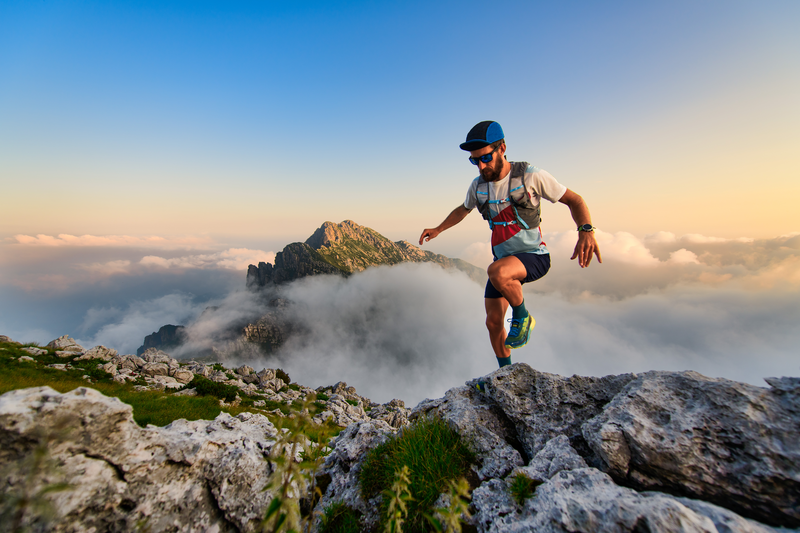
95% of researchers rate our articles as excellent or good
Learn more about the work of our research integrity team to safeguard the quality of each article we publish.
Find out more
ORIGINAL RESEARCH article
Front. Vet. Sci. , 18 November 2024
Sec. Animal Nutrition and Metabolism
Volume 11 - 2024 | https://doi.org/10.3389/fvets.2024.1489480
This article is part of the Research Topic Natural Compounds/Products and Livestock Productivity: Enhancing Antioxidant Levels, Gut Health, Mitigating Greenhouse Gas Emissions, and Disease Control View all 27 articles
The fermented Codonopsis pilosula residue (FCPR) has a promoting effect on animal health, but it has not been studied in livestock feed. This study investigated the effects of FCPR addition on the milk performance, milk metabolites, and blood biochemical parameters and metabolites of lactating donkeys. Twelve healthy multiparous lactating Dezhou donkeys were randomly divided into two groups (with 6 donkeys in each group): control group (without FCPR, C) and FCPR group (200 g of FCPR per head per day, FF). The experiment lasted for 5 weeks. The milk and blood samples were collected at the end of the experiment. The results showed that addition of FCPR significantly increased the daily milk yield and the milk components (protein, lactose, solids, solids-not-fat, and lactoferrin) yield of lactating donkeys and the weight gain of foals (p < 0.05). A total of 568 metabolites were detected in donkey milk, including 21 differential metabolites between group FF and group C. Compared to group C, the significantly up-regulated metabolic pathway in the FF group was renin secretion (p < 0.05). In addition, the FCPR significantly increased the concentrations of urea, complement C4 (C4), total antioxidant capacity (T-AOC), and catalase (CAT) in the blood, while reducing the concentration of malondialdehyde (MDA) (p < 0.05). A total of 753 metabolites were detected in serum of donkeys, including 86 differential metabolites between group FF and group C. Compared to group C, the significantly up-regulated metabolic pathways in the FF group were renin secretion, cAMP signaling pathway, regulation of lipolysis in adipocytes, and fatty acid biosynthesis (p < 0.05). The above results indicated that FCPR addition enhance the milk performance of lactating donkeys by activating the immune system, increasing the antioxidant capacity, and improving the glucose and lipid metabolism. These results provide a foundation for the development and utilization of FCPR additives, which is beneficial for livestock production and improving animal welfare.
Chinese herbal medicines (CHM) and plant extractive have been used in livestock and aquaculture and play an important role in animals’ healthy growth (1, 2). Some CHM or Chinese herbal residues (CHR) are added as feed additives to animal diets. It has been found that addition of CHR improved the gut environment of weaned piglets (2). Samanta et al. (3) studied the effects of ginger residue and inulin on pig fecal microbiota and found that they can improve intestinal microbiota and promote healthy growth of pigs. The results of Du et al. (1) found that dietary supplementation of Taraxacum mongolicum flavonoids protected Channa argus from lipopolysaccharide-induced inflammatory injury, improved digestion, activated immune responses, and enhanced antioxidant capacity. In study of Samanta et al. (3), the effects of Lonicera japonica extract on heat-stressed mid-lactation dairy cows were investigated, and the results found that Lonicera japonica extract helped to alleviate heat stress by improving antioxidant status and promoting endocrine and immune functions. The results of Abulaiti et al. (4) also showed that CHM supplementation improved the milk yield, milk composition, and serum metabolites in dairy cows.
