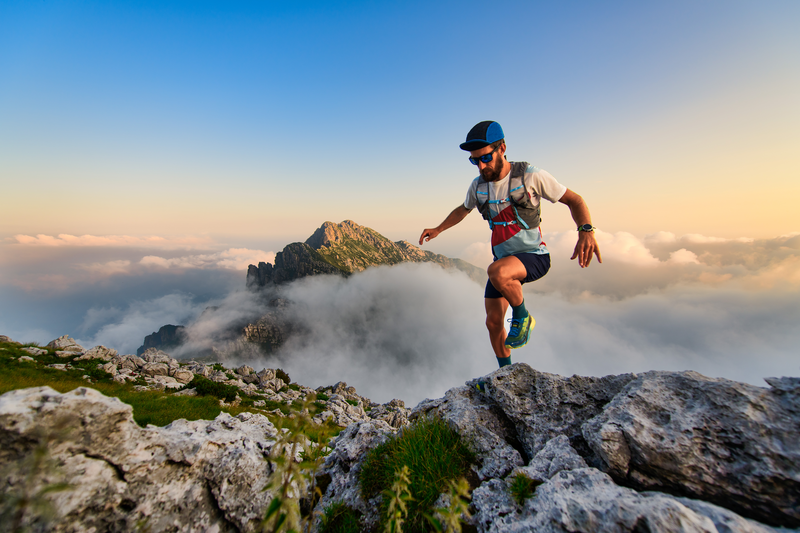
94% of researchers rate our articles as excellent or good
Learn more about the work of our research integrity team to safeguard the quality of each article we publish.
Find out more
REVIEW article
Front. Vet. Sci. , 11 February 2025
Sec. Veterinary Infectious Diseases
Volume 12 - 2025 | https://doi.org/10.3389/fvets.2025.1492144
Haemophilus parasuis (HPS) is the causative agent of porcine Glässer’s disease, which has become prevalent in China in recent years. It is characterized by fibrinous polyserositis, arthritis, and meningitis, but often shows mixed infection with other upper respiratory tract pathogens, causing heavy economic losses to the pig industry. Vaccination is an important means to prevent and control HPS infection, and the currently available vaccines are mainly the inactivated type or subunit vaccines containing immunogenic HPS proteins. This study reviews recent advances in HPS vaccines, analyzes the relative effectiveness of the components of subunit vaccines and discusses the advantages and disadvantages of each vaccine type. The goal is to provide insights for the development of more effective vaccines against Haemophilus parasuis infections in pigs.
Haemophilus parasuis (HPS) is an opportunistic Gram-negative bacterium in the Pasteurella family that causes upper respiratory tract infections of pigs (1). It is motile and diverse (2, 3) and requires factor V (nicotinamide adenine dinucleotide, NAD) for its growth (4). There are 15 serotypes defined so far with significant differences in pathogenicity: strains of serotypes 1, 5 and 12 are highly virulent, while those of serotypes 6 and 9 are of low pathogenicity (5–7). The strains circulating in China are mainly serotypes 4, 5, 12, 13 and 14 (8). HPS affects both piglets and adult pigs and is generally transmitted via the respiratory system. This bacterium is commonly found in the upper respiratory tract of healthy pigs, but under certain conditions, such as stress, overcrowding, or poor ventilation, it can cause a systemic infection known as Glässer’s disease, with high morbidity and mortality (9). The main manifestations of the disease are fibrinous polyserositis, arthritis, and meningitis (3, 10). The disease is widespread in the swine industry in China and around the world, causing huge economic losses to hog farmers.
HPS is a typical opportunistic pathogen which can cause infection in piglets when their immune system is compromised by infection with other pathogens or environmental stress. Usually, the maternal antibodies and innate immunity are sufficient to prevent severe HPS infection, but the pathogenic factors of HPS are not well understood. Common antibiotics are the usual first line of treatment for Glässer’s disease, but antibiotic resistance has been reported in clinical HPS isolates (11, 12), and prevention by vaccination is preferred. Tests have been done on inactivated vaccines, attenuated vaccines, subunit vaccines, genetically engineered vaccines, and DNA vaccines, and they have all been reported to combat HPS infection to some degree. The major problem with HPS vaccines is low cross-protection against the multiple heterologous serotypes that can have significant differences in virulence. The number of approved and commercially available vaccines are also limited and there have been cases of vaccination failure (13). Immunization of sows with HPS vaccine could affect the nasal microbiota of offspring piglets during the first 15 days of life, by reducing the relative abundance of Haemophilus parasuis and modifying the composition of the microbiota to promote species with anti-pathogenic activity (14). No information is available about the effects of the various types of HPS vaccines on the nasal microbiota of piglets. In this article, we review the current literature on advances in Haemophilus parasuis vaccines with an assessment of the pros and cons of each vaccine type to provide insights for the development of novel HPS vaccines that are more effective and offer broader coverage of multiple serotypes.
The pathogenic mechanism of HPS is relatively complex with many virulence factors involving colonization, invasion, and evasion of the host immune defense system. Upon colonizing the lower respiratory tract, HPS effectively evades the host’s immunoglobulin A (IgA)-mediated mucosal defenses by producing IgA proteases (15). In the early stages of infection in pigs, some virulent strains can block phagocytosis by alveolar macrophages and evade antibody-dependent complement-mediated killing (16), causing systemic infection and widespread seroresistance. Some progress has been made in the role of bacterial surface components, toxin proteins and virulence-related transcriptional regulators of HPS, such as lipo-oligosaccharides (LOS), capsular compounds, outer membrane proteins, and virulence-related transcriptional regulatory molecules. LOS have been shown to play an important role in HPS adsorption and invasion of host cells, in addition to inducing inflammatory factors (17, 18). Eberle et al. constructed an HPS capsular mutant strain that lacked CapD, a key gene for the synthesis of capsular polysaccharides, and confirmed that the mutant strain elicited only a weak immune response (19). Zhang et al. found that an outer membrane protein (OMP P2) deletion mutant of the HPS SC096 strain exhibited increased sensitivity to serum due to activation of the classical complement pathway and increased serum IgG content (20, 21), and that OMP P2 was associated with adhesion of porcine alveolar macrophage cell lines, thereby reducing the adhesion of HPS to epithelial and endothelial cell lines and the capacity for in vitro invasion (22). Tang et al. revealed that the extracellular serine protease EspP2 promoted adhesion of HPS to the host through the Rap1 signaling pathway (23).
Melniow et al. used gene chip technology to determine transcriptional profiles in response to simulated conditions of the physiological environment of HPS-infected hosts in vitro, such as acidity, temperature, pressure and iron limitation (24). They found 75 genes that were involved in regulating HPS protein expression, and most of these genes were involved in synthesis of iron and glucose metabolite transporters, metabolic enzymes, and DNA metabolism-related proteins. Because of the limited availability of free iron ions in the host, HPS takes up iron directly through hemoglobin, transferrin and lactoferrin (25). Álvarez-Estrada mimicked iron restriction in a host naturally infected with serotype 5 HPS and found that the expression of six iron restriction genes (tbpA, tbpB, hxuA, hxuB, hxuC, and fhuA) was upregulated (26). These included porcine transferrin binding proteins, hemophores, and the transporter and receptor of the heme/hemopexin-binding protein (hxu) operon, a receptor for siderophores (27). However, in Melniow’s study, the fhuA gene was not upregulated during infection. This discrepancy might be due to upregulation of the fhuA gene that requires two or more factors acting together.
