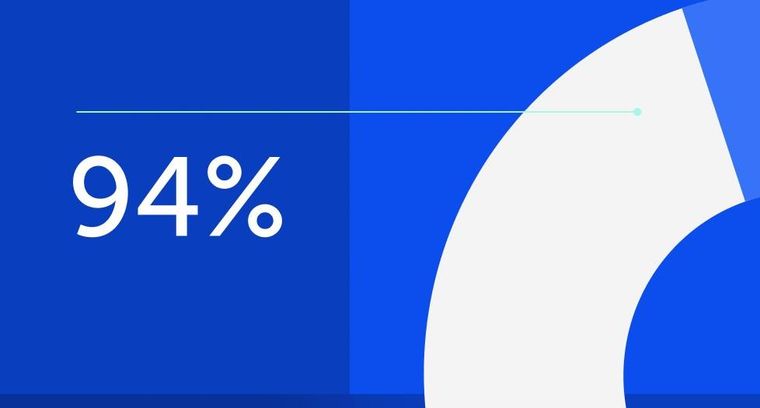
94% of researchers rate our articles as excellent or good
Learn more about the work of our research integrity team to safeguard the quality of each article we publish.
Find out more
ORIGINAL RESEARCH article
Front. Vet. Sci., 10 January 2025
Sec. Animal Nutrition and Metabolism
Volume 11 - 2024 | https://doi.org/10.3389/fvets.2024.1528126
This article is part of the Research TopicCrosslinking of feed nutrients, microbiome and production in ruminantsView all 16 articles
Introduction: The residual black wolfberry fruit (RBWF) is rich in nutrients and contains a diverse range of active substances, which may offer a viable alternative to antibiotics. This experiment was conducted to investigate the impact of varying levels of RBWF on the growth performance and rumen microorganisms of fattening sheep, and to quantify its economic benefits.
Methods: In this experiment, 40 three-month-old and male Duolang sheep with an average weight of 29.85 kg, selected for their propensity to gain weight, were randomly assigned to one of four groups, with ten sheep in each group. To this end, each group was fed with a different proportion of RBWF (0%, 2%, 5%, 8%), and rumen fluid samples were collected to detect differences in fermentation parameters and microbial structure.
Results: The findings indicate that the dry matter intake, OM and NDF apparent digestibility of Duolang sheep in the H2 group were found to be significantly higher than those observed in the other groups (P < 0.05). The concentration of volatile fatty acids (VFAs), including acetate, propionate, iso-butyrate, butyrate and iso-valerate, in rumen fluid demonstrated a linear increase with the supplementation of RBWF in the diet (P < 0.05). The dominant bacteria in the rumen of Duolang sheep were identified as Prevotella, Christensenellaceae R7 group, NK4A214 group, Ruminococcus, and Rikenellaceae RC9 gut group. Compared with the CK group, the relative abundance of Prevotella, NK4A214 group, unclassified Prevotellaceae and Lachnospiraceae NK3A20 group in the rumen of sheep in each experimental group increased to varying degrees. The gross profit of the H2 group was significantly higher than that of the other groups.
Conclusion: In conclusion, the supplementation of RBWF has been demonstrated to enhance the growth performance of Duolang sheep, optimise rumen fermentation parameters, and ultimately increase gross profit, of which 5 % is the best.
Lycium barbarum is a deciduous shrub belonging to the Solanaceae family (1). It is a widely utilized ingredient in the food and medical industries, due to the presence of bioactive metabolites in the branches, leaves and fruits (2). From a global perspective, China is the world’s foremost producer of wolfberries, accounting for over 80% of global cultivation. The majority of China’s wolfberry cultivation is concentrated in the provinces of Ningxia, Qinghai, Gansu and Xinjiang. It is reported that in 2023, the total area devoted to wolfberry cultivation in China will reach 1216.67 km2, with an estimated output of 1.4 Mt. of fresh fruit and 0.24 Mt. of dry fruit. In the production of fresh wolfberry, a residual quantity of 5 to 10% of the fruit is inevitable. This is typically discarded as waste, resulting in a considerable amount of wastage. The contents of crude protein (CP), crude fat (EE), crude ash (Ash), total carbohydrates and dietary fiber were found to be 9.2, 3.0, 5.6, 61.6 and 12.1%, respectively (3). Furthermore, Lycium ruthenicum Murray is a rich source of polyphenols, polysaccharides, alkaloids, anthocyanins and other biochemical components. These component confer a range of beneficial effects, including immune regulation, antioxidant activity, anti-aging, anti-tumor, anti-fatigue and anti-inflammatory actions (4). Zhao et al. (5) demonstrated that the inclusion of 0.6% Lycium barbarum polysaccharide (LBP) in the diet of dairy cows resulted in a notable enhancement in their production performance, accompanied by an augmentation in the body’s antioxidant capacity and immunity. Zhang et al. (6) discovered that fermented wolfberry residue not only enhances carcass weight in fattening sheep but also stimulates the expression of chemokines and immune-related pathways in sheep. Gan et al. (7) demonstrated that LBP can induce an immune response, thereby regulating the immune response to diseases such as cancer.
In comparison to red wolfberry, black wolfberry exhibited a higher concentration of phenols, concentrated tannins and monomeric anthocyanins, and also demonstrated enhanced anti-inflammatory and antioxidant activities (8, 9). Currently, there is a plethora of research exploring the utilization of by-products derived from red fruit wolfberry. However, despite the unique functional components present in black fruit wolfberry, it has not yet been subjected to the same degree of investigation. A substantial body of evidence attests to the potential value of incorporating red wolfberry by-products into livestock and poultry production (10). It may therefore be hypothesized that the inclusion of black wolfberry fruit in the diet of animals could have a beneficial effect on their production performance. The objective of this experiment was to investigate the impact of varying levels of residual black wolfberry fruit (RBWF) on the growth performance, nutrient apparent digestibility, rumen fermentation parameters, microflora and gross profit of Duolang sheep. This was done in order to establish a theoretical basis for the application of residual black wolfberry fruit in sheep production.
The study was carried out in accordance with the procedures sanctioned for this research, which have been approved by the Science and Technology Ethics Committee of Xinjiang Academy of Animal Sciences, China (ethics number 20230508). These procedures adhere to the principles and regulations for ethical protection in human and animal biological science and technology in China.
In this experiment, following the collection of the black wolfberry fruit in October 2022, the fruit that did not meet the quality standard was identified as RBWF. The nutritional components of RBWF were then determined, as illustrated in Table 1. Based on the principle of equal energy and nitrogen, four diets containing different proportions (0, 2, 5, and 8%) of RBWF were formulated (NRC 2007).