In addition, fermented Chinese herbal medicines (FCHM) or herbal residues (FCHR) are also widely used in animal husbandry. Lactic acid bacteria fermented herbs can regulate immune responses by activating innate or adaptive immune systems and maintaining intestinal immune homeostasis (5). The results of Fujaya et al. (6) showed that the fermented herbal extract enhanced weight gain, growth and feed efficiency of Nile Tilapia by increasing nutrient absorption and improving intestinal immune function. FCHM (3 kg/t) improved the weaned piglets growth performance by improving intestinal health and promoting nutrients digestibility (7). The probiotic-fermented herbal blend prevented death and improved growth performance of Salmonella pullorum infected chicks by increasing cecal lactobacilli, reducing cecal Escherichia coli and Salmonella, and enhancing immune function (8). The results of Su et al. (2) also found that addition of FCHR improved the gut environment of weaned piglets. In addition, fermented herbal tea residue (FHTR) increased the concentrations of hydroxyl radicals, urea, and glutathione peroxidase (GSH-Px) in serum of goats (9). Zhuang et al. (10) found that FHTR replacing 30% of the forage significantly increased the immunoglobulin G (IgG), total antioxidant capacity (T-AOC), and superoxide dismutase (SOD) levels, changed the fecal microbiota composition, increased the daily feed intake and daily gain of fattening cattle. It was also found that adding FHTR increased the concentration of immunoglobulin A (IgA) and immunoglobulin G (IgG) in serum, as well as the activity of SOD and GSH-Px, thereby improving the growth of heifers (11).
Codonopsis pilosula, the root of C. pilosula (Franch.) Nannf., which is known as “Dangshen” in Chinese (12) has been used in food (tea, wine, soup and porridge) and prescribed in traditional folk medicine in Asian countries like China for thousands of years (13, 14). Codonopsis pilosula has anti-inflammatory, antioxidant, prebiotic, and immune regulation biological activities, playing an important role in the treatment of cardiovascular diseases, digestive diseases, and immune diseases (15). The Codonopsis pilosula polysaccharides (CPPs) are the major bioactive substance with biological activities (13). It was found that CPPs significantly enriched the intestinal probiotic Lactobacillus and decreased the abundance of Enterococcus and Shigella, which was beneficial for the invigorating (16). Research has also found that a polysaccharide extracted from Codonopsis pilosula residue also has a regulatory effect on glucose metabolism (17). Furthermore, the Codonopsis pilosula extract, inulin-type fructan, reduced the mucosa ulcer index of rats by reducing the malondialdehyde (MDA) and nitric oxide (NO) content and promoting the myeloperoxidase (MPO), superoxide dismutase (SOD), and glutathione peroxidase (GSH-Px) activity (18). It was also found that the A. membranaceus and Codonopsis pilosula extract mixture improved the growth performance and immunity of growing-finishing pigs (19, 20).
It is speculated that fermented Codonopsis pilosula residue (FCPR) also has a promoting effect on animal health. Thus, effects of FCPR on milk performance, milk metabolites, and blood biochemical parameters and metabolites of lactating Dezhou donkeys was investigated in this study.
Milk and serum samples were from 12 healthy multiparous lactating Dezhou donkeys with an age of 3–5 years and an average bodyweight 278 ± 30 kg at 70 ± 5 days in milk (DIM). The donkeys were housed in a farm in Liaocheng City, China. The donkeys were raised in a semi-closed house. Each donkey was kept in a separate pen. All donkeys drank freely and offered the same diet of grass hay (ad libitum) supplemented with 2.5 kg concentrate per head per day (Table 1) (21). The donkeys were randomly divided into two groups, with 6 donkeys in each group. One group did not add FCPR (control group, C), while the other group added 200 g of FCPR per head per day (the FCPR group, FF). The fermentation substrate was the Codonopsis pilosula residue. And fermentation was conducted using Bacillus and Lactobacillus plantarum (0.2%). The nutritional composition of the FCPR was as follows: dry matter (50%), crude protein (8%), nitrogen free extract (18%), crude fiber (15%), and ash (9%). The experiment lasted for 5 weeks. The milk and blood samples were collected at the end of the experiment.
The lactating donkeys were manual-milked twice a day (11 am and 3 pm). The foals were removed from the mother for 4 h before the milking. The milk yield of the donkey was recorded. The milk samples were collected during milking twice a day, frozen quickly in liquid nitrogen and stored at −80°C until analysis. The blood samples were collected from the jugular vein before feeding in the morning. After stratification, the blood samples were centrifuged at 3500 rpm, 4°C for 15 min to separate the serum. Then, the serum was frozen quickly with liquid nitrogen and stored at −80°C.
The milk compositions (fat, protein, lactose, urea, lactoferrin, solids, and solids-not-fat) were determined using the CombiScope FTIR300 (Delta Instruments B.V., Drachten, The Netherlands). There were three replicates for each sample.
The concentration or international units (IU) of serum glucose (GLU), total protein (TP), albumin (ALB), triglyceride (TG), urea, aspartate aminotransferase (AST), alanine aminotransferase (ALT), lactate dehydrogenase (LDH), alkaline phosphatase (ALP), complement C3 (C3), complement C4 (C4), IgA, IgG, immunoglobulin M (IgM), SOD, GSH-Px, T-AOC, catalase (CAT), and MDA were measured using the automatic biochemical analyzer (Mindray BS-420, Shenzhen, China), and the test kits were provided by BioSino Bio-Technology and Science Inc. (Beijing, China).
