- 1College of Veterinary Medicine, Huazhong Agricultural University, Wuhan, China
- 2Veterinary Biological Technology Innovation Center of Hebei Province, College of Veterinary Medicine, Hebei Agricultural University, Baoding, China
- 3College of Veterinary Medicine, Hebei Agricultural University, Baoding, China
Metabolomics is a science that takes small molecular metabolites in organisms as the research object and determines the dynamic changes of metabolites at the overall level through a variety of modern analytical techniques. At present, metabolomics technology has been widely used in biological significance interpretation, food safety and quality, breeding, disease diagnosis, functional compound identification, and other fields. Its application in poultry science has also become the focus of widespread attention. With the sustainable development of analytical techniques, metabolomics has great potential in the application of poultry science. In this paper, the research progress of metabolomics in poultry growth and development, genetics and breeding, egg quality, meat quality, and disease is reviewed and concluded, which is expected to provide scientific ideas for the research of metabolomics in poultry.
1 Introduction
Poultry meat and eggs are welcomed by consumers because of their rich nutritional value, low price, low fat, and cholesterol content (1). Therefore, with an increasing demand for poultry products, there is more and more research on the production technologies of healthy and high-quality poultry products.
Metabolomics is a branch of omics developed in the mid-1990s, and the concept comes from the metabolome. Metabolomics refers to qualitatively and quantitatively analyzing small molecular metabolites (≤1,000 Da) in cells, tissue, organ, or organism, which reflect the metabolic pathway of endogenous metabolites affected by internal and external factors (2). With the characteristics of high throughput and high sensitivity, metabolomics is developed after genomics and proteomics and is called the peak of the trilogy of genomics (3). According to the analysis scope, metabolomics can be divided into two types: non-targeted metabolomics and targeted metabolomics. Non-targeted metabolomics aims at all endogenous metabolites in the organism, while targeted metabolomics aims at several target compounds (4, 5). Targeted metabolomics has better sensitivity and specificity than non-targeted metabolomics, so targeted metabolomics can verify and expand the results of non-targeted metabolomics analysis. At present, the commonly used analytical techniques include nuclear magnetic resonance (NMR), gas chromatography coupled to mass spectrometry (GC-MS), and liquid chromatography coupled with mass spectrometry (LC-MS). The comparison between the three analytical techniques showed that the sensitivity of NMR is lower than that of LC-MS, but its repeatability is very high. GC-MS has preferably been applied to analyze volatile metabolites such as fatty acids with sample derivatization pretreatment. With high sensitivity, high throughput and wide range, LC-MS analytical platform is becoming increasingly popular in the field of metabolomics. Researchers usually choose different analytical techniques according to the experimental needs. The basic technical route of poultry metabolomics is shown below (Figure 1).
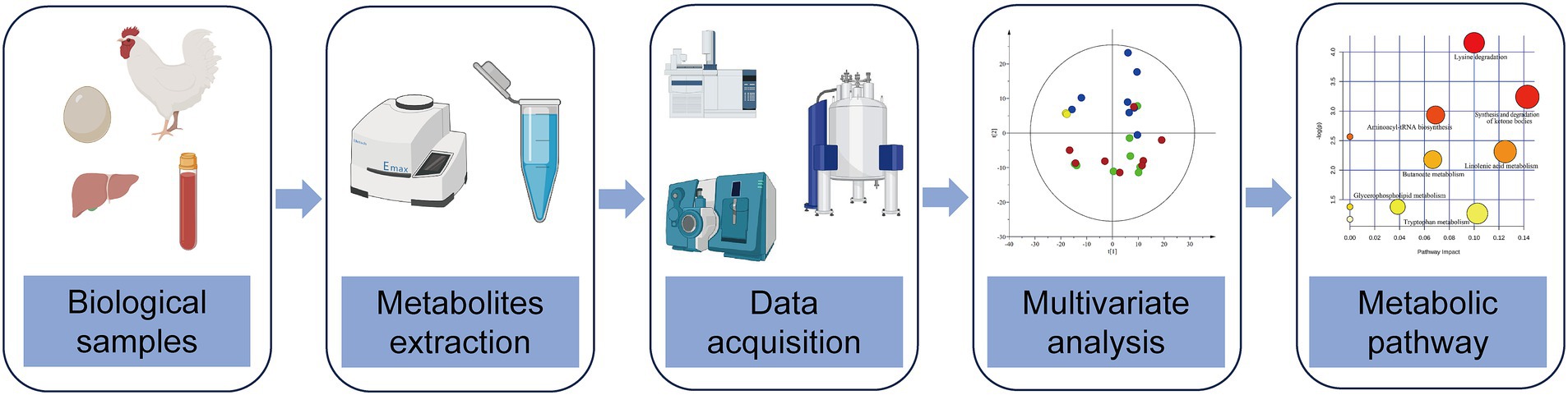
Figure 1. Schematic diagram of the technical route of poultry metabolomics, including biological samples selection, metabolites extraction, data acquisition, multivariate analysis and metabolic pathway.
Metabolomics is widely used in various fields, such as disease diagnosis and mechanism (6–8), drug development (9, 10), nutrition science (11, 12), microbial research (13, 14) and food science. With the continuous development of metabolomics, it has been well applied in physiology, pathology, nutrition and food safety in poultry science. Metabolomics analysis of poultry serum, muscle, kidney, liver, and egg products can reflect health status, meat quality, egg quality, pathological mechanism, and so on (Table 1). This will be beneficial to the work of farmers or the researchers, and provide a strong guarantee for health. Xiao et al. performed the 1H-NMR method to examine the metabolic composition of Wuding chicken of different ages, so as to evaluate the quality of chicken meat (15). In order to quickly and sensitively identify whether the meat product was mixed with other kinds of meat, some researchers used high-resolution MS for experimental analysis (16). In summary, the application of metabolomics technology in poultry has great potential.
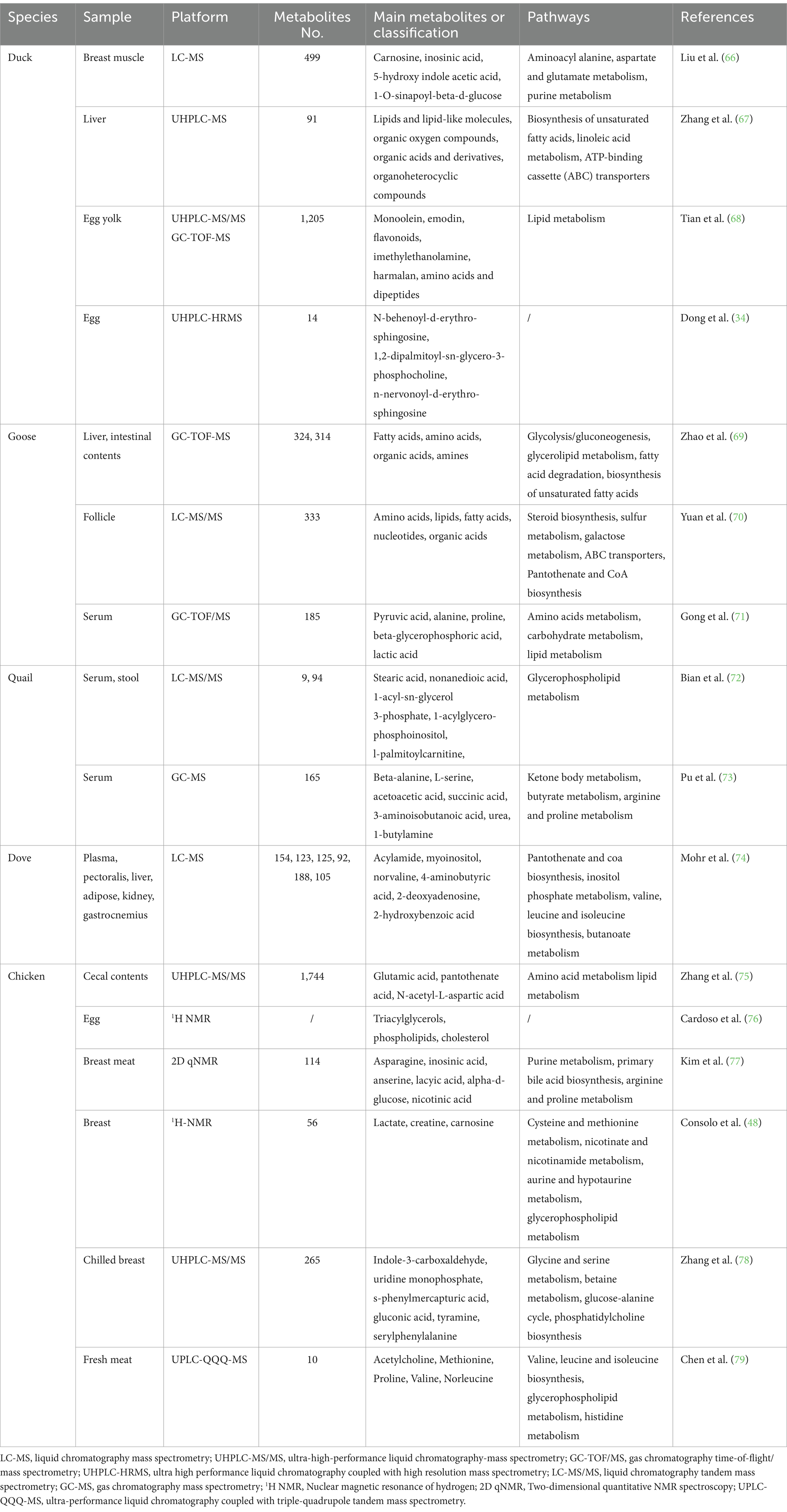
Table 1. Application of different metabolomics platforms to different samples from different poultry species.