Forty male lambs, 3 months aged and with a mean body weight of 29.85 ± 2.00 kg, were used in this experiment. Following deworming, a single-factor completely randomized experimental design was implemented, with the lambs randomly divided into four groups, each with 10 replicates. Four distinct proportions of RBWF diets were provided, comprising the following: a control group (CK, 0% RBWF), experimental group 1 (H1, 2% LBL), experimental group 2 (H2, 5% LBL) and experimental group 3 (H3, 8%LBL). The specific diet composition and nutrient composition are presented in Table 2. The experiment spanned 70 days, comprising a 10-day pre-experimental period and a 60-day experimental period.
Prior to the commencement of the experiment, the sheep house was thoroughly cleaned and disinfected, and the test sheep were subjected to a series of preparatory procedures, including cutting, deworming, and medicated bathing. During the course of the experiment, the sheep were maintained in separate columns and provided with food twice daily, at 10:00 and 18:00, respectively. The Duolang sheep were permitted to feed and drink freely throughout the duration of the experiment.
The quantity of feed provided and the quantity of residual feed were recorded on a daily basis. Prior to the morning feeding on the 1st, 30th and 60th days of the experiment, each sheep was weighed. Thereafter, the average daily feed intake (ADFI), average daily gain (ADG) and feed-to-weight ratio (F:G) were calculated. A digestion test was conducted over the final 10 days of the formal test period, comprising a 5-day adaptation period. Fecal samples were collected using the total fecal collection method over 5 consecutive days. The total fecal matter of each sheep was weighed prior to morning feeding on a daily basis, with 10% of the total fecal matter collected from each sheep. Following the conclusion of the experiment, the collected fecal samples were combined in a uniform manner, with 10% of the samples obtained through the quartering method and stored at −20°C for subsequent analysis.
An analysis was conducted on samples of diet, ingredients, and feces for dry matter (method 930.15), CP (method 990.03), EE (method 920.39), Ca (method 978.02), and P (method 946.06) using the AOAC procedures (11). NDF and ADF content were determined following Van Soest’s (12) method. ME was calculated based on the measured nutritional value:
Immediately following the formal test, the test sheep were slaughtered after 16 h of fasting and rumen fluid was collected. The pH value was immediately measured following filtration through four layers of gauze using a portable pH meter (PHS-3C, Shanghai, China), and the measurement was repeated 3 times. To assess rumen fermentation parameters and rumen microflora, the remaining samples were transferred to 15 mL and 5 mL freezing tubes and stored in −20°C and − 80°C freezers, respectively. The concentration of NH3-N was determined by phenol sodium hypochlorite colorimetry; the concentration of volatile fatty acids (VFAs) was determined by gas chromatography.
The TGuide S96 Magnetic Bead Method Soil/Fecal Genomic DNA Extraction Kit (Tiangen, Beijing, China) was used to extract DNA from the rumen specimens for 16S rDNA sequencing analysis. The concentration of the extracted nucleic acids was determined using an enzyme marker (GeneCompany Limited, Hong Kong, China, model Synergy HTX), and their integrity was assessed through agarose electrophoresis at a concentration of 1.8% (Beijing Bomei Fuxin Technology Co., Ltd., Beijing, China).
The highly variable V3-V4 regions of bacterial 16S rDNA were amplified by PCR using universal bacterial primers 338F (5′- ACTCCTACGGGGAGGCAGCA-3′) and 806R (5′- GGACTACHVGGGGTWTCTAAT-3′) after extracting total DNA from the samples. The final products were cleaned, quantified, and mixed to form a sequencing collection. After passing quality assessments, the collection was analyzed on the Illumina NovaSeq 6,000 instrument (San Diego, CA, USA). Raw data files from various high-throughput sequencers, including the Illumina NovaSeq, were processed to generate sequenced reads. These reads include both the actual sequences and quality metrics. The initial reads were refined with the Trimmomatic v0.33 tool. To further refine the data, Cutadapt 1.9.1 was utilized to eliminate primer sequences and produce polished reads.
The preliminary sorting of the experimental data was conducted using Excel 2023 software, while the significance test was performed with the one-way ANOVA program in SPSS 26.0 statistical software. Subsequently, linear and quadratic analyses were carried out on rumen fermentation parameters, employing the Duncan method for multiple comparison differences. The level of significance was determined by p < 0.05, while 0.05 < p ≤ 0.10 indicated a trend. The taxonomy annotation of the OTUs was conducted by classifying representative organisms using a Bayesian classifier based on the SILVA database (version 138). Alpha diversity analyzes species diversity and complexity using ACE, Chao1, Simpson, and Shannon indices. Beta diversity analyses were conducted using principal coordinate analysis (PCoA) and nonmetric multidimensional scaling (NMDS) to evaluate distinctions between groups. LEfSe (Line Discriminant Analysis (LDA) Effect Size) was employed to facilitate a comparative analysis of the various treatment groups. The differences between the classification levels were then analyzed individually, from the lowest level (species) to the highest (phylum), with the objective of identifying biomarkers exhibiting statistically significant differences between the groups.
Table 3 illustrates the impact of incorporating varying levels of RBWF into the diet on the growth performance of Duolang sheep. It can be observed that following a 60-day feeding period, the final weight gain of sheep in the H2 group was 14.89 kg, representing a 4.78, 12.97, and 13.32% increase compared to the CK, H1, and H3 groups (14.21 kg, 13.18 kg, and 13.14 kg, respectively). The H2 group exhibited the most favorable outcome, although no statistically significant difference was observed (p > 0.05). Concurrently, the dry matter intake of the H2 group was markedly higher than that of the CK group (p < 0.05).
Table 4 demonstrated that dietary RBWF supplementation had no statistically significant impact on the apparent digestibility of dry matter (DM), crude protein (CP), ether extract (EE), acid detergent fiber (ADF), calcium (Ca) and phosphorus (P) in Duolang sheep when compared with the CK group (p > 0.05). However, the apparent digestibility of organic matter (OM) and NDF in the H2 group was found to be significantly higher than that observed in the H3 group (p < 0.05).