Milk or serum sample (100 μL) was added with prechilled 80% methanol. Then, the sample was placed into the Eppendorf tube and vortexed. Afterwards, the samples were incubated on ice for 5 min. Then the samples were centrifuged for 20 min at 15,000 g, 4°C. Some of supernatant was diluted with LC–MS grade water to the final concentration containing 53% methanol. After that, the samples were transferred to a fresh Eppendorf tube and then were centrifuged for 20 min at 15,000 g, 4°C. Finally, the supernatant was analyzed by liquid chromatography–tandem mass spectrometry (LC–MS/MS).
The Vanquish UHPLC system (Thermo Fisher, Germany) coupled with an Orbitrap Q Exactive TM HF mass spectrometer (Thermo Fisher, Germany) were used in UHPLC–MS/MS analyses. Samples were injected onto a Hypersil Goldcolumn (100 × 2.1 mm, 1.9 μm) at a flow rate of 0.2 mL/min using a 12-min linear gradient. The eluents for the positive and negative polarity modes were eluent A (0.1% formic acid in Water) and eluent B (methanol). The solvent gradient was set as follows: 2% B, 1.5 min; 2–85% B, 3 min; 85–100% B, 10 min; 100–2% B, 10.1 min; 2% B, 12 min. Q Exactive TM HF mass spectrometer was operated in positive/negative polarity mode with spray voltage of 3.5 kV, capillary temperature of 320°C, sheath gas flow rate of 35 psi and aux gas flow rate of 10 L/min, S-lens RF level of 60, Aux gas heater temperature of 350°C.
The Compound Discoverer 3.3 (CD3.3, ThermoFisher) was used to process the raw data files for peak alignment, peak picking, and quantitation for each metabolite. After that, peak intensities were normalized to the total spectral intensity. Then predicted the molecular formula using the normalized data based on additive ions, molecular ion peaks and fragment ions. And then peaks were matched with the mzCloud,1 mzVault and MassList database to obtain the accurate qualitative and relative quantitative results. The statistical software R (R version R-3.4.3), Python (Python 2.7.6 version) and CentOS (CentOS release 6.6) were used in statistical analyses. Throughout the analytical run, aliquots of all the milk or plasma samples were mixed to create quality control (QC) samples, which were injected at regular intervals to assess the stability of the analytical system.
These metabolites were annotated using the Kyoto Encyclopedia of Genes and Genomes (KEGG) database,2 HMDB database3 and LIPIDMaps database.4 Principal components analysis (PCA) and Partial least squares discriminant analysis (PLS-DA) was performed at metaX. The statistical significance (p-value) was calculated using univariate analysis (t-test). The metabolites were considered to be differential metabolites when VIP > 1, p < 0.05, and fold change (FC) ≥ 2 or FC ≤ 0.5. For clustering heat maps, the data were normalized using z-scores of the intensity areas of differential metabolites and were ploted by Pheatmap package in R language. The functions of these metabolites and metabolic pathways were studied using the KEGG database. The metabolic pathways enrichment of differential metabolites was performed, when ratio was satisfied by x/n > y/N and p < 0.05, the metabolic pathway was considered as statistically significant enrichment. The Pearson correlation coefficient R2 between QC samples was between 0.991 and 0.996, which was very close to 1, indicating good stability and high data quality of the detection process.
The other data were analyzed by t-test in GraphPad Prism 6 software. The results were shown as mean ± SD. The significant difference was defined when p < 0.05.
The FCPR had no effect on the average daily feed intake of lactating donkeys, and had significant impacts on donkey milk yield, foals’ weight gain, and milk component yield (Table 2). The donkey daily milk yield and foals’ weight gain in FF group were significantly higher than that in the C group (p < 0.05). Compared with the control group, the weight gain of lactating donkeys in the FF group showed an increasing trend (p = 0.0909). As for milk composition, there was no difference between the two groups. However, except for milk fat and polyunsaturated fatty acid, the yield of other milk components such as protein, lactose, solids, solids-not-fat, and lactoferrin in the FF group was significantly higher than that in the control group (p < 0.05).
The data of milk metabolites from donkeys with or without FCPR were analyzed using PLS-DA. Figure 1A displayed the PLS-DA score plot of milk metabolites from groups C and FF. Figures 1B,C showed the volcano plots and heatmap of differential metabolites in different groups of donkey milk. A total of 568 metabolites were detected in donkey milk, including 21 differential metabolites, of which 13 were up-regulated and 8 were down-regulated. The differential metabolites were displayed in Table 3 (p < 0.05). The main metabolic pathways associated with the differential metabolites and the metabolic pathways classification were shown as Figure 1D. Compared to group C, the significantly up-regulated metabolic pathway in the FF group was renin secretion (p < 0.05). And the metabolic pathways of arginine and proline metabolism (p = 0.0735), adrenergic signaling in cardiomyocytes (p = 0.0804), and regulation of lipolysis in adipocytes (p = 0.0804) showed an increasing trend.
