- Instituto de Medicina Molecular João Lobo Antunes, Faculdade de Medicina, Universidade de Lisboa, Lisbon, Portugal
Human Endogenous Retroviruses (HERVs) constitute up to 8% of the human genome and have been emerging as important modulators of the immune system, being associated with cancer, autoimmunity and infectious diseases. Here, we investigated the expression of three HERV families in the human thymus. HERV-K, -W, and -R envelope (env) and HERV-K gag transcriptional levels were quantified in the main thymocyte subsets, thymic epithelial cells (TECs), B cells and myeloid populations, and Env protein expression was studied in thymic tissue. We found that HERV mRNA decreased with T cell development, which was in agreement with the identification of HERV-K Env protein in CD3 negative cortical cells. These results suggest a distinct regulation of HERV expression along T cell development, prompting us to evaluate the interplay with host restriction factors and potential underlying pathways. The transcriptional levels of some HERVs were found to positively correlate with the expression of the host restriction factors APOBEC3G and SLFN11, and, conversely, a negative correlation was found with SAMHD1. Moreover, IFN-α and IFN-γ induced the upregulation of HERV-K env and gag in purified CD4 single-positive thymocytes. Additionally, we found high levels of HERV mRNAs in TECs. Overall, our data support a tight regulation of HERV expression during human T cell development, with possible implications for the process of T cell selection.
Introduction
The thymus is the specialized primary lymphoid organ that supports the development of T cells. This is a tightly controlled process allowing the generation of cells featuring a diverse receptor repertoire and potentially able to respond to any antigen with controlled auto-reactivity (1). It relies on proper antigen presentation through many layers of regulation involving several cell types and proliferative/survival pathways (2, 3). Perturbations in those processes impact T cell homeostasis and favor autoimmunity (4).
The control of gene expression is a main determinant of T cell selection, as illustrated by several genes uniquely regulating these processes in thymic epithelial cells, such as AIRE (5–7). Therefore, human endogenous retroviruses (HERVs), which represent close to 8% of the human genome, are likely to be important players in the thymus. HERVs are known to be expressed in stem cells, including hematopoietic stem cells, and have been shown to play a role in lineage commitment (8, 9). Moreover, several HERV families have been shown to be expressed in the human thymus (10–14). The association of HERV expression with the development of autoimmune diseases has been suggested to be also linked to T cell selection in the thymus (8, 15–17), but there are no detailed studies on HERV expression throughout human thymocyte development as well as on thymic stromal cells.
Recent data from several cell types and tissues have also been highlighting host restriction factors that limit HERV expression (9), and the interplay with other viruses, including HIV and SARS-CoV-2 (7, 18–22). It is likely that the interplay between HERV expression and the activation of the cell-intrinsic pathways that detect cytoplasmic DNA and/or RNA may play a role in the response to viral infections, as well as in the human thymus, including possible modulation during cell selection (10).
We provide here a comprehensive evaluation of the expression of 3 major HERV families, HERV-K, -W, and –R, along thymocyte differentiation and in the other main cell populations in the human thymus, in parallel with the assessment of host restriction factors. Additionally, we investigated whether stimulation, cytokine exposure and HIV infection impact on HERV expression in human thymocytes.
Methods
Ethical Statement
Thymic specimens were obtained from routine thymectomy performed during pediatric (newborns to 4-year-old children) corrective cardiac surgery at Hospital de Santa Cruz, Carnaxide, Portugal. Cord blood was collected at the Obstetric Department of Hospital de Santa Maria, Lisbon, Portugal. Pediatric tonsils were obtained from routine pediatric tonsillectomy at the Centro Hospitalar Universitário Lisboa Norte (CHULN), Portugal. Adult peripheral blood was collected from volunteer healthy donors at Instituto de Medicina Molecular João Lobo Antunes, Lisbon, Portugal. All samples were collected after participants or parent's/legal guardians' written informed consent. The study was approved by the Ethical Boards of Faculty of Medicine of the University of Lisbon, of the CHULN and of the Hospital de Santa Cruz, Portugal.
Sample Processing
Total thymocytes and tonsillar cells were recovered by tissue dispersion using a 70μM filter (BD Biosciences). Thymocytes, tonsillar, cord blood and peripheral blood mononuclear cells were obtained through separation on Ficoll-Paque PLUS (GE Healthcare) density gradient.
Flow Cytometry
Surface staining was performed for 20 min at room temperature and always included Fixable Viability Dye (eBioscience) for dead cell exclusion. Cells were fixed, permeabilized and stained using an intracellular staining kit (eBioscience), as described (23, 24). Anti-human monoclonal antibodies (mAb) used were (clones in brackets): CD3 (UCHT1 or OKT3), CD4 (RPA-T4), CD8α (RPA-T8), CD11c (3.9), CD14 (61D3), CD16 (eBioCB16), CD19 (HIB19), CD20 (2H7), CD34 (4H11), CD45 (HI30), CD45RA (HI100), CD45RO (UCHL1), CD56 (MEM188), CD163 (eBioGH1/61), CD123 (6H6), CCR7 (150503), CXCR5 (51505), BDCA-3 (LS17-9), EpCAM (1B7), HLA-DR (G46-6), PD1 (MIH4) and TCRγδ (B1.1) from eBioscience. Cells were acquired using LSRFortessa (BD Biosciences) and data was analyzed in FlowJo (FlowJo).
