- Department of Obstetrics, Gynecology, and Reproductive Sciences, Larner College of Medicine, University of Vermont, Burlington, VT, United States
Pregnant mice infected with Lymphocytic Choriomeningitis Virus (Armstrong) (LCMV-Arm) experience high viral loads in the placenta and uterine tissue by 5–8 days post-infection, a time when the virus is nearly completely cleared from the spleen and blood. Interleukin 10 (IL-10) plays a crucial role in T cell responses associated with systemic viral clearance. Using the LCMV-arm model of infection, we examined first, whether IL-10 is involved in viral clearance in the placenta and uterine tissue and secondly, the potential mechanisms underlying this phenomenon. C57BL/6 (WT) and mice deficient in IL-10 (IL-10 KO) were infected with LCMV-Arm on day 10 of pregnancy. Placenta and uterine tissue, collected 2 and 8 days later, were analyzed using real time RT-PCR, plaque assays for viral load, and flow cytometry. In WT mice placenta and uterine tissue expression of IL-10 was elevated with LCMV-Arm infection. Fetus resorption was elevated in WT on days 2 and 8 post-infection as compared to IL-10 KO, and by day 19 of gestation delivery was greater. Viral loads in the placenta and uterine tissue were resolved early in IL-10 KO mice, but persistent in tissues of WT mice. Levels of NRF2 and FAS were equivalent, but BCL2L11 was higher in IL-10 KO uterus. IL-6, Interferon-β (IFN-β), CCL2, and IL-17 levels were also equivalent. IL-10 KO tissues tended toward higher expression of interferon-γ (IFN-γ) and had significantly lower expression of Transforming growth factor beta (TGF-β). The proportion of placenta and uterine tissue CD8 T cells expressing the activation markers CD44hi and PD1 were elevated in IL-10 KO mice. These data suggest that high IL-10 expression at the fetal-maternal interface following LCMV-Arm infection prevents clearance of viral load by impairing CD8 T cell activation and poses a significant threat to successful pregnancy outcome. The ability to modulate IL-10 expression at the maternal-fetal interface may help overcome negative pregnancy outcomes arising during acute LCMV and other viral infections in humans.
Introduction
Emerging infections with such agents as Zika virus and Coronavirus, and more common agents such as Influenza and Cytomegalovirus continue to serve as reminders about our deficient understanding of maternal immunity. This is especially true with regard to the maternal fetal interface. In spite of potentially relevant conflicting data [reviewed elsewhere (1)], there still exist models of maternal tolerance which rely on mechanisms of inherent suppression of maternal immunity. These mechanisms are thought to act systemically or at the maternal-fetal interface, and at the tissue or cellular level. The study of infections during pregnancy offers an opportunity to examine and determine the relevance of some of these mechanisms. In addition, it presents a means to uncover possible subtle elements and regulatory circuits in the maternal immune system. These may support fetal tolerance, but at the same time protect fetus and mother against overwhelming disease. Accumulating evidence suggests that pregnancy drives the expansion of unique cellular phenotypes particularly at the maternal fetal interface (2–4) which may specifically express mechanisms which can both support immune surveillance and minimize harmful anti-fetal tissue responses (5, 6).
Lymphocytic choriomeningitis virus (LCMV) is an ambisense single stranded RNA virus belonging to the family Arenaviridae, which can cause human disease (7, 8). Generally, infection occurs through contact with aerosolized mouse feces and urine, causing a self-limited febrile illness (9). However, the virus can be transmitted vertically in mice (10) and humans (11). In humans, transmission vertically results in congenital neurologic abnormalities (12, 13) while death is an outcome if viral transmission occurs via transplantation of infected organs (14, 15) in immune suppressed patients. A recent estimate is that ~4% of pregnant women may be seropositive with LCMV specific IgG (16).
Laboratory strains of this virus have long been used to study mechanistic aspects of the elicited immune response in mice. Infection with the LCMV-Armstrong strain results in an acute infection with a peak systemic viral load about 3 days after inoculation. This is followed by a rise in CD8 virus-specific effector T cells that peaks between 5 and 8 days post-infection (17) and which contributes to the resulting clearance of virus. By day 15 of infection, the CD8 T cell population consolidates and forms a memory T cell pool (18). In contrast, infection with Clone 13 leads to persistent infection and is associated with dysregulation of T cell function (19).
We have previously observed that infection with the LCMV-Arm on day 8–10 of pregnancy results in systemic (spleen, peripheral blood) immunity, including specific CD8 T cell expansion, interferon-γ (IFN-γ) production, cytotoxicity and viral clearance that is equivalent to that found in non-pregnant mice infected at the same time. However, by 8 days after infection, when the systemic circulation is cleared of virus, the placenta and uterus remain infected (20) until birth.
Interleukin-10 (IL-10) is an important driver of “anti-inflammatory” and regulatory immune functioning in subsets of T cells (21), B cells (22) “alternative” macrophages (23), and dendritic cells (24). It is a complex moderator of autoimmunity (25) and anti-tumor immunity (26). Particularly, the activity of IL-10 is thought to be a driver of pathogen persistence and chronicity in parasitic (27) and viral infections (28–31), including infection with LCMV Clone 13 in non-pregnant mice. This activity is thought to be related to its role in inhibiting T cell production of IFN-γ and other immunomodulatory mechanisms (28, 31, 32). IL-10 may also have pleiotropic functions as it may control angiogenesis (33) vascular function (34) and apoptosis (35). In pregnancy, IL-10 is expressed by trophoblast (36) and decidua (37). It is also thought to be important in early pregnancy development, as relative deficiency is a contributor to early pregnancy loss in both mouse models (38, 39) and in human recurrent early pregnancy loss (40). In addition, mid-gestation deficiency in IL-10 has been linked to the inflammatory response leading to lipopolysaccharide-induced preterm birth in mouse models (41–43). However, early higher expression of IL-10 (44) or a group of factors that include IL-10 (45) in humans identifies women who subsequently have preterm birth, and is elevated in chronic placental infection [e.g., malaria (46)] in humans. This suggests a complex relationship between viral infection, immunity, IL-10 and homeostasis in pregnancy-related tissues. To begin to understand this relationship, we returned to our finding of viral persistence in mice infected with LCMV-Arm, which does not cause systemic viral persistence, but does cause prolonged infection in the placenta with mid-gestation infection (20). We hypothesized that IL-10 modulates the local immune response to LCMV-Arm. We report that deficiency in IL-10 led to a significantly lower viral load in the placenta and uterine tissue 8 days post mid-gestation infection. This was correlated with alterations of cytokine expression in these tissues, and an increase in activated CD8 T cells, but a decrease in the extent of fetal resorption. These data point to a complex interaction between maternal immunity, acute LCMV infection and the fetal-placental unit, and support an important role for IL-10 in aberrant pregnancy outcome following acute infection with LCMV.
Materials and Methods
Mice and Infection
Adult male and female C57BL/6 (B6, or “Wild Type” WT stock # 00664) and IL-10 KO mice of the same genetic background (Stock # 002251) were obtained from The Jackson Laboratory (Bar Harbor, ME). Mice were maintained under specific pathogen-free conditions and used in procedures approved by the Institutional Animal Care and Use Committee at the University of Vermont and in accordance with The Association for Assessment and Accreditation of Laboratory Animal Care. Females were mated with same-strain males and the males were removed 24 h later which was taken as ~day 0 of pregnancy. On day 10 of gestation, pregnant B6 and IL-10 KO females were infected with LCMV-Arm (2 × 105 PFU/mouse) by intraperitoneal (IP) injection in 100 ul PBS or left uninfected and unmanipulated (20). At days 2 and 8 post-infection (i.e., ~days 12 and 18 of gestation) mice were euthanized by CO2 inhalation. Fresh samples from the spleen, uterine tissue (wall and decidua), placenta and uterine draining lymph nodes were harvested and either used immediately for flow cytometry or stored in RPMI (Sigma, St. Louis, MO) or frozen at −80°C until further use for either plaque assay or RNA expression. Analysis elaborated here include one representative, healthy-appearing (e.g., not necrotic) placental sample or one uterine sample (2–3 implantation sites and underlying nonplacental, e.g., decidua/endometrium/myometrium, tissue plus intervening non-implantation uterus) per mouse. All infection-related experiments were carried out in an ABSL2+ facility at the University of Vermont.
