- 1Australian Centre for Disease Preparedness, Commonwealth Scientific and Industrial Research Organisation, Geelong, VIC, Australia
- 2Flinders University College of Medicine and Public Health, Adelaide, SA, Australia
- 3Departments of Ophthalmology, and Cell, Developmental and Regenerative Biology, Icahn School of Medicine at Mount Sinai, New York, NY, United States
- 4Department of Ophthalmology and Visual Sciences, Truhlsen Eye Institute, University of Nebraska Medical Center, Omaha, NE, United States
Uveitis is a common manifestation of post-Ebola syndrome, associated with persistence of Ebola virus (EBOV; Zaire ebolavirus) inside the eye. The iris and retinal pigment epithelia are key components of the blood-ocular barriers, but have the capacity to act as hosts for microorganisms. We investigated the ability of EBOV to productively infect these cell populations. Donor-matched human iris and retinal pigment epithelial isolates (n = 5) were infected with EBOV at a multiplicity of infection of 1 for up to 72 hours. Parallel cultures were infected with Reston virus (RESTV; Reston ebolavirus) or Zika virus (ZIKV), or held uninfected under the same conditions. Viral transcript expression by RT-qPCR on total cellular RNA, cytoimmunofluorescence, and assays of 50% tissue culture infected dose of culture supernatant showed that both iris and retinal pigment epithelial isolates were permissive to infection, and supported replication and release of EBOV, as well as RESTV and ZIKV. However, in comparison to cells isolated from iris, those from retina demonstrated obvious EBOV-induced cytopathic effect, had higher intracellular EBOV nucleoprotein transcript, expressed intracellular EBOV protein more widely, and released EBOV at higher titer. Comparable results were obtained for isolates infected with RESTV and ZIKV. Consistent with observations of retinal pigment epithelial scars in Ebola survivors, our results suggest that an early event in post-Ebola uveitis is infection of the retinal pigment epithelium. Relative susceptibility of retinal pigment epithelial cells to infection with RESTV and ZIKV, as well as EBOV, implies this phenomenon may relate to a cell-specific attribute, such as high phagocytic activity.
1 Introduction
The majority of individuals who become infected with Ebola virus (EBOV; Zaire ebolavirus) and survive the acute hemorrhagic Ebola virus disease, develop a chronic inflammatory condition that is often referred to as ‘post-Ebola syndrome’ (1). This syndrome is characterized by arthritis, neuro-inflammation, and fatigue, and it has been linked to long-term persistence of live virus in immune-privileged sites (2). One of the most serious manifestations of post-Ebola syndrome is uveitis, or inflammation inside the eye, seen in up to 33% of Ebola survivors (3). Uveitis may affect the anterior and/or posterior segments of the eye, and lead to complications that include cataract, glaucoma and macular oedema. The inflammation or its complications cause vision loss in as many as 60% of survivors (4).
Ocular pigment epithelial cells play central roles in ocular infection and inflammation. These cells form an important component of the blood-ocular barriers that regulate the movement of molecules, cells, microorganisms and other foreign products between the bloodstream and the eye (5, 6). They have molecular properties that contribute to ocular immune privilege, which describes the mechanisms that the immune system uses to control inflammation within the eye in order to protect intraocular tissues that are critical for vision (7). However, these cells are also implicated in ocular pathology, with capacity to produce inflammatory cytokines and other molecules (8, 9), and the potential to act as host to a range of microorganisms (10–12).
These cells include the iris pigment epithelium protecting the anterior segment, and the retinal pigment epithelium protecting the posterior segment, which form a continuous layer with the intervening ciliary body epithelium. While the iris and retinal pigment epithelia play similar roles in the anterior and posterior eye, respectively, they are phenotypically distinct cells with different molecular profiles, that interact differently with microorganisms. The ocular pigment epithelial cell line – ARPE-19 (13) – is susceptible to infection with EBOV when exposed to high titer (14), but this cell has a different phenotype and often behaves differently to primary cells (15), including in the setting of ocular infection (16).
To understand how EBOV infects the human eye, to cause uveitis, we prepared multiple donor-matched, phenotyped iris and retinal pigment epithelial cell isolates from human cadaveric eyes, and infected these isolates in parallel with EBOV. We measured susceptibility of the cells to infection by multiple qualitative and quantitative methods, and we also compared susceptibility to Reston virus (RESTV; Reston ebolavirus, another member of the Ebolavirus genus, but understood to be non-pathogenic in humans) and Zika virus (ZIKV, another single-stranded RNA virus that causes systemic disease and uveitis, but of the family, Flaviviridae). This work represents the first effort to establish infectious mechanisms of EBOV in human ocular pigment epithelial cells.
2 Methods
2.1 Ocular Pigment Epithelial Cells
Donor-matched iris and retinal pigment epithelial cell isolates were prepared from paired human cadaver eyes, using methods we have previously published (10, 11). In brief, irises and retinal pigment epithelium-choroid were dissected from the two posterior eyecups. Irises were digested in 0.25% trypsin (Thermo Fisher Scientific-Gibco, Grand Island, NY), and pigment epithelial cells were brushed from the digested tissue. Cells were plated in Epithelial Cell Medium (ScienCell Research Laboratories, Carlsbad, CA; catalogue number 4101, containing 2% FBS and penicillin-streptomycin). The retinal pigment epithelium-choroid was digested with 0.5 mg/mL collagenase IA and 0.5 mg/mL collagenase IV, scraped off in sheets, and collected by sucrose density gradient centrifugation. Cell sheets were plated in 50% Minimum Essential Medium Eagle alpha modification (with sodium bicarbonate) [MEM], 25% Dulbecco’s Modified Eagle Medium [DMEM] and 25% F-12 with 1x N1 Medium Supplement, 1x Non-Essential Amino Acids Solution, 1x GlutaMAX Supplement, 0.25 mg/mL taurine, 0.02 μg/mL hydrocortisone, 0.013 ng/mL 3,3’,5-triiodo-L-thyronine sodium, 100 U/mL Penicillin-Streptomycin (all obtained from Merck-Sigma Aldrich, St Louis, MO or Thermo Fisher Scientific-GIBCO) and 10% FBS (Bovogen Biologicals, Keilor East, Australia).