Cell Sorting
Total thymocytes, cord blood or adult PBMCs, and mononuclear tonsillar cells were centrifuged and incubated at 4°C in the dark for 30 min with the antibodies listed above. After washing, cells were sorted to a high degree of purity (routinely >98%) using a FACSAria (BD Biosciences). Total thymocytes were sorted according to human αβ T-cell developmental stages: triple-negative (TN) CD34+ (Lineage-CD4-CD8-CD3-CD34+), CD4ISP (CD4+CD8-CD3-), double-positive (DP - CD4+CD8+CD3-/+), CD4SP (CD4+CD8-CD3+) and CD8SP (CD4-CD8+CD3+). Lineage=CD14, CD16, CD19, CD20, CD56, CD123, CD11c, TCRγδ. The following thymic stromal populations were also sorted: B cells (CD3-CD19+DR+), monocytes/macrophages (CD3-CD163+), plasmacytoid dendritic cells (pDCs - CD3-CD8-DR+CD123+), CD11c+ myeloid dendritic cells (mDCs - CD3-CD8-DR+CD123-BDCA3-CD11c+), BDCA-3+ mDCs (CD3-CD8-DR+CD123-BDCA3+), thymic epithelial cells (TECs - CD3-CD8-CD45-EpCAM+) and TCRγδ T cells (CD3+TCRγδ+). Cord blood cells were sorted as CD34+. Adult peripheral blood T cells were sorted into CD4 (CD3+CD4+CD8-) and CD8 (CD3+CD4-CD8+) T cells, as well as naïve (CCR7+CD45RA+) and effector plus central memory (CCR7-CD45RA-, CCR7+CD45RA- and CCR7-CD45RA+) T cell subsets. Memory CD4 (CD4+CD45RO+) pediatric mononuclear tonsillar cells were sorted according to the T follicular helper differentiation stages: PD1-CXCR5-, PD1lowCXCR5-, PD1lowCXCR5+, PD1brightCXCR5bright.
HERV and HRF Quantification
Total RNA was extracted using ZR-Duet DNA/RNA MiniPrep (Zymo Research), according to the manufacturer's guidelines. RNA from all samples was treated with DNase I (Sigma-Aldrich) to remove any genomic DNA contamination. The purified RNA was quantified using NanoDrop™ 1,000 Spectrophotometer (Thermo Scientific) and 50–200 ng of RNA were used to synthesize complementary DNA (cDNA) using oligo (dT)20 or random primers (300 ng/μL; Invitrogen) and Superscript III reverse transcriptase (Invitrogen). mRNA levels were determined by Real-Time RT-PCR using the appropriate set of primers (Table 1) and Power SYBR Green PCR Master mix (Applied Biosystems) on a 7500 Fast or the ViiA™ 7 Real-Time PCR System (both from Applied Biosystems). Relative mRNA levels, normalized to β-actin, were calculated using the 2-ΔCT or 2-ΔΔCT method.
Immunohistochemistry
Fixed thymic tissue was embedded in paraffin and cut into 3 μm sections (Minot Microtome Leica RM2145). Epitope-retrieval was performed at pH9 (Leica Biosystems buffer) for 15 min using a microwave (800W). Sections were stained with antibodies against HERV-K Env TM (Austral Biologicals, HERM-18115), APOBEC3G (NIH AIDS Reagents Program), SAMHD1 (Sigma-Aldrich, SAB1101454), and SLFN11 (Sigma-Aldrich, HPA023030). Samples were then incubated with a peroxidase/DAB detection system (EnVision, Dako) and counterstained with Harris' hematoxylin (BioOptica). Images were acquired using a Leica DM2500 microscope.
Immunofluorescence Staining
After protein blocking, 3 μm tissue sections were stained by sequential incubations with the above mentioned anti-HERV-K Env antibody and anti-CD3 (eBioscience, OKT3) antibody and secondary antibodies as previously described (23, 24), and DAPI was used for nuclear counterstaining. Images were acquired with Zeiss LSM 710 confocal microscope (Carl Zeiss, Oberkochen, Germany) using a EC Plan-Neofluar 40x/1.30 objective; Digital zoom inserts acquired with ZEN 2012 SP5 FP3 (black) software.
Thymocyte Stimulation
FACS-sorted CD4SP T cells (200.000–500.000 cells/condition) were stimulated in a 96 well U-bottom tissue culture plate at 37°C in the presence of complete medium alone or supplemented with Interleukin-2 (IL-2; 100 U/mL; R&D), IL-4 (100 ng/mL; R&D), IL-7 (50 ng/mL; R&D), Tumor Necrosis Factor-α (TNF-α, 20 ng/mL; eBiosciences), Interferon-α (IFNα, 50 ng/mL; eBiosciences), IFN-γ (66 ng/mL; BD Biosciences), PHA (5 μg/mL; Sigma-Aldrich), or PMA (500 ng/mL; Sigma-Aldrich) + Ionomycin (500 ng/mL; Calbiochem, Merck Biosciences, Nottingham, UK) for 4 or 23 h. After culture, cells were washed and cell pellets were frozen at −80°C.
HIV Infection
CD4 SP thymocytes were cultured for 4 h at 3.5 × 107 cells/mL, in the presence of 0.3 ng RT of HIV-1 or HIV-2/106 thymocytes, washed and cell pellets frozen at −80°C. All viruses used were primary isolates using either CCR5 as co-receptor, namely HIV-192US660 and HIV-260415K (NIH AIDS Reagent Program, Division of AIDS, the latter given by Dr. Feng Gao and Dr. Beatrice Hahn), or CXCR4 as co-receptor, namely HIV-192HT599 (NIH AIDS Reagent Program, Division of AIDS, given by Dr. Neal Halsey) and HIV-220.04 (kindly provided by Dr. Nuno Taveira). We have previously validated the infection protocol using the same viral strains in human thymocytes (24).
Statistical Analysis
Statistical analysis was performed using GraphPad Prism v9 (GraphPad Software Inc.). Two-sample data were compared using Wilcoxon-matched pairs test. Data from more than two samples were compared using Friedman test or Kruskal-Wallis test with Dunn's multiple comparison post-test. P values < 0.05 were considered significant.
Results
HERV Expression in Human Thymus
It is likely that HERV expression in the thymus impacts on the patterning of the peripheral T cell repertoire, with possible implications in autoimmune conditions. Here, we analyzed, for the first time, the transcriptional expression of the major HERV genes, namely HERV-K (HML-2), HERV-W and HERV-R env, as well as HERV-K (HML-2) gag, during T cell development and in other main thymic populations. In the future will be important to address the expression of other HERVs, such as the HERV-H (12), for which we were unable to optimize a reliable quantitative assay for human thymic cells.