Flow Cytometry
The spleen, uterine tissue, placenta and uterine draining lymph nodes of pregnant B6 and IL-10 KO mice were placed in “Ghost special” medium [Iscove's Modified Dubeco's Medium, IMDM, without bicarbonate (Gibco, Carlsbad, CA)], supplemented with 1% fetal bovine serum (FBS; Gibco). From spleen and uterine draining nodes, single cell suspensions were generated through mechanical dissociation using 70 um nylon mesh. Uterine tissue and placenta were washed once in Ghost medium, cut into small pieces and subjected to enzymatic digestion by incubation in Hanks Balanced Salt Solution (HBSS, Gibco/Invitrogen, Grand Island, NY) containing 200 U/ml hyaluronidase (Sigma), 0.2 mg/ml DNAse I (Sigma) and 28 U/ml Liberase Blendzyme 3 (Roche, Indianapolis, IN) for 20 min in a 37°C warm water bath. After digestion, samples were pressed through 70-μm mesh. The resulting cell suspension was washed with phosphate buffered saline (27) (Mediatech, Manassas, VA) supplemented with bovine serum albumin (0.1%; PBS-BSA; Sigma). Approximately 1 × 106 cells were incubated in a 1:50 dilution of 2.4G2 (BD Biosciences, San Jose, CA) in order to block non-specific antibody staining due to Fc receptors, and then incubated for 1 h at room temperature in a specific antibody mix, washed twice with PBS-BSA and then fixed with PBS-BSA+1% paraformaldehyde (Fisher Scientific, Fair Lawn, New Jersey). The antibodies used in these studies included CD45.2 (clone 104, PerCP-Cy™ 5.5), PD1(Clone J43 PE), from e-Bioscience (San Diego, CA); CD8 (Clone 5H10-PE-Texas Red), Invitrogen, and CD44 (clone IM7 APC BD-Biosciences, San Jose, CA). Samples were processed with a LSRII flow cytometer (BD Biosciences) and the results of ~10K cells were analyzed using FLOWJO software (Version 8.8, Tree Star, Inc, Ashland, OR). The gating scheme used was previously described (47).
Plaque Assay
Placenta and uterine tissue were dissected, weighed and kept in 200 μl of RPMI (Sigma) supplemented with 1% FBS at −80°C until they were homogenized using a Mini-BeadBeater setup (BioSpec, Bartlesville, OK). Plaque assays were then performed on homogenates as previously described (20). Briefly, serial dilutions of virus-infected homogenates were placed on monolayers of VeroE6 cells and incubated for 1 h at 37°C. A warm mix of 1 × M199 media (Sigma) and 0.5% agarose was overlaid and allowed to solidify for 20 mins. Plates were then incubated for another 6 days, fixed with 25% paraformaldehyde (Fisher Scientific) and stained with 0.1% crystal violet. Excess stain was washed with water and then the plaques were visually counted. Data are expressed as PFU (number of plaques × 106) / gm of tissue used in the assay).
QRT-PCR
Placenta (one representative healthy-appearing per mouse) and samples of uterine tissue (2–3 implantation sites with intervening tissue) were dissected, immediately frozen and stored at −80° C until ready for processing. Total RNA was extracted from 0.5 to 1 mg of tissue using the PrepEase RNA spin kit from USB [The iScript cDNA synthesis kit (Bio-Rad Laboratories, Hercules, CA)] was used to synthesize cDNA from 250 ng of RNA template using a mix of random hexamers and oligo dTs. From each sample 1μl cDNA was used to amplify the genes of interest. QRT-PCR was performed on an ABI Prism 7000 (Applied biosystems-CA) using Power Sybrgreen master mix. Each sample was run in triplicate and the CTs were averaged. The following primers (forward and reverse, each in 5′-3′ orientation) were used for amplification:
Beta-2 microglobulin (β2m) ATGCTATCCAGAAAACCCCTCAAA and CAGTTCAGTATGTTCGGCTTCCC; Fas, AACAAAGTCCCAGAAATCGCCTATG and TCCTGTCTCCTTTTCCAGCACTT; Forkhead box protein P3 (Foxp3), AATGGGTGTCCAGGGAGC and TGGCAGTGCTTGAGAAACTC; Transforming growth factor beta (Tgf-β), CGCAACAACGCCATCTATGAG and TGCTCCACACTTGATTTTAATCTCTGC; Interferon gamma (Ifn-γ), CCTCATGGCTGTTTCTGGCTGTTA and CATTGAATGCTTGGCGCTGGACC; Ifn-γ receptor, CAGGTAAAGGTGTATTCGGGTTCC and CCAGGCAGATACATCAGGATACATAAT; Interleukin-10 (IL-10), TTACTGACTGGCATGAGGATCA and GAAAGAAAGTCTTCACCTGGCTGA; IL-17, GACTCTCCACCGCAATGAAGACC and CCCACACCCACCAGCATCT; IL-6, AGAAAGACAAAGCCAGAGTCCTTCAG and GTCCTTAGCCACTCCTTCTGTGACT; Bcl-2-like protein 11 (Bcl2L11) GACGGAAGATAAAGCGTAACAGTTGT and TCCATACGACAGTCTCAGGAGGAA; Nuclear factor (Erythroid-derived 2)-like Nrf2 ATGATGGACTTGGAGTTGCCA and GCTCATAGTCCTTCTGTCGC; Interferon beta (Ifn-β )TGTCCTCAACTGCTCTCCAC and CCTGCAACCACCACTCATTC; CCL2 (monocyte chemoattractant protein-1, MCP-1), TGATCCCAATGAGTAGGCTGGAG and ATGTCTGGACCCATTCCTTCTTG. Relative expression was determined using the ΔΔCT method with an uninfected uterine sample as a comparator, and the results of PCR with β2m as a “loading control.”
Statistics
Data was graphed, visualized for trends and analyzed using GraphPad Prism 7 (GraphPad Software, San Diego, CA). Data points are displayed individually with lines delineating means or the tops of columns delineating medians. Data passing at least one of three normality tests were compared using parametric analysis. Two groups were compared with the t test while more-than-two group analysis used ANOVA with post-test correction for multiple-comparison testing. For data not passing normality, two group comparisons utilized the Mann-Whitney test, while more than two groups utilized Kruskal Wallis testing, and adjusted using the Dunn's multiple comparisons test. Significance was set at p < 0.05, although some trends are noted.
Results
Previous studies of LCMV infection in the mouse placenta and decidua showed that 8 days after infection with an intraperitoneal dose of LCMV (20), the systemic circulation developed an increase in peripheral blood and spleen LCMV-specific CD8 cells and activated CD4 cells as occurs in non-pregnant mice. However, while the spleen is cleared of LCMV by 8 days post infection, the decidua (uterus) and placenta is not. One possible explanation for this difference is enhanced viral uptake, as subsequent studies revealed the increased expression of a potential receptor for the virus in mid gestation placenta (48). However, one potential rational for prolonged viral infection in the relevant tissues are the adaptive and innate immune responses generated in those tissues, as deficiency in tissue specific immunity is a potential contributor to viral persistence in other models of infection. To begin to assess the local immune and inflammatory response to LCMV infection, we examined the expression of a variety of cytokines relevant to innate and adaptive immunity in uterine and placental tissues of C57BL/6 (Wild Type, WT) mice. Adult mice were mated to same-strain males and infected on ~day 10 of pregnancy, since previous studies suggested a very high rate of resorption with infection earlier in gestation (20). Figure 1 shows a comparison to the tissues in uninfected mice. Two timepoints are examined. “Early” refers to 2 days post infection, ~ 12 days of gestation, while “late” refers to 8 days post infection, ~18 days of gestation. While expression of molecules such as IFNγ-R (Figure 1C) and FOXp3 (Figure 1G) are not significantly changed over time and infection, three other patterns of RNA expression are discernable. In one pattern (e.g., Figure 1B, IFN-γ) expression does not appear to be different from uninfected tissues until late in infection. In another (e.g., Figure 1D, TGFβ) infection over time is associated with decreased expression. In a third, (e.g., Figure 1E, IL-17, uterus) expression is elevated soon after infection and continues to be elevated late in infection, raising the speculation of pleiotropic functions in the context of infection.
