- 1Institute of Animal Nutrition Sichuan Agricultural University, Chengdu, China
- 2Anyou Biotechnology Group Co., Ltd., Taicang, China
Genistin is an isoflavone of soybean, with estrogenic activity. This experiment was conducted to investigate its effect on reproductive performance, antioxidant capacity, and immunity in gestating sows. Seventy-two sows (Landrace × Yorkshire) were selected and randomly divided into two treatment groups (n = 36) based on their backfat thickness, parity and fed with basal diet or supplementation of 150 mg/ kg genistin to the basal diet based on DMI for the entire gestation period. Results showed that dietary genistin supplementation significantly increased the average number of live born per litter (p < 0.05), and tended to increase the number of healthy piglets per litter (p = 0.058), but decreased the average weight of live born per litter (p < 0.05). Dietary genistin supplementation significantly decreased the number of mummified and stillbirths per litter (p < 0.05). Moreover, the average daily feed intake (ADFI) and total feed intake of the gestating sows were also increased in the genistin-supplemented group (p < 0.05). Genistin significantly increased the serum concentrations of catalase (CAT), immunoglobulin A (IgA), IgG, and IgM at 35 days of gestation (p < 0.05). The serum concentrations of interleukin-10 (IL-10) and interferon-γ (IFN-γ) were also increased upon genistin supplementation (p < 0.05). However, genistin supplementation tended to decrease the serum concentrations of total cholesterol (TC), low-density lipoprotein cholesterol (LDL-C), and leptin at 85 days of gestation (p = 0.081 and p = 0.096, respectively). Interestingly, genistin supplementation decreased the transcript abundance of interferon-γ (IFN-γ) and placental imprinting gene H19, but significantly increased the transcript abundance of insulin-like growth factor I (IGF-I) and amino acid transporters such as the sodium-coupled neutral amino acid transporter 2 (SNTA2) and SNAT4 in the placenta (p < 0.05). These results suggested that dietary genistin supplementation during gestation can improve the reproductive performance of sows, which was probably associated with improving of antioxidant capacity and immunity, as well as changes of transcript abundance of critical functional genes in the placenta.
Introduction
The number of stillbirths, mummies, abortions, and early embryonic death has a significant impact on both the reproductive performance metrics of sows and the economic viability of farms (1). Previous studies indicated that the mortality rate of pre-implantation embryos in sows falls within the range of 20 to 50%, with the majority of losses occurring between 12 to 18 days post-breeding, during the embryonic implantation period (2–4). Furthermore, a separate study underscores that over 70% of embryonic fatalities occur within the initial 25 days of gestation (5).
In the intricate choreography of mammalian reproduction, a diverse array of hormones played a pivotal role. Among these, estrogen, which was secreted by the ovary and placenta, held particular significance. It was instrumental in inducing estrus, fostering oocyte maturation, sustaining gestation, and triggering parturition mechanisms in the later stages of gestation (6–8). Prior research had shown that sows in their second to fifth parity demonstrated superior reproductive performance (9). However, as the number of mammalian litters increases, it was often accompanied by a lack of estrogen production, leading to a rapid decline in mammalian reproductive performance (10). Studies had demonstrated that estrogen supplementation could effectively enhance the physiological state and reproductive capabilities of animals. For example, studies have found that estrogen could improve the physiological state of menopausal mice, increase body weight and feed intake, and increase vitality (11). Another study showed that estrogen could promote the development and activation of chick primordial follicles (12).
Phytoestrogens have similar chemical structure as natural estrogens and can bind to estrogen receptors to exert estrogenic function (13). Phytoestrogens may be divided into chalcones, flavonoids (flavones, flavonols, flavanones, isoflavones), lignans, stilbenoids and miscellaneous classes according to their chemical structure and biosynthesis (14, 15). The isoflavonoids has attracted more attention than other groups, as they are abundant in a wide variety of natural plants (16). Recent studies have shown that animal reproductive performance can be improved by dietary supplementation of various phytoestrogens. For instance, daidzein and genistein are two major components of isoflavones. Daidzein could improve the reproductive performance of female Zhedong White geese by altering serum hormone concentrations (17). Genistein has been reported to improve the reproductive performance of breeder hens during the late egg-laying period (18) and might exhibit therapeutic potential for polycystic ovarian syndrome mice the ER-Nrf2-Foxo1-ROS pathway (19). However, an extremely high dose of genistein may lead to infertility in female mice (20, 21). Although, numerous studies investigated the influence of phytoestrogens on animal production, there are few reports on the application of genistin in sows. Therefore, this study was aimed to investigate the effects of dietary supplementation with genistin during gestation on reproductive performance, antioxidant capacity, and immunity of sows.
Materials and methods
Animals and experimental design
A total of 72 multiparous sows (3 to 6 parity, Yorkshire × Landrace) with an average backfat thickness of 13.79 ± 2.45 mm were selected from Sanmenxia Tianyuan swine farms. From the first day of artificial insemination, the sows were randomly divided into two groups (n = 36); (I) control group [basal diet (BD)], (II) genistin group (BD was supplemented with 150 mg/kg genistin). Genistin was provided by Guilin Fengpeng Biotechnology Co., Ltd. (Guilin, China), purity: 10% soybean isoflavones, genistin accounted for 8.5%. Before the start of the trial, genistin was thoroughly blended with the premix and subsequently incorporated into the compound feed to ensure even distribution, resulting in a genistin concentration of 150 mg/kg in the feed. One week prior to parturition, the sows were transferred from the gestation house to the farrowing room. Five sows in the control group and two sows in the genistin group were empty or had an abortion.
Diets and feeding management
The nutritional requirements recommended by the National Research Council (U.S.) in 2012 were met or slightly exceeded by the basic diet (Table 1). In this experiment, the concentration of genistin in the basal diet was 24.7 mg/kg (detected by Qingdao Kechuang Quality Testing Co., Ltd.). Gestation sows are raised in a single column in the gestation house, and the gestation house adopts a full fecal floor. The sows were fed twice a day (7:30 and 15:00), 1.8–2.0 kg/d (1–30 d); 2.3–2.7 kg/d (31–85 d); 3–3.5 kg/d (86–114 d). The gestation house was kept ventilated and breathable, dry and clean, and the room temperature was controlled at 20–25°C. Sows had ad libitum access to drinking water. Management and treatment plans were performed according to the swine farm plan.
Sample collection and farrowing record
At 7:00 in the morning of 35 and 85 days of gestation, 6 sows with similar average backfat thickness and parity were randomly selected from each group. These sows were fasted, and the standing Baoding method was employed to secure them with Baoding ropes, ensuring both their safety and comfort. Subsequently, blood samples were obtained via venipuncture of the anterior vena cava. The collected blood was allowed to stand at room temperature for 30 min. Thereafter, serum separation was accomplished by centrifugation at 3,500 r/min for 15 min and stored at −20°C for later analysis.