The pigment epithelial cells were expanded in plating medium supplemented with 2% FCS, refreshed twice a week, at 37°C and 5% CO2 in air, and stored frozen in liquid nitrogen. Cell phenotype was verified for all cell isolates by immunocytochemical detection of cytokeratin-8, indicating epithelial lineage, and absence of α-smooth muscle actin, which is expressed during mesenchymal differentiation; expression of retinal pigment epithelial cell specific markers (i.e. cytokeratin-8, retinal pigment epithelium-specific protein 65 [RPE65] and zonula occludens 1 [ZO1]) were also assessed in those isolates (see ‘Cytoimmunofluorescence’). All cell isolates were demonstrated to be free of Mycoplasma species contamination by quantitative real-time polymerase chain reaction (qPCR) of DNA extracted from culture supernatant.
2.2 Viruses
The following viruses were used in this work: EBOV, variant Mayinga; RESTV, Philippines, 2008; and Zika virus (ZIKV), strain PRVABC59. These viruses were amplified in Vero C1008 cells (European Collection of Authenticated Cell Cultures [ECACC], Salisbury, UK), cultured with DMEM supplemented with 10% FBS at 37°C and 5% CO2 in air, and titrated by end-point dilution of culture supernatant in fresh Vero C1008 cell monolayers.
2.3 Viral Infection of Ocular Pigment Epithelial Cells
Ocular pigment epithelial cells suspended in Epithelial Cell Medium or supplemented 50% MEM/25% DMEM/25% F-12, both with 2% FBS, were plated at passage 2, in 6-well (growth area = 10 cm2) or 24-well (growth area = 2 cm2) multi-well plates, and incubated for 2 days at 37°C and 5% CO2 in air. Subconfluent cell monolayers were infected with EBOV, RESTV or ZIKV at a multiplicity of infection (MOI) of 1, or mock-infected, in minimum volumes of DMEM with 2% FBS (250 µL and 100 µL in 6-well or 24 well-plates, respectively). After 30-40 minutes, the medium was added back to standard volumes (2 ml and 1 ml in 6-well or 24 well-plates, respectively) with fresh medium. At intervals of 24, 48 and 72 hours, supernatant was collected and frozen at -80°C, and cells were either fixed or lysed with TRIzol Reagent (Thermo Fisher Scientific-Invitrogen, Carlsbad, CA), and stored at -80°C ahead of RNA extraction for reverse transcription (RT)-qPCR. At 48 hours, 24-well plates were fixed in 10% neutral buffered formalin for 48 hours, and stored at 4°C for cytoimmunofluorescence. All work with live virus was conducted under biosafety level 4 conditions, including the use of positive pressure personnel suits with segregated air supply.
2.4 Cytoimmunofluorescence
For cell phenotyping, 4% paraformaldehyde-fixed cell monolayers were labelled overnight at 4°C with one of following rabbit polyclonal antibodies diluted in 0.05% Triton X-100 and 2% bovine serum albumin in PBS: anti-human cytokeratin 8 (Abcam, Cambridge, United Kingdom; catalogue number ab53280; working dilution, 1:250, equivalent to 0.132 µg/mL), α-smooth muscle actin (Abcam, catalogue number ab5694; working dilution, 1:100, equivalent to 2 µg/mL) and rabbit immunoglobulin (Vector Laboratories, Burlingame, CA; catalogue number I-1000, working concentration, 2 µg/mL). Additional retinal pigment epithelial cell monolayers were labelled with: mouse anti-human RPE65 (Novus Biologicals, Centennial, CO; catalogue number NB100-355; working concentration, 4 µg/mL), rabbit anti-human ZO1 (Thermo Fisher Scientific-Invitrogen, catalogue number 40-2200; working dilution, 1:100, equivalent to 2.5 µg/mL) and mouse immunoglobulin (BD Pharmingen-BD Biosciences, San Diego, CA; catalogue number 555746, working concentration, 4 µg/mL). Cell monolayers were incubated with Alexa Fluor 488-tagged donkey anti-rabbit immunoglobulin antibody or anti-mouse immunoglobulin antibody (Thermo Fisher Scientific-Molecular Probes, Eugene, OR; catalogue numbers A11008 and A11029; working concentration, 1 µg/mL) for 1 hour at room temperature, counterstained with 4′,6-diamidino-2-phenylindole (DAPI), and imaged by fluorescence microscopy at 200x magnification.
To demonstrate viral infection, 10% neutral buffered formalin-fixed virus-infected and uninfected cell monolayers were permeabilized with 0.1% Nonidet P-40 (Merck-Sigma Aldrich), and labelled overnight at room temperature with rabbit anti-ebolavirus nucleoprotein (NP) antiserum (17), diluted 1:2000 to detect the ebolaviruses, or mouse anti-double stranded (ds)RNA monoclonal antibody (SCICONS, Budapest, Hungary) at 5 µg/mL to detect ZIKV in phosphate buffered saline with 1% bovine serum albumin. Subsequently, monolayers were incubated with Alexa Fluor 488-conjugated goat anti-rabbit immunoglobulin or anti-mouse immunoglobulin antibody (Thermo Fisher Scientific-Molecular Probes, catalogue numbers A11008 and A11001; working concentration, 2 µg/mL) for 1 hour at room temperature, counterstained with DAPI, and imaged on the EVOS FL Cell Imaging System (Thermo Fisher Scientific-Invitrogen) at 10x magnification.
2.5 RNA Extraction and Reverse Transcription
RNA was extracted by TRIzol Reagent (Thermo Fisher Scientific-Invitrogen), according to the manufacturer’s instructions, and stored at -80°C. Concentration was measured by spectrophotometry using the Nanodrop 2000 (Thermo Fisher Scientific, Wilmington, DE). For all samples, the cDNA synthesis reaction was performed using iScript Reverse Transcription Supermix for RT-qPCR (Bio-Rad Laboratories, Hercules, CA), with 500 ng of RNA input resulting in 20 μL of cDNA.