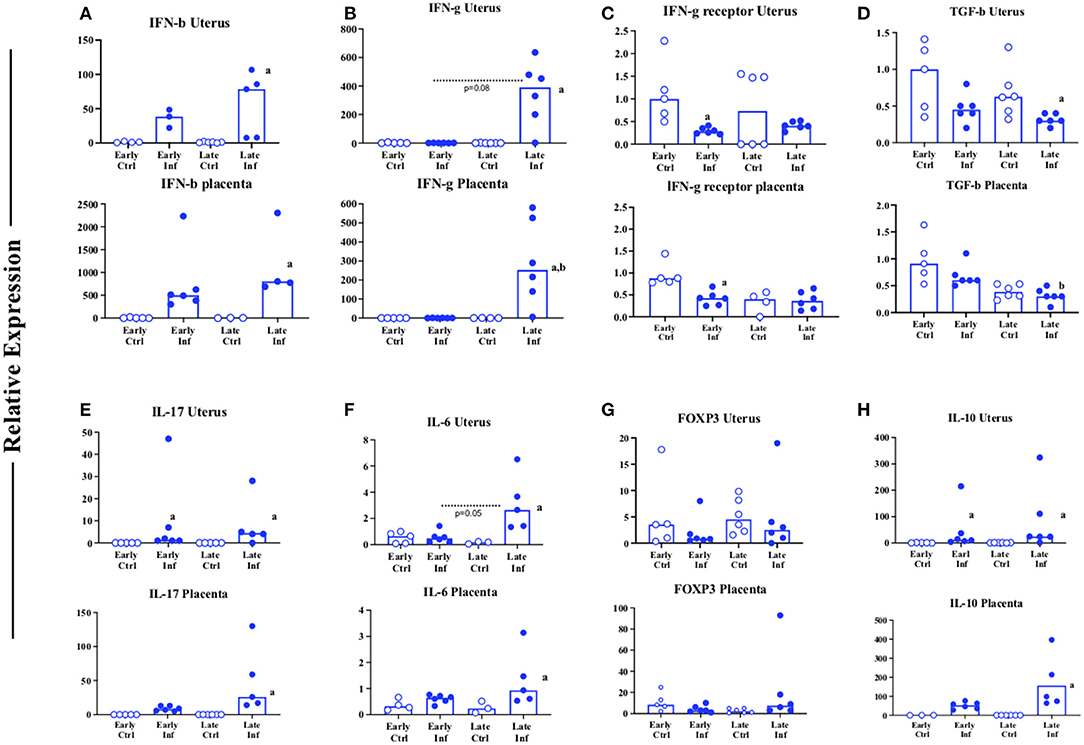
Figure 1. Cytokine profile of uterine and placental tissues in normal mice infected with LCMV during pregnancy. C57BL/6 (B6) mice were infected (closed symbols) or not infected (open symbols) ip with 2 × 105 pfu of LCMV Armstrong on day 10 of pregnancy. (A–H) Values for the uterus (top) or the placenta (bottom) of the samples collected. ~Day 12 (early), or ~18 (late) of pregnancy the mice were euthanized, and the tissues indicated were tested by QT-PCR for expression. Y axis: relative expression using the ΔΔCT method compared to sample from an unmanipulated animal. X axis: time grouping. Each symbol represents an individual sample from an individual (distinct) mother (n = 4–6 mothers). Inf denotes samples from an infected mouse while Ctrl denotes samples from an unmanipulated mouse. Data was analyzed using Kruskal Wallis test with Dunn's correction for multiple comparisons testing. Letters: a denotes significance (e.g., p < 0.05) when comparing infected to control samples obtained at the same gestational age; b denotes significance between samples obtained early or late post infection. Trends (p < 0.1) are noted numerically.
One cytokine with this pattern raised this same speculation: IL-10 (Figure 1H). Because of our finding, and the existing evidence in the literature, we examined pregnancy outcome in infected WT mice and in mice deficient in Interleukin-10 (IL-10 KO, Figure 2). Because we suspected a high level of resorption in the IL-10 KO mice based on a mouse model of early pregnancy loss (38), we examined the uteri of WT and IL-10 KO mice 2 and 8 days after infection. When we reviewed the proportion of resorption sites with necrotic fetal-placental units consistent with resorption, WT mice had a significantly greater proportion at both 2 (Figure 2A, left) and 8 days (Figure 2A, right) after infection as compared to IL-10 KO mice who experienced a level roughly similar to that found in uninfected WT mice (49). Because IL-10 deficient mice are more susceptible to preterm birth in response to toll like receptor activation with agents such as LPS (42), we expected that deficiency in IL-10 in the face of LCMV infection would lead to earlier delivery. Observations suggest that mice on this genetic (e.g., C57BL/6) background have a gestational length of ~19.5 days (50). Because of this we undertook an investigation the number of non-resorbed implantation sites did or did not have an attached fetus (non-delivered vs. delivered Figure 2B). Unexpectedly, analysis of the calculated proportions suggested that proportionately more WT pups of infected mothers had delivered by day 19 of gestation (Figure 2B). Parturition is the outcome of a complex developmental process [reviewed in (51)] that likely is influenced by infection. To begin to probe the potential factors that might mediate oxidative stress (which could also lead to pathways such as senescence), cell death, and tissue dysregulation in infected WT and IL-10 infected tissues at day ~18 of gestation, we examined tissue RNA expression of NRF2 (52), BIM/BCL2L11 (53, 54) and FAS/CD95 (35, 54) (Figure 2). Although uterine NRF2 is decreased by infection in WT mice (Supplementary Figure 1), we observed that both NRF (Figure 2C), and FAS (Figure 2E) were similarly expressed in tissues of IL-10 KO mice as compared to WT. In addition, RNA expression of the pro-apoptotic mitochondrial molecule BCL2L11 was increased only in the placenta in IL-10 KO mice (Figure 2D).
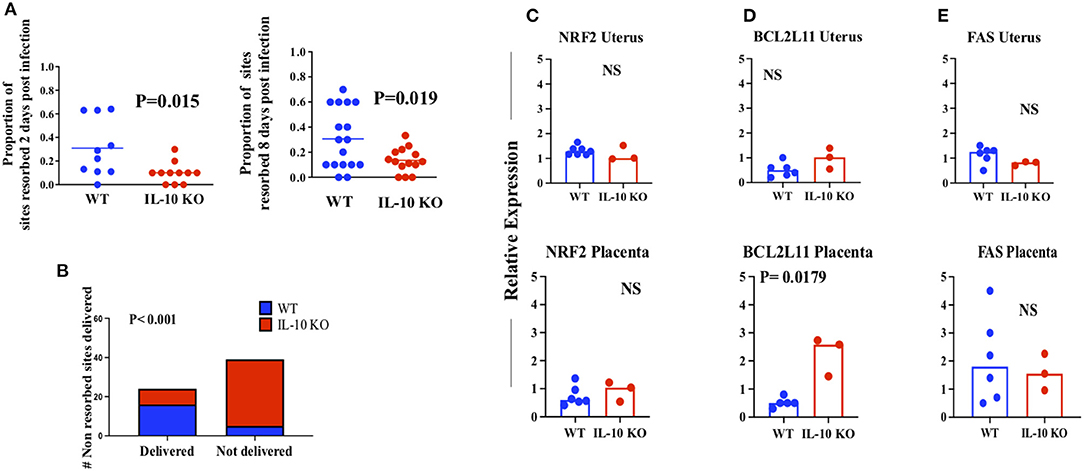
Figure 2. Effect of LCMV infection on pregnancy outcome in WT and IL-10 KO mice. (A) Proportion of implantation sites resorbed (necrotic) 2 days (left, n = 10 WT and 11 IL-10 KO mothers euthanized) and 8 days (right, n = 17 WT and 14 IL-10 KO) post infection. Each symbol represents a mother euthanized. (B) Number of non-resorbed sites delivered. Uteri (n = 5 WT and 7 IL-10 KO) were opened on day 19 of gestation and the presence of a pup was counted as a “non-delivered” site. Lines, sample means. p values, analysis of significance by the t test. (C–E) RNA expression by QT-PCR of the indicated molecules in the indicated tissues on day ~18 of gestation. Bars, sample medians. p values, analysis of significance by the Mann Whitney test. Blue symbols, WT; Red symbols IL-10 KO. NS, not significant at the p < 0.05 level.
Differences in reproductive outcome in WT and IL-10 KO mice could be due to viral load over time after infection in reproductive tissues, even if virus is cleared from systemic lymphoid tissues (20) (Supplementary Figure 2). We thus examined uterus and placenta of WT and IL-10 KO mice using a plaque assay (Figure 3). We infected mice on ~day 10 of gestation and harvested tissues 2 (early) and 8 days later. We found that viral loads appeared equivalent and low early post infection in both uterus (Figure 3A) and placenta (Figure 3B) from WT and IL-10 KO mice. However, late post infection, the viral load was statistically greater in both tissues from WT as compared to IL-10 KO mice.
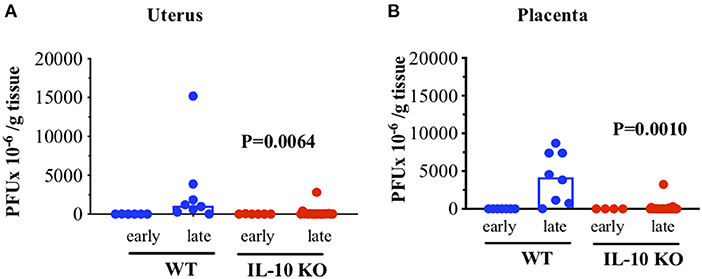
Figure 3. Viral load in WT versus IL-10 KO mice infected with LCMV. WT (C57BL/6) females or IL-10 KO females were mated to same-strain males and on day ~10 of gestation were infected as in Figure 1 and euthanized on days ~12 and ~18 of gestation. Placentas were homogenized and then tested for viral load by plaque assay. (A) Uterine tissues (n = ~10–20/strain/time). (B) Placental tissues (n = ~10–20/strain/time). Y axis: PFU × 10−6 virus per gram tissue as assessed by plaque assay. X axis: early (2 days post infection or ~12 of gestation) or late (8 days post infection or day ~18 of pregnancy). Each symbol is a unique tissue representing a distinct mother. Blue, WT tissues; Red, IL-10 KO tissues. Bars indicate median for the sample group. p values generated by Kruskal Wallis testing with Dunn's multiple comparisons test to compare WT vs IL-10 KO late values. Significance set at the p < 0.05 level.
