- 1College of Veterinary Medicine, Inner Mongolia Agricultural University, Hohhot, China
- 2Key Laboratory of Clinical Diagnosis and Treatment Techniques for Animal Disease, Ministry of Agriculture, Hohhot, China
- 3Laboratory of Young Animal Physiology and Pathology, Institute of Veterinary Medicine, Mongolian University of Life Sciences, Ulaanbaatar, Mongolia
- 4Shenyang Fengmei Biotechnology Co., Ltd., Shenyang, China
During the late laying period, the intestinal barrier of laying hens is susceptible to damage, resulting in enteric infections and even systemic inflammatory responses, posing a major challenge for the poultry industry. Therefore, it is crucial to investigate methods for addressing intestinal inflammation in late laying hens. In order to maximize the production potential of egg laying chickens, farmers usually use various feed additives to prevent damage to the intestinal barrier. Composite yeast cultures have shown advantages in broiler applications. This study aims to assess the impact of composite yeast culture (CYC) on the intestinal barrier function, inflammatory cytokines, and microbial community structure of Hy-Line Brown laying hens. A total of 160 healthy Hy-Line Brown hens, aged 58 weeks and of similar weight, were randomly assigned to two groups, with four replicates per group and 20 hens in each replicate. The control group was fed a basal diet (Con), while the experimental group was provided with a diet supplemented with 40 g/kg of composite yeast culture (CYC). The test period was 25 days. The results indicated that: compared to the control group, CYC significantly improved the egg production rates of hens during days 11–15, 16–20, and 21–25 (p < 0.05). CYC significantly enhanced the relative mRNA expression levels of occludin, claudin-1, zonula occludens-1 (ZO-1), and mucin 2 (Muc2) in the intestinal tract (p < 0.05), while reducing the relative expression levels of pro-inflammatory factors TNF-α and IFN-γ (p < 0.05), and increasing the levels of anti-inflammatory factors IL-4, IL-10, and TGF-β1 (p < 0.05). CYC significantly increased the abundance of Bifidobacterium pseudocatenulatum and Faecalibacterium prausnitzii in the intestine. These findings suggest that the composite yeast culture (CYC) can improve the structure of the intestinal microbial community. In conclusion, CYC may enhance egg production rates, reduce inflammatory responses, and strengthen intestinal barrier function by modulating the composition of the intestinal microbiota in late laying hens.
1 Introduction
As the rearing time of laying hens extends from 72 weeks to 100 weeks, the late laying period accounts for more than half of the laying cycle (1). In intensive poultry farming systems, the metabolic activity of hens during peak laying, coupled with various oxidative stresses, leads to a decline in intestinal barrier function as the hens age. This deterioration can result in gut-derived infections and even systemic inflammatory responses, subsequently causing a decrease in egg production and a reduction in their productive lifespan, resulting in significant economic losses (2, 3). Therefore, the production potential of hens in the late laying period largely depends on a healthy gastrointestinal tract, with a complete intestinal barrier being particularly crucial.
At the same time, issues such as the misuse of antibiotics and illegal additives in livestock farming have come to light (4). To ensure the safety of animal-derived food products, China implemented a comprehensive ban on the addition of antibiotics to animal feed in 2020. Consequently, to address these issues, various feed additives have been sought and adopted to prevent damage to the intestinal barrier in laying hens, thereby reducing the occurrence of chronic intestinal inflammation in hens during the late laying period (5, 6). In recent years, probiotics and their cultures have emerged as environmentally friendly and efficient growth promoters and immune modulators, demonstrating significant potential for positive impacts on animal health and human welfare, and are considered ideal alternatives to antibiotics (7).
It has been demonstrated that yeast cultures can improve the immune function of broiler chickens, enhance nutrient metabolism, and increase production performance (8). Furthermore, yeast culture (specifically, Saccharomyces cerevisiae) additives have been shown to improve nutrient utilization and meat quality in broilers, as well as to enhance the ecological environment (9, 10). Yeast is commonly used as a feed additive in the forms of live yeast, dried yeast, fermentation products, and components of yeast cell walls (11). Beta-glucans and mannan oligosaccharides, the primary components of yeast cell walls, have been shown to play a significant role in the antioxidant capacity and immune response in animals such as broilers, sheep, and calves (12–15). Additionally, it has been widely reported that yeasts can enhance food digestion and nutrient absorption in broiler chickens by modulating or optimizing the composition of the intestinal microbiota, thereby improving growth performance (16, 17).
Currently, numerous probiotic fermentation products for poultry have emerged, but their quality and efficacy vary significantly. The composite yeast culture (CYC) is a microbial fermentation preparation previously developed and commercialized by our research group. It is derived from two high-activity yeast strains isolated from naturally fermented milk in the Inner Mongolian grasslands, fermented with a specific medium using a specialized fermentation process (18). The main active components of CYC include beta-glucans, mannan oligosaccharides, peptides, amino acids, and organic acids. Preliminary studies have shown that this microbial ecological preparation promotes growth and improves immune function in sheep (18). Additionally, research has found that beta-glucans can enhance production performance, egg quality, and immune function in laying hens (19). Mannan interacts with transmembrane glycoprotein receptors that recognize glycosylated compounds, stimulating macrophages in vivo and promoting phagocytosis (20). Although many studies indicate that CYC is beneficial for host health, this has yet to be confirmed in laying hens during the late laying period. Therefore, this study aims to investigate the effects of CYC feeding on growth performance, intestinal barrier function, inflammatory responses, and the intestinal microbiota structure in Hy-Line Brown laying hens during the late laying period, with the goal of providing a theoretical basis for the clinical application of such composite yeast cultures in the poultry industry.
2 Materials and methods
2.1 Preparation of composite yeast culture
The composite yeast culture (CYC) is a product of previous research conducted by our group, containing five primary active components, with their minimum concentrations listed in Table 1. A strain of Saccharomyces cerevisiae (BC) and a strain of Kluyveromyces marxianus (XR4) from the microbial ecological preparation strain bank of the Veterinary College of Inner Mongolia Agricultural University were mixed in a 1:1 ratio with distilled water to create a microbial suspension with a concentration of 109 CFU/mL. This suspension was inoculated at an 8% inoculum rate into a solid-state fermentation substrate, to which water was added to achieve a moisture content of 40%. The fermentation lasted for 72 h. The solid-state fermentation substrate was composed of corn, rice bran, cottonseed, wheat, and bran. The fermentation process was carried out in a laboratory environment, with the temperature of the substrate recorded every 3 h. When the fermentation time reached 24 h and the core temperature reached 40°C, the substrate was turned over and continued to ferment. After 72 h of fermentation, the composite yeast culture was dried and ground at low temperatures (45–50°C) and then packaged for use.
2.2 Experimental design and feeding management
The basic diet was formulated based on the NRC (1994) guidelines, utilizing a corn-soybean meal diet. The composition and nutritional levels of the basic diet are presented in Table 2. Prior to the start of the experiment, strict disinfection of the housing environment was conducted, and during the trial, manure was cleaned and the chicken house was disinfected daily.
This feeding trial was conducted at the Hy-Line Brown laying hen farm of Fengmei Biotechnology Co., Ltd. in Shenyang, Liaoning Province. A total of 160 healthy Hy-Line Brown laying hens, approximately 58 weeks old and of similar body weight, were randomly assigned to two experimental groups, each consisting of four replicates of 20 hens. The control group was fed a basic diet, while the experimental group received a diet supplemented with CYC (40 g/kg), with the supplementation level determined based on previous research conducted by our group on composite yeast cultures. The pre-feeding period lasted for 3 days, followed by a 25 day trial period. The hens were housed in four-tier stepped cages with three hens per cage, and automatic waterers were available. Feed three times a day (at 04:00, 11:00, and 17:00), and during the trial period, they can eat as they please. A 16-h photoperiod was maintained with natural lighting supplemented by artificial light controlled by a lighting program controller. The henhouse temperature was kept at 22 ± 2°C, utilizing natural ventilation along with longitudinal negative pressure ventilation.
