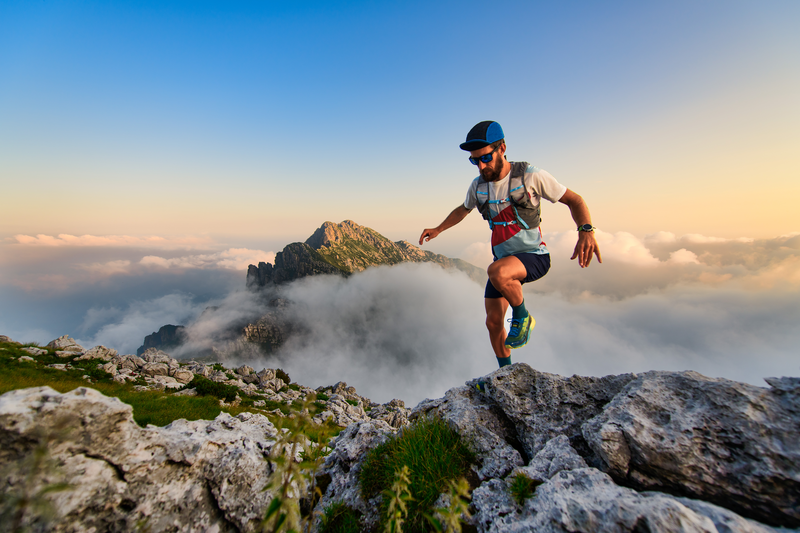
95% of researchers rate our articles as excellent or good
Learn more about the work of our research integrity team to safeguard the quality of each article we publish.
Find out more
ORIGINAL RESEARCH article
Front. Vet. Sci. , 11 February 2025
Sec. Parasitology
Volume 12 - 2025 | https://doi.org/10.3389/fvets.2025.1529253
Introduction: Ticks are important ectoparasites of livestock. Ticks and tick-borne diseases (TBDs) cause losses to the animal husbandry industry and also present a major hidden danger to public health and safety. However, the tick species and prevalence of TBDs in border regions of China, Russia, and North Korea remain unknown. The purpose of this study was to identify the tick species and tick-borne pathogens endemic in these regions.
Methods: Morphological and molecular identification of ticks was performed by microscopy and polymerase chain reaction (PCR), and the distribution of tick species, pathogen, and risk factors of infection were analyzed.
Results: In total, 1,187 ticks were collected from the border areas of 13 localities in eight cities. Five tick species were identified: Haemaphysalis longicornis (39.68%), Ixodes persulcatus (25.36%), Haemaphysalis japonica (15.50%), Dermacentor silvarum (15.42%), and Haemaphysalis concinna (4.04%). There were more female than male ticks, and nymphs were the least frequently collected. I. persulcatus was the main species in the forest environment, while H. longicornis was the main species in grasslands and animal surface. Four pathogens were detected: Rickettsia, Bartonella, Anaplasma, and Babesia.
Discussion: Pathogen detection in ticks differed significantly among the environments and between Sexes. There were significant differences in the proportion of ticks infected with Rickettsia, Bartonella, Anaplasma, and Babesia among regions, species, sexes, and environments. The results of this survey of the tick species in border areas of China, Russia, and North Korea provided a scientific basis for the prevention and control of TBDs.
Ticks are important ectoparasites of livestock and can be divided into three families: Ixodidae, Argasidae, and Nuttalliellidae (1). Although most common tick species are distributed throughout various provinces and cities in China, some are unique to a certain region, which may be related to environmental differences (2). Currently, 907 species of ticks have been reported worldwide. About 120 tick species have been identified in China alone, with most (80%) being hard ticks (3). Under normal circumstances, the body length of ticks is approximately 2–15 mm, although body size can rapidly increase while sucking blood (4). Tick development occurs as a process of incomplete metamorphosis, which can be divided into the egg, larva, nymph, and adult stages (5). Ticks can be classified based on the number of hosts and molting sites (6). In addition, ticks can readily adapt to harsh environmental conditions and some species can survive without food for long periods (7). Tick distribution is closely related to climate, soil, water, geographical environment, hosts, and other factors (8, 9). Ticks and tick-borne diseases (TBDs) not only cause harm to the animal husbandry industry, but also pose a major hidden threat to public safety and health (10). Moreover, many tick species can cause anemia and other diseases, as well as transmit various pathogens to the hosts, including Anaplasma, Bartonella, Rickettsia, Babesia, and Tick-borne encephalitis virus (11).