The following chapters will mainly introduce the application of metabolomics in poultry growth and development, genetics and breeding, disease prevention and control, egg quality, and meat quality. This review is focused on the cutting-edge research of metabolomics in the field of poultry science in recent years, which will help to provide a reference for the further development of poultry science.
2 Metabolomics in the growth and development of poultry
Through the detection of metabolites in serum, egg yolk, liver, and other tissues, some small molecular metabolites can be screened and selected as biomarkers for different breeds and developmental stages of poultry. It not only provides a theoretical basis for the different phenotypic traits of poultry across different breeds and at different growth stages, but also offers new insights into the changes in metabolites at these growth stages. The changes of tissue metabolites and metabolic pathways at different growth stages are shown in Figure 2.
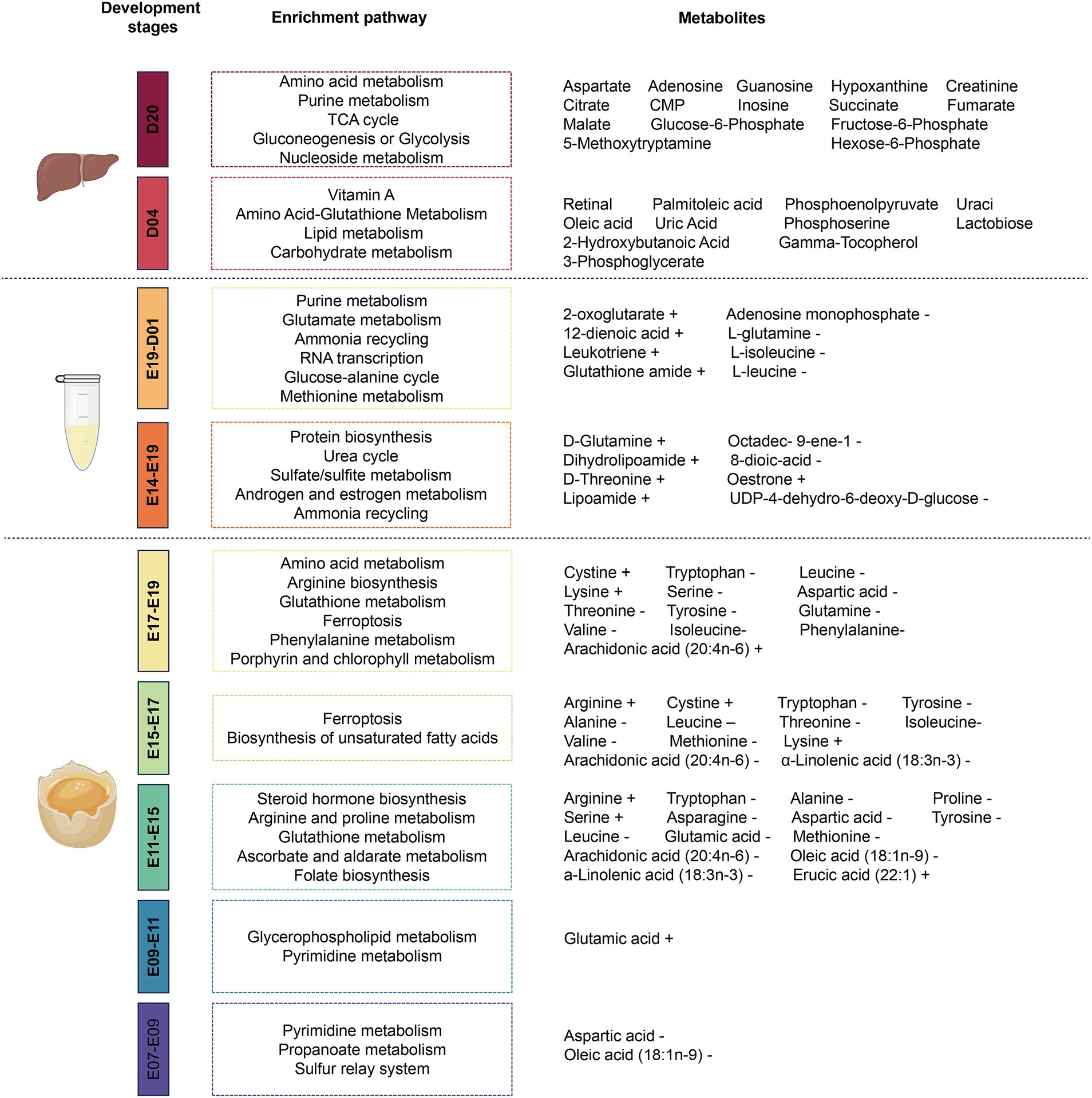
Figure 2. Metabolites changing at different developmental stages in poultry. The changes of egg yolk in the five stages of E07-E09, E09-E11, E11-E15, E15-E17, and E17-E19, serum in the two stages of E14-E19 and E19-D01, and liver metabolites and metabolic pathways in the two stages of D04 and D20. E07 indicates the seventh day of unhatched and D01 indicates the first day after hatching.
The hatching egg is a complex structure, and the growing breeding chicken embryo spends more than 30% of its life cycle in it. As the first diet of the early embryo, the nutrients in the hatching egg play a crucial role in the growth and development of chicks and the improvement of immune function (17). The yolk part of the hatching egg structure is considered to be the main nutrient provider throughout the development of the embryo.
Liu et al. used UHPLC-MS/MS metabolomics to study the changes of yolk metabolites on the 7th, 9th, 11th, 15th, 17th, and 19th day of the embryo. It was found that the yolk metabolites were changed when compared with other days of the embryo, and most of the metabolites were annotated in the amino acid metabolic pathway on the 11th, 15th, 17th, and 19th day of the embryo (18). It can be seen from the above results that the dynamic monitoring of metabolites (nutrient providers) in egg yolk can help understand the nutritional requirements of embryos at different developmental stages. Therefore, metabolomics technology can provide precise nutrition with benefits to promote healthier and stronger chicks during hatching.
