- 1Institute of Clinical Medicine, Aarhus University, Aarhus, Denmark
- 2Department of Infectious Diseases, Aarhus University Hospital, Aarhus, Denmark
- 3Department of Infectious Diseases, Regional Hospital West Jutland, Herning, Denmark
- 4Department of Biology, University of Nebraska at Omaha, Omaha, NE, United States
A cure for human immunodeficiency virus (HIV-1) is restricted by the continued presence of a latent reservoir of memory CD4+ T cells with proviruses integrated into their DNA despite suppressive antiretroviral therapy (ART). A predominant strategy currently pursued in HIV-1 cure-related research is the “kick and kill” approach, where latency reversal agents (LRAs) are used to reactivate transcription from integrated proviruses. The premise of this approach is that “kicking” latent virus out of hiding allows the host immune system to recognize and kill infected cells. Clinical trials investigating the efficacy of LRAs, such as romidepsin, have shown that these interventions do induce transient spikes in viral RNA in HIV-1-infected individuals. However, since these trials failed to significantly reduce viral reservoir size or significantly delay time to viral rebound during analytical treatment interruptions, it is questioned how much each individual latent provirus is actually “kicked” to produce viral transcripts and/or proteins by the LRA. Here, we developed sensitive and specific digital droplet PCR-based assays with single-provirus level resolution. Combining these assays allowed us to interrogate the level of viral RNA transcripts from single proviruses in individuals on suppressive ART with or without concomitant romidepsin treatment. Small numbers of proviruses in peripheral blood memory CD4+ T cells were triggered to become marginally transcriptionally active upon romidepsin treatment. These novel assays can be applied retrospectively and prospectively in HIV-1 cure-related clinical trials to gain crucial insights into LRA efficacy at the single provirus level.
Introduction
Human immunodeficiency virus (HIV-1) is a major part of the global disease burden causing suppression of the immune system by depletion of its central regulators, the CD4+ T cells. Introduction of antiretroviral therapy (ART) in the mid 1990's has greatly reduced morbidity and mortality associated with HIV-1 by preventing depletion of CD4+ T cells and suppressing viral loads to undetectable levels (1, 2). However, ART is not curative. Soon after HIV-1 individuals stop taking their medication, the virus rebounds, and thus lifelong ART adherence is necessary to prevent disease progression (3).
Developing a cure for HIV-1 have so far been limited by the establishment of a reservoir of latently infected memory CD4+ T cells harboring integrated HIV-1 DNA. Most of these proviruses are transcriptionally silent and they ensure viral persistence despite suppressive treatment of the infection with ART (4–6). Therefore, elimination of cells harboring integrated proviruses in order to achieve a cure for HIV-1 has been the goal since this latent proviral reservoir was discovered. One of the dominant strategies currently used in the search for a cure is the “kick and kill” approach (7–12). This approach intends to reverse latency by forcing the latently infected cells to (re)activate proviral transcription thus allowing the host immune system to clear the infected cells (8). Different latency reversal agents (LRAs) have been tested in clinical trials since 2005 (13, 14). Our research team have tested the HDAC inhibitors panobinostat and romidepsin as well as a TLR9 agonist as LRAs (15–18). We and another group have observed transient spikes in cell-associated unspliced HIV-1 RNA copies following romidepsin infusion in HIV-1 infected individuals on suppressive ART as a marker of the “kick” (15, 17, 19). However, limited changes were found in the size of the viral reservoir after treatment with romidepsin in these studies and romidepsin treatment alone did not lead to significant delays to viral rebound during analytical treatment interruptions.
Previously, a single-cell, microfluidics-based analysis strategy was employed to estimate the number of HIV-1 transcripts in human CD4+ T cells stimulated ex vivo (20). However, only bulk populations of cells have been used to analyze viral transcription in samples from individuals on suppressive ART as well as in clinical samples from latency reversal trials. It is well-documented that there is an increase in HIV-1 transcription in response to LRAs, yet the contribution of individual proviruses to these increased transcript levels has never been assessed. This is important because the overall efficacy of LRAs has been below what was originally hypothesized or desired. Thus, we need to increase the resolution whereby we can define LRA efficacy to have single-provirus resolution. With that granularity of understanding, it will be easier to assess whether a given LRA, or combination of agents is likely to have sufficient impact on the latent viral reservoir to allow for ART-free remission. A single-provirus resolution assay for HIV-1 transcription is therefore needed to comprehensively evaluate whether observed increases in viral transcription in individuals upon stimulation with LRAs results from a small number of transcriptional productive proviruses or from a larger proportion of transcriptional active proviruses. Such differentiation is critical as these distinctive mechanisms of transcriptional activity upon latency reversal will have very different impacts on the latent HIV-1 reservoir.
To this end, we determined that there are four possible models that can account for the observed increased HIV-1 transcription following LRA treatment. The LRA activates: (1) few proviruses to each produce high levels of viral transcripts; (2) many proviruses to each produce high levels of viral transcripts: (3) few proviruses to each produce low levels of viral transcripts; or (4) many proviruses to each produce low levels of viral transcripts. To distinguish between these four outcomes, we invented a pair of novel assays to determine the number of viral transcripts present on single-provirus level.
Here, we first present the development and validation of the two interrelated assays, referred to as the quantitative viral transcription assay (QVTA) and single-provirus transcription assay (SPTA). Then we present the outcomes of our assays after we determined the breadth of provirus activation by romidepsin in vivo.
Materials and Methods
Ethical Statement and Samples From HIV-1 Individuals
Herein, cryopreserved PBMC samples from three different groups of HIV-1 individuals were used (Table 1). All individuals provided written informed consent. To ensure anonymity of participants, only de-identified data were utilized to complete this study. Participants in the “ART naïve” group comprise a group of five HIV-1 individuals from whom samples were collected prior to ART initiation [Danish Data Protection Agency approval #1-16-02-424-15 and approved by the National Committee on Health Research Ethics (#1-10-72-260-15)]. Participants in “suppressive ART—group 1” were part of a clinical study approved by the National Committee on Health Research Ethics (#1-10-72-311-13) and the Danish Data Protection Agency (#1-16-02-464-13) (21). This was a non-interventional, longitudinal study. Samples from participants from suppressive ART—group 1 included two longitudinal visits with 1–2 months between sample collections. Participants in “suppressive ART—group 2” were part of a separate clinical study approved by the National Committee on Health Research Ethics (#M-2013–364–13) (15). This was an interventional, longitudinal study wherein individuals received three infusions of romidepsin 1 week apart. Samples from participants from suppressive ART—group 2 included pre-romidepsin samples which were taken before the first infusion of romidepsin and post-romidepsin samples which were taken 4 h after third infusion of romidepsin (except ID36 which is after second infusion).
Cell Isolation, Well Seeding, and RNA Extraction
Cryopreserved PBMCs from study participants were thawed and subjected to negative magnetic bead selection of memory CD4+ T cells according to the manufacturer's instruction (Miltenyi Biotec, Bergisch Gladbach, Germany; cat# 130-091-893). Purified cells were seeded in dilutions of proviruses in 96-well plates. In order to get a specific number of proviruses in each well for ddPCR analyses as described below, we first calculated the number of HIV-1 DNA copies per memory CD4+ T cells as follows: total HIV-1 DNA copies in bulk divided by one minus the proportion of naïve T cells revealed by flow cytometry based on prior observations that naïve CD4+ T cells harbor very few proviruses (22–24). This number was per million cells, which allowed us to determine the number of cells to seed in a given well in order to have the prespecified number of HIV-1 proviruses present. Quantification of HIV-1 DNA copies in bulk was available from previous studies or performed on a different aliquot of the same sample as described in section Total HIV-1 DNA Measurements. In order to ensure the presence of sufficient cellular material for efficient RNA extraction from each well, we added 5,000 MOLT-4/CCR5 cells (NIH-ARP; Cat# 4984-447, RRID:CVCL_S545) to each well that received proviruses. Cells in each well were lysed in 100 μL RA1 lysis buffer containing 10 mM DTT, and RNA was extracted using nucleoSpin 96 RNA 96-well kit (Macherey-Nagel, Dueren, Germany; cat# 740709.4) according to the manufacturer's instruction with the following changes: we used 100 μL RA4 buffer, and we eluted RNA in 50 μL RNase-free water.