To calculate the average weight of piglets per litter and average weight of live born piglets, the weight of each piglet was recorded during farrowing (before drinking colostrum). The number of piglets per litter (including total born, live born, healthy piglets, weak piglets, and mummified and stillborn) was recorded. Healthy piglets refer to piglets with birth weight ≥0.9 kg, and weak piglets refer to piglets with birth weight <0.9 kg (farm regulations). Sow natural childbirth. The farrowing duration was recorded. The duration of farrowing means the time from the birth of the first piglet to the expulsion of all placentas. The daily feed intake of each sow was recorded to calculate the total feed intake and average daily feed intake.
After delivery, 6 sows with successful delivery were selected from each group, and the placenta was washed with saline water. Placental tissues of 3 cm × 3 cm were collected in sterilized cryopreservation tubes, which was quickly frozen in liquid nitrogen and transferred to −80°C for storage.
Analysis of serum hormone and metabolite contents
The contents of estradiol (E2, Item No. MM-0480O1), leptin (LEP, Item No. MM-0395O1), progesterone (PROG, Item No. MM-1205O1) and insulin growth factor-1 (IGF-1, Item No. MM-78075O1) were analyzed using the respective enzyme-linked immunosorbent assay (ELISA) kits (Jiangsu Meimian Industrial Co., Ltd., China) following the manufacturer’s instructions; and the serum concentrations of total protein (TP), albumin (ALB), glucose (GLU), blood urea nitrogen (BUN), triglyceride (TG), total cholesterol (TC), high-density lipoprotein cholesterol (HDL-C) and low-density lipoprotein cholesterol (LDL-C) were determined using Hitachi Automatic Biochemical Analyzer 3100 (Hitachi Diagnostic Products Co., Ltd., Shanghai). The minimal detection limits for E2, PROG, LEP and IGF-1 were 8 pmol/L, 80 pmol/L, 80 ng/L and 3 ng/L, respectively. And the intra-assay coefficient of variation (CV) of all kits was 10%, and the inter-assay CV was 12%.
Analysis of serum immunoglobulins and cytokines contents
The contents of immunoglobulin A (IgA, Item No. MM-0905O1), IgG (Item No. MM-0403O1), IgM (Item No. MM-0402O1), interleukin-1 (IL-1, Item No. MM-0426O1), IL-6 (Item No. MM-0418O1), IL-10 (Item No. MM-0425O1), interferon-γ (IFN-γ, Item No. MM-0412O1) and tumor necrosis factor-α (TNF-α, Item No. MM-0383O1) were analyzed using the respective enzyme-linked immunosorbent assay (ELISA) kits (Jiangsu Meimian Industrial Co., Ltd., China) following the manufacturer’s instructions. The lowest detectable levels of kit IgA, IgG, IgM, IL-1, IL-6, IL-10, IFN-γ and TNF-α were 1 μg/mL, 1.2 μg/mL, 12 μg/mL, 3 ng/L, 50 ng/L, 8 ng/L, 100 pg/mL and 10 pg/mL, respectively, and the intra-assay coefficient of variation (CV) of all kits was 10%, and the inter-assay CV was 12%.
Analysis of serum oxidant and antioxidant contents
The contents of superoxide dismutase (SOD, Item No. A001-2-2), total antioxidant capacity (T-AOC, Item No. A015-1-2), glutathione peroxidase (GSH-Px, Item No. A005-1-2), catalase (CAT, Item No. A0071-1) and malondialdehyde (MDA, Item No. A005-1-2) were analyzed using the respective assay kits (Nanjing Jiancheng Bioengineering Institute Co., Ltd.). All measurements were carried out in accordance with the manufacturer’s procedures.
RNA extraction and quantitative real-time polymerase chain reaction (qPCR)
Total RNA was extracted from frozen placenta samples in strict accordance with the reagent instructions of RNAiso Plus (TaKaRa, Japan). The concentration and purity of total RNA were determined by a Thermo NanoDrop-ND2000 spectrophotometer. Samples with OD260:OD280 ratios ranging from 1.8 to 2.0 were regarded as suitable for cDNA synthesis. Additionally, we determined the RNA integrity of term placentas using 1% agarose gel electrophoresis, which showed 5S, 18S, and 28S rRNA bands. Moreover, the 28S:18S ribosomal RNA band ratio was found to be ≥1.8.
The RNA samples were reverse transcribed into cDNA using a reverse transcriptase HiScript III RT SuperMix for qPCR (+gDNA wiper) kit (Vazyme, Nanjing, China) with approximately 1.0 μL RNA sample by following the manufacturer’s protocols. Detection the glutamate cysteine ligase modified subunit gene GCLM in placental tissue; fatty acid transporter 1 (FATP1); glucose transporters gene SLC2A3 and SLC2A13; amino acid transporter gene SANT1, SNAT2 SNAT4 and SLC38A10; insulin-like growth factors-1 (IGF1) (22); placental imprinting genes IGF2 and H19; placental pro-inflammatory cytokine gene IL1-β and TNF-α; the transcript abundance of antioxidant gene SOD2 and GSH-Px. TB Green® Premix Ex TaqTM II (Takara, Japan) reagent instructions are referred to for the operation steps and reaction system. The reaction system was 10 μL, containing TB Green premix Ex Taq II 5 μL, forward primer 0.4 μL, reverse primer 0.4 μL, ROX reference 0.2 μL, DEPC water 2 μL and cDNA 2 μL, The concentration of primers used was 10 μM. The thermal cycling conditions were as follows: initial denaturation at 95°C for 5 s, annealing at 60°C for 34 s and finally, extension at 72°C for 5 min. After amplification, a melting curve analysis was performed following each qPCR assay to check the specificity and purity of the resulting PCR products. The primers were consulted in the NCBI database and the primers of target gene sequence were synthesized by Sangon Biotech (Shanghai) Co., Ltd. The Primer and probe sequences are shown in the Table 2. The mRNA transcriptional abundance of the 2 groups were calculated using the method with b-actin as the reference gene (23).
Statistical analysis
All data were initially collated using Excel 2021 and analyzed using the t-test in SAS 9.4 for analysis. Normality of the data was assessed with the Shapiro–Wilk statistic (W > 0.05). In analyzing the reproductive performance of sows, the sows and their litter size were analyzed as the experimental unit. A Poisson regression analysis was carried out to analyze the number of live born, healthy piglets per litter, weak piglets per litter, mummified and stillborn by using the total born as a covariate and other data were analyzed using the relevant samples of randomly sampled sows from each group as the experimental unit. Mean and SEM were used to express the results of the experiments. When p < 0.05, differences were considered significant, while 0.05 ≤ p < 0.1 were considered to indicate a tendency.
Results
Reproductive performance and feed intake
As shown in Table 3, dietary genistin supplementation significantly increased the average number of live born per litter (p < 0.05), and tended to increase the number of healthy piglets per litter (p = 0.058), but decreased the average weight of live burns per litter (p < 0.05). Dietary genistin supplementation significantly decreased the number of mummified and stillbirths per litter (p < 0.05), and significantly increased the total feed intake of sows in gestation and their average daily intake (p < 0.05).
