterovirus and parechovirus infections in europe. Microorganisms (2021) 9:1827. doi: 10.3390/microorganisms9091827
10. Pfeiffer HC, Bragstad K, Skram MK, Dahl H, Knudsen PK, Chawla MS, et al. Two cases of acute severe flaccid myelitis associated with enterovirus D68 infection in children, Norway, autumn 2014. Euro Surveill (2015) 20(10):21062. doi: 10.2807/1560-7917.ES2015.20.10.21062
11. Knoester M, Helfferich J, Poelman R, Van Leer-Buter C, Brouwer OF, Niesters HGM. Twenty-nine cases of enterovirus-D68-associated acute flaccid myelitis in europe 2016: A case series and epidemiologic overview. Pediatr Infect Dis J (2019) 38(1):16–21. doi: 10.1097/INF.0000000000002188
12. Hixon AM, Frost J, Rudy MJ, Messacar K, Clarke P, Tyler KL. Understanding enterovirus D68-induced neurologic disease: A basic science review. Viruses (2019) 11(9):821. doi: 10.3390/v11090821
13. Hixon AM, Frost J, Rudy MJ, Messacar K, Clarke P, Tyler KL. Recommendations for enterovirus diagnostics and characterisation within and beyond Europe. J Clin Virol (2018) 101:11–7. doi: 10.1016/j.jcv.2018.01.008
14. World Health Organization Enterovirus Surveillance Guidelines. Guidelines for enterovirus surveillance in support of the polio eradication initiative. Available at: http://www.euro.who.int/:data/assets/pdf_file/0020/272810/EnterovirusSurveillanceGuidelines.
15. Verstrepen WA, Kuhn S, Kockx MM, Van De Vyvere ME, Mertens AH. Rapid detection of enterovirus RNA in cerebrospinal fluid specimens with a novel single-tube real-time reverse transcription-PCR assay. J Clin Microbiol (2001) 39(11):4093–6. doi: 10.1128/JCM.39.11.4093-4096.2001
16. Rotbart HA. Enzymatic RNA amplification of the enteroviruses. J Clin Microbiol (1990) 28(3):438–42. doi: 10.1128/jcm.28.3.438-442.1990
17. Nielsen ACY, Böttiger B, Midgley SE, Nielsen LP. A novel enterovirus and parechovirus multiplex one-step real-time PCR-validation and clinical experience. J Virological Methods (2013) 193(2):359–63. doi: 10.1016/j.jviromet.2013.06.038
18. Nix WA, Oberste MS, Pallansch MA. Sensitive, seminested PCR amplification of VP1 sequences for direct identification of all enterovirus serotypes from original clinical specimens. J Clin Microbiol (2006) 44(8):2698–704. doi: 10.1128/JCM.00542-06
19. Brown DM, Zhang Y, Scheuermann RH. Epidemiology and sequence-based evolutionary analysis of circulating non-polio enteroviruses. Microorganisms (2020) 8:1856. doi: 10.3390/microorganisms8121856
20. Yang X, Duan L, Zhan W, Tang Y, Liang L, Xie J, et al. Enterovirus B types cause severe infection in infants aged 0–3 months. Virol J (2023) 20(5). doi: 10.1186/s12985-023-01965-9
21. Benschop KSM, Broberg EK, Hodcroft E, Schmitz D, Albert J, Baicus A, et al. Molecular epidemiology and evolutionary trajectory of emerging echovirus 30, europe. Emerg Infect Dis (2021) 27(6):1616–26. doi: 10.3201/eid2706.203096
22. Alhazmi A, Nekoua MP, Mercier A, Vergez I, Sane F, Alidjinou EK, et al. Combating coxsackievirus B infections. Rev Med Virol (2023) 33(1):e2406. doi: 10.1002/rmv.2406
23. Jmii H, Fisson S, Aouni M, Jaidane H. Type B coxsackieviruses and central nervous system disorders: critical review of reported associations. Rev Med Virol (2021) 31(4):e2191. doi: 10.1002/rmv.2191
Keywords: enterovirus, surveillance, coxsackievirus, poliovirus, meningitis
Citation: Dudman S, Klundby I, Øverbø J, Numanovic S, Nilsen M, Lind A, Holberg-Petersen M and Landaas ET (2024) Trends in the enterovirus surveillance in Oslo, Norway before and during the COVID-19 pandemic. Front. Virol. 3:1343781. doi: 10.3389/fviro.2023.1343781
Received: 24 November 2023; Accepted: 20 December 2023;
Published: 08 January 2024.
Edited by:
Thea Kølsen Fischer, Nordsjællands Hospital, DenmarkReviewed by:
Hubert G. Niesters, University Medical Center Groningen, NetherlandsCopyright © 2024 Dudman, Klundby, Øverbø, Numanovic, Nilsen, Lind, Holberg-Petersen and Landaas. This is an open-access article distributed under the terms of the Creative Commons Attribution License (CC BY). The use, distribution or reproduction in other forums is permitted, provided the original author(s) and the copyright owner(s) are credited and that the original publication in this journal is cited, in accordance with accepted academic practice. No use, distribution or reproduction is permitted which does not comply with these terms.
*Correspondence: Susanne Dudman, c3VzYW5ubWdAbWVkaXNpbi51aW8ubm8=
Disclaimer: All claims expressed in this article are solely those of the authors and do not necessarily represent those of their affiliated organizations, or those of the publisher, the editors and the reviewers. Any product that may be evaluated in this article or claim that may be made by its manufacturer is not guaranteed or endorsed by the publisher.
Research integrity at Frontiers
Learn more about the work of our research integrity team to safeguard the quality of each article we publish.