Table 5 illustrates the impact of dietary supplementation with RBWF on rumen fermentation parameters in Duolang sheep. It can be observed that no statistically significant difference was evident in pH and NH3-N concentration between the experimental groups (p > 0.05). In comparison to the CK group, the pH of the rumen in sheep exhibited a linear decline (p = 0.080), while the NH3-N concentration demonstrated a notable linear increase (p < 0.05). The concentrations of acetate and propionate in the rumen of the H2 group were found to be significantly higher than those observed in the other experimental groups. The concentrations of iso-butyrate, butyrate, iso-valerate and total volatile fatty acids (TVFAs) in the rumen of the H2 and H3 groups were significantly higher than those of the CK group (p < 0.05). As the ratio of RBWF supplementation increased, the concentrations of acetate, propionate, iso-butyrate, butyrate, iso-valerate, n-valeric acid and total volatile fatty acids (VFAs) in the rumen exhibited a linear increase (p < 0.05). However, the quadratic effect of acetate and iso-valerate was statistically significant (p < 0.05).
A total of 1,348,210 readings were obtained from 16 samples in four groups (5 in CK, 4 in H1, 3 in H2 and 4 in H3). Following quality control and splicing, 1,269,853 clean readings were obtained, with an average of 79,365 clean readings obtained for each sample. The number of unique OUTs in each group was as follows: 3,295 in CK, 2,260 in H1, 1,608 in H2 and 2,175 in H3. The total number of OUTs across all four groups was 4,170 (Figure 1).
As illustrated in Figure 2, dietary RBWF supplementation had no discernible impact on the alpha diversity of rumen microbiota in sheep. With regard to beta diversity, the results of PCoA (Figure 3A) and NMDS (Figure 3B) analysis demonstrated that there was no notable separation between the distribution of rumen microbial representative points across different treatment groups, thereby substantiating that the addition of RBWF had no substantial influence on the beta diversity of rumen microorganisms in Duolang sheep.
Figure 2. Alpha diversity analysis of rumen flora. (A) ACE index of species richness; (B) Chao1 index of species richness; (C) Shannon index of species diversity; (D) Simpson index of species diversity.
Figure 3. Beta diversity analysis of rumen flora. (A) PCoA principal axis analysis; (B) NMDS non-metric multidimensional scaling analysis.
A LEfSe (linear discriminant analysis effect size) analysis was conducted to identify discrepancies in the taxonomic composition of bacterial species. The figure presents a representative branch diagram of the main microbiome structure (Figure 4), illustrating the most notable differences between the groups of different treatment components at the phylum, class, order, family, genus, and species levels. The data indicated that 12 branches were more abundant in the CK group, 3 branches were more abundant in the H1 group, 11 branches were more abundant in the H2 group, and 9 branches were more abundant in the H3 group. The abundance differences of different bacterial groups among CK, H1, H2, and H3 are shown in Figure 5. The figure illustrates the abundance differences of various bacterial groups between the CK, H1, H2, and H3 groups. Notably, the Lachnospiraceae ND3007 group, Eubacterium coprostanoligenes group, and Bifidobacterium longum exhibited the most pronounced differences in abundance within the CK group. In contrast, the Prevotellaceae UCG-001 and Parasutterella excrementihominis genera demonstrated the most significant abundance differences within the HC group. The bacterial genera Terrisporobacter, Dubosiella and Enterococcus were more abundant in H2, while Muribaculum and Pseudomonadales were more abundant in H3.
Figure 4. LEfSe (linear discriminant analysis effect size) cladogram comparing microbial communities among the 2.5 elevations.
The differences in rumen microbial species abundance between the various treatment groups were subjected to analysis. At the phylum level (Figure 6A), the dominant bacterial species in the rumen of Duolang sheep were identified as Firmicutes, Bacteroidota, Proteobacteria, Fibrobacterota, and Patescibacteria. Following the addition of RBWF, no significant overall effect on the abundance of phylum-level flora was observed (p > 0.05). However, the relative abundance of Bacteroidota and Fibrobacterota in H1 group increased (Supplementary Table S1). At the genus level (Figure 6B), the dominant bacteria in the rumen bacteria of Duolang sheep were Prevotella, Christensenellaceae R7 group, NK4A214 group, Ruminococcus, and Rikenellaceae RC9 gut group. After the addition of RBWF, the overall effect on the abundance of genus-level bacteria was not significant (p > 0.05). Nevertheless, there was a notable increase in the relative abundance of Prevotella, NK4A214 group and Lachnospiraceae NK3A20 group, while the relative abundance of Prevotellaceae UCG 001 decreased (Supplementary Table S2).
Figure 6. Distribution of bacterial taxa averaged under phyla (A) and genera (B) levels across the different treatment groups (as a percentage of the total sequence).
As illustrated in the Figure 7, the relative abundance of rumen-dominant bacteria exhibited a correlation with growth performance, nutrient apparent digestibility, and rumen fermentation parameters. The abundances of F082, Christensenellaceae R7 group, NK4A214 group and Ruminococcus were found to be significantly and positively correlated with ADG (p < 0.05). In contrast, Prevotellaceae UCG 001 was observed to be negatively correlated with FCR and pH, and positively correlated with other indexes. Of these, it was found to be significantly and positively correlated with OM apparent digestibility (p < 0.05).
Figure 7. Spearman correlation and clustering analysis between growth performance, nutrient apparent digestibility and rumen fermentation parameters with main bacteria in Genus level. Different colors represent positive correlation (red) or negative correlation (blue), and the color shade indicates the magnitude of the correlation, * represents 0.01 < p ≤ 0.05 and ** represents p ≤ 0.01.
The feed unit price of each group was found to be between 2.25 and 2.39 yuan/kg. As illustrated in the Table 5, as increase in the proportion of RBWF added to the diet was associated with a gradual decrease in feed unit price. The gross profit of the H2 group was observed to have increased by 19.34% in comparison to the CK group (Table 6).
The current global concern surrounding antibiotic resistance is well documented (13). The intensification of animal husbandry practices at home and abroad has resulted in the pervasive use of antibiotics in animal husbandry production, which has introduced significant risks to food safety and environmental safety. The utilization of antibiotics in human and livestock production will inevitably result in the emergence of drug resistance (14). In order to mitigate the adverse effects of antibiotic resistance on human health, a number of healthcare institutions have introduced a series of policies aimed at promoting the rational use of antibiotics (15). Lycium barbarum, a traditional Chinese herbal medicine and health food, has been the subject of considerable interest due to the diversity of its functional components (16). Yin et al. (17) demonstrated that the inclusion of LBP in the diet of weaned piglets resulted in enhanced growth performance, antioxidant capacity, and immunity, as well as the regulation of intestinal microbial composition. Furthermore, they established that LBP can serve as an effective substitute for antibiotics in the feed of weaned piglets. Tian et al. (18) observed that Lycium barbarum partially alleviated the intestinal ecological imbalance caused by antibiotics by regulating the intestinal flora, and also produced short-chain fatty acids (SCFAs) to improve intestinal barrier function. Prior research has demonstrated that the bioactive components present in Lycium barbarum exhibit a range of biological functions that can prevent and treat chronic diseases, thereby positioning it as a potential alternative to antibiotics (19) The current research mainly focuses on the role of mining and extracting some active ingredients in Lycium barbarum as feed additives in livestock and poultry production and clinical practice (20, 21). However, the extraction method also affects the chemical properties of the active ingredients of Lycium barbarum (22). At the same time, there are few studies on the effects of wolfberry fruit on growth performance, nutrient apparent digestibility, rumen fermentation parameters and microbial flora structure of fattening sheep. Therefore, the objective of this study was to elucidate the impact of incorporating RBWF into the diet of fattening Duolang sheep and evaluate its economic benefits.