2.3 Production performance
During the experiment, eggs were collected and weighed from each replicate daily at 9:00 AM. Additionally, leftover feed was collected and weighed every 3 days. The calculations were performed over a 5-day cycle, and the following metrics were calculated: egg production rate, average egg weight, average feed intake, and feed-to-egg ratio.
2.4 Sample collection
At the end of the feeding period, 8 chickens from each group (2 chickens per replicate) were randomly selected. They were rendered unconscious via CO2 intravenous injection, followed by euthanasia through cervical dislocation. Subsequently, laboratory personnel wearing sterile gloves performed dissections. Approximately 10 g of intestinal content from the jejunum was collected using sterilized sampling tools and immediately transferred to a 2 mL sterile, enzyme-free cryogenic tube. A 2 cm segment of the jejunum was also collected under sterile conditions and transferred to another 2 mL sterile, enzyme-free cryogenic tube. The collected intestinal tissue and contents were rapidly frozen in liquid nitrogen and stored at −80°C for subsequent assessments of tight junction proteins, inflammatory cytokines, and metagenomic analysis. Additionally, intestinal tissue samples were collected for later histological analysis.
2.5 Histological analysis
For each hen, a 2 cm segment of the jejunum was collected, and the intestinal contents were carefully washed away using sterile PBS solution. Excess moisture was removed with filter paper, and the tissue was then fixed in 4% paraformaldehyde solution (Biosharp, Hefei, China) for at least 48 h. The samples were subsequently embedded in paraffin, and sections of 4 μm thickness were prepared using a microtome. Hematoxylin and eosin (HE) staining was performed on the tissue sections. Finally, the samples were observed under an optical microscope (NiKon DS-Fi3, Tokyo, Japan) at 40× magnification, and the heights of the intestinal villi (VH), crypt depths (CD), and the ratio of villus height to crypt depth (VH/CD) were measured and calculated using an image processing and analysis system (NIS-Elements F 5.21.00).
2.6 Gene expression measurement
Total RNA was extracted from the jejunal samples using the Total RNA Mini Kit (GeneBetter, Beijing, China). The quality and concentration of the obtained total RNA were measured using a microplate reader (BioTek Synergy HT, Richmond, United States). Using HiScript III RT SuperMix (Vazyme, Nanjing, China), 1 ng of total RNA was reverse transcribed to synthesize complementary DNA (cDNA).
Amplification was performed using the CFX96 Touch™ Real-time PCR System (Bio-Rad, California, United States) with SYBR Green (Biosharp, Hefei, China), ddH₂O, cDNA, and both forward and reverse primers. The entire RT-qPCR process was monitored to obtain the cycle threshold (Ct) values, and the expression levels of target genes were calculated using the method. The primer sequences for all genes (β-actin, claudin-1, occludin, ZO-1, Muc2, IL-1β, TNF-α, IFN-γ, IL-4, IL-10, TGF-β1) can be found in Table 3. The reaction system consisted of 10 μL, and the program included an initial denaturation step (95°C, 2 min), followed by 40 cycles of denaturation (95°C, 15 s) and annealing/extension (60°C, 30 s). The entire experimental procedure was conducted according to the manufacturer’s instructions.
2.7 Metagenome sequencing
DNA extraction from the jejunal contents was carried out by Magergene (Guangdong Magigene Biotechnology Co., Ltd., Guangzhou, China) using a commercial kit in accordance with the manufacturer’s instructions. The integrity and purity of the extracted DNA were assessed using 1% agarose gel electrophoresis. Additionally, the concentration and purity of the DNA were measured using a Qubit 3.0 (Thermo Fisher Scientific, Waltham, United States) and a NanoDrop One (Thermo Fisher Scientific, Waltham, United States).
Following the manufacturer’s recommendations, the ALFA-SEQ DNA Library Prep Kit was used to generate sequencing libraries, including the addition of index codes. The quality of the libraries was assessed using the Qubit 4.0 fluorometer (Life Technologies, Grand Island, NY) and the QSEP400 high-throughput nucleic acid analysis system (Houze Biology Technology Co., China). Finally, the libraries were sequenced on the Illumina NovaSeq 6000 platform, yielding 150 bp paired-end reads for subsequent analysis. Use QIIME software to evaluate the alpha diversity index and display the results in a box plot. Use R software to draw PCoA and abundance maps.
2.8 Statistical analysis
GraphPad Prism 9.5.1 (GraphPad Software, Inc., San Diego, CA, United States) was used to analyze the data. Independent samples t-tests were conducted to compare the differences between groups. If the data are normally distributed but have heterogeneous variances, Welch’s correction t-test was employed. For non-normally distributed data, the Mann–Whitney U test, a non-parametric test, was utilized. The Spearman statistical method was used to analyze the correlation coefficients between the gut microbiota and the indicators of gut barrier function and inflammatory cytokines. Moreover, a p < 0.05 was considered statistically significant.
3 Results
3.1 Production performance
Based on the results presented in Table 4, there were no significant differences in average daily feed intake and feed-to-egg ratio between the two groups during the experimental phases (days 1–5, 6–10, 11–15, 16–20, and 21–25) (p > 0.05). However, during the periods of days 11–15, 16–20, and 21–25, the egg production rates of the CYC group were higher than those of the control group by 4.75, 6.25, and 7%, respectively, with significant differences (p < 0.05). In the period from days 6–10, the average egg weight of the CYC group was 0.49 g heavier than that of the control group, which was also statistically significant (p < 0.05). Additionally, the CYC group showed an increasing trend in average egg weight compared to the control group during the periods of days 11–15, 16–20, and 21–25, although these differences were not statistically significant (p > 0.05).
Overall, the inclusion of CYC in the diet of laying hens during the late egg-laying period positively influences egg production rates and average egg weight without adversely affecting feed intake or feed efficiency. These findings suggest that CYC could be a beneficial supplement for improving the production performance of laying hens during this critical phase.
3.2 Investigation results on the barrier function of the jejunum
HE staining of jejunal tissue was performed (Figure 1A). The results showed that the villi in the Con group were relatively loosely arranged, while the villi in the CYC group were closely packed and exhibited relatively intact structures. The height of the jejunal villi in the CYC group (Figure 1B) and the ratio of villus height to crypt depth (Figure 1D) were significantly higher than those in the Con group (p < 0.01). There was no significant difference in crypt depth between the two groups (Figure 1C) (p > 0.05).