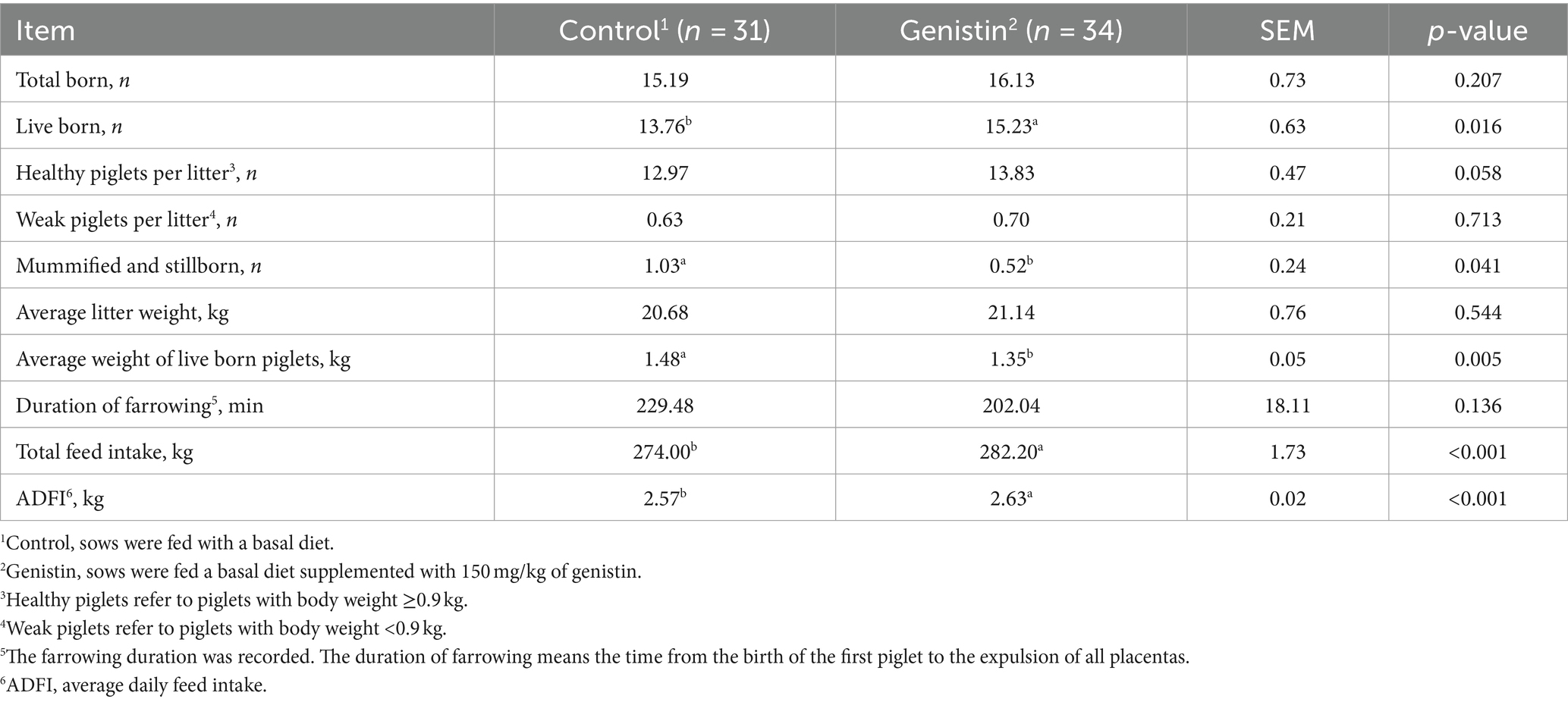
Table 3. Effects of dietary supplementation with genistin on reproductive performance and feed intake of sows.
Serum hormones and metabolites
As compared to the control group, genistin supplementation tended to decrease the serum concentrations of TC (p = 0.081) and LDL-C (p = 0.096) at 85 days of gestation (Table 4). Moreover, the serum leptin (LEP) concentration also tended to be decreased in the genistin group (p = 0.073).
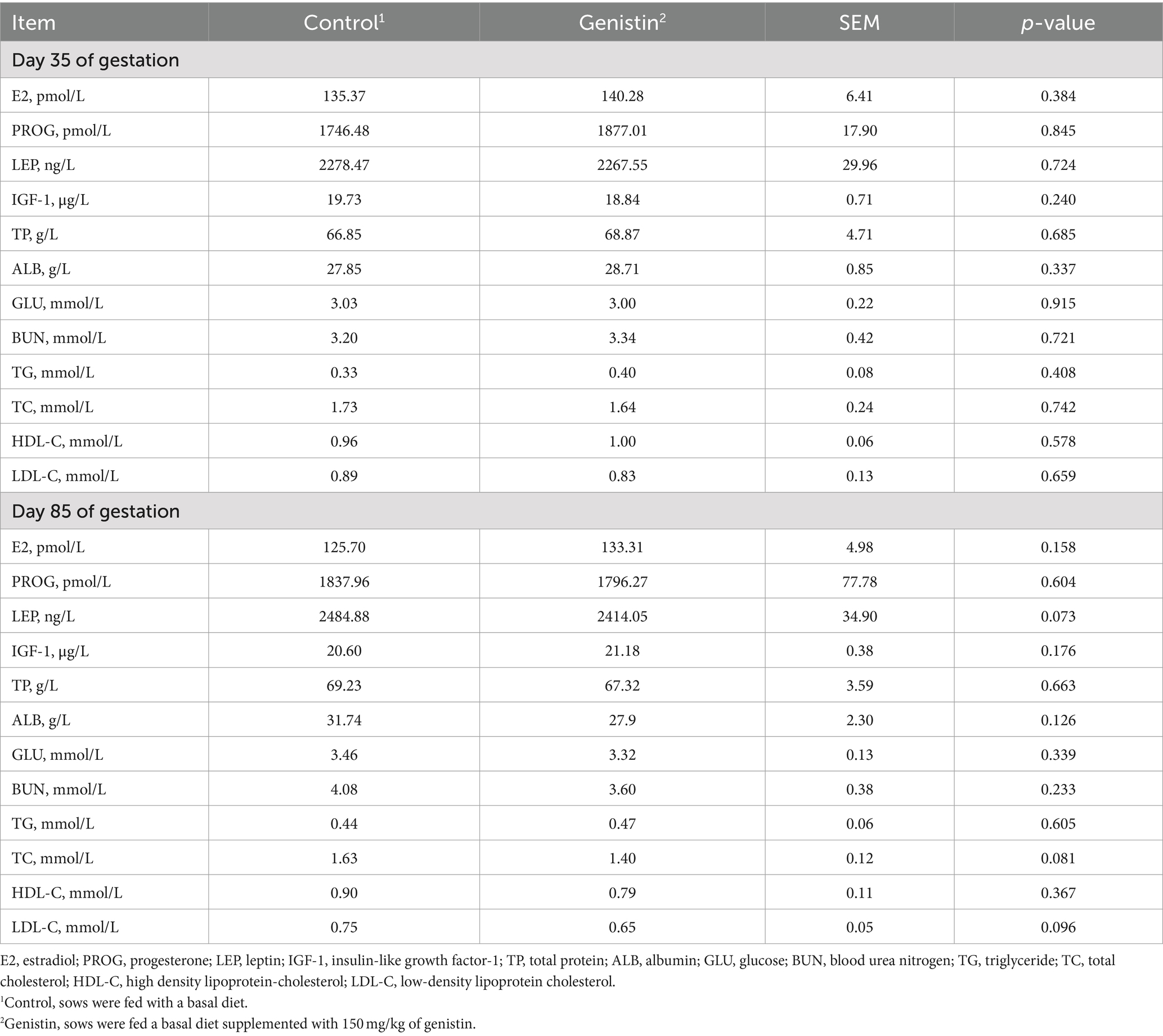
Table 4. Effects of dietary supplementation with genistin on serum hormones and metabolite levels of sows.
