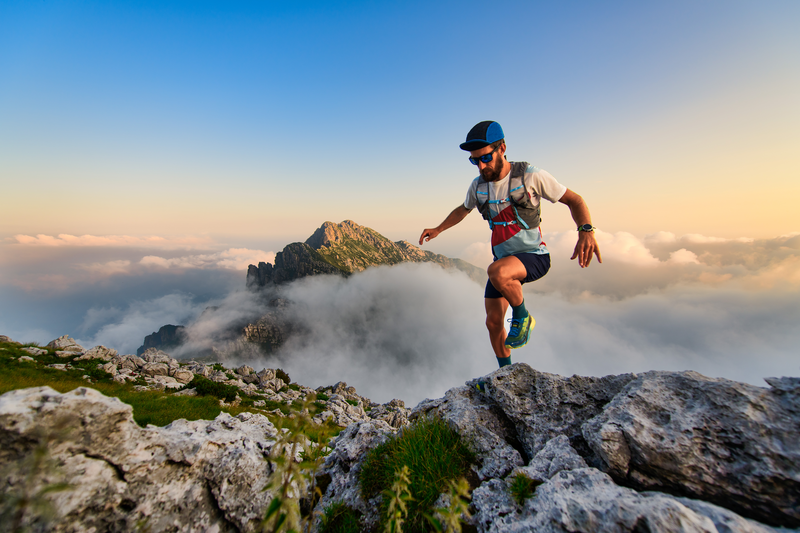
94% of researchers rate our articles as excellent or good
Learn more about the work of our research integrity team to safeguard the quality of each article we publish.
Find out more
BRIEF RESEARCH REPORT article
Front. Virol. , 02 August 2022
Sec. Emerging and Reemerging Viruses
Volume 2 - 2022 | https://doi.org/10.3389/fviro.2022.929715
This article is part of the Research Topic Emerging and Re-emerging Viral Diseases View all 37 articles
Hendra virus and Nipah virus are considered to be emerging viruses and cause severe zoonotic diseases, which occur in humans who have had close contact with horses and pigs in Australia and Asia, respectively. Both viruses belong to the genus Henipavirus. Although there are large populations of horses and pigs in northern Nigeria, no previous studies in this region have investigated henipavirus sero-surveillance in horses and pigs using the gold standard test, the serum neutralization test (SNT). A total of 536 apparently healthy horses and 508 apparently healthy pigs were sampled in northern Nigeria in 2018. Serum samples were tested for Hendra virus and Nipah virus-specific antibodies using either the Henipavirus Luminex binding assays for horses or the Hendra virus Competitive ELISA and Nipah virus Indirect ELISA for pigs as initial screening tests, followed by the confirmatory Hendra and Nipah virus SNT for both species, according to accredited protocols at the Australian Centre for Disease Preparedness. Although some horse and pig samples crossed-reacted or reacted weakly in the screening test, confirmatory SNT for all of them proved negative. This study reveals the absence of Hendra and Nipah antibodies in horses and pigs in northern Nigeria, which is consistent with the absence of observable disease in the field. However, the continuous inter and intra-trans-boundary animal movement and trade in Nigeria calls for the continuous evaluation of the henipavirus status of susceptible animals to safeguard both animal and human health.
Viral diseases of humans and horses are emerging and reemerging, including diseases caused by henipaviruses, which emerged from wildlife hosts in the late 20th century (1). The Henipavirus genus comprises the zoonotic and highly lethal viruses, Hendra virus (HeV) and Nipah virus (NiV), within the family Paramyxoviridae (2). Cedar virus is also in this genus but is not known to cause disease. HeV and NiV have similar reservoir host preferences but different geographic ranges (3).
HeV was first isolated in 1994 from a disease outbreak involving 20 horses and two humans in the Brisbane suburb of Hendra, Australia (4). Since then, it is known to have caused sporadic and relatively small outbreaks with high mortality associated with equine and human infections (5). HeV infection is a serious emerging zoonotic disease, and human infection is acquired through contact with acutely infected horses (5, 6). A severe outbreak of febrile encephalitis in humans in Malaysia in 1998 led to the discovery of NiV, which was attributable to a concurrent disease outbreak in pigs (7). It is assumed that pig trading contributed to the spread of the outbreak between farms, including into Singapore (7, 8). A total of 265 human encephalitis cases with approximately 105 fatalities, all involving those associated with pig farming and slaughtering activities, occurred during the initial outbreak (9). This led to the culling of approximately one million pigs to control the outbreak (10).