HPS infection stimulates host cells to produce signaling molecules through a set of virulence factors, which bind to cognate receptors to activate downstream signaling pathways and promote host production of multiple proinflammatory factors. Earlier studies indicated that the OMP P2 protein of HPS played a key role in pathogenesis by increasing expression of IL-1α, IL-1β, IL-6, and IL-8 mRNAs in porcine alveolar macrophages (PAMs) and inducing cytokine release in host cells (28–32). Huang et al. reported that excessive and persistent production of proinflammatory cytokines was responsible for severe pulmonary injury in the HPS-infected hosts (33). In the study by Zhou (34), OMP P2 was shown to upregulate mRNA expression of the cytokines IL-17 and IL-23 as well as the chemokines CCL-4 and CCL-5. IL-17. This can lead to excessive inflammation and significant tissue damage due to binding of IL-23 to IL-1, thus maintaining the expansion of Th17 with subsequent release of IL-6, IL-17, IL-22 and TNF-α (35–37). In addition, it was further demonstrated that both the surface-exposed Loop7 and Loop8 structures of OMP P2 could induce the transcription and expression of pro-inflammatory cytokines and chemokines such as IL-1α, IL-1β, IL-6, IL-8 and TNF-α in PAM and PK-15 by activating the NF-κB and MAPK signaling pathways (38). The diagram in Figure 1, shows that HPS OMP-P2 binds to Toll-like receptors (TLRs) 1, 2, 4 and 6, recruiting linker molecules, MyD88 and TRIF, and linking key proteins TRIF, IRAK4, IRAK1, TRAF6, TAK1 and TAB1, which then activate the NF-κB pathway, inducing the pro-inflammatory factors, IL-8 and CCL4, and activating the p38 and JNK MAPK pathways.
Figure 1. OMP P2 induces IL-8 and CCL4 expression through the NF-κB and MAPK pathways (100), respectively.
At present, inactivated vaccines are the most widely used type for Haemophilus parasuis prevention, but there are a few subunit vaccines. Highly virulent strains are employed for inactivated vaccines, and these can be monovalent, bivalent, or trivalent, covering single or multiple serotypes. However, inactivated vaccines only show good efficacy against infections of the same serotype, while their protective effect against infections of different serotypes is poor or ineffective. Thus, developing a vaccine with high cross-protection against multiple serotypes is a challenge that needs to be met. Table 1 lists the relevant vaccines currently available.
In addition, there are two multivalent vaccines applying for clinical trials, namely the porcine circovirus type 2, swine streptococcal disease, Haemophilus parasuis triple subunit vaccine filed by Wuhan Keqian Biological Co. Ltd., China; and the swine pseudorabies-Haemophilus parasuis dual inactivated vaccine against TY-ΔgE, type 4 BJ02, type 5 GS04, and type 13 HN02 strains filed by Beijing Shengtaier Technology Co. Ltd., Beijing Huaxia Xingyang Biotechnology Co. Ltd., and Huaxia Xingyang (Jiangsu) Biotechnology Co., Ltd., all based in China. Although there are many vaccines available on the market, the disease is still widespread. The reason for this could be that most of the existing vaccines are the inactivated type against serotypes 4, 5 and 12, which do not have good protective efficiency against other serotypes. Haemophilus parasuis has less impact than other diseases such as Mycoplasma pneumoniae, porcine circovirus disease, and African swine fever, and the swine industry gives priority to vaccination against diseases with greater impact in order to save costs and avoid the need for multiple vaccinations. Therefore, vaccination against Haemophilus parasuis is not considered a priority. Thus, it is particularly important to design a vaccine that offers good protection efficiency against the most prevalent serotypes, and the simultaneous immunization against Haemophilus parasuis and other diseases will provide the missing incentive for vaccination against lower priority diseases.
Inactivated vaccines are currently the most widely used against Haemophilus parasuis, and these are mainly based on the combination of bivalent or multivalent vaccines of highly virulent serotypes such as type 1, 4, 5, 12 and 13, or multiple vaccines together with porcine circovirus disease, swine streptococcal disease and other porcine epidemic diseases. The results showed that high levels of neutralizing antibodies were produced about three weeks after vaccination with inactivated strains (39, 40), and that piglets born from sows after two inoculations had higher levels of maternal antibodies, which could produce early immune protection (7, 41, 42). In 2001, Takahashi (43) developed an inactivated vaccine against serotypes 2 and 5 and evaluated its safety and efficacy in laboratory and field experiments, where it showed high immune protection against infection by serotypes 2 and 5 along with significant reduction of clinical symptoms in response to challenge infection. Martin (44) prepared an inactivated vaccine in Spain against serotypes 2, 4 and 5 that provided 100% protection in piglets and induced higher levels of cytokines in vivo than those of the subunit vaccine group containing outer membrane protein and transferrin-binding protein. Inactivated vaccines against HPS serotypes 4 and 5 have been reported to reduce mortality and clinical symptoms in piglets after challenge with strains of serotypes 4, 5, 13 and 14; however, it did not protect piglets from serotype 12 infection (45, 46). Zhao et al. (47) assessed the efficacy of the trivalent inactivated vaccine for HPS serotypes 4, 5 and 12 in piglets, and the results showed that the protection was 100%.
Although inactivated vaccines still dominate the market, they do have some limitations. Inactivated vaccines cannot contain all disease-causing serotypes at the same time, and the cross-protection efficiency is low. According to one study (48), it is possible to provide heterologous protection against different serotypes if differences in antibody specificity and ability to induce antibody fixation of complement are taken into consideration. Cross-protection against different strains can also be achieved by choosing the proper target proteins as components of subunit vaccines.
Compared with traditional vaccines, subunit vaccines only contain part of the antigenic components of pathogenic bacteria or viruses, eliminating the possible side effects caused by irrelevant components and greatly improving the safety of vaccines (49). At present, two subunit vaccines of Haemophilus parasuis have been approved for marketing, namely Streptococcus suis-Haemophilus parasuis Dual Subunit Vaccine jointly developed by Wuhan Keqian Biological Co. Ltd., China and other units, and Porcine Circovirus type 2-Haemophilus parasuis Double Subunit Vaccines. The main antigenic HPS component in these two vaccines is the PalA protein, and the latter also contains HPS 06257 protein. These two antigenic proteins are the outer membrane proteins of Haemophilus parasuis, and the other outer membrane proteins, such as D15, OmpP2, OppA, HPS-0675 and GAPDH, have been confirmed as potential candidate antigens with good immunogenicity (49). Related studies have shown that HPS 06257 and PalA proteins are well-conserved in different HPS serotypes. These two proteins can induce high-level specific antibodies in vivo and provide good immune protection in the piglet challenge models. Importantly, the PalA protein is highly similar to the P6 protein of Haemophilus parasuis, and D15 is highly similar to D15 of Haemophilus parasuis and OMA87 of Pasteurella multocida (50, 51) as revealed by the proteomes of diverse microorganisms (52). The P6 protein is involved in the immune response during Haemophilus influenzae infection (53, 54). These characteristics provide strong support for these two proteins as effective target antigens for an HPS subunit vaccine.
In addition to the subunit vaccines that have been successfully approved for marketing, a number of other recombinant proteins associated with HPS immunogenicity are being studied for potential use in vaccines. Recombinant PilA (rPilA) binds to PK-15 cells, porcine tracheal epithelial cells, and the extracellular matrix components, laminin and fibronectin. The rPilA can react with convalescent and ultra-immune serum of Glässer’s disease patients (55). Purified rPilA elicited a robust immune response and produced strong immune protection against HPS serotype 5 challenge in a murine model. Transferrin-binding protein B (TbpB) also showed outstanding performance in a series of animal immunogenicity challenge and evaluation experiments, and was considered the most promising antigen for the formulation of a subunit vaccine with broad-spectrum protection against HPS (56–58). Jia et al. (59) screened the isolates of serotype 13, cloned the lpxC- and gmhA-related genes, and these proteins induced high levels of IgG antibodies and an immune response with secretion of IL-4, IL-10, and IFN-γ in mice. They found that immunization with GmhA and LpxC together could stimulate the production of both Th1 and Th2 immune responses, while recombinant LpxC and inactivated bacteria could only produce a Th2 immune response. In terms of protection from HPS challenge in mice, immunization with recombinant LpxC or GmhA individually resulted in 50% protection, while the combination, LpxC + GmhA, provided 60% protection against infection with a lethal dose of HPS. Dai et al. (60) tested the immunoprotective effect of vaccination with the recombinant polyamine transporter, PotD, in mice and showed that this protein could effectively stimulate both humoral and cellular immune responses. PotD immunization enhanced lymphocyte proliferation, and triggered a Th1-type immune response, protecting the mice from a lethal HPS infection and possibly conferring resistance to HPS colonization. Álvaro et al. (61) evaluated the protective effect of a vaccine containing the three recombinant proteins, rOmpP2, rOmpP5 and rOmpD15, against infection by the HPS Nagasaki strain in piglets lacking colostrum. All three recombinant proteins were recognized and induced specific antibodies in pig serum, but they were not sufficient to protect pigs from HPS challenge under experimental conditions.
