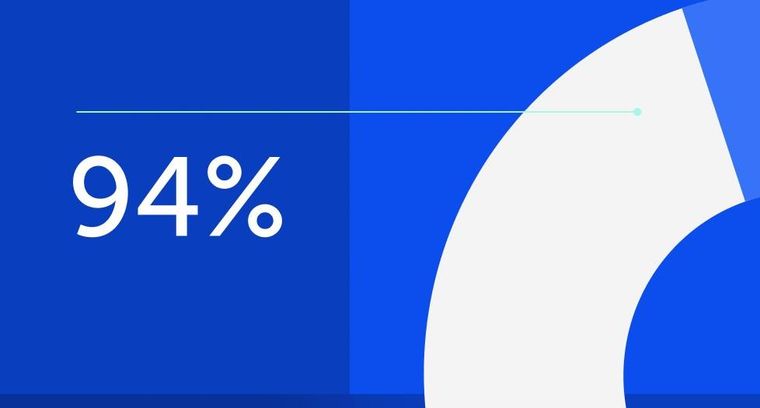
94% of researchers rate our articles as excellent or good
Learn more about the work of our research integrity team to safeguard the quality of each article we publish.
Find out more
ORIGINAL RESEARCH article
Front. Vet. Sci., 02 April 2025
Sec. Parasitology
Volume 12 - 2025 | https://doi.org/10.3389/fvets.2025.1572944
Introduction: The recent discovery of Theileria haneyi, a tick-borne hemoparasite that causes mild clinical signs of equine piroplasmosis, has added complexity to the diagnosis of this reportable disease, which is prevalent among equids globally. Knowledge gaps regarding competent tick vectors that can transmit T. haneyi and the recent outbreak of Haemaphysalis longicornis in the US has prompted us to conduct this study. Our objective was to investigate whether H. longicornis can transstadially transmit T. haneyi to horses.
Materials and methods: Haemaphysalis longicornis larvae (0.5 g) and nymphs (n = 500) were fed on a splenectomized T. haneyi-infected horse for parasite acquisition. During the tick feeding period, parasitemia was monitored using nested PCR (nPCR) and blood smear analysis. The acquisition ticks fed until repletion and were transferred to an incubator for molting. Concomitantly, red blood cells (RBCs) were collected from the acquisition horse for further infection. Freshly molted nymphs (n = 282) and adults (n = 212), 22 offsprings of the acquisition larvae and nymphs, respectively, were placed on two individual naïve spleen-intact horses for transstadial parasite transmission. Another naïve horse was inoculated with 1 mL of RBCs from the acquisition horse. After tick infestation and RBC inoculation, the transmission horses were monitored for 38 days for the presence of T. haneyi DNA in their peripheral blood using nPCR, as well as for any clinical signs of infection.
Results and discussion: The splenectomized acquisition horse developed canonical signs of acute T. haneyi infection during tick acquisition. The percentage of parasitized RBCs in the acquisition horse varied between 2.2 and 8.1% during the tick feeding stage. Out of a subset of 10 engorged larvae that fed on the acquisition horse, all ticks tested nPCR positive for T. haneyi. However, only 4 out of 10 engorged nymphs that fed on the acquisition horse tested PCR positive for T. haneyi. We found no evidence for the presence of parasite DNA in the transmission ticks or in the horse’s blood nor did we observe any clinical signs of T. haneyi infection in the transmission horses. In contrast, the horse inoculated with RBCs from the acquisition horse tested nPCR positive for T. haneyi 15 days after inoculation. It showed parasites in blood smear and developed canonical clinical signs of acute infection.
Conclusion: The findings show that H. longicornis ticks cannot transstadially transmit T. haneyi to horses.
Theileria haneyi, a recently discovered apicomplexan tick-borne hemoparasite, infects horses globally (1–4). Along with Theileria equi and Babesia caballi, T. haneyi is one of the causative agents of equine piroplasmosis, a disease with significant economic implications that affects equids, including horses, donkeys, and zebras. According to the current regulations of the World Organization for Animal Health (formerly Office International des Epizooties—OIE), equine piroplasmosis is a reportable disease that poses severe barriers to animal movement globally (5–7). While countries free from this disease, such as the US, Japan, and New Zealand, enforce strict regulations to prevent the importation of infected horses and to maintain their disease-free status, countries already affected by the disease also limit the import of infected animals to avoid the introduction of potentially diverse and virulent strains.
The severity of T. haneyi acute infection is lower than that of T. equi and B. caballi infections, and clinical signs include hemolytic anemia, fever, lethargy, petechiation, parasitemia, which is indicated by the presence of infected red blood cells (RBCs) in a blood smear, and in some cases, sudden death (3–5). Animals that survive the acute phase of the disease may later develop chronic asymptomatic infection and serve as reservoirs for tick and iatrogenic transmission of the parasite (3–5). It is important to note that vaccines against equine piroplasmosis have not yet been developed. The strategies to monitor and control the infection and disease include the use of molecular and serological diagnostics, and anti-parasitic prophylactic and therapeutic drugs (8–10).
Experimental studies have demonstrated that T. haneyi is less virulent to immunocompetent horses than T. equi and B. caballi. However, the impact of the parasite on high-performance racing horses and working equids is currently unknown (1, 11). Research has shown that T. haneyi is resistant to the most commonly used anti-parasitic therapeutic drugs, including imidocarb dipropionate, buparvaquone, tulathromycin, and diclazuril (12, 13). In addition, a previous study has shown that the co-infection of T. haneyi and T. equi decreases the efficacy of imidocarb dipropionate (14). This decrease in the efficacy of imidocarb dipropionate is highly concerning, given that it is the drug of choice for the treatment of equine piroplasmosis and is widely used for controlling the clinical signs of the disease and curing T. equi-infected equids worldwide (8, 9). As T. haneyi is considered a novel tick-borne hemoparasite of equids, little is currently known about its lifecycle (1). There are gaps in the literature regarding the knowledge of tick species that are competent in transmitting the parasite to horses.
Haemaphysalis longicornis, also known as the Asian longhorned tick, is endemic to East Asia, including countries such as China, Korea, and Japan (15–17). From the Asia-Pacific region, H. longicornis has spread to New Zealand and Australia, where it has become a major tick vector for the transmission of the Theileria orientalis Ikeda genotype, a hemoparasite with significant economic implications that infects cattle (18–23). H. longicornis is a highly aggressive, multihost tick species that can feed on a variety of mammalian hosts (24, 25). As reported in previous studies, it is a competent biological vector for several pathogens that can infect humans and animals, including hemoparasites, bacteria, and viruses (26–32). The invasion of this tick species in the US was confirmed in 2017, and it has been linked to the emerging outbreaks of T. orientalis Ikeda infections in cattle across the country (25, 33). Since then, H. longicornis has spread to several states in the eastern US, posing a major threat to the country’s public and animal health (34–38).