2.6 Ebolavirus Primers
Genomic sequences for EBOV and RESTV were obtained from the Nucleotide database of the US National Library of Medicine National Center for Biotechnology Information Nucleotide under the following accession identifiers: EBOV, AF086833.2; RESTV, AB050936.1. The sequences were aligned using the European Molecular Biology Laboratory-European Bioinformatics Institute Clustal Omega multiple sequence alignment web tool (18). Primers were designed that amplified 194 base pair (bp) of the ebolavirus NP transcript: forward 5’- TGGCAATCTGTCGGACAAATGATG-3’, reverse 5’- AGGATATGATCAAGGACGGTTTTGAC-3’. Primers included intentional mismatches (3 forward and 3 reverse) to ensure that transcript from the two viruses would be amplified with approximately equal efficiency: EBOV, 89.4%; RESTV, 92.8%. Products were sequenced to confirm amplification of the correct transcript.
2.7 Quantitative Real-Time Polymerase Chain Reaction
The qPCR was performed on the CFX Connect Real-Time PCR System (Bio-Rad Laboratories). In addition to SsoAdvanced Universal SYBR Green Supermix (Bio-Rad Laboratories) and nuclease-free water, each reaction contained 0.375 μM forward and 0.375 μM reverse primer, and 2 μL of undiluted cDNA. The ZIKV envelope (Env) primers were: forward 5’-GCTGGDGCRGACACHGGRACT-3’, reverse 5’-RTCYACYGCCATYTGGRCTG-3’ (304 bp amplicon, 76.5% amplification efficiency) (10). The GAPDH primers were: forward 5’-AGCTGAACGGGAAGCTCACTGG-3’, reverse 5’-GGAGTGGGTGTCGCTGTTGAAGTC-3’ (209 bp amplicon, 85.1% amplification efficiency) (19). Cycling conditions were as follows: pre-amplification hold of 95°C for 30 seconds; 45 cycles of denaturation at 95°C for 30 seconds, annealing at 54°C (NP) or 59°C (Env) for 30 seconds, extension; and fluorescence reading at 72°C for 30 seconds. Melting curves were performed from 70°C to 95°C for each run to confirm a single product. Absolute number of NP or Env transcripts was calculated from target starting quantity, which was determined from standard curves generated by serial dilution of purified PCR product in CFX Manager v3.0 (Bio-Rad Laboratories), from the formula: [target starting quantity (ng) x 6.022x1023]/[product length (bp) x 109 x 660]. Each result was normalized to glyceraldehyde 3-phosphate dehydrogenase (GAPDH) transcript number in the same sample.
2.8 Measurement of Viral Titer
Confluent monolayers of Vero C1008 cells were incubated in triplicate with 10-fold serial dilutions of supernatant collected from virus-infected pigment epithelial cells. After 7 days, cells were fixed for 48 hours with 10% neutral buffered formalin and immunolabeled to detect infected cells, following the method described in ‘Cytoimmunofluorescence’. The 50% tissue culture infective dose (TCID50) was determined according to the method described by Reed and Muench (20).
2.9 Statistical Analysis
Data were analyzed using GraphPad Prism (GraphPad Software, La Jolla, CA). The Mann-Whitney U test was used to compare cellular viral load and supernatant TCID50 between iris and retinal pigment epithelial cells. For all tests, a statistically significant difference was defined by a p-value of less than 0.05.
2.10 Research Compliance
Use of human cadaver donor eyes from the Eye Bank of South Australia (Adelaide, Australia) for this research was approved by the Southern Adelaide Clinical Human Research Ethics Committee (protocol number: 175.13).
3 Results
Ocular pigment epithelial cells were isolated separately from the posterior eyecups of 5 cadaveric donors (1 man and 4 women), whose ages at death ranged from 56 to 71 years (median = 64 years). Time from death to processing of the eyecups extended from 12 to 41 hours (median = 29 hours). Cytoimmunofluorescent labelling of all 5 paired cell isolates at the passage used for infections demonstrated strong expression cytokeratin-8 and no expression of α-smooth muscle actin, indicating an epithelial phenotype with no mesenchymal differentiation; in addition, all retinal cell isolates expressed the retinal pigment epithelial cell-specific proteins, RPE65 and ZO1 (Figure 1A).