With the development of next-generation sequencing technology, availability of a host of complete genomes has made it possible to use the strategy of reverse vaccinology for development of subunit vaccines on a large scale. The basic strategy of reverse vaccinology begins with the bioinformatics analysis of genome-wide genetic information of the target pathogens, and the prediction of potential antigens. The main steps include screening and analysis of genomic information (62), identification of open reading frames with unknown functions (63, 64), homology alignment, subcellular localization of unknown functional ORF-encoded proteins, and functional annotation to identify surface proteins and toxin proteins (65). Comparative genomics and pan-genomics (66, 67) can help researchers respond more efficiently to genetic mutations and immune evasion due to differences between pathogen strains. The precursor LolA protein which comprises the HPS outer membrane lipoprotein carrier protein, and the two HPS outer membrane proteins, RlpB and VacJ (68, 69), were identified by bioinformatics methods as candidates for immunoprotective studies of Haemophilus parasuis. The results showed that mice could produce high levels of IgG antibodies in response to immunization with recombinant LolA, resulting in 50% protection against challenge with the virulent strain HPS01, serotype 13. LolA was found to induce Th1 and Th2 immune responses in mice as shown by the level of cytokines of IL-4, IL-10 and interferon-γ. The recombinant proteins RlpB and VacJ induced strong antibody responses and high IFN-γ levels in the immune sera of inoculated animals, but they did not afford sufficient protection of the pigs in the challenge test. Li et al. (49) identified three outer membrane proteins TolC, LppC and HAPS_0926 by bioinformatics methods. Mice immunized with these three outer membrane proteins produced humoral and host cell-mediated responses with significantly increased antigen-specific IgG level and lymphoproliferative responses. CD4+ and CD8+ T cells as well as three cytokines (IL-2, IL-4, and IFN-γ) were significantly increased in immunized mice. Antisera against the candidate antigens were effective in preventing HPS from surviving in a whole-blood survival assay. It is generally perceived that multi-component subunit vaccines induce a more pronounced immune responses than single-component vaccines.
In addition to the above-mentioned candidate antigens for subunit vaccines, the antigenic proteins listed in Table 2 have also been shown to provide partial protection in HPS challenge experiments. It should be noted, however, that in the majority of these trials, the immunized animals were challenged with highly virulent strains, requiring the highest degree of protection.
Among the candidate antigens, the OmpA family of OMPs function as adhesins and invasins in the respiratory system that bind to airway epithelial cells, which constitute the principle defensive barrier in the lung. Their detection of pathogens like HPS through Toll-like receptors results in the activation of signaling pathways and release of antimicrobial and pro-inflammatory molecules. VtaA was selected as a candidate antigen for its passenger domains with an extensive mosaic structure and serum cross-reactivity among VtaA from different strains. Most of the remaining candidate antigens that have high immunogenicity were identified through bioinformatics screening.
Subunit vaccines are being widely studied, because they have the advantage over live vaccines of avoiding the risk of HPS dissemination and potential side effects from viral components. Candidate proteins play vital roles in nutrient uptake and virulence, so blocking them can repress HPS infection (70), but there are also problems such as poor immunogenicity and cross-protection against heterologous serotypes. Although the subunit vaccines tested so far have shown promising protective effects in mouse and piglet models, there are other potential protective antigens that remain unexplored. Future development of multi-component subunit vaccines should look for common antigens in different serotypes for cross-protection. Extensive research is still needed, and the choice of proper adjuvant (s) to improve the immunogenicity of subunit antigens is equally important.
Bacterial ‘ghosts’ (BGs) are empty bacterial particles obtained by the evacuation of cytoplasm and nucleic acids by gentle biological or chemical perforation (71). BGs are a new type of inactivated bacterial vaccine, which maintains antigenicity but is safer because of the absence of cytoplasm and nucleic acids (72). BG vaccines can retain a host of antigens and protein epitopes with adjuvant and drug delivery properties (73), and can induce a stronger immune response because of the native conformation of the epitopes on the BG surface. Hu et al. (74) constructed a BG vaccine from the HPS serotype 5 strain using phage bacteriolytic technology which produced stronger antibody responses in immunized piglets, higher levels of IFN-γ and IL-4, and more CD4+ T lymphocytes than the inactivated vaccine. However, the researchers did not conduct an in-depth investigation of cross-protection. There are few studies on BG vaccines of Haemophilus parasuis due to the difficulty of their production and relatively high cost, although the stronger protective effect could greatly reduce livestock losses to pig farmers from Glässer’s disease.
Live attenuated vaccines are primarily based on manipulation of the main virulence factors by live-passaging or genetic deletion, which make them less- or non-pathogenic, but still immunogenic. Compared with inactivated vaccines, stable live attenuated vaccines can induce longer-lasting immune responses in the body and are important vaccine candidates for Haemophilus parasuis (75). In 2020, Eberle (19) demonstrated that the HPS HS069 mutant with deletion of the cap gene (polysaccharide biosynthetic protein and glycosyltransferase protein) had enhanced biofilm formation ability compared with the wildtype, could effectively adhere to 3D4/21 cells, and had reduced resistance to macrophage phagocytosis. This study not only confirmed the important role of capsular polysaccharides in HPS infection in piglets but also demonstrated how a genetic deletion could be used to generate an attenuated vaccine. Cytolethal distending toxin (CDT) is an important virulence determinant of many bacterial pathogens that acts by blocking the cell cycle. Zhang et al. (21) constructed an attenuated strain of HPS SC096 by deleting the CDT gene and found that the mutant exhibited reduced adherence to and invasion of porcine umbilical vein endothelial cells (PUVEC) and a porcine renal epithelial cell line (PK-15). Deletion of the rfaE gene, a core biosynthetic enzyme gene in LOS, an important virulence factor of HPS, reduced adhesion to PUVECs and PK-15 cells by 10-fold and 12-fold, respectively (76). In the study of Lin et al. (77), piglets infected with the wild-type JS0135 strain exhibited more significant tissue damage and pathological changes compared to piglets infected with a ΔCDT mutant. In addition, Lin et al. demonstrated that loss of the CDT gene cluster in JS0135 led to increased susceptibility to phagocytosis by porcine alveolar macrophages (3D4/2) than that of the wild-type strain. The two-component system senses the density modulator QseBC, which plays an important role in the virulence of the Enterobacteriaceae and Pasteurella families (78). Yan et al. (79) constructed a ΔQseBC mutant of HPS SC1401 and infected porcine alveolar macrophages and mouse alveolar epithelial cells (MLE-12) with this mutant. They found that the ability of the mutant to adhere to and invade PAM and MLE-12 was significantly reduced. In the mouse challenge model, the mortality rate of mice inoculated with HPS SC1401 was 87.5%, while that of mice inoculated with ΔQseBC was only 50%. Pathological and histological examination of spleen and lungs showed that the pathological effects of ΔQseBC were milder than those of the wild-type group, indicating that the deletion mutant weakened the virulence of HPS in mice. In addition, deletion mutants of capD, cheY, hfq, wza, and lgtF genes have all been reported to have reduced pathogenicity.