Considering the ability of H. longicornis to transmit the T. haneyi-related parasite T. orientalis to cattle (27) and given that this tick species feeds efficiently on horses (39, 40), we conducted a controlled experiment to investigate the ability of H. longicornis to transstadially transmit T. haneyi to horses.
All procedures in this study were performed in accordance with the protocols approved by the Institutional Animal Care and Use Committees of the University of Idaho (protocol no. 2041–26) and Washington State University (protocol no. 6982). Furthermore, all procedures adhered to the US National Institutes of Health (NIH) Guide for the Care and Use of Laboratory Animals.
In this study, the T. haneyi Eagle Pass isolate was used (1). The T. haneyi blood stabilate was prepared and horses were infected based on protocols mentioned in previous studies (11–13).
The H. longicornis tick colony maintained at the USDA-ARS Animal Disease Research Unit, Pullman, WA, United States, was used in this study. This colony comprised ticks that were collected in the field by Dr. Dana Price (Rutgers University, New Jersey) in New Jersey and kindly provided to the USDA-ARS Animal Disease Research Unit in 2019 for colony rearing. In addition, ticks from this colony had also been used for competence studies in Theileria and Babesia parasites (27, 41).
A Welsh horse chronically infected with the T. haneyi Eagle Pass isolate was used for tick parasite acquisition. The horse was infected with T. haneyi blood stabilate and monitored throughout the development of chronic infection, as previously described (14). The horse was used as an untreated control in a previous investigation (13). Prior to tick parasite acquisition, the horse was splenectomized to increase parasitemia, which was performed at the Washington State University Veterinary Teaching Hospital (WSU VTH), following standard procedures. Briefly, before the surgery, the horse was kept off feed for 24 h and water for 6–8 h and anesthesia was administered by a veterinary anesthesiologist. A skin incision of approximately 30 cm was made over the sixteenth rib beginning at the paralumbar muscles and extending distally to 5 cm ventral to the costochondral junction. The incision was extended through the subcutaneous tissues and muscles. Then, a careful stab incision was made through the periosteum and peritoneum. The apex of the spleen was exteriorized, exposing the gastrosplenic ligament and associated vessels, which were ligated and transected. The spleen was partially elevated and shifted ventrally to access the nephrosplenic ligament. The ligament was bluntly and sharply dissected off the axial splenic surface. Then, phenylephrine was injected directly into the spleen to cause splenic contraction. The hilus was double-ligated and cauterized, and the spleen was removed. Post-surgery recovery followed standard procedures at WSU VTH. Post-surgery pain was managed by the administration of non-steroidal anti-inflammatory drugs at recommended dosages. The horse was discharged from WSU VTH 1 week after the surgery. It was monitored for parasitemia by Giemsa staining of blood smear followed by microscopy analysis, T. haneyi nPCR, and evaluation of clinical signs of equine piroplasmosis. Fourteen days after splenectomy, when evident signs of parasite replication were observed in peripheral blood, the acquisition horse was infested with 0.5 g of unfed H. longicornis larvae and 500 unfed, freshly molted H. longicornis nymphs. The ticks were placed under separate cloth patches on the acquisition horse (Figure 1). Once the larvae and nymphs fed to repletion, they were collected, placed in the incubator for molting, and used for transmission steps. Furthermore, blood from the acquisition horse was collected on day 3 post-tick application. The blood was washed to remove leukocytes, and RBCs were suspended in one volume of Puck’s Saline G (Fisher Scientific) with 20% polyvinylpyrrolidone (PVP-40) (Sigma-Aldrich) and frozen in liquid nitrogen until use.
Figure 1. Schematic of the experimental design to investigate the competence of Haemaphysalis longicornis to transstadially transmit Theileria haneyi to horses. H. longicornis larvae (0.5 g) and nymphs (n = 500) were fed on a splenectomized T. haneyi-infected horse (acquisition horse). Nymphal (n = 282) and adult (212) offspring were subsequently fed on naïve horses for transmission (transmission horses 1 and 2, respectively). In addition, red blood cells (RBCs) from the acquisition horse were intravenously inoculated into transmission horse 3. Figure created using BioRender.com.
Freshly molted, unfed nymphs and adults, which were the offspring of the acquisition larvae and nymphs, respectively, were placed on two naïve, spleen-intact horses for transmission (Figure 1). A total of 282 nymphs and 212 adults were placed under a cloth patch on transmission horses 1 and 2, respectively (Figure 1). After 3 days of feeding, 10 partially fed nymphs and 10 partially fed adults were collected for PCR analysis. DNA extracted from whole individual partially fed nymphs and salivary glands (SGs) dissected from individual adults was used for this analysis. The remaining ticks fed to repletion. After tick feeding, both horses were monitored for 38 days for the presence of T. haneyi DNA in peripheral blood and signs of acute infection. In addition, 1 mL of RBCs from the acquisition horse was intravenously inoculated into another horse (transmission horse 3) (Figure 1). After RBC inoculation, transmission horse 3 was monitored for 30 days to detect T. haneyi DNA in peripheral blood and signs of acute infection.
The blood cell count of the acquisition and transmission horses was evaluated using the ProCyte One™ Hematology Analyzer (IDEXX Laboratories, Inc., Westbrook, ME, United States) in accordance with the manufacturer’s protocol. Briefly, peripheral blood was collected into Vacutainer® tubes containing EDTA (BD Company, Franklin Lakes, NJ, United States) at several time points post-splenectomy, post-tick infestation, and post-RBC inoculation. The collected whole-blood samples were mixed for approximately 5 min, and the number of total leukocytes, lymphocytes, monocytes, neutrophils, and RBCs was measured. White blood cell (WBC) populations were counted as 1,000 cells/mL of blood, and RBC populations were counted as 1,000,000 cells/mL of blood.
The presence of T. haneyi DNA in the acquisition and transmission horses and in the acquisition and transmission ticks was monitored using nested PCR (nPCR) targeting a hypothetical gene (GenBank accession number: MT896770.1), syntenic to the T. equi ema-1 gene, as described in previous studies (1, 12, 13). Briefly, DNA extraction was performed on whole-blood and tick samples using the Qiagen DNeasy® Blood & Tissue Kit (Qiagen, Germantown, MD, United States) in accordance with the manufacturer’s instructions. As positive and negative controls for nPCR, DNA obtained from a horse that had consistently tested positive for T. haneyi and sterile 1X phosphate-buffered saline (PBS) (Thermo Fisher Scientific, Waltham, MA. United States) were used, respectively, as described in previous studies (1, 2, 12, 13, 42).