Rickettsia are small Gram-negative bacteria with an obligate intracellular life cycle circulating between mammalian hosts and hematophagous arthropod vectors in nature. Rickettsia are transmitted to mammalian hosts during blood feeding by infected ticks and mites (12). Rickettsia are categorized as belonging to the spotted fever group (SFG), typhus group (TG), transitional group (TRG), and ancestral group (AG) (13). The main clinical symptoms of Rickettsia infection in humans are fever, headache and nausea. Severe patients may die. Overall, the public health burden of tick-borne Rickettsioses remains significantly underestimated (14). Anaplasma belongs to the family Anaplasmataceae of order Rickettsiales. The genus Anaplasma includes Anaplasma marginale, Anaplasma centrale, Anaplasma bovis, Anaplasma ovis, and Anaplasma phagocytophilum transmitted by ticks. Different types of Anaplasma cause different clinical symptoms. A. phagocytophilum mainly cause fever, abortion, and decreased milk production (15). A. marginale has the most severe symptoms and may lead to death of livestock if not treated in time (16). A. centrale is the least pathogenic and is often used in vaccines (17). Today, Anaplasma still has effects on human and animal health at the global level. Bartonella species are gram-negative, and zoonotic bacteria belonging to the α2-subgroup of proteobacteria (18). It is spread to mammals mainly by blood-sucking arthropods. Cat scratch disease (CDS) is the most harmful disease to humans caused by Bartonella, with approximately 12,000 cases reported annually in the United States (19). Although the incidence is not high, we still need to take it seriously. Babesia is a protozoan parasite of the phylum Apicomplexa. Babesiosis is a worldwide tick-borne zoonosis caused by hemoprotozoan parasites of the genus Babesia (20). Ixodes ticks are the main vectors of Babesia spp. Clinical manifestations of Babesiosis are mainly related to the immune function of the host. Babesia bovis can have a serious impact on the livestock industry. The economic loss to China is up to 60 million dollars per year (21). Therefore, scientific prevention and control of Babesia is crucial for the livestock industry.
In terms of incidence, TBDs are the most serious vector-borne diseases in the animal husbandry and veterinary fields, and the second most common human vector-borne diseases after mosquito-borne diseases (22). Tick species and the prevalence of TBDs in border areas of China, Russia, and North Korea remain unknown. Therefore, the aim of this study was to identify the tick species and pathogens in border areas of China, Russia and North Korea, and to analyze potential risk factors, so as to provide a scientific basis for the prevention and control of TBDs.
Free ticks were collected using the cloth flag method. The collection sites consisted of grasslands and forests with lush vegetation close to a water source. When sampling, the gauze was laid flat on the grass and moved slowly by hand with a stick. At regular intervals, a magnifying glass and tweezers were used to transfer ticks from the gauze to a 15 mL centrifuge tube and relative information was recorded. Farms and villages were randomly selected. After obtaining the consent of farmers, the surfaces of livestock (cattle and sheep) were checked for the presence of ticks at the preferred attachment sites, such as behind the ear, perineum, and lower abdomen. During collection, the head of the tick was clamped with elbow tweezers and the mouthparts were gently rotated and pulled out perpendicular to the body surface. The samples were then placed into a labeled plain 15 mL centrifuge tube.
Adult ticks with relatively complete morphology of different species were selected and washed three times with sterile water to remove dust from the surface of the tick and soaked in phosphate-buffered saline (23). The morphological structures of different species of male and female ticks were observed with a stereomicroscope. Images were captured and stored following appropriate taxonomical keys (24).
All ticks were used to extract DNA, and each tick was tagged individually. The ticks were ground to powder in liquid nitrogen. Tick DNA was extracted using a tissue Genomic DNA Extraction Kit (Tiangen Biotech (Beijing) Co., Ltd., Beijing, China), in accordance with the manufacturer’s instructions, and stored at-20°C until further use.
Species-specific primers were used to amplify the 16S ribosomal DNA (16SrDNA) gene of tick (45), outer membrane protein-A (ompA) gene of Rickettsia (41), citrate synthase (gltA) gene of Bartonella (42), 16S ribosomal RNA (16SrRNA) gene of Anaplasma (40), chaperonin-containing t-complex polypeptide 1 (CCTeta) gene of Babesia (37), and major piroplasm surface protein (MPSP) gene of Theileria sinensis and Theileria orientalis (43, 44). The primers used in this study are listed in Table 1. The PCR reaction was conducted with a 25-μL reaction volume comprising 1 μL of each primer (10 pmol), 3 μL of template DNA (50–60 ng/μl), 2 μL of deoxynucleotide triphosphates (Takara Biotechnology (Dalian) Co., Ltd., Dalian, China), 2.5 μL of 10× Ex Taq buffer, 0.25 μL of Ex Taq, and 15.25 μL of distilled water. The PCR reaction conditions are presented in Table 2.