Compared with inactivated vaccines, live attenuated vaccines have the advantage of reducing the risk that inactivated vaccines are not completely inactivated and still partially infective. They can distinguish between infected and vaccinated animals, and have a relatively long immune protection period. The primary limitation of live attenuated vaccines is that the main virulence factors and associated pathways of HPS are still not fully understood. With continued in-depth research, live attenuated vaccines are expected to become more widely accepted as a highly effective vaccination strategy against Haemophilus parasuis.
Compared to subunit vaccines and live attenuated vaccines, DNA vaccines are safer, more stable, and easier to prepare. Fu et al. (80) constructed a novel DNA vaccine (pCgap) encoding GAPDH and explored its immunoprotective efficacy in mice. They showed that the DNA vaccine was highly expressed in mammalian cells and could induce significant humoral immunity as well as adaptive Th1 and Th2 responses in mice. In the mouse model the pCgap DNA vaccine provided 83.3 and 50% protection against challenge with HPS MD0322 and SH0165 strains, respectively. Since the pCgap gene is quite conserved in HPS and is widely present in 15 serotypes, the vaccine has the potential to be protective against all serotypes and is expected to be a vaccine candidate with high cross-protection efficacy. But it also has certain limitations: DNA vaccines may not elicit robust immune responses, requiring additional measures to enhance their effectiveness (81, 82).
With the development of reverse vaccinology and immunoinformatics, different types of vaccines have become more available. In addition to conventional inactivated vaccines and attenuated vaccines, multi-epitope vaccines are also developing rapidly. A methodology called pan-genomics analysis is used to identify the core genome of a pathogen and predict the B cell and T cell epitopes of the outer membrane proteins encoded by the core genome (83). With this approach, the genes encoding a multi-epitope vaccine could be identified, the antigenicity and physicochemical properties of the proteins could be predicted, and the three-dimensional structure, molecular docking, and molecular dynamics could be simulated (84, 85). Pang et al. (86) retrieved the complete genomes of 105 HPS strains and extracted 8 core genes and their protein sequences using the Roary (87) program for pan-genomic analysis. Next, they designed a multi-epitope vaccine with high scores through linker ligation according to the predicted signal peptide (4), subcellular localization (88, 89), T cell epitope prediction (90, 91), the immunogenicity and physicochemical properties of the antigen (92), and its secondary and tertiary structure (93–95). HPS strains of different serotypes or unclassifiable HPS could be combined to produce polyclonal antibodies in mice after immunization, and the serum levels of IgM + IgG and IgG1 + IgG2 in mice were significantly increased after the second and third immunization, and high levels of B cell populations, cytotoxicity, and T helper lymphocytes were observed, indicating that a cell-mediated immune response was activated. Preliminary evidence suggests that the protein is a promising vaccine candidate.
Multi-epitope vaccines are composed of epitopes of several antigens with high immunogenicity, which solves the problem of low immunogenicity caused by a single antigen and is predicted by computer simulation. Multi-epitope vaccines have been widely used in various animal and human vaccine research, and compared with traditional vaccines, the cost is low. In addition to low production cost and high safety, they can also be composed of a variety of immunogenic peptides of different pathogens to generate protective immune responses to these pathogens as well. However, there are certain limitations. Epitope prediction analysis is typically based on linear sequences and does not consider the influence of the spatial conformation of proteins. With the advancing capability for AI-assisted in-depth interdisciplinary approaches of bioinformatics and multi-omics, the application of multi-epitope vaccines for preventing Glässer’s disease has great prospects (96).
In summary, this review provides a general overview of six types of Haemophilus parasuis vaccines. Table 3 summarizes the advantages and disadvantages of each type, showing a clearer and more intuitive comparison of their differences.
Haemophilus parasuis is an opportunistic pathogen in the upper respiratory tract of pigs that usually have co-infections with a variety of viruses, bacteria and mycoplasmas, resulting in severe respiratory diseases. Such co-infections render diagnosis and treatment of the diseases more difficult and bring serious economic losses to the swine industry (97). The approach to effectively prevent and control HPS infection relies on accurate diagnosis, biosafety measures and good vaccines (98, 99). Although the research results obtained so far show good protective efficacy of inactivated and subunit vaccines, the problem of low cross-protection still exists because HPS has multiple serotypes. Therefore, by multicomponent vaccines derived from different serotypes to enhance the cross-protection capability of a vaccines and inclusion of effective adjuvants to boost immunogenicity should be major goals in developing vaccines against HPS infection.
YD: Writing – original draft, Writing – review & editing. YHa: Writing – review & editing. HF: Supervision, Writing – review & editing. JS: Conceptualization, Funding acquisition, Writing – review & editing. YHe: Conceptualization, Funding acquisition, Writing – review & editing.
The author(s) declare that financial support was received for the research, authorship, and/or publication of this article. This research was supported by “Pioneer” and “Leading Goose” R&D Program of Zhejiang (No.2025C01138, 2023C02023 and 2023C02047).
The authors declare that the research was conducted in the absence of any commercial or financial relationships that could be construed as a potential conflict of interest.
All claims expressed in this article are solely those of the authors and do not necessarily represent those of their affiliated organizations, or those of the publisher, the editors and the reviewers. Any product that may be evaluated in this article, or claim that may be made by its manufacturer, is not guaranteed or endorsed by the publisher.
1. Morozumi, T, and Nicolet, J. Some antigenic properties of Haemophilus Parasuis and a proposal for serological classification. J Clin Microbiol. (1986) 23:1022–5. doi: 10.1128/jcm.23.6.1022-1025.1986
2. Oliveira, S, and Pijoan, C. Computer-based analysis of Haemophilus Parasuis protein fingerprints. Can J Vet Res. (2004) 68:71–5.
3. Oliveira, S, and Pijoan, C. Haemophilus Parasuis: new trends on diagnosis, epidemiology and control. Vet Microbiol. (2004) 99:1–12. doi: 10.1016/j.vetmic.2003.12.001
4. Almagro Armenteros, JJ, Tsirigos, KD, Sønderby, CK, Petersen, TN, Winther, O, Brunak, S, et al. Signalp 5.0 improves signal peptide predictions using deep neural networks. Nat Biotechnol. (2019) 37:420–3. doi: 10.1038/s41587-019-0036-z
5. Kielstein, P, and Rapp-Gabrielson, VJ. Designation of 15 Serovars of Haemophilus Parasuis on the basis of immunodiffusion using heat-stable antigen extracts. J Clin Microbiol. (1992) 30:862–5. doi: 10.1128/jcm.30.4.862-865.1992
6. Aragon, V, Bouchet, B, and Gottschalk, M. Invasion of endothelial cells by systemic and nasal strains of Haemophilus Parasuis. Vet J. (2010) 186:264–7. doi: 10.1016/j.tvjl.2009.08.013
7. Nielsen, R. Pathogenicity and immunity studies of Haemophilus Parasuis serotypes. Acta Vet Scand. (1993) 34:193–8. doi: 10.1186/BF03548209
8. Zhang, J, Xu, C, Guo, L, Shen, H, Deng, X, Ke, C, et al. Prevalence and characterization of genotypic diversity of Haemophilus Parasuis isolates from southern China. Can J Vet Res. (2012) 76:224–9.