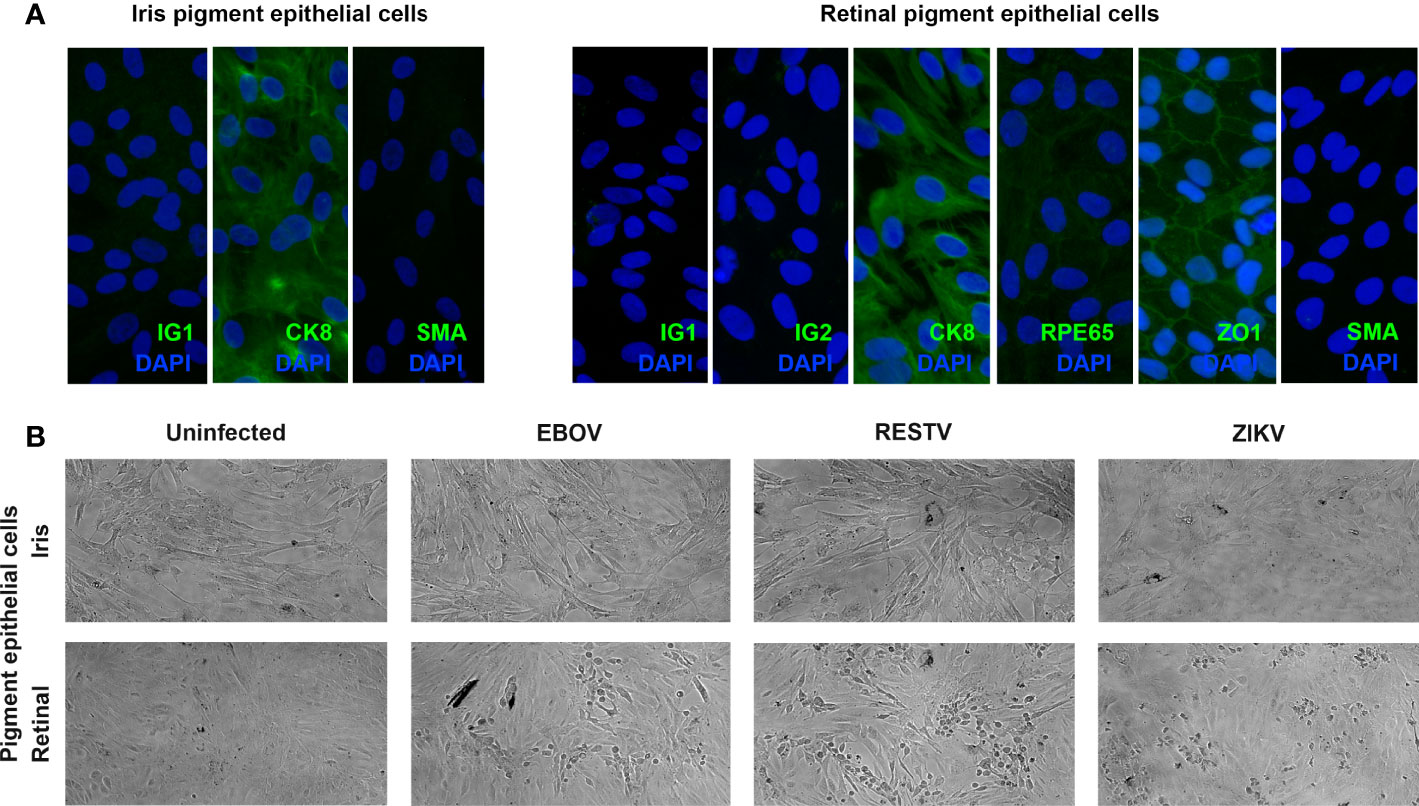
Figure 1 (A) Representative fluorescence photomicrographs of donor-matched human iris and retinal pigment epithelial cells immunolabelled to detect the presence of cytokeratin 8 (CK8) and absence of alpha-smooth muscle actin (SMA), plus human retinal pigment epithelial cells immunolabelled for retinal pigment epithelium-specific protein 65 (RPE65), and zonula occludens 1 (ZO1), with negative controls labelled with species-matched immunoglobulin (IG1 and IG2). Alexa Fluor 488 (green) with DAPI nuclear counterstain (blue). Original magnification: 400x. (B) Representative light photomicrographs of EBOV-, RESTV- and ZIKV-infected, plus uninfected donor-matched human iris and retinal pigment epithelial cell monolayers 72 hours following inoculation at a multiplicity of infection of 1. Original magnification: 10x.
To investigate the susceptibility of human ocular pigment epithelial cells to infection with EBOV, subconfluent donor-matched iris and retinal pigment epithelial cell monolayers were inoculated with virus at the MOI of 1 for intervals of 24, 48 and 72 hours. For comparison, additional cell monolayers from the same donors were inoculated in parallel with the non-pathogenic ebolavirus, RESTV, or the unrelated uveitogenic dsRNA virus, ZIKV, or incubated in parallel without inoculation. By 72 hours, virus-induced cytopathic effect was observed in retinal pigment epithelial cells inoculated with EBOV, as well as RESTV and ZIKV; this effect was not observed in the infected iris pigment epithelial cells (Figure 1B). This observation suggests that human retinal pigment epithelial cells are more susceptible to EBOV infection than iris pigment epithelial cells, and that a similar cell differential exists for other viruses.
Viral transcript in human ocular pigmented epithelial cells was quantified over time by RT-qPCR of total RNA extracted from the cell monolayers harvested at 24, 48 and 72 hours post-inoculation (Figure 2). Additional cell monolayers were fixed at 48 hours and immunolabelled for viral antigen: NP for EBOV and RESTV, and dsRNA for ZIKV (Figure 3). Level of viral transcript was similar across the different viruses for the same cell populations and time points; however, for each virus, transcript was higher in the retinal compared to the iris pigment epithelial cells across all time points (EBOV NP, p < 0.05 at 72 hours; RESTV NP and ZIKV Env, p < 0.05 at 48 hours). Immunolabelling of cell monolayers for viral antigen showed a clear difference in cell subset infection across all 5 paired human ocular cell isolates, with more infected cells in the retinal versus the iris pigment epithelial cell monolayers. These two results indicate that both human iris and retinal pigment epithelial cells are susceptible to infection with EBOV, as well as RESTV and ZIKV, but also suggest that retinal pigment epithelial cells are more readily infected.
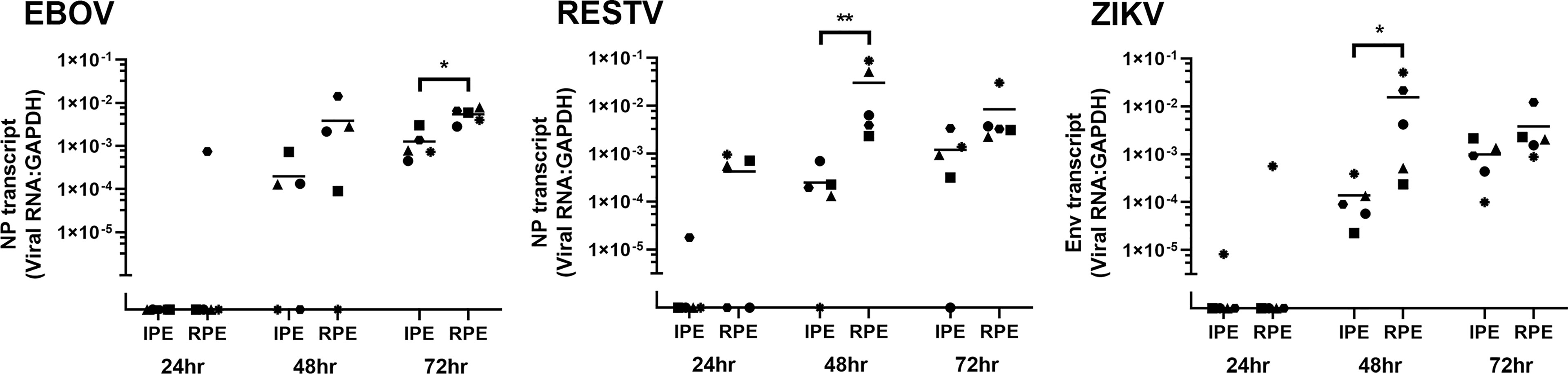
Figure 2 Graphs showing viral transcript in EBOV-, RESTV- and ZIKV-infected human iris (IPE) and retinal (RPE) pigment epithelial cell monolayers 24, 48 and 72 hours (hr) following inoculation at a multiplicity of infection of 1 (n = 5 donors/condition). NP = ebolavirus nucleoprotein; Env = ZIKV envelope. Shapes represent individual cell isolates, and crossbars represent means. Statistical comparisons were made between IPE and RPE by Mann-Whitney U test (*p < 0.05; **p < 0.01).