Serum immunoglobulins and cytokines
As shown in Table 5, genistin supplementation significantly increased the serum concentrations of IgA, IgG, and IgM at 35 days of gestation (p < 0.05). The serum concentrations of IL-10 and IFN-γ were both increased upon genistin supplementation (p < 0.05). Moreover, genistin supplementation tended to increase the serum IgM concentration at 85 days of gestation (p = 0.098).
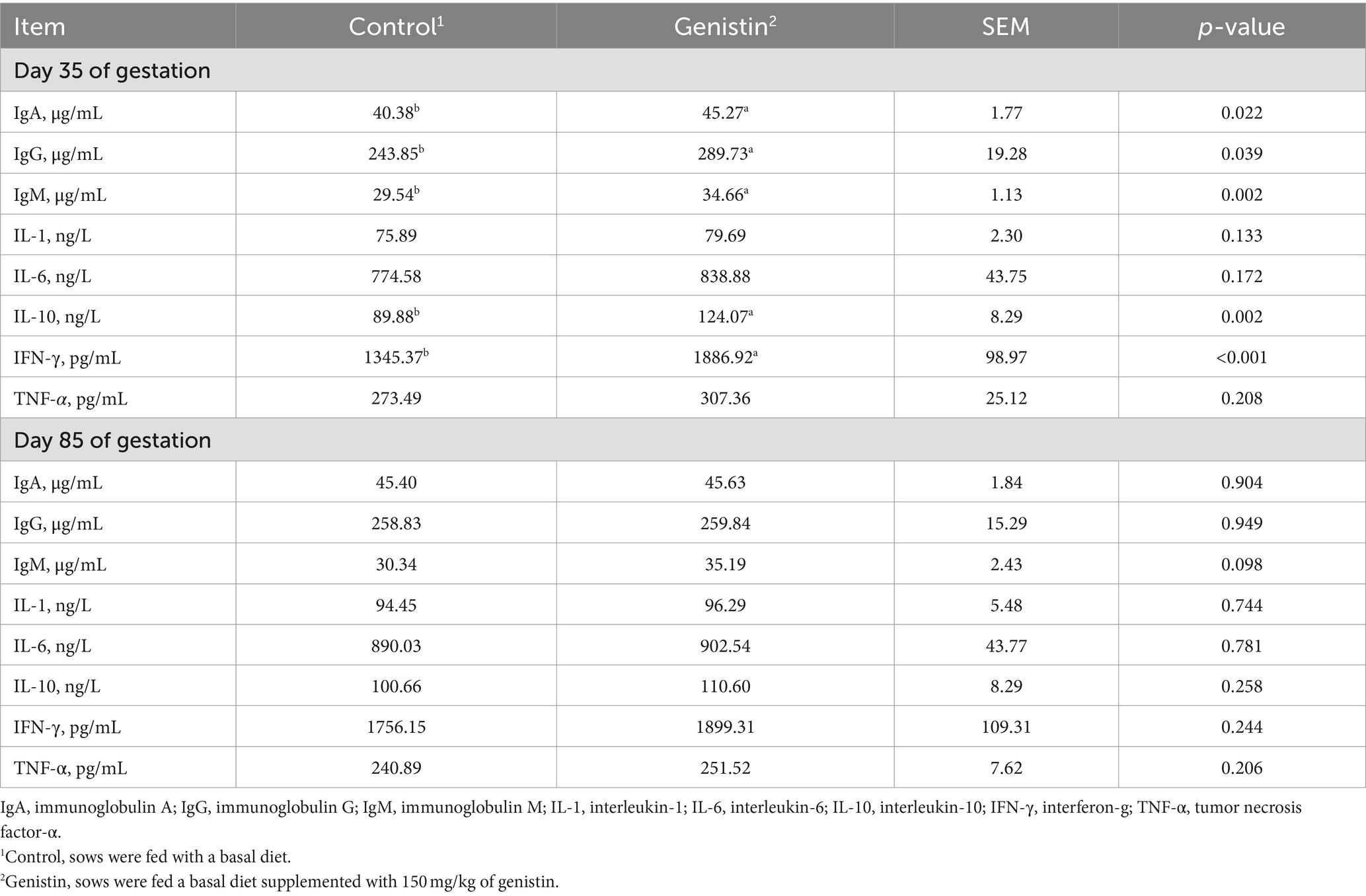
Table 5. Effects of dietary supplementation with genistin on serum immunoglobulin and cytokines of sows.
Antioxidant capacity
As shown in Table 6, dietary genistin supplementation significantly increased the serum concentration of CAT at 35 days of gestation (p < 0.05). It has no influences on the serum concentrations of antioxidative enzymes such as the SOD, GSH-Px, and CAT at 85 days of gestation (p > 0.05).
Transcriptional abundance of placental genes
As shown in Table 7, genistin supplementation significantly decreased the transcript abundance of IL-1β and H19 in the placental (p < 0.05), but increased the transcript abundance of critical imprinting genes such as the SNTA2, SNAT4, and IGF-1 (p < 0.05). However, transcript abundance of nutrient transporters (e.g., SLC2A3, SLC2A13, SLC38A10, and FATP1) did not differ between the two groups (p > 0.1).