Figure 1. The PLS-DA analysis (A) of milk metabolites and volcano plots (B), heatmap (C), and the involved metabolic pathways (D) of donkey milk differential metabolites between group FF and group C. a (b): the negative (positive) ion mode. (A) The horizontal axis PC1 and vertical axis PC2 represent the scores of the principal components ranked first and second, respectively. Different colored dots represent samples from different groups. Ellipses represent a 95% confidence interval. (B) FC = fold change. (C) Red indicates functions with relatively high values (0–4/3), while blue represents a relatively low value [0-(−4)/(−3)]. The milk samples were taken from 12 lactating donkeys with (FF) or without fermented Codonopsis pilosula residue addition (C).
The changes in blood biochemical parameters of lactating donkeys were shown in Table 4. The addition of FCPR to the diet of lactating donkeys significantly increased the blood urea and C4 concentration and activity of T-AOC, CAT (p < 0.05). And the concentration of MDA of group FF was significantly lower than group C (p < 0.01). Compared with the control group, the activity of SOD in the FF group showed an increasing trend (p = 0.0885). The other detected blood biochemical parameters were not affected by FCPR addition.
The data of serum metabolites from donkeys with or without FCPR were analyzed using PLS-DA. Figure 2A displayed the PLS-DA score plot of serum metabolites from groups C and FF. Figures 2B,C showed the volcano plots and heatmap of differential metabolites in different groups of donkey serum. A total of 753 metabolites were detected in donkey serum, including 86 differential metabolites, of which 31 were up-regulated and 55 were down-regulated. Some differential metabolites were shown in Table 5 (FC > 2, p < 0.05). The main metabolic pathways associated with the differential metabolites and the metabolic pathways classification were shown as Figure 2D. Compared to group C, the significantly up-regulated metabolic pathways in the FF group included renin secretion, cAMP signaling pathway, regulation of lipolysis in adipocytes, and fatty acid biosynthesis (p < 0.05). And the metabolic pathways of fatty acid elongation, fatty acid degradation, riboflavin metabolism, vitamin B6 metabolism, fatty acid metabolism, and adrenergic signaling in cardiomyocytes showed an increasing trend (p = 0.0885).
Figure 2. The PLS-DA analysis (A) of serum metabolites and volcano plots (B), heatmap (C), and the involved metabolic pathways (D) of donkey serum differential metabolites between group FF and group C. a (b): the negative (positive) ion mode. (A) The horizontal axis PC1 and vertical axis PC2 represent the scores of the principal components ranked first and second, respectively. Different colored dots represent samples from different groups. Ellipses represent a 95% confidence interval. (B) FC = fold change. (C) Red indicates functions with relatively high values (0–4), while blue represents a relatively low value [0-(−4)]. The serum samples were taken from 12 lactating donkeys with (FF) or without fermented Codonopsis pilosula residue addition (C).
Some studies have shown that adding FCHM to the diet can improve animal production performance. It was found that addition of FCHM improved the growth performance of weaned piglets (7). Addition of FHTR improved the rumen fermentation, and thus affected serum indicators and meat quality of black goats (22). The results of Zhuang et al. (10) showed that FHTR significantly increased the daily feed intake and daily gain of fattening cattle. In study of Shan et al. (23), the effects of dietary FCHM supplementation on milk performance were investigated, and the results found that FCHM improved the milk yield, milk fat and protein content, and immune function of dairy cows under heat stress conditions. In this study, the addition of FCPR significantly increased the donkeys’ milk yield, weight gain of foals, and the milk components (protein, lactose, solids, solids-not-fat, and lactoferrin) yield (p < 0.05). And FCPR also changed the metabolites in donkey milk. A total of 21 differential metabolites in donkey milk were detected between FF and C groups. Compared to group C, the significantly up-regulated metabolic pathway in the FF group was renin secretion. The composition of donkey milk is influenced by various factors such as breed, lactation stage, season, and dietary composition (24–26). In this study, the milk was from 12 healthy multiparous lactating Dezhou donkeys with the similar age, weight, and lactation stage. In addition, except for FCPR, lactating donkeys were fed with the same diet. Thus, the above results indicated that FCPR improved the lactation performance of female donkeys and altered the metabolites of donkey milk. Our results were consistent with the previous conclusion.
It has been found that Codonopsis pilosula has anti-inflammatory, antioxidant, and immune regulation biological activities (13, 15). Sun and Liu (27) investigated the immunity activity of CPPs, and the results found that it can stimulated lymphocyte proliferation in a dose-dependent manner. The CPPs exerted antitumor activity by enhancing immune system function (28). Results of Fan et al. (29) showed that Codonopsis pilosula glucofructan (CPG) improved both the humoral and cellular immunity and effectively inhibited tumor growth in mice. CPG could enhance immunoregulatory function and can be used as a functional food for humans (30). In this study, FCPR exhibited similar immunomodulatory biological activity. Addition of FCPR improved the immune function of lactating donkeys by increasing the level of C4 in the blood, thereby enhancing their milk production performance.