All samples verified as positive by agarose gel electrophoresis were sent to Shanghai Shenggong Biotechnology Company for Sanger sequencing. Newly obtained sequences were compared with the National Center for Biotechnology Information database1 using the Basic Local Alignment Search Tool2 and related sequences were retrieved from the GenBank database.3 Selection of representative samples for use ClustalW software4 multiple sequence alignment. Phylogenetic trees were constructed using the Maximum Likelihood method with a Tamura 3-parameter model and bootstrapping of 1,000 replicates to calculate the evolutionary relationship using Molecular Evolutionary Genetics Analysis software (25).5
Prism9 software (GraphPad Software, LLC, San Diego, CA, United States) was used for statistical analysis of tick-borne pathogen infections under different conditions. A Fisher score algorithm was used to select the optimal model. Univariate logistic regression was used to identify potential risk factors. A probability (p) value <0.05 was considered statistically significant. The odds ratio (OR) and 95% confidence interval (CI) were calculated to explore the correlation between the prevalence of pathogens and different factors. Ref represents the reference value for each set of data. Relevant data were expressed with reference to Zhao et al. (26).
In total, 1,187 ticks, were collected from 2020 to 2021 among eight counties of border areas of China (Hunchun, Yanji, Tumen, Longjing, Dunhua, Helong, Wangqing, and Antu), Russia, and North Korea (Figure 1). Of the 1,187 ticks, 632 were female, 376 were male, and 179 were nymphs. Regarding the environments, 343 ticks were collected in forests, 351 in grasslands, and 493 on animal surfaces. According to the identification results, there were three genera and five species of ticks in border areas of China, Russia, and North Korea, which included 471 Haemaphysalis longicornis, 184 Haemaphysalis japonica, 48 Haemaphysalis concinna, 301 Ixodes persulcatus, and 183 Dermacentor silvarum with proportions of 39.68, 15.50, 4.04, 25.36, and 15.42%, respectively. Haemaphysalis accounted for 59.22%, indicating that it was the dominant tick genus in border areas of China, Russia, and North Korea.
Figure 1. Map of in border areas of China, Russia and North Korea. Gray ranges represent sampling areas. Black dots represent sampling points.
The average infection rate of Rickettsia, Bartonella, Anaplasma, and Babesia was 48.78, 22.91, 35.05, and 5.14%, respectively. Among these pathogens, four were detected in Hunchun, Wangqing, Tumen, Dunhua and Longjing. Anaplasma was not detected in Yanji. Rickettsia and Bartonella were only detected in Helong and Antu. Four pathogens were detected in H. longicornis, H. japonica, and I. persulcatus, while Rickettsia, Bartonella, and Anaplasma were found in D. silvarum, and Bartonella and Anaplasma were identified in H. concinna. Bartonella was confirmed in all tick species. No T. sinensis and T. orientalis were detected in this survey (Table 3).
The Rickettsia sequences were compared with known sequences in the GenBank database. Three genotypes of Rickettsia were detected in ticks in border areas of China, Russia, and North Korea: Rickettsia raoultii, Candidatus rickettsia jingxinensis and Candidatus rickettsia tarasevichiae. Among these, the YB-BJ-4 (PQ487798) strains were located in the same branch as isolates from India (MN537561), Sichuan province, China (MF590726), and Dandong, China (MH177456). The YB-BJ-5 (PQ487799) and YB-BJ-6 (PQ487800) strains were situated on the same branch as isolates from Siberia (MK304548), Turkey (MG920563), and Xinjiang, China (KU723511). The YB-BJ-2 (PQ487796) and YB-BJ-3 (PQ487797) strains were highly homologous and on the same branch with isolates from Harbin, China (MT019661), Mudanjiang, China (KF008247), and Hokkaido, Japan (LC379461) (Figure 2). The Bartonella gltA gene sequence obtained from this study (PQ487795) formed one clade with the Korea (MT362935) and Shandong province, China (KX655838) (Figure 3). Also, the Anaplasma sequence from this study (PQ461353) was compared with known Anaplasma sequences in the GenBank database. The isolates of Anaplasma capra from Luoyang, China (MT799937), Shanxi, China (MG869594), and Korea were located on the same branch and had the highest homology (Figure 4). Lastly, the Babesia sequence (PQ487801) was compared with known Babesia ovata sequences in the GenBank database. The isolate obtained in this study clustered with sequences from Japan (AB367928) with high homology (Figure 5).