HeV can experimentally infect cats, guinea pigs, and pigs (11). In the field, HeV infects horses and causes disease, and it has been identified in asymptomatic dogs on two occasions (12). HeV has been isolated from the uterine fluid and fetal tissues of a pregnant fruit bat, also known as a flying fox belonging to the genus Pteropus, implicating this species as a reservoir host (13). Humans become infected after close contact with HeV-infected horses. Similarly, humans became infected after close contact with NiV-infected pigs in the only Malaysian outbreak (7). Other species capable of becoming naturally infected with NiV include cats, horses, and dogs (7, 14). The presence of NiV neutralizing antibodies and the isolation of the virus from two species of fruit bats (Pteropus vampyrus and P. hypomelanus) indicates the reservoir host role of these bats in the transmission of NiV infection (7). HeV and NiV outbreaks are found in accordance with the geographical distribution of the natural reservoir species. HeV is found in Australia and NiV is found in Southeast Asia, with more recent outbreaks of NiV occurring in Kerala, India (15, 16).
Clinical signs associated with henipavirus infections in pigs can manifest as neurological disease syndromes such as trembling, twitches, muscle spasms, and uncoordinated gait, or as a respiratory syndrome with manifestations (8).
In horses, infections are characterized predominantly by respiratory syndrome with increased heart rate and fever, as well as sudden death. Neurological manifestations have also been reported (5). In Nigeria’s horse and pig populations, these clinical signs may occur covertly, but since the country does not have a diagnostic capacity for HeV and NiV, infections may go unnoticed or misdiagnosed.
Animal husbandry practices in Nigeria allow close contact between bat reservoirs and animals susceptible to henipaviruses (17). The vast majority of animal husbandry practices in Nigeria are extensive systems. Animals forage in the wild for food, encroaching on the natural habitat of bats and potentially putting these foraging animals at a higher risk of exposure to bat-associated pathogens. The proximity of humans to domestic animal viral amplification hosts will play a significant role in the dynamics of an enzootic virus outbreak.
Human infection with HeV and NiV can occur via exposure to infectious urine, saliva, or nasopharyngeal fluid from infected horses and pigs (5). This study investigated the presence of henipavirus antibodies in horses and pigs in seven states in Nigeria and could provide useful insight into the epidemiology of the disease in Nigeria and the sub-Saharan African region in general. As many clinical cases of human HeV and NiV infection have occurred via close contact with infected horses and pigs and/or their tissues, the results of this study will inform the henipavirus infection risk of sub-Saharan African animal handlers and those involved with animal necropsies (7, 18–21). In this study, sero-surveillance of henipaviruses was conducted in horses and pigs in seven states of Nigeria using screening tests, followed by confirmatory serum neutralization tests (SNT), to determine the health status.
Using convenience sampling, a cross-sectional study was carried out on horses. Verbal consent was obtained from the horse owners. Horses (all adults) were sampled from stables, including horse racing stables in Kaduna (Kaduna State), Suleja (Niger State), Gombe (Gombe State), Keffi (Nasarawa State), and Abuja (Federal Capital Territory; FCT). In total, 536 apparently healthy horses were sampled between January and June 2018. Table 1 contains the locations and numbers of animals sampled for this study. Figure 1 shows the sampling locations. Blood (5 ml) was collected via jugular venipuncture from restrained horses and transported to the laboratory where sera were separated by centrifugation at 5,000g for 10 min. The harvested sera were siphoned using a sterile Pasteur pipette and transferred into 2 ml cryovials where they were kept at −20°C until transported to the Australian Centre for Disease Preparedness (ACDP) for testing. Sera from horses were screened using NiV and HeV Luminex binding assays, which use soluble G (glycoprotein) as the binding antigen (22). All reactors were subsequently tested by the NiV and/or HeV SNTs.
Figure 1 Map of Nigeria showing the states (pink) (Federal Capital Territory, Niger, Nasarawa, Kaduna, Gombe, Plateau and Benue) and locations where horses and pigs were sampled.