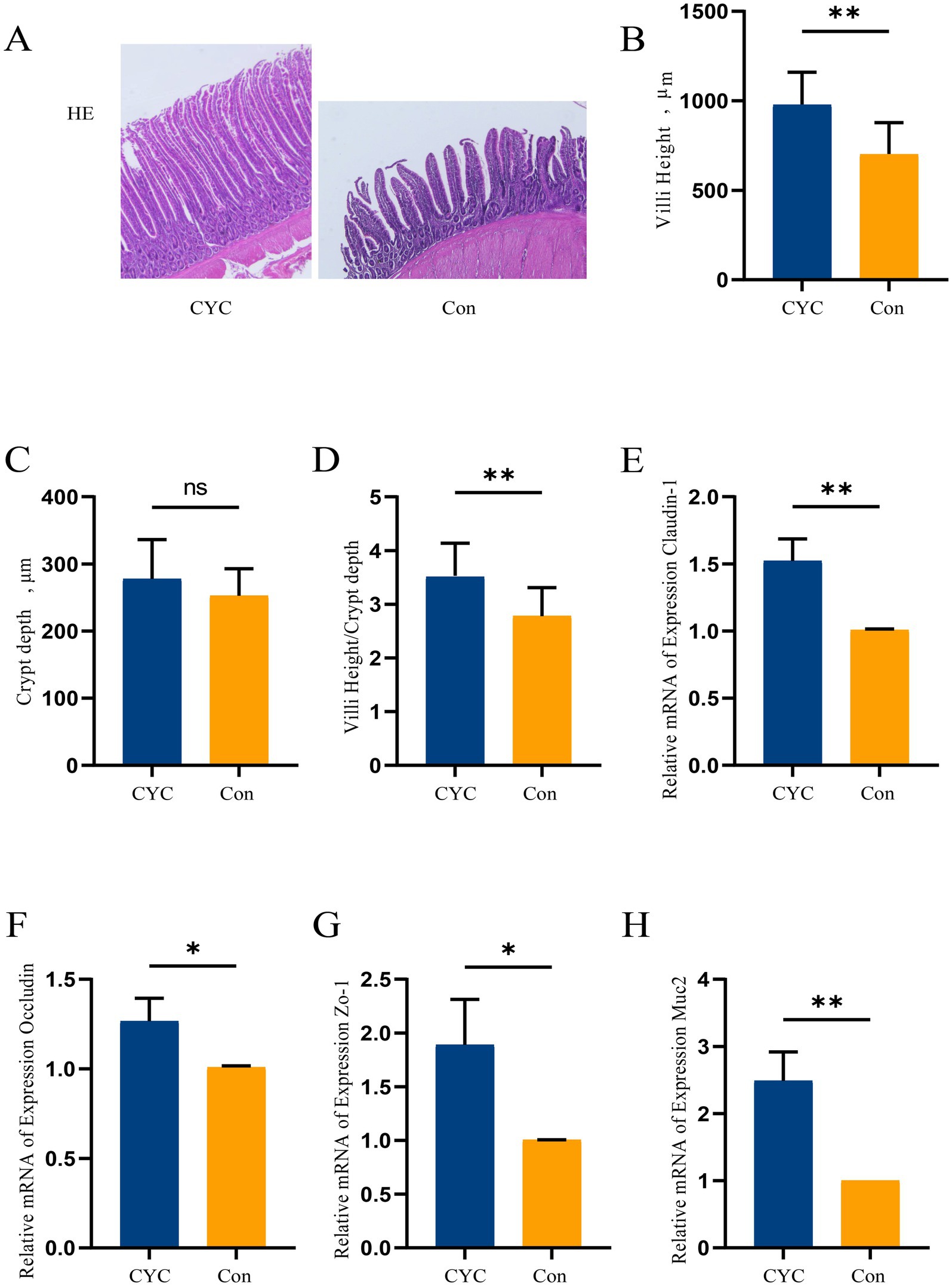
Figure 1. The impact of adding CYC on the jejunal barrier in laying hens during the late egg-laying period. (A) Observation of jejunal tissue morphology under 40× magnification using HE staining. (B) Measurement and calculation of jejunal villus height (VH). (C) Measurement and calculation of jejunal crypt depth (CD). (D) Calculation of the ratio of jejunal villus height to crypt depth (VII/CD). (E) Relative expression level of claudin-1 mRNA in jejunal tissue. (F) Relative expression level of occludin mRNA. (G) Relative expression level of zonula occludens-1 (ZO-1) mRNA in jejunal tissue. (H) Relative expression level of mucin 2 (Muc2) mRNA in jejunal tissue. *p < 0.05 and **p < 0.01.
According to the qPCR results, the mRNA expression levels of claudin-1 (Figure 1E) and Muc2 (Figure 1H) in the jejunal tissue of the CYC group were significantly higher than those in the Con group (p < 0.01). Additionally, the mRNA expression levels of occludin (Figure 1F) and ZO-1 (Figure 1G) in the CYC group were also significantly higher than those in the Con group (p < 0.05). Taken together, these findings suggest that feeding CYC may improve the structure of intestinal villi and enhance the intestinal barrier function in laying hens.
3.3 I results of the study on inflammatory cytokines in jejunal tissue
Results of gene expression of inflammatory cytokines (Figure 2). The study found that the relative expression level of the pro-inflammatory cytokine TNF-α (Figure 2B) in the jejunal tissue of the CYC group was significantly lower than that of the Con group (p < 0.01). Additionally, the relative expression level of IFN-γ (Figure 2C) in the CYC group was significantly lower than that in the Con group (p < 0.05). There was a trend towards decreased expression of IL-1β (Figure 2A) in the CYC group, although this did not reach statistical significance (p > 0.05). Conversely, the relative expression level of the anti-inflammatory cytokine IL-4 (Figure 2D) in the CYC group was significantly higher than that of the Con group (p < 0.01). The relative expression levels of IL-10 (Figure 2E) and TGF-β1 (Figure 2F) in the CYC group were also significantly higher than those in the Con group (p < 0.05). In summary, feeding CYC to laying hens may primarily modulate intestinal inflammatory responses by reducing levels of IFN-γ and TNF-α, while enhancing levels of IL-4, IL-10, and TGF-β1, thereby influencing immune function.
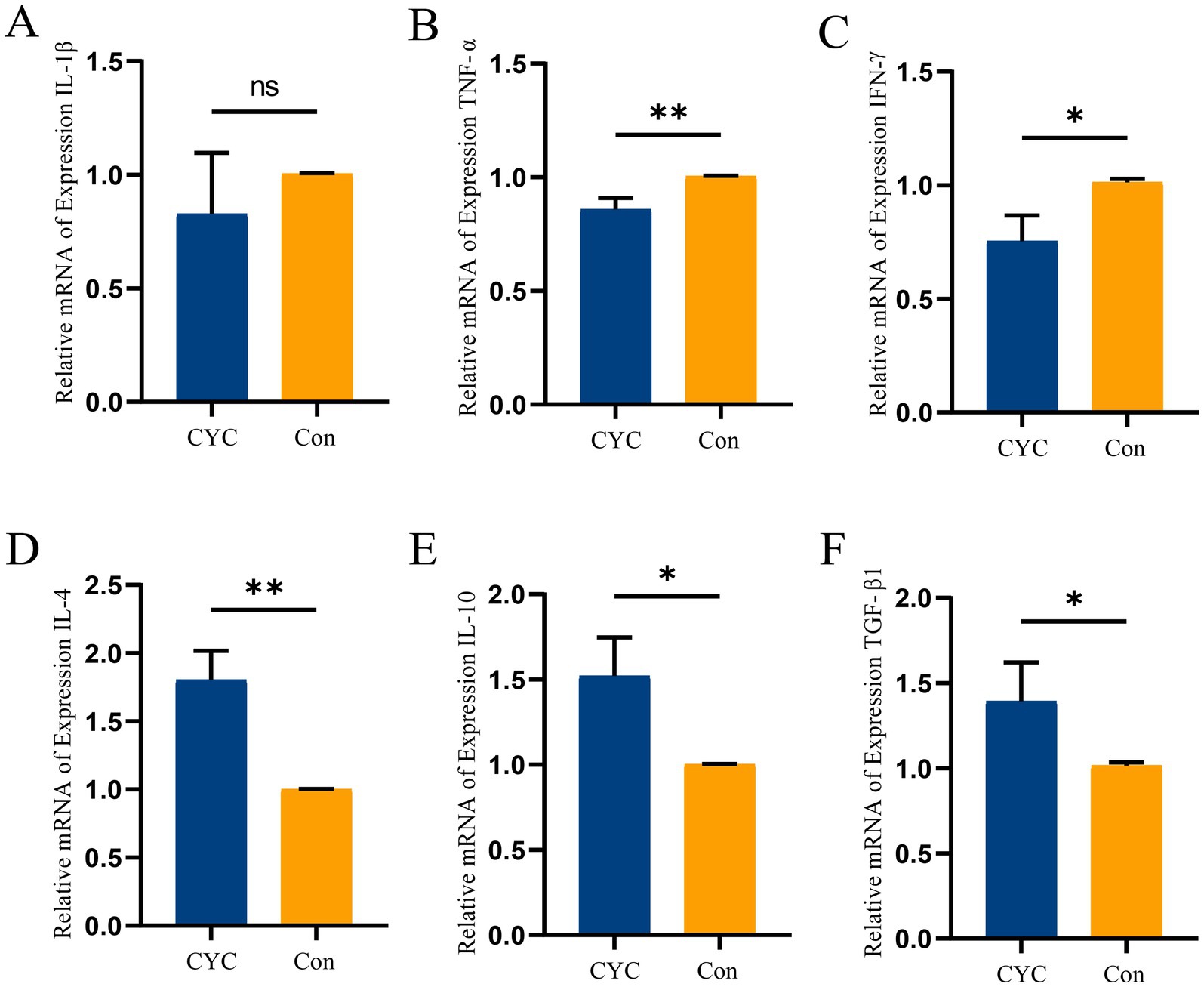
Figure 2. The impact of CYC on the expression of cytokine genes in the jejunum of laying hens during the late laying period. (A) Relative expression levels of mRNA for IL-1β in jejunal tissue. (B) Relative expression levels of mRNA for TNF-α in jejunal tissue. (C) Relative expression levels of mRNA for IFN-γ in jejunal tissue. (D) Relative expression levels of mRNA for IL-4 in jejunal tissue. (E) Relative expression levels of mRNA for IL-10 in jejunal tissue. (F) Relative expression levels of mRNA for TGF-β1 in jejunal tissue. *p < 0.05 and **p < 0.01.