9. Zhang, N-Z, Zhou, D-H, Huang, S-Y, Wang, M, Shi, X-C, Ciren, D, et al. Seroprevalence and risk factors associated with Haemophilus Parasuis infection in Tibetan pigs in Tibet. Acta Trop. (2014) 132:94–7. doi: 10.1016/j.actatropica.2013.12.021
10. Zhang, B, Tang, C, Liao, M, and Yue, H. Update on the pathogenesis of Haemophilus Parasuis infection and virulence factors. Vet Microbiol. (2014) 168:1–7. doi: 10.1016/j.vetmic.2013.07.027
11. Moleres, J, Santos-López, A, Lázaro, I, Labairu, J, Prat, C, Ardanuy, C, et al. Novel Blarob-1-bearing plasmid conferring resistance to Β-lactams in Haemophilus Parasuis isolates from healthy weaning pigs. Appl Environ Microbiol. (2015) 81:3255–67. doi: 10.1128/AEM.03865-14
12. Liu, H, Xue, Q, Zeng, Q, and Zhao, Z. Haemophilus Parasuis Vaccines. Vet Immunol Immunopathol. (2016) 180:53–8. doi: 10.1016/j.vetimm.2016.09.002
13. Huang, J, Yang, X, Wang, A, Huang, C, Tang, H, Zhang, Q, et al. Pigs overexpressing porcine Β-Defensin 2 display increased resilience to Glaesserella Parasuis. Infection Antibiotics (Basel). (2020) 9:903. doi: 10.3390/antibiotics9120903
14. Blanco-Fuertes, M, Correa-Fiz, F, López-Serrano, S, Sibila, M, and Aragon, V. Sow vaccination against virulent Glaesserella Parasuis shapes the nasal microbiota of their offspring. Sci Rep. (2022) 12:3357. doi: 10.1038/s41598-022-07382-2
15. Wang, H, Liu, L, Cao, Q, Mao, W, Zhang, Y, Qu, X, et al. Haemophilus Parasuis Α-2,3-Sialyltransferase-mediated Lipooligosaccharide sialylation contributes to bacterial pathogenicity. Virulence. (2018) 9:1247–62. doi: 10.1080/21505594.2018.1502606
16. Wang, X, Xu, X, Wu, Y, Li, L, Cao, R, Cai, X, et al. Polysaccharide biosynthesis protein Capd is a novel pathogenicity-associated determinant of Haemophilus Parasuis involved in serum-resistance ability. Vet Microbiol. (2013) 164:184–9. doi: 10.1016/j.vetmic.2013.01.037
17. Xu, Z, Yue, M, Zhou, R, Jin, Q, Fan, Y, Bei, W, et al. Genomic characterization of Haemophilus Parasuis Sh0165, a highly virulent strain of Serovar 5 prevalent in China. PLoS One. (2011) 6:e19631. doi: 10.1371/journal.pone.0019631
18. Harper, M, Boyce, JD, and Adler, B. The key surface components of Pasteurella Multocida: capsule and lipopolysaccharide. Curr Top Microbiol Immunol. (2012) 361:39–51. doi: 10.1007/82_2012_202
19. Eberle, KC, Hau, SJ, Luan, S-L, Weinert, LA, Stasko, JA, Wang, J, et al. Generation and evaluation of a Glaesserella (Haemophilus) Parasuis capsular mutant. Infect Immun. (2020) 88:e00879–19. doi: 10.1128/IAI.00879-19
20. Zhang, B, Feng, S, Xu, C, Zhou, S, He, Y, Zhang, L, et al. Serum resistance in Haemophilus Parasuis Sc096 strain requires outer membrane protein P2 expression. FEMS Microbiol Lett. (2012) 326:109–15. doi: 10.1111/j.1574-6968.2011.02433.x
21. Zhang, B, He, Y, Xu, C, Xu, L, Feng, S, Liao, M, et al. Cytolethal distending toxin (Cdt) of the Haemophilus Parasuis Sc096 strain contributes to serum resistance and adherence to and invasion of Pk-15 and Puvec cells. Vet Microbiol. (2012) 157:237–42. doi: 10.1016/j.vetmic.2011.12.002
22. Zhang, B, Xu, C, and Liao, M. Outer membrane protein P2 of the Haemophilus Parasuis Sc096 strain contributes to adherence to porcine alveolar macrophages cells. Vet Microbiol. (2012) 158:226–7. doi: 10.1016/j.vetmic.2012.01.023
23. Tang, X, Xu, S, Yang, Z, Wang, K, Dai, K, Zhang, Y, et al. Espp2 regulates the adhesion of Glaesserella Parasuis via Rap1 signaling pathway. Int J Mol Sci. (2024) 25:4570. doi: 10.3390/ijms25084570
24. MELNIKOW, E, DORNAN, S, SARGENT, C, DUSZENKO, M, EVANS, G, GUNKEL, N, et al. Microarray analysis of Haemophilus Parasuis gene expression under in vitro growth conditions mimicking the in vivo environment. Vet Microbiol. (2005) 110:255–63. doi: 10.1016/j.vetmic.2005.08.007
25. Xie, Q, Jin, H, Luo, R, Wan, Y, Chu, J, Zhou, H, et al. Transcriptional responses of Haemophilus Parasuis to Iron-restriction stress in vitro. Biometals. (2009) 22:907–16. doi: 10.1007/s10534-009-9243-2
26. Álvarez-Estrada, Á, Gutiérrez-Martín, CB, Rodríguez-Ferri, EF, and Martínez-Martínez, S. Transcriptomics of Haemophilus (Glässerella) Parasuis Serovar 5 subjected to culture conditions partially mimetic to natural infection for the search of new vaccine antigens. BMC Vet Res. (2018) 14:326. doi: 10.1186/s12917-018-1647-1
27. Morton, DJ, Seale, TW, Madore, LL, VanWagoner, TM, Whitby, PW, and Stull, TL. The Haem-Haemopexin utilization gene cluster (Hxucba) as a virulence factor of Haemophilus Influenzae. Microbiology (Reading). (2007) 153:215–24. doi: 10.1099/mic.0.2006/000190-0
28. Galdiero, M, Galdiero, M, Finamore, E, Rossano, F, Gambuzza, M, Catania, MR, et al. Haemophilus Influenzae Porin induces toll-like receptor 2-mediated cytokine production in human monocytes and mouse macrophages. Infect Immun. (2004) 72:1204–9. doi: 10.1128/IAI.72.2.1204-1209.2004
29. Galdiero, S, Capasso, D, Vitiello, M, D'Isanto, M, Pedone, C, and Galdiero, M. Role of surface-exposed loops of Haemophilus Influenzae protein P2 in the mitogen-activated protein kinase Cascade. Infect Immun. (2003) 71:2798–809. doi: 10.1128/IAI.71.5.2798-2809.2003
30. Massari, P, Ram, S, Macleod, H, and Wetzler, LM. The role of Porins in Neisserial pathogenesis and immunity. Trends Microbiol. (2003) 11:87–93. doi: 10.1016/S0966-842X(02)00037-9
31. Vitiello, M, Finamore, E, Cantisani, M, Bevilacqua, P, Incoronato, N, Falanga, A, et al. P2 Porin and loop L7 from Haemophilus Influenzae modulate expression of Il-6 and adhesion molecules in astrocytes. Microbiol Immunol. (2011) 55:347–56. doi: 10.1111/j.1348-0421.2011.00318.x
32. Zhou, S, He, X, Xu, C, Zhang, B, Feng, S, Zou, Y, et al. The outer membrane protein P2 (Ompp2) of Haemophilus Parasuis induces Proinflammatory cytokine Mrna expression in porcine alveolar macrophages. Vet J. (2014) 199:461–4. doi: 10.1016/j.tvjl.2013.12.010
33. Huang, C, Wang, Y, Li, X, Ren, L, Zhao, J, Hu, Y, et al. Clinical features of patients infected with 2019 novel coronavirus in Wuhan, China. Lancet. (2020) 395:497–506. doi: 10.1016/S0140-6736(20)30183-5
34. Zhou, Y, Feng, S, He, X, Zhou, Q, Wang, Y, Yue, H, et al. Surface-exposed loops L7 and L8 of Haemophilus (Glaesserella) Parasuis Ompp2 contribute to the expression of Proinflammatory cytokines in porcine alveolar macrophages. Vet Res. (2019) 50:105. doi: 10.1186/s13567-019-0721-4