It is worth noting that the lipid nutrients in egg yolk are mainly stored in the liver in the form of cholesterol esters after being ingested and absorbed by the embryo (19). The accumulation of lipids accounts for 10% of the mass of the liver until 3 days before breaking the shell (20). After hatching, the chicks use the remaining egg yolk and stored lipids as nutrients for their growth. However, after absorption and consumption on the fifth day after birth, the fat mass in the liver was only one-tenth of the initial storage (21). And the metabolic burden on the liver and how to quickly and effectively maintain a stable energy balance are the main problems faced by the chicks after birth. With the application of high-throughput metabolomics and combined with transcriptome, a study was performed to monitor the liver metabolic network under natural conditions on day 4 (D4) and day 20 (D20) after hatching (22). It was observed that the liver of D4 had a feature of hypoxia, and the TCA cycle was inhibited as compared with that of D20. These results might be due to the mismatch between the rapid growth of liver cells and the delayed formation of blood vessels. Meanwhile, in the liver on D4 from the chicken, pyruvate metabolism and 3-phosphoglycerate metabolism became important metabolic forks under hypoxia conditions, and the polyamine synthesis pathway was enriched and promoted the increase of urea level. During the anaerobic phase of D20, the metabolic pattern in the liver shifted from protecting on its own growth mode to supporting metabolic mode in other tissues, and the enrichment of proline and lysine metabolites suggested the increase of collagen generation and crosslinking in the liver at this stage. At the same time, the anaerobic effect of carbohydrate metabolism was reduced, glucose storage and fatty acid synthesis were increased, and the liver was gradually transformed into a mature liver. The comparison between the two time points from the metabolomic results provided insights into the regulation of metabolites in the liver during the transition from an anoxic to oxic environment in the chicken.
Peng et al. used LC/MS-QTOF based metabolomics to determine the changes of main metabolites in the serum on the 14th and 19th day of embryo incubation and 1 day after hatching, and analyze the related pathways of the differential metabolites during chicken embryo development (23). It was found that 39 metabolites changed from the 14th to 19th day of embryo incubation, and 68 metabolites changed significantly from the 19th day of embryo incubation to the first day after hatching. Among them, the changes in serum glutamine, threonine, and oestrone contents could be used as candidate indicators for the evaluation of early embryonic development because they contributed to promoting the growth and development of chicken embryos from 14 to 19 days old. Additionally, the metabolomic results revealed that L-glutamine, L-isoleucine, and L-leucine could be used as early feed additives for broilers to meet their early growth needs. The above metabolomic results provided insights into the role of serum metabolites in different stages of chicken embryo development. Therefore, metabolomics can be used to screen differential metabolites in the process of growth and development in poultry, which can be treated as biomarkers, indicators or feed additives to explain the mechanism of growth and development and meet the needs of their growth and development.
In the above study, both UHPLC-MS/MS and GC-TOF MS technologies were employed. UHPLC-MS/MS is suitable for detecting a wide range of metabolites, including amino acids and fatty acids, offering highly accurate quantitative analysis. GC-TOF MS is particularly suited for primary metabolites like sugars and short-chain fatty acids, making it ideal for high-throughput analysis. Moreover, Peng et al. employed quality control samples to enhance the reliability of data analysis, thus reducing data fluctuations caused by experimental errors, which is essential for the analysis of high-throughput samples. Therefore, selecting the appropriate technology based on the specific type of target metabolites and experimental requirements is vital for studying the changes in metabolomics during poultry growth and development.
3 Metabolomics in poultry product quality
Eggs are highly nutritious, containing essential amino acids, macroelements, microelements, vitamins, etc., and are considered indispensable food for humans (1, 24). Metabolomics technology offers a powerful means of identifying differential metabolites related to egg quality, thus providing insights into enhancing egg quality.
Previous studies have shown that the yolk is rich in free fatty acids and different types of lipids (25, 26). The lipids can significantly enrich the neuronal and subcellular structure of the hippocampus, which plays an important role in brain development and cognition (27). But the extreme claims about dietary yolks increasing cholesterol risk have gradually overshadowed the physiological value of lipids (28, 29). In order to change this bias, Paul et al. used high-resolution MS to characterize the complex structure of lipids in order to clarify the potential nutrients of lipids in egg yolk (30). The results showed that egg yolk is rich in phospholipids, ceramides and triglycerides, and structural lipids have ω-3 and ω-6 fatty acids, which play an important role as essential precursors of endogenous anti-inflammatory lipid mediators. Interestingly, the yolk structure glycerophospholipids could reduce the absorption of dietary cholesterol in the view of cholesterol risk (31–33). In fact, the nutrient composition in the yolk changes dynamically with the continuous metabolism of the yolk sac, which becomes an important factor affecting the embryo development of broilers. Dong et al. employed a non-targeted metabolomics approach utilizing ultra high-performance liquid chromatography coupled with high resolution mass spectrometry (UHPLC-HRMS) to profile the metabolites of sea duck and caged duck eggs (34). It was observed that the levels of n-behenoyl-d-erythro-sphingosine, 1,2-dipalmitoyl-sn-glycero-3-phosphocholine, and n-nervonoyl-d-erythro-sphingosine in sea duck eggs were significantly higher than those in the caged duck eggs. These findings provided robust evidence for the identification of sea duck eggs and effective means to combat fraud in the poultry and egg industry. Goto et al. utilized metabolomics technology to explore the effects of hen breed and feed conditions on egg composition (35). The researchers analyzed 138 yolk metabolites and 132 albumen metabolites using the GC-MS method. The study revealed that feed conditions significantly altered the levels of three yolk metabolites (erythritol, threitol, and urea) and 12 albumen metabolites (erythritol, threitol, ribitol, linoleic acid, isoleucine, dihydrouracil, 4-hydroxyphenyllactic acid, alanine, glycine, N-butyrylglycine, pyruvic acid, and valine). These findings indicate that genetic and environmental factors play a vital role in the composition of eggs, thereby offering a basis for designing eggs that meet the nutritional needs of consumers in the future. Liu et al. employed LC-MS based metabolomics to analyze the albumen and yolk of long-term stored duck eggs and fresh duck eggs (36). For duck eggs, the long-term storage resulted in the degradation of nutrients such as amino acids, fatty acids, nucleotides, sugars, and vitamins, and the production of harmful substances such as ammonia and biogenic amines, which ultimately compromised the quality of duck eggs. So the consumption of long-term stored duck eggs will have a negative impact on health. In summary, at the level of metabolites, metabolomics technology provides evidence and insights into enhancing the nutritional value, quality and safety of eggs.
Eggshell quality is a crucial factor in poultry egg production, with eggshell rupture accounting for a significant loss of total egg yield, typically around 8–10% (37). The eggshell, which takes approximately 19 h to form, is a highly organized and well-calcified structure that consists of large quantities of calcium, carbonate, proteins, and other chemicals released by the expanding uterus as the egg passes through the isthmus (37–39). Deposited in large quantities and highly organized, these substances constitute the main components of the eggshell. Endogenous metabolites present in the uterine fluid play a vital role in the deposition of calcium carbonate and the subsequent calcification of the eggshell. To investigate whether metabolite differences in the intra-uterine fluid significantly impact eggshell quality, Wang et al. utilized the LC-MS metabolomics approach to identify the metabolites in the uterine fluid of chickens that produced eggs with varying eggshell quality (40). The results revealed that phosphatidylcholine, diacylglycerol, verapamil, risedronate, coproporphyrinogen III, biliverdin phosphatidylcholine, diacylglycerol, verapamil, and biliverdin are essential components involved in eggshell calcification. In addition, it was found that eutectic protein 3 and biliverdin could enhance eggshell strength. Regulating the composition of uterine fluid could potentially improve eggshell quality, and the metabolomics analysis opens up new avenues to increase economic returns in poultry farms.
As a kind of high-quality protein, poultry meat is easy to be absorbed and used by the human body. In recent years, the quality of poultry meat is a research focus. Metabolomics technology can deeply understand the correlation between meat quality and breed, day age and storage time, and then screen and label differential metabolites related to meat quality, which is helpful to improve the speed and accuracy of key metabolite identification and establish a high-quality evaluation system for meat quality.