All HERV genes analyzed were expressed in all sorted subsets, confirming that they are transcriptionally active during T cell development (Figure 1). There was a trend for all the HERV genes analyzed to decrease expression with differentiation, except for HERV-W env, which presented higher levels (although not statistically significant) at the DP stage. Of note, HERV-W env was the least expressed gene of all HERVs analyzed. Furthermore, we found the expression of HERV-K (HML-2) gag lower than that of HERV-K (HML-2) env at all stages, indicating different regulation of these viral genes. The triple-negative (TN) CD34 + progenitors presented the highest HERV-R env expression levels, then decreasing upon T cell differentiation, which was further confirmed in progenitors isolated from the cord blood (Figure 1A).
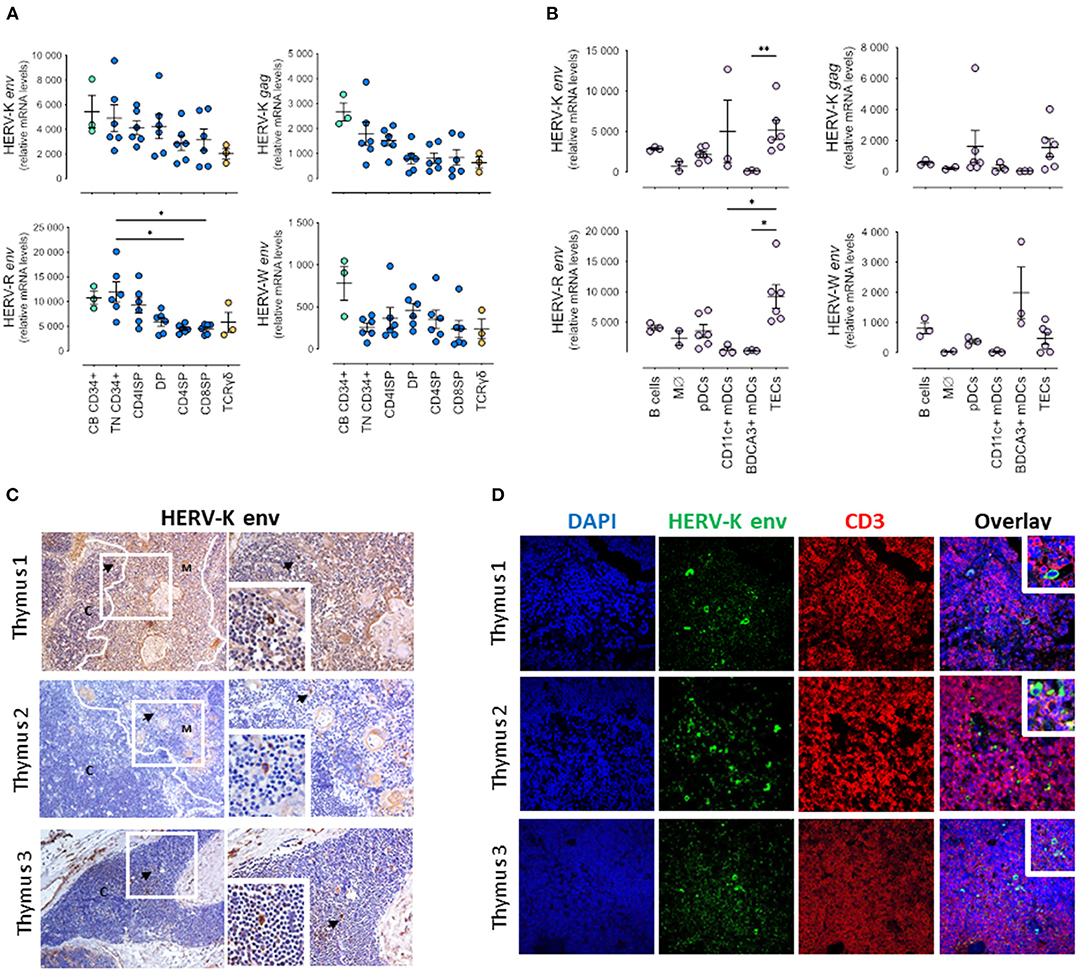
Figure 1. HERV expression in pediatric thymuses. Relative transcriptional levels of HERV-K, -W, -P and -R env normalized to β-actin expression levels in sort-purified: (A) CD34 + cells from cord blood, thymocyte subsets along T cell development (TN, Triple Negative; CD4ISP, Immature Single Positive CD4; DP, Double Positive; CD4SP, CD4 Single Positive and CD8SP, CD8 Single Positive) and γδ thymocytes; (B) Major non-T cell populations in the thymus, namely B cells, monocytes/macrophages (Mϕ), plasmacytoid dendritic cells (pDCs), both CD11c + and BDCA3 + myeloid dendritic cells (DCs), and thymic epithelial cells (TECs); each dot represents one different thymus; results were compared using Kruskal-Wallis with Dunn's multiple comparisons test; *P < 0.05; **P < 0.001. (C) HERV-K Env protein expression evaluated by immunohistochemistry; left images amplification 200x; right images amplification 400x; Digital zoom insert - the image down to a centered area with the same ratio as the original; black arrows indicate positive cells stained in brown; white lines shows delimitation cortex/medulla area; C, Cortex; M, Medulla. (D) Double staining for HERV-K Env protein and CD3 by immunofluorescence; image amplification 400x; Digital zoom insert - the image down to a centered area with the same ratio as the original.
Notably, we were able to quantify the HERV transcripts in the main antigen-presenting populations in the thymus, namely B cells, myeloid and plasmacytoid dendritic cells, and monocytes/macrophages, as well as in thymic epithelial cells (TECs) that featured the highest levels (Figure 1B). Interestingly, the expression of HERV-K (HML-2) gag was also lower than that of HERV-K (HML-2) env at all cell populations, further supporting a distinct regulation of transcription or splicing of the gag.
We also evaluated at the protein level the expression of the HERV-K Env, and confirmed its expression in the thymic tissue both by immunohistochemistry (Figure 1C) and by immunofluorescence (Figure 1D). HERV-K (HML-2) Env + cells were found in CD3 negative cells with thymocyte morphology in the cortex, but were more frequently detected in larger CD3 negative cells in the medulla, near Hassall's corpuscles, that were likely stromal cells (Figures 1C,D).