3.4 Metagenomic analysis
We investigated the jejunal microbial structure of laying hens in the Con and CYC groups during the late laying period. The alpha diversity of microbial communities (Figure 3A) showed that there was no significant difference (p > 0.05) in Chao1, Shannon, Simpson, and Species indices between the CYC group and the Con group. The results of the β-diversity principal coordinate analysis (Figure 3B) indicated a significant separation distance between the Con and CYC groups, with a more pronounced intra-group clustering, suggesting a distinct difference in microbial composition between the two groups. At the species level, the composition results (Figures 3C,D) revealed that the abundances of Escherichia coli, Bifidobacterium pseudocatenulatum, Faecalibacterium prausnitzii, Pseudomonas stutzeri, Hydrogenophaga pseudoflava, Pseudomonas oryzae, and Deltaproteobacteria bacterium were significantly increased in the CYC group. In contrast, Ligilactobacillus salivarius, Lactobacillus crispatus, Enterococcus cecorum, Limosilactobacillus reuteri, and Ligilactobacillus murinus showed higher abundances in the Con group.
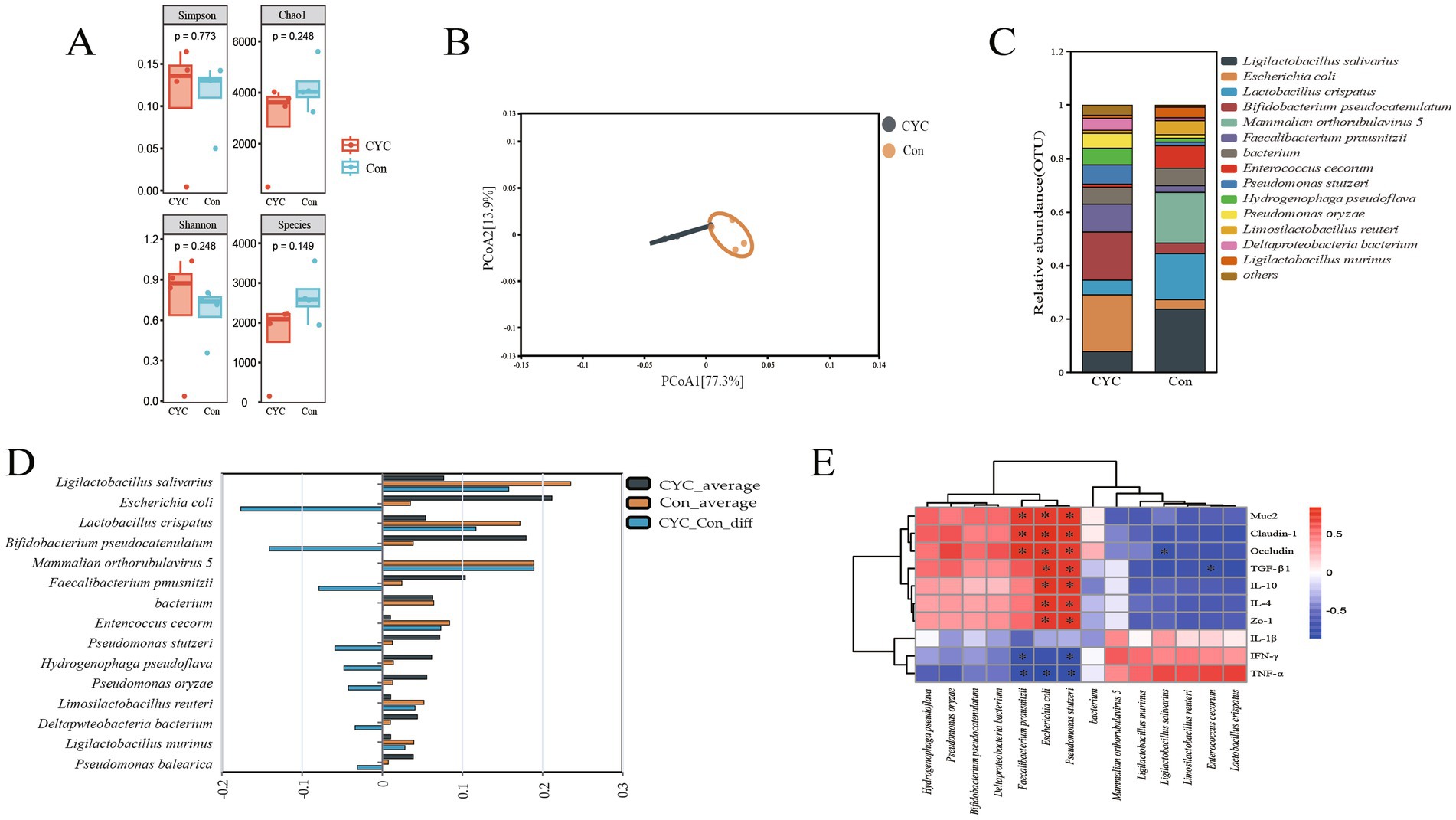
Figure 3. Effect of CYC supplementation on microbial structure in the jejunum during the late laying period. (A) α-diversity analysis. (B) Principal coordinate analysis (PCoA). (C) Bar chart of the relative abundance of the top 15 species at the species level. (D) Analysis of difference between two groups at different levels. (E) Heatmap of the correlation between the microbiota and relevant physiological indicators, where red indicates positive correlations and blue indicates negative correlations. *p < 0.05.