Furthermore, many studies have also shown Codonopsis pilosula has antioxidant activity. Codonopsis pilosula extract significantly alleviated mitochondrial damage and activated autophagy, thereby improving hippocampal tissue damage and alleviating cognitive impairment in aging mice (31). CPPs significantly reduced serum blood glucose, insulin level and MDA content increased SOD activity, and significantly improved insulin resistance of diabetes mice (32). In study of Liu et al. (33), the polysaccharides increased the activities of total SOD and GSH-Px, and decreased the level of MDA, and thereby increased the BW of red swamp crayfish. The neutral polysaccharide (CPP-1) could protect cells from oxidative damage (increasing the activity of SOD and CAT and reducing the MDA content), reduce the body fat index of nonalcoholic fatty liver disease mice, and improve the liver function (34). Results of other studies also found CPPs exerts a protective effect by enhancing antioxidant activity (increasing GSH-Px, SOD, and T-AOC activity and reducing MDA levels), regulating the dynamic balance of NO and endothelin-1, and significantly improving the survival rate of cells treated with hydrogen peroxide (35–37). The SOD, GSH Px, and CAT are antioxidant enzymes that catalyze the conversion of free superoxide anion radicals into non-toxic compounds, which are crucial for reducing oxidative stress and maintaining animal health (38). In this study, the addition of FCPR to the diet of lactating donkeys significantly increased the concentrations of T-AOC and CAT in the blood, while reducing the concentration of MDA (p < 0.01). Our findings were consistent with the conclusions of other studies. Our results indicated that FCPR improved the milk performance of lactating donkeys by enhancing their blood antioxidant capacity.
In addition, the blood metabolites of lactating donkeys were also detected in this study. A total of 753 metabolites were detected in donkey serum, including 86 differential metabolites, of which 31 were up-regulated and 55 were down-regulated. The main up-regulated differential metabolites including DL-α-aminocaprylic acid, isobutyryl carnitine, epinephrine, DL-m-tyrosine, ethyl (E)-2-cyano-3-(2,5-dichloroanilino)prop-2-enoate, L-Adrenaline, LPC O-22:6, 4-(4-nitrophenylazo) aniline, 2-{(3S)-1-[4-(trifluoromethyl) benzyl]-3-pyrrolidinyl}-1,3-benzoxazole, gamma-glutamylcysteine, and LPC 12:0 (FC > 2, p < 0.05). The differential metabolic pathways associated with these differential metabolites were renin secretion, cAMP signaling pathway, regulation of lipolysis in adipocytes, and fatty acid biosynthesis (p < 0.05). The above metabolic pathways were mainly related to glucose metabolism and lipid metabolism. It has been found that CPPs improved the lipid metabolism and glucose metabolism impairment, and reduce insulin resistance of obesity mice, and has the potential for the treatment of obesity (39). Bai et al. (40) investigated the effects of Codonopsis pilosula oligosaccharides (CPO) supplementation on obesity, and the results found that CPO decreased body weight and fat accumulation and improved glucose tolerance in high-fat diet induced obese mice. The results of Hu et al. (41) also found that Codonopsis pilosula polyynes participated in lipid metabolism by inhibiting the expression of squalene monooxygenase gene, which may be used in treatment of hypercholesterolemia and atherosclerosis. Liu et al. (17) also found that the polysaccharide extracted from Codonopsis pilosula residue improved the glucose metabolism by alleviating oxidative stress, improving lipid metabolism, and increasing glycolytic enzymes activity. Therefore, in this study, FCPR may enhance the milk performance of lactating donkeys by improving glucose and lipid metabolism and enhancing energy utilization efficiency.
In summary, the addition of FCPR significantly increased the daily milk yield, the yield of milk protein, lactose, solids, solids-not-fat, and lactoferrin, as well as the foals’ weight gain. A total of 21 differential metabolites between FF and C groups, which were related to global and overview maps, amino acid metabolism, and endocrine system metabolic pathways. The addition of FCPR also significantly increased the concentrations of urea, C4, T-AOC, and CAT in the blood, while reducing the concentration of MDA. And 86 differential metabolites were detected between FF and C groups, which were associated with renin secretion, cAMP signaling pathway, regulation of lipolysis in adipocytes, and fatty acid biosynthesis. In conclusion, FCPR may enhance the milk performance of lactating donkeys by activating the immune system, increasing the antioxidant capacity, and improving the glucose and lipid metabolism. These results provide a foundation for the development and utilization of FCPR additives, which are beneficial for livestock production and improve animal welfare.
The original contributions presented in the study are included in the article/supplementary material, further inquiries can be directed to the corresponding authors.
The animal study was approved by the Animal Care and Use Committee of Liaocheng University (Shandong, China) (2022111002). The study was conducted in accordance with the local legislation and institutional requirements.
MZ: Conceptualization, Methodology, Writing – original draft. FH: Investigation, Visualization, Writing – review & editing. XD: Investigation, Visualization, Writing – review & editing. GL: Funding acquisition, Methodology, Supervision, Writing – review & editing. CW: Funding acquisition, Supervision, Writing – review & editing.