Market-weight adult pigs were sampled at slaughterhouses prior to slaughter. Using convenience sampling, the abattoirs in Makurdi (Benue State) and Jos (Plateau State) were visited twice weekly between the months of March to June 2018. In total, 508 pigs were sampled (Table 1; Figure 1). Blood samples were collected from the cranial vena cava of the pigs presented for slaughter. After transportation to the laboratory, the blood was centrifuged at 5000g for 10 min for separation of serum. Harvested sera were kept at -20°C until transported to ACDP for testing. Sera from pigs were screened using NiV iELISA and HeV bELISA (23, 24). The NiV iELISA used a whole virus NiV antigen, whereas the HeV bELISA used a soluble G antigen fromHeV and a monoclonal antibody (mAb 1.2). All reactors in either ELISAs were subsequently tested by the NiV and/or HeV SNTs.
The Henipavirus Luminex binding assays have been described previously (22). For example, HeV and NiV soluble G proteins were first coupled to individual microsphere sets (25). Then, a predetermined number of coupled magnetic beads (Fisher Biotech Pty Ltd, Australia) were added to each well of a 96-well Nunc™ Microwell™ microplate (Thermo Fisher Scientific), covered in foil and placed on a plate shaker for 30 min. The plate was washed with 3× Phosphate Buffered Saline with Tween® 20 (PBST) using a magnetic binding plate so the beads did not disappear (bind to the magnetic plate). The test sera were diluted 1:100 in 2% skim milk and 100 µl added to each well. The plate was covered in foil and placed on the plate shaker for 30 min. Following incubation, the plate was washed with 3× PBST (as above), and 100 µl per well of biotinylated Protein G (Pierce, Rockford, USA) and biotinylated Protein A (Pierce, Rockford, USA) was added to each well. The plate was covered in foil and placed on the plate shaker for 30 min. Next, 100 µl of streptavidin-phycoerythrin (Qiagen, Pty Ltd, Australia) was added to each well. The plate was read using a Bio-Plex Protein Array System integrated with Bio-Plex Manager Software (Bio Rad Laboratories, Inc., CA, USA). Results were recorded as median fluorescence intensity (MFI) and transformed as a percentage relative to the MFI for the positive control to yield a percentage positive (%P) value. Results greater than 5%PI and greater than 1,500 MFI were considered positive. Results of less than 5%PI and less than 1,500 MFI were negative. Results that were either greater than 5%PI or greater than 1,500 MFI in duplicate wells were considered indeterminate. Indeterminate and positive samples were then tested in the SNT.
A HeV competitive blocking ELISA as described previously (23) was used to assess the sera for the presence of antibody against HeV. Briefly, Nunc™ Maxisorp™ 96 well ELISA plates (Thermo Fisher Scientific) were coated with 50 µl of 4.4 ng HeV-soluble G tetramer antigen (26) diluted in PBS. Plates were incubated at 37°C on a plate shaker for 1 h. The plates were blocked with 50 µl of casein blocking buffer (Sigma Aldrich) diluted 1/10 in ddH20 (BB). Following 30 min on a plate shaker at 37°C, the plates were washed 3× PBST. The test sera were diluted 1/5 in BB and 50 µl added to each well. The plates were incubated at 37°C on a plate shaker for 1 h. Then, mAb 1.2 (27) diluted 1/500 in BB was added. Plates were incubated at 37°C for 30 min on a plate shaker. Next, the plates were washed 3× PBST, and 50 µl of goat anti-mouse IgG HRP conjugate (Jackson ImmunoResearch Laboratories, Inc.) diluted ½,000 in BB was added to all wells. Plates were again incubated at 37°C for 30 min on a plate shaker. The plates were developed with 50 µL of 3,3´5,5´-tetramethylbenzidine (TMB) substrate (Sigma Aldrich) added to each well. After 8–10 min, the plates were stopped by adding 50 µl per well of 1 M sulfuric acid (Ajax Finechem). Plates were read at 450 nm using a plate reader and the results were calculated as percentage inhibition of the mAb wells. A cut off value of ≥33% inhibition was used as a positive result.