As a validation control we quantified in parallel the HERV transcripts in purified CD4 T cell subsets from pediatric human tonsils (Supplementary Figure 1A), as well as the expression of HERV-K Env by immunohistochemistry (Supplementary Figure 1B) and by immunofluorescence (Supplementary Figure 1C). The transcriptional levels observed tended to be lower than those observed in the thymus, though the HERV-K (HML-2) Env expression was more frequently detected, and in cells co-expressing CD3 located outside the germinal centers, raising the possibility of a translational control of HERV-K (HML-2) Env protein in the thymus.
Overall, we demonstrated, for the first time, the expression of 3 HERV families, HERV-K, -W, and –R, in the human thymus and show that their transcriptional levels tended to decrease along T cell development, and were highly expressed in stromal cells, particularly TECs.
Host Restriction Factor Expression in Human Thymus
It is plausible to speculate that HERV expression may induce a cell response with up-regulation of host restriction factors. Therefore, we evaluated in the purified populations described above the transcriptional levels of APOBEC3G (apolipoprotein B mRNA editing enzyme, catalytic polypeptide-like G), APOBEC3F, SAMHD1 (SAM And HD Domain Containing Deoxynucleoside Triphosphate Triphosphohydrolase 1) and SLFN11 (Schlafen 11), as shown in Figures 2A,B. Interestingly, the pattern of expression of APOBEC3G, APOBEC3F, and SLFN11 followed the one observed with HERVs with higher levels in progenitors and early thymocyte stages (Figure 2A). Conversely, the levels of SAMHD1 were lower in these populations (Figure 2A), possibly due to their high proliferative rates since SAMHD1 is known to decrease during the cell cycle (25). However, SAMHD1 levels were high in monocytes/macrophages and dendritic cells (Figure 2B). APOBECs, particularly APOBEC3F, were highly expressed in B cells and TECs, whereas SLFN11 was distinctly found in plasmacytoid dendritic cells (Figure 2B). These results are in agreement with the findings that we observed at the protein level by immunohistochemistry, namely preferential expression of APOBEC3G, SAMHD1 and SLFN11 in the thymic medulla (Figure 2C).
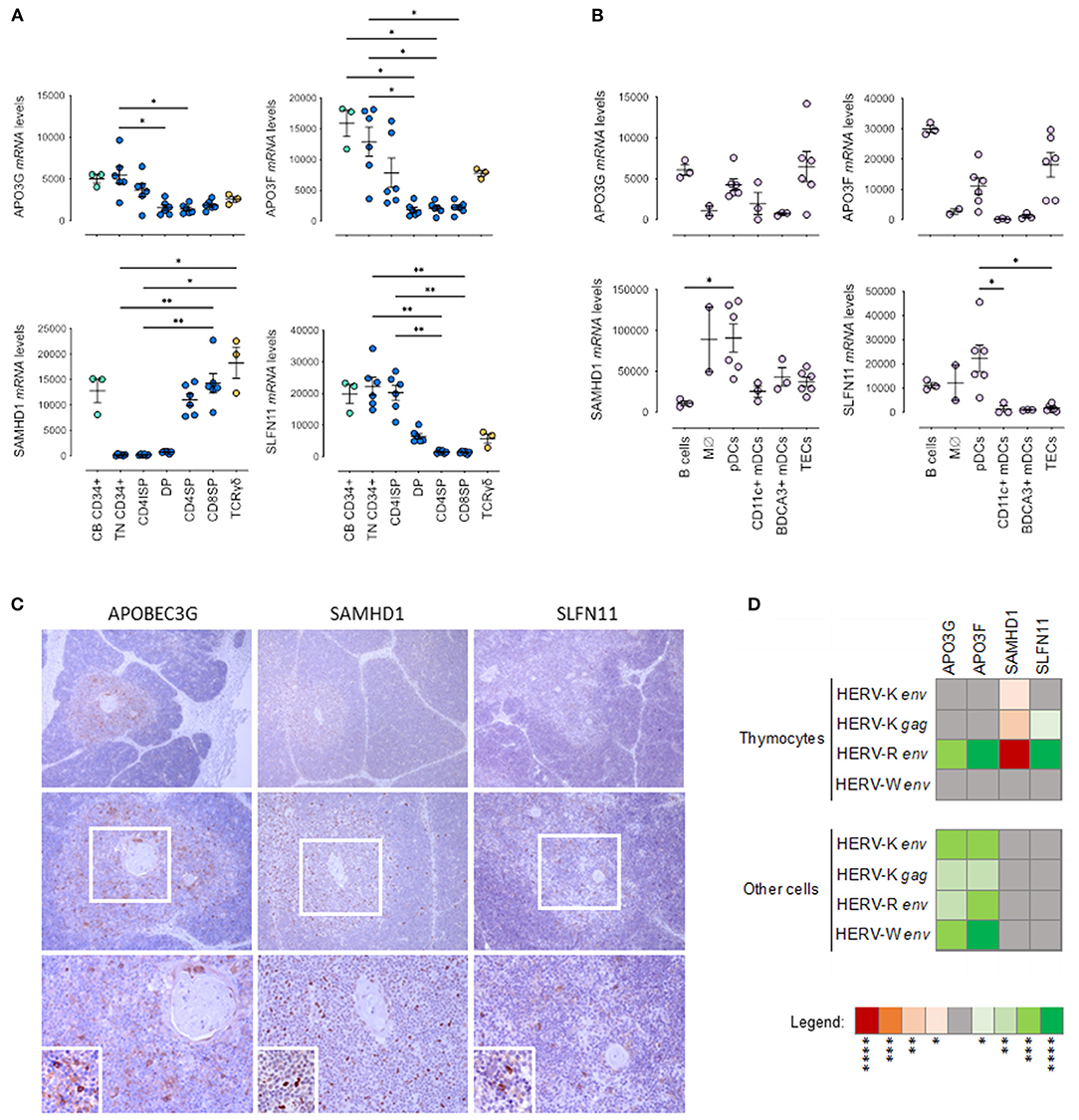
Figure 2. Host Restriction Factor (HRF) expression in pediatric thymuses. Relative transcriptional levels of the host restriction factors APOBEC3G, APOBEC3F, SAMHD1 and SLFN11 normalized to β-actin expression levels in sort-purified: (A) CD34 + cells from cord blood, thymocyte subsets along T cell development (TN, Triple Negative; CD4ISP, Immature Single Positive CD4; DP, Double Positive; CD4SP, CD4 Single Positive and CD8SP, CD8 Single Positive) and γδ thymocytes; (B) Major non-T cell populations in the thymus, namely B cells, monocytes/macrophages (Mϕ), plasmacytoid dendritic cells (pDCs), both CD11c + and BDCA3 + myeloid dendritic cells (DCs), and thymic epithelial cells (TECs); each dot represents one different thymus; results were compared using Kruskal-Wallis with Dunn's multiple comparisons test; *P < 0.05; **P < 0.001. (C) APOBEC3G, SAMHD1 and SLFN11 protein expression evaluated by immunohistochemistry in representative thymus samples; upper images amplification 100x; middle images amplification 200x; bottom images 400x - Digital zoom insert - the image down to a centered area with the same ratio as the original; Positive cells stained in brown; (D) Correlogram of the transcriptional levels of factors APOBEC3G, APOBEC3F, SAMHD1 and SLFN11 with transcriptional levels of HERV-K, -W, and -R env in total thymocytes and in the other cells from the thymus, using non-parametric Spearman correlation test (red/negative and green/positive). *p < 0.05; **p < 0.01; ***p < 0.001; ****p < 0.0001.