The author(s) declare that financial support was received for the research, authorship, and/or publication of this article. This research was funded by the Shandong Provincial Natural Science Foundation (ZR2023MC149), the National Key R&D Program of China (2022YFD1600103, 2023YFD130200404), the Key Technology Research and Development Program of Shandong (2021TZXD012), and the Open Project of Liaocheng Universtiy Animal husbandry discipline (319312105–5).
Thanks to Wenxia Li from Liaocheng Fengyuan Biotechnology Co., Ltd. for providing fermented Codonopsis pilosula residue.
The authors declare that the research was conducted in the absence of any commercial or financial relationships that could be construed as a potential conflict of interest.
All claims expressed in this article are solely those of the authors and do not necessarily represent those of their affiliated organizations, or those of the publisher, the editors and the reviewers. Any product that may be evaluated in this article, or claim that may be made by its manufacturer, is not guaranteed or endorsed by the publisher.
1. Du, JH, Xu, MY, Wang, Y, Lei, Z, Yu, Z, and Li, MY. Evaluation of Taraxacum mongolicum flavonoids in diets for Channa argus based on growth performance, immune responses, apoptosis and antioxidant defense system under lipopolysaccharide stress. Fish Shellfish Immun. (2022) 131:1224–33. doi: 10.1016/j.fsi.2022.11.034
2. Su, J, Zhu, Q, Zhao, Y, Han, L, Yin, Y, Blachier, F, et al. Dietary supplementation with Chinese herbal residues or their fermented products modifies the colonic microbiota, bacterial metabolites, and expression of genes related to colon barrier function in weaned piglets. Front Microbiol. (2018) 9:3181. doi: 10.3389/fmicb.2018.03181
3. Samanta, AK, Jayaram, C, Jayapal, N, Sondhi, N, Kolte, AP, Senani, S, et al. Assessment of fecal microflora changes in pigs supplemented with herbal residue and prebiotic. PLoS One. (2015) 10:e0132961. doi: 10.1371/journal.pone.0132961
4. Abulaiti, A, Ahsan, U, Naseer, Z, Ahmed, Z, Liu, W, Ruan, C, et al. Effect of dietary Chinese herbal preparation on dry matter intake, milk yield and milk composition, serum biochemistry, hematological profile, and reproductive efficiency of Holstein dairy cows in early postpartum period. Front Vet Sci. (2024) 11:1434548. doi: 10.3389/fvets.2024.1434548
5. Zhu, H, Guo, L, Yu, D, and Du, X. New insights into immunomodulatory properties of lactic acid bacteria fermented herbal medicines. Front Microbiol. (2022) 13:1073922. doi: 10.3389/fmicb.2022.1073922
6. Fujaya, Y, Hidayani, AA, Sari, DK, Aslamyah, S, Rukminasari, N, Muthalib, A, et al. The optimal dosage of fermented herbal extract on growth and feed efficiency of Nile Tilapia (Oreochromis niloticus). Trop Life Sci Res. (2023) 34:39–56. doi: 10.21315/tlsr2023.34.2.3
7. Chen, G, Li, Z, Liu, S, Tang, T, Chen, Q, Yan, Z, et al. Fermented Chinese herbal medicine promoted growth performance, intestinal health, and regulated bacterial microbiota of weaned piglets. Animals. (2023) 13:476. doi: 10.3390/ani13030476
8. Wang, Y, Li, J, Xie, Y, Zhang, H, Jin, J, Xiong, L, et al. Effects of a probiotic-fermented herbal blend on the growth performance, intestinal flora and immune function of chicks infected with Salmonella pullorum. Poult Sci. (2021) 100:101196. doi: 10.1016/j.psj.2021.101196
9. Gao, C, Wu, L, Zhao, W, Chen, Y, Deng, M, Liu, G, et al. Effects of fermented herbal tea residue on serum indices and fecal microorganisms of Chuanzhong black goats. Microorganisms. (2022) 10:1228. doi: 10.3390/microorganisms10061228
10. Zhuang, X, Chen, Z, Sun, X, Li, F, Luo, J, Chen, T, et al. Fermentation quality of herbal tea residue and its application in fattening cattle under heat stress. BMC Vet Res. (2021) 17:348. doi: 10.1186/s12917-021-03061-y
11. Xie, Y, Chen, Z, Wang, D, Chen, G, Sun, X, He, Q, et al. Effects of fermented herbal tea residues on the intestinal microbiota characteristics of Holstein heifers under heat stress. Front Microbiol. (2020) 11:1014. doi: 10.3389/fmicb.2020.01014
12. He, JY, Zhu, S, Goda, Y, Cai, SQ, and Komatsu, K. Quality evaluation of medicinally-used Codonopsis species and Codonopsis Radix based on the contents of pyrrolidine alkaloids, phenylpropanoid and polyacetylenes. J Nat Med Tokyo. (2014) 68:326–39. doi: 10.1007/s11418-013-0801-0
13. Luan, F, Ji, Y, Peng, L, Liu, Q, Cao, H, Yang, Y, et al. Extraction, purification, structural characteristics and biological properties of the polysaccharides from Codonopsis pilosula: a review. Carbohydr Polym. (2021) 261:117863. doi: 10.1016/j.carbpol.2021.117863