Figure 2. Phylogenetic analysis of Rickettsia based on ompA (530 bp). The phylogenetic trees were constructed by maximum-likelihood method and Tamura 3-parameter model with 1,000 bootstrap replications. The sequences obtained in this study are indicated with red color. The sequences of Rickettsia felis (AY727036) were included as outgroup.
Figure 3. Phylogenetic analysis of Bartonella based on gltA (356 bp). The phylogenetic trees were constructed by maximum-likelihood method and Tamura 3-parameter model with 1,000 bootstrap replications. The sequences obtained in this study are indicated with red color. The sequences of Bartonella grahamii (DQ334256) were included as outgroup.
Figure 4. Phylogenetic analysis of Anaplasma based on 16SrRNA (426 bp). The phylogenetic trees were constructed by maximum-likelihood method and Tamura 3-parameter model with 1,000 bootstrap replications. The sequences obtained in this study are indicated with red color. The sequences of Rickettsia slovaca (NR179179) were included as outgroup.
Figure 5. Phylogenetic analysis of Babesia based on CCTeta (1,008 bp). The phylogenetic trees were constructed by maximum-likelihood method and Tamura 3-parameter model with 1,000 bootstrap replications. The sequences obtained in this study are indicated with red color. The sequences of Theileria sergenti (AY727036) were included as outgroup.
Our results suggest that regionally, ticks from four regions, Hunchun, Wangqing, Dunhua, and Longjing, may be more likely to carry Rickettsia; ticks from Yanji, Dunhua, and Longjing had a higher detection rate of Bartonella; ticks from two regions, Hunchun and Dunhua, were more likely to be infected with Anaplasma; and there was no significant difference in the distribution of Babesia across regions. When analyzed from the perspective of tick species, H. longicornis, I. persulcatus, and D. silvarum are more likely to carry Rickettsia and Anaplasma; Bartonella is more likely to be present in all four species of ticks except H. longicornis; and for the Babesia, H. longicornis is a likely potential vector. The sex of the tick is also an important factor in the prevalence of TBDs. Our study found that female ticks were more likely to carry Rickettsia, Bartonella, and Babesia; male ticks were more likely to carry Anaplasma. Finally, analyzing the collection environment we found that ticks from animal body surfaces are more likely to carry pathogens compared to the natural environment. In summary, region, tick species, sex, and collection environment may be potential risk factors for TBDs transmission (Tables 4–7).
China has a vast border with many countries. The border between China, North Korea, and Russia lies in northeast China. Because the forest hydrology resources in this area are very rich, the endemic tick species have gradually diversified. Ticks and other vectors in the border zone can freely migrate to another country through a variety of routes, which may increase the risk of tick-borne diseases. In this study, 1,187 ticks collected from eight counties and cities in border areas of China, Russia, and North Korea were classified and analyzed. Among the five identified species, Haemaphysalis were the dominant tick species. H. longicornis was the most commonly detected species in this survey. H. longicornis, commonly known as the New Zealand cattle tick, is found mainly in East Asia and the Pacific region (27), and more recently in the United States and other countries in the Americas. H. longicornis can parasitize most warm-blooded animals, including humans and domestic animals, and spread a variety of pathogens (28), thus posing major hidden dangers to public safety and the animal husbandry industry.
In this study, three types of Rickettsia were detected. In fact, R. raoultii and C. rickettsia tarasevichiae have been endemic along the China-Russia border areas in recent years. Related studies have shown that R. raoultii and C. rickettsia tarasevichiae were detected in I. persulcatus and D. silvarum along the China-Russia border areas in 2014 (29, 30). This is almost consistent with the results of our survey. Notably, in previous studies, C. rickettsia jingxinensis have been reported mainly in Southwestern China and Korea (31, 32). The C. rickettsia jingxinensis detected in this survey were also consistent with the above areas in terms of their affinities. This suggests that there is potential for the spread of C. rickettsia jingxinensis to the border areas. Bartonella is widely prevalent around the world, and usually lice and fleas are considered to be the main vectors of Bartonella (33). Whether ticks are capable of transmitting Bartonella remains controversial. In 2022, some researchers from Portugal surveyed 268 ticks in Portugal and found that none of the ticks had infected Bartonella (34). However, a total of 272 Bartonella infections were detected in 1,187 ticks in our survey, indicating that ticks do have the ability to carry Bartonella. It remains to be investigated whether ticks can transmit Bartonella to their hosts through blood-sucking. A. capra is an emerging zoonotic tick-borne pathogen with a broad host range, including many mammals. In 2012, A. capra was detected in goats in China. Although current studies are not sufficient, domestic ruminants are considered the main host (35). Prior to this, A. capra was mainly prevalent in south-central China. A. capra have been reported to be detected in H. longicornis in Hubei Province, China, with a positivity rate of 1.32% (36). This is the first report of A. capra detected at the border areas of China, Russia, and North Korea. Gene sequences were in the same clade as the isolates from Luoyang and Shanxi. The positivity rate in this survey was significantly higher than in previous studies, a result that reminds us to pay close attention to the prevalence of A. capra. B. ovata is more frequently reported in Japan (37). The isolates from this investigation showed the highest homology with isolates from Japan. There are fewer reports on the epidemiology of B. ovata. It has been reported that 646 bovine bloods from various regions of China were positive for B. ovata at a rate of 1.5% (38). The positivity rate of B. ovata was also low in this survey. There are many reasons for this phenomenon, but of course scientific prevention and control is essential.