Again, we compared the expression of HRFs in the thymus with the levels observed in pediatric tonsils (Supplementary Figure 2). We found that the thymic levels tended to be higher than those found in the tonsil even when we excluded the follicular/germinal center T cells, which expressed very low levels of HRFs (Supplementary Figure 2A). Moreover, the transcriptional levels of HERVs and HRFs were also poorly correlated in the tonsil subsets (Supplementary Figure 2B).
Interestingly, HERV-R env levels in thymocytes significantly correlated with APOBEC3G, APOBEC3F and SLFN11 and inversely with SAMHD1 expression (Figure 2D). Moreover, the APOBEC3G and APOBEC3F expression levels were also directly correlated the levels of expression of HERV- K gag and HERV-K, -R, -W env in thymic stromal cells (Figure 2D).
Altogether, our data support the possibility that HERVs may modulate the up-regulation of the main host restriction factors in the human thymus.
Changes in HERV and HRF Transcriptional Levels Upon Thymocyte Stimulation or HIV Exposure
HERVs levels, and specifically HERV-K (HML-2), have been shown to be modulated by cytokines, hormones and chemical agents in different types of cells, but their regulation in thymocytes has not been addressed. We thus studied the modulation of HERV-K (HML-2) env and gag by cytokines and activation conditions in CD4SP thymocytes (Figure 3A).
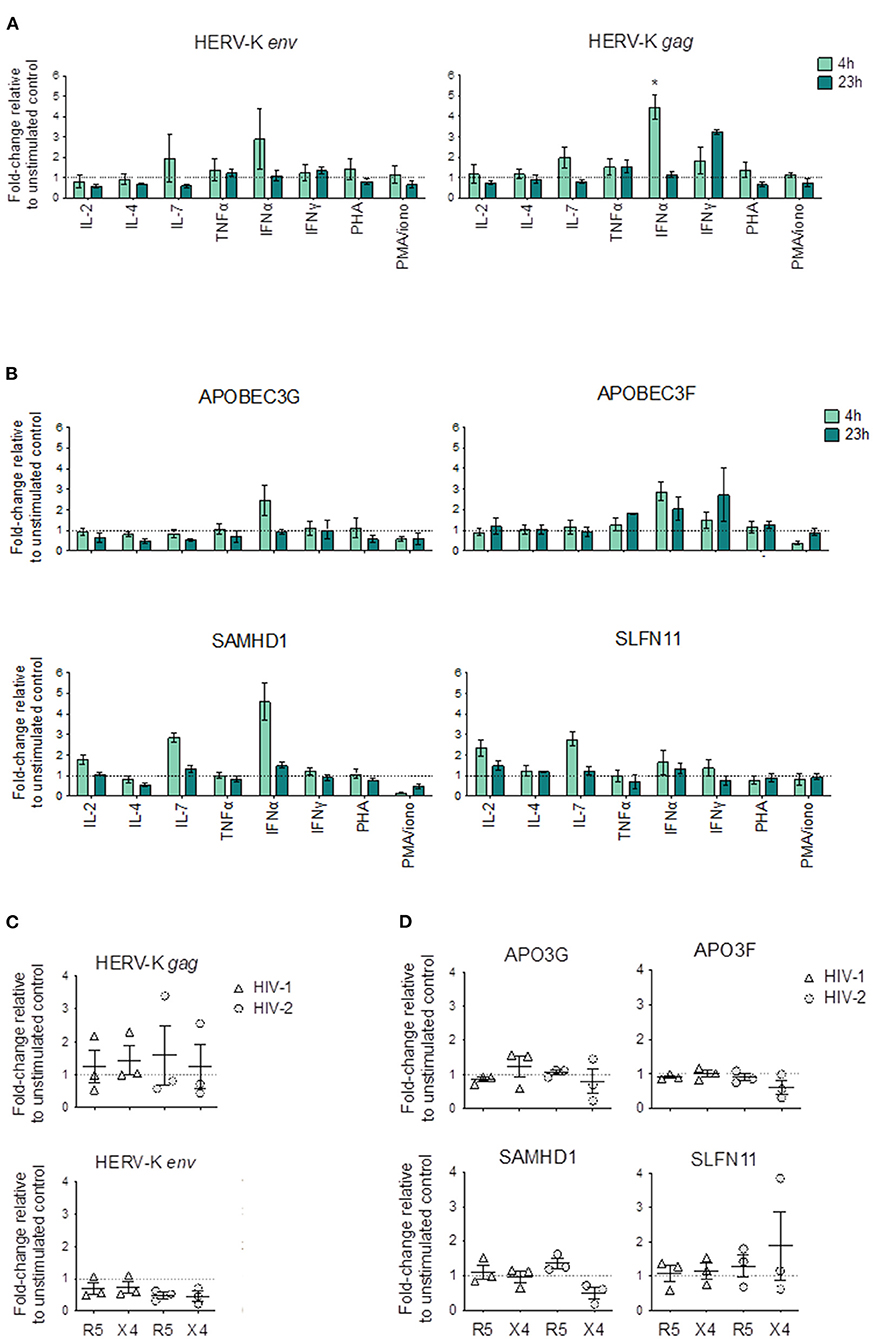
Figure 3. Changes in HERV and HRF transcriptional levels upon cytokine and mitogenic stimulation of CD4SP thymocytes and upon HIV exposure. (A,B) Sorted CD4SP thymocytes were stimulated for 4 h (light green) or 23 h (dark green) with medium alone or in the presence of IL-2, IL-4, IL-7, TNF-α, IFN-α, IFN-γ, PHA or PMA + Ionomycin, and the relative expression levels of HERVs (A) and HRFs (B) calculated as the fold-change relative to the unstimulated control by ΔΔCT in 3 thymuses; Bars represent mean ± SEM, Kruskal-Wallis test with Dunn's multiple comparison post-test was used to compare each condition with the unstimulated control, and the significant comparisons were shown, *p < 0.05. (C,D) Sorted CD4SP thymocytes were infected by HIV-1 (triangles) with or with HIV-2 (circles) with primary isolates using CXCR4 (X4) or CCR5 (R5) as co-receptors; cells were collected after 4 h and the relative expression levels of HERVs (C) and HRFs (D) calculated as the fold-change relative to the non-infected control by ΔΔCT; Each symbol represents a thymus.