An indirect ELISA was used to assess for antibodies against NiV (23). Firstly, cell lysates of NiV-infected cells (Ag) and Vero mock uninfected cells (Mock) were prepared using detergent treatment and irradiation, diluted in PBS, and used to coat NUNC Maxisorp 96 well ELISA plates (Thermo Fisher Scientific) using 50 µl per well in rotating columns of two. The plates were incubated at 37°C for 1 h on a plate shaker. The sera were inactivated using a 1:5 dilution in PBSA containing 0.5% Tween 20 and 0.5% Triton X-100 solution and heat-inactivated at 56°C for 30 min. Then 25 µl of Vero mock diluted 1:100 in PBS was added to 25 µl of the diluted heat inactivated sera. This was incubated at room temperature for 30 min. Next, 450 µl of blocking solution (5% v/v normal chicken sera and 5% w/v skim milk in the required volume of PBS) was added to each tube of pre-treated sera (final dilution of 1:100) and incubated for 30 min at room temperature. The ELISA plates were washed 4× PBST and 100 µl of blocking solution was added to each well. The plates were incubated at 37°C for 30 min on a plate shaker. The plates were washed 4× PBST and 100 µl of the pre-treated sera was added to each well. The plates were incubated at 37°C for 1 h without a plate shaker (stationary). The ELISA plates were washed 4× PBST and protein A/G conjugate diluted in PBST and 1% skim milk powder was added 100 µl/per well except the blank wells. The plates were again incubated at 37°C for 1 h without shaking. Following incubation, the plates were washed 4× PBST and 50 µl of TMB substrate was added to each well. The color development was stopped after 10 min by adding 50 µl of 1 M sulfuric acid. The plates were read at 450 nm using a plate reader. The optical density (OD) results were used to calculate an OD ratio which equaled the average OD sample against NiV antigen divided by the average OD sample against Vero mock antigen. Results are shown in Table 2. Samples with an OD value (NiV antigen) <0.2 were negative. Samples with an OD value (NiV antigen) between 0.2 and 0.5 were weak reactors except when the OD ratio was less than 2.0, in which case they were non-specific reactors. An OD value (NiV antigen) >0.5 and an OD ratio >2.0 were positive. All reactors and positives were then tested in the NiV SNT.
Table 2 Serology results for horses in the screening (HeV sG Luminex and NiV sG Luminex) and confirmatory (HeV SNT and NiV SNT) assays.
The SNTs were performed as previously described (23). These tests are conducted at BSL4 at ACDP. Serial doubling dilutions of sera from 1/2 to 1/4,096, were carried out in singlicate in a final volume of 50 μl/well in 96 well plates. To this, 50 μl of 100 TCID50 units of virus (HeV or NiV) was added and incubated for 30 min at 37°C. Following incubation, 100 μl of Vero cells at 2 × 105 cells/ml were added to each well on the 96-well plate, and the plate was incubated for 3 days at 37°C, 5% CO2 in a humidified incubator. Serum antibody titers are determined as the highest dilution at which there is complete neutralization of the virus and the absence of a cytopathic effect (CPE).
The results for 536 horses are shown in Table 3. Antibodies against G protein showed a reaction rate of 1.86% (10/536) for HeV and 0.56% (3/536) for NiV in the Luminex binding assay for antibodies against G protein from horse samples. All indeterminates and positives were negative when subjected to HeV and NiV SNT. Specific details of the individual horses, which were positive or indeterminate in the screening assays can be found in Table S2.
Table 3 Serology results for pigs in the screening (HeV bELISA and NiV iELISA) and confirmatory (HeV SNT and NiV SNT) assays.
The results for 508 pigs are shown in Table 3. Specific details of the individual pigs, which were positive, non-specific or weak reactors, can be found in Table S3. One pig from Markudi in Benue State tested positive in the HeV bELISA (percentage positive 0.2%; 1/508) but tested negative using the HeV SNT and was thus considered HeV antibody negative.
In the NiV iELISA, there were no positives but two were weak reactors (0.39%; 1/508) and 11 were non-specific reactors (2.17%; 11/508). Further investigation of these samples gave negative results when they were subjected to the NiV SNT.
This was a large-scale surveillance study targeting seven states in Nigeria, with 1,044 animals sampled in total. With very low reactivity in the screening assays and no positive test results in the gold standard tests, the HeV and NiV SNTs, this study showed that there were no antibodies specific to HeV or NiV in the horse and pig sera collected from northern Nigeria (19). Olufemi et al. (17), reported 15.5% and 20.0% prevalence of henipavirus antibodies in the sera of horses and pigs from Zaria, Nigeria, using the HeV sG iELISA method. However, this study did not verify the performance characteristics of this screening assay, nor did they follow up with the confirmatory SNT, thereby shedding doubt on the reported seroprevalence. The Hendra bELISA used in the current study has diagnostic sensitivity (DSe) and diagnostic specificity (DSp) values of 100% (95% CI 95.3–100.0) and 99.5% (95% CI 98.8–99.8) (24).