35. Bettelli, E, Carrier, Y, Gao, W, Korn, T, Strom, TB, Oukka, M, et al. Reciprocal developmental pathways for the generation of pathogenic effector Th17 and regulatory T cells. Nature. (2006) 441:235–8. doi: 10.1038/nature04753
36. Gu, C, Wu, L, and Li, X. Il-17 family: cytokines, receptors and signaling. Cytokine. (2013) 64:477–85. doi: 10.1016/j.cyto.2013.07.022
37. Kuwabara, T, Ishikawa, F, Kondo, M, and Kakiuchi, T. The role of Il-17 and related cytokines in inflammatory autoimmune diseases. Mediat Inflamm. (2017) 2017:3908061. doi: 10.1155/2017/3908061
38. Wang, Y, Liu, C, Fang, Y, Liu, X, Li, W, Liu, S, et al. Transcription analysis on response of porcine alveolar macrophages to Haemophilus Parasuis. BMC Genomics. (2012) 13:68. doi: 10.1186/1471-2164-13-68
39. Macedo, N, Rovira, A, and Torremorell, M. Haemophilus Parasuis: infection, immunity and Enrofloxacin. Vet Res. (2015) 46:128. doi: 10.1186/s13567-015-0263-3
40. Li, X-H, Zhao, G-Z, Qiu, L-X, Dai, A-L, Wu, W-W, and Yang, X-Y. Protective efficacy of an inactive vaccine based on the Ly02 isolate against acute Haemophilus Parasuis infection in piglets. Biomed Res Int. (2015) 2015:649878. doi: 10.1155/2015/649878
41. Galina Pantoja, L, Stammen, B, Minton, B, and Amodie, D. Serologic profiling of Haemophilus Parasuis-vaccinated sows and their litters using a novel oligopeptide permease a enzyme-linked immunosorbent assay reveals unexpected patterns of serological response and maternal antibody transfer. J Vet Diagn Invest. (2014) 26:125–30. doi: 10.1177/1040638713510163
42. Baumann, G, and Bilkei, G. Effect of vaccinating sows and their piglets on the development of Glässer's disease induced by a virulent strain of Haemophilus Parasuis Serovar 5. Vet Rec. (2002) 151:18–21. doi: 10.1136/vr.151.1.18
43. Takahashi, K, Naga, S, Yagihashi, T, Ikehata, T, Nakano, Y, Senna, K, et al. A cross-protection experiment in pigs vaccinated with Haemophilus Parasuis Serovars 2 and 5 Bacterins, and evaluation of a bivalent vaccine under laboratory and field conditions. J Vet Med Sci. (2001) 63:487–91. doi: 10.1292/jvms.63.487
44. de la Fuente, AJM, Ferri, EFR, Tejerina, F, Frandoloso, R, Martínez, SM, and Martín, CBG. Cytokine expression in colostrum-deprived pigs immunized and challenged with Haemophilus Parasuis. Res Vet Sci. (2009) 87:47–52. doi: 10.1016/j.rvsc.2008.12.012
45. Huang, X, Li, Y, Fu, Y, Ji, Y, Lian, K, Zheng, H, et al. Cross-protective efficacy of recombinant transferrin-binding protein a of Haemophilus Parasuis in Guinea pigs. Clin Vaccine Immunol. (2013) 20:912–9. doi: 10.1128/CVI.00621-12
46. Yuan, F, Fu, S, Hu, J, Li, J, Chang, H, Hu, L, et al. Evaluation of recombinant proteins of Haemophilus Parasuis strain Sh0165 as vaccine candidates in a mouse model. Res Vet Sci. (2012) 93:51–6. doi: 10.1016/j.rvsc.2011.04.020
47. Zhao, Z, Liu, H, Xue, Y, Chen, K, Liu, Z, Xue, Q, et al. Analysis of efficacy obtained with a trivalent inactivated Haemophilus Parasuis Serovars 4, 5, and 12 vaccine and commercial vaccines against Glässer's disease in piglets. Can J Vet Res. (2017) 81:22–7.
48. Hau, SJ, Eberle, KC, and Brockmeier, SL. Importance of strain selection in the generation of heterologous immunity to Glaesserella (Haemophilus) Parasuis. Vet Immunol Immunopathol. (2021) 234:110205. doi: 10.1016/j.vetimm.2021.110205
49. Li, M, Cai, R-J, Song, S, Jiang, Z-Y, Li, Y, Gou, H-C, et al. Evaluation of immunogenicity and protective efficacy of recombinant outer membrane proteins of Haemophilus Parasuis Serovar 5 in a murine model. PLoS One. (2017) 12:e0176537. doi: 10.1371/journal.pone.0176537
50. Robb, CW, Orihuela, CJ, Ekkelenkamp, MB, and Niesel, DW. Identification and characterization of an in vivo regulated D15/Oma87 homologue in Shigella Flexneri using differential display polymerase chain reaction. Gene. (2001) 262:169–77. doi: 10.1016/S0378-1119(00)00537-0
51. Wei, X, Cao, S, Zhang, L, Wen, X, Wen, Y, Wu, R, et al. Comparative proteome analysis of the extracellular proteins of two Haemophilus Parasuis strains Nagasaki and Sw114. Biochem Biophys Res Commun. (2014) 446:997–1001. doi: 10.1016/j.bbrc.2014.03.049
52. Antelmann, H, Williams, RC, Miethke, M, Wipat, A, Albrecht, D, Harwood, CR, et al. The extracellular and cytoplasmic proteomes of the non-virulent Bacillus Anthracis strain Um23c1-2. Proteomics. (2005) 5:3684–95. Epub 2005/08/27. doi: 10.1002/pmic.200401218
53. Nelson, MB, Murphy, TF, van Keulen, H, Rekosh, D, and Apicella, MA. Studies on P6, an important outer-membrane protein antigen of Haemophilus Influenzae. Rev Infect Dis. (1988) 10:S331–6. doi: 10.1093/cid/10.Supplement_2.S331
54. See, SB, and Thomas, WR. Protective anti-outer membrane protein immunity against Pasteurella Pneumotropica infection of mice. Microbes Infect. (2013) 15:470–9. doi: 10.1016/j.micinf.2013.04.003
55. An, J, Cai, J, Zhang, B, and Li, Y. Pili subunit Pila contributes to the Cytoadhesion of Glaesserella Parasuis to host cells and provides Immunoprotection. Appl Environ Microbiol. (2023) 89:e0200222. doi: 10.1128/aem.02002-22
56. Martín de la Fuente, AJ, Gutiérrez Martín, CB, Pérez Martínez, C, García Iglesias, MJ, Tejerina, F, and Rodríguez Ferri, EF. Effect of different vaccine formulations on the development of Glässer's disease induced in pigs by experimental Haemophilus Parasuis infection. J Comp Pathol. (2009) 140:169–76. doi: 10.1016/j.jcpa.2008.10.007
57. Guizzo, JA, Chaudhuri, S, Prigol, SR, Yu, R-H, Dazzi, CC, Balbinott, N, et al. The amino acid selected for generating mutant Tbpb antigens defective in binding transferrin can compromise the in vivo protective capacity. Sci Rep. (2018) 8:7372. doi: 10.1038/s41598-018-25685-1
58. Fernández, AG, Martín, CBG, Rilo, MP, Fernández, EP, Pérez, RM, Frandoloso, R, et al. Phylogenetic study and comparison of different Tbpb obtained from Glaesserella Parasuis present in Spanish clinical isolates. Res Vet Sci. (2023) 157:35–9. doi: 10.1016/j.rvsc.2023.02.003
59. Jia, Y, Chen, X, Zhou, Y, Yan, P, Guo, Y, Yin, R, et al. Application of mouse model for evaluation of recombinant Lpxc and Gmha as novel antigenic vaccine candidates of Glaesserella Parasuis serotype 13. J Vet Med Sci. (2021) 83:1500–8. doi: 10.1292/jvms.21-0298