Of all the stimuli tested, only IFNα clearly increased HERV-K (HML-2) levels (Figure 3A). IFNγ also increased HERV-K (HML-2) gag but not env levels, although with slower kinetics than IFNα. In addition, IL-7 increased HERV-K (HML-2) gag expression. Of note, we found no evidence of increased HERV-K (HML-2) env or gag expression upon stimulation or in any of the activation conditions tested (Figure 3A).
In parallel, we quantified the changes in the expression of HRFs, and found that IFNα stimulation induced increase in the transcriptional levels of APOBEC3G, APOBEC3F, and SAMHD1 in CD4SP, whereas IL-7 increased the levels of SAMHD1 and SLFN11 (Figure 3B).
Next, we asked whether HIV exposure of CD4SP thymocytes modulate the expression of HERV genes. For this purpose, we selected both HIV-1 and HIV-2 primary isolates with known co-receptor usage, namely CCR5 or CXCR4, that were previously used in our laboratory to infect human thymocytes (24). Interestingly, none of the viral strains were able to significantly change the levels of expression of HERV-K (HML-2) env and gag (Figure 3C). Moreover, no consistent changes were also observed in the levels in the evaluated HRFs in CD4SP upon HIV exposure (Figure 3D).
In conclusion, our data support that IFN and IL-7, but not cell activation, may modulate HERV-K (HML-2) env and gag expression in CD4SP thymocytes.
Discussion
We show here that the expression of HERV-K, -W, and –R decrease along T cell development in the human thymus. The highest levels in the early stages are in line with the high levels previously described in hematopoietic progenitors (8, 9), and may be in part related to the high proliferative rate of immature thymocytes (26). HERV expression in thymocytes is particularly important in the human context given the possible contribute of T-T interactions to the T cell selection through the expression of MHC class II (4, 26). Our data further support a possible impact of HERV expression for the TCR repertoire selection (10).
It is worth noting that the HERV expression follows the same kinetics of the expression of APOBECs, HRFs that play a determinant role in the control of retroviral infections (27). Given the role of APOBECs in cell biology (28), their possible impact in T cell selection deserves exploration. The same observation has been reported in the peripheral blood, particularly in patients with autoimmune diseases, namely systemic lupus erythematous and Sjogren Syndrome (15, 29, 30). The same profile was observed with SLFN11, a putative DNA/RNA helicase that induces irreversible replication block, which is emerging as an important regulator of cellular response to DNA damage in cancer cells (31, 32), not yet explored in T cell development. Conversely, we find an opposite profile between SAMHD1 and HERV expression. The low levels of SAMHD1 in early stages is likely related to its transcriptional down-regulation in proliferating cells (25), being plausible that they may play a role in the control of HERV expression in mature quiescent thymocytes, as suggested for HIV infection (33). The role of HRFs in these cells is at least in part mediated by IFN (19). In this regard it is worth emphasizing our findings of up-regulation of both SAMHD1 and SLFN11 in CD4SP thymocytes parallel with HERV up-regulation. Our preliminary data also suggest a possible impact of IL-7 that should be further investigated given the central role of this cytokine in T cell development and human naïve CD4 T cell homeostasis (34).
Surprisingly we were unable to reveal an impact of either HIV-1 or HIV-2 infection in HERV expression in CD4SP thymocytes using both CCR5- and CXCR4-tropic primary isolates. Although we did not exclude a possible later up-regulation of HERV expression, these results suggest a wired control of their expression in these mature quiescent thymocytes, in contrast with peripheral and activated CD4 T cells (18, 20, 35).
Of major interest are the high levels of HERVs expression in TECs, B cells and dendritic cells isolated from the human thymus, supporting an impact on T cell selection (10). Moreover, our finding of HERV protein expression in the thymic medulla further reinforces their possible role in negative selection, with implications for autoimmune diseases (15). We found high levels of HERV-W in the BDCA-3 + dendritic cells isolated from the 3 thymuses evaluated which deserves confirmation and exploration given the recent association of HERV-W with pediatric onset of type 1 Diabetes (17). The relative high levels of HERV expression in TECs point to the relevance of investigating their contribution to the transcriptional regulation in these cells central for T cell selection (7). Interestingly, we found distinct patterns of HRFs in human thymic stromal cells, with TECs and B cells relying mostly in APOBECs, whereas plasmacytoid dendritic cells expressed the highest levels of SLFN11 and SAMHD1. The latter have been previously reported in peripheral dendritic cells with relevance for HIV infection (36).
Overall, we found that the profile of HERV expression along human T cell development and in thymic stromal cell populations parallels the expression levels of key host restriction factors, supporting a relevant role for HERVs in human thymus biology.
Data Availability Statement
The raw data supporting the conclusions of this article will be made available by the authors, without undue reservation.