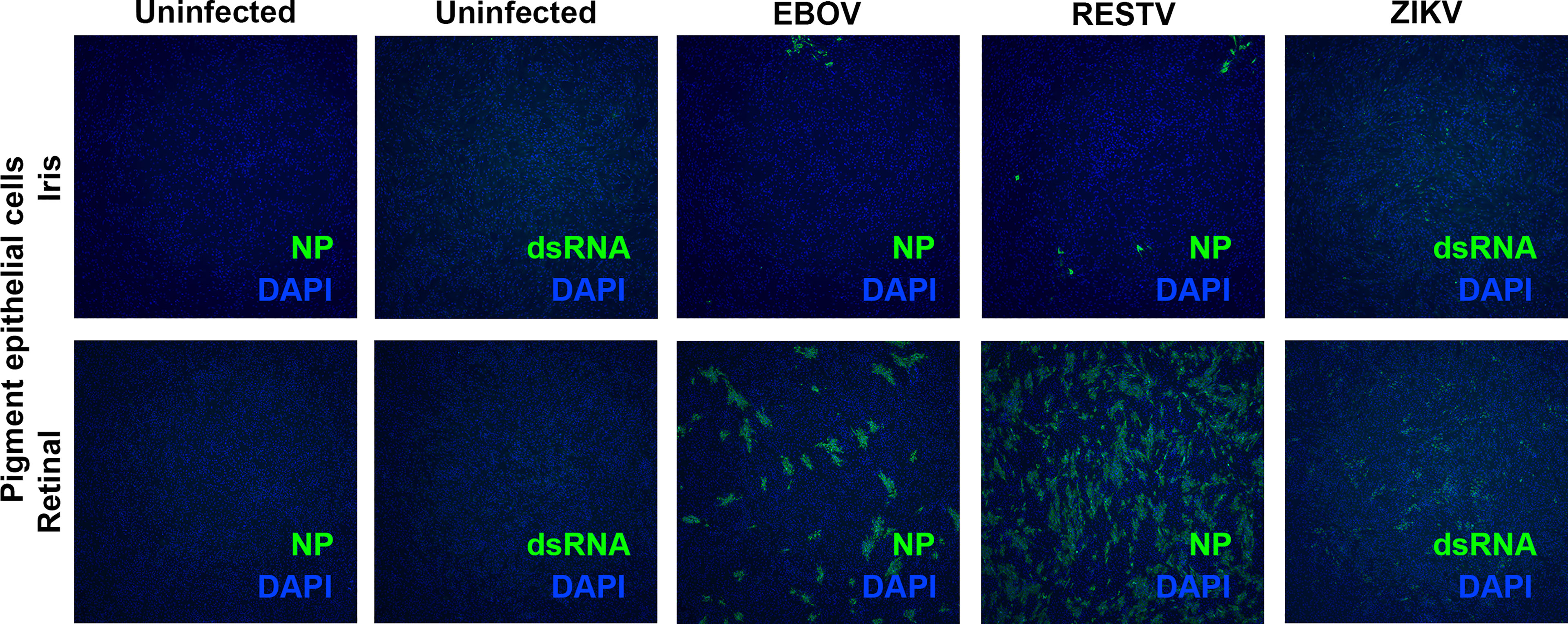
Figure 3 Representative fluorescence photomicrographs of EBOV-, RESTV- and ZIKV-infected, plus uninfected donor-matched human iris and retinal pigment epithelial cell monolayers 48 hours following inoculation at a multiplicity of infection of 1 immunolabelled to detect ebolavirus nucleoprotein (EBOV and RESTV) or double-stranded RNA (ZIKV). Alexa Fluor 488 (green) with DAPI nuclear counterstain (blue). Original magnification: 10x.
To confirm these observations of differential human ocular pigment cell infectivity with EBOV by another method, viral titer in supernatant collected from the cell monolayers at 24, 48 and 72 hours was determined as TCID50 (Figure 4). For infected cultures, the TCID50 increased across the time intervals, particularly for the retinal pigment epithelial cells, which released significantly more EBOV, plus RESTV and ZIKV, than the iris pigment epithelial cells by 48 hours (p < 0.01). Interestingly, the TCID50 for RESTV-infected pigment epithelial cells was higher than that for EBOV-infected cells across all time points, suggesting the cells have greater capacity to release infectious RESTV than EBOV up to 72 hours. For uninfected cultures, there was consistently no TCID50 measured. Taken together, these findings demonstrate that human retinal pigment epithelial cells are relatively more susceptible to infection with EBOV than iris pigment epithelial cells, and that this susceptibility extends to other viruses, here RESTV and ZIKV.
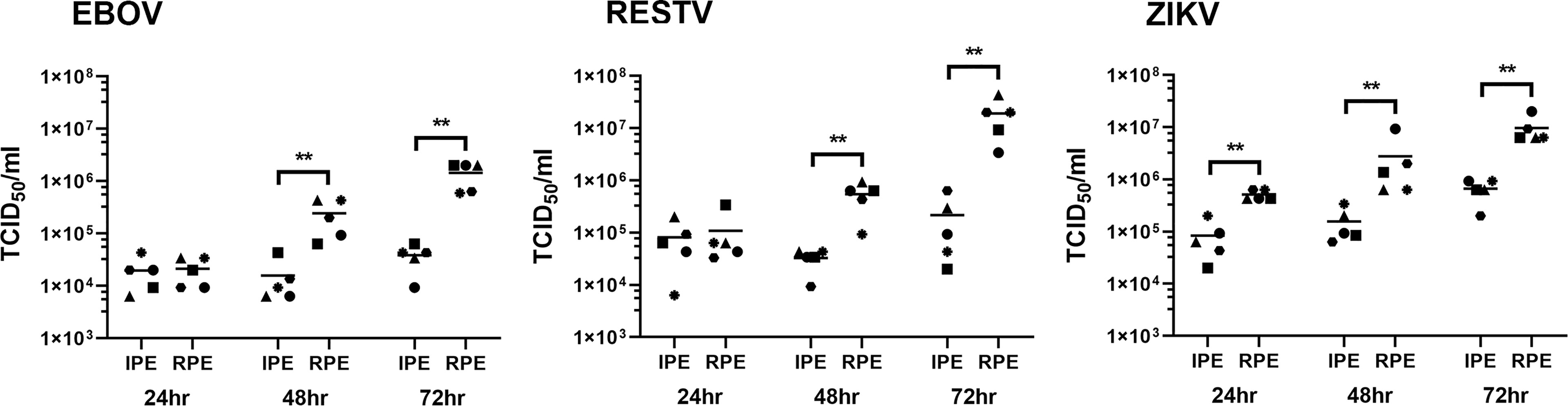
Figure 4 Graphs showing the 50% tissue culture infective dose (TCID50) in culture supernatant collected from EBOV-, RESTV- and ZIKV-infected human iris (IPE) and retinal (RPE) pigment epithelial cell monolayers 24, 48 and 72 hours (hr) following inoculation at a multiplicity of infection of 1 (n = 5 donors/condition). Shapes represent individual cell isolates, and crossbars represent means. Statistical comparisons were made between IPE and RPE by Mann-Whitney U test (**p < 0.01).