60. Dai, K, Ma, X, Yang, Z, Chang, Y-F, Cao, S, Zhao, Q, et al. Polyamine transport protein PotD protects mice against Haemophilus parasuis and elevates the secretion of pro-inflammatory cytokines of macrophage via JNK-MAPK and NF-κB signal pathways through TLR4. Vaccines (Basel). (2019) 7:216. doi: 10.3390/vaccines7040216
61. Álvarez-Estrada, Á, Martínez-Martínez, S, Martín, C-BG, García-Iglesias, M-J, Pérez-Martínez, C, Yubero-Delgado, S, et al. Immunogenic characterization of vaccines based on Haemophilus Parasuis Nagasaki strain, Ompp2, Ompp5 and Ompd15, in colostrum-deprived pigs experimentally challenged with the same strain. Res Vet Sci. (2018) 119:292–301. doi: 10.1016/j.rvsc.2018.07.009
62. Mora, M, Veggi, D, Santini, L, Pizza, M, and Rappuoli, R. Reverse Vaccinology. Drug Discov Today. (2003) 8:459–64. doi: 10.1016/S1359-6446(03)02689-8
63. Altschul, SF, Gish, W, Miller, W, Myers, EW, and Lipman, DJ. Basic local alignment search tool. J Mol Biol. (1990) 215:403–10. doi: 10.1016/S0022-2836(05)80360-2
64. Altschul, SF, Madden, TL, Schäffer, AA, Zhang, J, Zhang, Z, Miller, W, et al. Gapped blast and psi-blast: a new generation of protein database search programs. Nucleic Acids Res. (1997) 25:3389–402. doi: 10.1093/nar/25.17.3389
65. Sharma, A, Sanduja, P, Anand, A, Mahajan, P, Guzman, CA, Yadav, P, et al. Advanced strategies for development of vaccines against human bacterial pathogens. World J Microbiol Biotechnol. (2021) 37:67. doi: 10.1007/s11274-021-03021-6
66. Sharma, A, Arya, DK, Sagar, V, Bergmann, R, Chhatwal, GS, and Johri, AK. Identification of potential universal vaccine candidates against group a Streptococcus by using high throughput in silico and proteomics approach. J Proteome Res. (2013) 12:336–46. doi: 10.1021/pr3005265
67. Maione, D, Margarit, I, Rinaudo, CD, Masignani, V, Mora, M, Scarselli, M, et al. Identification of a universal group B Streptococcus vaccine by multiple genome screen. Science. (2005) 309:148–50. doi: 10.1126/science.1109869
68. Guo, Z, Jia, Y, Huang, C, Zhou, Y, Chen, X, Yin, R, et al. Immunogenicity and protection against Glaesserella Parasuis serotype 13 infection after vaccination with recombinant protein Lola in mice. J Vet Med Sci. (2022) 84:1527–35. doi: 10.1292/jvms.22-0203
69. Hau, SJ, Luan, S-L, Loving, CL, Nicholson, TL, Wang, J, Peters, SE, et al. Evaluation of the recombinant proteins Rlpb and Vacj as a vaccine for protection against Glaesserella Parasuis in pigs. BMC Vet Res. (2020) 16:167. doi: 10.1186/s12917-020-02377-5
70. Hensel, M, Shea, JE, Gleeson, C, Jones, MD, Dalton, E, and Holden, DW. Simultaneous identification of bacterial virulence genes by negative selection. Science. (1995) 269:400–3. doi: 10.1126/science.7618105
71. Witte, A, Wanner, G, Sulzner, M, and Lubitz, W. Dynamics of Phix174 protein E-mediated lysis of Escherichia Coli. Arch Microbiol. (1992) 157:381–8. doi: 10.1007/BF00248685
72. Witte, A, Bläsi, U, Halfmann, G, Szostak, M, Wanner, G, and Lubitz, W. Phi X174 protein E-mediated lysis of Escherichia Coli. Biochimie. (1990) 72:191–200. doi: 10.1016/0300-9084(90)90145-7
73. Mayr, UB, Walcher, P, Azimpour, C, Riedmann, E, Haller, C, and Lubitz, W. Bacterial ghosts as antigen delivery vehicles. Adv Drug Deliv Rev. (2005) 57:1381–91. doi: 10.1016/j.addr.2005.01.027
74. Hu, M, Zhang, Y, Xie, F, Li, G, Li, J, Si, W, et al. Protection of piglets by a Haemophilus Parasuis ghost vaccine against homologous challenge. Clin Vaccine Immunol. (2013) 20:795–802. doi: 10.1128/CVI.00676-12
75. Zhang, L, Li, Y, Dai, K, Wen, X, Wu, R, Huang, X, et al. Establishment of a successive Markerless mutation system in Haemophilus Parasuis through natural transformation. PLoS One. (2015) 10:e0127393. doi: 10.1371/journal.pone.0127393
76. Zhang, B, Yu, Y, Zeng, Z, Ren, Y, and Yue, H. Deletion of the Rfae gene in Haemophilus Parasuis Sc096 strain attenuates serum resistance, Adhesion and Invasion. Microb Pathog. (2014) 74:33–7. doi: 10.1016/j.micpath.2014.07.006
77. Lin, Y, Zhu, N, Liu, J, Wen, S, Xu, Y, Xu, X, et al. The role of Cytolethal distending toxin in Glaesserella Parasuis Js0135 strain infection: cytotoxicity, Phagocytic Resistance and Pathogenicity. Vet Microbiol. (2024) 295:110168. doi: 10.1016/j.vetmic.2024.110168
78. Weigel, WA, and Demuth, DR. Qsebc, a two-component bacterial adrenergic receptor and global regulator of virulence in Enterobacteriaceae and Pasteurellaceae. Mol Oral Microbiol. (2016) 31:379–97. doi: 10.1111/omi.12138
79. Yan, X, Dai, K, Gu, C, Yu, Z, He, M, Xiao, W, et al. Deletion of two-component system Qsebc weakened virulence of Glaesserella Parasuis in a murine acute infection model and adhesion to host cells. PeerJ. (2022) 10:e13648. doi: 10.7717/peerj.13648
80. Fu, S, Zhang, M, Ou, J, Liu, H, Tan, C, Liu, J, et al. Construction and immune effect of Haemophilus Parasuis DNA vaccine encoding Glyceraldehyde-3-phosphate dehydrogenase (Gapdh) in mice. Vaccine. (2012) 30:6839–44. doi: 10.1016/j.vaccine.2012.09.014
81. Khalid, K, and Poh, CL. The development of DNA vaccines against Sars-Cov-2. Adv Med Sci. (2023) 68:213–26. doi: 10.1016/j.advms.2023.05.003
82. Hobernik, D, and Bros, M. DNA vaccines-how far from clinical use? Int J Mol Sci. (2018) 19:3605. doi: 10.3390/ijms19113605
83. Parvizpour, S, Pourseif, MM, Razmara, J, Rafi, MA, and Omidi, Y. Epitope-based vaccine design: a comprehensive overview of bioinformatics approaches. Drug Discov Today. (2020) 25:1034–42. doi: 10.1016/j.drudis.2020.03.006
84. Alzarea, SI. Identification and construction of a multi-epitopes vaccine design against Klebsiella Aerogenes: molecular modeling study. Sci Rep. (2022) 12:14402. doi: 10.1038/s41598-022-18610-0
85. Albekairi, TH, Alshammari, A, Alharbi, M, Alshammary, AF, Tahir Ul Qamar, M, Ullah, A, et al. Designing of a novel multi-antigenic epitope-based vaccine against E. hormaechei: An Intergraded Reverse Vaccinology and Immunoinformatics Approach. Vaccines (Basel). (2022) 10. doi: 10.3390/vaccines10050665
86. Pang, M, Tu, T, Wang, Y, Zhang, P, Ren, M, Yao, X, et al. Design of a Multi-Epitope Vaccine against Haemophilus Parasuis based on Pan-genome and Immunoinformatics approaches. Front Vet Sci. (2022) 9:1053198. doi: 10.3389/fvets.2022.1053198
87. Page, AJ, Cummins, CA, Hunt, M, Wong, VK, Reuter, S, Holden, MTG, et al. Roary: rapid large-scale prokaryote Pan genome analysis. Bioinformatics. (2015) 31:3691–3. doi: 10.1093/bioinformatics/btv421