The presence of amplifiable DNA in the tick samples, including larvae, nymphs, and adult SG, was evaluated using PCR by employing specific primers for the H. longicornis GAPDH gene (GenBank accession number: MW148932.1). Briefly, DNA from individual larvae, nymphs, and SG was extracted using the Qiagen DNeasy® Blood & Tissue Kit in accordance with the manufacturer’s instructions. Of each sample, 2 ul was used for PCR with 1 μM of each of the following primers: 5-tgggcgcggagtacgtggt-3 and 5-cgtgcacggtggacatgagg-3. To achieve an amplicon size of 270 bp, DreamTaq green PCR Master Mix (Thermo Fisher Scientific) was used with an annealing temperature of 55°C. The amplicon was analyzed using 1.5% agarose gel, and ChemiDoc™ Touch Imaging System (Bio-Rad, Hercules, CA, United States) was used for image acquisition and subsequent visualization. In addition, the H. longicornis GAPDH amplicon was sequenced for confirmation.
Horse blood samples were used for DNA extraction as described earlier. The samples were subjected to PCR using specific primers for equine β-actin (GenBank accession number: XM_023655002.1) to demonstrate the presence of amplifiable DNA. Of each sample, 2 μl was used for PCR with 1 μM of each of the following primers: 5-tggcatccacgaaactacct-3 and 5-tctgctggaaggtggacaat-3. To achieve an amplicon size of 248 bp, DreamTaq green PCR Master Mix (Thermo Fisher Scientific) was used with an annealing temperature of 55°C. The amplicon was analyzed using 1.5% agarose gel, and ChemiDoc™ Touch Imaging System (Bio-Rad) was used for image acquisition and subsequent visualization. In addition, the horse β-actin amplicon was sequenced for confirmation.
To investigate the competence of H. longicornis in acquiring and transmitting T. haneyi, an infected horse (i.e., the acquisition horse) with a high percentage of parasitized erythrocytes (PPE) was selected for tick acquisition. This chronically T. haneyi-infected horse was subjected to splenectomy and monitored for PPE and clinical signs of acute infection (Figures 2–4). Fourteen days after splenectomy, ticks were placed on the acquisition horse. During the tick feeding period, variations in PPE were observed, 8.1, 8.6, 2.7, and 2.2% on days 1, 3, 5, and 7 post-infestation, respectively (Figure 2A). The presence of T. haneyi-infected erythrocytes was observed in blood smear via Giemsa staining. A representative blood smear staining from the acquisition horse is shown in Figure 2B. In addition, peripheral blood of the acquisition horse was collected on day 3 post-tick infestation and used for transmission.
Figure 2. Percentage of parasitized erythrocytes (PPE) during the larval and nymphal Haemaphysalis longicornis feeding period on the acquisition horse (A). A representative blood smear Giemsa staining. Arrows indicate Theileria haneyi-infected erythrocytes (B).
Figure 3. Percentage of packed cell volume (PCV) (A) and total number of red blood cells (RBCs) (B) in the Theileria haneyi-infected acquisition horse during tick infestation. Dashed red lines represent the lower physiological limit for PCV and total RBCs in equines.
Figure 4. Total number of white blood cells (WBCs) (A), lymphocytes (B), neutrophils (C), and monocytes (D) in the Theileria haneyi-infected acquisition horse during tick infestation. Dashed red lines represent the upper and lower physiological limits for each cell population in equines.
During the tick feeding period, packed cell volume (PCV), total number of RBCs, and complete blood cell count were examined (Figure 3). PCV decreased from 27.6% on day 0 when ticks were placed on the animal to 15.3% on day 7 when all ticks had dropped. Notably, it was below the physiological values for equines, decreasing by more than 50% during the tick feeding period (Figure 3A). Similarly, the total number of RBCs decreased by nearly 50% (from 6.6 to 3.65 M/μL) during tick feeding (Figure 3B). The changes in the peripheral blood cells of the acquisition horse during the tick feeding period are shown in Figure 4. The total number of WBCs was increased above the physiological level for equines during days 1 to 7 post-tick infestation (Figure 4A). This increase was not due to the change in the number of lymphocytes as it was within the normal levels during the period (Figure 4B). Interestingly, the levels of both neutrophils and monocytes were markedly increased (Figures 4C,D). All these observations on parasitemia and blood cell alterations show that H. longicornis larvae and nymphs fed on the acquisition horse during the development of high levels of T. haneyi PPE and canonical signs of acute parasite infection.
A total of 7, 116, 82, and 70 engorged larvae were collected from the acquisition horse on days 4, 5, 6, and 7 post-infestation, respectively (Figure 5A). Regarding nymphs, a total of 100, 148, 19, and 1 engorged ticks were collected from the acquisition horse on days 4, 5, 6, and 7 post-infestation, respectively (Figure 5B). Engorged larvae and nymphs were kept in an incubator for molting and hardening and used for the transmission phase of the study.
Figure 5. The number of engorged Haemaphysalis longicornis larvae (A) and nymphs (B) collected every day post-infestation on the acquisition horse.
A portion of the engorged larvae and nymphs that fed on the acquisition horse, whose number decreased between days 4 and 6 post-infection, was evaluated using nPCR for the presence of T. haneyi DNA (Table 1). The results of the nPCR conducted with DNA extracted from 10 individual engorged larvae showed the presence of T. haneyi DNA in all ticks tested. Interestingly, the presence of parasite DNA was detected in only 4 of 10 engorged nymphs measured. In the PCR analysis, the H. longicornis GAPDH gene consistently showed the presence of amplifiable tick DNA in all samples analyzed (Table 1). Altogether, these observations show that a representative number of larvae and nymphs fed to repletion on the acquisition horse. Furthermore, nPCR results showed the presence of T. haneyi DNA in the engorged larvae and nymphs.
Table 1. Results of Theileria haneyi nPCR and Haemaphysalis longicornis GAPDH PCR in 10 acquisition larvae (L1–10) and 10 acquisition nymphs (N1–10).
Freshly molted H. longicornis nymphs (n = 282) and adults (212), offspring of T. haneyi acquisition larvae and nymphs, respectively, were placed on transmission horses 1 and 2, respectively, to investigate the transmission of the tick parasite (Figure 1). To examine the presence of T. haneyi DNA, 10 partially fed nymphs and 10 partially fed adults were collected for nPCR on day 3 of feeding. The results of nPCR using individual nymphs and individual SG dissected from adult ticks showed no evidence of the presence of T. haneyi DNA in the transmission ticks (Table 2). The presence of amplifiable DNA in the tick samples was confirmed by the PCR of the H. longicornis GAPDH gene (Table 2). A total of 79 replete nymphs were recovered from transmission horse 1, over 8 days of feeding, while 65 replete adults were obtained from transmission horse 2, over 10 days of feeding.