Prior research has demonstrated a significant correlation between the average daily feed intake of fattening sheep and feed conversion efficiency, with feed conversion efficiency being closely associated with growth performance (23). The administration of LBP to a diet regimen was observed to enhance the growth performance and digestive enzyme activity of broilers (24). Hao et al. demonstrated that the administration of Chinese wolfberry and astragalus extracts in lieu of a 1% basal diet can enhance the growth performance of Tibetan fragrant pigs (25). The results of this experiment demonstrated that the incorporation of 5% RBWF into the diet led to a notable increase in the dry matter intake of fattening sheep. This was accompanied by a similar trend in both the daily gain and total weight gain. However, no significant effect was observed, which may be attributed to the considerable inter-individual variability within the experimental group. In contrast to the aforementioned findings, Guo et al. (26) observed that the addition of Lycium barbarum to the basal diet resulted in a notable enhancement in the average body weight and growth performance of rats. This discrepancy may be attributed to the variation in nutrient digestion and absorption rates resulting from the disparate digestive tract structures of the experimental animals.
Apparent digestibility is a significant indicator of the rate of digestion and absorption of nutrients in the experimental diet. It provides insight into whether the diet meets the nutritional requirements for animal growth (27). The polysaccharide derived from Lycium barbarum is a complex mixture of active ingredients with a multitude of potential applications and a promising future in development (28). The study demonstrated that the intake of polysaccharides in the diet exerts a discernible influence on the feed intake and apparent digestibility of nutrients in ruminants (29). Lin et al. (30) demonstrated that LBP enhanced the digestive and absorptive processes and immune function of immunosuppressed mice by inhibiting the activation of the MLCK signaling pathway, and regulated the immune system of the intestinal mucosal barrier. The addition of 5% RBWF resulted in a significantly higher apparent digestibility of OM and NDF compared to the control group. Additionally, there was a notable increase in the apparent digestibility of ADF, from 24.93 to 27.74%. However, this difference was not found to be statistically significant. In contrast to the aforementioned findings, Ju et al. (31) observed that the addition of Lycium barbarum polysaccharides to the lamb diet resulted in a notable enhancement in the apparent digestibility of DM, OM, and CP. This discrepancy could be attributed to the distinct processing forms of Lycium barbarum products. In this experiment, the fruit was not subjected to extraction, and it was directly incorporated into the lamb diet, consequently influencing the chemical composition of the Lycium barbarum additives. In conclusion, the incorporation of RBWF into the diet has been demonstrated to enhance the feed intake of fattening sheep and augment the apparent digestibility of OM and NDF, as well as the daily gain of lambs. This is may achieved by elevating the relative abundance of fiber-degrading bacteria within the rumen, which in turn increases the feed reward.
The compound stomach is the most prominent anatomical feature of ruminants, and the rumen, situated within the four stomachs, serves as the primary site for ruminant digestion of feed. The key indicators for measuring the maintenance of an internal steady state of rumen fermentation in animals are pH, NH3-N and VFAs. The pH directly affects the growth and interaction of microorganisms in the rumen, and maintaining an appropriate range is closely related to rumen fermentation. At the same time, the concentration of volatile fatty acids (VFAs) and ammonia nitrogen (NH3-N) within the rumen also exert a direct influence on rumen pH (32). NH3-N represents the primary nitrogen source for protein synthesis in rumen microorganisms. The microorganisms in the rumen engage in cooperative degradation of nutrients in the feed, converting them into volatile fatty acids (VFAs) that provide energy for body growth (33). The concentration and proportion of VFAs in the rumen are related to dietary composition and energy level, and have an effect on the energy utilization efficiency, growth performance and methane production of the host (34). The addition of 0.6% LBP to the diet of dairy cows has been found to significantly improve the production performance of dairy cows and significantly increase the total volatile fatty acid content of rumen ammonia nitrogen (5). In this study, the concentration of volatile fatty acids (VFAs), including acetate, propionate, iso-butyrate, butyrate and iso-valerate, in rumen fluid demonstrated a linear increase with the elevation of dietary RBWF supplemental level. This outcome aligns with the findings of Duan et al. (35). The pH of rumen fluid in sheep is typically within the range of 5.5–7.0, while the normal range of NH3-N is 5.0 to 30 mg/dL (36). The rumen fluid pH and NH3-N of all sheep were maintained within the normal range in this experiment. As a consequence of the considerable rise in VFAs concentration in rumen fluid, the pH in the rumen of Duolang sheep in each experimental group declined in a linear fashion with the increase of RBWF supplemental level in the diet, while the concentration of NH3-N in rumen increased linearly. It may therefore be hypothesized that the addition of RBWF to the diet can increase the protein synthesis of rumen microorganisms, thereby promoting microbial fermentation in the rumen of animals and the absorption and utilization of nutrients in the diet. Further investigation is required to elucidate the precise effects.
The rumen is a digestive organ that contains a diverse range of microorganisms, which work collectively to degrade nutrients in the diet, thereby providing the host with energy (37). Simultaneously, the host provides a stable and conducive environment for the growth of rumen microorganisms, while obtaining energy from the final product (38). The structure and abundance of the rumen microbial flora can exert a direct influence on the health and growth of the host. Conversely, the structure of rumen microbial flora is also influenced by host species, nutritional energy level and environment (39). Prior research has demonstrated that dietary supplementation of wolfberry fruit can regulate the composition of gastrointestinal microbiota and cecal fermentation in rabbits (40). The findings of the study conducted indicated that the incorporation of LBP into the weaned piglets diet could potentially enhance the abundance of Bacteroidetes in the ileum and cecum, while increasing the levels of Lactobacillus and Bifidobacterium in the cecum, and the intestinal microflora was shown to be improved (41). Alpha diversity is primarily indicative of the richness and diversity of species distribution within the rumen, whereas beta diversity predominantly reflects the dissimilarities in microbial communities between disparate samples. In this experiment, the rumen microorganisms of Duolang sheep were sequenced, and the alpha diversity results demonstrated that the dietary RBWF addition level had no significant impact on the four indexes of Chao1, Ace, Shannon and Simpson in the rumen of Duolang sheep. The PCoA and NMDS plots revealed that the bacterial community structures of the different treatment groups were not statistically different from one another, as indicated by the overlap of the respective groups on the plots. The aforementioned results demonstrate that the incorporation of RBWF into the diet does not exert any deleterious effects on the rumen microbial flora structure of Duolang sheep.