Ethics Statement
The studies involving human participants were reviewed and approved by Ethical boards of the Faculdade de Medicina da Universidade de Lisboa, of the Centro Hospitalar Universitário Lisboa Norte, and of the Hospital de Santa Cruz, Portugal. Written informed consent to participate in this study was provided by the participants' legal guardian/next of kin.
Author Contributions
VP, RF, AS, and HN-C: conceptualize. VP, AP, RF, and HN-C: perform research. VP and HN-C: analyze data. VP, AS, and HN-C: write the manuscript. All authors contributed to the article and approved the submitted version.
Funding
This work was supported by Fundação para a Ciência e a Tecnologia (FCT) and by Programa Operacional Ciência e Inovação 2010 (POCI2010), grant PTDC/SAU-MII/66248/2006 to AS, and a Gilead Genesis Grant to HN-C. HN-C received a scholarship from FCT co-financed by POCI2010.
Conflict of Interest
The authors declare that the research was conducted in the absence of any commercial or financial relationships that could be construed as a potential conflict of interest.
Publisher's Note
All claims expressed in this article are solely those of the authors and do not necessarily represent those of their affiliated organizations, or those of the publisher, the editors and the reviewers. Any product that may be evaluated in this article, or claim that may be made by its manufacturer, is not guaranteed or endorsed by the publisher.
Acknowledgments
The authors thank Dr. Miguel Abecasis and Dr. Rui Anjos for human thymus sample collection; the ENT department of CHULN for tonsil collections; Dr. Francisca Matos and nurses and staff at Hospital de Santa Cruz for technical assistance; Diana Santos and Pedro Rosmaninho for technical support; Afonso Almeida and Alexandre ASF Raposo for their critical reading of the manuscript; patients and families.
Supplementary Material
The Supplementary Material for this article can be found online at: https://www.frontiersin.org/articles/10.3389/fviro.2022.826393/full#supplementary-material
References
1. Kondo K, Takada K, Takahama Y. Antigen processing and presentation in the thymus: implications for T cell repertoire selection. Curr Opin Immunol. (2017) 46:53–7. doi: 10.1016/j.coi.2017.03.014
2. Daley SR, Teh C, Hu DY, Strasser A, Gray DHD. Cell death and thymic tolerance. Immunol Rev. (2017) 277:9–20. doi: 10.1111/imr.12532
3. Breed ER, Lee ST, Hogquist KA. Directing T cell fate: how thymic antigen presenting cells coordinate thymocyte selection. Semin Cell Dev Biol. (2018) 84:2–10. doi: 10.1016/j.semcdb.2017.07.045
4. Caramalho Í, Nunes-Cabaço H, Foxall RB, Sousa AE. Regulatory T-cell development in the human thymus. Front Immunol. (2015) 6:395. doi: 10.3389/fimmu.2015.00395
5. Takahama Y, Ohigashi I, Baik S, Anderson G. Generation of diversity in thymic epithelial cells. Nat Rev Immunol. (2017) 17:295–05. doi: 10.1038/nri.2017.12
6. Kaiser C, Bradu A, Gamble N, Caldwell JA, Koh AS. AIRE in context: leveraging chromatin plasticity to trigger ectopic gene expression. Immunol Rev. (2022) 305:59–76. doi: 10.1111/imr.13026
7. Martinez-Ruíz GU, Morales-Sánchez A, Bhandoola A. Transcriptional and epigenetic regulation in thymic epithelial cells. Immunol Rev. (2022) 305:43–58. doi: 10.1111/imr.13034
8. Wang T, Medynets M, Johnson KR, Doucet-O'Hare TT, DiSanza B, Li W, et al. Regulation of stem cell function and neuronal differentiation by HERV-K via mTOR pathway. Proc Natl Acad Sci USA. (2020) 117:17842–53. doi: 10.1073/pnas.2002427117
9. Babarinde IA, Ma G, Li Y, Deng B, Luo Z, Liu H, et al. Transposable element sequence fragments incorporated into coding and noncoding transcripts modulate the transcriptome of human pluripotent stem cells. Nucleic Acids Res. (2021) 49:9132–53. doi: 10.1093/nar/gkab710
10. Meylan F, De Smedt M, Leclercq G, Plum J, Leupin O, Marguerat S, et al. Negative thymocyte selection to HERV-K18 superantigens in humans. Blood. (2005) 105:4377–82. doi: 10.1182/blood-2004-07-2596
11. Yi J-M, Kim H-S. Expression analysis of endogenous retroviral elements belonging to the HERV-F family from human tissues and cancer cells. Cancer Lett. (2004) 211:89–96. doi: 10.1016/j.canlet.2004.01.026
12. Yi J-M, Kim H-M, Kim H-S. Human endogenous retrovirus HERV-H family in human tissues and cancer cells: expression, identification, and phylogeny. Cancer Lett. (2006) 231:228–39. doi: 10.1016/j.canlet.2005.02.001
13. Yi J-M, Kim T-H, Huh J-W, Park KS, Jang SB, Kim H-M, et al. Human endogenous retroviral elements belonging to the HERV-S family from human tissues, cancer cells, and primates: expression, structure, phylogeny and evolution. Gene. (2004) 342:283–92. doi: 10.1016/j.gene.2004.08.007
14. Kim H-S, Yi J-M, Hirai H, Huh J-W, Jeong M-S, Jang S-B, et al. Human endogenous retrovirus (HERV)-R family in primates: chromosomal location, gene expression, and evolution. Gene. (2006) 370:34–42. doi: 10.1016/j.gene.2005.11.008
15. Gröger V, Cynis H. Human endogenous retroviruses and their putative role in the development of autoimmune disorders such as multiple sclerosis. Front Microbiol. (2018) 9:265. doi: 10.3389/fmicb.2018.00265
16. Xue B, Sechi LA, Kelvin DJ. Human endogenous retrovirus K (HML-2) in health and disease. Front Microbiol. (2020) 11:1690. doi: 10.3389/fmicb.2020.01690