Quantification of HIV-1 Nucleic Acid Levels From Proviruses
Digital droplet PCR were used to quantify HIV-1 nucleic acid levels from the number of proviruses seeded in each well in the 96-well plates. More precisely, we are quantifying proviruses with intact packaging signals present in the unspliced HIV-1 RNA transcript based on previously published primer/probe pairs (21). Complementary DNA synthesis and denaturation were performed as previously described (21) with the following modifications: we used 50 pmol/μL HIV-1 specific reverse primer located in pol and 50 pmol/μL gene specific reverse primer for TBP (listed in Supplementary Table S1) (25). Reverse transcription was performed at 50°C for 45 min followed by heat inactivation of the reverse transcriptase at 80°C for 15 min. Triplicate technical replicates were used to assess HIV-1 nucleic acid levels from each well using the HIV-1 RNA detection combination of primers listed in Supplementary Table S1. The reference gene TBP (Applied Biosystems, Foster City, California; cat# 4448485) were analyzed in either duplicates or quadruplicates. Droplet generation was performed according to the manufacturer's instructions using a QX100 droplet generator (Bio-Rad, Hercules, California). The PCR reaction was carried out on a C1000 thermal cycler (Bio-Rad) and reading of droplets was performed by the QX100 droplet reader (Bio-Rad). Data were analyzed using QuantaSoft™ software (Bio-Rad).
QVTA and SPTA
The assays we developed, referred to as QVTA and SPTA quantify HIV-1 nucleic acid levels from single-proviruses seeded in 96-well plates using ddPCR as described above. The assays generate two interrelated readouts: transcriptional units per million proviruses (TUPM) and copies of HIV-1 RNA per active provirus (CAP), respectively. The TUPM value is calculated based upon Poisson statistics as applied to the number of HIV-1 transcription-positive wells within a sample. The TUPM value is analogous to an IUPM (infectious units per million resting CD4+ T cells) value from the quantitative viral outgrowth assay and it is built upon the same statistical basis (26). The distinction of TUPM from IUPM is that infectious units are not estimated in the TUPM results. Rather TUPM is an estimate of the total number of transcriptionally active proviruses in a given sample. The CAP value is a direct representation of the transcripts generated from an individual provirus and CAP measures HIV-1 RNA copies per active provirus in a given well.
Total HIV-1 DNA Measurements
The majority of total HIV-1 DNA levels presented herein were first reported in our previous studies (15, 21). Pre-romidepsin viral DNA levels for ID41 and ID44 are measurements performed herein (cells were not available to determine post-romidepsin viral DNA levels for ID41 and ID44) according to our previously described methods. Also, HIV-1 DNA level measurements are performed herein for the ART naïve individuals. Briefly, CD4+ T cells were enriched from thawed PBMCs using a negative-selection magnetic bead CD4+ T cell isolation kit (Miltenyi Biotec; cat# 130-096-533) according to the manufacturer's instructions. Genomic DNA was isolated from 1 × 106 purified CD4+ T cells using AllPrep DNA/RNA Mini Kit (Qiagen, Hilden, Germany; cat# 80204) according to the manufacturer's instructions. Total HIV-1 DNA was measured using ddPCR as previously described (21) with the HIV-1 gag and pol detection primer-probes combinations provided in Supplementary Table S1 (27–34).
Cell-Associated Unspliced HIV-1 RNA Measurements
Cell associated unspliced HIV-1 RNA levels from 1 × 106 CD4+ T cells were adapted from our previous studies for the individuals on suppressive ART (15, 21). For the ART naïve individuals, measurements are performed herein. Briefly, CD4+ T cells were enriched from thawed PBMCs using a negative-selection magnetic bead CD4+ T cell isolation kit (Miltenyi Biotec) according to the manufacturer's instructions. Genomic RNA was isolated from 1 × 106 purified CD4+ T cells using AllPrep DNA/RNA Mini Kit (Qiagen; cat# 80204) according to the manufacturer's instructions. Cell-associated unspliced HIV-1 RNA levels were measured using ddPCR as previously described (21) with the same modifications as described therein for quantification of HIV-1 nucleic acid from proviruses.
Statistics
All statistical analyses (alpha: 0.05) were carried out in GraphPad Prism version 9.0.2. Specific statistical tests performed are called out in the figure legends alongside the data that were analyzed by that approach.
Results
Defining the Number of Transcriptionally Active Proviruses in HIV-1 Individuals
The first goal of this work was to develop a method to determine contributions to viral transcription levels in HIV-1 infected individuals with single-provirus resolution using a digital droplet PCR (ddPCR) platform. Therefore, we first needed to assess the sensitivity of proviral transcription measurements by the ddPCR platform. For this, we used a relatively homogeneous sample pool consisting of 8E5/LAV cells (NIH-ARP Cat# 95, RRID:CVCL_3484) harboring approximately one integrated provirus per cell (35). 8E5/LAV cells were stimulated with 10 nM PMA for 2 h and seeded within a 10-fold dilution series in 96-well plates with 100, 10, or 1 cell per well, respectively. To ensure the presence of sufficient cellular material for efficient RNA extraction, we added 5,000 MOLT-4/CCR5 cells to each well that received 8E5/LAV cells. RNA was extracted from each well-and HIV-1 transcription was measured by ddPCR. We found that transcriptional activity could be measured from all three dilutions. Thus, the ddPCR platform can be used to measure transcription from single 8E5/LAV cells which corresponds to transcription per active provirus (Supplementary Figure S1A). From this result, we concluded that ddPCR sensitivity is sufficient to measure HIV-1 transcription from as little as one transcriptionally active provirus.
However, since many proviruses are transcriptionally silent in HIV-1 individuals (5, 36), seeding one provirus per well from individuals would result in an unwieldy number of wells to be analyzed with many wells lacking a transcriptionally active provirus. Therefore, we next determined the number of proviruses that must be assessed in order to measure transcription from a single active provirus in each reaction (Figure 1). To accomplish this, memory CD4+ T cells from three ART naïve individuals as well as from two individuals on suppressive ART—group 1 (Table 1) were seeded in limiting dilutions based upon principles for the design and interpretation of dilution assays (26). RNA was extracted from the cells and the total number of HIV-1 RNA copies per number of proviruses seeded per well were determined by ddPCR. A graphical illustration of the experimental setup is presented in Figure 1A. When analyzing the changes in HIV-1 RNA copies per number of proviruses seeded, we observed the expected decline in HIV-1 transcripts with lower numbers of proviruses seeded per well (Figures 1B,E). To have only one transcriptionally active provirus per well, the frequency of positive wells should hit approximately 33% according to Poisson statistics. Therefore, we calculated the frequency of positive wells from the four dilutions of proviruses for each single individual (Figures 1C,F) and for each group of individuals (Figures 1D,G). In both groups of individuals, the frequency of positive wells was 39% when 200 proviruses were seeded per well (Figures 1D,G). Therefore, assaying 200 proviruses is sufficient to detect individual transcriptionally active proviruses in both groups of individuals.