Table 2. Results of Theileria haneyi nPCR and Haemaphysalis longicornis GAPDH PCR in 10 transmission nymphs (N1–10) and 10 individual salivary glands (SG1–10) dissected from adult transmission ticks.
Transmission horses 1 and 2 were monitored for 38 days after tick infestation for the presence of T. haneyi DNA in peripheral blood, changes in blood cell count, and signs of acute infection. T. haneyi nPCR results showed no evidence of the parasite DNA in the peripheral blood of the transmission horses (Supplementary Tables S1, S2). Both horses’ PCV values remained within the normal range, except when the PCV of transmission horse 1 fluctuated from 28 to 29% between days 10 and 22 post-infestation and when the PCV of transmission horse 2 decreased to 22.9% on day 20 post-infestation. After these fluctuations, PCV values returned to normal levels (Supplementary Tables S1, S2). No significant increase in body temperature was observed in the transmission horses during the post-infestation monitoring period. In addition, no significant changes in the absolute number of total WBCs, lymphocytes, neutrophils, and monocytes were observed during the monitoring period (Supplementary Tables S1, S2). Together, these results demonstrate no evidence of T. haneyi transstadial transmission by H. longicornis nymphs and adults.
To investigate the presence of viable, infectious T. haneyi parasites in the blood of the acquisition horse, its RBCs were inoculated into a naïve horse (transmission horse 3) (Figure 1). After inoculation, transmission horse 3 was monitored for 30 days for signs of acute infection (Figure 1). PCR results showed the presence of T. haneyi DNA in its peripheral blood, starting from day 15 post-inoculation (Table 3). Subsequently, on day 21 post-inoculation, parasites were visualized using microscopy of blood smear. The presence of amplifiable DNA extracted from peripheral blood samples of transmission horse 3 was confirmed using endpoint PCR of the horse β-actin gene (Table 3).
Table 3. Results of Theileria haneyi nPCR, blood smear, and horse β-actin PCR in transmission horse 3 infected with blood from the transmission animal.
Theileria haneyi infection in transmission horse 3 was also confirmed by a marked decrease in PCV and total RBCs in peripheral blood, starting from day 15 post-inoculation (Figure 5A). The total number of WBCs and leukocytes showed a tendency to decrease after inoculation; however, the levels remained within the normal range for equines (Figures 6B,C). In addition, the total number of neutrophils in peripheral blood decreased below the physiological levels on days 29 and 30 post-inoculation (Figure 6D). Two marginal peaks in the number of monocytes were observed on day 3 and day 28 post-inoculation, but these levels were within the physiological range. Taken together, the results of PCR, blood smear analysis, and changes in blood cell counts demonstrated that transmission horse 3 was infected with T. haneyi following inoculation with RBCs from the acquisition horse (Figure 6).
Figure 6. Percentage of packed cell volume (PCV) (A; the left y-axis) and total number of red blood cells (RBCs) (A; the right y-axis) in transmission horse 3 following inoculation of RBCs from the Theileria haneyi acquisition horse. Panels (B–E) present the absolute numbers of white blood cells (WBCs), lymphocytes, neutrophils, and monocytes in peripheral blood, respectively, of transmission horse 3 following inoculation of RBCs from the acquisition horse. Red arrows indicate the time point that the animal became nPCR positive for T. haneyi. Dashed red lines indicate the lowest physiological levels for PCV and RBCs (A), WBCs (B), lymphocytes (C), neutrophils (D), and monocytes (E).
Studies investigating the competence of tick species in transmitting both endemic and exotic tick-borne pathogens are crucial for developing and implementing effective control strategies to protect human, livestock, and wildlife health. Such studies are especially important in light of the adaptation of arthropods to diverse environments and global interconnectivity, which significantly affect tick populations and their habitats. The recent prevalence of H. longicornis in the eastern USA represents a serious threat to public and animal health (25, 34, 35, 38, 43). H. longicornis is a major vector for several pathogens, including Anaplasma, Babesia, Borrelia, Ehrlichia, Rickettsia, Theileria, and numerous viruses, which affect several mammalian hosts (18, 44–46). This pathogen-transmitting nature of H. longicornis is attributable to important aspects of its lifecycle, such as its multihost characteristic and remarkable adaptability to environmental conditions (47, 48). It has been recently demonstrated that H. longicornis is a competent vector for transmitting the US isolate of T. orientalis genotype Ikeda to cattle, posing a serious risk to the country’s bovine industry (27, 33, 49).
This study examined T. haneyi, a recently discovered tick-borne hemoparasite of equids, and investigated the ability of H. longicornis to transstadially transmit this parasite to horses. H. longicornis larvae and nymphs were used for parasite acquisition on a splenectomized infected horse, and their respective offspring (nymphs and adults) were evaluated for parasite transmission to naïve hosts. The ticks fed efficiently to repletion on the acquisition horse, which showed high PPE during the feeding period. Engorged larvae and nymphs molted successfully into nymphs and adults, respectively, which were then used for parasite transmission. H. longicornis nymphs and adults were placed on naïve horses (transmission horses) to investigate T. haneyi transmission. Partially fed transmission nymphs and adults tested negative for nPCR for the presence of T. haneyi DNA, and the transmission horses remained healthy and tested nPCR negative for T. haneyi during the observation period. The transmission horses were monitored for 38 days post-tick infestation, which is more than twice the average prepatent time for the T. haneyi-related parasite T. equi to show signs of infection in horses following tick infestation (3–5, 50). The results of this controlled experiment showed no evidence of transstadial transmission of T. haneyi to horses via H. longicornis. In contrast, a naïve horse inoculated with RBCs from the acquisition horse developed canonical signs of acute T. haneyi infection, including anemia, the presence of parasites in blood smear, and nPCR-positive results for parasite DNA.
The observations on the acquisition horse reinforce that the spleen is a major immunological site for controlling parasite replication during the chronic phase of infection with apicomplexan hemoparasites (14, 51, 52). In the present study, the removal of the spleen in the chronically infected acquisition horse aggravated the infection, which was characterized by increased parasitemia in peripheral blood, a decrease in the total number of RBCs, and a decrease in PCV, consistent with previous studies (1, 14, 53). The spleen-intact transmission horse 3 that received RBCs from the acquisition horse showed the presence of parasite DNA, starting from day 15 post-inoculation, and developed mild but canonical signs of acute infection, which is also in line with previous studies (1, 11).