A potential connection between late pregnancy loss (or shortened gestation) and viral load could be related to innate immunity, especially as this connection has been documented in other infections (55). In contrast, the driver of our observations could be related to adaptive immunity. To begin to examine this potential dichotomy, we examined the expression of molecules related to innate vs. adaptive immunity in tissues from WT or IL-10 KO mice. Tissue samples from WT and IL-10 KO mice taken 8 days after infection e.g., day ~18 of gestation were harvested and examined for RNA expression of IL-6 (56, 57), IFN-β (58), and CCL2 (59) which are part of the innate response to viral infection (Figure 4A). Further, we examined IFN-γ (60), IL-17 (61) and TGF-β (60, 62) (Figure 4B) which are considered important for adaptive immunity. At this time point, there was no difference in expression of innate immune mediators (Figure 4A). However, we observed a trend toward increase in IFN-γ in the placenta of IL-10 KO mice and lower TGFβ in both tissues of IL-10 KO as compared to WT mice (Figure 4B).
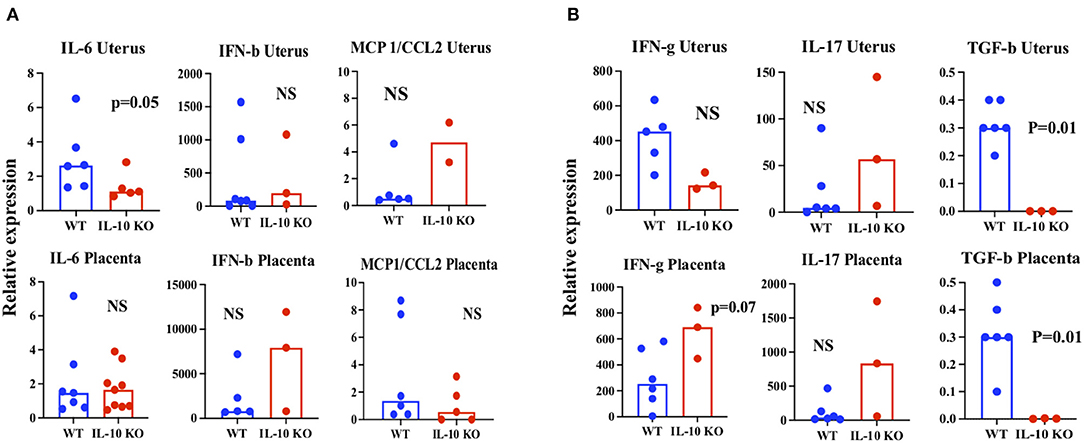
Figure 4. RNA expression of cytokines in WT vs. IL-10 KO tissues. Tissues from WT (blue) or IL-10 KO (red) mice who were infected on ~day 10 of pregnancy and harvested 8 days later were harvested and RNA extracted to determine expression by QT-PCR as in Figure 1. Each symbol denotes a single tissue from a unique mother. (A) “Innate immunity” cytokines IL-6 (left), Interferon-β (middle) and CCL2 (right). (B) “Adaptive immunity” cytokines Interferon–γ, IL-17, Transforming growth factor-β. Upper panels, uterus. Lower panels, placenta. Bars—medians. p values analysis of significance utilized the Mann Whitney test. NS, not significant at the p < 0.05 level. Trends (p < 0.1) are noted.
This finding allowed speculation that the local CD8 T cell response might be different in the tissues of WT and IL-10 KO mice late in infection. To test this, we harvested tissues for examination by flow cytometry. We first analyzed single cell suspensions of tissues to estimate the proportion of relevant cells (Figure 5A and see Supplementary Figure 4A). CD45+ cells were increased in proportion in IL-10 KO uterus as compared to WT (Figure 5A, top row, middle panel), while the proportion of CD8+ cells was equivalent regardless of tissue (Figure 5A, middle row). However, the proportion of CD8+cells that were positive for the activation marker CD44 was higher in both the uterus and placenta of IL-10 KO mice as compared to WT (Figure 5A, bottom row, middle and right panels and see Supplementary Figure 4B, left panel). This suggested that either there was increased trafficking and or local activation of CD8+ cells in IL-10-infected vs. WT- infected utero-placental tissues (Figure 5A).
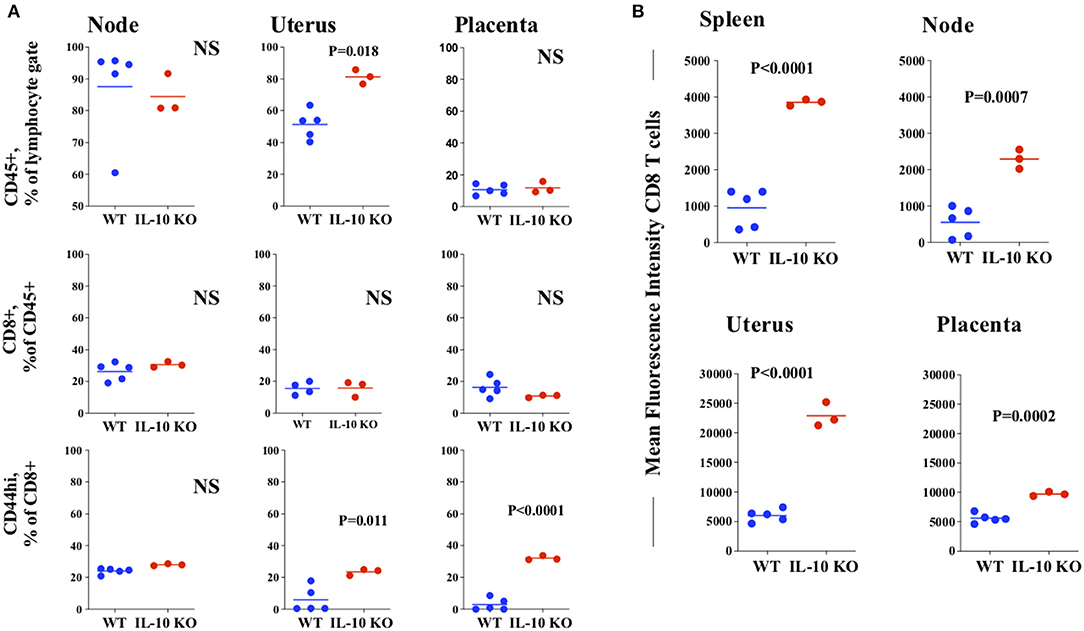
Figure 5. Flow cytometric analysis of tissues from infected normal and IL-10 deficient mice. Single cells suspensions were obtained from tissues harvested from LCMV-infected pregnant WT (blue symbols) and IL-10 KO (red symbols) mice 8 days after infection on ~18 of gestation. Each symbol represents a single tissue from a unique mother. These were stained with antibodies to CD45, CD8, CD44 and PD1 (A) Top panel, percentage of lymphoid gate (by side and forward scatter) cells positive for CD45; Middle panel, percentage of CD45+ cells observed to be CD8+; Bottom panel, percentage of CD8+ cells highly expressing CD44 consistent with activation. (B) Expression of PD1 on CD8+ cells in each of the tissues assayed. Lines, means. p values indicate analysis by t test. NS, not significant at the p < 0.05 level, Node: uterine draining lymph node.
The molecule programmed death-1, PD1/CD279 (22, 63) is expressed on T cells and is associated with regulation of T cell homeostasis, activation and tolerance. Expression is upregulated on activation (64) and expression is associated with modulation of the response to viral infection. LCMV infection with less virulent strains leads to transient upregulation of PD1 and retained functional capacity, while chronic infection with more virulent strains leads to retained PD1 expression as part of the “exhausted” T cell phenotype (65). Based on previous findings of infection with a more virulent strain (28), we expected to see a decreased level of PD1 on CD8 T cells from IL-10 KO mice. Although the size of the systemic CD8 T cell pool is similar in infected WT and IL-10 deficient mice (Figure 5A, middle row, left panel), population-based PD1 expression was increased in IL-10 KO CD8 T cells of both the spleen and uterine draining lymph nodes (Figure 5B, top row, and see Supplementary Figure 4B, right panel) as compared to WT. In addition, the expression of PD1 in both uterine and placental CD8 T cells was elevated in IL-10 deficient tissues compared to WT (Figure 5B, bottom row). Taken together, this data suggests tissue specific regulation of the CD8 T cell pool in the face of viral infection.
Discussion
These studies are driven by an earlier finding that infection with LCMV leads to persistent infection of the uterus and placenta, but not the fetus, despite the fact that the strain used is deemed less virulent and does not usually lead to persistent infection in adult animals (66). We were interested in the role of IL-10 because of its potential role in modulation of both innate and adaptive immunity.