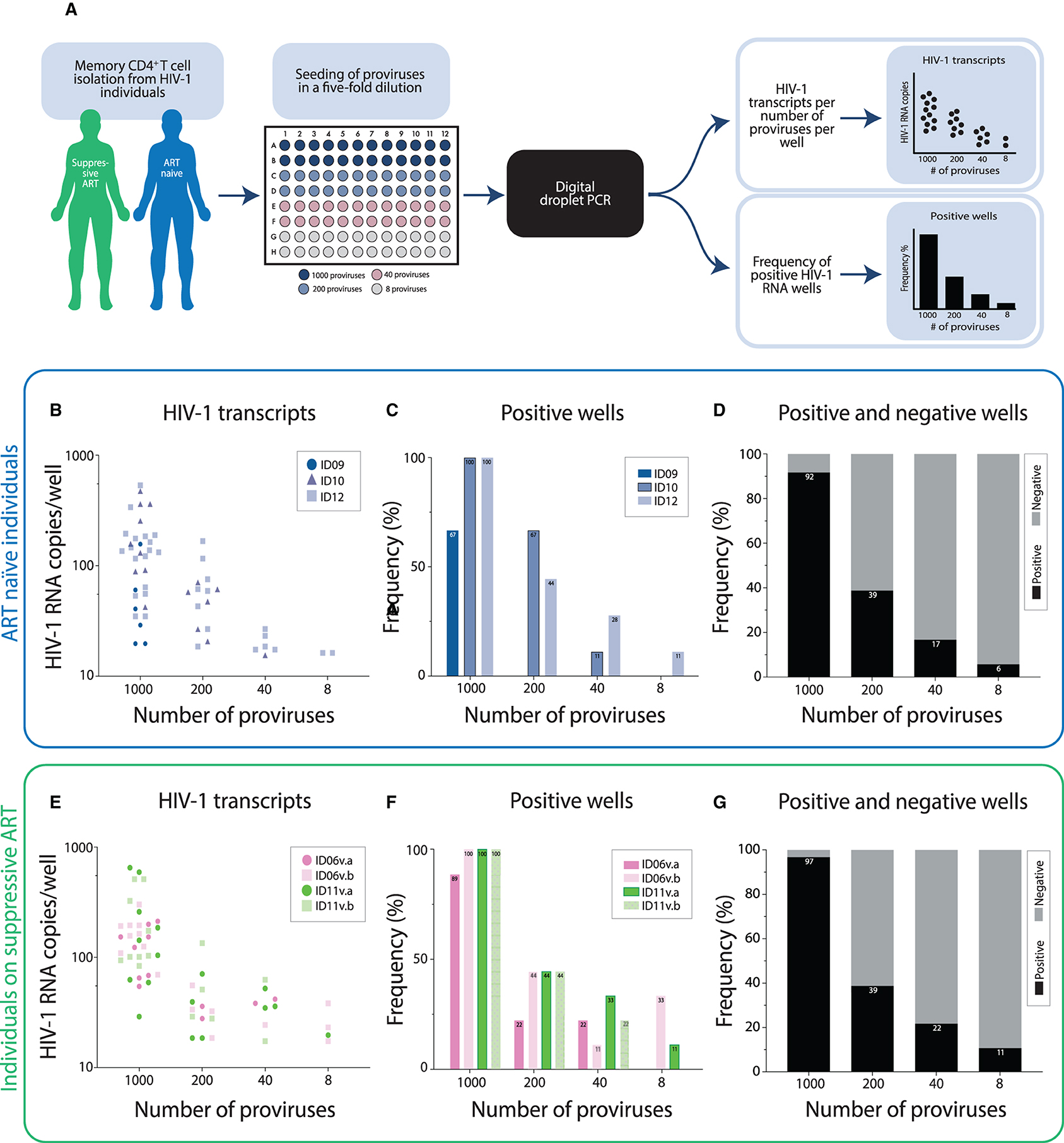
Figure 1. Limiting dilution digital droplet PCR (ddPCR) reveals that assaying 200 proviruses is sufficient to detect single transcriptionally active proviruses in ART naïve individuals as well as in individuals on suppressive ART. (A) Graphical illustration of the experimental setup. (B) Proviruses from three ART naïve individuals were seeded in limiting dilutions with 1,000, 200, 40, or 8 proviruses per well. The transcriptional activity in each well was measured by ddPCR. Each dot represents a positive well-measurement and shows the number of HIV-1 RNA copies measured in that well. In total, 36 wells were assayed for each dilution (9 wells for ID09, 9 wells for ID10, and 18 wells for ID12). (C,D) The frequencies of positive HIV-1 RNA measurements per ART naïve individual at the differing dilutions tested are depicted (C) and weighted averages accounting for all wells tested, both positive (black bars) and negative (gray bars) wells, are shown in (D). (E) HIV-1 transcripts from a dilution series of proviruses in individuals on suppressive ART are presented as indicated for (B) (9 wells per visit: v, visit). (F,G) The frequency of positive measurements of HIV-1 RNA by ddPCR in individuals on suppressive ART are presented as indicated for (C,D).
The QVTA and the SPTA Generate Stable Values of Transcriptional Units per Million Proviruses as Well as Stable Values of Copies per Active Provirus in HIV-1 Individuals on Suppressive ART
After defining the number of proviruses needed to detect transcription from a single provirus, we went on to establish and validate the reproducibility of the assays longitudinally in individuals that received no intervention between sample collection and had a previously demonstrated stable viral reservoir (21) as well as cross-sectionally in ART naïve individuals. The assays we developed, referred to as QVTA and SPTA generate two interrelated readouts: TUPM (transcriptional units per million proviruses) and CAP (copies per active provirus). The readouts are generated using ddPCR technology. We first examined TUPM- and CAP-values over time in suppressive ART—group 1 individuals. A graphical illustration of the QVTA and SPTA experimental setup generating the TUPM- and CAP-values is shown in Supplementary Figure 2A. We purified memory CD4+ T cells from longitudinal samples collected from three suppressive ART—group 1 individuals and seeded the cells in 96-well plates with 200 proviruses per well. Then, RNA was extracted from the cells in each well, and HIV-1 transcription from each individual well was determined by ddPCR. No difference in TUPM (p > 0.9999) or CAP (p = 0.3322) was observed between visit a and visit b (Figures 2B,C). In addition, we looked at the stability of TUPM outcomes across cell subtypes where proviruses are found. For this, we seeded total PBMCs as well as purified CD4+ T cells and purified memory CD4+ T cells from a single individual on suppressive ART—group 1 using the same experimental setup as shown in Figure 2A. We did not observe any differences in TUPM or CAP between total PBMCs and CD4+ T cells (p = 0.7677), between total PBMCs and memory CD4+ T cells (p > 0.9999) nor between CD4+ T cells and memory CD4+ T cells (p > 0.9999) (Supplementary Figure S2). Together these data show that our assays generated stable TUPM- and CAP-values in individuals on suppressive ART.