A similar study conducted in Uganda between 2015 and 2016 reported that 2.1% of apparently healthy pigs sampled at a slaughterhouse were sero-reactive in at least one screening assay (HeV G and/or NiV G indirect ELISAs). However, none of the sera-neutralized viruses in the plaque reduction neutralization tests with either HeV or NiV, supporting the finding that neither HeV nor NiV was present, but suggesting the presence of other henipa-like viruses (9). The current Nigerian study has similar findings, with 2.8% of pigs being sero-reactive in at least one screening assay and none testing positive in the neutralization test.
Higher levels of sero-reactivity have been found in studies with bats. Using a Luminex multiplexed microsphere assay, Hayman et al. found that 22.0% and 39.0% of Ghanian fruit bats (Epomophorus gambianus and E. helvum) were seropositive for NiV and HeV, respectively (28).
In this study, the negative results from the confirmatory and gold standard HeV SNT and NiV SNT showed the absence of a prior HeV and/or NiV infection in the sampled horses and pigs from Nigeria using validated diagnostic tests. Caution should be exercised when using unvalidated screening tests, particularly in new populations. These screening tests give non-specific cross-reactions resolvable with the use of HeV and NiV SNTs at Laboratory Bio-safety-Level 4 Containment (PC4) (22).
Further investigations, such as Western blots using the henipavirus antigens, may help clarify the ELISA and Luminex results in the future. Atherstone et al. used Western blots as a confirmatory test after screening pig serum in ELISAs, and while some samples were positive in both screening and confirmatory tests, none of the sera-neutralized HeV or NiV in plaque reduction neutralization tests (9). This most likely supports the presence of antibodies against a closely related virus, as neutralizing antibodies should be present if exposed to a homologous virus.
The low seroprevalence was expected, especially as there has been no report of disease in either pigs or horses in Nigeria or in Africa. However, with bats, which might be carrying undescribed henipaviruses in parts of Africa, it is pertinent to monitor this situation, and we would recommend under-camp and under-roost surveillance. The risk to humans remains very low as we would expect to see disease in pigs and/or horses before we see disease in humans as these animals act as amplifying hosts.
Sero-surveillance is a convenient way of monitoring the health status of susceptible host species. The negative serology results for HeV and NiV SNTs in horse and pig sera sampled from seven states in Nigeria in 2018 showed the absence of HeV and NiV in Nigeria then. However, unrestricted transboundary movements of susceptible hosts highlight the need for continuing sero-surveillance of these and other viruses to monitor the risk of henipavirus disease in Nigeria and to safeguard both animal and human health.
The original contributions presented in the study are included in the article/supplementary material. Further inquiries can be directed to the corresponding author.
The animal study was reviewed and approved by the University of Abuja Ethics Committee on Animal Use; Reference number UAECAU/2018/04. Written informed consent was obtained from the owners for the participation of their animals in this study.
AMA conceived the idea for this project. AMA, AAA, YJ, SI, AT, SA, RE, GE, and MA organized the planning, logistics and collection of samples, and submission of samples to the reference laboratory. LM conducted the laboratory work. AMA, LM, and KH wrote, reviewed, and edited the manuscript before submission. All authors listed have made a substantial, direct, and intellectual contribution to the work and approved it for publication.
The authors wish to thank all the various pig and horse stakeholders for their cooperation during the sampling.
The authors declare that the research was conducted in the absence of any commercial or financial relationships that could be construed as a potential conflict of interest.
All claims expressed in this article are solely those of the authors and do not necessarily represent those of their affiliated organizations, or those of the publisher, the editors and the reviewers. Any product that may be evaluated in this article, or claim that may be made by its manufacturer, is not guaranteed or endorsed by the publisher.