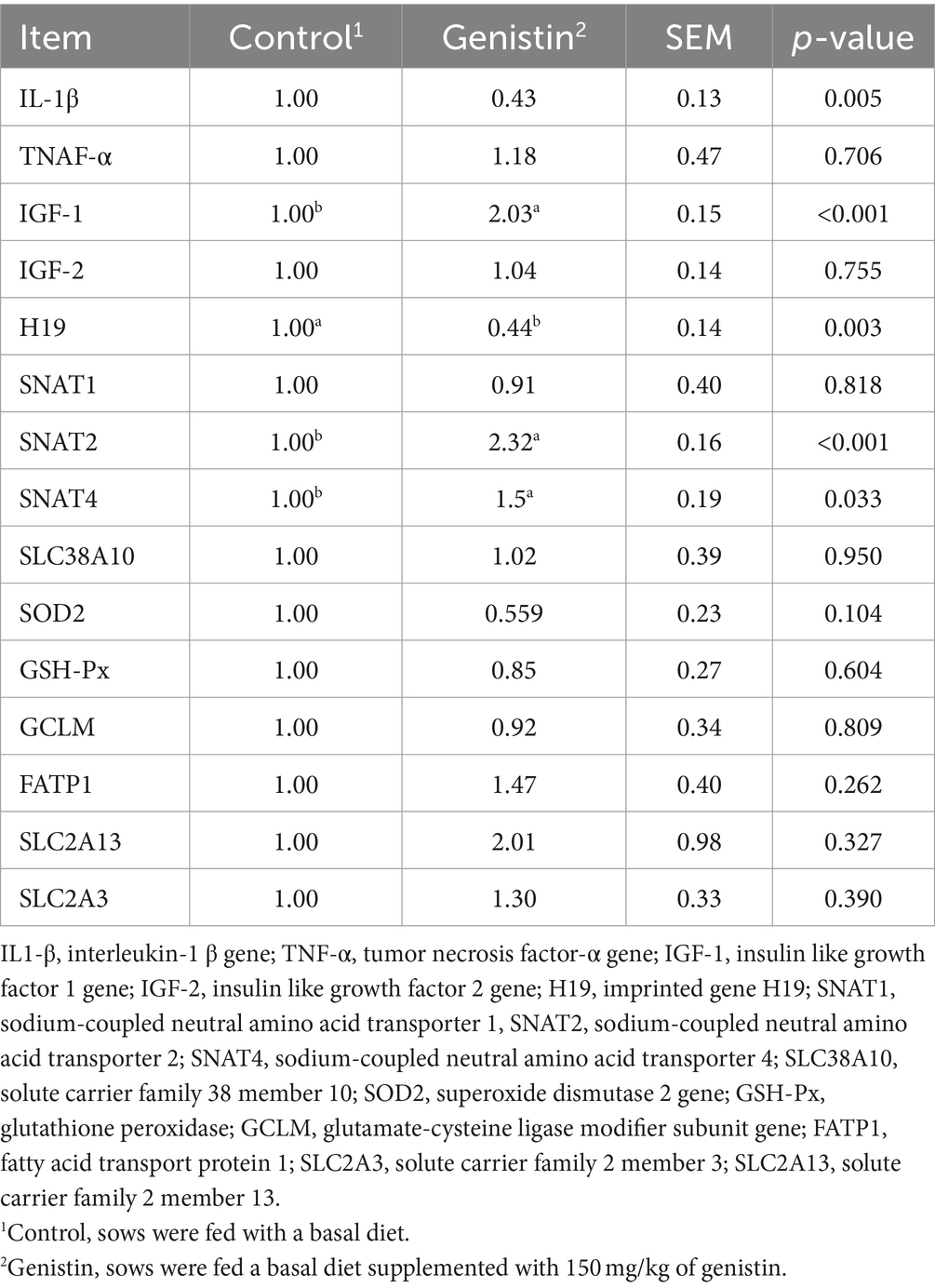
Table 7. Effect of dietary supplementation with genistin on the transcript abundance of placental functional genes IL1β, TNF-α, IGF-1, IGF-2, H19, SNAT1, SNAT2, SNAT4, SLC38A10, SOD2, GSH-Px, GCLM, FATP1, SLC2A13, and SLC2A3.
Discussion
The physiological significance of soy isoflavones has been widely elucidated over the past decade, with genistin, the main component of natural isoflavones, receiving particular attention for its estrogenic, anti-inflammatory and antioxidant properties (24). In the present study, we found that genistin supplementation at a dose of 150 mg/kg during gestation significantly increased the number of live born piglets, which is consistent with previous studies on sows (22). It is noteworthy that genistin supplementation reduced the average weight of live born piglets, which may be attributed to the limited uterine volume. Research has shown that uterine volume during gestation of sows does have an impact on litter size to some extent (25). As a result of the limited uterine volume and increased litter size, piglets may experience reduced birth weight (26). Modern pig breeds are known to have a high ovulation rate, but excessive ovulation in sows can negatively affect embryo development and survival during gestation (27). However, in this study, genistin was found to significantly reduce the number of stillbirths and mummies, corroborating earlier findings in sows (28).
Estrogen and progesterone are two important hormones that regulate reproductive processes in mammals (29). During gestation, it can promote embryo implantation and development, inhibit uterine contraction, and ensure the safe development of the fetus (8, 30, 31). Inadequate estrogen production is more likely to result in fetal loss during gestation (32). Previous research has indicated that dietary daidzein supplementation can result in a significant increase in serum progesterone and estrogen levels by the 35 days of gestation (22). However, this study showed that dietary genistin supplementation had no significant effect on progesterone and estrogen on either 35 days or 85 days of gestation. This discrepancy may be attributed to several factors: (I) distinct mechanisms of action between genistin and daidzein; (II) variances in animal species, dosages, and physiological states. Insulin-like growth factor 1 (IGF-1) has a positive regulatory effect on the supply of fetal glucose, affecting the transport of glucose and amino acids in the placenta, and is also an important factor in determining fetal size (33). IGF-I also plays an important role in controlling skeletal muscle growth (34, 35). In the present study, we found that genistin had no significant effect on serum IGF-1, probably because the 35 days of gestation and the 85 days of gestation were not the peak period of IGF-1 secretion. Studies have shown that the serum concentration of IGF-1 in sows at 10–12 days of gestation is the highest (36). The uterus or placenta of a sow can also produce IGF-1. By detecting the transcript abundance of IGF-1 gene in placental tissue, it was found that genistin significantly increased the relative expression of IGF-1, which was similar to the results of daidzein treatment (22). It is hypothesized that genistin may increase the transfer of maternal nutrients to the fetus and reduce the incidence of mummification and stillbirth by enhancing the expression of the IGF-I gene. Late gestation is an important period of fetal development. During this period, the mother needs to increase feed intake and fat deposition to meet the energy needs of fetal growth. Leptin is a key hormone involved in the body’s regulation of energy balance. A high concentration of leptin acts on the hypothalamus, leading to a decrease in animal feed intake (37). The present study found that genistin had a decreasing trend in serum leptin content on the 85 days of gestation, which may have the effect of promoting feed intake in sows and increasing nutrient supply to the fetus in the later stages of gestation.