To further investigate the impact of the microbial composition in the jejunal tract on gut barrier function and inflammatory cytokines in laying hens during the late laying period, this study employed Spearman correlation analysis to assess the relationships between the top 14 most abundant microbial species and relevant physiological indicators. The correlation analysis results for the intestinal microbial structure of laying hens during the late laying period (Figure 3E) revealed that Faecalibacterium prausnitzii exhibited a significant positive correlation with Muc2, occludin, and claudin-1 (p < 0.05), while showing a significant negative correlation with IFN-γ and TNF-α (p < 0.05). Additionally, Escherichia coli was significantly positively correlated with Muc2, claudin-1, occludin, TGF-β1, IL-10, IL-4, and ZO-1 (p < 0.05) and negatively correlated with TNF-α (p < 0.05). Pseudomonas stutzeri displayed significant positive correlations with Muc2, claudin-1, occludin, TGF-β1, IL-10, IL-4, and ZO-1 (p < 0.05), alongside significant negative correlations with IFN-γ and TNF-α (p < 0.05). Lastly, Ligilactobacillus salivarius showed a significant negative correlation with occludin (p < 0.05), while Enterococcus cecorum and Lactobacillus crispatus were both significantly negatively correlated with TGF-β1 (p < 0.05).
In summary, the addition of CYC not only altered the microbial diversity in the jejunal tract of laying hens but also enriched beneficial bacterial species, which could contribute to enhanced gut health and improved gut barrier function during the late laying period.
4 Discussion
In poultry, digestion and absorption primarily occur in the jejunum, a process influenced by the intestinal morphology and enzyme activity (21). In this study, we assessed the effects of composite yeast culture (CYC) as a feed additive on growth and intestinal health-related parameters in late-stage laying Hy-Line Brown hens, including growth performance, intestinal barrier function, cytokine levels, and gut microbial structure. Our findings demonstrate that the feed additive CYC can serve as a suitable alternative to antibiotics, reducing intestinal-related inflammation in late laying hens.
Due to the intense metabolic activity experienced by laying hens during peak egg production, the metabolic level gradually declines in hens during the later stages of egg-laying, leading to a decrease in egg production capacity (22). This study found that hens fed CYC exhibited a significant increase in egg production rate starting from day 11 (p < 0.05). This result may be associated with the presence of β-glucans and mannans in CYC, which can enhance intestinal morphology and barrier function in poultry, thereby promoting digestion and absorption (23). Furthermore, probiotics may compete with harmful bacteria for adhesion sites and nutrients on the intestinal mucosa (24, 25), potentially inhibiting the growth of pathogenic bacteria, protecting gut health, and consequently improving protein utilization and egg production rates. Previous studies have confirmed the positive effects of various yeast strains on improving poultry production performance; for example, yeast cultures can significantly enhance average daily weight gain and feed efficiency in broilers (26), and the addition of β-glucans to feed can improve egg production rates in heat-stressed hens (19). In earlier research, incorporating yeast cultures into feed significantly increased average daily feed intake and reduced feed-to-weight ratio in sheep (18). In this experiment, no significant differences were observed in average daily feed intake and feed-to-egg ratio between the two groups, suggesting that the appetite-stimulating effects of yeast cultures may vary among different animal species.
The jejunum is a crucial organ for digestion and nutrient absorption in poultry, where its structural and functional integrity is essential not only for nutrient assimilation but also for reducing the invasion of external pathogenic microorganisms (27, 28). The villus height to crypt depth ratio (VH/CD) is a key indicator that reflects the digestive and absorptive capacity of the small intestine; a higher ratio indicates a more effective intestinal barrier function (29, 30). Research has shown that feeding yeast and other probiotics significantly increases the jejunal villus height in late laying hens (31). In this study, CYC was found to enhance jejunal villus height (p < 0.05), which supports improved digestive and absorptive capabilities, thereby promoting animal health. Furthermore, the CYC group exhibited a significant increase in the VH/CD ratio, consistent with previous findings.
The intestinal barrier is primarily composed of intestinal epithelial cells and tight junction complexes, serving as the structural basis for maintaining epithelial permeability and barrier function. An intact intestinal barrier is essential not only for nutrient absorption but also for reducing the invasion of external pathogenic microorganisms (32, 33). The tight junctions between intestinal epithelial cells mainly consist of claudin-1, occludin, ZO-1, and junctional adhesion molecules (JAM). Claudin-1 and occludin are key proteins in the transmembrane protein family, with claudin-1 playing a critical role in stabilizing intestinal permeability (34), while occludin primarily maintains tight junction stability (35). ZO-1 supports and maintains tight junction structure, participating in the linkage of transmembrane proteins, substance transport, and signal transduction (36). In our study, CYC was shown to enhance the mRNA expression of tight junction proteins occludin, claudin-1, ZO-1, and Muc2 in late laying hens (p < 0.05), thereby strengthening intestinal barrier function. Muc2 has been found to assist probiotics in colonizing the gut (37). Furthermore, yeast culture has significantly increased the expression of Muc2 in broiler ileum (38), and microbial preparations from yeast cell walls have raised the expression of tight junction protein genes in broilers (39). The combined application of β-glucans and mannans has been shown to improve gut barrier function in broilers (23), and these findings align with our results. This phenomenon further suggests that CYC’s enhancement of egg production may be related to the ability of β-glucans and mannans to improve intestinal barrier function in late laying hens, facilitating digestion and absorption, and consequently enhancing protein utilization.
Poultry lack lymph nodes and instead rely on dispersed lymphoid tissues, making the gut a crucial organ for immune function (40). Intestinal immune regulation primarily depends on cytokines secreted by immune cells. Based on this, we measured changes in the mRNA expression of pro-inflammatory cytokines IL-1β, TNF-α, and IFN-γ, as well as anti-inflammatory cytokines IL-4, IL-10, and TGF-β1, aiming to reflect the health status of the hens through these expression changes. Our study revealed that after CYC supplementation, late laying hens showed reduced levels of the pro-inflammatory cytokines TNF-α and IFN-γ (p < 0.05), while the levels of anti-inflammatory cytokines IL-4, IL-10, and TGF-β1 increased (p < 0.05). That elevated expression of pro-inflammatory cytokines IL-1β, TNF-α, and IFN-γ can inhibit the expression of tight junction proteins occludin and claudin-1, which leads to increased intestinal permeability and damage to barrier function (41). Therefore, in this study, the promotion of occludin and claudin-1 expression by CYC may be related to the reduced expression of IL-1β, TNF-α, and IFN-γ. Pro-inflammatory cytokine IFN-γ and anti-inflammatory cytokines IL-4 and IL-10 are secreted by Th1 and Th2 cells, respectively, and play significant roles in the regulation of intestinal tight junctions and the maintenance of epithelial barrier integrity (42). These cytokines are essential components of cellular immunity (43). TGF-β1 can inhibit inflammation in the gut by directly acting on immune cells, thereby modulating intestinal immune function (44). Additionally, the anti-inflammatory cytokine IL-10 can enhance immune function by reducing the expression of the pro-inflammatory cytokine IL-1β (45). Therefore, this study suggests that CYC may improve intestinal barrier function in late laying hens by regulating the expression of pro-inflammatory cytokines TNF-α and IFN-γ, as well as anti-inflammatory cytokines IL-4, IL-10, and TGF-β1. Furthermore, our research group has confirmed at the cellular gene and protein levels that β-glucans and mannans can activate the TLR4-mediated NF-κB/MAPK signaling pathway, leading to a reduction in the expression of pro-inflammatory cytokines IL-1β and TNF-α and an increase in anti-inflammatory cytokine IL-10 expression, thereby modulating cellular inflammatory responses. In summary, this study demonstrates that CYC supplementation effectively alleviates intestinal inflammation and enhances immune function in late laying hens.