14. Yang, J, Yang, XZ, Li, B, Lu, XY, Kang, JF, and Cao, XY. Establishment of in vitro culture system for Codonopsis pilosula transgenic hairy roots. 3 Biotech. (2020) 10:137. doi: 10.1007/s13205-020-2130-9
15. Guo, H, Lou, Y, Hou, X, Han, Q, Guo, Y, Li, Z, et al. A systematic review of the mechanism of action and potential medicinal value of codonopsis pilosula in diseases. Front Pharmacol. (2024) 15:1415147. doi: 10.3389/fphar.2024.1415147
16. Cao, L, Du, C, Zhai, X, Li, J, Meng, J, Shao, Y, et al. Codonopsis pilosula polysaccharide improved spleen deficiency in mice by modulating gut microbiota and energy related metabolisms. Front Pharmacol. (2022) 13:862763. doi: 10.3389/fphar.2022.862763
17. Liu, W, Lv, X, Huang, W, Yao, W, and Gao, X. Characterization and hypoglycemic effect of a neutral polysaccharide extracted from the residue of Codonopsis Pilosula. Carbohydr Polym. (2018) 197:215–26. doi: 10.1016/j.carbpol.2018.05.067
18. Li, JK, Wang, T, Zhu, ZC, Yang, FR, Cao, LY, and Gao, JP. Structure features and anti-gastric ulcer effects of inulin-type fructan CP-A from the roots of Codonopsis pilosula (Franch.) Nannf. Molecules. (2017) 22:2258. doi: 10.3390/molecules22122258
19. Cheng, L, Lei, Y, and Kim, IH. Dietary Astragalus membranaceus and Codonopsis pilosula extracts mixture supplementation increases the growth performance and foot-and mouth disease antibody titers in growing-finishing pigs. Livest. Sci. (2020) 240:104134. doi: 10.1016/j.livsci.2020.104134
20. Cheng, M, Pi, WX, Lu, TL, Bai, DT, Chen, J, and Xiao, SX. Determination of lobetyolin and polysaccharides in aerial part of Codonopsis pilosula and analysis of influencing factors. J Chin Med Mater. (2020) 43:1094–100. doi: 10.13863/j.issn1001-4454.2020.05.009
21. Huang, F, Du, X, Ma, Z, Liu, G, Wang, C, and Zhou, M. Effects of methionine on milk performance and milk constituents of lactating donkeys. Animals. (2024) 14:3027. doi: 10.3390/ani14203027
22. Wang, M, Wu, L, Guo, Y, Sun, J, Deng, M, Liu, G, et al. Effects of fermented herbal tea residue on meat quality, rumen fermentation parameters and microbes of black goats. AMB Express. (2023) 13:106. doi: 10.1186/s13568-023-01610-2
23. Shan, CH, Guo, J, Sun, X, Li, N, Yang, X, Gao, Y, et al. Effects of fermented Chinese herbal medicines on milk performance and immune function in late-lactation cows under heat stress conditions. J Anim Sci. (2018) 96:4444–57. doi: 10.1093/jas/sky270
24. Li, Y, Ma, Q, Zhou, M, Zhang, Z, Zhan, Y, Liu, G, et al. A metabolomics comparison in milk from two Dezhou donkey strains. Eur Food Res Technol. (2022) 248:1267–75. doi: 10.1007/s00217-022-03962-8
25. Zhou, M, Huang, F, Du, X, Liu, G, and Wang, C. Analysis of the differentially expressed proteins in donkey milk in different lactation stages. Food Secur. (2023) 12:4466. doi: 10.3390/foods12244466
26. Zhou, M, Huang, F, Du, X, Wang, C, and Liu, G. Microbial quality of donkey milk during lactation stages. Food Secur. (2023) 12:4272. doi: 10.3390/foods12234272
27. Sun, Y, and Liu, J. Structural characterization of a water-soluble polysaccharide from the roots of Codonopsis pilosula and its immunity activity. Int J Biol Macromol. (2008) 43:279–82. doi: 10.1016/j.ijbiomac.2008.06.009
28. Li, N, Xiong, YX, Ye, F, Jin, B, Wu, JJ, Han, MM, et al. Isolation, purification, and structural characterization of polysaccharides from Codonopsis pilosula and their anti-tumor bioactivity by immunomodulation. Pharmaceuticals (Basel). (2023) 16:895. doi: 10.3390/ph16060895
29. Fan, Y, Long, Y, Gong, Y, Gao, X, Zheng, G, and Ji, H. Systemic immunomodulatory effects of Codonopsis pilosula glucofructan on S180 solid-tumor-bearing mice. Int J Mol Sci. (2023) 24:15598. doi: 10.3390/ijms242115598
30. Ji, HY, Yu, J, Jiao, JS, Dong, XD, Yu, SS, and Liu, AJ. Ultrasonic-assisted extraction of Codonopsis pilosula glucofructan: optimization, structure, and immunoregulatory activity. Nutrients. (2022) 14:927. doi: 10.3390/nu14050927
31. Wang, X, Kang, J, Li, X, Wu, P, Huang, Y, Duan, Y, et al. Codonopsis pilosula water extract delays D-galactose-induced aging of the brain in mice by activating autophagy and regulating metabolism. J Ethnopharmacol. (2024) 327:118016. doi: 10.1016/j.jep.2024.118016
32. Fu, PP, Hong, T, and Yang, Z. Effect of polysaccharides from Radix Codonopsis on insulin resistance in diabetic mice. Lishizhen Med Mater Med Res. (2008) 19:2414–6.