Serum metabolites serve as indicators reflecting the nutritional metabolic status in animals. Abnormal lipid metabolism often leads to increased lipid levels and increases the susceptibility of polyunsaturated fatty acids to free radical oxidative damage, thereby affecting the reproductive performance of animals (38). Studies have shown that TC and LDL-C are related to the occurrence of preterm birth, TC concentration is negatively correlated with the occurrence of preterm birth, and high concentration of LDL-C in the third trimester of pregnancy may also lead to the occurrence of preterm birth (39, 40). In this experiment, by measuring the concentration of serum metabolites, it was found that dietary genistin supplementation trend to decrease the total cholesterol (TC) and low-density lipoprotein cholesterol (LDL-C) in serum metabolites of pregnant sows on the 85 days. It is concluded that genistin may reduce the incidence of miscarriage by reducing serum TC and LDL-C concentrations.
Oxidative stress in animals is often accompanied by inflammatory response, which is characterized by over-expression of pro-inflammatory factors and activation of the immune system (41). In the rat model of myocardial ischemia-reperfusion injury, it was found that 40–60 mg/kg genistin could significantly reduce the levels of TNF-α, IL-6 and IL-8 in serum (42). Another experiment showed that different doses of genistin significantly reduced the production of TNF-α, IL-1β and IL-6 in serum of rats (43). IL-10 is an anti-inflammatory factor and IFN-γ is a lymph factor with a wide range of immunomodulatory effects. In the present study, we found that genistin could significantly increase the levels of IL-10 and IFN-γ in the serum of sows on the 35 days of gestation. At the same time, it was found that genistin could significantly increase the content of IgA, IgM and IgG in the serum of sows during the 35 days of gestation, and IgM in the serum of sows on the 85 days of gestation had an increasing trend. These are similar to the results that dietary supplementation of 40 mg/kg genistein can significantly increase the content of IgM and IgG in the serum of broilers at 21 days of age (44), and diets were supplemented with 200 mg/kg daidzein can significantly increase the content of IgG in the serum of sows on the 35 days of gestation (22). Therefore, it is speculated that genistin can reduce inflammation and improve immunity by increasing the content of IL-10, IFN-γ, IgA, IgM and IgG in serum, so as to improve the reproductive performance of sows.
In the gestation stage, due to the rapid development needs of the fetus and envir that genistin has a scavenging effect on superoxide anion (O2−) and 200 μM concentration is equivalent to 0.08 U/mg superoxide dismutase (45). In the rat model of myocardial ischemia-reperfusion injury, it was found that 40–60 mg/kg genistin onmental factors, it may cause metabolic disorders in pregnant sows and produce excessive free radicals in the body, resulting in oxidative stress in rat (46, 47). Free radicals can be scavenged by antioxidant enzymes such as SOD, GSH-Px and CAT (48). Genistin has been proved to have antioxidant properties. Previous studies have shown genistin could significantly increase the concentration of CAT, SOD and GSH and significantly reduce the concentration of MDA in serum (42). In the present study, we found that genistin supplementation could significantly increase the concentration of CAT in the serum of sows on the 35 days of gestation, indicating that genistin could also play an antioxidant role in sows. Therefore, genistin may improve the antioxidant capacity in early pregnancy and improve the reproductive performance of sows.
The placenta has a crucial role in fetal growth by facilitating the exchange of gases, nutrients, and metabolites between the mother and the fetus (49). Both pro-inflammatory and anti-inflammatory cytokines are produced by the placenta, and the overproduction of these cytokines, such as IL-1β, is linked to the development of gestational complications (50). Studies have found that IL-1β is more capable of inducing apoptosis of fetal membrane cells than IL-6, and then affecting fetal development (51). The present study found that dietary genistin supplementation significantly decreased the transcript abundance of IL-1β in the placenta of sows. It is inferred that genistin can reduce the fetal inflammatory response by reducing the transcript abundance of IL-1β gene in the placenta, thereby reducing the fetal death. H19 and IGF-2 are maternal and paternal imprinted genes, respectively. Placental imprinted genes can regulate placental nutrient transport and are the main regulators of placental development and fetal growth. In general, maternally imprinted genes inhibit fetal growth, while paternal imprinted genes promote fetal growth (52–54). In the present study, we found that genistin supplementation significantly reduced the transcript abundance of H19 gene in placental tissue of sows, but had no significant effect on the transcript abundance of IGF-2 gene, indicating that genistin may affect the transcript abundance of placental imprinting gene H19 gene to reduce the inhibition of fetal growth. Both SNAT2 and SNAT4 are amino acid transporter genes that are crucial for transporting both essential and non-essential amino acids from the mother to the fetus (55). In the current study, genistin supplementation significantly increased the gene expression of SNAT2 and SNAT4 in placental tissue. Therefore, it is presumed that genistin can aid in the deposition and growth of fetal proteins by facilitating the transfer of amino acids from the mother to the fetus. In summary, genistin may promote fetal growth and ensure fetal health by affecting the transcript abundance of inflammatory genes, placental imprinting genes and nutrient transport genes to improve the reproductive performance of sows.
Conclusion
In conclusion, these results suggest that genistin can be used as an effective additive to improve the reproductive performance of sows. The benefits of genistin supplementation may be attributed to enhanced immune and antioxidant capacity, as well as regulation of placental essential genes.
Data availability statement
The original contributions presented in the study are included in the article/supplementary material, further inquiries can be directed to the corresponding authors.
Ethics statement
All experimental protocols used in the animal experiment were approved by the Institutional Animal Care and Use Committee of Sichuan Agricultural University (No. 20210709). The studies were conducted in accordance with the local legislation and institutional requirements. Written informed consent was obtained from the owners for the participation of their animals in this study.
Author contributions
YLi: Data curation, Validation, Visualization, Writing – original draft. BY: Supervision, Writing – review & editing. CL: Funding acquisition, Project administration, Supervision, Writing – review & editing. SX: Supervision, Writing – review & editing. YLu: Supervision, Writing – review & editing. PZ: Supervision, Writing – review & editing. GC: Supervision, Validation, Writing – review & editing. JY: Supervision, Writing – review & editing. JL: Supervision, Writing – review & editing. HY: Supervision, Writing – review & editing. JH: Funding acquisition, Methodology, Project administration, Writing – review & editing.
Funding
The author(s) declare that financial support was received for the research, authorship, and/or publication of this article. The National Key R&D Program of China (2023YFD130120), and the Innovation Team of Sichuan Province (SCCXTD-2024-8) supported this work.
Acknowledgments
The authors thank Jiangsu Anyou Biotechnology Group Co., Ltd. for the support and help of this experiment. The authors thank GC, Zhao Bo, Ren Qiang, Fu Jiawei, Yu Hanjing, Zhang Yuzhu, and SX for their help in the process of experiment and sample collection. The authors thank teacher Wang Huifen for her help in the sample determination process.
Conflict of interest
CL, SX, and GC were employed by Anyou Biotechnology Group Co., Ltd.
The remaining authors declare that the research was conducted in the absence of any commercial or financial relationships that could be construed as a potential conflict of interest.
The author(s) declared that they were an editorial board member of Frontiers, at the time of submission. This had no impact on the peer review process and the final decision.