Our survey identified four potential risk factors that influence the prevalence of TBDs. Tick species are one of the most important factors in the prevalence of TBDs. Different species of ticks can carry different pathogens. It has been reported that H. longicornis can carry up to 44 pathogens. It’s one of the tick species that carries the highest number of pathogens (39). Our study found similar problems. Among the ticks we collected, H. longicornis was the most abundant and infected with four pathogens. The relationship between region and tick species is inextricably linked, and the distribution of ticks is significantly regional. In China, tick species are more abundant in the Northwestern and Southwestern regions, TBDs epidemics are also more severe. Although the samples were collected only in the border area of Northeast China, we can see from the results that some species of ticks were detected only in specific areas. Interestingly, sex and collection environment were also found to be risk factors for TBDs, and although the exact reasons for this are unclear, this phenomenon deserves to be studied in depth.
Haemaphysalis are the dominant tick genus in border areas of China, Russia, and North Korea. Four pathogens (Rickettsia, Bartonella, Anaplasma, and Babesia) were detected in the tick species collected in this study. Based on our results, scientific exclusion of potential risk factors may provide a new idea for controlling the spread of tick-borne diseases. These findings provide epidemiological data to support the prevention and control of ticks and tick-borne diseases in the border region of China, Russia, and North Korea.
The datasets presented in this study can be found in online repositories. The names of the repository/repositories and accession number(s) can be found in the article/Supplementary material.
PM: Conceptualization, Data curation, Writing – original draft, Writing – review & editing. JS: Data curation, Writing – original draft. SZ: Data curation, Writing – review & editing. ZM: Data curation, Formal analysis, Supervision, Writing – review & editing. YM: Writing – review & editing. ZT: Supervision, Writing – review & editing. ZW: Writing – review & editing. SL: Writing – review & editing. FZ: Writing – review & editing. ML: Formal analysis, Writing – review & editing. LW: Methodology, Writing – review & editing. LJ: Conceptualization, Data curation, Funding acquisition, Investigation, Methodology, Project administration, Resources, Supervision, Visualization, Writing – original draft, Writing – review & editing.
The author(s) declare that financial support was received for the research, authorship, and/or publication of this article. This research was supported by the Scientific Research and Innovation Team Project of Yanbian University and Jilin Province (no. 20200301034RQ) and supported by the 111 Project (D20034) and supported by State Key Laboratory for Diagnosis and Treatment of Severe Zoonotic Infectious Diseases, Jilin University.
The authors declare that the research was conducted in the absence of any commercial or financial relationships that could be construed as a potential conflict of interest.
The authors declare that no Gen AI was used in the creation of this manuscript.
All claims expressed in this article are solely those of the authors and do not necessarily represent those of their affiliated organizations, or those of the publisher, the editors and the reviewers. Any product that may be evaluated in this article, or claim that may be made by its manufacturer, is not guaranteed or endorsed by the publisher.