Shi et al. used LC-MS/MS metabolomics to explore the effect of selective breeding on chicken quality (41). The results showed that glycerol phospholipid metabolism played an important role in chicken flavor and development and affected chicken quality. Li et al. detected the dynamic changes of metabolites in chicken breast meat during different growth and development stages by LC-MS/MS metabolomics (42). In this study, 573 metabolites were identified in five age stages, which directly reflected the nutritional changes of chicken meat and helped to understand the biological process in the development of meat quality. With the application of NMR-based metabolomics, Xiao et al. evaluated the chemical components in the muscle of Wuding chickens at the age of 110, 140, 170, 200, and 230d (15). The total metabolite content in the chicken breast and leg meat was significantly higher in the other four periods compared to 110 days. Moreover, the levels of lactic acid, creatine, inosinic acid, glucose, and other precursors were different in the chest muscle and leg muscle at 230d. The findings were significant to further understand the relationship between chicken metabolism and age, and also could be used to evaluate chicken meat quality.
The flavor and nutritional value of the poultry in the process of storage is also one of the focused issues. Frozen broilers may rot before sale due to the rampant growth of microorganisms and the erosion of spoilage bacteria. The rotten frozen chicken will devour a large amount of water and nutrients and produce excess harmful metabolites. In order to characterize the microbial species and metabolite profile of frozen chicken, Zhang et al. used 16S rRNA gene sequencing and UHPLC-MS/MS non-targeted metabonomic techniques to monitor and observe frozen chicken samples (43). The results showed that Acinetobacter, Serratia, Curella, Shivella, and Obesobacter were the main bacteria causing spoilage of frozen chicken, and 10 metabolic pathways such as histidine metabolism and purine metabolism were the potential pathways to lead to spoilage of frozen chicken. This study was conducive to understanding the changes of metabolites in frozen chickens during storage and provided a basis for the development of new methods for detecting the freshness of frozen chickens. In addition to the traditional metabonomic techniques, Zhou et al. developed a new metabolomic method with the employment of laser ablation electrospray ionization mass spectrometry (LAESI-MS), for direct analysis and identification of meat samples without sample pretreatment (44). Combined with the use of principal component analysis and partial least squares discriminant analysis, the metabolomics technique based on LAESI-MS was proved to be a rapid and accurate method for the distinction of meat samples including chicken, duck, pork, beef, and mutton.
Metabolomics, with its high sensitivity, high throughput, and robustness, has emerged as a powerful tool for both the identification and quality assessment of poultry products such as poultry meat and egg. Screening and labeling of differential metabolites related to meat and egg quality requires statistical analysis of metabolomics data. Multivariate analysis (PCA, PLS-DA, or OPLS-DA) was used in all of the above studies to identify significant metabolites and to analyze between-group differences, and VIP values and p-values from t-tests were used to screen for significant metabolites. The focus on data handling varies from study to study. For instance, Goto et al. analyzed the data by using a two-way mixed-design analysis of variance (ANOVA), and the raw data in the other two articles were transformed by ProteoWizard and the XCMS project was employed for peak alignment, retention time correction as well as peak area extraction. With continuous advances in metabolomics technology, it is crucial to explore simpler and more effective data processing methods to investigate the molecular mechanisms behind egg quality and poultry meat characteristics.
4 Metabolomics in poultry breeding
Poultry with excellent breeds and strong production performance is welcomed by more farms. Genetics and breeding are of great significance in increasing production level and efficiency. In order to improve the selection efficiency and speed up the genetic process, molecular genetic markers can be used to assist poultry breed selection.
Liu et al. used LC-MS to analyze the metabolites of Chahua Chicken No. 2 (CH) and Yao Chicken (Y) (45). The results showed that CH and Y had 85 different metabolites involved in the oxidation of amino acids and fatty acids, which could provide the basis for the molecular breeding of chicken quality traits in the future. Ji et al. used LC-MS based metabolomics to detect the metabolite profile in the adipose tissue of lean Leghorn and Fayoumi chickens, and compared the results to the commercial broiler chickens to explore the pathways associated with heritable leanness in chickens (46). Metabolomic results found that the lipolysis and fatty acid oxidation in white adipose were up-regulated in the lean chickens, indicating the essential roles of these pathways in heritable differences in fatness.
Both studies utilized metabolomics and gene expression profiling, but focused on different aspects. Liu et al. provided a scientific foundation for genetic improvement and breeding of local chicken breeds, emphasizing the potential of the identified genes for improving meat quality and fat deposition in poultry. Ji et al. offered insight into the mechanisms underlying leanness in chickens, suggesting potential pathways for future research on obesity and adipose metabolism in humans. The study also highlights chickens as a unique model for studying human obesity and metabolic disorders.
5 Metabolomics in poultry disease
Poultry disease is the most disturbing thing in the process of poultry breeding. Once the disease breaks out, it will affect a large number of birds and bring huge economic losses to the farmers. There are still some problems such as difficulty and high cost in the prevention and treatment of poultry diseases. Metabolomics has been proven to have great potential in predicting the incidence, severity, and progression of the disease (47–49). With the development of an analysis platform, metabolomics technology can be used to screen differential metabolites as markers for the early detection and evaluation of diseases (Figure 3).
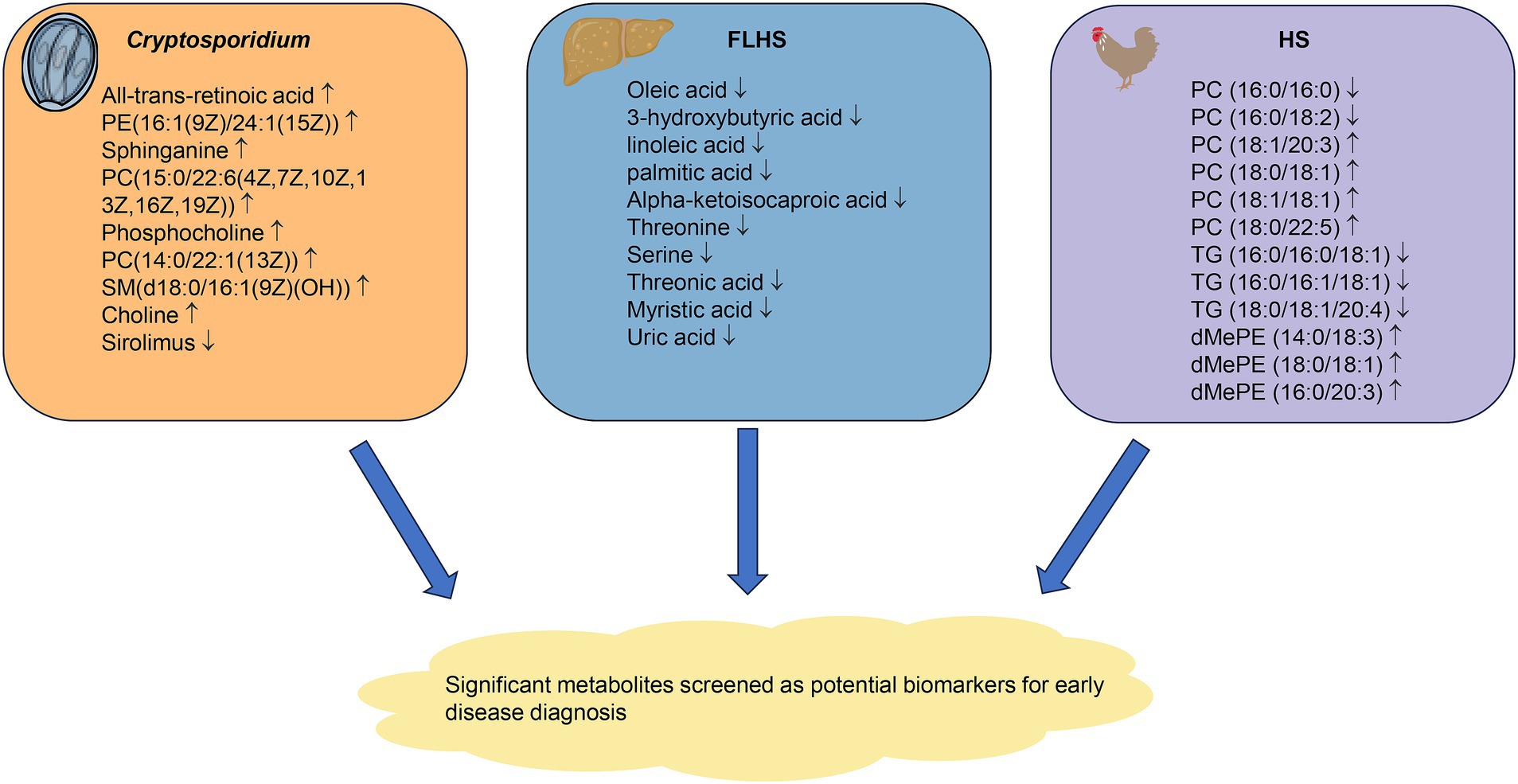
Figure 3. Metabolomics technology screens for significantly different metabolites that can be used as biomarkers for early diagnosis of disease. The changes of metabolites of cryptosporidiosis, FLHS and HS are shown in the figure. FLHS, fatty liver hemorrhage syndrome; HS, heat stress.