4 Discussion
Up to one-third of Ebola survivors will develop uveitis, associated with the persistence of live EBOV inside the eye. The ocular pigment epithelial cells are key players in ocular inflammation, and our work represents the first effort to examine the susceptibility of primary human ocular pigment epithelial cells to EBOV infection. Using donor-matched human iris and retinal pigment epithelial cell isolates and EBOV, variant Mayinga, we observed that both cell populations were permissive to infection, but that retinal pigment epithelial cells were substantially more susceptible. In comparison to pigment epithelial cells isolated from the iris, those from the retina demonstrated obvious viral-induced cytopathic effect, had higher intracellular viral transcript and more widely expressed protein, and released the virus at higher titer as the infection progressed. Our study design, with low MOI, was chosen to establish differences in infectivity of these two cell populations, but host cell responses to infection – including production of inflammatory, immunomodulatory and anti-viral cytokines and chemokines – would be of interest and could be addressed in future studies.
In order to access the eye from the blood stream, EBOV must interact with the blood-aqueous barrier or the blood-retinal barrier, placing the virus in contact with the iris pigment epithelium or the retinal pigment epithelium, respectively. The difference in susceptibility of these two cell populations to infection could reflect differences in effectiveness of EBOV entry into, replication within and/or exit from the cells. Overall, EBOV is able to enter a broad range of cells, as its surface protein, glycoprotein (GP)1, interacts with diverse, common cell surface proteins that include lectins, glycosaminoglycans, integrins, receptor tyrosine kinases, folate receptor 1, and T-cell immunoglobulin and mucin domain 1 (21). Once inside the host cell, EBOV uses cellular transcriptional, translational and post-transcriptional machinery during viral replication, and hijacks the host endosomal sorting complexes required for transport (ESCRT) pathway in order to bud from the cell membrane (21). Despite molecular promiscuity, differential infectivity by EBOV has been demonstrated amongst leukocyte subsets (22).
Another explanation for the relative susceptibility of retinal pigment epithelial cells to infection with EBOV may relate to their specialized cellular phenotype. Retinal pigment epithelial cells are considered an epithelial-derived subset of tissue-resident phagocytes (23). They mediate turn-over of photoreceptor outer segments by phagocytosis, which is essential for vision. However, retinal pigment epithelial cells may ingest other materials including apoptotic cells and microbial antigens (24, 25). For example, retinal pigment epithelial cells phagocytose Mycobacterium tuberculosis and permit intracellular replication (26). The fact that we saw similar differences for two comparison viruses – the closely related ebolavirus strain that is understood to be non-pathogenic in humans, and has a modified and less active GP1, RESTV (27), and the unrelated single-stranded virus that causes infectious disease and uveitis, ZIKV (10) – supports this possibility.
Across multiple cohort studies, there are reports of Ebola survivors with retinal scars (4, 28, 29) that by optical coherence tomography involve the outer neural retina and extend to the retinal pigment epithelium (29). The characteristic appearance of hyperpigmentation with a hypopigmented halo also is consistent with retinal pigment epithelial involvement. Of strong relevance, during a routine eye examination prior to the onset of uveitis, a patient whose clinical course has been described in considerable detail, was found to have retinal pigment epithelial scars; during uveitis extremely high levels of intraocular virus were detected in the affected eye (30). These findings all would be consistent with a high susceptibility of human retinal pigment epithelial cells to infection, suggesting the disease starts in these cells. Multiple types of post-Ebola uveitis have been reported, including anterior (based at the iris and ciliary body), intermediate (based in the vitreous), posterior (based at the choroid and/or retina) and panuveitis, with relative frequency of these different forms varying across studies by different groups (4, 28, 29); in the largest reported group of 564 Ebola survivors in Liberia (the PREVAIL III longitudinal cohort study), there was a slight predominance of posterior uveitis (29).
Our research has some limitations. The study necessarily involved in vitro infections of human ocular pigment epithelial cells. There is always potential for phenotypic drift in cultured cells, and retinal pigment epithelial cells in particular are prone to differentiation (31); thus, we used cells in earliest possible passage, and we confirmed the phenotype with cytoimmunofluorescence. We addressed inter-individual differences by studying isolates from multiple eyes, and using donor-matched iris and retinal pigment epithelial cells. We limited the comparison to iris and retinal pigment epithelial cells, and did not also study ciliary body epithelial cells; while one can readily separately identify iris and retina, given that the boundaries between iris and ciliary body, and ciliary body and retina are blurred, it is difficult to be certain of pure cell populations (32). Infections were not carried beyond 72 hours, when a virus-induced cytopathic effect in retinal pigment epithelial cells was observed; the infection may continue to progress in iris pigment epithelial cells past this time, to achieve higher intracellular expression of viral RNA and increased release of infectious virus. In the 2014 Ebola outbreak in West Africa, the Makona EBOV strain predominated, and we worked with the Mayinga strain, which was isolated during the 1976 Ebola outbreak in the Democratic Republic of Congo. Although unrelated research with dengue virus shows viral strain may impact ocular pathology (12), and EBOV strain-specific differences have not been studied, uveitis has been reported in both Congolese and West African Ebola survivors (4, 33).