1. ^https://www.ncbi.nlm.nih.gov/
2. ^https://blast.ncbi.nlm.nih.gov/Blast.cgi
3. ^https://www.ncbi.nlm.nih.gov/genbank/
5. ^v.11.0; https://www.megasoftware.net/
1. Nava, S, Guglielmone, AA, and Mangold, AJ. An overview of systematics and evolution of ticks. Front Biosci (Landmark Ed). (2009) 14:2857–77. doi: 10.2741/3418
2. Horak, IG, Camicas, JL, and Keirans, JE. The Argasidae, Ixodidae and Nuttalliellidae (Acari: Ixodida): a world list of valid tick names. Exp Appl Acarol. (2002) 28:27–54. doi: 10.1023/a:1025381712339
3. Lu, X, Lin, XD, Wang, JB, Qin, XC, Tian, JH, Guo, WP, et al. Molecular survey of hard ticks in endemic areas of tick-borne diseases in China. Ticks Tick Borne Dis. (2013) 4:288–96. doi: 10.1016/j.ttbdis.2013.01.003
4. Song, R, Ma, Y, Hu, Z, Li, Y, Li, M, Wu, L, et al. MaxEnt modeling of Dermacentor marginatus (Acari: Ixodidae) distribution in Xinjiang, China. J Med Entomol. (2020) 57:1659–67. doi: 10.1093/jme/tjaa063
5. Brophy, M, Walker, KR, Adamson, JE, and Ravenscraft, A. Tropical and temperate lineages of Rhipicephalus sanguineus s.l. ticks (Acari: Ixodidae) host different strains of Coxiella-like endosymbionts. J Med Entomol. (2022) 59:2022–9. doi: 10.1093/jme/tjac132
6. Chen, Z, and Liu, J. A review of argasid ticks and associated pathogens of China. Front Vet Sci. (2022) 9:865664. doi: 10.3389/fvets.2022.865664
7. Mansfield, KL, Schilling, M, Sanders, C, Holding, M, and Johnson, N. Arthropod-borne viruses of human and animal importance: overwintering in temperate regions of Europe during an era of climate change. Microorganisms. (2024) 12:1307. doi: 10.3390/microorganisms12071307
8. McCoy, KD, Toty, C, Dupraz, M, Tornos, J, Gamble, A, Garnier, R, et al. Climate change in the Arctic: testing the poleward expansion of ticks and tick-borne diseases. Glob Chang Biol. (2023) 29:1729–40. doi: 10.1111/gcb.16617
9. Chisu, V, Giua, L, Bianco, P, Masala, G, Sechi, S, Cocco, R, et al. Molecular survey of Hepatozoon canis infection in domestic dogs from Sardinia, Italy. Vet Sci. (2023) 10:640. doi: 10.3390/vetsci10110640
10. Barker, SC, and Walker, AR. Ticks of Australia. The species that infest domestic animals and humans. Zootaxa. (2014) 3816:1–144. doi: 10.11646/zootaxa.3816.1.1
11. Rodriguez, Y, Rojas, M, Gershwin, ME, and Anaya, JM. Tick-borne diseases and autoimmunity: a comprehensive review. J Autoimmun. (2018) 88:21–42. doi: 10.1016/j.jaut.2017.11.007
12. Kim, HK. Rickettsia-host-tick interactions: knowledge advances and gaps. Infect Immun. (2022) 90:e0062121. doi: 10.1128/iai.00621-21
13. Gillespie, JJ, Williams, K, Shukla, M, Snyder, EE, Nordberg, EK, Ceraul, SM, et al. Rickettsia phylogenomics: unwinding the intricacies of obligate intracellular life. PLoS One. (2008) 3:e2018. doi: 10.1371/journal.pone.0002018
14. Biswal, M, Krishnamoorthi, S, Bisht, K, Sehgal, A, Kaur, J, Sharma, N, et al. Rickettsial diseases: not uncommon causes of acute febrile illness in India. Trop med. Infect Dis. (2020) 5:59. doi: 10.3390/tropicalmed5020059
15. Woldehiwet, Z. The natural history of Anaplasma phagocytophilum. Vet Parasitol. (2010) 167:108–22. doi: 10.1016/j.vetpar.2009.09.013
16. Kocan, KM, de la Fuente, J, Blouin, EF, Coetzee, JF, and Ewing, SA. The natural history of Anaplasma marginale. Vet Parasitol. (2010) 167:95–107. doi: 10.1016/j.vetpar.2009.09.012
17. Kolo, A. Anaplasma species in Africa-a century of discovery: a review on molecular epidemiology, genetic diversity, and control. Pathogens. (2023) 12:702. doi: 10.3390/pathogens12050702
18. Deng, H, Le Rhun, D, Buffet, JP, Cotte, V, Read, A, Birtles, RJ, et al. Strategies of exploitation of mammalian reservoirs by Bartonella species. Vet Res. (2012) 43:15. doi: 10.1186/1297-9716-43-15