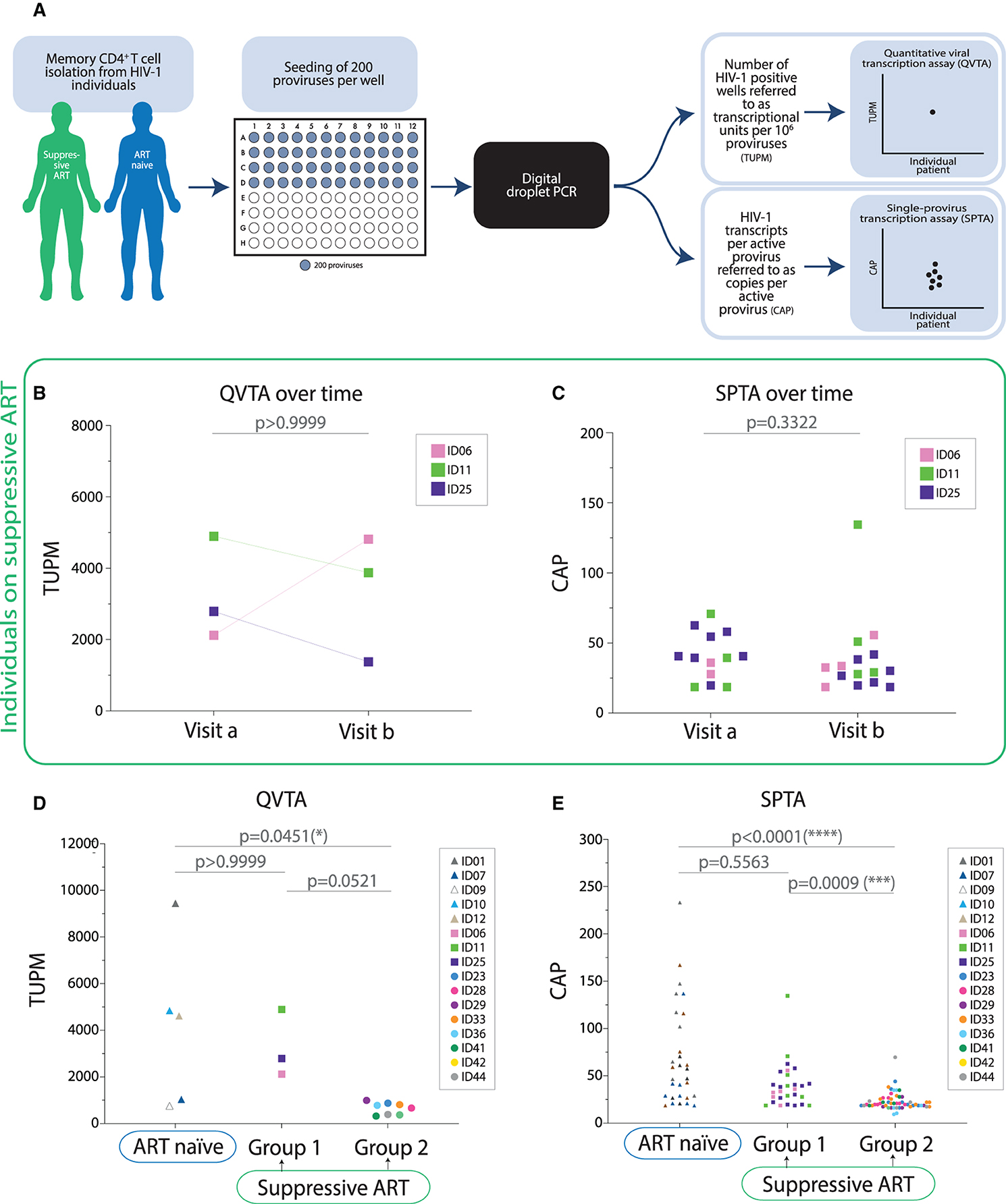
Figure 2. Transcriptional units per million proviruses as well as transcription number per each transcriptionally active provirus are higher in ART naïve individuals compared to individuals on suppressive ART with low baseline cell-associated unspliced HIV-1 RNA. (A) Graphical illustration of the experimental setup. (B) Assessment of transcriptional units per million proviruses (TUPM) over time in individuals on suppressive ART. The Wilcoxon matched-pairs signed rank test was performed to test for the difference in the transcriptional activity between visit a and b (1–2 months between sample collections) in individuals on suppressive ART (n = 3). (C) Assessment of copies of HIV-1 RNA per active provirus (CAP) in individuals on suppressive ART over time. Each symbol represents a positive measurement of HIV-1 RNA in a well containing one active provirus. The Mann Whitney test was used to assess potential differences in HIV-1 RNA copies per active provirus between two visits for each individual on suppressive ART. Paired analysis was not performed given that data included were generated from multiple wells per individual per visit (v, visit). (D) Assessment of TUPM in ART naïve individuals vs. individuals on suppressive ART [group 1 and group 2 are graphed independently given the substantial difference in baseline cell-associated unspliced HIV-1 RNA levels between these groups at inclusion (Table 1)]. For ID6, ID11, and ID25 TUPM are depicted as an average between the two visits. Statistical evaluation: Kruskal-Wallis test with Dunn's multiple comparison tests. (E) Assessment of CAP in ART naïve individuals vs. individuals on suppressive ART evaluated using Dunn's multiple comparison test. Each symbol represents a positive measurement of HIV-1 RNA in a well containing one active provirus.
TUPM and CAP Are Higher in ART Naïve Individuals Compared to Individuals on Suppressive ART
Given the reproducibility of the QVTA and SPTA over time, we next determined the contribution of single-proviruses to HIV-1 transcription in ART naïve individuals as well as in individuals on suppressive ART. Due to ongoing viral replication in untreated HIV-1 individuals and therefore high plasma viral loads compared to individuals on suppressive ART (37), we hypothesized that ART naïve individuals would have higher transcriptional activity on a per provirus basis. We purified memory CD4+ T cells from five ART naïve individuals and 11 individuals on suppressive ART (suppressive ART—group 1 and suppressive ART—group 2). Because suppressive ART—group 1 individuals exhibited higher cell-associated unspliced HIV-1 RNA levels relative to suppressive ART—group 2 individuals (median difference = 94.3 copies/106 CD4+ T cells; Mann-Whitney p = 0.012), the groups were analyzed separately (Table 1; Figure 2). ART groups 1 & 2 also differed in their time on ART (median difference = 50 months; Mann-Whitney p = 0.042), but not in their time with HIV-1 below 50 copies/mL (median difference = 48 months; Mann-Whitney p = 0.183) (Table 1). Cells from the two groups of individuals were seeded and analyzed as illustrated in Figure 2A. We found that TUPM in ART naïve individuals was significantly higher compared to individuals on suppressive ART—group 2 (p = 0.0451) (Figure 2D; Table 1). When looking at the SPTA for the same groups of individuals, we observed significantly higher CAP-values in ART naïve individuals compared to suppressive ART—group 2 (p < 0.0001) (Figure 2E). Moreover, we found that CAP-values are significantly higher in individuals on suppressive ART with high cell-associated unspliced HIV-1 (group 1) compared to individuals on suppressive ART with low cell-associated unspliced HIV-1 RNA levels (group 2) (p = 0.0009).
Romidepsin Treatment Reactivates Proviruses to Each Produce Few Viral Transcripts in HIV-1 Individuals on Suppressive ART
We had eight individuals on suppressive ART with elevated viral transcription in response to romidepsin and sufficient PBMCs cryopreserved for evaluation with our novel QVTA and SPTA (suppressive ART—group 2) (15). Figure 3A shows the cell-associated unspliced HIV-1 RNA data (copies/106 CD4+ T cells) for these eight individuals pre- and post-romidepsin treatment that was observed by Leth et al. (15). We defined the TUPM + CAP outcome combination that would be observed with each of the given reactivation scenario models described in the introduction (Figure 3B). We purified memory CD4+ T cells from the eight individuals pre- and post-romidepsin treatment and performed the QVTA and SPTA as illustrated in Figure 2A. We first examined the TUPM outcomes generated by the QVTA. Two out of the eight individuals (ID33 and ID42) had elevated TUPM values post-romidepsin treatment. However, we did not observe significant changes group-wide in transcriptional units at the per million proviruses level in the individuals (Figure 3C). Similarly, we saw no overall increases in the levels of transcripts generated from a single provirus after treatment with romidepsin (Figure 3D). Together, these outcomes support the third model depicted in Figure 3B. To ensure that our result was not driven by relative differences in the number of memory CD4+ T cells or proviruses analyzed, we calculated the number of cells as well as the number of proviruses analyzed per individual pre- and post-romidepsin treatment. No significant differences were observed between the total number of memory CD4+ T cells analyzed pre- and post-romidepsin treatment (p = 0.6875) (Supplementary Figure S3A). Also, no significant differences were observed in the number of proviruses analyzed pre- and post-romidepsin treatment in these individuals (p = 0.5312) (Supplementary Figures S3B,C). When examining total HIV-1 DNA levels for the same individuals, no significant changes were observed after treatment with romidepsin (Supplementary Figure S4). In conclusion, the general lack of changes in TUPM and CAP post-romidepsin reveal that few proviruses are stimulated to generate viral transcripts by this intervention and that each provirus that is activated is only mildly stimulated to produce low levels of viral transcripts in HIV-1 individuals.