Maintaining the microbial ecological balance in the gastrointestinal tract is crucial for overall health. Dominant microbial communities play an important role in achieving this balance (46). Numerous studies have demonstrated that gut microbiota can form a microbial barrier, participate in protecting the host’s intestinal defenses and nutrition, and regulate immune function (47, 48). In this study, PCoA and relative abundance results indicated that feeding CYC can adjust the richness and diversity of the microbial community structure in the jejunum. Specifically, at the species level, there was a notable increase in the abundance of Bifidobacterium pseudocatenulatum and Faecalibacterium prausnitzii. It has been reported that B. pseudocatenulatum alleviates DSS-induced colitis in mice by increasing Muc2 mRNA expression, thereby enhancing intestinal barrier function while reducing pro-inflammatory cytokines TNF-α, IL-1β, and IL-6, and increasing the anti-inflammatory cytokine IL-10 (49), indicating the anti-inflammatory and immune-regulatory benefits of B. pseudocatenulatum for the gut. F. prausnitzii is a major producer of butyrate in the intestine (50), and a reduction in F. prausnitzii has been negatively correlated with disease activity (51). Butyrate is reported to be a primary energy source for intestinal epithelial cells (IEC), which are essential for maintaining barrier integrity (52). Therefore, butyrate plays a crucial role in preserving the integrity of the intestinal barrier. Research has shown that butyrate and its derivatives can reduce intestinal inflammation by promoting mucus secretion (53), and effectively enhance gut development (54). In this study, feeding CYC resulted in an increased abundance of B. pseudocatenulatum and F. prausnitzii, which elevated the expression of Muc2 and reduced the levels of pro-inflammatory cytokines IL-1β and TNF-α. These observations may be related to the effects of butyrate. We conducted a Spearman correlation analysis to examine the relationship between changes in gut microbial community structure and intestinal barrier function, as well as cytokines, to validate the reasoning behind our observations. The study found that F. prausnitzii in the intestines of laying hens showed a significant positive correlation with Muc2, occludin, and claudin-1 (p < 0.05), while it exhibited a significant negative correlation with IFN-γ and TNF-α (p < 0.05). Similarly, B. pseudocatenulatum was positively correlated with Muc2, claudin-1, occludin, TGF-β1, IL-10, IL-4, and Zo-1, and negatively correlated with IFN-γ and TNF-α. We hypothesize that B. pseudocatenulatum and F. prausnitzii have a regulatory effect on the mRNA expression of Muc2, claudin-1, occludin, TGF-β1, IL-10, IL-4, and Zo-1, which aligns with the results mentioned above. These findings further suggest that the complex yeast culture (CYC) may promote butyrate production by increasing the relative abundance of B. pseudocatenulatum and F. prausnitzii, thereby improving intestinal barrier function and modulating the expression of associated inflammatory cytokines. However, the underlying mechanisms involved require further investigation.
5 Conclusion
In conclusion, the complex yeast culture (CYC) may enhance the intestinal microbial community structure in late-stage Hy-Line Brown hens, improve intestinal barrier function, and reduce the occurrence of intestinal inflammation. These effects contribute to increased egg production and alleviation of intestinal inflammation. Therefore, CYC can serve as an effective feed additive to strengthen the intestinal structure of laying hens, promoting better nutrient absorption and immune defense during the later stages of egg production. This study provides a theoretical basis for the clinical application of complex yeast cultures in the poultry industry.
Data availability statement
The datasets presented in this study can be found in online repositories. The names of the repository/repositories and accession number(s) can be found in the article/supplementary material.
Ethics statement
The animal studies were approved by Inner Mongolia Agricultural University Animal Protection and Utilization Committee. The studies were conducted in accordance with the local legislation and institutional requirements. Written informed consent was obtained from the owners for the participation of their animals in this study.
Author contributions
QL: Conceptualization, Investigation, Methodology, Validation, Writing – original draft. LY: Project administration, Software, Writing – review & editing. BT: Investigation, Writing – original draft. SL: Investigation, Writing – original draft. JM: Investigation, Writing – original draft. JN: Investigation, Writing – original draft. ZY: Formal analysis, Writing – original draft. XZ: Formal analysis, Writing – original draft. YW: Formal analysis, Writing – original draft. YL: Formal analysis, Writing – original draft. XW: Formal analysis, Writing – original draft. LW: Resources, Writing – original draft. SD: Conceptualization, Writing – original draft, Writing – review & editing. DL: Conceptualization, Funding acquisition, Writing – original draft, Writing – review & editing.
Funding
The author(s) declare that financial support was received for the research, authorship, and/or publication of this article. This research was funded by the Inner Mongolia Autonomous Region Science and Technology Plan Project (2020GG0036; 2022YFDZ0051), Basic Research Business Project of Directly Affiliated Universities in Inner Mongolia Autonomous Region (BR22-11-17), Inner Mongolia First Class Discipline Research Project (YLXKZX-NND-012), and Cooperative Research and Development of Mutton Sheep in China and Mongolia (CHN-2023/68).
Acknowledgments
We thank all the members who participated in this study for their support and the experimental site keepers for their help.
Conflict of interest
LW was employed by Shenyang Fengmei Biotechnology Co., Ltd.
The remaining authors declare that the research was conducted in the absence of any commercial or financial relationships that could be construed as a potential conflict of interest.
Generative AI statement
The authors declare that no Generative AI was used in the creation of this manuscript.
Publisher’s note
All claims expressed in this article are solely those of the authors and do not necessarily represent those of their affiliated organizations, or those of the publisher, the editors and the reviewers. Any product that may be evaluated in this article, or claim that may be made by its manufacturer, is not guaranteed or endorsed by the publisher.