A substantial body of research has demonstrated that the predominant bacteria in the rumen of ruminants are members of the Firmicutes and Bacteroidota phyla. These bacteria play a pivotal role in the host’s degradation of complex carbohydrates and promotion of the decomposition and absorption of nutrients (42, 43). The results indicate that Firmicutes and Bacteroidota play an important role in maintaining the stability of the rumen microbial community. Among them, Bacteroidota mainly promotes rumen fermentation to degrade cellulose, soluble sugar and carbohydrates to produce small molecules such as VFAs, which are utilized by the host (44). The role of Firmicutes is mainly to enhance host lipid metabolism and promote its energy absorption (45). Fujisaka et al. (46) observed that Firmicutes and Bacteroidota constituted between 70 and 90% of the total bacterial population. In this experiment, the relative abundance of Firmicutes and Bacteroidota accounted for approximately 90% of the total bacteria, which was consistent with the aforementioned reports. Furthermore, the relative abundance of Bacteroidota and Fibrobacterota increased following the addition of RBWF in this experiment, which may facilitate the degradation of cellulose in the rumen and is associated with the enhancement of the apparent digestibility of NDF in this experiment.
In addition to decomposing and metabolizing complex nutrients such as cellulose and protein, Prevotella can also engage in cooperative interactions with other microorganisms to facilitate the growth and development of the body (47). The NK4A214 group is primarily responsible for the degradation of fibers, which provides energy to the host. Additionally, it has been observed that this process enhances the host’s immunity by inhibiting the growth of harmful bacteria (48). The Lachnospiraceae NK3A20 group has also been identified as a potentially beneficial bacterium, with involvement in the metabolism of various carbohydrates and the production of acetic acid and butyric acid, which provide energy for the host (49). Prevotellaceae UCG 001 primarily degrades proteins and amino acids, producing short-chain fatty acids, and facilitates the degradation of fiber in collaboration with cellulose-decomposing bacteria (50). Following the addition of RBWF to the experimental diet, a notable increase was observed in the relative abundance of Prevotella, NK4A214 group, unclassified Prevotellaceae and Lachnospiraceae NK3A20 group. These bacteria are capable of decomposing complex dietary fibers, such as cellulose and pectin, into smaller molecular structures that can be utilized by other bacteria within the rumen, resulting in the production of volatile fatty acids (VFAs). Zhu et al. (51) observed that a reduction in the relative abundance of Prevotellaceae UCG 001 was associated with an improvement in depressive behavior. This finding was hypothesized to be linked to a decrease in short-chain fatty acids and a reduction in intestinal inflammation. The results of this experiment demonstrated a reduction in the relative abundance of Prevotellaceae UCG 001, which may be associated with autoimmune regulation. Furthermore, our study elucidated the correlation between growth performance, nutrient apparent digestibility, rumen fermentation parameters and major bacteria at the genus level.
Prior research has demonstrated a positive correlation between Prevotellaceae UCG 001 and the expression of inflammatory factors (52). In this experiment, Prevotellaceae UCG 001 was found to be negatively correlated with feed conversion ratio (FCR) and pH, and positively correlated with other indicators. Furthermore, it was significantly positively correlated with organic matter (OM) apparent digestibility. The concentration of acetic acid and butyric acid in the rumen of Duolang sheep in the experimental group increased, which enhanced the absorption of nutrients in the rumen and thus promoted growth performance. The abundance of F082, Christensenellaceae R7 group, NK4A214 group and Ruminococcus was significantly and positively correlated with ADG. An increase in the relative abundance of NK4A214 group was observed, resulting in an increase in ADG for the experimental group. However, further study is required to elucidate the specific mechanisms through which these strains affect growth performance and rumen fermentation.
In the context of sheep breeding, economic benefits are closely associated with feed costs and growth performance (53). The results of this experiment indicate that an increase in the proportion of RBWF added to the diet is associated with a reduction in feed unit price. This suggests that the inclusion of RBWF in the diet may offer a potential means of reducing feed costs. However, the total weight gain of sheep in the experimental group with RBWF was found to decrease. In contrast, the H2 group exhibited a 4.78% increase in weight gain compared to the control group. Additionally, the gross profit was observed to increase by 17.45% compared to the control group. These findings suggest that the appropriate amount of RBWF can enhance the profitability of mutton sheep breeding. Furthermore, the 5% addition was identified as the optimal dosage. Han et al. (54) demonstrated that the incorporation of wolfberry as a functional or nutritional feed ingredient in broiler diets offers a promising economic benefit. Similarly, Wang et al. (55) revealed that the administration of 0.1–0.2% LBP nutrition lick blocks can markedly enhance the economic viability of fattening Tan sheep. Collectively, these studies highlight the potential of Lycium barbarum products to enhance livestock productivity.
The results of this experiment demonstrate that the administration of RBWF can enhance the growth performance of Duolang sheep, optimize rumen fermentation parameters, exert no deleterious effect on the structure and abundance of rumen microbial flora, and augment gross profit. In the context of the experimental conditions, the administration of 5% RBWF yielded more favorable outcomes.
The datasets presented in this study can be found in online repositories. The names of the repository/repositories and accession number(s) can be found below: https://www.ncbi.nlm.nih.gov/, PRJNA1170910, PRJNA1170932.
The animal studies were approved by the animal study protocol was approved by the Ethics Committee of Institute of Feed Research, Xinjiang Academy of Animal Science (protocol code no. 320230508 and May 10, 2023 of approval). The studies were conducted in accordance with the local legislation and institutional requirements. Written informed consent was obtained from the owners for the participation of their animals in this study.