Tick vector competence is the ability of a specific tick species to acquire, maintain, and transmit a pathogen (54, 55). It is a complex process involving multiple factors related to ticks, pathogens, and susceptible hosts. First, ticks should efficiently feed on an infected host to acquire the pathogen. Second, they should maintain the pathogen through molting and transmit it by injecting their saliva during feeding on a naïve host. This process becomes even more complex with apicomplexan hemoparasites, such as Theileria spp. and Babesia spp., which undergo sexual reproduction during their stages in competent tick vectors (5, 56, 57). Moreover, H. longicornis ticks efficiently feed on a wide range of mammalian hosts, including humans and domestic and wildlife animals (23, 48). Our results reinforce this observation by showing that H. longicornis larvae, nymphs, and adults feed efficiently on horses, regardless of the equine status (either infected or naïve). Irrespective of the efficient feeding of all H. longicornis stages on horses, our results indicate that this tick species is not competent in transstadially transmitting T. haneyi to horses. Our findings showed the presence of T. haneyi DNA in all 10 tested acquisition larvae, whereas only 4 of the 10 analyzed acquisition nymphs tested positive for the parasite DNA. This finding is interesting considering that both larvae and nymphs fed simultaneously on a horse with T. haneyi parasitemia ranged from 8% down to 2% during the tick feeding period. It was beyond the scope of this study to investigate the tick and/or parasite factors associated with the ability of H. longicornis to transmit some Theileria species, such as T. orientalis, but not others, such as T. haneyi, which deserves further studies.
Theileria haneyi is genetically closely related to T. equi, with both hemoparasites infecting equids and causing similar clinical signs during acute infection, including hemolytic anemia, fever, anorexia, and prostration (1, 5, 11, 14). However, there are marked differences between these two parasites. Whereas experimental studies have shown that T. haneyi is only mildly virulent to immunocompetent horses, experimental and field data show that T. equi causes severe anemia and fever and can be lethal in some cases (1, 5, 11, 14). In addition, T. haneyi is resistant to imidocarb dipropionate, the drug of choice for treating equine piroplasmosis, whereas T. equi is susceptible to this drug, with only sporadic cases of resistance being reported (4, 8, 9, 53). Experimental studies have demonstrated that Rhipicephalus microplus ticks are competent vectors for transmitting T. equi (58, 59). However, given that R. microplus are monoxenic ticks and that horses are not natural hosts to this species, the epidemiological implications of this finding remain to be determined. Currently, no information is available on whether R. microplus is competent in transmitting T. haneyi. Therefore, considering these biological differences between T. haneyi and T. equi, which specific characteristics would allow or prevent these parasites from being transmitted by a particular tick species remains unknown. Thus, although in the present study, we showed that H. longicornis is not a competent vector for the transstadial transmission of T. haneyi, whether this tick species is a competent vector for T. equi remains to be determined. Furthermore, the present findings do not rule out the possibility that as H. longicornis becomes more widespread and adapted to new environments, such as the eastern, northern, and southern USA, it may acquire the capacity to transmit T. haneyi to equids, as well as new pathogens to humans, livestock, and wildlife.
Considering the recent invasion of H. longicornis into the eastern USA and the wide host range of this tick species, how this invasion will affect the resident tick populations and the dynamics of tick-borne diseases in humans, livestock, and wildlife remains to be determined. Without doubt, this is a multifaceted scenario that could affect already endemic diseases, such as Lyme disease (60), and facilitate the emergence of previously exotic pathogens, such as the recent outbreaks of T. orientalis Ikeda in the USA (27, 33, 34, 38). In the present study, we used 0.5 g of larvae and 500 nymphs for acquisition and 282 and 212 nymphal and adult offspring, respectively, for transmission. Our findings on tick infection status and animal health showed no evidence for the transstadial transmission of T. haneyi by H. longicornis. Although this raises several questions about the biological factors that prevent H. longicornis from being a competent vector for T. haneyi, these findings can be reassuring for stakeholders in the equine industry, considering that equids may not be at risk of infection with this Theileria species due to H. longicornis infestation. However, this conclusion is based on a controlled experiment, and constant interactions between ticks, pathogens, and mammalian hosts, including equids, may change this scenario. Therefore, maintaining robust livestock and wildlife animal health surveillance programs is essential to control tick-borne pathogens, particularly T. haneyi, T. equi, and B. caballi, the causative agents of equine piroplasmosis.
In conclusion, the findings of the present study demonstrated that H. longicornis cannot transstadially transmit the T. haneyi Eagle Pass isolate to horses. Although the acquisition horse developed high T. haneyi parasitemia in peripheral blood during tick acquisition and parasite DNA was detected in larval and nymphal acquisition ticks, no evidence was found for the competence of H. longicornis to transmit T. haneyi to horses.
The datasets presented in this study can be found in online repositories. The names of the repository/repositories and accession number(s) can be found in the article/Supplementary material.
All procedures in this study were performed according to protocols approved by the Institutional Animal Care and Use Committees of the University of Idaho (protocol # 2041–26) and Washington State University (protocol # 6982). The study was conducted in accordance with the local legislation and institutional requirements.
KP: Conceptualization, Data curation, Formal analysis, Investigation, Methodology, Supervision, Validation, Visualization, Writing – original draft, Writing – review & editing. KO: Conceptualization, Data curation, Formal analysis, Investigation, Methodology, Supervision, Validation, Visualization, Writing – original draft, Writing – review & editing. CO: Conceptualization, Data curation, Formal analysis, Investigation, Methodology, Supervision, Validation, Visualization, Writing – original draft, Writing – review & editing. LK: Conceptualization, Data curation, Formal analysis, Investigation, Methodology, Validation, Visualization, Writing – original draft, Writing – review & editing. RB: Conceptualization, Data curation, Formal analysis, Funding acquisition, Investigation, Methodology, Project administration, Resources, Supervision, Validation, Visualization, Writing – original draft, Writing – review & editing.
The author(s) declare that financial support was received for the research and/or publication of this article. The research was funded by the USDA-ARS CRIS# 2090–32000-044-000-D.
The authors thank Shelby Beckner, Sarah Therrian, Elizabeth Hart, Emma Karel, Kristin Erickson, Megan Jacks, Gavin Scoles, and Ellie Higgins for their excellent technical assistance and animal care.
The authors declare that the research was conducted in the absence of any commercial or financial relationships that could be construed as a potential conflict of interest.
The authors declare that no Gen AI was used in the creation of this manuscript.