References
1. Bain, MM, Nys, Y, and Dunn, IC. Increasing persistency in lay and stabilising egg quality in longer laying cycles. What are the challenges? Br Poult Sci. (2016) 57:330–8. doi: 10.1080/00071668.2016.1161727
2. Xie, T, Bai, SP, Zhang, KY, Ding, XM, Wang, JP, Zeng, QF, et al. Effects of Lonicera confusa and Astragali Radix extracts supplementation on egg production performance, egg quality, sensory evaluation, and antioxidative parameters of laying hens during the late laying period. Poult Sci. (2019) 98:4838–47. doi: 10.3382/ps/pez219
3. Wang, W, Wang, J, Zhang, H, Wu, S, and Qi, G. Effects of Clostridium butyricum on production performance and intestinal absorption function of laying hens in the late phase of production. Anim Feed Sci Technol. (2020) 264:114476. doi: 10.1016/j.anifeedsci.2020.114476
4. Low, CX, Tan, LT-H, Ab Mutalib, N-S, Pusparajah, P, Goh, B-H, Chan, K-G, et al. Unveiling the impact of antibiotics and alternative methods for animal husbandry: a review. Antibiotics. (2021) 10:578. doi: 10.3390/antibiotics10050578
5. Emami, NK, Calik, A, White, MB, Kimminau, EA, and Dalloul, RA. Effect of probiotics and multi-component feed additives on microbiota, gut barrier and immune responses in broiler chickens during subclinical necrotic enteritis. Front Vet Sci. (2020) 7:572142. doi: 10.3389/fvets.2020.572142
6. Ahmad, R, Yu, Y-H, Hsiao, FS-H, Su, C-H, Liu, H-C, Tobin, I, et al. Influence of heat stress on poultry growth performance, intestinal inflammation, and immune function and potential mitigation by probiotics. Animals. (2022) 12:2297. doi: 10.3390/ani12172297
7. Attia, YA, Basiouni, S, Abdulsalam, NM, Bovera, F, Aboshok, AA, Shehata, AA, et al. Alternative to antibiotic growth promoters: beneficial effects of Saccharomyces cerevisiae and/or Lactobacillus acidophilus supplementation on the growth performance and sustainability of broilers’ production. Front Vet Sci. (2023) 10:1259426. doi: 10.3389/fvets.2023.1259426
8. Kim, E, Kyoung, H, Hyung Koh, N, Lee, H, Lee, S, Kim, Y, et al. Supplementation of live yeast culture modulates intestinal health, immune responses, and microbiota diversity in broiler chickens. J Anim Sci. (2022) 100:skac122. doi: 10.1093/jas/skac122
9. Sun, Z, Wang, T, Demelash, N, Zheng, S, Zhao, W, Chen, X, et al. Effect of yeast culture (Saccharomyces cerevisiae) on broilers: a preliminary study on the effective components of yeast culture. Animals. (2019) 10:68. doi: 10.3390/ani10010068
10. Hoque, M-R, Jung, H-I, and Kim, I-H. Effect of yeast culture (Saccharomyces cerevisiae) supplementation on growth performance, excreta microbes, noxious gas, nutrient utilization, and meat quality of broiler chicken. J Poult Sci. (2021) 58:216–21. doi: 10.2141/jpsa.0190144
11. Bilal, RM, Hassan, FU, Saeed, M, Rafeeq, M, Zahra, N, Fraz, A, et al. Role of yeast and yeast-derived products as feed additives in broiler nutrition. Anim Biotechnol. (2023) 34:392–401. doi: 10.1080/10495398.2021.1942028
12. Yang, C, Zhang, T, Tian, Q, Cheng, Y, Gebeyew, K, Liu, G, et al. Supplementing mannan oligosaccharide reduces the passive transfer of immunoglobulin G and improves antioxidative capacity, immunity, and intestinal microbiota in neonatal goats. Front Microbiol. (2022) 12:795081. doi: 10.3389/fmicb.2021.795081
13. Zhen, W, Shao, Y, Wu, Y, Li, L, Pham, VH, Abbas, W, et al. Dietary yeast β-glucan supplementation improves eggshell color and fertile eggs hatchability as well as enhances immune functions in breeder laying hens. Int J Biol Macromol. (2020) 159:607–21. doi: 10.1016/j.ijbiomac.2020.05.134
14. Maamouri, O, and Ben, SM. Effect of yeast culture feed supply on growth, ruminal pH, and digestibility of fattening calves. Food Sci Nutr. (2021) 9:2762–7. doi: 10.1002/fsn3.2238
15. Sastré-Calderón, N, Gómez-Verduzco, G, Cortés-Cuevas, A, Juárez-Ramírez, M, Arce-Menocal, J, Márquez-Mota, CC, et al. Growth performance and immunity of broilers fed sorghum-soybean meal diets supplemented with phytases and Β-mannanases. Animals. (2024) 14:924. doi: 10.3390/ani14060924
16. Zhou, J, Fu, Y, Qi, G, Dai, J, Zhang, H, Wang, J, et al. Yeast cell-wall polysaccharides improve immunity and attenuate inflammatory response via modulating gut microbiota in LPS-challenged laying hens. Int J Biol Macromol. (2023) 224:407–21. doi: 10.1016/j.ijbiomac.2022.10.133
17. Lin, J, Comi, M, Vera, P, Alessandro, A, Qiu, K, Wang, J, et al. Effects of Saccharomyces cerevisiae hydrolysate on growth performance, immunity function, and intestinal health in broilers. Poult Sci. (2023) 102:102237. doi: 10.1016/j.psj.2022.102237
18. Chen, H, Liu, S, Li, S, Li, D, Li, X, Xu, Z, et al. Effects of yeast culture on growth performance, immune function, antioxidant capacity and hormonal profile in Mongolian ram lambs. Front Vet Sci. (2024) 11:1424073. doi: 10.3389/fvets.2024.1424073
19. Ezzat, W, Mahrose, KM, Rizk, AM, Ouda, MMM, Fathey, IA, Othman, SI, et al. Impact of β-glucan dietary supplementation on productive, reproductive performance and physiological response of laying hens under heat stress conditions. Poult Sci. (2024) 103:103183. doi: 10.1016/j.psj.2023.103183
20. Korcová, J, Machová, E, Filip, J, and Bystrický, S. Biophysical properties of carboxymethyl derivatives of mannan and dextran. Carbohydr Polym. (2015) 134:6–11. doi: 10.1016/j.carbpol.2015.07.008
21. Khan, S, Moore, RJ, Stanley, D, and Chousalkar, KK. The gut microbiota of laying hens and its manipulation with prebiotics and probiotics to enhance gut health and food safety. Appl Environ Microbiol. (2020) 86:e00600–20. doi: 10.1128/AEM.00600-20
22. Silversides, FG, and Scott, TA. Effect of storage and layer age on quality of eggs from two lines of hens. Poult Sci. (2001) 80:1240–5. doi: 10.1093/ps/80.8.1240
23. Teng, P-Y, Adhikari, R, Llamas-Moya, S, and Kim, WK. Effects of combination of mannan-oligosaccharides and β-glucan on growth performance, intestinal morphology, and immune gene expression in broiler chickens. Poult Sci. (2021) 100:101483. doi: 10.1016/j.psj.2021.101483
24. Cazorla, SI, Maldonado-Galdeano, C, Weill, R, De Paula, J, and Perdigón, GDV. Oral Administration of probiotics increases paneth cells and intestinal antimicrobial activity. Front Microbiol. (2018) 9:736. doi: 10.3389/fmicb.2018.00736
25. Tous, N, Marcos, S, Goodarzi Boroojeni, F, Pérez De Rozas, A, Zentek, J, Estonba, A, et al. Novel strategies to improve chicken performance and welfare by unveiling host-microbiota interactions through hologenomics. Front Physiol. (2022) 13:884925. doi: 10.3389/fphys.2022.884925
26. Wang, T, Cheng, K, Yu, CY, Li, QM, Tong, YC, Wang, C, et al. Effects of a yeast-derived product on growth performance, antioxidant capacity, and immune function of broilers. Poult Sci. (2021) 100:101343. doi: 10.1016/j.psj.2021.101343
27. Thanabalan, A, and Kiarie, EG. Body weight, organ development and jejunal histomorphology in broiler breeder pullets fed n-3 fatty acids enriched diets from hatch through to 22 weeks of age. Poult Sci. (2022) 101:101514. doi: 10.1016/j.psj.2021.101514
28. Alyileili, SR, El-Tarabily, KA, Belal, IEH, Ibrahim, WH, Sulaiman, M, and Hussein, AS. Intestinal development and histomorphometry of broiler chickens fed Trichoderma reesei degraded date seed diets. Front Vet Sci. (2020) 7:349. doi: 10.3389/fvets.2020.00349