In summary, our work has showed human retinal pigment epithelial cells to be relatively susceptible to infection with EBOV. This suggests that they may be a primary target within the eye, and also suggests that they could potentially be monitored during acute infection to identify patients at highest risk of uveitis: ophthalmic imaging modalities such as ‘fundus autofluorescence’ that demonstrates retinal pigment epithelial activity, which may not be visible clinically (34), would be particularly valuable in this context.
Data Availability Statement
The original contributions presented in the study are included in the article. Further inquiries can be directed to the corresponding author.
Ethics Statement
The use of human cadaver donor eyes for this research was approved by the Southern Adelaide Clinical Human Research Ethics Committee.
Author Contributions
SY, GM and JS conceptualized the study. ST, YM, LA, BA, MM and TB developed the methodology. ST, YM, LA and JS wrote the original draft. BA, MM, TB and SY reviewed and edited the manuscript. GM and JS supervised the study. All authors contributed to the article and approved the submitted version.
Funding
This work was supported by a grant from the National Health & Medical Research Council (GNT1139857 to JS).
Conflict of Interest
The authors declare that the research was conducted in the absence of any commercial or financial relationships that could be construed as a potential conflict of interest.
Publisher’s Note
All claims expressed in this article are solely those of the authors and do not necessarily represent those of their affiliated organizations, or those of the publisher, the editors and the reviewers. Any product that may be evaluated in this article, or claim that may be made by its manufacturer, is not guaranteed or endorsed by the publisher.
Acknowledgments
The authors wish to thank Ms. Janet Matthews for administrative support in preparing this manuscript.
References
1. Burki TK. Post-Ebola Syndrome. Lancet Infect Dis (2016) 16:780–1. doi: 10.1016/S1473-3099(15)00259-5
2. Jacob ST, Crozier I, Fischer WA 2nd, Hewlett A, Kraft CS, Vega MA, et al. Ebola Virus Disease. Nat Rev Dis Primers. (2020) 6:13. doi: 10.1038/s41572-020-0147-3
3. PREVAIL III Study Group, Sneller MC, Reilly C, Badio M, Bishop RJ, Eghrari AO, et al. A Longitudinal Study of Ebola Sequelae in Liberia. N Engl J Med (2019) 380:924–34. doi: 10.1056/NEJMoa1805435
4. Shantha JG, Crozier I, Hayek BR, Bruce BB, Gargu C, Brown J, et al. Ophthalmic Manifestations and Causes of Vision Impairment in Ebola Virus Disease Survivors in Monrovia, Liberia. Ophthalmology. (2017) 124:170–7. doi: 10.1016/j.ophtha.2016.10.011
5. Freddo TF. A Contemporary Concept of the Blood-Aqueous Barrier. Prog Retin Eye Res (2013) 32:181–95. doi: 10.1016/j.preteyeres.2012.10.004
6. O'Leary F, Campbell M. The Blood Retina Barrier in Health and Disease. FEBS J (2021). doi: 10.1111/febs.16330
7. Mochizuki M, Sugita S, Kamoi K. Immunological Homeostasis of the Eye. Prog Retin Eye Res (2013) 33:10–27. doi: 10.1016/j.preteyeres.2012.10.002
8. Chui JJ, Li MW, Di Girolamo N, Chang JH, McCluskey PJ, Wakefield D. Iris Pigment Epithelial Cells Express a Functional Lipopolysaccharide Receptor Complex. Invest Ophthalmol Vis Sci (2010) 51:2558–67. doi: 10.1167/iovs.09-3923
9. Fukuoka Y, Strainic M, Medof ME. Differential Cytokine Expression of Human Retinal Pigment Epithelial Cells in Response to Stimulation by C5a. Clin Exp Immunol (2003) 131:248–53. doi: 10.1046/j.1365-2249.2003.02087.x
10. Ryan FJ, Carr JM, Furtado JM, Ma Y, Ashander LM, Simoes M, et al. Zika Virus Infection of Human Iris Pigment Epithelial Cells. Front Immunol (2021) 12:644153. doi: 10.3389/fimmu.2021.644153
11. Lie S, Rochet E, Segerdell E, Ma Y, Ashander LM, Shadforth AMA, et al. Immunological Molecular Responses of Human Retinal Pigment Epithelial Cells to Infection With Toxoplasma Gondii. Front Immunol (2019) 10:708. doi: 10.3389/fimmu.2019.00708
12. Ashander LM, Lumsden AL, Dawson AC, Ma Y, Ferreira LB, Oliver GF, et al. Infection of Human Retinal Pigment Epithelial Cells With Dengue Virus Strains Isolated During Outbreaks in Singapore. Microorganisms (2022) 10(2):310. doi: 10.3390/microorganisms10020310
13. Dunn KC, Aotaki-Keen AE, Putkey FR, Hjelmeland LM. ARPE-19, a Human Retinal Pigment Epithelial Cell Line With Differentiated Properties. Exp Eye Res (1996) 62:155–69. doi: 10.1006/exer.1996.0020
14. Smith JR, Todd S, Ashander LM, Charitou T, Ma Y, Yeh S, et al. Retinal Pigment Epithelial Cells are a Potential Reservoir for Ebola Virus in the Human Eye. Transl Vis Sci Technol (2017) 6:12. doi: 10.1167/tvst.6.4.12
15. Strunnikova NV, Maminishkis A, Barb JJ, Wang F, Zhi C, Sergeev Y, et al. Transcriptome Analysis and Molecular Signature of Human Retinal Pigment Epithelium. Hum Mol Genet (2010) 19:2468–86. doi: 10.1093/hmg/ddq129
16. Smith JR, Ashander LM, Arruda SL, Cordeiro CA, Lie S, Rochet E, et al. Pathogenesis of Ocular Toxoplasmosis. Prog Retin Eye Res (2021) 81:100882. doi: 10.1016/j.preteyeres.2020.100882