All claims expressed in this article are solely those of the authors and do not necessarily represent those of their affiliated organizations, or those of the publisher, the editors and the reviewers. Any product that may be evaluated in this article, or claim that may be made by its manufacturer, is not guaranteed or endorsed by the publisher.
The Supplementary material for this article can be found online at: https://www.frontiersin.org/articles/10.3389/fvets.2025.1572944/full#supplementary-material
SUPPLEMENTARY TABLE S1 | Transmission horse 1.
SUPPLEMENTARY TABLE S2 | Transmission horse 2.
1. Knowles, DP, Kappmeyer, LS, Haney, D, Herndon, DR, Fry, LM, Munro, JB, et al. Discovery of a novel species, Theileria haneyi n. sp., infective to equids, highlights exceptional genomic diversity within the genus Theileria: implications for apicomplexan parasite surveillance. Int J Parasitol. (2018) 48:679–90. doi: 10.1016/j.ijpara.2018.03.010
2. Bastos, RG, Sears, KP, Dinkel, KD, Kappmeyer, L, Ueti, MW, Knowles, DP, et al. Development of an indirect ELISA to detect equine antibodies to Theileria haneyi. Pathogens. (2021) 10:270. doi: 10.3390/pathogens10030270
3. Tamzali, Y. Equine piroplasmosis: an updated review. Equine Vet. Educ. (2013) 25:590–8. doi: 10.1111/eve.12070
4. Mendoza, FJ, Pérez-Écija, A, Kappmeyer, LS, Suarez, CE, and Bastos, RG. New insights in the diagnosis and treatment of equine piroplasmosis: pitfalls, idiosyncrasies, and myths. Front Vet Sci. (2024) 11:1459989. doi: 10.3389/fvets.2024.1459989
5. Wise, LN, Pelzel-McCluskey, AM, Mealey, RH, and Knowles, DP. Equine piroplasmosis. Vet Clin North Am Equine Pract. (2014) 30:677–93. doi: 10.1016/j.cveq.2014.08.008
6. Knowles, D Jr. Equine babesiosis (piroplasmosis): a problem in the international movement of horses. Br Vet J. (1996) 152:123–6. doi: 10.1016/S0007-1935(96)80066-2
7. Brüning, A. Equine piroplasmosis an update on diagnosis, treatment and prevention. Br Vet J. (1996) 152:139–51. doi: 10.1016/S0007-1935(96)80070-4
8. Grause, JF, Ueti, MW, Nelson, JT, Knowles, DP, and Kappmeyer, LSBunn TO. Efficacy of imidocarb dipropionate in eliminating Theileria equi from experimentally infected horses. Vet J. (2013) 196:541–6. doi: 10.1016/j.tvjl.2012.10.025
9. Ueti, MW, Mealey, RH, Kappmeyer, LS, White, SN, Kumpula-McWhirter, N, Pelzel, AM, et al. Re-emergence of the apicomplexan Theileria equi in the United States: elimination of persistent infection and transmission risk. PLoS One. (2012) 7:e44713. doi: 10.1371/journal.pone.0044713
10. Vial, HJ, and Gorenflot, A. Chemotherapy against babesiosis. Vet Parasitol. (2006) 138:147–60. doi: 10.1016/j.vetpar.2006.01.048
11. Sears, KP, Kappmeyer, LS, Wise, LN, Silva, M, Ueti, MW, White, S, et al. Infection dynamics of Theileria equi and Theileria haneyi, a newly discovered apicomplexan of the horse. Vet Parasitol. (2019) 271:68–75. doi: 10.1016/j.vetpar.2019.06.009
12. Onzere, CK, Hulbert, M, Sears, KP, Williams, LBA, and Fry, LM. Tulathromycin and Diclazuril lack efficacy against Theileria haneyi, but Tulathromycin is not associated with adverse clinical effects in six treated adult horses. Pathogens. (2023) 12:453. doi: 10.3390/pathogens12030453
13. Onzere, CK, Hassan, A, Sears, K, Kappmeyer, LS, Villarino, NF, Fry, LM, et al. Transient efficacy of buparvaquone against Theileria haneyi in chronically infected horses. Parasit Vectors. (2024) 17:337. doi: 10.1186/s13071-024-06397-0
14. Sears, KP, Knowles, DP, and Fry, LM. Clinical progression of Theileria haneyi in Splenectomized horses reveals decreased virulence compared to Theileria equi. Pathogens. (2022) 11:254. doi: 10.3390/pathogens11020254
15. Morii, K, Sakamoto, Y, Watari, Y, Iijima, H, Doi, K, Morishima, K, et al. Genetic population structure of the Asian longhorned tick, Haemaphysalis longicornis, in Japan. Exp Appl Acarol. (2024) 94:8. doi: 10.1007/s10493-024-00971-y
16. Xiang, Y, He, L, Zhu, L, Xiao, C, Pan, Y, Chen, T, et al. Pathogenetic identification in ticks and yaks from Zoige County, China. Front Cell Infect Microbiol. (2024) 14:1474519. doi: 10.3389/fcimb.2024.1474519
17. Jang, H, Casel, MAB, Sg, J, Choi, JH, Gil, J, Rollon, R, et al. Seasonal dynamics of Haemaphysalis tick species as SFTSV vectors in South Korea. Microbiol Spectr. (2024) 12:e0048924. doi: 10.1128/spectrum.00489-24
18. Lakew, BT, Eastwood, S, and Walkden-Brown, SW. Epidemiology and transmission of Theileria orientalis in Australasia. Pathogens. (2023) 12:1187. doi: 10.3390/pathogens12101187
19. Hammer, JF, Emery, D, Bogema, DR, and Jenkins, C. Detection of Theileria orientalis genotypes in Haemaphysalis longicornis ticks from southern Australia. Parasit Vectors. (2015) 8:229. doi: 10.1186/s13071-015-0839-9
20. Emery, DL. Approaches to integrated parasite management (IPM) for Theileria orientalis with an emphasis on immunity. Pathogens. (2021) 10:1153. doi: 10.3390/pathogens10091153
21. Onizawa, E, and Jenkins, C. Epidemiology, clinical signs, and risk factors associated with theileriosis in Australian cattle (2006-2022). Pathogens. (2024) 13:253. doi: 10.3390/pathogens13030253
22. Lawrence, K, Gedye, K, McFadden, A, Pulford, D, Heath, A, and Pomroy, W. Review of the New Zealand Theileria orientalis Ikeda type epidemic and epidemiological research since 2012. Pathogens. (2021) 10:1346. doi: 10.3390/pathogens10101346