29. Munoz, LR, Bailey, MA, Krehling, JT, Bourassa, DV, Hauck, R, Pacheco, WJ, et al. Effects of dietary yeast cell wall supplementation on growth performance, intestinal Campylobacter jejuni colonization, innate immune response, villus height, crypt depth, and slaughter characteristics of broiler chickens inoculated with Campylobacter jejuni at d 21. Poult Sci. (2023) 102:102609. doi: 10.1016/j.psj.2023.102609
30. Ducatelle, R, Goossens, E, De Meyer, F, Eeckhaut, V, Antonissen, G, Haesebrouck, F, et al. Biomarkers for monitoring intestinal health in poultry: present status and future perspectives. Vet Res. (2018) 49:43. doi: 10.1186/s13567-018-0538-6
31. Soren, S, Mandal, GP, Mondal, S, Pradhan, S, Mukherjee, J, Banerjee, D, et al. Efficacy of Saccharomyces cerevisiae fermentation product and probiotic supplementation on growth performance, gut microflora and immunity of broiler chickens. Animals. (2024) 14:866. doi: 10.3390/ani14060866
32. Horowitz, A, Chanez-Paredes, SD, Haest, X, and Turner, JR. Paracellular permeability and tight junction regulation in gut health and disease. Nat Rev Gastroenterol Hepatol. (2023) 20:417–32. doi: 10.1038/s41575-023-00766-3
33. Chelakkot, C, Ghim, J, and Ryu, SH. Mechanisms regulating intestinal barrier integrity and its pathological implications. Exp Mol Med. (2018) 50:1–9. doi: 10.1038/s12276-018-0126-x
34. Findley, MK, and Koval, M. Regulation and roles for claudin-family tight junction proteins. IUBMB Life. (2009) 61:431–7. doi: 10.1002/iub.175
35. Chen, Y, Merzdorf, C, Paul, DL, and Goodenough, DA. COOH terminus of occludin is required for tight junction barrier function in early Xenopus embryos. J Cell Biol. (1997) 138:891–9. doi: 10.1083/jcb.138.4.891
36. Itoh, M, Furuse, M, Morita, K, Kubota, K, Saitou, M, and Tsukita, S. Direct binding of three tight junction-associated maguks, Zo-1, Zo-2, and Zo-3, with the COOH termini of claudins. J Cell Biol. (1999) 147:1351–63. doi: 10.1083/jcb.147.6.1351
37. Liu, Y, Yu, Z, Zhu, L, Ma, S, Luo, Y, Liang, H, et al. Orchestration of MUC2—the key regulatory target of gut barrier and homeostasis: a review. Int J Biol Macromol. (2023) 236:123862. doi: 10.1016/j.ijbiomac.2023.123862
38. Zhen, W, Zhu, T, Wang, P, Guo, F, Zhang, K, Zhang, T, et al. Effect of dietary Saccharomyces-derived prebiotic refined functional carbohydrates as antibiotic alternative on growth performance and intestinal health of broiler chickens reared in a commercial farm. Poult Sci. (2023) 102:102671. doi: 10.1016/j.psj.2023.102671
39. Kyoung, H, Kim, E, Cho, JH, Lee, H, Kim, Y, Park, KI, et al. Dietary yeast cell wall enhanced intestinal health of broiler chickens by modulating intestinal integrity, immune responses, and microbiota. Poult Sci. (2023) 102:102660. doi: 10.1016/j.psj.2023.102660
40. Casteleyn, C, Doom, M, Lambrechts, E, Van den Broeck, W, Simoens, P, and Cornillie, P. Locations of gut-associated lymphoid tissue in the 3-month-old chicken: a review. Avian Pathol. (2010) 39:143–50. doi: 10.1080/03079451003786105
41. Biasi, F, Leonarduzzi, G, Oteiza, PI, and Poli, G. Inflammatory bowel disease: mechanisms, redox considerations, and therapeutic targets. Antioxid Redox Signal. (2013) 19:1711–47. doi: 10.1089/ars.2012.4530
42. Akdis, M, Aab, A, Altunbulakli, C, Azkur, K, Costa, RA, Crameri, R, et al. Interleukins (from IL-1 to IL-38), interferons, transforming growth factor β, and TNF-α: receptors, functions, and roles in diseases. J Allergy Clin Immunol. (2016) 138:984–1010. doi: 10.1016/j.jaci.2016.06.033
43. Guo, W, Xu, L, Guo, X, Wang, W, Hao, Q, Wang, S, et al. The impacts of fermented feed on laying performance, egg quality, immune function, intestinal morphology and microbiota of laying hens in the late laying cycle. Animal. (2022) 16:100676. doi: 10.1016/j.animal.2022.100676
44. Lodyga, M, and Hinz, B. TGF-β1—a truly transforming growth factor in fibrosis and immunity. Semin Cell Dev Biol. (2020) 101:123–39. doi: 10.1016/j.semcdb.2019.12.010
45. Saraiva, M, and O’Garra, A. The regulation of IL-10 production by immune cells. Nat Rev Immunol. (2010) 10:170–81. doi: 10.1038/nri2711
46. Dai, D, Qi, G, Wang, J, Zhang, H, Qiu, K, and Wu, S. Intestinal microbiota of layer hens and its association with egg quality and safety. Poult Sci. (2022) 101:102008. doi: 10.1016/j.psj.2022.102008
47. Abd El-Hack, ME, MohamedT, E-S, Alqhtani, AH, Swelum, AA, Salem, HM, AhmedR, E, et al. The relationship among avian influenza, gut microbiota and chicken immunity: an updated overview. Poult Sci. (2022) 101:102021. doi: 10.1016/j.psj.2022.102021
48. Gao, W, An, K, Li, P, Li, L, and Xia, Z. Dietary Saccharomyces cerevisiae improves intestinal flora structure and barrier function of Pekin ducks. Poult Sci. (2023) 102:101940. doi: 10.1016/j.psj.2022.101940
49. Wang, H, Zhang, X, Kou, X, Zhai, Z, and Hao, Y. A ropy exopolysaccharide-producing strain Bifidobacterium pseudocatenulatum Bi-OTA128 alleviates dextran sulfate sodium-induced colitis in mice. Nutrients. (2023) 15:4993. doi: 10.3390/nu15234993
50. Zhang, M, Zhou, L, Wang, Y, Dorfman, RG, Tang, D, Xu, L, et al. Faecalibacterium prausnitzii produces butyrate to decrease c-Myc-related metabolism and Th17 differentiation by inhibiting histone deacetylase 3. Int Immunol. (2019) 31:499–514. doi: 10.1093/intimm/dxz022
51. Martín, R, Rios-Covian, D, Huillet, E, Auger, S, Khazaal, S, Bermúdez-Humarán, LG, et al. Faecalibacterium: a bacterial genus with promising human health applications. FEMS Microbiol Rev. (2023) 47:fuad039. doi: 10.1093/femsre/fuad039
52. Melaku, M, Zhong, R, Han, H, Wan, F, Yi, B, and Zhang, H. Butyric and citric acids and their salts in poultry nutrition: effects on gut health and intestinal microbiota. IJMS. (2021) 22:10392. doi: 10.3390/ijms221910392
53. Bedford, A, and Gong, J. Implications of butyrate and its derivatives for gut health and animal production. Anim Nutr. (2018) 4:151–9. doi: 10.1016/j.aninu.2017.08.010
Keywords: composite yeast culture, production performance, intestinal barrier, cytokines, intestinal microbiota
Citation: Luo Q, Yang L, Tumenjargal B, Liu S, Ma J, Ning J, Yun Z, Zhang X, Wu Y, Lu Y, Wu X, Wang L, Demberel S and Liu D (2024) Effect of composite yeast culture on the jejunal barrier function, inflammatory response, and microbial community structure of laying hens during the late stage of egg production. Front. Vet. Sci. 11:1524726. doi: 10.3389/fvets.2024.1524726
Edited by:
Yun Ji, China Agricultural University, ChinaReviewed by:
Zhigang Zhang, Northeast Agricultural University, ChinaBaoqing Guo, Iowa State University, United States
Copyright © 2024 Luo, Yang, Tumenjargal, Liu, Ma, Ning, Yun, Zhang, Wu, Lu, Wu, Wang, Demberel and Liu. This is an open-access article distributed under the terms of the Creative Commons Attribution License (CC BY). The use, distribution or reproduction in other forums is permitted, provided the original author(s) and the copyright owner(s) are credited and that the original publication in this journal is cited, in accordance with accepted academic practice. No use, distribution or reproduction is permitted which does not comply with these terms.
*Correspondence: Shirchin Demberel, c2guZGVtYmVyZWxAZ21haWwuY29t; Dacheng Liu, bm1nbGRjQDE2My5jb20=
†These authors have contributed equally to this work