17. Marsh GA, Haining J, Robinson R, Foord A, Yamada M, Barr JA, et al. Ebola Reston Virus Infection of Pigs: Clinical Significance and Transmission Potential. J Infect Dis (2011) 204 Suppl 3:S804–9. doi: 10.1093/infdis/jir300
18. Madeira F, Park YM, Lee J, Buso N, Gur T, Madhusoodanan N, et al. The EMBL-EBI Search and Sequence Analysis Tools APIs in 2019. Nucleic Acids Res (2019) 47(W1):W636–41. doi: 10.1093/nar/gkz268
19. Silverman MD, Zamora DO, Pan Y, Texeira PV, Baek SH, Planck SR, et al. Constitutive and Inflammatory Mediator-Regulated Fractalkine Expression in Human Ocular Tissues and Cultured Cells. Invest Ophthalmol Vis Sci (2003) 44:1608–15. doi: 10.1167/iovs.02-0233
20. Reed LJ, Muench LH. A Simple Method of Estimating Fifty Percent Endpoints. Am J Hyg (1938) 27:493–7. doi: 10.1093/oxfordjournals.aje.a118408
21. Rojas M, Monsalve DM, Pacheco Y, Acosta-Ampudia Y, Ramirez-Santana C, Ansari AA, et al. Ebola Virus Disease: An Emerging and Re-Emerging Viral Threat. J Autoimmun (2020) 106:102375. doi: 10.1016/j.jaut.2019.102375
22. Kotliar D, Lin AE, Logue J, Hughes TK, Khoury NM, Raju SS, et al. Single-Cell Profiling of Ebola Virus Disease In Vivo Reveals Viral and Host Dynamics. Cell. (2020) 183:1383–401 e19. doi: 10.1016/j.cell.2020.10.002
23. Kwon W, Freeman SA. Phagocytosis by the Retinal Pigment Epithelium: Recognition, Resolution, Recycling. Front Immunol (2020) 11:604205. doi: 10.3389/fimmu.2020.604205
24. Mayerson PL, Hall MO. Rat Retinal Pigment Epithelial Cells Show Specificity of Phagocytosis In Vitro. J Cell Biol (1986) 103:299–308. doi: 10.1083/jcb.103.1.299
25. Finnemann SC, Rodriguez-Boulan E. Macrophage and Retinal Pigment Epithelium Phagocytosis: Apoptotic Cells and Photoreceptors Compete for Alphavbeta3 and Alphavbeta5 Integrins, and Protein Kinase C Regulates Alphavbeta5 Binding and Cytoskeletal Linkage. J Exp Med (1999) 190:861–74. doi: 10.1084/jem.190.6.861
26. Nazari H, Karakousis PC, Rao NA. Replication of Mycobacterium Tuberculosis in Retinal Pigment Epithelium. JAMA Ophthalmol (2014) 132:724–9. doi: 10.1001/jamaophthalmol.2014.270
27. Fujihira H, Usami K, Matsuno K, Takeuchi H, Denda-Nagai K, Furukawa JI, et al. A Critical Domain of Ebolavirus Envelope Glycoprotein Determines Glycoform and Infectivity. Sci Rep (2018) 8:5495. doi: 10.1038/s41598-018-23357-8
28. Hereth-Hebert E, Bah MO, Etard JF, Sow MS, Resnikoff S, Fardeau C, et al. Ocular Complications in Survivors of the Ebola Outbreak in Guinea. Am J Ophthalmol (2017) 175:114–21. doi: 10.1016/j.ajo.2016.12.005
29. Eghrari AO, Bishop RJ, Ross RD, Davis B, Larbelee J, Amegashie F, et al. Characterization of Ebola Virus-Associated Eye Disease. JAMA Netw Open (2021) 4:e2032216. doi: 10.1001/jamanetworkopen.2020.32216
30. Varkey JB, Shantha JG, Crozier I, Kraft CS, Lyon GM, Mehta AK, et al. Persistence of Ebola Virus in Ocular Fluid During Convalescence. N Engl J Med (2015) 372:2423–7. doi: 10.1056/NEJMoa1500306
31. Fronk AH, Vargis E. Methods for Culturing Retinal Pigment Epithelial Cells: A Review of Current Protocols and Future Recommendations. J Tissue Eng. (2016) 7:2041731416650838. doi: 10.1177/2041731416650838
32. Janssen SF, Gorgels TG, Bossers K, Ten Brink JB, Essing AH, Nagtegaal M, et al. Gene Expression and Functional Annotation of the Human Ciliary Body Epithelia. PloS One (2012) 7:e44973. doi: 10.1371/journal.pone.0044973
33. Kibadi K, Mupapa K, Kuvula K, Massamba M, Ndaberey D, Muyembe-Tamfum JJ, et al. Late Ophthalmologic Manifestations in Survivors of the 1995 Ebola Virus Epidemic in Kikwit, Democratic Republic of the Congo. J Infect Dis (1999) 179(Suppl 1):S13–4. doi: 10.1086/514288
Keywords: ebola virus, human, retina, iris, pigment epithelium, uveitis
Citation: Todd S, Ma Y, Ashander LM, Appukuttan B, Michael MZ, Blenkinsop TA, Yeh S, Marsh GA and Smith JR (2022) Brief Research Report: Ebola Virus Differentially Infects Human Iris and Retinal Pigment Epithelial Cells. Front.Virol. 2:892394. doi: 10.3389/fviro.2022.892394
Received: 09 March 2022; Accepted: 23 May 2022;
Published: 16 June 2022.
Edited by:
Velpandi Ayyavoo, University of Pittsburgh, United StatesReviewed by:
Robert W. Cross, University of Texas Medical Branch at Galveston, United StatesLogan Banadyga, Public Health Agency of Canada (PHAC), Canada
Copyright © 2022 Todd, Ma, Ashander, Appukuttan, Michael, Blenkinsop, Yeh, Marsh and Smith. This is an open-access article distributed under the terms of the Creative Commons Attribution License (CC BY). The use, distribution or reproduction in other forums is permitted, provided the original author(s) and the copyright owner(s) are credited and that the original publication in this journal is cited, in accordance with accepted academic practice. No use, distribution or reproduction is permitted which does not comply with these terms.
*Correspondence: Justine R. Smith, anVzdGluZS5zbWl0aEBmbGluZGVycy5lZHUuYXU=
†These authors have contributed equally to this work and share first authorship
‡These authors have contributed equally to this work and share senior authorship