The key findings presented here are the decreased viral load late in infection in IL-10 KO as compared to WT mice, and that IL-10 deficiency in the face of infection leads to comparatively less pregnancy loss and potentially prolonged gestation. Based on previous data in IL-10 deficient mice, and the increased expression of proapoptotic proteins such as BCL2L11 (Figure 2C) the later finding is somewhat counterintuitive. The trend for increased expression of genes such as IL-6 (Figure 4A) is however consistent with developmental dysregulation (57) in the WT pregnancies examined. Although representative of distinct pregnancies, the relatively small sample size in our expression studies demands cautious interpretation. Despite the likelihood that more significant differences might be revealed with a much larger, potentially challenging number of mice, we were able to detect differences consistent with the idea that our findings may be related to differential activity of tissue-localized CD8 T cells.
In studies of neonatal male mice, intracerebral infection with LCMV-Arm, the strain used in these studies, leads to persistent infection as adults, with viral nucleic acid present in several tissues (67). However, in adult mice, infection with LCMV-Arm leads to systemic tissue clearance which occurs in ~ 8 days. This clearance is associated with long-term LCMV- specific immunity and protection against more virulent strains. Key mechanisms of viral persistence in neonatally infected mice include central tolerance, as the virus is persistent in the thymus as well as other tissues and adoptive transfer of T cells from immune mice leads to viral clearance (68).
Viral persistence can be achieved in adult mice through administration of certain clones of LCMV (e.g., clone 13) which, interestingly enough, were isolated from the spleen of mice infected neonatally (66). Viral persistence was initially related to lack of functional cytoxic T cells. Recently it has been observed that strains of LCMV which cause either limited or chronic LCMV infection systemically, alter male urinary scent proteins (69). The effect on urinary scent proteins appears to last longer than it takes for systemic clearance, and this leads to the speculation that the reproductive tract may harbor “non-virulent” virus for a longer than expected (as compared to systemic circulation) time in males as well as pregnant females. This may support extended examination of different viral strains and LCMV specific immunity in the non-pregnant reproductive track of females (70).
The fact that injection of LCMV-Arm may generate different clones of varying immunity (66) raises the possibility that uteroplacental tissues may generate sub-strains with enhanced tissue specific binding or tropism (48). This may lead to clones which cause persistent infection and would thus be termed “virulent.” Further studies are needed to examine the potential presence of altered virus genetic elements, such as those relevant to the L protein (71) in placental tissues.
The existing data may support a model whereby there are three different biologies of LCMV infection during pregnancy. First, infection (more likely early) in pregnancy tends to embryonic loss (20), observed in our studies as “resorption” (Figure 2). Second, infection leads to utero-placental prolonged viral presence at a time during enhanced expression of a putative viral receptor (48). This may lead to labor complications [e.g., dystocia (20)] and increased perinatal mortality. The third, perhaps with infection in late gestation exists along the same continuum as infection in the brain within the first day of life. During which the placental status is not clear, but the fetus becomes chronically infected due to infection of the immature thymus. These three “biologies” might be related to inherent elements of anatomy (72), viral tropism (48) and mechanisms of host defense and innate immunity which may differ by strain [e.g., SWR/J and HA/ICR (10) vs. C57BL/6 (our studies)]. This should be examined in future investigations.
Since IL-10 is expressed in trophoblast, one could expect that it plays a developmental role as do cytokines such as IFN-γ (73) as well as a protective immune regulatory role in pregnancy. Indeed, this is how we interpreted early expression of IL-10 in women who would go on to have preterm birth (44). We posited that these women experienced some dysregulatory insult for which IL-10 expression was enhanced in a compensatory manner. Because of the proposed role of IL-10 in “anti-inflammatory responses” IL-10 has been examined in models of recurrent pregnancy loss and preterm birth. In models of recurrent pregnancy loss, utero-placental deficiency in IL-10 leads to increased loss (38). Moreover, in models of Toll-like receptor mediated preterm birth, deficiency is related to increase in innate immune activation [e.g., NK cells (41, 74)] and a more profound phenotype. However, in the context of LCMV infection, where we would expect that deficiency would lead to enhanced inflammation, we did not observe increased expression of inflammatory cytokines (Figure 4A), an early-expressed interferon (Figures 1A, 4A) or a modulator of innate immune cell trafficking (Figure 4A; Supplementary Figure 3). Further, we did not see overall decreased “resiliency” in uteroplacental tissues of IL-10-KO vs. WT tissues (Figure 2) as pregnancy outcome was improved, and there was no difference in RNA expression of molecules such as NRF2 which should be defense against oxidative stress and FAS which should mediate apoptosis. Our only finding was increased RNA expression of the mitochondrial pro-apoptotic molecule BCL2L11. This may support examination of mitochondrial function and overall metabolism in the context of LCMV infection.
Cell death and tissue destruction in LCMV infection is said to be related to T cell activation and effector function since the virus is considered to be non-lytic (75). However, the correlation between viral load and poor pregnancy outcome we observed could be explained by subtle (e.g., nonlytic), toxic effects of the virus on utero-placental tissues or innate mechanisms not examined. If this would be the case, then viral clearance related to immunity would be expected to be protective. This is consistent with our findings. The ability for pregnant animals to clear systemic LCMV, generate anti LCMV memory, and protect against virulent strains (20) tends to mitigate against both central (e.g., deletion in thymus) and systemic (e.g., global, non-thymic) tolerance as a cause for viral persistence in utero-placental tissues. Moreover, it appears in these studies and those previously published that CD8 T cells can traffic to uteroplacental tissues in response to LCMV infection, although this may be due to dysregulation of mechanisms which limit trafficking to this site (76, 77) in the uninfected case.
Existing data suggests that not only chronic infection, but also homeostatic expansion in response to niche, cytokines such as IL-7, or environmental antigen (78) can lead to T cells with a molecular and phenotypic signature similar to “exhaustion” (78), but that the majority of these cells are cleared via FAS/FAS ligand interactions. We have observed that maternal T cells may undergo homeostatic expansion, possibly in response to fetal antigen (54, 79). This is associated with the increased expression of molecules such as Il-7 receptor and, in the uterus, expression of PD1, granzyme and downregulation of CD8 (49, 54). This suggests a “quasi exhausted” phenotype which can be relieved systemically (80) and possibly locally through inflammation. The factors driving this phenotype may explain the relative persistence of virus in uteroplacental as opposed to systemic tissues.
Chronic LCMV infection is associated with increased IL-10 production in antigen presenting cells and decreased T cell function. Further, antibody mediated blockade of the IL-10 receptor restores T cell function and enhances viral clearance (28). Consistent with this, in our studies, deficiency in IL-10 is associated with decreased viral load in utero-placental tissues and with a calculated increase in the proportion of CD8 T cells (uterus, Figure 5A). This correlated with increased expression of the bona fide activation marker CD44 (81) on uterine and placental CD8 T cells (Figure 5A). We were intrigued by the increased CD8 T cell expression of PD1 in both systemic and local tissues of infected IL-10 KO as compared to WT (Figure 5B), when we expected this molecule to be downregulated in IL-10 KO cells. We were further somewhat surprised by the contrast with CD44, which occurred at a relatively higher level in CD8 T cells of only local IL-10 KO tissues (Figure 5A). We attribute this to the short time-frame of these experiments, as PD1 may be elevated for a time after antigen is cleared and during the revival of exhausted cells [reviewed in (22)]. In addition, we could speculate that genetic deficiency in IL-10 may developmentally alter (upregulate) PD1 even in the uninfected state. PD1 is actually a complex modulator of immunity (82) via interaction with a number of ligands at least one of which, PD-L1 is upregulated by IL-10 (22). PD1 signaling is also closely linked to T cell metabolism (83). As such, the highly metabolically active state of the placenta may influence the cellular expression and function of PD1 and its ligands.
Our data, taken in this context, is consistent with a model for a unique phenotype in uterine/placental tissues compared to systemic CD8 T cells (3, 84), which should in the future be analyzed with respect to exact anatomic site (85) and fetal sex (43). From our point of view (1, 86), these data suggest that in local tissues this may not be a phenotype of inherent suppression or limitation. This state may be one of heightened early activation, which may lead to a state which is like exhaustion, but which can be “cured” by tissue-derived factors when needed to clear viral infections. The concept of a unique intrauterine CD8 T cell phenotype- one that exhibits elements of the “exhaustion-that-can-be-relieved” phenotype is supported by studies in other animal models (87–89) and in humans (90–92). Our hope is that consideration of this concept can be utilized to develop therapeutic interventions to prevent congenital infection.
Data Availability Statement
Requests to access the datasets should be directed to Elizabeth A. Bonney, elizabeth.bonney@med.uvm.edu.
Ethics Statement
The animal study was reviewed and approved by Institutional Animal Care and Use Committee University of Vermont.
Author Contributions
EB prepared manuscript. All authors participated in experiments and data analysis. All authors contributed to the article and approved the submitted version.