19. Nelson, CA, Saha, S, and Mead, PS. Cat-scratch disease in the United States, 2005-2013. Emerg Infect Dis. (2016) 22:1741–6. doi: 10.3201/eid2210.160115
20. Vannier, EG, Diuk-Wasser, MA, Ben Mamoun, C, and Krause, PJ. Babesiosis. Infect Dis Clin N Am. (2015) 29:357–70. doi: 10.1016/j.idc.2015.02.008
21. Bal, MS, Mahajan, V, Filia, G, Kaur, P, and Singh, A. Diagnosis and management of bovine babesiosis outbreaks in cattle in Punjab state. Vet World. (2016) 9:1370–4. doi: 10.14202/vetworld.2016.1370-1374
22. Wikel, SK. Tick-host-pathogen systems immunobiology: an interactive trio. Front Biosci (Landmark Ed). (2018) 23:265–83. doi: 10.2741/4590
23. Li, J, Zhang, S, Liang, W, Zhao, S, Wang, Z, Li, H, et al. Survey of tick species and molecular detection of selected tick-borne pathogens in Yanbian, China. Parasite. (2022) 29:38. doi: 10.1051/parasite/2022039
24. Keirans, JE, and Litwak, TR. Pictorial key to the adults of hard ticks, family Ixodidae (Ixodida: Ixodoidea), east of the Mississippi River. J Med Entomol. (1989) 26:435–48. doi: 10.1093/jmedent/26.5.435
25. Alkathiri, B, Lee, S, Ahn, K, Cho, YS, Youn, SY, Seo, K, et al. 16S rRNA metabarcoding for the identification of tick-borne bacteria in ticks in the Republic of Korea. Sci Rep. (2024) 14:19708. doi: 10.1038/s41598-024-70815-7
26. Zhao, S, Wang, H, Zhang, S, Xie, S, Li, H, Zhang, X, et al. First report of genetic diversity and risk factor analysis of equine piroplasm infection in equids in Jilin, China. Parasit Vectors. (2020) 13:459. doi: 10.1186/s13071-020-04338-1
27. Gunton, AL, and Jenkins, SJ. Chemical softness in aromatic adsorption: benzene, nitrobenzene and anisole on Pt111. J Phys Chem A. (2024) 128:6296–304. doi: 10.1021/acs.jpca.4c02214
28. Beard, CB, Occi, J, Bonilla, DL, Egizi, AM, Fonseca, DM, Mertins, JW, et al. Multistate infestation with the exotic disease-vector tick Haemaphysalis longicornis - United States, august 2017-September 2018. MMWR Morb Mortal Wkly Rep. (2018) 67:1310–3. doi: 10.15585/mmwr.mm6747a3
29. Wen, J, Jiao, D, Wang, JH, Yao, DH, Liu, ZX, Zhao, G, et al. Rickettsia raoultii, the predominant Rickettsia found in Dermacentor silvarum ticks in China-Russia border areas. Exp Appl Acarol. (2014) 63:579–85. doi: 10.1007/s10493-014-9792-0
30. Yi, S, Hongrong, J, Wuchun, C, Weiming, F, Wendong, J, and Xin, W. Prevalence of Candidatus Rickettsia tarasevichiae-like Bacteria in Ixodid ticks at 13 sites on the Chinese-Russian border. J Med Entomol. (2014) 51:1304–7. doi: 10.1603/ME13189
31. Park, HJ, Kim, J, Choi, YJ, Kim, HC, Klein, TA, Chong, ST, et al. Tick-borne rickettsiae in Midwestern region of Republic of Korea. Acta Trop. (2021) 215:105794. doi: 10.1016/j.actatropica.2020.105794
32. Lu, M, Meng, C, Zhang, B, Wang, X, Tian, J, Tang, G, et al. Prevalence of spotted fever group Rickettsia and Candidatus Lariskella in multiple tick species from Guizhou Province, China. Biomol Ther. (2022) 12:701. doi: 10.3390/biom12111701
33. Spitalska, E, Minichova, L, Hamsikova, Z, Stanko, M, and Kazimirova, M. Bartonella, Rickettsia, Babesia, and Hepatozoon species in fleas (Siphonaptera) infesting small mammals of Slovakia (Central Europe). Pathogens. (2022) 11:886. doi: 10.3390/pathogens11080886
34. Torrejon, E, Sanches, GS, Moerbeck, L, Santos, L, Andre, MR, Domingos, A, et al. Molecular survey of Bartonella species in stray cats and dogs, humans, and questing ticks from Portugal. Pathogens. (2022) 11:749. doi: 10.3390/pathogens11070749
35. Altay, K, and Erol, USahin OF. Anaplasma capra: a new emerging tick-borne zoonotic pathogen. Vet Res Commun. (2024) 48:1329–40. doi: 10.1007/s11259-024-10337-9