LH: Conceptualization, Data curation, Formal analysis, Investigation, Methodology, Writing – original draft, Funding acquisition, Project administration, Resources, Visualization, Writing – review & editing. PD: Conceptualization, Data curation, Formal analysis, Investigation, Methodology, Writing – original draft, Software, Writing – review & editing. YY: Conceptualization, Data curation, Formal analysis, Investigation, Methodology, Resources, Validation, Visualization, Writing – review & editing. AS: Supervision, Writing – review & editing. JL: Investigation, Software, Validation, Writing – review & editing. CX: Investigation, Software, Validation, Writing – review & editing. TG: Conceptualization, Funding acquisition, Methodology, Project administration, Resources, Supervision, Writing – review & editing.
The author(s) declare that financial support was received for the research, authorship, and/or publication of this article. This study was financially supported by the China-Kazakhstan forage basic information sharing platform construction and planting base, diversified processing enterprise technical services (2022E01039) and the Study on the effect of Apocynum venetum on alleviating heat stress in dairy cows (2024D01A44).
The authors declare that the research was conducted in the absence of any commercial or financial relationships that could be construed as a potential conflict of interest.
The author(s) declare that no Gen AI was used in the creation of this manuscript.
All claims expressed in this article are solely those of the authors and do not necessarily represent those of their affiliated organizations, or those of the publisher, the editors and the reviewers. Any product that may be evaluated in this article, or claim that may be made by its manufacturer, is not guaranteed or endorsed by the publisher.
The Supplementary material for this article can be found online at: https://www.frontiersin.org/articles/10.3389/fvets.2024.1528126/full#supplementary-material
1. Dong, JZ, Yang, JJ, and Wang, Y. Resources of Lycium species and related research progress. Zhongguo Zhong Yao Za Zhi. (2008) 33:2020–7.
2. Zenggen, L, Baolong, L, Huaixiu, W, and Yuchuan, S. Phytochemical profiles, nutritional constituents and antioxidant activity of black wolfberry (Lycium Ruthenicum Murr.). Ind Crop Prod. (2020) 154:112692. doi: 10.1016/j.indcrop.2020.112692
3. Su, L, Guzelinur, E, Zhang, Z, Hou, L, Li, X, Cui, Y, et al. Development and application of nutritional components of Lycium Ruthenicum Murray and its by-products. Xinjiang Anim Husb. (2024) 40:37–46. doi: 10.16795/j.cnki.xjxmy.2024.3.008
4. Jianfei, L, Yuan, G, Yang, J-L, and Duolong, D. Advance on alkaloids of Lycium genus. Chin Sci Bull. (2021) 67:332–50. doi: 10.1360/tb-2021-0229
5. Zhao, Y, and Lei, Y. Effects of Lycium Barbarum polysaccharides on production performance, rumen fermentation parameters, antioxidant capacity, and immune capacity of dairy cows. China Feed. (2024) 29:43–6. doi: 10.15906/j.cnki.cn11-2975/s.20241011
6. Zhang, Y, Guo, Y, Luo, Y, Du, M, Yin, X, Xu, X, et al. Integrated metabolomics and transcriptome revealed the effect of fermented Lycium Barbarum residue promoting Ovis Aries immunity. Front Immunol. (2022) 13:9436. doi: 10.3389/fimmu.2022.889436
7. Liu, G, Shenghua, Z, Qiong, L, and Huibi, X. A polysaccharide-protein complex from Lycium Barbarum upregulates cytokine expression in human peripheral blood mononuclear cells. Eur J Pharmacol. (2003) 471:217–22. doi: 10.1016/s0014-2999(03)01827-2
8. Vanessa, M, Rita, SA, Bruna, S, Xiaoying, Z, and Pires, DAC. Comparative studies on the anti-Neuroinflammatory and antioxidant activities of black and red goji berries. J Funct Foods. (2022) 92:105038. doi: 10.1016/j.jff.2022.105038
9. Tahidul, I, Xiaoming, Y, Singh, BT, and Baojun, X. Comparative studies on phenolic profiles, antioxidant capacities and carotenoid contents of red goji berry (Lycium Barbarum) and black goji berry (Lycium Ruthenicum). Chem Cent J. (2017) 11:59. doi: 10.1186/s13065-017-0287-z
10. Po-Yen, C, Tin-Han, S, Kai-Chieh, C, Jhin-Syuan, W, Chi-Ming, Y, and Yu-Sen, C. Potential of galled leaves of goji (Lycium Chinense) as functional food. BMC Nutr. (2020) 6:26. doi: 10.1186/s40795-020-00351-w
11. William, H, and George, WL. Official methods of analysis of AOAC International. Oxford: Oxford Academic (2006).
12. Van Soest, PJ, Robertson, JB, and Lewis, BA. Methods for dietary Fiber, neutral detergent Fiber, and nonstarch polysaccharides in relation to animal nutrition. J Dairy Sci. (1991) 74:3583–97. doi: 10.3168/jds.s0022-0302(91)78551-2
13. Mark, A, and Blaskovich, T. Antibiotics special issue: challenges and opportunities in antibiotic discovery and development. ACS Infect Dis. (2020) 6:1286–8. doi: 10.1021/acsinfecdis.0c00331
14. Gerard, DW. Antibiotic adjuvants: rescuing antibiotics from resistance. Trends Microbiol. (2016) 24:862–71. doi: 10.1016/j.tim.2016.06.009
15. Ha, DR, Haste, NM, and Gluckstein, DP. The role of antibiotic stewardship in promoting appropriate antibiotic use. Am J Lifestyle Med. (2017) 13:376–83. doi: 10.1177/1559827617700824
16. Bojana, V, Milinčić Danijel, D, Mirjana, M, Jelena, D, Tijana, I, Kostić Aleksandar, Ž, et al. Health benefits and applications of goji berries in functional food products development: a review. Antioxidants. (2022) 11:248. doi: 10.3390/antiox11020248
17. Yuebang, Y, Fang, W, Mei, Y, Bin, T, Yulong, Y, Jiashun, C, et al. Lycium Barbarum polysaccharides as antibiotic substitutes improve growth performance, serum immunity, antioxidant status, and intestinal health for weaned piglets. Front Microbiol. (2022) 12:993. doi: 10.3389/fmicb.2021.819993