Funding
This work was supported by NIH RO1HD047224 and NIHP20 RR021905 (the Vermont Center for Immunology and Infectious Diseases) and the University of Vermont, Department of Obstetrics, Gynecology and Reproductive Sciences.
Conflict of Interest
The authors declare that the research was conducted in the absence of any commercial or financial relationships that could be construed as a potential conflict of interest.
Publisher's Note
All claims expressed in this article are solely those of the authors and do not necessarily represent those of their affiliated organizations, or those of the publisher, the editors and the reviewers. Any product that may be evaluated in this article, or claim that may be made by its manufacturer, is not guaranteed or endorsed by the publisher.
Acknowledgments
We thank Koela Ray and Karen Oppenheimer for technical assistance. Studies reported here made use of the UVM-COM Flow Cytometry and Cell Sorting Facility.
Supplementary Material
The Supplementary Material for this article can be found online at: https://www.frontiersin.org/articles/10.3389/fviro.2022.829991/full#supplementary-material
References
1. Bonney EA. Alternative theories: pregnancy and immune tolerance. J Reprod Immunol. (2017) 123:65–71. doi: 10.1016/j.jri.2017.09.005
2. Gamliel M, Goldman-Wohl D, Isaacson B, Gur C, Stein N, Yamin R, et al. Trained memory of human uterine NK cells enhances their function in subsequent pregnancies. Immunity. (2018) 48: 951-962.e955. doi: 10.1016/j.immuni.2018.03.030
3. van der Zwan A, Bi K, Norwitz ER, Crespo  C, Claas FHJ, Strominger JL, et al. Mixed signature of activation and dysfunction allows human decidual CD8(+) T cells to provide both tolerance and immunity. Proc Natl Acad Sci USA. (2018) 115:385–90. doi: 10.1073/pnas.1713957115
4. Hu XH Li ZH, Muyayalo KP, Wang LL, Liu CY, Mor G, et al. A newly intervention strategy in preeclampsia: Targeting PD-1/Tim-3 signaling pathways to modulate the polarization of decidual macrophages. FASEB J. (2022) 36:e22073. doi: 10.1096/fj.202101306R
5. Tilburgs T, Strominger JL. CD8+ effector T cells at the fetal-maternal interface, balancing fetal tolerance and antiviral immunity. Am J Reprod Immunol. (2013) 69:395–407. doi: 10.1111/aji.12094
6. Crespo  C, van der Zwan A, Ramalho-Santos J, Strominger JL, Tilburgs T. Cytotoxic potential of decidual NK cells and CD8+ T cells awakened by infections. J Reprod Immunol. (2017) 119:85–90. doi: 10.1016/j.jri.2016.08.001
7. Jahrling PB, Peters CJ. Lymphocytic choriomeningitis virus: a neglected pathogen of man. Arch Pathol Lab Med. (1992) 116:486–8.
8. Jamieson DJ, Kourtis AP, Bell M, Rasmussen SA. Lymphocytic choriomeningitis virus: An emerging obstetric pathogen? Am J Obstet Gynecol. (2006) 194:1532–6. doi: 10.1016/j.ajog.2005.11.040
9. Bowen GS, Calisher CH, Winkler WG, Kraus AL, Fowler EH, Garman RH, et al. Laboratory studies of a lymphocytic choriomeningitis virus outbreak in man and laboratory animals. Am J Epidemiol. (1975) 102:233–40. doi: 10.1093/oxfordjournals.aje.a112152
10. Oldstone MB, Dixon FJ. Disease accompanying in utero viral infection. The role of maternal antibody in tissue injury after transplacental infection with lymphocytic choriomeningitis virus. J Exp Med. (1972) 135:827–38. doi: 10.1084/jem.135.4.827
11. Barton LL, Peters CJ, Ksiazek TG. Lymphocytic choriomeningitis virus: an unrecognized teratogenic pathogen. Emerg Infect Dis. (1995) 1:152–153. doi: 10.3201/eid0104.950410
12. Barton LL, Mets MB. Congenital lymphocytic chroiomeningitis virus infection: decade of rediscovery. Clin Infect Dis. (2001) 33:370–4. doi: 10.1086/321897
13. Sheinbergas MM. Antibody to lymphocytic choriomeningitis virus in children with congenital hydrocephalus. Acta Virol. (1975) 19:165–6.
14. Fischer SA, Graham MB, Kuehnert MJ, Kotton CN, Srinivasan A, Marty FM, et al. Transmission of lymphocytic choriomeningitis virus by organ transplantation. N Engl J Med. (2006) 354:2235–49. doi: 10.1056/NEJMoa053240
15. Macneil A, Stroher U, Farnon E, Campbell S, Cannon D, Paddock CD, et al. Solid organ transplant-associated lymphocytic choriomeningitis, United States, 2011. Emerg Infect Dis. (2012) 18:1256–62. doi: 10.3201/eid1808.120212
16. Vilibic-Cavlek T, Oreski T, Korva M, Kolaric B, Stevanovic V, Zidovec-Lepej S, et al. Prevalence and risk factors for lymphocytic choriomeningitis virus infection in continental Croatian regions. Trop Med Infect Dis. (2021) 6:67. doi: 10.3390/tropicalmed6020067
17. Murali-Krishna K, Altman J, Suresh M, Sourdive DJ, Zajac AJ, Miller JD, et al. Counting antigen-specific CD8 T cells: a reevaluation of bystander activation during viral infection. Immunity. (1998) 8:1–20. doi: 10.1016/S1074-7613(00)80470-7
18. Kaech SM, Tan JT, Wherry EJ, Konieczny BT, Surh CD, Ahmed R. Selective expression of the interleukin 7 receptor identifies effector CD8 T cells that give rise to long-lived memory cells. Nat Immunol. (2003) 4:1191–8. doi: 10.1038/ni1009
19. Mothe BR, Stewart BS, Oseroff C, Bui H-H, Stogiera S, Garcia Z, et al. Chronic lymphocytic choriomeningitis virus infection actively down-regulates CD4+ T cell responses directed against a broad range of epitopes. J Immunol. (2007) 179:1058–67. doi: 10.4049/jimmunol.179.2.1058
20. Constantin CM, Masopust D, Gourley T, Grayson J, Strickland OL, Ahmed R, et al. Normal establishment of virus-specific memory CD8 T cell pool following primary infection during pregnancy. J Immunol. (2007) 179:4383–9. doi: 10.4049/jimmunol.179.7.4383
21. Asseman C, Mauze S, Leach MW, Coffman RL, Powrie F. An essential role for interleukin 10 in the function of regulatory cells that inhibit intestinal inflammation. J Exp Med. (1999) 190:995–1003. doi: 10.1084/jem.190.7.995
22. Sharpe AH, Pauken KE. The diverse functions of the PD1 inhibitory pathway. Nat Rev Immunol. (2018) 18:153–67. doi: 10.1038/nri.2017.108
23. Antoniv TT, Park-Min KH, Ivashkiv LB. Kinetics of IL-10-induced gene expression in human macrophages. Immunobiology. (2005) 210:87–95. doi: 10.1016/j.imbio.2005.05.003
24. Ng CT, Oldstone MB. Infected CD8alpha- dendritic cells are the predominant source of IL-10 during establishment of persistent viral infection. Proc Natl Acad Sci USA. (2012) 109:14116–21. doi: 10.1073/pnas.1211910109
25. Georgescu L, Vakkalanka RK, Elkon KB, Crow MK. Interleukin-10 promotes activation-induced cell death of SLE lymphocytes mediated by Fas ligand. J Clin Investig. (1997) 100:2622–33. doi: 10.1172/JCI119806
26. Hagenbaugh A, Sharma S, Dubinett SM, Wei SHY, Aranda R, Cheroutre H, et al. Altered immune responses in interleukin 10 transgenic mice. J Exp Med. (1997) 185:2101–10. doi: 10.1084/jem.185.12.2101
27. Montenegro SML, Miranda P, Mahanty S, Abath FGC, Teixeira KM, Coutinho EM, et al. Cytokine production in acute versus chronic human schistosomiasis mansoni: the cross-regulatory role of interferon-γ and interleukin-10 in the responses of peripheral blood mononuclear cells and splenocytes to parasite antigens. J Infect Dis. (1999) 179:1502–14. doi: 10.1086/314748
28. Brooks DG, Trifilo MJ, Edelmann KH, Teyton L, McGavern DB, Oldstone MB. Interleukin-10 determines viral clearance or persistence in vivo. Nat Med. (2006) 12:1301–9. doi: 10.1038/nm1492
29. Moore KW, Viera P, Florentino DF, Trounstine ML, Khan TA, Mosmann TR. Homology of the cytokine synthesis inhibatory factor (IL-10) to to the Epstein Barr Virus gene BCRF1. Science. (1990) 248:1230–4. doi: 10.1126/science.2161559
30. Filippi CM, von Herrath MG. IL-10 and the resolution of infections. J Pathol. (2008) 214:224–30. doi: 10.1002/path.2272
31. Ejrnaes M, Filippi CM, Martinic MM, Ling EM, Togher LM, Crotty S, et al. Resolution of a chronic viral infection after interleukin-10 receptor blockade. J Exp Med. (2006) 203:2461–72. doi: 10.1084/jem.20061462
32. Richter K, Perriard G, Behrendt R, Schwendener RA, Sexl V, Dunn R, et al. Macrophage and T cell produced IL-10 promotes viral chronicity. PLoS Pathog. (2013) 9:e1003735. doi: 10.1371/journal.ppat.1003735
33. Wang X, Zhao H, Yang N, Jin Y, Chen J. Antiangiogenic effect of platelet P2Y(12) inhibitor in ischemia-induced angiogenesis in mice hindlimb. Biomed Res Int. (2021) 2021:5529431. doi: 10.1155/2021/5529431
34. Cheng SB, Sharma S. Interleukin-10: a pleiotropic regulator in pregnancy. Am J Reprod Immunol. (2015) 73:487–500. doi: 10.1111/aji.12329
35. Aschkenazi S, Straszewski S, Verwer KM, Foelmer H, Rutherford T, Mor G. Differential regulation and function of the Fas/Fas ligand system in human trophoblast cells. Biol Reprod. (2002) 66:1853–61. doi: 10.1095/biolreprod66.6.1853
36. Roth I, Fisher SJ. IL-10 is an autocrine inhibitor of human placental cytotrophoblast MMP-9 production and invasion. Dev Biol. (1999) 205:194–204. doi: 10.1006/dbio.1998.9122
37. Dudley DJ, Edwin SS, Dangerfield A, Jackson K, Trautman MS. Regulation of decidual cell and chorion cell production of interleukin-10 by purified bacterial products. Am J Reprod Immunol. (1997) 38:246–51. doi: 10.1111/j.1600-0897.1997.tb00510.x
38. Chaouat G, Meliani AA, Martal J, Raghupathy R, Eliot J, Mosmann T, et al. IL-10 prevents naturally occuring fetal loss in the CBAxDBA/2 mating combination, and local defect in IL-10 production in the abortion-prone combination is corrected by in vivo injection of IFN-t. J Immunol. (1995) 154:4261–8.