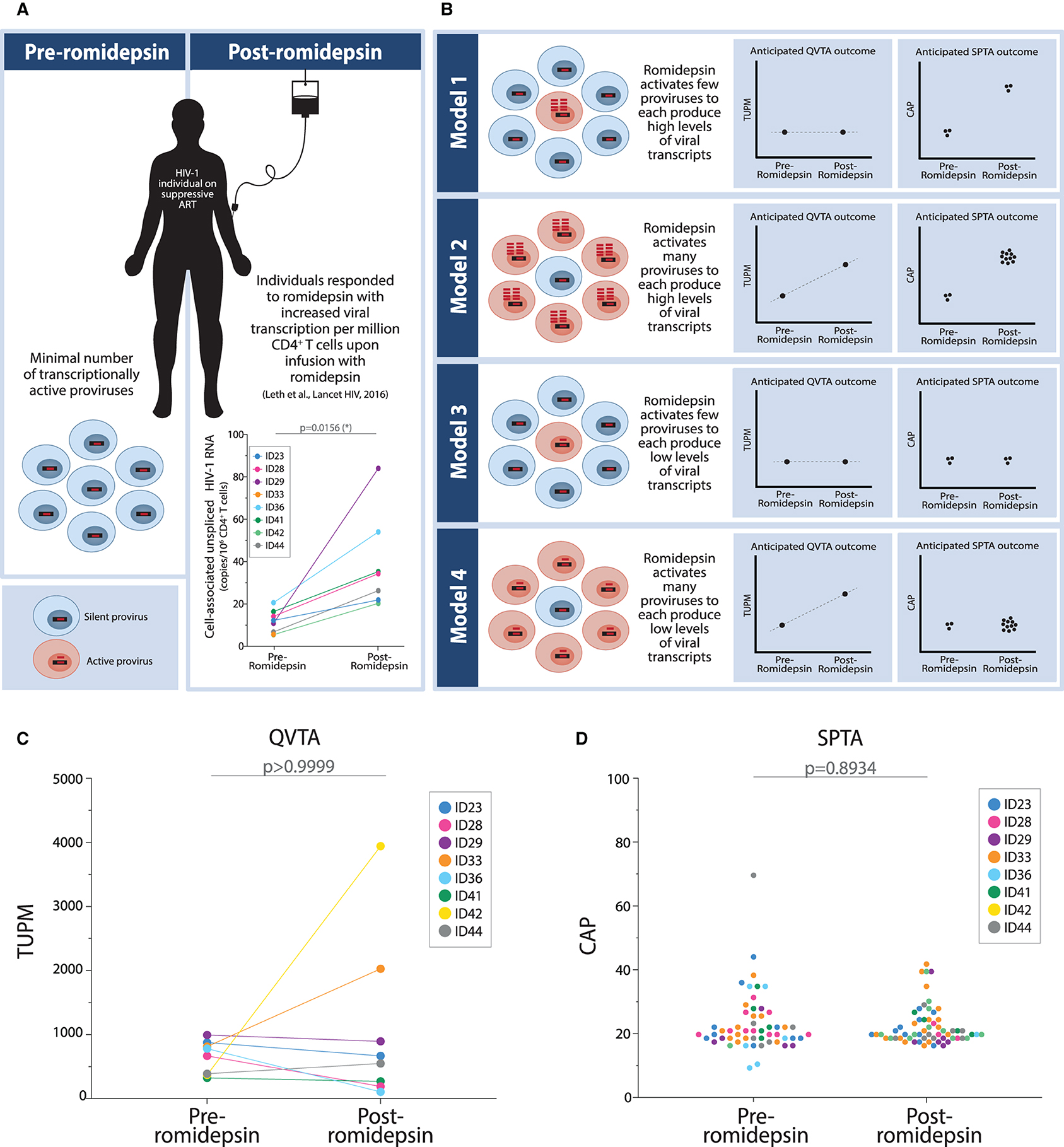
Figure 3. Treatment with romidepsin causes few proviruses to each produce low numbers of HIV-1 transcripts. (A) Graphical illustration of clinical trial participants included in this study. Specifically, participants who exhibited romidepsin-induced HIV-1 transcription were included. (B) Four models depicting possible combinations of outcomes for changes in HIV-1 transcription post-romidepsin treatment as determined using the quantitative viral transcription assays (QVTA) alongside the single-provirus transcription assays (SPTA). (C) TUPM pre- and post-romidepsin treatment in individuals on suppressive ART. We performed the Wilcoxon matched-pairs signed rank test to test for differences in TUPM between individuals before and after treatment with romidepsin. (D) CAP pre- and post-romidepsin treatment in individuals on long-term suppressive ART. We performed the Mann Whitney test to test for differences in HIV-1 RNA copies per 200 proviruses in individuals before and after receiving romidepsin infusion.
Discussion
In the HIV-1 cure research field, multiple interventional approaches have been proposed as strategies to achieve ART-free remission (7, 38, 39). Among the proposed interventions is one that has been quite extensively evaluated in clinical trials—namely the “kick and kill” latency reversal concept (8, 13). Our group and others have successfully used LRAs to “kick” latent HIV-1 such that viral transcription and virion production are induced by the intervention(s) (13, 15–17, 19, 40, 41). Two common questions raised when LRAs are used in clinical studies are: How much is each individual latent provirus actually “kicked” by a given intervention and what is the frequency of proviruses being activated? Here we report a pair of sensitive and specific ddPCR-based assays that can be used in tandem to answer these critical questions facing the HIV-1 cure field. Our two interrelated assays, the QVTA and SPTA, can be used to quantify viral transcription from single transcribing proviruses derived from HIV-1 infected individuals. Control experiments of the generated assays revealed that the QVTA and SPTA generates stable TUPM- and CAP-values, respectively, in longitudinal clinical samples in the absence of a clinical intervention. One of the most effective LRAs reported to date in the literature is romidepsin (15, 17, 19, 42). We report here that few proviruses are only moderately activated by romidepsin such that they produce low levels of viral transcripts.
We found that romidepsin-induced increases in viral transcription in individuals on suppressive ART result from a small number of transcriptional productive proviruses each producing low levels of viral transcripts. This finding agrees with the fact that romidepsin treatment did not change the viral DNA reservoir size in the same individuals we analyzed (15) (Supplementary Figure S4). Together, these results, our previously reported outcomes of analytical treatment interruption in these individuals (15) and the recent pharmacodynamic data of McMahon et al. (43) show that romidepsin alone is insufficient as a LRA to lead to ART-free remission. Importantly, the data provided herein present a mechanistic explanation for why romidepsin is insufficient as single LRA: Not only are too few proviruses activated out of latency, but the proviruses that are activated are insufficiently stimulated to generate enough viral proteins to boost a sufficiently robust immune response that will lead to the killing of individual cells harboring an activated provirus. In this context, it is relevant to discuss how a robust stimulation of proviruses might look like. In Supplementary Figure S1B we have plotted CAP for PMA-stimulated 8E5/LAV cells (one cell per well). A quarter of the positive wells containing one 8E5/LAV cell have CAP-values above 800 copies per active provirus, and more than half of the positive wells have CAP-values above 100 copies per active provirus. These data suggest how a robust LRA-mediated stimulation of proviruses may look with the SPTA in future ex vivo or in vivo clinical studies.