As one of the most harmful zoonotic parasitic diseases, Cryptosporidium was first discovered in the early 20th century. It can cause gastroenteritis symptoms in a variety of vertebrates. In poultry and birds, some Cryptosporidium subtypes induce obvious respiratory symptoms such as cough, runny nose and dyspnea, and even cause death in severe cases (50). In addition, the study found that Cryptosporidium infection can lead to the suppression of the host immune system, resulting in increased susceptibility to avian influenza, Newcastle disease, and other diseases (51). Metabolomics have been used to detect the iconic metabolites in infected chickens. Wu et al. used UPLC-MS metabolomic technique and multivariate statistical analysis to study the metabolic characteristics of serum samples of chickens infected with Cryptosporidium baileyi in order to distinguish the potential metabolites between infected and uninfected chickens (52). The results showed metabolomics profiles in the serum were significantly changed after the infection of Cryptosporidium baileyi, and a total of 138 differential serum metabolites enriched in energy, lipid metabolism, and immune-related pathways were detected. Notably, it was found that nine metabolites such as choline, all-trans retinoic acid, phosphocholine and sphinganine could be used as serum diagnostic markers after Cryptosporidium baileyi infection, which provided the information for exploring the invasion mechanism of Cryptosporidium and the pathological changes of the host.
Fatty liver hemorrhage syndrome (FLHS) is a nutritional metabolic disease characterized by lipid metabolism disorders. Its clinical manifestations are fat deposition, rupture, and bleeding in the liver (53, 54). Mostly occurring in caged laying hens, FLHS leads to a decrease in egg production, the shortening of the peak period of egg production, and the increase in mortality, which causes huge economic losses to the layer industry (55). Guo et al. found that metabolomics technology could be applied to the clinical diagnosis of FLHS in laying hens, which provided the potential biomarkers for further understanding the development of FLHS (56). Serum metabolites of the laying hens with FLHS and the healthy control were analyzed by GC-TOF-MS at two-time points (40 and 80 days). Forty different metabolites were screened, which were mainly related to lipid metabolism, amino acid metabolism, and energy metabolism disorders. These studies proved that metabolomics is a powerful tool to study and identify the diagnostic biomarkers related to FLHS in laying hens, and provide new insights into its biological mechanism. Meng et al. revealed the potential pathogenesis of FLHS and abnormal arachidonic acid metabolism in laying hens by applying non-targeted and targeted metabolomics based on UPLC-QTOF/MS (57). Through the analysis of the changes of the liver metabolic profile, 42 liver metabolites were found. Pathway analysis of these liver metabolites revealed that the disorder of arachidonic acid metabolism had an important role in the pathogenesis of FLHS.
For the growing environment, temperature plays an important role in the growth and metabolism of poultry. Heat stress (HS) is an important factor affecting poultry production in tropical and subtropical areas, which mainly causes the decrease in feed intake and growth performance of broilers (58), and leads to pathological changes such as abnormal lipid metabolism and secretion disorder of ketone body components (59, 60). HS can cause fat deposition in broilers, inhibit the proliferation of poultry liver cells, and induce liver cell apoptosis. In order to clarify the metabolomic-level mechanism of the influence of HS, Guo et al. used LC-MS based metabolomics to examine the liver lipid metabolites of Chinese indigenous slow-growing broilers (Huaixiang chickens) (61). It was found that HS could change the content levels of 12 hepatic lipid metabolites mainly including phosphatidylcholine, triglyceride and dimethyl-phosphatidyl ethanolamine. Meanwhile, pathway analysis found that HS altered the pathways of linoleic acid, alpha-linolenic acid, glycerolipid and glycerophospholipid metabolism in the liver of broiler chickens.
It is worth noting that corticosterone (CORT) is one of the stress hormones in multiple types of poultry stress including HS (62). Stressors can trigger the hypothalamic-pituitary-adrenal axis and produce large amounts of CORT in chickens (62, 63). Excessive production of CORT can damage the immune system of chickens and negatively affect the growth and production performance (64). In order to explore the effects of physiological stress brought by CORT on metabolic groups of the liver, kidney, and breast muscle of chickens, a chicken stress model was established by administering CORT (dissolved in ethanol) in drinking water (65). With the application of an untargeted 1H-NMR-based metabolomics approach, it was found that CORT, respectively, altered 11, 46, and 14 metabolites in the liver, kidney and breast muscle, which were linked to amino acid and sugar metabolism. In addition, some metabolites such as dimethylglycine, galactose, and carnosine in breast muscle were regulated by CORT, which might be associated with meat quality. In summary, the identification of metabolites as biomarkers of stress will help to develop and evaluate mitigation strategies to enhance bird health.
The studies mentioned above suggest that metabolomics can identify metabolic differences between diseased and healthy animals. The selection of samples for testing depends on the specific disease being investigated. For example, serum samples are used for diagnosing Cryptosporidium infection, serum and liver metabolites are analyzed for FLHS, and liver lipid metabolites are tested for HS. Disease-specific biomarkers vary, and the metabolome can be influenced by factors such as physiological states, environmental stimuli, and other variables. Thus, when using metabolomics for early disease diagnosis and prevention in poultry, it is critical to select samples that accurately reflect the current physiological state.
6 Conclusion
As an emerging technology, metabolomics is employed to identify and monitor the changes of small-molecule metabolites in organisms caused by exogenous disturbances, which will help to explore the metabolic mechanism. The wide applications of metabolomics technology in poultry disease diagnosis, genetics and breeding, growth and development, egg quality, and meat quality detection indicate this approach is highly promising. However, there are still many problems to be solved in the current metabolomics technology, for example, there are still some limitations in the sensitivity and coverage of some detection instruments, and the metabolites detected may only be a small part of the gene variation. Therefore, the sensitivity, stability, specificity, and broad spectrum of the detection technology still need to be further improved. Moreover, multi-omics technology is the future trend that the integration of genomics, transcriptomics, proteomics and metabolomics can provide comprehensive information at the different levels for poultry research.
As omics technologies continue to evolve, metabolomics is increasingly recognized as a crucial tool in poultry research. The future development of this field will likely be propelled by advancements in metabolite detection instruments, improvements in metabolite databases, and the widespread application of diverse metabolomics analysis strategies. To address the challenges posed by high-dimensional, complex datasets in current metabolomics analyses, it will be essential to establish a more comprehensive and standardized reference database, enabling the effective use of data from many unknown metabolites. Furthermore, future research trends are expected to focus on integrating multiple analytical platforms to enable a more holistic analysis of metabolomics data from various dimensions, offering deeper insights into poultry metabolic mechanisms. At the same time, the rapid development of artificial intelligence technologies will significantly enhance the ability to process metabolomics data, providing innovative approaches for dimensionality reduction and more nuanced interpretations of metabolomics information.