18. Baoming, T, Mengyao, L, Wei, A, Li, Y, Jiawei, Z, Yilin, L, et al. Lycium Barbarum relieves gut microbiota Dysbiosis and improves colonic barrier function in mice following antibiotic perturbation. J Funct Foods. (2020) 71:103973. doi: 10.1016/j.jff.2020.103973
19. Duan, P, Zhang, L, Lihong, H, and Guishan, X. Biological function and application in livestock and poultry production of Lycium Barbarum and its extracts. Chin J Anim Nutr. (2023) 35:4207–15. doi: 10.12418/CJAN2023.390
20. Liu, YL, Runsheng, Y, Saisai, L, Yulan, D, Jun, Y, and Xin, Y. Effect of dietary Lycium Barbarum polysaccharide on growth performance and immune function of broilers. J Appl Poult Res. (2017) 26:200–8. doi: 10.3382/japr/pfw063
21. Zhuang, H, Zhen, L, Jinjin, H, Jiandong, L, and Peng, Y. Effects of Chinese wolfberry and Astragalus extract on the antioxidant capacity of Tibetan pig liver. PLoS One. (2021) 16:5749. doi: 10.1371/journal.pone.0245749
22. Hao, W, Shenfei, W, Jing, Z, and Shao-Ping, L. Effects of extraction methods on immunology activity and chemical profiles of Lycium Barbarum polysaccharides. J Pharm Biomed Anal. (2020) 185:113219. doi: 10.1016/j.jpba.2020.113219
23. Xiaoxue, Z, Guoze, L, Fadi, L, Deyin, Z, Lvfeng, Y, Yuan, Z, et al. Effect of feed efficiency on growth performance, body composition, and fat deposition in growing Hu lambs. Anim Biotechnol. (2021) 34:183–98. doi: 10.1080/10495398.2021.1951747
24. Lina, L, Baoju, K, Qian, J, and Chen, JS. Effects of dietary Lycium Barbarum polysaccharides on growth performance, digestive enzyme activities, antioxidant status, and immunity of broiler chickens. Poult Sci. (2020) 99:744–51. doi: 10.1016/j.psj.2019.10.043
25. Hao, Z, Li, Z, Huo, J, Chu, Y, Li, J, Yu, X, et al. Effects of Chinese wolfberry and Astragalus extracts on growth performance, pork quality, and unsaturated fatty acid metabolism regulation in Tibetan fragrant pigs. Anim Sci J. (2021) 92:e13581. doi: 10.1111/asj.13581
26. Guo, Y, Liu, J, Tuo, Q, Zhang, D, Wanapat, M, and Xin, G. The effect of dietary supplementation of Lycium Barbarum leaves on the growth performance, organ indexes and intestinal microflora of rats. Front Vet Sci. (2024) 11:1416793. doi: 10.3389/fvets.2024.1416793
27. Zhentao, H, Shuai, L, Xiaolu, W, Shuting, C, Xianliang, Z, Lei, H, et al. Effect of mixed meal replacement of soybean meal on growth performance, nutrient apparent digestibility, and gut microbiota of finishing pigs. Front Vet Sci. (2024) 11:1486. doi: 10.3389/fvets.2024.1321486
28. Dawei, Y, Yamei, Y, and Jun, L. Isolation, structure and biological activity of polysaccharides from the fruits of Lycium Ruthenicum Murr: a review. Carbohydr Polym. (2022) 291:119618. doi: 10.1016/j.carbpol.2022.119618
29. Xulei, L, Fuyao, L, Tianhai, Y, Shenghua, C, Metha, W, and Fujiang, H. Cistanche Deserticola addition improves growth, digestibility, and metabolism of sheep fed on fresh forage from alfalfa/tall fescue pasture. Animals. (2020) 10:668. doi: 10.3390/ani10040668
30. Lin, R, Lin, Y, Wang, J, and Peng, L. Regulation of mouse digestive function, intestinal mucosal barrier function, and inflammatory reaction by Lycium Barbarum polysaccharide pathway through myosin light chain kinase. Heliyon. (2024) 10:e29795. doi: 10.1016/j.heliyon.2024.e29795
31. Ju, H, Hao, X, Zhang, X, Liu, S, Zhang, H, Xiang, B, et al. Effects of dietary Lycium Barbarum polysaccharide on nutrient apparent digestibility, energy metabolism, nitrogen metabolism and serum immune indexes of lambs. Chin J Anim Nutr. (2022) 34:7960–9. doi: 10.3969/j.issn.1006-267x.2022.12.044
32. Liu, H, Zhou Jianwei, A, Degen, A, Hongshan, L, Xu-Liang, C, Lizhuang, H, et al. A comparison of average daily gain, apparent Digestibilities, energy balance, rumen fermentation parameters, and serum metabolites between yaks (Bos Grunniens) and Qaidam cattle (Bos Taurus) consuming diets differing in energy level. Anim Nutr. (2023) 12:77–86. doi: 10.1016/j.aninu.2022.07.015
33. Chuan, L, Jiantong, Z, Yanjiao, L, Xianghui, Z, Huan, L, Li Kairong, Q, et al. Glutamate supplementation improves growth performance, rumen fermentation, and serum metabolites in heat-stressed Hu sheep. Front Nutr. (2022) 9:1386. doi: 10.3389/fnut.2022.851386
34. Yao, G, Ruochen, Y, Chunhui, D, Yong, W, Qing-Hong, H, Shoukun, J, et al. Effect of Dioscorea opposite waste on growth performance, blood parameters, rumen fermentation and rumen bacterial Community in Weaned Lambs. J Integr Agric. (2023) 22:1833–46. doi: 10.1016/j.jia.2022.10.002
35. Duan, P, Rehemujiang, H, Zhang, L, Mulong, L, Li, C, Lihong, H, et al. Lycium Barbarum (wolfberry) branches and leaves enhance the growth performance and improve the rumen microbiota in Hu sheep. Animals. (2024) 14:1610. doi: 10.3390/ani14111610
36. Hongjin, L, Xu Tianwei, X, Shixiao, ML, Xueping, H, Xungang, W, Zhang Xiaoling, H, et al. Effect of dietary concentrate to forage ratio on growth performance, rumen fermentation and bacterial diversity of Tibetan sheep under barn feeding on the Qinghai-Tibetan plateau. PeerJ. (2019) 7:e7462. doi: 10.7717/peerj.7462
37. Xin, Y, Shoukun, J, Chunhui, D, Tian Peizhi, J, Sisi, YH, Yingjie, Z, et al. Age-related changes in the ruminal microbiota and their relationship with rumen fermentation in lambs. Front Microbiol. (2021) 12:135. doi: 10.3389/fmicb.2021.679135
38. Kansagara, YK, Savsani, HH, Chavda, MR, Chavda, JA, Belim, SY, Makwana, KR, et al. Rumen microbiota and nutrient metabolism: a review. Bhart Kris Anus Patr. (2022) 37:320–7. doi: 10.18805/bkap486