The Supplementary Material for this article can be found online at: https://www.frontiersin.org/articles/10.3389/fviro.2022.929715/full#supplementary-material
1. Clayton BA. Nipah virus: transmission of a zoonotic paramyxovirus. Curr Opin Virol (2017) 22:97–104. doi: 10.1016/j.coviro.2016.12.003
3. Fischer K, Diederich S, Smith G, Reiche S, Pinho Dos Reis V, Stroh E, et al. Indirect ELISA based on hendra and nipah virus proteins for the detection of henipavirus specific antibodies in pigs. PloS One (2018) 13(4):e0194385. doi: 10.1371/journal.pone.0194385
4. Murray K, Selleck P, Hooper P, Hyatt A, Gould A, Gleeson L, et al. A morbillivirus that caused fatal disease in horses and humans. Science (1995) 268(5207):94–7. doi: 10.1126/science.7701348
5. Tulsiani SM, Graham GC, Moore PR, Jansen CC, Van Den Hurk AF, Moore FAJ, et al. Emerging tropical diseases in australia. part 5. hendra virus. Ann Trop Med Para (2011) 105(1):1–11. doi: 10.1179/136485911X12899838413547
6. Mahalingam S, Herrero LJ, Playford EG, Spann K, Herring B, Rolph MS, et al. Hendra virus: an emerging paramyxovirus in Australia. Lancet Infect Dis (2012) 12(10):799–807. doi: 10.1016/S1473-3099(12)70158-5
7. Chua KB, Bellini WJ, Rota PA, Harcourt BH, Tamin A, Lam SK, et al. Nipah virus: A recently emergent deadly paramyxovirus. Science (2000) 288(5470):432–1435. doi: 10.1126/science.288.5470.1432
8. Mohd Nor MN, Gan CH, Ong BL. Nipah virus infection of pigs in peninsular Malaysia. Rev Sci Tech (2000) 19(1):160–5. doi: 10.20506/rst.19.1.1202
9. Atherstone C, Diederich S, Weingartl HM, Fischer K, Balkema-Buschmann A, Grace D, et al. Evidence of exposure to henipaviruses in domestic pigs in Uganda. Transbound Emerg Dis (2019) 66:921–8. doi: 10.1111/tbed.13105
10. Enserink M. New virus fingered in Malaysian epidemic. Science (1999) 284(5413):407–10. doi: 10.1126/science.284.5413.407
11. Li M, Embury-Hyatt C, Weingartl HM. Experimental inoculation study indicates swine as a potential host for hendra virus. Vet Res (2010) 41:33. doi: 10.1051/vetres/2010005
12. Kirkland PD, Gabor M, Poe I, Neale K, Chaffey K, Finlaison DS, et al. Hendra virus infection in dog, Australia, 2013. Emerg Infect Dis (2015) 21(12):2182–5. doi: 10.3201/eid2112.151324
13. Halpin K, Young PL, Field HE, Mackenzie JS. Isolation of hendra virus from pteropid bats: a natural reservoir of hendra virus. J Gen Virol (2000) 81(8):1927–32. doi: 10.1099/0022-1317-81-8-1927
14. Ching PKG, de Los Reyes VC, Sucaldito MN, Tayag E, Columna-Vingno AB, Malbas FF Jr, et al. Outbreak of henipavirus infection, Philippines, 2014. Emerg Infect Dis (2015) 21(2):328–31. doi: 10.3201/ejd2102.141433
15. Weingartl HM. Hendra and nipah viruses: Pathogenesis, animal models and recent breakthroughs in vaccination. Vacc Dev Ther (2015) 5:59–74. doi: 10.2147/VDT.S86482
16. Yadav PD, Shete AM, Kumar GA, Sarkale P, Sahay RR, Radhakrishnan C, et al. Nipah virus sequences from humans and bats during nipah outbreak, kerala, India, 2018. Emerg Infect Dis (2019) 25(5):1003–6. doi: 10.3201/eid2505.181076
17. Olufemi OT, Umoh JU, Azikwi AA, Olufemi YO. Serological evidence of henipavirus among horses and pigs in zaria and environs in kaduna state, Nigeria. Adv Biol (2015) 2015:1–7. doi: 10.1155/2015/632158
18. Hooper P, Zaki S, Daniels P, Middleton D. Comparative pathology of the diseases caused by hendra and nipah viruses. Microbes Infect (2001) 3(4):315–22. doi: 10.1016/s1286-4579(01)01385-5
19. World Organisation for Animal Health (OIE). Chapter 3.1.14. nipah and hendra virus diseases, in: Manual of diagnostic tests and vaccines for terrestrial animals (2018). Paris, France: OIE. Available at: https://www.oie.int/fileadmin/Home/eng/Health_standards/tahm/3.01.14_NIPAH_HENDRA.pdf (Accessed March 15, 2022).