39. Schumacher A, Wafula PO, Bertoja AZ, Sollwedel A, Thuere C, Wollenberg I, et al. Mechanisms of action of regulatory T cells specific for paternal antigens during pregnancy. Obstetr Gynecol. (2007) 110:1137–45. doi: 10.1097/01.AOG.0000284625.10175.31
40. Lee SK, Na BJ, Kim JY, Hur SE, Lee M, Gilman-Sachs A, et al. Determination of clinical cellular immune markers in women with recurrent pregnancy loss. Am J Reprod Immunol. (2013) 70:398–411. doi: 10.1111/aji.12137
41. Murphy SP, Hanna NN, Fast LD, Shaw SK, Berg G, Padbury JF, et al. Evidence for participation of uterine natural killer cells in the mechanisms responsible for spontaneous preterm labor and delivery. Am J Obstetr Gynecol. (2009) 200:308–9. doi: 10.1016/j.ajog.2008.10.043
42. Robertson SA, Skinner RJ, Care AS. Essential role for IL-10 in resistance to lipopolysaccharide-induced preterm labor in mice. J Immunol. (2006) 177:4888–96. doi: 10.4049/jimmunol.177.7.4888
43. Na Q, Chudnovets A, Liu J, Lee JY, Dong J, Shin N, et al. Placental macrophages demonstrate sex-specific response to intrauterine inflammation and may serve as a marker of perinatal neuroinflammation. J Reprod Immunol. (2021) 147:103360. doi: 10.1016/j.jri.2021.103360
44. Pearce BD, Grove J, Bonney EA, Bliwise N, Dudley DJ, Schendel DE, et al. Interrelationship of cytokines, hypothalamic-pituitary-adrenal axis hormones, and psychosocial variables in the prediction of preterm birth. Gynecol Obstet Invest. (2010) 70:40–6. doi: 10.1159/000284949
45. Keenan-Devlin LS, Caplan M, Freedman A, Kuchta K, Grobman W, Buss C, et al. Using principal component analysis to examine associations of early pregnancy inflammatory biomarker profiles and adverse birth outcomes. Am J Reprod Immunol. (2021) 86:e13497. doi: 10.1111/aji.13497
46. Sarr D, Aldebert D, Marrama L, Frealle E, Gaye A, Brahim HO, et al. Chronic infection during placental malaria is associated with up-regulation of cycloxygenase-2. Malar J. (2010) 9:45. doi: 10.1186/1475-2875-9-45
47. Bonney EA, Krebs K, Kim J, Prakash K, Torrance BL, Haynes L, et al. Protective intranasal immunization against influenza virus in infant mice is dependent on IL-6. Front Immunol. (2020) 11:568978. doi: 10.3389/fimmu.2020.568978
48. Santhanakrishnan M, Ray K, Oppenheimer K, Bonney EA. Dynamic regulation of alpha-dystroglycan in mouse placenta. Placenta. (2008) 29:932–6. doi: 10.1016/j.placenta.2008.08.021
49. Shepard MT, Bonney EA. PD-1 regulates T cell proliferation in a tissue and subset-specific manner during normal mouse pregnancy. Immunol Invest. (2013) 42:385–408. doi: 10.3109/08820139.2013.782317
50. Murray SA, Morgan JL, Kane C, Sharma Y, Heffner CS, Lake J, et al. Mouse gestation length is genetically determined. PLoS ONE [Electronic Resource]. (2010) 5:e12418. doi: 10.1371/journal.pone.0012418
51. Menon R, Bonney EA, Condon J, Mesiano S, Taylor RN. Novel concepts on pregnancy clocks and alarms: redundancy and synergy in human parturition. Hum Reprod Update. (2016) 22:535–60. doi: 10.1093/humupd/dmw022
52. Tantengco OAG, de Castro Silva M, Shahin H, Bento GFC, Cursino GC, Cayenne S, et al. The role of nuclear factor erythroid 2-related factor 2 (NRF2) in normal and pathological pregnancy: a systematic review. Am J Reprod Immunol. (2021) 86:e13496. doi: 10.1111/aji.13496
53. Luo S, Rubinsztein DC. BCL2L11/BIM: a novel molecular link between autophagy and apoptosis. Autophagy. (2013) 9:104–5. doi: 10.4161/auto.22399
54. Norton MT, Fortner KA, Oppenheimer KH, Bonney EA. Evidence that CD8 T-cell homeostasis and function remain intact during murine pregnancy. Immunology. (2010) 131:426–37. doi: 10.1111/j.1365-2567.2010.03316.x
55. Racicot K, Kwon JY, Aldo P, Abrahams V, El-Guindy A, Romero R, et al. Type I interferon regulates the placental inflammatory response to bacteria and is targeted by virus: mechanism of polymicrobial infection-induced preterm birth. Am J Reprod Immunol. (2016) 75:451–60. doi: 10.1111/aji.12501
56. Rincon M. Interleukin-6: from an inflammatory marker to a target for inflammatory diseases. Trends Immunol. (2012) 33:571–7. doi: 10.1016/j.it.2012.07.003
57. Omere C, Richardson L, Saade GR, Bonney EA, Kechichian T, Menon R. Interleukin (IL)-6: a friend or foe of pregnancy and parturition? Evidence from functional studies in fetal membrane cells. Front Physiol. (2020) 11:891. doi: 10.3389/fphys.2020.00891
58. Racicot K, Aldo P, El-Guindy A, Kwon JY, Romero R, Mor G. Cutting edge: fetal/placental type I IFN can affect maternal survival and fetal viral load during viral infection. J Immunol. (2017) 198:3029–32. doi: 10.4049/jimmunol.1601824
59. Gajewski T, Rouhani S, Trujillo J, Pyzer A, Yu J, Fessler J, et al. Severe COVID-19 infection is associated with aberrant cytokine production by infected lung epithelial cells rather than by systemic immune dysfunction. Res Sq. (2021). doi: 10.21203/rs.3.rs-1083825/v1
60. Hosking MP, Flynn CT, Whitton JL. TCR independent suppression of CD8(+) T cell cytokine production mediated by IFNγ in vivo. Virology. (2016) 498:69–81. doi: 10.1016/j.virol.2016.08.003
61. Jie Z, Liang Y, Hou L, Dong C, Iwakura Y, Soong L, et al. Intrahepatic innate lymphoid cells secrete IL-17A and IL-17F that are crucial for T cell priming in viral infection. J Immunol. (2014) 192:3289–300. doi: 10.4049/jimmunol.1303281
62. Tinoco R, Alcalde V, Yang Y, Sauer K, Zuniga EI. Cell-intrinsic transforming growth factor-beta signaling mediates virus-specific CD8+ T cell deletion and viral persistence in vivo. Immunity. (2009) 31:145–57. doi: 10.1016/j.immuni.2009.06.015
63. Riley JL. PD-1 signaling in primary T cells. Immunol Rev. (2009) 229:114–25. doi: 10.1111/j.1600-065X.2009.00767.x
64. Agata Y, Kawasaki A, Nishimura H, Ishida Y, Tsubata T, Yagita H, et al. Expression of the PD-1 antigen on the surface of stimulated mouse T and B lymphocytes. Int Immunol. (1996) 8:765–72. doi: 10.1093/intimm/8.5.765
65. Wherry EJ, Ha SJ, Kaech SM, Haining WN, Sarkar S, Kalia V, et al. Molecular signature of CD8+ T cell exhaustion during chronic viral infection [Erratum appears in Immunity. 2007 Nov; 27(5):824]. Immunity. (2007) 27:670–84. doi: 10.1016/j.immuni.2007.11.006
66. Ahmed R, Oldstone MB. Organ-specific selection of viral variants during chronic infection. J Exp Med. (1988) 167:1719–24. doi: 10.1084/jem.167.5.1719
67. Fazakerley JK, Southern P, Bloom F, Buchmeier MJ. High resolution in situ hybridization to determine the cellular distribution of lymphocytic choriomeningitis virus RNA in the tissues of persistently infected mice: relevance to arenavirus disease and mechanisms of viral persistence. J Gen Virol. (1991) 72:1611–25. doi: 10.1099/0022-1317-72-7-1611
68. Jamieson BD, Somasundaram T, Ahmed R. Abrogation of tolerance to a chronic viral infection. J Immunol. (1991) 147:3521–9.