88. Ong, E, Wang, H, Wong, MU, Seetharaman, M, Valdez, N, and He, Y. Vaxign-ml: supervised machine learning reverse vaccinology model for improved prediction of bacterial protective antigens. Bioinformatics. (2020) 36:3185–91. doi: 10.1093/bioinformatics/btaa119
89. Jespersen, MC, Peters, B, Nielsen, M, and Marcatili, P. Bepipred-2.0: improving sequence-based B-cell epitope prediction using conformational epitopes. Nucleic Acids Res. (2017) 45:W24–9. doi: 10.1093/nar/gkx346
90. Peters, B, Bulik, S, Tampe, R, Van Endert, PM, and Holzhütter, H-G. Identifying Mhc class I epitopes by predicting the tap transport efficiency of epitope precursors. J Immunol. (2003) 171:1741–9. doi: 10.4049/jimmunol.171.4.1741
91. Nielsen, M, and Nn-Align, LO. An artificial neural network-based alignment algorithm for Mhc class ii peptide binding prediction. BMC Bioinform. (2009) 10:296. doi: 10.1186/1471-2105-10-296
92. Doytchinova, IA, and Flower, DR. Vaxijen: a server for prediction of protective antigens, tumour antigens and subunit vaccines. BMC Bioinform. (2007) 8:4. doi: 10.1186/1471-2105-8-4
93. McGuffin, LJ, Bryson, K, and Jones, DT. The Psipred protein structure prediction server. Bioinformatics. (2000) 16:404–5. doi: 10.1093/bioinformatics/16.4.404
94. Wang, S, Li, W, Liu, S, and Xu, J. Raptorx-property: a web server for protein structure property prediction. Nucleic Acids Res. (2016) 44:W430–5. doi: 10.1093/nar/gkw306
95. Kim, DE, Chivian, D, and Baker, D. Protein structure prediction and analysis using the Robetta server. Nucleic Acids Res. (2004) 32:W526–31. doi: 10.1093/nar/gkh468
96. Khalid, K, and Poh, CL. The promising potential of reverse vaccinology-based next-generation vaccine development over conventional vaccines against antibiotic-resistant Bacteria. Vaccines (Basel). (2023) 11:1264. doi: 10.3390/vaccines11071264
97. Ni, H-B, Gong, Q-L, Zhao, Q, Li, X-Y, and Zhang, X-X. Prevalence of Haemophilus Parasuis"Glaesserella Parasuis" in pigs in China: a systematic review and Meta-analysis. Prev Vet Med. (2020) 182:105083. doi: 10.1016/j.prevetmed.2020.105083
98. Hao, J, Jia, M, Liu, Y, Lv, Z, Chen, J, Xiong, W, et al. Application of a rapid and sensitive Rpa-Crispr/Cas12a assay for naked-eye detection of Haemophilus Parasuis. Anal Chim Acta. (2024) 1287:342101. doi: 10.1016/j.aca.2023.342101
99. Zhang, K, Sun, Z, Shi, K, Yang, D, Bian, Z, Li, Y, et al. Rpa-Crispr/Cas12a-based detection of Haemophilus Parasuis. Animals (Basel). (2023) 13:3317. doi: 10.3390/ani13213317
100. Chen, Y, Liu, T, Langford, P, Hua, K, Zhou, S, Zhai, Y, et al. Haemophilus Parasuis induces activation of Nf-Κb and map kinase signaling pathways mediated by toll-like receptors. Mol Immunol. (2015) 65:360–6. doi: 10.1016/j.molimm.2015.02.016
101. Confer, AW, and Ayalew, S. The Ompa family of proteins: roles in bacterial pathogenesis and immunity. Vet Microbiol. (2013) 163:207–22. doi: 10.1016/j.vetmic.2012.08.019
102. Olvera, A, Pina, S, Pérez-Simó, M, Aragón, V, Segalés, J, and Bensaid, A. Immunogenicity and protection against Haemophilus Parasuis infection after vaccination with recombinant virulence associated trimeric autotransporters (Vtaa). Vaccine. (2011) 29:2797–802. doi: 10.1016/j.vaccine.2011.01.105
103. Li, M, Song, S, Yang, D, Li, C, and Li, G. Identification of secreted proteins as novel antigenic vaccine candidates of Haemophilus Parasuis Serovar 5. Vaccine. (2015) 33:1695–701. doi: 10.1016/j.vaccine.2015.02.023
104. Fu, S, Zhang, M, Xu, J, Ou, J, Wang, Y, Liu, H, et al. Immunogenicity and protective efficacy of recombinant Haemophilus Parasuis Sh0165 putative outer membrane proteins. Vaccine. (2013) 31:347–53. doi: 10.1016/j.vaccine.2012.11.003
105. Yang, Z, Zhang, Y, Zhao, Q, Du, S, Huang, X, Wu, R, et al. Hbpa from Glaesserella Parasuis induces an inflammatory response in 3d4/21 cells by activating the Mapk and Nf-Κb Signalling pathways and protects mice against G. Parasuis when used as an immunogen. Vet Res. (2024) 55:93. doi: 10.1186/s13567-024-01344-4
106. Li, G, Xie, F, Li, J, Liu, J, Li, D, Zhang, Y, et al. Identification of novel Haemophilus Parasuis Serovar 5 vaccine candidates using an Immunoproteomic approach. J Proteome. (2017) 163:111–7. doi: 10.1016/j.jprot.2017.05.014
107. Zhang, NZ, Chu, YF, Gao, PC, Zhao, P, He, Y, and Lu, ZX. Immunological identification and characterization of extracellular serine protease-like protein encoded in a putative gene of. J Vet Med Sci. (2012) 74:983–7. doi: 10.1292/jvms.11-0260
108. Zhang, L, Li, Y, Wen, Y, Lau, GW, Huang, X, Wu, R, et al. Htra is important for stress resistance and virulence in Haemophilus Parasuis. Infect Immun. (2016) 84:2209–19. doi: 10.1128/IAI.00147-16
Keywords: Haemophilus parasuis, Glässer’s disease, vaccines, pathogenesis, Glaesserella parasuis
Citation: Duan Y, Hao Y, Feng H, Shu J and He Y (2025) Research progress on Haemophilus parasuis vaccines. Front. Vet. Sci. 12:1492144. doi: 10.3389/fvets.2025.1492144
Received: 06 September 2024; Accepted: 22 January 2025;
Published: 11 February 2025.
Edited by:
Faham Khamesipour, Ministry of Health and Medical Education, IranReviewed by:
Nattawooti Sthitmatee, Chiang Mai University, ThailandCopyright © 2025 Duan, Hao, Feng, Shu and He. This is an open-access article distributed under the terms of the Creative Commons Attribution License (CC BY). The use, distribution or reproduction in other forums is permitted, provided the original author(s) and the copyright owner(s) are credited and that the original publication in this journal is cited, in accordance with accepted academic practice. No use, distribution or reproduction is permitted which does not comply with these terms.
*Correspondence: Jianhong Shu, c2h1amlhbmhvbmdAenN0dS5lZHUuY24=; Yulong He, aGV5bDc5QHpzdHUuZWR1LmNu
†ORCID: Yulong He, orcid.org/0000-0002-0265-5946
Jianhong Shu, orcid.org/0000-0002-1080-9108
Disclaimer: All claims expressed in this article are solely those of the authors and do not necessarily represent those of their affiliated organizations, or those of the publisher, the editors and the reviewers. Any product that may be evaluated in this article or claim that may be made by its manufacturer is not guaranteed or endorsed by the publisher.
Research integrity at Frontiers
Learn more about the work of our research integrity team to safeguard the quality of each article we publish.