20. Field H, Young P, Yob JM, Mills J, Hall L, Mackenzie J. The natural history of hendra and nipah viruses. Microbes Infect (2001) 3(4):307–14. doi: 10.1016/s1286-4579(01)01384-3
21. Islam MS, Sazzad HM, Satter SM, Sultana S, Hossain MJ, Hasan M, et al. Nipah virus transmission from bats to humans associated with drinking traditional liquor made from date palm sap, Bangladesh, 2011–2014. Emerg Infect Dis (2016) 22(4):664–70. doi: 10.3201/eid2204.151747
22. McNabb L, Barr J, Crameri G, Juzva S, Riddell S, Colling A, et al. Henipavirus microsphere immuno-assays for detection of antibodies against hendra virus. J Virol Methods (2014) 200:22–8. doi: 10.1016/j.jviromet.2014.01.010
23. Daniels P, Ksiazek T, Eaton BT. Laboratory diagnosis of nipah and hendra virus infections. Microbes Infect (2001) 3:289–95. doi: 10.1016/S1286-4579(01)01382-X
24. Di Rubbo A, McNabb L, Klein R, White JR, Colling A, Dimitrov DS, et al. Optimization and diagnostic evaluation of monoclonal antibody-based blocking ELISA formats for detection of neutralizing antibodies to hendra virus in mammalian sera. J Virol Methods (2019) 274:113731. doi: 10.1016/j.jviromet.2019.113731
25. Bossart KN, McEachern JA, Hickey AC, Choudhry V, Dimitrov DS, Eaton BT, et al. Neutralization assays for differential henipavirus serology using bio-plex protein array systems. J Virol Methods (2007) 142:29–40. doi: 10.1016/j.jviromet.2007.01.003
26. Bossart KN, Crameri G, Dimitrov AS, Mungall BA, Feng YR, Patch JR, et al. Receptor binding, fusion inhibition, and induction of cross-reactive neutralizing antibodies by a soluble G glycoprotein of hendra virus. J Virol (2005) 79(11):6690–702. doi: 10.1128/JVI.79.11.6690-6702.2005
27. White JR, Boyd V, Crameri GS, Duch CJ, van Laar RK, Wang L-F, et al. Location of immunogenicity of and relationships between neutralization epitopes on the attachment protein (G) of hendra virus. J Gen Virol (2005) 86:2839–48. doi: 10.1099/vir.0.81218-0
Keywords: sero-surveillance, Hendra virus, Nipah virus, horses, pigs
Citation: Adamu AM, McNabb L, Adikwu A A, Jibril YJ, Idoko SI, Turaki AU, Abalaka SE, Edeh RE, Egwu GO, Adah MI and Halpin K (2022) Henipavirus sero-surveillance in horses and pigs from Northern Nigeria. Front. Virol. 2:929715. doi: 10.3389/fviro.2022.929715
Received: 27 April 2022; Accepted: 29 June 2022;
Published: 02 August 2022.
Edited by:
Shuofeng Yuan, The University of Hong Kong, ChinaReviewed by:
Amy Schuh, Centers for Disease Control and Prevention (CDC), United StatesCopyright © 2022 Adamu, McNabb, Adikwu, Jibril, Idoko, Turaki, Abalaka, Edeh, Egwu, Adah and Halpin. This is an open-access article distributed under the terms of the Creative Commons Attribution License (CC BY). The use, distribution or reproduction in other forums is permitted, provided the original author(s) and the copyright owner(s) are credited and that the original publication in this journal is cited, in accordance with accepted academic practice. No use, distribution or reproduction is permitted which does not comply with these terms.
*Correspondence: Kim Halpin, a2ltLmhhbHBpbkBjc2lyby5hdQ==
†ORCID: Kim Halpin, orcid.org/0000-0002-1279-5010
Disclaimer: All claims expressed in this article are solely those of the authors and do not necessarily represent those of their affiliated organizations, or those of the publisher, the editors and the reviewers. Any product that may be evaluated in this article or claim that may be made by its manufacturer is not guaranteed or endorsed by the publisher.
Research integrity at Frontiers
Learn more about the work of our research integrity team to safeguard the quality of each article we publish.