Publisher’s note
All claims expressed in this article are solely those of the authors and do not necessarily represent those of their affiliated organizations, or those of the publisher, the editors and the reviewers. Any product that may be evaluated in this article, or claim that may be made by its manufacturer, is not guaranteed or endorsed by the publisher.
References
1. Christianson, WT. Stillbirths, mummies, abortions, and early embryonic death. Vet Clin North Am Food Anim Pract. (1992) 8:623–39. doi: 10.1016/S0749-0720(15)30708-8
2. Stroband, HW, and Van der Lende, T. Embryonic and uterine development during early pregnancy in pigs. J Reprod Fertil Suppl. (1990) 40:261–77.
3. Bazer, FW, Wu, G, Spencer, TE, Johnson, GA, Burghardt, RC, and Bayless, K. Novel pathways for implantation and establishment and maintenance of pregnancy in mammals. Mol Hum Reprod. (2010) 16:135–52. doi: 10.1093/molehr/gap095
4. Ye, Q, Zeng, X, Cai, S, Qiao, S, and Zeng, X. Mechanisms of lipid metabolism in uterine receptivity and embryo development. Trends Endocrinol Metab. (2021) 32:1015–30. doi: 10.1016/j.tem.2021.09.002
5. Eilts, BE, and Paccamonti, DL. Embryonic mortality in domestic species In: MT Zavy and RD Geisert, editors. Preventive veterinary medicine. Boca Raton, FL: CRC Press (1994). 236.
6. Geisert, RD, Zavy, MT, Moffatt, RJ, Blair, RM, and Yellin, T. Embryonic steroids and the establishment of pregnancy in pigs. J Reprod Fertil Suppl. (1990) 40:293–305.
7. Zhang, S, Lin, H, Kong, S, Wang, S, Wang, H, Wang, H, et al. Physiological and molecular determinants of embryo implantation. Mol Asp Med. (2013) 34:939–80. doi: 10.1016/j.mam.2012.12.011
8. Duan, J, Chen, H, Xu, D, Li, Y, Li, X, Cheng, J, et al. 17β-estradiol improves the developmental ability, inhibits reactive oxygen species levels and apoptosis of porcine oocytes by regulating autophagy events. J Steroid Biochem Mol Biol. (2021) 209:105826. doi: 10.1016/j.jsbmb.2021.105826
9. Koketsu, Y, and Dial, GD. Factors influencing the postweaning reproductive performance of sows on commercial farms. Theriogenology. (1997) 47:1445–61. doi: 10.1016/S0093-691X(97)00135-0
10. Albrecht, ED, Aberdeen, GW, and Pepe, GJ. The role of estrogen in the maintenance of primate pregnancy. Am J Obstet Gynecol. (2000) 182:432–8. doi: 10.1016/S0002-9378(00)70235-3
11. Yin, W, Borniger, JC, Wang, X, Maguire, SM, Munselle, ML, Bezner, KS, et al. Estradiol treatment improves biological rhythms in a preclinical rat model of menopause. Neurobiol Aging. (2019) 83:1–10. doi: 10.1016/j.neurobiolaging.2019.08.029
12. Zhao, D, Lv, C, Liu, G, Mi, Y, and Zhang, C. Effect of estrogen on chick primordial follicle development and activation. Cell Biol Int. (2017) 41:630–8. doi: 10.1002/cbin.10766
13. Canivenc-Lavier, MC, and Bennetau-Pelissero, C. Phytoestrogens and health effects. Nutrients. (2023) 15:317. doi: 10.3390/nu15020317
14. Sirotkin, AV, and Harrath, AH. Phytoestrogens and their effects. Eur J Pharmacol. (2014) 741:230–6. doi: 10.1016/j.ejphar.2014.07.057
15. Dixon, RA. Phytoestrogens. Annu Rev Plant Biol. (2004) 55:225–61. doi: 10.1146/annurev.arplant.55.031903.141729
16. Michel, T, Halabalaki, M, and Skaltsounis, AL. New concepts, experimental approaches, and dereplication strategies for the discovery of novel phytoestrogens from natural sources. Planta Med. (2013) 79:514–32. doi: 10.1055/s-0032-1328300
17. Zhao, X, Shao, T, Wang, YQ, Lu, XL, Luo, JB, and Zhou, WD. The phytoestrogen daidzein may affect reproductive performance of Zhedong White geese by regulating gene mRNA levels in the HPG axis. Br Poult Sci. (2013) 54:252–8. doi: 10.1080/00071668.2013.767439
18. Lv, ZP, Yan, SJ, Li, G, Liu, D, and Guo, YM. Genistein improves the reproductive performance and bone status of breeder hens during the late egg-laying period. Poult Sci. (2019) 98:7022–9. doi: 10.3382/ps/pez367
19. Luo, M, Zheng, LW, Wang, YS, Huang, JC, Yang, ZQ, Yue, ZP, et al. Genistein exhibits therapeutic potential for PCOS mice via the ER-Nrf2-Foxo1-ROS pathway. Food Funct. (2021) 12:8800–11. doi: 10.1039/D1FO00684C
20. Jefferson, WN, Padilla-Banks, E, and Newbold, RR. Disruption of the developing female reproductive system by phytoestrogens: genistein as an example. Mol Nutr Food Res. (2007) 51:832–44. doi: 10.1002/mnfr.200600258
21. Nagao, T, Yoshimura, S, Saito, Y, Nakagomi, M, Usumi, K, and Ono, H. Reproductive effects in male and female rats of neonatal exposure to genistein. Reprod Toxicol. (2001) 15:399–411. doi: 10.1016/S0890-6238(01)00141-1
22. Li, Y, He, G, Chen, D, Yu, B, Yu, J, Zheng, P, et al. Supplementing daidzein in diets improves the reproductive performance, endocrine hormones and antioxidant capacity of multiparous sows. Anim Nutr. (2021) 7:1052–60. doi: 10.1016/j.aninu.2021.09.002
23. Livak, KJ, and Schmittgen, TD. Analysis of relative gene expression data using real-time quantitative PCR and the 2−ΔΔCT. Methods. (2001) 25:402–8. doi: 10.1006/meth.2001.1262
24. Messina, M. Soy and health update: evaluation of the clinical and epidemiologic literature. Nutrients. (2016) 8:754. doi: 10.3390/nu8120754
25. Vallet, JL. Fetal erythropoiesis and other factors which influence uterine capacity in swine. J Appl Anim Res. (2000) 17:1–26. doi: 10.1080/09712119.2000.9706289
26. Vallet, JL, McNeel, AK, Miles, JR, and Freking, BA. Placental accommodations for transport and metabolism during intra-uterine crowding in pigs. J Anim Sci Biotechnol. (2014) 5:55. doi: 10.1186/2049-1891-5-55
27. Da Silva, CL, van den Brand, H, Laurenssen, BF, Broekhuijse, ML, Knol, EF, Kemp, B, et al. Relationships between ovulation rate and embryonic and placental characteristics in multiparous sows at 35 days of pregnancy. Animal. (2016) 10:1192–9. doi: 10.1017/S175173111600015X
28. Xie, K, Li, Y, Chen, D, Yu, B, Luo, Y, Mao, X, et al. Daidzein supplementation enhances embryo survival by improving hormones, antioxidant capacity, and metabolic profiles of amniotic fluid in sows. Food Funct. (2020) 11:10588–600. doi: 10.1039/D0FO02472D
29. Sinchak, K, Mills, RH, Tao, L, LaPolt, P, Lu, JK, and Micevych, P. Estrogen induces de novo progesterone synthesis in astrocytes. Dev Neurosci. (2003) 25:343–8. doi: 10.1159/000073511
30. Ozturk, S, and Demir, R. Particular functions of estrogen and progesterone in establishment of uterine receptivity and embryo implantation. Histol Histopathol. (2010) 25:1215–28. doi: 10.14670/HH-25.1215
31. Tripathy, S, Nallasamy, S, and Mahendroo, M. Progesterone and its receptor signaling in cervical remodeling: mechanisms of physiological actions and therapeutic implications. J Steroid Biochem Mol Biol. (2022) 223:106137. doi: 10.1016/j.jsbmb.2022.106137
32. Balogh, A, Ditrói, F, and Lampé, G. Serum progesterone levels in early imminent abortion. Acta Physiol Hung. (1985) 65:275–9.