69. Oldstone MBA, Ware BC, Davidson A, Prescott MC, Beynon RJ, Hurst JL. Lymphocytic choriomeningitis virus alters the expression of male mouse scent proteins. Viruses. (2021) 13:1180. doi: 10.3390/v13061180
70. Suvas PK, Dech HM, Sambira F, Zeng J, Onami TM. Systemic and mucosal infection program protective memory CD8 T cells in the vaginal mucosa. J Immunol. (2007) 179:8122–7. doi: 10.4049/jimmunol.179.12.8122
71. Salvato M, Borrow P, Shimomaye E, Oldstone MB. Molecular basis of viral persistence: a single amino acid change in the glycoprotein of lymphocytic choriomeningitis virus is associated with suppression of the antiviral cytotoxic T-lymphocyte response and establishment of persistence. J Virol. (1991) 65:1863–9. doi: 10.1128/jvi.65.4.1863-1869.1991
72. Mims CA. Effect on the fetus of maternal infection with lymphocytic choriomeningitis (LCM) virus. J Infect Dis. (1969) 120:582–97. doi: 10.1093/infdis/120.5.582
73. Ashkar AA, Di Santo JP, Croy BA. Interferon gamma contributes to initiation of uterine vascular modification, decidual integrity, and uterine natural killer cell maturation during normal murine pregnancy. J Exp Med. (2000) 192:259–70. doi: 10.1084/jem.192.2.259
74. Murphy SP, Fast LD, Hanna NN, Sharma S. Uterine NK cells mediate inflammation-induced fetal demise in IL-10-null mice. Journal of Immunology. (2005) 175:4084–90. doi: 10.4049/jimmunol.175.6.4084
75. Buchmeier MJ, Welsh RM, Dutko FJ, Oldstone MB. The virology and immunobiology of lymphocytic choriomeningitis virus infection. Adv Immunol. (1980) 30:275–331. doi: 10.1016/S0065-2776(08)60197-2
76. Fernekorn U, Butcher EC, Behrends J, Hartz S, Kruse A. Functional involvement of P-selectin and MAdCAM-1 in the recruitment of alpha4beta7-integrin-expressing monocyte-like cells to the pregnant mouse uterus. Eur J Immunol. (2004) 34:3423–33. doi: 10.1002/eji.200425223
77. Nancy P, Tagliani E, Tay CS, Asp P, Levy DE, Erlebacher A. Chemokine gene silencing in decidual stromal cells limits T cell access to the maternal-fetal interface. Science. (2012) 336:1317–21. doi: 10.1126/science.1220030
78. Fortner KA, Bond JP, Austin JW, Boss JM, Budd RC. The molecular signature of murine T cell homeostatic proliferation reveals both inflammatory and immune inhibition patterns. J Autoimmun. (2017) 82:47–61. doi: 10.1016/j.jaut.2017.05.003
79. Norton MT, Fortner KA, Bizargity P, Bonney EA. Pregnancy alters the proliferation and apoptosis of mouse splenic erythroid lineage cells and leukocytes. Biol Reprod. (2009) 81:457–64. doi: 10.1095/biolreprod.109.076976
80. Bonney EA, Matzinger P. The maternal immune system's interaction with circulating fetal cells. J Immunol. (1997) 158:40–7.
81. DeGrendele HC, Kosfiszer M, Estess P, Siegelman MH. CD44 activation and associated primary adhesion is inducible via T cell receptor stimulation. J Immunol. (1997) 159:2549–53.
82. Zhang JY, Zhang Z, Jin B, Zhang SY, Zhou CB, Fu JL, et al. Cutting edge: programmed death-1 up-regulation is involved in the attrition of cytomegalovirus-specific CD8+ T cells in acute self-limited hepatitis B virus infection. J Immunol. (2008) 181:3741–4. doi: 10.4049/jimmunol.181.6.3741
83. Kalia V, Yuzefpolskiy Y, Vegaraju A, Xiao H, Baumann F, Jatav S, et al. Metabolic regulation by PD-1 signaling promotes long-lived quiescent CD8 T cell memory in mice. Sci Transl Med. (2021) 13:eaba6006. doi: 10.1126/scitranslmed.aba6006
84. Tilburgs T, Claas FH, Scherjon SA. Elsevier Trophoblast Research Award Lecture: unique properties of decidual T cells and their role in immune regulation during human pregnancy. Placenta. (2010) 31 Suppl:S82–86. doi: 10.1016/j.placenta.2010.01.007
85. Sojka DK, Yang L, Plougastel-Douglas B, Higuchi DA, Croy BA, Yokoyama WM. Cutting edge: local proliferation of uterine tissue-resident NK cells during decidualization in mice. J Immunol. (2018) 201:2551–6. doi: 10.4049/jimmunol.1800651
86. Bonney EA, Johnson MR. The role of maternal T cell and macrophage activation in preterm birth: cause or consequence? Placenta. (2019) 79:53–61. doi: 10.1016/j.placenta.2019.03.003
87. Moström MJ, Scheef EA, Sprehe LM, Szeltner D, Tran D, Hennebold JD, et al. Immune profile of the normal maternal-fetal interface in rhesus macaques and its alteration following zika virus infection. Front Immunol. (2021) 12:719810. doi: 10.3389/fimmu.2021.719810
88. McLendon BA, Seo H, Kramer AC, Burghardt RC, Bazer FW, Johnson GA. Pig conceptuses secrete interferon gamma to recruit T cells to the endometrium during the peri-implantation period†. Biol Reprod. (2020) 103:1018–29. doi: 10.1093/biolre/ioaa132
89. Vasudevan S, Kamat MM, Walusimbi SS, Pate JL, Ott TL. Effects of early pregnancy on uterine lymphocytes and endometrial expression of immune-regulatory molecules in dairy heifers. Biol Reprod. (2017) 97:104–18. doi: 10.1093/biolre/iox061
90. Tilburgs T, Scherjon SA, Roelen DL, Claas FH. Decidual CD8+CD28- T cells express CD103 but not perforin. Hum Immunol. (2009) 70:96–100. doi: 10.1016/j.humimm.2008.12.006
91. Slutsky R, Romero R, Xu Y, Galaz J, Miller D, Done B, et al. Exhausted and senescent T cells at the maternal-fetal interface in preterm and term labor. J Immunol Res. (2019) 2019:3128010. doi: 10.1155/2019/3128010
Keywords: CD8 T cells, cytokines, pregnancy, lymphocytic choriomeningitis (LCMV), mouse
Citation: Negi VD, Khurana S and Bonney EA (2022) Interleukin-10 Delays Viral Clearance in the Placenta and Uterus of Mice With Acute Lymphocytic Choriomeningitis Virus Infection During Pregnancy. Front. Virol. 2:829991. doi: 10.3389/fviro.2022.829991
Received: 06 December 2021; Accepted: 18 January 2022;
Published: 15 February 2022.
Edited by:
Vikki M. Abrahams, Yale University, United StatesReviewed by:
Dorothy K. Sojka, Loyola University Chicago, United StatesElizabeth Ann Lieser Enninga, Mayo Clinic, United States
Copyright © 2022 Negi, Khurana and Bonney. This is an open-access article distributed under the terms of the Creative Commons Attribution License (CC BY). The use, distribution or reproduction in other forums is permitted, provided the original author(s) and the copyright owner(s) are credited and that the original publication in this journal is cited, in accordance with accepted academic practice. No use, distribution or reproduction is permitted which does not comply with these terms.
*Correspondence: Elizabeth A. Bonney, elizabeth.bonney@med.uvm.edu