23. Heath, A. A history of the introduction, establishment, dispersal and management of Haemaphysalis longicornis Neumann, 1901 (Ixodida: Ixodidae) in New Zealand. NZ J Zool. (2020) 47:241–71. doi: 10.1080/03014223.2020.1772326
24. Trout Fryxell, RT, Chavez-Lindell, T, Butler, RA, and Odoi, A. Environmental variables serve as predictors of the invasive Asian longhorned tick (Haemaphysalis longicornis Neumann): an approach for targeted tick surveillance. PLoS One. (2023) 18:e0292595. doi: 10.1371/journal.pone.0292595
25. Beard, CB, Occi, J, Bonilla, DL, Egizi, AM, Fonseca, DM, Mertins, JW, et al. Multistate infestation with the exotic disease-vector tick Haemaphysalis longicornis – United States, august 2017-September 2018. MMWR Morb Mortal Wkly Rep. (2018) 67:1310–3. doi: 10.15585/mmwr.mm6747a3
26. Chu, CY, Jiang, BG, Liu, W, Zhao, QM, Wu, XM, Zhang, PH, et al. Presence of pathogenic Borrelia burgdorferi sensu lato in ticks and rodents in Zhejiang, south-East China. J Med Microbiol. (2008) 57:980–5. doi: 10.1099/jmm.0.47663-0
27. Dinkel, KD, Herndon, DR, Noh, SM, Lahmers, KK, Todd, SM, Ueti, MW, et al. A U.S. isolate of Theileria orientalis, Ikeda genotype, is transmitted to cattle by the invasive Asian longhorned tick, Haemaphysalis longicornis. Parasit Vectors. (2021) 14:157. doi: 10.1186/s13071-021-04659-9
28. Luo, LM, Zhao, L, Wen, HL, Zhang, ZT, Liu, JW, Fang, LZ, et al. Haemaphysalis longicornis ticks as reservoir and vector of severe fever with thrombocytopenia syndrome virus in China. Emerg Infect Dis. (2015) 21:1770–6. doi: 10.3201/eid2110.150126
29. Heath, A. Biology, ecology and distribution of the tick, Haemaphysalis longicornis Neumann (Acari: Ixodidae) in New Zealand. N Z Vet J. (2016) 64:10–20. doi: 10.1080/00480169.2015.1035769
30. Zhuang, L, Du, J, Cui, XM, Li, H, Tang, F, Zhang, PH, et al. Identification of tick-borne pathogen diversity by metagenomic analysis in Haemaphysalis longicornis from Xinyang, China. Infect Dis Poverty. (2018) 7:45. doi: 10.1186/s40249-018-0417-4
31. Eisen, L. Vector competence studies with hard ticks and Borrelia burgdorferi sensu lato spirochetes: a review. Ticks Tick Borne Dis. (2020) 11:101359. doi: 10.1016/j.ttbdis.2019.101359
32. Zheng, W, Umemiya-Shirafuji, R, Chen, S, Okado, K, Adjou Moumouni, PF, Suzuki, H, et al. Identification of Haemaphysalis longicornis genes differentially expressed in response to Babesia microti infection. Pathogens. (2020) 9:378. doi: 10.3390/pathogens9050378
33. Oakes, VJ, Yabsley, MJ, Schwartz, D, LeRoith, T, Bissett, C, Broaddus, C, et al. Theileria orientalis Ikeda genotype in cattle, Virginia, USA. Emerg Infect Dis. (2019) 25:1653–9. doi: 10.3201/eid2509.190088
34. Myers, SA, and Scimeca, RC. First report of Haemaphysalis longicornis (Neumann) in Oklahoma, USA. Pathogens. (2024) 13:861. doi: 10.3390/pathogens13100861
35. Adams, DR, Barbarin, AM, and Reiskind, MH. New report of Haemaphysalis longicornis (Ixodida: Ixodidae) in Mecklenburg County, Virginia from field collections. J Med Entomol. (2024) 61:1261–5. doi: 10.1093/jme/tjae090
36. Fausett, E, Kirstein, OD, Bellman, S, Long, A, Roeske, I, Cheng, C, et al. Surveillance and detection of Haemaphysalis longicornis (Acari: Ixodidae) in protected areas from Georgia, USA. J Med Entomol. (2024) 61:1071–6. doi: 10.1093/jme/tjae051
37. Eleftheriou, A, Zeiger, B, Jennings, J, and Pesapane, R. Phenology and habitat associations of the invasive Asian longhorned tick from Ohio, USA. Med Vet Entomol. (2024) 38:314–24. doi: 10.1111/mve.12719
38. Butler, RA, and Trout Fryxell, RT. Management of Haemaphysalis longicornis (Acari: Ixodidae) on a cow-calf farm in East Tennessee, USA. J Med Entomol. (2023) 60:1374–9. doi: 10.1093/jme/tjad121
39. Seo, HJ, Truong, AT, Kim, KH, Lim, JY, Min, S, Kim, HC, et al. Molecular detection and phylogenetic analysis of tick-borne pathogens in ticks collected from horses in the Republic of Korea. Pathogens. (2021) 10:1069. doi: 10.3390/pathogens10091069
40. Egizi, A, Bulaga-Seraphin, L, Alt, E, Bajwa, WI, Bernick, J, Bickerton, M, et al. First glimpse into the origin and spread of the Asian longhorned tick, Haemaphysalis longicornis, in the United States. Zoonoses Public Health. (2020) 67:637–50. doi: 10.1111/zph.12743
41. Poh, KC, Aguilar, M, Capelli-Peixoto, J, Davis, SK, and Ueti, MW. Haemaphysalis longicornis (Acari: Ixodidae) does not transmit Babesia bovis, a causative agent of cattle fever. Ticks Tick Borne Dis. (2024) 15:102374. doi: 10.1016/j.ttbdis.2024.102374
42. Onzere, CK, Fry, LM, Bishop, RP, Da Silva, M, Madsen-Bouterse, SA, Bastos, RG, et al. Theileria equi RAP-1a and RAP-1b proteins contain immunoreactive epitopes and are suitable candidates for vaccine and diagnostics development. Int J Parasitol. (2022) 52:385–97. doi: 10.1016/j.ijpara.2022.01.004
43. Rochlin, I, Benach, JL, Furie, MB, Thanassi, DG, and Kim, HK. Rapid invasion and expansion of the Asian longhorned tick (Haemaphysalis longicornis) into a new area on Long Island, New York, USA. Ticks Tick Borne Dis. (2023) 14:102088. doi: 10.1016/j.ttbdis.2022.102088
44. Dehhaghi, M, Kazemi Shariat Panahi, H, Holmes, EC, Hudson, BJ, Schloeffel, R, and Guillemin, GJ. Human tick-borne diseases in Australia. Front Cell Infect Microbiol. (2019) 9:3. doi: 10.3389/fcimb.2019.00003
45. Namjoshi, P, Lubembe, DM, Sultana, H, and Neelakanta, G. Antibody-blocking of a tick transporter impairs Anaplasma phagocytophilum colonization in Haemaphysalis longicornis ticks. Sci Rep. (2024) 14:9003. doi: 10.1038/s41598-024-59315-w