33. Liu, F, Geng, C, Qu, YK, Cheng, BX, Zhang, Y, and Wang, AM. The feeding of dietary Codonopsis pilosula polysaccharide enhances the immune responses, the expression of immune-related genes and the growth performance of red swamp crayfish (Procambarus clarkii). Fish Shellfish Immun. (2020) 103:321–31. doi: 10.1016/j.fsi.2020.05.034
34. Ma, K, Yi, X, Yang, ST, Zhu, H, Liu, TY, Jia, SS, et al. Isolation, purification, and structural characterization of polysaccharides from Codonopsis pilosula and its therapeutic effects on non-alcoholic fatty liver disease in vitro and in vivo. Int J Biol Macromol. (2024) 265:130988. doi: 10.1016/j.ijbiomac.2024.130988
35. Qin, T, Ren, Z, Liu, XP, Luo, Y, Long, Y, and Peng, S. Study of the selenizing Codonopsis pilosula polysaccharides protects RAW264.7 cells from hydrogen peroxide-induced injury. Int J Biol Macromol. (2019) 125:534–43. doi: 10.1016/j.ijbiomac.2018.12.025
36. Wang, HY, Li, YB, Xie, RD, and Zhang, F. Protective effect of Codonopsis pilosula polysaccharides on oxidative damage of EA. hy926 cells induced by hydrogen peroxide. Pharm J Chin People Liberat Army. (2018) 34:195–200. doi: 10.3969/j.issn.1008-9926.2018.03.001
37. Zou, YF, Zhang, YY, Paulsen, BS, Rise, F, Chen, ZL, Jia, RY, et al. New pectic polysaccharides from Codonopsis pilosula and Codonopsis tangshen: structural characterization and cellular antioxidant activities. J Sci Food Agric. (2021) 101:6043–52. doi: 10.1002/jsfa.11261
38. Yu, Z, Zhao, L, Zhao, JL, Xu, W, Guo, Z, Zhang, AZ, et al. Dietary Taraxacum mongolicum polysaccharide ameliorates the growth, immune response, and antioxidant status in association with NF-κB, Nrf2 and TOR in Jian carp (Cyprinus carpio var. Jian). Aquaculture. (2022) 547:737522. doi: 10.1016/j.aquaculture.2021.737522
39. Su, Q, Huo, J, Wang, Y, Zhou, Y, Luo, D, Hou, J, et al. The obesity amelioration effect in high-fat-diet fed mice of a homogeneous polysaccharide from Codonopsis pilosula. Molecules. (2022) 27:5348. doi: 10.3390/molecules27165348
40. Bai, R, Cui, F, Li, W, Wang, Y, Wang, Z, Gao, Y, et al. Codonopsis pilosula oligosaccharides modulate the gut microbiota and change serum metabolomic profiles in high-fat diet-induced obese mice. Food Funct. (2022) 13:8143–57. doi: 10.1039/d2fo01119k
Keywords: donkeys, fermented Codonopsis pilosula residue, milk performance, metabolites, blood biochemical parameters
Citation: Zhou M, Huang F, Du X, Liu G and Wang C (2024) Fermented Codonopsis pilosula residue improved milk performance of lactating donkeys by enhancing antioxidant capacity and regulating metabolism. Front. Vet. Sci. 11:1489480. doi: 10.3389/fvets.2024.1489480
Received: 01 September 2024; Accepted: 29 October 2024;
Published: 18 November 2024.
Edited by:
Moyosore Joseph Adegbeye, University of Africa, NigeriaReviewed by:
Muyang Li, Heilongjiang Bayi Agricultural University, ChinaCopyright © 2024 Zhou, Huang, Du, Liu and Wang. This is an open-access article distributed under the terms of the Creative Commons Attribution License (CC BY). The use, distribution or reproduction in other forums is permitted, provided the original author(s) and the copyright owner(s) are credited and that the original publication in this journal is cited, in accordance with accepted academic practice. No use, distribution or reproduction is permitted which does not comply with these terms.
*Correspondence: Miaomiao Zhou, emhvdW1tMDMyOUAxNjMuY29t; Changfa Wang, d2FuZ2NoYW5nZmFAbGN1LmVkdS5jbg==
Disclaimer: All claims expressed in this article are solely those of the authors and do not necessarily represent those of their affiliated organizations, or those of the publisher, the editors and the reviewers. Any product that may be evaluated in this article or claim that may be made by its manufacturer is not guaranteed or endorsed by the publisher.
Research integrity at Frontiers
Learn more about the work of our research integrity team to safeguard the quality of each article we publish.