36. Tang, J, Xu, J, Liu, XH, Lv, FZ, Yao, QJ, Zhou, XF, et al. Prevalence and genetic diversity of Anaplasma and Ehrlichia in ticks and domesticated animals in Suizhou County, Hubei Province, China. Sci Rep. (2024) 14:12621. doi: 10.1038/s41598-024-63267-6
37. Sivakumar, T, Tattiyapong, M, Okubo, K, Suganuma, K, Hayashida, K, Igarashi, I, et al. PCR detection of Babesia ovata from questing ticks in Japan. Ticks Tick Borne Dis. (2014) 5:305–10. doi: 10.1016/j.ttbdis.2013.12.006
38. Niu, Q, Liu, Z, Yu, P, Yang, J, Abdallah, MO, Guan, G, et al. Genetic characterization and molecular survey of Babesia bovis, Babesia bigemina and Babesia ovata in cattle, dairy cattle and yaks in China. Parasit Vectors. (2015) 8:518. doi: 10.1186/s13071-015-1110-0
39. Zhao, GP, Wang, YX, Fan, ZW, Ji, Y, Liu, MJ, Zhang, WH, et al. Mapping ticks and tick-borne pathogens in China. Nat Commun. (2021) 12:1075. doi: 10.1038/s41467-021-21375-1
40. Yang, J, Liu, Z, Niu, Q, Liu, J, Xie, J, Chen, Q, et al. Evaluation of different nested PCRs for detection of Anaplasma phagocytophilum in ruminants and ticks. BMC Vet Res. (2016) 12:35. doi: 10.1186/s12917-016-0663-2
41. Jia, N, Jiang, JF, Huo, QB, Jiang, BG, and Cao, WC. Rickettsia sibirica subspecies sibirica BJ-90 as a cause of human disease. N Engl J Med. (2013) 369:1176–8. doi: 10.1056/NEJMc1303625
42. Qin, XR, Han, HJ, Han, FJ, Zhao, FM, Zhang, ZT, Xue, ZF, et al. Rickettsia japonica and novel Rickettsia species in ticks, China. Emerg Infect Dis. (2019) 25:992–5. doi: 10.3201/eid2505.171745
43. Liu, A, Guan, G, Liu, Z, Liu, J, Leblanc, N, Li, Y, et al. Detecting and differentiating Theileria sergenti and Theileria sinensis in cattle and yaks by PCR based on major piroplasm surface protein (MPSP). Exp Parasitol. (2010) 126:476–81. doi: 10.1016/j.exppara.2010.05.024
44. Ota, N, Mizuno, D, Kuboki, N, Igarashi, I, Nakamura, Y, Yamashina, H, et al. Epidemiological survey of theileria orientalis infection in grazing cattle in the eastern part of Hokkaido, Japan. Nippon Juigaku Zasshi. (2009) 71:937–44. doi: 10.1292/jvms.71.937
Keywords: tick species, tick-borne diseases, risk factors, border areas of China, tick-borne pathogen distribution
Citation: Min P, Song J, Zhao S, Ma Z, Meng Y, Tang Z, Wang Z, Lin S, Zhao F, Liu M, Wang L and Jia L (2025) Tick species, tick-borne pathogen distribution and risk factor analysis in border areas of China, Russia and North Korea. Front. Vet. Sci. 12:1529253. doi: 10.3389/fvets.2025.1529253
Received: 16 November 2024; Accepted: 28 January 2025;
Published: 11 February 2025.
Edited by:
Reginaldo G. Bastos, Agricultural Research Service (USDA), United StatesReviewed by:
Yongchang Li, Xinjiang Agricultural University, ChinaCopyright © 2025 Min, Song, Zhao, Ma, Meng, Tang, Wang, Lin, Zhao, Liu, Wang and Jia. This is an open-access article distributed under the terms of the Creative Commons Attribution License (CC BY). The use, distribution or reproduction in other forums is permitted, provided the original author(s) and the copyright owner(s) are credited and that the original publication in this journal is cited, in accordance with accepted academic practice. No use, distribution or reproduction is permitted which does not comply with these terms.
*Correspondence: Lijun Jia, bGlqdW5qaWExMDE1QHNvaHUuY29t
†These authors share first authorship
Disclaimer: All claims expressed in this article are solely those of the authors and do not necessarily represent those of their affiliated organizations, or those of the publisher, the editors and the reviewers. Any product that may be evaluated in this article or claim that may be made by its manufacturer is not guaranteed or endorsed by the publisher.
Research integrity at Frontiers
Learn more about the work of our research integrity team to safeguard the quality of each article we publish.