Author contributions
MZ: Writing – original draft, Writing – review & editing. MY: Writing – original draft. NM: Writing – review & editing. JL: Writing – review & editing.
Funding
The author(s) declare that financial support was received for the research, authorship, and/or publication of this article. This review was supported by Science Research Project of Hebei Education Department (BJK2024077) and S&T Program of Hebei (21326617D).
Conflict of interest
The authors declare that the research was conducted in the absence of any commercial or financial relationships that could be construed as a potential conflict of interest.
Publisher’s note
All claims expressed in this article are solely those of the authors and do not necessarily represent those of their affiliated organizations, or those of the publisher, the editors and the reviewers. Any product that may be evaluated in this article, or claim that may be made by its manufacturer, is not guaranteed or endorsed by the publisher.
References
1. Kralik, G, and Kralik, Z. Poultry products enriched with Nutricines have beneficial effects on human health. Med Glas (Zenica). (2017) 14:1–7. doi: 10.17392/879-16
2. Muthubharathi, BC, Gowripriya, T, and Balamurugan, K. Metabolomics: small molecules that matter more. Mol Omics. (2021) 17:210–29. doi: 10.1039/d0mo00176g
3. Patti, GJ, Yanes, O, and Siuzdak, G. Metabolomics: the apogee of the omics trilogy. Nat Rev Mol Cell Biol. (2012) 13:263–9. doi: 10.1038/nrm3314
4. Griffiths, WJ, Koal, T, Wang, YQ, Kohl, M, Enot, DP, and Deigner, HP. Targeted metabolomics for biomarker discovery. Angew Chem-Int Edit. (2010) 49:5426–45. doi: 10.1002/anie.200905579
5. Liu, PF, Li, RT, Antonov, AA, Wang, LH, Li, W, Hua, YF, et al. Discovery of metabolite biomarkers for acute ischemic stroke progression. J Proteome Res. (2017) 16:773–9. doi: 10.1021/acs.jproteome.6b00779
6. Ussher, JR, Elmariah, S, Gerszten, RE, and Dyck, JRB. The emerging role of metabolomics in the diagnosis and prognosis of cardiovascular disease. J Am Coll Cardiol. (2016) 68:2850–70. doi: 10.1016/j.jacc.2016.09.972
7. Perakakis, N, Stefanakis, K, and Mantzoros, CS. The role of omics in the pathophysiology, diagnosis and treatment of non-alcoholic fatty liver disease. Metab Clin Exp. (2020) 111S:24. doi: 10.1016/j.metabol.2020.154320
8. Pereira, PR, Carrageta, DF, Oliveira, PF, Rodrigues, A, Alves, MG, and Monteiro, MP. Metabolomics as a tool for the early diagnosis and prognosis of diabetic kidney disease. Med Res Rev. (2022) 42:1518–44. doi: 10.1002/med.21883
9. Ramana, P, Adams, E, Augustijns, P, and Van Schepdael, A. Metabonomics and drug development. Methods Mol Biol (Clifton, NJ). (2015) 1277:195–207. doi: 10.1007/978-1-4939-2377-9_14
10. Aminov, R . Metabolomics in antimicrobial drug discovery. Expert Opin Drug Discov. (2022) 17:1047–59. doi: 10.1080/17460441.2022.2113774
11. Gibbons, H, O'Gorman, A, and Brennan, L. Metabolomics as a tool in nutritional research. Curr Opin Lipidol. (2015) 26:30–4. doi: 10.1097/mol.0000000000000140
12. LeVatte, M, Keshteli, AH, Zarei, P, and Wishart, DS. Applications of metabolomics to precision nutrition. Lifestyle Genom. (2022) 15:1–9. doi: 10.1159/000518489
13. Mielko, KA, Jablonski, SJ, Milczewska, J, Sands, D, Lukaszewicz, M, and Mlynarz, P. Metabolomic studies of Pseudomonas Aeruginosa. World J Microbiol Biotechnol. (2019) 35:178. doi: 10.1007/s11274-019-2739-1
14. Bauermeister, A, Mannochio-Russo, H, Costa-Lotufo, LV, Jarmusch, AK, and Dorrestein, PC. Mass spectrometry-based metabolomics in microbiome investigations. Nat Rev Microbiol. (2022) 20:143–60. doi: 10.1038/s41579-021-00621-9
15. Xiao, ZC, Ge, CR, Zhou, GH, Zhang, WG, and Liao, GZ. H-1 Nmr-based metabolic characterization of Chinese Wuding chicken meat. Food Chem. (2019) 274:574–82. doi: 10.1016/j.foodchem.2018.09.008
16. Zhang, MY, Li, YY, Zhang, YY, Kang, CD, Zhao, WT, Ren, N, et al. Rapid Lc-Ms/Ms method for the detection of seven animal species in meat products. Food Chem. (2022) 371:11. doi: 10.1016/j.foodchem.2021.131075
17. Cherian, G . Nutrition and metabolism in poultry: role of lipids in early diet. J Anim Sci Biotechnol. (2015) 6:28. doi: 10.1186/s40104-015-0029-9
18. Liu, HC, Ding, P, Tong, YY, He, X, Yin, YL, Zhang, HH, et al. Metabolomic analysis of the egg yolk during the embryonic development of broilers. Poult Sci. (2021) 100:101014. doi: 10.1016/j.psj.2021.01.036
19. Sahan, U, Ipek, A, and Sozcu, A. Yolk sac fatty acid composition, yolk absorption, embryo development, and Chick quality during incubation in eggs from young and old broiler breeders. Poult Sci. (2014) 93:2069–77. doi: 10.3382/ps.2013-03850
20. Feast, M, Noble, RC, Speake, BK, and Ferguson, MWJ. The effect of temporary reductions in incubation temperature on growth characteristics and lipid utilisation in the Chick embryo. J Anat. (1998) 193:383–90. doi: 10.1046/j.1469-7580.1998.19330383.x
21. Noble, RC, and Ogunyemi, D. Lipid changes in the residual yolk and liver of the Chick immediately after hatching. Biol Neonate. (1989) 56:228–36. doi: 10.1159/000243127
22. Van Every, HA, and Schmidt, CJ. Transcriptomic and Metabolomic characterization of post-hatch metabolic reprogramming during hepatic development in the chicken. BMC Genomics. (2021) 22:21. doi: 10.1186/s12864-021-07724-w
23. Peng, ML, Li, SN, He, QQ, Zhao, JL, Li, LL, and Ma, HT. Based serum metabolomics analysis reveals simultaneous interconnecting changes during chicken embryonic development. J Anim Physiol Anim Nutr. (2018) 102:1210–9. doi: 10.1111/jpn.12925
24. Everson, GJ, and Souders, HJ. Composition and nutritive importance of eggs. J Am Diet Assoc. (1957) 33:1244–54. doi: 10.1016/S0002-8223(21)16913-5
25. Jing, M, Zhao, S, and House, JD. Performance and tissue fatty acid profile of broiler chickens and laying hens fed hemp oil and Hempomega (tm). Poult Sci. (2017) 96:1809–19. doi: 10.3382/ps/pew476
26. Westbrook, LA, and Cherian, G. Egg quality, fatty-acid composition and gastrointestinal morphology of layer hens fed whole flaxseed with enzyme supplementation. Br Poult Sci. (2019) 60:146–53. doi: 10.1080/00071668.2018.1556783
27. Chen, XY, Du, YY, Boni, GF, Liu, X, Kuang, JL, and Geng, ZY. Consuming egg yolk decreases body weight and increases serum Hdl and brain expression of Trkb in male Sd rats. J Sci Food Agric. (2019) 99:3879–85. doi: 10.1002/jsfa.9610