46. Herb, H, González, J, Ferreira, FC, and Fonseca, DM. Multiple piroplasm parasites (Apicomplexa: Piroplasmida) in northeastern populations of the invasive Asian longhorned tick, Haemaphysalis longicornis Neumann (Ixodida: Ixodidae), in the United States. Parasitology. (2023) 150:1063–9. doi: 10.1017/S0031182023000914
47. Butler, RA, Muller, LI, Grove, D, and Fryxell, RTT. Ecological relationships of Haemaphysalis longicornis Neumann with other tick species on wildlife hosts at cow-calf farms implementing integrated pest management in eastern Tennessee. Parasitology. (2024) 151:1001–11. doi: 10.1017/S0031182024001380
48. Nwanade, CF, Wang, M, Li, S, Yu, Z, and Liu, J. The current strategies and underlying mechanisms in the control of the vector tick, Haemaphysalis longicornis: implications for future integrated management. Ticks Tick Borne Dis. (2022) 13:101905. doi: 10.1016/j.ttbdis.2022.101905
49. Thompson, AT, White, S, Shaw, D, Egizi, A, Lahmers, K, Ruder, MG, et al. Theileria orientalis Ikeda in host-seeking Haemaphysalis longicornis in Virginia, U.S.A. Ticks Tick Borne Diseases. (2020) 11:101450. doi: 10.1016/j.ttbdis.2020.101450
50. Scoles, GA, Hutcheson, HJ, Schlater, JL, Hennager, SG, Pelzel, AM, and Knowles, DP. Equine piroplasmosis associated with Amblyomma cajennense ticks, Texas, USA. Emerg Infect Dis. (2011) 17:1903–5. doi: 10.3201/eid1710.101182
51. Goff, WL, Bastos, RG, Brown, WC, Johnson, WC, and Schneider, DA. The bovine spleen: interactions among splenic cell populations in the innate immunologic control of hemoparasitic infections. Vet Immunol Immunopathol. (2010) 138:1–14. doi: 10.1016/j.vetimm.2010.07.006
52. Suarez, CE, Alzan, HF, Silva, MG, Rathinasamy, V, Poole, WA, and Cooke, BM. Unravelling the cellular and molecular pathogenesis of bovine babesiosis: is the sky the limit? Int J Parasitol. (2019) 49:183–97. doi: 10.1016/j.ijpara.2018.11.002
53. Sears, K, Knowles, D, Dinkel, K, Mshelia, PW, Onzere, C, Silva, M, et al. Imidocarb dipropionate lacks efficacy against Theileria haneyi and fails to consistently clear Theileria equi in horses co-infected with T. Haneyi. Pathogens. (2020) 9:1035. doi: 10.3390/pathogens9121035
54. Porcelli, S, Deshuillers, PL, Moutailler, S, and Lagrée, AC. Meta-analysis of tick-borne and other pathogens: co-infection or co-detection? That is the question. Curr Res Parasitol Vector Borne Dis. (2024) 6:100219. doi: 10.1016/j.crpvbd.2024.100219
55. Phillips, P, and Sundaram, M. From invasion to outbreak: tick introductions and disease. Trends Parasitol. (2024) 41:24–7. doi: 10.1016/j.pt.2024.11.005
56. Jalovecka, M, Sojka, D, Ascencio, M, and Schnittger, L. Babesia life cycle – when phylogeny meets biology. Trends Parasitol. (2019) 35:356–68. doi: 10.1016/j.pt.2019.01.007
57. Jalovecka, M, Hajdusek, O, Sojka, D, Kopacek, P, and Malandrin, L. The complexity of piroplasms life cycles. Front Cell Infect Microbiol. (2018) 8:248. doi: 10.3389/fcimb.2018.00248
58. Ueti, MW, Palmer, GH, Kappmeyer, LS, Statdfield, M, Scoles, GA, and Knowles, DP. Ability of the vector tick Boophilus microplus to acquire and transmit Babesia equi following feeding on chronically infected horses with low-level parasitemia. J Clin Microbiol. (2005) 43:3755–9. doi: 10.1128/jcm.43.8.3755-3759.2005
59. Ueti, MW, Palmer, GH, Kappmeyer, LS, Scoles, GA, and Knowles, DP. Expression of equi merozoite antigen 2 during development of Babesia equi in the midgut and salivary gland of the vector tick Boophilus microplus. J Clin Microbiol. (2003) 41:5803–9. doi: 10.1128/JCM.41.12.5803-5809.2003
Keywords: Theileria haneyi, Haemaphysalis longicornis, Asian longhorned ticks, tick transmission, equine piroplasmosis, horses
Citation: Poh KC, Oyen K, Onzere CK, Kappmeyer LS and Bastos RG (2025) Haemaphysalislongicornis ticks are unable to transstadially transmit Theileria haneyi to horses. Front. Vet. Sci. 12:1572944. doi: 10.3389/fvets.2025.1572944
Received: 07 February 2025; Accepted: 17 March 2025;
Published: 02 April 2025.
Edited by:
Calin Mircea Gherman, University of Agricultural Sciences and Veterinary Medicine of Cluj-Napoca, RomaniaReviewed by:
Marius Stelian Ilie, Banat University of Agricultural Sciences and Veterinary Medicine, RomaniaCopyright © 2025 Poh, Oyen, Onzere, Kappmeyer and Bastos. This is an open-access article distributed under the terms of the Creative Commons Attribution License (CC BY). The use, distribution or reproduction in other forums is permitted, provided the original author(s) and the copyright owner(s) are credited and that the original publication in this journal is cited, in accordance with accepted academic practice. No use, distribution or reproduction is permitted which does not comply with these terms.
*Correspondence: Reginaldo G. Bastos, cmVnaW5hbGRvLmJhc3Rvc0B1c2RhLmdvdg==
†These authors have contributed equally to this work and share first authorship
Disclaimer: All claims expressed in this article are solely those of the authors and do not necessarily represent those of their affiliated organizations, or those of the publisher, the editors and the reviewers. Any product that may be evaluated in this article or claim that may be made by its manufacturer is not guaranteed or endorsed by the publisher.
Research integrity at Frontiers
Learn more about the work of our research integrity team to safeguard the quality of each article we publish.