The conclusions presented in the previous paragraph are true at the individual cohort level. However, there is much more nuance to the interpretation when individuals are examined more closely. These nuances open doors for more investigations. For example, romidepsin reactivated many proviruses to each produce low levels of HIV-1 transcripts in individual ID33 and individual ID42, fitting with model 4. When looking into the clinical data from these two individuals, we could not identify a clinical parameter that could explain why romidepsin appears to be activating more proviruses in those two individuals. It is the paired QVTA/SPTA outcomes that identified these individuals as unique in their response to romidepsin. Using the paired QVTA/SPTA to identify such differential responders from other latency reversal clinical trials will help to focus LRA efficacy biomarker search efforts. Seeking a biomarker(s) of QVTA/SPTA robust responders to various LRAs, or combinations thereof, is fertile ground for future studies.
As with any study, there are limitations to our work. We acknowledge that our data needs to be interpreted in the light of two important aspects of HIV-1 persistence. With our current setup we are not able to infer the replication competence of the reactivated proviruses and further we are unable to account for any potential effects that clonal expansion of provirus containing cells may have on the breadth of romidepsin's impact. In addition, comparisons between data from viremic individuals and individuals on suppressive ART is complicated by the fact that short-lived HIV-1 DNA copies (such as 2LTR circles, and other un-integrated forms) are more frequent in viremic individuals. It is possible that romidepsin could have reactivated few proviruses to each become extremely transcriptionally active to produce many hundreds or thousands of HIV-1 RNA copies each. However, such an outcome would be extraordinarily rare considering that we analyzed nearly 2.5 million memory CD4+ T cells on average for each individual on suppressive ART pre- and post-romidepsin treatment as well as 11,500 proviruses on average for each of these individuals pre- and post-romidepsin treatment. With that level of coverage, we believe that our conclusions are reflective of the overall reality present in the peripheral blood of the individuals at the time of sampling. What our peripheral blood analyses cannot account for is the possibility that other anatomical sites may exhibit differential latency reversal outcomes. For example, lymphoid tissues, intestinal tissues and central nervous system tissues have been described as persistent HIV-1 reservoirs, and that viral replication here maintains local tissue reservoirs in infected individuals and non-human primates on suppressive ART (44–48). To this end, the diversity of cell types residing in the blood and in different tissues that could harbor latent HIV-1 is considered one of the major barriers to implementing a successful “kick and kill” strategy in the clinic (38). Thus, it could be important to apply our paired QVTA/SPTA in other anatomical compartments and with other cell types to gain the greatest possible insights into LRA efficacy.
Interestingly, we found that cell-associated unspliced HIV-1 RNA levels in individuals on suppressive ART seems to have an impact on the QVTA and SPTA outcomes. We found that TUPM as well as CAP in ART naïve individuals were significantly higher compared to suppressive ART—group 2 individuals who exhibited lower cell-associated unspliced HIV-1 RNA levels, while no difference was observed in TUPM or CAP between the ART naïve individuals and suppressive ART—group 1 individuals. Also, we found that CAP was higher in suppressive ART—group 1 individuals compared to suppressive ART—group 2 individuals. Besides having notably different levels of cell-associated unspliced HIV-1 RNA levels, these two groups of individuals also differed in the median time they have been on ART (Table 1). It has been shown that the half-life of the latent reservoir is 43.9 months in individuals treated with ART (49). Thus, the median time on ART might therefore explain why we see a difference in HIV-1 transcription at the individual provirus level between the two groups of individuals on suppressive ART. These data also indicate that individuals who have been on suppressive ART for long time (like the group 2 individuals) have lower levels of single-provirus transcription. This is in accordance with a recent finding showing preliminary evidence of a selection, over time, for proviruses that are in a deeper state of latency (50). Which also bolsters the hypotheses that treating with latency reactivating agents early during ART may be more effective than treating individuals that have been on suppressive ART for many years (42, 51, 52).
In conclusion, we show that our novel QVTA/SPTA can measure the response to a LRA in vivo with single cell resolution. When we applied our assays to assess the clinical impact of the LRA romidepsin, we determined that a small number of proviruses were activated to be marginally transcriptionally active. The QVTA/SPTA have the potential to be used to evaluate the efficacy of any LRA using bio banked samples from the past as well as future latency reversal trials. Gaining a comprehensive understanding of the impact of such interventions at the individual provirus level will facilitate the development of more efficacious HIV-1 cure intervention strategies.
Data Availability Statement
The original contributions presented in the study are included in the article/Supplementary Material, further inquiries can be directed to the corresponding author.
Ethics Statement
The studies involving human participants were reviewed and approved by Danish Data Protection Agency National Committee on Health Research Ethics. The patients/participants provided their written informed consent to participate in this study.
Author Contributions
PD, MT, SL, OS, and MS: conceptualization. OS, SL, RN, and JG: clinical study and collection of material from HIV-1 individuals. KK and MS: performed laboratory work and data collection. KK, MS, MT, OS, and PD: performed data analyses and interpretation of the results. KK: first draft of the manuscript. All authors critically revising the manuscript for important intellectual content.
Funding
This work was funded by Aarhus University Student Stipend (KK), Aarhus University Research Foundation (AUFF-E-2016-FLS-8-9; PD), and Aarhus University Hospital. The funders had no role in study design, data analyses, interpretation, or writing of this paper.
Conflict of Interest
The authors declare that the research was conducted in the absence of any commercial or financial relationships that could be construed as a potential conflict of interest.
Publisher's Note
All claims expressed in this article are solely those of the authors and do not necessarily represent those of their affiliated organizations, or those of the publisher, the editors and the reviewers. Any product that may be evaluated in this article, or claim that may be made by its manufacturer, is not guaranteed or endorsed by the publisher.
Acknowledgments
We thank our colleague Bente Ladegaard for her laboratory assistance on this study.