28. Feng, ZH, Gong, JG, Zhao, GX, Lin, X, Liu, YC, and Ma, KW. Effects of dietary supplementation of resveratrol on performance, egg quality, yolk cholesterol and antioxidant enzyme activity of laying hens. Br Poult Sci. (2017) 58:544–9. doi: 10.1080/00071668.2017.1349295
29. Puertas, G, and Vazquez, M. Advances in techniques for reducing cholesterol in egg yolk: a review. Crit Rev Food Sci Nutr. (2019) 59:2276–86. doi: 10.1080/10408398.2018.1448357
30. Wood, PL, Muir, W, Christmann, U, Gibbons, P, Hancock, CL, Poole, CM, et al. Lipidomics of the chicken egg yolk: high-resolution mass spectrometric characterization of nutritional lipid families. Poult Sci. (2021) 100:887–99. doi: 10.1016/j.psj.2020.11.020
31. Cohn, JS, Kamili, A, Wat, E, Chung, RWS, and Tandy, S. Dietary phospholipids and intestinal cholesterol absorption. Nutrients. (2010) 2:116–27. doi: 10.3390/nu2020116
32. Kullenberg, D, Taylor, LA, Schneider, M, and Massing, U. Health effects of dietary phospholipids. Lipids Health Dis. (2012) 11:16. doi: 10.1186/1476-511x-11-3
33. Ballesteros, MN, Valenzuela, F, Robles, AE, Artalejo, E, Aguilar, D, Andersen, CJ, et al. One egg per day improves inflammation when compared to an oatmeal-based breakfast without increasing other Cardiometabolic risk factors in diabetic patients. Nutrients. (2015) 7:3449–63. doi: 10.3390/nu7053449
34. Dong, XY, Wang, XJ, Xu, XL, Song, YX, Nie, XM, Jia, W, et al. An untargeted metabolomics approach to identify markers to distinguish duck eggs that come from different poultry breeding systems by ultra high performance liquid chromatography-high resolution mass spectrometry. J Chromatogr B. (2021) 1179:8. doi: 10.1016/j.jchromb.2021.122820
35. Goto, T, Mori, H, Shiota, S, and Tomonaga, S. Metabolomics approach reveals the effects of breed and feed on the composition of chicken eggs. Meta. (2019) 9:12. doi: 10.3390/metabo9100224
36. Liu, HH, Yang, QL, Guo, R, Hu, JW, Tang, Q, Qi, JJ, et al. Metabolomics reveals changes in metabolite composition of duck eggs under the impact of long-term storage. J Sci Food Agric. (2022) 102:4647–56. doi: 10.1002/jsfa.11825
37. Ketta, M, and Tumova, E. Eggshell structure, measurements, and quality-affecting factors in laying hens: a review. Czeh J Anim Sci. (2016) 61:299–309. doi: 10.17221/46/2015-cjas
38. Eastin, WC Jr, and Spaziani, E. On the mechanism of calcium secretion in the avian Shell gland (uterus). Biol Reprod. (1978) 19:505–18. doi: 10.1095/biolreprod19.3.505
39. Nys, Y, Hincke, MT, Arias, JL, Garcia-Ruiz, JM, and Solomon, SE. Avian eggshell mineralization. Poult Avian Biol Rev. (1999) 10:143–66.
40. Wang, XQ, Zhu, P, Sun, ZH, Zhang, JN, and Sun, CJ. Uterine Metabolomic analysis for the regulation of eggshell calcification in chickens. Meta. (2021) 11:16. doi: 10.3390/metabo11090575
41. Shi, K, Zhao, Q, Shao, MH, Duan, Y, Li, DF, Lu, YQ, et al. Untargeted metabolomics reveals the effect of selective breeding on the quality of chicken meat. Meta. (2022) 12:16. doi: 10.3390/metabo12050367
42. Li, JJ, Zhang, DH, Yin, LQ, Li, ZQ, Yu, CL, Du, HR, et al. Integration analysis of metabolome and transcriptome profiles revealed the age-dependent dynamic change in chicken meat. Food Res Int. (2022) 156:111171. doi: 10.1016/j.foodres.2022.111171
43. Zhang, T, Ding, H, Chen, L, Zhang, SS, Wu, PF, Xie, KZ, et al. Characterization of chilled chicken spoilage using an integrated microbiome and metabolomics analysis. Food Res Int. (2021) 144:12. doi: 10.1016/j.foodres.2021.110328
44. Zhou, WZ, Xia, L, Huang, CQ, Yang, JW, Shen, CY, Jiang, HH, et al. Rapid analysis and identification of meat species by laser-ablation electrospray mass spectrometry (Laesi-Ms). Rapid Commun Mass Spectrom. (2016) 30:116–21. doi: 10.1002/rcm.7647
45. Liu, Y, Liang, SM, Wang, K, Zi, XN, Zhang, R, Wang, GZ, et al. Physicochemical, nutritional properties and metabolomics analysis fat deposition mechanism of Chahua chicken no. 2 and Yao chicken. Genes. (2022) 13:22. doi: 10.3390/genes13081358
46. Ji, B, Middleton, JL, Ernest, B, Saxton, AM, Lamont, SJ, Campagna, SR, et al. Molecular and metabolic profiles suggest that increased lipid catabolism in adipose tissue contributes to leanness in domestic chickens. Physiol Genomics. (2014) 46:315–27. doi: 10.1152/physiolgenomics.00163.2013
47. Lu, Z, He, XF, Ma, BB, Zhang, L, Li, JL, Jiang, Y, et al. Serum metabolomics study of nutrient metabolic variations in chronic heat-stressed broilers. Br J Nutr. (2018) 119:771–81. doi: 10.1017/s0007114518000247
48. Consolo, NRB, Samuelsson, LM, Barbosa, L, Monaretto, T, Moraes, TB, Buarque, VLM, et al. Characterization of chicken muscle disorders through metabolomics, pathway analysis, and water Relaxometry: a pilot study. Poult Sci. (2020) 99:6247–57. doi: 10.1016/j.psj.2020.06.066
49. Huang, SC, Zhang, CD, Xu, TT, Shaukat, A, He, YF, Chen, P, et al. Integrated fecal microbiome and metabolomics reveals a novel potential biomarker for predicting Tibial dyschondroplasia in chickens. Front Physiol. (2022) 13:16. doi: 10.3389/fphys.2022.887207
50. Bouzid, M, Hunter, PR, Chalmers, RM, and Tyler, KM. Cryptosporidium pathogenicity and virulence. Clin Microbiol Rev. (2013) 26:115–34. doi: 10.1128/cmr.00076-12
51. Eladl, AH, Hamed, HR, and Khalil, MR. Consequence of cryptosporidiosis on the immune response of vaccinated broiler chickens against Newcastle disease and/or avian influenza. Vet Res Commun. (2014) 38:237–47. doi: 10.1007/s11259-014-9610-5
52. Wu, XM, Yang, X, Fan, XC, Chen, X, Wang, YX, Zhang, LX, et al. Serum metabolomics in chickens infected with Cryptosporidium Baileyi. Parasites Vectors. (2021) 14:12. doi: 10.1186/s13071-021-04834-y
53. Rozenboim, I, Mahato, J, Cohen, NA, and Tirosh, O. Low protein and high-energy diet: a possible natural cause of fatty liver hemorrhagic syndrome in caged white Leghorn laying hens. Poult Sci. (2016) 95:612–21. doi: 10.3382/ps/pev367
54. Peng, G, Huang, EF, Ruan, JM, Huang, LM, Liang, HP, Wei, Q, et al. Effects of a high energy and low protein diet on hepatic and plasma characteristics and Cidea and Cidec Mrna expression in liver and adipose tissue of laying hens with fatty liver hemorrhagic syndrome. Anim Sci J. (2019) 90:247–54. doi: 10.1111/asj.13140
55. Shini, A, Shini, S, and Bryden, WL. Fatty liver Haemorrhagic syndrome occurrence in laying hens: impact of production system. Avian Pathol. (2019) 48:25–34. doi: 10.1080/03079457.2018.1538550