17. Noli M, Meloni G, Manca P, Cossu D, Palermo M, Sechi LA. HERV-W and mycobacterium avium subspecies paratuberculosis are at play in pediatric patients at onset of type 1 diabetes. Pathogens. (2021) 10:1135. doi: 10.3390/pathogens10091135
18. Srinivasachar Badarinarayan S, Shcherbakova I, Langer S, Koepke L, Preising A, Hotter D, et al. HIV-1 infection activates endogenous retroviral promoters regulating antiviral gene expression. Nucleic Acids Res. (2020) 48:10890–908. doi: 10.1093/nar/gkaa832
19. Zhao X, Zhao Y, Du J, Gao P, Zhao K. The interplay among HIV, LINE-1, and the interferon signaling system. Front Immunol. (2021) 12:732775. doi: 10.3389/fimmu.2021.732775
20. Li X, Guo Y, Li H, Huang X, Pei Z, Wang X, et al. Infection by diverse HIV-1 subtypes leads to different elevations in HERV-K transcriptional levels in human T cell lines. Front Microbiol. (2021) 12:662573. doi: 10.3389/fmicb.2021.662573
21. Lima-Junior DS, Krishnamurthy SR, Bouladoux N, Collins N, Han S-J, Chen EY, et al. Endogenous retroviruses promote homeostatic and inflammatory responses to the microbiota. Cell. (2021) 184:3794–811.e19. doi: 10.1016/j.cell.2021.05.020
22. Balestrieri E, Minutolo A, Petrone V, Fanelli M, Iannetta M, Malagnino V, et al. Evidence of the pathogenic HERV-W envelope expression in T lymphocytes in association with the respiratory outcome of COVID-19 patients. Ebio Med. (2021) 66:103341. doi: 10.1016/j.ebiom.2021.103341
23. Caramalho I, Nunes-Silva V, Pires AR, Mota C, Pinto AI, Nunes-Cabaço H, et al. Human regulatory T-cell development is dictated by interleukin-2 and−15 expressed in a non-overlapping pattern in the thymus. J Autoimmun. (2015) 56:98–110. doi: 10.1016/j.jaut.2014.11.002
24. Nunes-Cabaço H, Matoso P, Foxall RB, Tendeiro R, Pires AR, Carvalho T, et al. Thymic HIV-2 infection uncovers posttranscriptional control of viral replication in human thymocytes. J Virol. (2015) 89:2201–8. doi: 10.1128/JVI.03047-14
25. Mauney CH, Hollis T. SAMHD1: recurring roles in cell cycle, viral restriction, cancer, and innate immunity. Autoimmunity. (2018) 51:96–110. doi: 10.1080/08916934.2018.1454912
26. Rothenberg EV. Single-cell insights into the hematopoietic generation of T-lymphocyte precursors in mouse and human. Exp Hematol. (2021) 95:1–12. doi: 10.1016/j.exphem.2020.12.005
27. Cervantes-Gracia K, Gramalla-Schmitz A, Weischedel J, Chahwan R. APOBECs orchestrate genomic and epigenomic editing across health and disease. Trends Genet TIG. (2021) 37:1028–43. doi: 10.1016/j.tig.2021.07.003
28. Mavragani CP, Kirou KA, Nezos A, Seshan S, Wild T, Wahl SM, et al. Expression of APOBEC family members as regulators of endogenous retroelements and malignant transformation in systemic autoimmunity. Clin Immunol. (2021) 223:108649. doi: 10.1016/j.clim.2020.108649
29. Khadjinova AI, Wang X, Laine A, Ukadike K, Eckert M, Stevens A, et al. Autoantibodies against the envelope proteins of endogenous retroviruses K102 and K108 in patients with systemic lupus erythematosus correlate with active disease. Clin Exp Rheumatol. (2021). [Epub ahead of print].
30. Balada E, Vilardell-Tarrés M, Ordi-Ros J. Implication of human endogenous retroviruses in the development of autoimmune diseases. Int Rev Immunol. (2010) 29:351–70. doi: 10.3109/08830185.2010.485333
31. Schwarz DA, Katayama CD, Hedrick SM. Schlafen, a new family of growth regulatory genes that affect thymocyte development. Immunity. (1998) 9:657–68. doi: 10.1016/s1074-7613(00)80663-9
32. Zhang B, Ramkumar K, Cardnell RJ, Gay CM, Stewart CA, Wang W-L, et al. A wake-up call for cancer DNA damage: the role of Schlafen 11 (SLFN11) across multiple cancers. Br J Cancer. (2021) 125:1333–40. doi: 10.1038/s41416-021-01476-w
33. Chen S, Bonifati S, Qin Z, St Gelais C, Wu L. SAMHD1 Suppression OF antiviral immune responses. Trends Microbiol. (2019) 27:254–67. doi: 10.1016/j.tim.2018.09.009
34. Silva SL, Sousa AE. Establishment and maintenance of the human naïve CD4+ T-cell compartment. Front Pediatr. (2016) 4:119. doi: 10.3389/fped.2016.00119
35. Monde K, Terasawa H, Nakano Y, Soheilian F, Nagashima K, Maeda Y, et al. Molecular mechanisms by which HERV-K gag interferes with HIV-1 gag assembly and particle infectivity. Retrovirology. (2017) 14:27. doi: 10.1186/s12977-017-0351-8
Keywords: human thymus, HERVs, host restriction factors, HIV, APOBEC3F, APOBEC3G, SLFN11, SAMHD1
Citation: Passos V, Pires AR, Foxall RB, Nunes-Cabaço H and Sousa AE (2022) Expression of Human Endogenous Retroviruses in the Human Thymus Along T Cell Development. Front. Virol. 2:826393. doi: 10.3389/fviro.2022.826393
Received: 30 November 2021; Accepted: 17 January 2022;
Published: 10 February 2022.
Edited by:
Mario Clerici, University of Milan, ItalyReviewed by:
Zsolt Ruzsics, University of Freiburg Medical Center, GermanyMassimiliano Bergallo, University of Turin, Italy
Copyright © 2022 Passos, Pires, Foxall, Nunes-Cabaço and Sousa. This is an open-access article distributed under the terms of the Creative Commons Attribution License (CC BY). The use, distribution or reproduction in other forums is permitted, provided the original author(s) and the copyright owner(s) are credited and that the original publication in this journal is cited, in accordance with accepted academic practice. No use, distribution or reproduction is permitted which does not comply with these terms.
*Correspondence: Ana E. Sousa, asousa@medicina.ulisboa.pt
†These authors have contributed equally to this work and share senior authorship