Supplementary Material
The Supplementary Material for this article can be found online at: https://www.frontiersin.org/articles/10.3389/fviro.2021.736395/full#supplementary-material
References
1. Li TS, Tubiana R, Katlama C, Calvez V, Mohand HA, Autran B. Long-lasting recovery in CD4 T-cell function and viral-load reduction after highly active antiretroviral therapy in advanced HIV-1 disease. Lancet. (1998) 351:1682–6. doi: 10.1016/S0140-6736(97)10291-4
2. Mocroft A, Vella S, Benfield T, Chiesi A, Miller V, Gargalianos P, et al. Changing patterns of mortality across Europe in individuals infected with HIV-1. Lancet. (1998) 352:1725–30. doi: 10.1016/S0140-6736(98)03201-2
3. Davey RT, Bhat N, Yoder C, Chun TW, Metcalf JA, Dewar R, et al. HIV-1 and T cell dynamics after interruption of highly active antiretroviral therapy (HAART) in individuals with a history of sustained viral suppression. Proc Natl Acad Sci USA. (1999) 96:15109–114. doi: 10.1073/pnas.96.26.15109
4. Chun T-W, Carruth L, Finzi D, Shen X, DiGiuseppe JA, Taylor H, et al. Quantification of latent tissue reservoirs and total body viral load in HIV-1 infection. Nature. (1997) 387:183–8. doi: 10.1038/387183a0
5. Chun T-W, Stuyver L, Mizell SB, Ehler LA, Mican JA, Baseler M, et al. Presence of an inducible HIV-1 latent reservoir during highly active antiretroviral therapy. Proc Nat. Acad Sci USA. (1997) 94:13193–7. doi: 10.1073/pnas.94.24.13193
6. Finzi D, Hermankova M, Pierson T, Carruth LM, Buck C, Chaisson RE, et al. Identification of a reservoir for HIV-1 in individuals on highly active antiretroviral therapy identification of a reservoir for HIV-1 in individuals on highly active antiretroviral therapy. Science. (1997) 278:1295–300. doi: 10.1126/science.278.5341.1295
7. Abner E, Jordan A. HIV “shock and kill” therapy: in need of revision. Antiviral Res. (2019) 166:19–34. doi: 10.1016/j.antiviral.2019.03.008
9. Kim Y, Anderson JL, Lewin SR. Getting the “kill” into “shock and kill”: strategies to eliminate latent HIV. Cell Host Microbe. (2018) 23:14–26. doi: 10.1016/j.chom.2017.12.004
10. Lewin SR, Rasmussen TA. Kick and kill for HIV latency. Lancet. (2020) 395:844–6. doi: 10.1016/S0140-6736(20)30264-6
11. Lichterfeld M. Reactivation of latent HIV moves shock-and-kill treatments forward. Nature. (2020) 578:42–3. doi: 10.1038/d41586-020-00010-x
12. Thorlund K, Horwitz MS, Fife BT, Lester R, Cameron DW. Landscape review of current HIV “kick and kill” cure research - some kicking, not enough killing. BMC Infect Dis. (2017) 17:1–12. doi: 10.1186/s12879-017-2683-3
13. Archin NM, Liberty AL, Kashuba AD, Choudhary SK, Kuruc JD, Crooks AM, et al. Administration of vorinostat disrupts HIV-1 latency in individuals on antiretroviral therapy. Nature. (2012) 487:482–5. doi: 10.1038/nature11286
14. Lehrman G, Hogue IB, Palmer S, Jennings C, Spina CA, Wiegand A, et al. Depletion of latent HIV-1 infection in vivo: a proof-of-concept study. Lancet. (2005) 366:549–55. doi: 10.1016/S0140-6736(05)67098-5
15. Leth S, Schleimann MH, Nissen SK, Højen JF, Olesen R, Graversen ME, et al. Combined effect of Vacc-4x, recombinant human granulocyte macrophage colony-stimulating factor vaccination, and romidepsin on the HIV-1 reservoir (REDUC): a single-arm, phase 1B/2A trial. Lancet HIV. (2016) 3:e463–72. doi: 10.1016/S2352-3018(16)30055-8
16. Rasmussen TA, Tolstrup M, Brinkmann CR, Olesen R, Erikstrup C, Solomon A, et al. Panobinostat, a histone deacetylase inhibitor, for latent virus reactivation in HIV-infected individuals on suppressive antiretroviral therapy: a phase 1/2, single group, clinical trial. Lancet HIV. (2014) 1:e13–21. doi: 10.1016/S2352-3018(14)70014-1
17. Søgaard OS, Graversen ME, Leth S, Olesen R, Brinkmann CR, Nissen SK, et al. The depsipeptide romidepsin reverses HIV-1 latency in vivo. PLoS Pathog. (2015) 11:e1005142. doi: 10.1371/journal.ppat.1005142
18. Vibholm L, Schleimann MH, Højen JF, Benfield T, Offersen R, Rasmussen K, et al. Short-course toll-like receptor 9 agonist treatment impacts innate immunity and plasma viremia in individuals with human immunodeficiency virus infection. Clin Infect Dis. (2017) 64:1686–95. doi: 10.1093/cid/cix201
19. Mothe B, Rosás-Umbert M, Coll P, Manzardo C, Puertas MC, Morón-López S, et al. HIVconsv vaccines and romidepsin in early-treated HIV-1-infected individuals: safety, immunogenicity and effect on the viral reservoir (study BCN02). Front Immunol. (2020) 11:1–15. doi: 10.3389/fimmu.2020.00823
20. Yucha RW, Hobbs KS, Hanhauser E, Hogan LE, Nieves W, Ozen MO, et al. High-throughput characterization of HIV-1 reservoir reactivation using a single-cell-in-droplet PCR assay. EBioMedicine. (2017) 20:217–29. doi: 10.1016/j.ebiom.2017.05.006
21. Leth S, Nymann R, Jørgensen S, Olesen R, Rasmussen TA, Østergaard L, et al. HIV-1 transcriptional activity during frequent longitudinal sampling in aviremic individuals on antiretroviral therapy. AIDS. (2016) 30:713–21. doi: 10.1097/QAD.0000000000000974
22. Brenchley JM, Hill BJ, Ambrozak DR, Price DA, Guenaga FJ, Casazza JP, et al. T-cell subsets that harbor human immunodeficiency virus (HIV) in vivo: implications for HIV pathogenesis. J Virol. (2004) 78:1160–8. doi: 10.1128/JVI.78.3.1160-1168.2004
23. Chomont N, El-Far M, Ancuta P, Trautmann L, Procopio FA, Yassine-Diab B, et al. HIV reservoir size and persistence are driven by T cell survival and homeostatic proliferation. Nat Med. (2009) 15:893–900. doi: 10.1038/nm.1972
24. Fromentin R, Bakeman W, Lawani MB, Khoury G, Hartogensis W, DaFonseca S, et al. CD4+ T cells expressing PD-1, TIGIT and LAG-3 contribute to HIV persistence during ART. PLoS Pathog. (2016) 12:1–19. doi: 10.1371/journal.ppat.1005761
25. Telan EFO, Samonte GMJ, Palaypayon N, Abellanosa-Tac-An IP, Leaño PSA, Tsuneki A, et al. Possible HIV transmission modes among at-risk groups at an early epidemic stage in the Philippines. J Med Virol. (2013) 85:2057–64. doi: 10.1002/jmv.23701
26. Rosenbloom DIS, Elliott O, Hill AL, Henrich TJ, Siliciano JM, Siliciano RF. Designing and interpreting limiting dilution assays: general principles and applications to the latent reservoir for human immunodeficiency virus-1. Open Forum Infect Dis. (2015) 2:1–9. doi: 10.1093/ofid/ofv123
27. Albert J, Fenyö EM. Simple, sensitive, and specific detection of human immunodeficiency virus type 1 in clinical specimens by polymerase chain reaction with nested primers. J Clin Microbiol. (1990) 28:1560–4. doi: 10.1128/jcm.28.7.1560-1564.1990
28. Chun T-W, Murray D, Justement JS, Hallahan CW, Moir S, Kovacs C, et al. Relationship between residual plasma viremia and the size of HIV proviral DNA reservoirs in infected individuals receiving effective antiretroviral therapy. J Infect Dis. (2011) 204:135–8. doi: 10.1093/infdis/jir208
29. Henrich TJ, Gallien S, Li JZ, Pereyra F, Kuritzkes RD. Low-Level Detection and Quantitation of Cellular HIV-1 DNA and 2-LTR Circles Using Droplet Digital PCR. Journal of Virological Methods, (2012) 186(1–2), 68–72. doi: 10.1016/j.jviromet.2012.08.019