56. Guo, LY, Kuang, J, Zhuang, Y, Jiang, JL, Shi, Y, Huang, C, et al. Serum Metabolomic profiling to reveal potential biomarkers for the diagnosis of fatty liver hemorrhagic syndrome in laying hens. Front Physiol. (2021) 12:13. doi: 10.3389/fphys.2021.590638
57. Meng, JC, Ma, N, Liu, HL, Liu, J, Liu, JX, Wang, JP, et al. Untargeted and targeted metabolomics profiling reveals the underlying pathogenesis and abnormal arachidonic acid metabolism in laying hens with fatty liver hemorrhagic syndrome. Poult Sci. (2021) 100:10. doi: 10.1016/j.psj.2021.101320
58. Abdelhameed, S, and Alexander, A. Nutritional strategies to alleviate heat stress effects through feed restrictions and feed additives (vitamins and minerals) in broilers under summer conditions. J Anim Behav Biometeorol. (2019) 7:123–31. doi: 10.31893/2318-1265jabb.v7n3p123-131
59. Slimen, IB, Najar, T, Ghram, A, and Abdrrabba, M. Heat stress effects on livestock: molecular, cellular and metabolic aspects, a review. J Anim Physiol Anim Nutr. (2016) 100:401–12. doi: 10.1111/jpn.12379
60. Goo, D, Kim, JH, Park, GH, Reyes, JBD, and Kil, DY. Effect of heat stress and stocking density on growth performance, breast meat quality, and intestinal barrier function in broiler chickens. Animals. (2019) 9:10. doi: 10.3390/ani9030107
61. Guo, Y, Liao, JH, Liang, ZL, Balasubramanian, B, and Liu, WC. Hepatic lipid metabolomics in response to heat stress in local broiler chickens breed (Huaixiang chickens). Vet Med Sci. (2021) 7:1369–78. doi: 10.1002/vms3.462
62. Cockrem, JF . Stress, corticosterone responses and avian personalities. J Ornithol. (2007) 148:169–78. doi: 10.1007/s10336-007-0175-8
63. Lattin, CR, Reed, JM, DesRochers, DW, and Romero, LM. Elevated corticosterone in feathers correlates with corticosterone-induced decreased feather quality: a validation study. J Avian Biol. (2011) 42:247–52. doi: 10.1111/j.1600-048X.2010.05310.x
64. Hangalapura, BN, Nieuwland, MGB, Buyse, J, Kemp, B, and Parmentier, HK. Effect of duration of cold stress on plasma adrenal and thyroid hormone levels and immune responses in chicken lines divergently selected for antibody responses. Poult Sci. (2004) 83:1644–9. doi: 10.1093/ps/83.10.1644
65. Brown, CLJ, Zaytsoff, SJM, Montina, T, and Inglis, GD. Corticosterone-mediated physiological stress alters liver, kidney, and breast muscle Metabolomic profiles in chickens. Animals. (2021) 11:17. doi: 10.3390/ani11113056
66. Liu, HH, Wei, B, Tang, Q, Chen, C, Li, YY, Yang, QL, et al. Non-target metabolomics reveals the changes of small molecular substances in duck breast meat under different preservation time. Food Res Int. (2022) 161:11. doi: 10.1016/j.foodres.2022.111859
67. Zhang, Y, Zhang, A, Wang, L, Yang, T, Dong, B, Wang, Z, et al. Metabolomics and proteomics characterizing hepatic reactions to dietary linseed oil in duck. Int J Mol Sci. (2022) 23:15690. doi: 10.3390/ijms232415690
68. Tian, Y, Li, G, Du, X, Zeng, T, Chen, L, Xu, W, et al. Integration of LC-MS-based and GC-MS-based metabolic profiling to reveal the effects of domestication and boiling on the composition of duck egg yolks. Meta. (2023) 13:135. doi: 10.3390/metabo13010135
69. Zhao, MM, Xing, Y, Liu, LD, Fan, X, Liu, L, Geng, TY, et al. Gc-Tof-Ms-based metabolomics analyses of liver and intestinal contents in the overfed vs. normally-fed geese. Animals. (2020) 10:14. doi: 10.3390/ani10122375
70. Yuan, X, Hu, S, Li, L, Liu, H, He, H, and Wang, J. Metabolomic analysis of scd during goose follicular development: implications for lipid metabolism. Genes (Basel). (2020) 11:1001. doi: 10.3390/genes11091001
71. Gong, YJ, Lyu, WT, Shi, XF, Zou, XT, Lu, LZ, Yang, H, et al. A serum metabolic profiling analysis during the formation of fatty liver in Landes geese via Gc-Tof/Ms. Front Physiol. (2020) 11:10. doi: 10.3389/fphys.2020.581699
72. Bian, M, Lin, Z, Wang, Y, Zhang, B, Li, G, and Wang, H. Bioinformatic and Metabolomic analysis reveal intervention effects of chicory in a quail model of hyperuricemia. Evid Based Complement Alternat Med. (2018) 2018:5730385. doi: 10.1155/2018/5730385
73. Pu, S, Usuda, K, Nagaoka, K, and Watanabe, G. Heat challenge influences serum metabolites concentrations and liver lipid metabolism in Japanese quail (Coturnix Japonica). J Vet Med Sci. (2019) 81:77–83. Epub 2018/11/23. doi: 10.1292/jvms.18-0615
74. Mohr, AE, Basile, AJ, and Sweazea, KL. An urban diet differentially alters the gut microbiome and Metabolomic profiles compared with a seed diet in mourning doves. Am J Physiol Regul Integr Comp Physiol. (2022) 323:R385–96. doi: 10.1152/ajpregu.00323.2021
75. Zhang, T, Ding, H, Chen, L, Lin, YY, Gong, YS, Pan, ZM, et al. Antibiotic-induced Dysbiosis of microbiota promotes chicken lipogenesis by altering metabolomics in the cecum. Meta. (2021) 11:19. doi: 10.3390/metabo11080487
76. Cardoso, PHS, de Oliveira, ES, Liao, LM, and de Almeida Ribeiro Oliveira, G. 1h Nmr as a simple methodology for differentiating barn and free-range chicken eggs. Food Chem. (2022) 396:133720. doi: 10.1016/j.foodchem.2022.133720
77. Kim, HC, Ko, YJ, and Jo, C. Potential of 2d Qnmr spectroscopy for distinguishing chicken breeds based on the metabolic differences. Food Chem. (2021) 342:128316. doi: 10.1016/j.foodchem.2020.128316
78. Zhang, T, Zhang, S, Chen, L, Ding, H, Wu, P, Zhang, G, et al. UHPLC-MS/MS-based nontargeted metabolomics analysis reveals biomarkers related to the freshness of chilled chicken. Food Secur. (2020) 9:1326. doi: 10.3390/foods9091326
Keywords: metabolomics, application, egg, pathway, metabolites, poultry
Citation: Zhang M, You M, Ma N and Lv J (2024) Advance in the application of metabolomics technology in poultry. Front. Vet. Sci. 11:1501630. doi: 10.3389/fvets.2024.1501630
Edited by:
Kai Wang, Chinese Academy of Agricultural Sciences (CAAS), ChinaReviewed by:
Tatsuhiko Goto, Obihiro University of Agriculture and Veterinary Medicine, JapanGuoqin Li, Zhejiang Academy of Agricultural Sciences, China
Copyright © 2024 Zhang, You, Ma and Lv. This is an open-access article distributed under the terms of the Creative Commons Attribution License (CC BY). The use, distribution or reproduction in other forums is permitted, provided the original author(s) and the copyright owner(s) are credited and that the original publication in this journal is cited, in accordance with accepted academic practice. No use, distribution or reproduction is permitted which does not comply with these terms.
*Correspondence: Ning Ma, bWFuaW5nOTYxOEAxNjMuY29t; Jiancun Lv, bHZqaWFuY3VuMDAxQDE2My5jb20=