39. Zhongyan, L, Zhihui, X, Zongzhuan, S, Yuanchun, T, and Hong, S. Dietary energy level promotes rumen microbial protein synthesis by improving the energy productivity of the ruminal microbiome. Front Microbiol. (2019) 10:847. doi: 10.3389/fmicb.2019.00847
40. Paola, C, Giulio, C, Filippo, B, Elisa, C, Laura, M, Federica, R, et al. Dietary supplementation with goji berries (Lycium Barbarum) modulates the microbiota of digestive tract and Caecal metabolites in rabbits. Animals. (2022) 12:121. doi: 10.3390/ani12010121
41. Jiashun, C, Lina, L, Qian, J, Baoju, K, Yinghui, L, and Jie, Y. Effects of dietary supplementation of Lycium Barbarum polysaccharides on growth performance, immune status, antioxidant capacity and selected microbial populations of weaned piglets. J Anim Physiol Anim Nutr. (2019) 104:1106–15. doi: 10.1111/jpn.13247
42. Xinyu, G, Yuzhu, S, Lv Weibing, P, Xiaoning, LX, Luo Yuzhu, H, Jiang, WJ, et al. Sex differences in rumen fermentation and microbiota of Tibetan goat. Microb Cell Factories. (2022) 21:55. doi: 10.1186/s12934-022-01783-8
43. Ruth, H, De, MMC, Hugo, J, Alejandro, R, and Alejandro, C-Q. Functional and phylogenetic characterization of Bacteria in bovine rumen using fractionation of ruminal fluid. Front Microbiol. (2022) 13:3002. doi: 10.3389/fmicb.2022.813002
44. Yang, X, Fan, X, Jiang, H, Zhang, Q, Basangwangdui, ZQ, Dang, S, et al. Simulated seasonal diets Alter yak rumen microbiota structure and metabolic function. Front Microbiol. (2022) 13:6285. doi: 10.3389/fmicb.2022.1006285
45. Ali, A, Ahmad, ZJ, Bo, ZL, Chao, Y, Qudratullah, K, Shah Tariq, D, et al. Dynamics of rumen bacterial composition of yak (Bos Grunniens) in response to dietary supplements during the cold season. PeerJ. (2021) 9:e11520. doi: 10.7717/peerj.11520
46. Shiho, F, Yoshiyuki, W, and Kazuyuki, T. The gut microbiome: A Core regulator of metabolism. J Endocrinol. (2023) 256:111. doi: 10.1530/joe-22-0111
47. Kou, X, Ma, Q, Liu, Y, Khan, MZ, Wu, B, Chen, W, et al. Exploring the effect of gastrointestinal Prevotella on growth performance traits in livestock animals. Animals. (2024) 14:965. doi: 10.3390/ani14131965
48. Giuseppe, C, Corrado, D, Matteo, D, Andrea, S, Federica, M, Breanne, MA, et al. Exploring the relationship between bacterial genera and lipid metabolism in bovine rumen. Animal. (2022) 16:100520. doi: 10.1016/j.animal.2022.100520
49. Ronald, K, Peter, R, Eric, A, Kenters Nikki, J, William, K, Joan, NS, et al. Rumen Lachnospiraceae isolate Nk3a20 exhibits metabolic flexibility in response to substrate and Coculture with a methanogen. Appl Environ Microbiol. (2023) 89:e0063423. doi: 10.1128/aem.00634-23
50. Masami, M, Fumiko, N, Hiroshi, S, and Ryuichiro, T. Paraprevotella Clara gen. Nov., Sp. Nov. and Paraprevotella Xylaniphila Sp. Nov., members of the family 'Prevotellaceae' isolated from human Faeces. Int J Syst Evol Microbiol. (2009) 59:1895–900. doi: 10.1099/ijs.0.008169-0
51. Hui-Zheng, Z, Yubin, L, Qingyu, M, Wenzhi, H, Li Xiaojuan, W, Man-Si, DL, et al. Xiaoyaosan improves depressive-like behavior in rats with chronic immobilization stress through modulation of the gut microbiota. Biomed Pharmacother. (2023) 112:108621. doi: 10.1016/j.biopha.2019.108621
52. Ruizhi, H, Ziyu, H, Ming, L, Jijun, T, Hongfu, Z, De-Xing, H, et al. Dietary Protocatechuic acid ameliorates inflammation and up-regulates intestinal tight junction proteins by modulating gut microbiota in Lps-challenged piglets. J Anim Sci Biotechno. (2020) 11:92. doi: 10.1186/s40104-020-00492-9
53. Tekletsadik, E, Solomon, MB, and Mengistu, U. The effect of barley bran, linseed meal and their mixes supplementation on the performances, carcass characteristics and economic return of arsi-bale sheep. Small Rumin Res. (2013) 114:35–40. doi: 10.1016/j.smallrumres.2013.05.010
54. Han, H, Yaozhong, W, and Chen, P. Effects of beer yeast and wolfberry on production performance and economic benefits of broilers. Shanghai J Anim Husb Vet Med. (2008) 44:63–5.
Keywords: residual black wolfberry fruit, Duolang sheep, growth performance, rumen microorganisms, economic benefits
Citation: Hou L, Duan P, Yang Y, Shah AM, Li J, Xu C and Guo T (2025) Effects of residual black wolfberry fruit on growth performance, rumen fermentation parameters, microflora and economic benefits of fattening sheep. Front. Vet. Sci. 11:1528126. doi: 10.3389/fvets.2024.1528126
Received: 14 November 2024; Accepted: 27 December 2024;
Published: 10 January 2025.
Edited by:
Qingbiao Xu, Huazhong Agricultural University, ChinaReviewed by:
Hao Lizhuang, Qinghai University, ChinaCopyright © 2025 Hou, Duan, Yang, Shah, Li, Xu and Guo. This is an open-access article distributed under the terms of the Creative Commons Attribution License (CC BY). The use, distribution or reproduction in other forums is permitted, provided the original author(s) and the copyright owner(s) are credited and that the original publication in this journal is cited, in accordance with accepted academic practice. No use, distribution or reproduction is permitted which does not comply with these terms.
*Correspondence: Tongjun Guo, Z3VvdGFveGpAMTI2LmNvbQ==
†These authors have contributed equally to this work and share first authorship
Disclaimer: All claims expressed in this article are solely those of the authors and do not necessarily represent those of their affiliated organizations, or those of the publisher, the editors and the reviewers. Any product that may be evaluated in this article or claim that may be made by its manufacturer is not guaranteed or endorsed by the publisher.
Research integrity at Frontiers
Learn more about the work of our research integrity team to safeguard the quality of each article we publish.