30. Kiselinova M, De Spiegelaere W, Buzon MJ, Malatinkova E, Lichterfeld M, Vandekerckhove L. Integrated and total HIV-1 DNA predict ex vivo viral outgrowth. PLoS Pathog. (2016) 12:1–17. doi: 10.1371/journal.ppat.1005532
31. Lewin SR, Vesanen M, Kostrikis L, Hurley A, Duran M, Zhang L, et al. Use of real-time PCR and molecular beacons to detect virus replication in human immunodeficiency virus type 1-infected individuals on prolonged effective antiretroviral therapy. J Virol. (1999) 73:6099–103. doi: 10.1128/JVI.73.7.6099-6103.1999
32. Rouet F, Chaix M-L, Nerrienet E, Ngo-Giang-Huong N, Plantier J-C, Burgard M, et al. Impact of HIV-1 genetic diversity on plasma HIV-1 RNA quantification. J Acquired Immune Deficiency Syndr. (2007) 45:380–8. doi: 10.1097/QAI.0b013e3180640cf5
33. Saksela K, Muchmore E, Girard M, Fultz P, Baltimore D. High viral load in lymph nodes and latent human immunodeficiency virus (HIV) in peripheral blood cells of HIV-1-infected chimpanzees. J Virol. (1993) 67:7423–7. doi: 10.1128/jvi.67.12.7423-7427.1993
34. Strain MC, Lada SM, Luong T, Rought SE, Gianella S, Terry VH, et al. Highly precise measurement of HIV DNA by droplet digital PCR. PLoS ONE. (2013) 8:e55943. doi: 10.1371/journal.pone.0055943
35. Busby E, Whale AS, Bridget Ferns R, Grant PR, Morley G, Campbell J, et al. Instability of 8E5 calibration standard revealed by digital PCR risks inaccurate quantification of HIV DNA in clinical samples by qPCR. Sci Rep. (2017) 7:1–7. doi: 10.1038/s41598-017-01221-5
36. Chun T-W, Finzi D, Margolick J, Chadwick K, Schwartz D, Siliciano RF. In vivo fate of HIV-1-infected T cells: quantitative analysis of the transition to stable latency. Nat Med. (1995) 1:1284–90. doi: 10.1038/nm1295-1284
37. Phillips AN, Staszewski S, Weber R, Kirk O, Francioli P, Miller V, et al. HIV viral load response to antiretroviral therapy according to the baseline CD4 cell count and viral load. J Am Med Assoc. (2001) 286:2560–7. doi: 10.1001/jama.286.20.2560
38. Ait-Ammar A, Kula A, Darcis G, Verdikt R, De Wit S, Gautier V, et al. Current status of latency reversing agents facing the heterogeneity of HIV-1 cellular and tissue reservoirs. Front Microbiol. (2020) 10:3060. doi: 10.3389/fmicb.2019.03060
39. Denton PW, Søgaard OS, Tolstrup M. Impacts of HIV cure interventions on viral reservoirs in tissues. Front Microbiol. (2019) 10:1–7. doi: 10.3389/fmicb.2019.01956
40. Elliott JH, McMahon JH, Chang CC, Lee SA, Hartogensis W, Bumpus N, et al. Short-term administration of disulfiram for reversal of latent HIV infection: a phase 2 dose-escalation study. Lancet HIV. (2015) 2:e520–9. doi: 10.1016/S2352-3018(15)00226-X
41. Wei DG, Chiang V, Fyne E, Balakrishnan M, Barnes T, Graupe M, et al. Histone deacetylase inhibitor romidepsin induces HIV expression in CD4 T cells from individuals on suppressive antiretroviral therapy at concentrations achieved by clinical dosing. PLoS Pathog. (2014) 10:e1004071. doi: 10.1371/journal.ppat.1004071
42. Gunst JD, Tolstrup M, Rasmussen TA, Søgaard OS. The potential role for romidepsin as a component in early HIV-1 curative efforts. Expert Rev Anti Infect Ther. (2016) 14:447–50. doi: 10.1586/14787210.2016.1164031
43. McMahon DK, Zheng L, Cyktor JC, Aga E, Macatangay BJ, Godfrey C, et al. A Phase 1/2 randomized, placebo-controlled trial of romidespin in persons with HIV-1 on suppressive antiretroviral therapy. J Infect Dis. (2021) 224:648–56. doi: 10.1093/infdis/jiaa777
44. Banga R, Procopio FA, Noto A, Pollakis G, Cavassini M, Ohmiti K, et al. PD-1+ and follicular helper T cells are responsible for persistent HIV-1 transcription in treated aviremic individuals. Nat Med. (2016) 22:754–61. doi: 10.1038/nm.4113
45. Estes JD, Kityo C, Ssali F, Swainson L, Makamdop KN, Del Prete GQ, et al. Defining total-body AIDS-virus burden with implications for curative strategies. Nat Med. (2017) 23:1271–6. doi: 10.1038/nm.4411
46. Lorenzo-Redondo R, Fryer HR, Bedford T, Kim EY, Archer J, Kosakovsky Pond SL, et al. Persistent HIV-1 replication maintains the tissue reservoir during therapy. Nature. (2016) 530:51–6. doi: 10.1038/nature16933
47. Telwatte S, Lee S, Somsouk M, Hatano H, Baker C, Kaiser P, et al. Gut and blood differ in constitutive blocks to HIV transcription, suggesting tissue-specific differences in the mechanisms that govern HIV latency. PLoS Pathog. (2018) 14:e1007357. doi: 10.1371/journal.ppat.1007357
48. Valcour V, Chalermchai T, Sailasuta N, Marovich M, Lerdlum S, Suttichom D, et al. Central nervous system viral invasion and inflammation during acute HIV infection. Journal of Infectious Diseases. (2012) 206:275–82. doi: 10.1093/infdis/jis326
49. Finzi D, Blankson J, Siliciano JD, Margolick JB, Chadwick K, Pierson T, et al. Latent infection of CD4+ T cells provides a mechanism for lifelong persistence of HIV-1, even in individuals on effective combination therapy. Nat Med. (1999) 5:512–7. doi: 10.1038/8394
50. Einkaif KB. Evolutionary Dynamics of HIV Reservoir Cells via a Novel Single-Cell Multiomics Assay [Oral abstract no 155]. Conference on Retroviruses and Opportunistic Infections (CROI), Virtual CROI 2021 (2021). Available online at: http://www.croiwebcasts.org/console/player/48321?mediaType=slideVideo&
51. Gunst JD, Tolstrup M, Søgaard OS. Beyond antiretroviral therapy: early interventions to control HIV-1 infection. AIDS. (2017) 31:1665–7. doi: 10.1097/QAD.0000000000001524
Keywords: HIV-1 latency, single-provirus, digital droplet PCR, latency reversal, romidepsin
Citation: Kjær K, Leth S, Konrad CV, Gunst JD, Nymann R, Østergaard L, Søgaard OS, Schleimann MH, Tolstrup M and Denton PW (2021) Modest de novo Reactivation of Single HIV-1 Proviruses in Peripheral CD4+ T Cells by Romidepsin. Front. Virol. 1:736395. doi: 10.3389/fviro.2021.736395
Received: 05 July 2021; Accepted: 07 September 2021;
Published: 01 October 2021.
Edited by:
Maximilian Muenchhoff, Ludwig Maximilian University of Munich, GermanyReviewed by:
Francesco Roberto Simonetti, Johns Hopkins University, United StatesLuis Menendez-Arias, Consejo Superior de Investigaciones Científicas (CSIC), Spain
Copyright © 2021 Kjær, Leth, Konrad, Gunst, Nymann, Østergaard, Søgaard, Schleimann, Tolstrup and Denton. This is an open-access article distributed under the terms of the Creative Commons Attribution License (CC BY). The use, distribution or reproduction in other forums is permitted, provided the original author(s) and the copyright owner(s) are credited and that the original publication in this journal is cited, in accordance with accepted academic practice. No use, distribution or reproduction is permitted which does not comply with these terms.
*Correspondence: Kathrine Kjær, kkjaer@clin.au.dk
†These authors have contributed equally to this work and share last authorship