33. Wathes, DC, Reynolds, TS, Robinson, RS, and Stevenson, KR. Role of the insulin-like growth factor system in uterine function and placental development in ruminants. J Dairy Sci. (1998) 81:1778–89. doi: 10.3168/jds.S0022-0302(98)75747-9
34. Florini, JR, Ewton, DZ, and Coolican, SA. Growth hormone and the insulin-like growth factor system in myogenesis. Endocr Rev. (1996) 17:481–517. doi: 10.1210/edrv-17-5-481
35. Li, Z, Xu, K, Guo, Y, Ping, L, Gao, Y, Qiu, Y, et al. A high-fat diet reverses metabolic disorders and premature aging by modulating insulin and IGF1 signaling in SIRT6 knockout mice. Aging Cell. (2020) 19:e13104. doi: 10.1111/acel.13104
36. Green, ML, Simmen, RC, and Simmen, FA. Developmental regulation of steroidogenic enzyme gene expression in the periimplantation porcine conceptus: a paracrine role for insulin-like growth factor-I. Endocrinology. (1995) 136:3961–70. doi: 10.1210/endo.136.9.7649105
37. Khant Aung, Z, Grattan, DR, and Ladyman, SR. Pregnancy-induced adaptation of central sensitivity to leptin and insulin. Mol Cell Endocrinol. (2020) 516:110933. doi: 10.1016/j.mce.2020.110933
38. Ciragil, P, Kurutas, EB, Gul, M, Kilinc, M, Aral, M, and Guven, A. The effects of oxidative stress in urinary tract infection during pregnancy. Mediat Inflamm. (2005) 2005:309–11. doi: 10.1155/MI.2005.309
39. Chen, Q, Chen, H, Xi, F, Sagnelli, M, Zhao, B, Chen, Y, et al. Association between maternal blood lipids levels during pregnancy and risk of small-for-gestational-age infants. Sci Rep. (2020) 10:19865. doi: 10.1038/s41598-020-76845-1
40. Wang, D, Ding, W, Ding, C, Chen, H, Zhao, W, Sun, B, et al. Higher peripheral cholesterol and a positive correlation with risk for large-for-gestational-age neonates in pre-pregnancy underweight women. Front Endocrinol. (2021) 12:760934. doi: 10.3389/fendo.2021.760934
41. Lauridsen, C. From oxidative stress to inflammation: redox balance and immune system. Poult Sci. (2019) 98:4240–6. doi: 10.3382/ps/pey407
42. Gu, M, Zheng, AB, Jin, J, Cui, Y, Zhang, N, Che, ZP, et al. Cardioprotective effects of genistin in rat myocardial ischemia-reperfusion injury studies by regulation of P2X7/NF-κB pathway. Evid Based Complement Alternat Med. (2016) 2016:5381290. doi: 10.1155/2016/5381290
43. Zhao, JH, Arao, Y, Sun, SJ, Kikuchi, A, and Kayama, F. Oral administration of soy-derived genistin suppresses lipopolysaccharide-induced acute liver inflammation but does not induce thymic atrophy in the rat. Life Sci. (2006) 78:812–9. doi: 10.1016/j.lfs.2005.05.104
44. Lv, Z, Fan, H, Zhang, B, Xing, K, and Guo, Y. Dietary genistein supplementation for breeders and their offspring improves the growth performance and immune function of broilers. Sci Rep. (2018) 8:5161. doi: 10.1038/s41598-018-23530-z
45. Russo, A, Cardile, V, Lombardo, L, Vanella, L, and Acquaviva, R. Genistin inhibits UV light-induced plasmid DNA damage and cell growth in human melanoma cells. J Nutr Biochem. (2006) 17:103–8. doi: 10.1016/j.jnutbio.2005.05.011
46. Agarwal, A, Gupta, S, and Sharma, RK. Role of oxidative stress in female reproduction. Reprod Biol Endocrinol. (2005) 3:28. doi: 10.1186/1477-7827-3-28
47. Gupta, S, Agarwal, A, Banerjee, J, and Alvarez, JG. The role of oxidative stress in spontaneous abortion and recurrent pregnancy loss: a systematic review. Obstet Gynecol Surv. (2007) 62:335–47. doi: 10.1097/01.ogx.0000261644.89300.df
48. Kurata, M, Suzuki, M, and Agar, NS. Antioxidant systems and erythrocyte life-span in mammals. Comp Biochem Physiol B. (1993) 106:477–87. doi: 10.1016/0305-0491(93)90121-K
49. Gude, NM, Roberts, CT, Kalionis, B, and King, RG. Growth and function of the normal human placenta. Thromb Res. (2004) 114:397–407. doi: 10.1016/j.thromres.2004.06.038
50. Kaneko, Y, Sano, M, Seno, K, Oogaki, Y, Takahashi, H, Ohkuchi, A, et al. Olive leaf extract (OleaVita) suppresses inflammatory cytokine production and NLRP3 inflammasomes in human placenta. Nutrients. (2019) 11:970. doi: 10.3390/nu11050970
51. Fortunato, SJ, and Menon, R. IL-1 beta is a better inducer of apoptosis in human fetal membranes than IL-6. Placenta. (2003) 24:922–8. doi: 10.1016/S0143-4004(03)00160-7
52. Constância, M, Hemberger, M, Hughes, J, Dean, W, Ferguson-Smith, A, Fundele, R, et al. Placental-specific IGF-II is a major modulator of placental and fetal growth. Nature. (2002) 417:945–8. doi: 10.1038/nature00819
53. Reik, W, Constância, M, Fowden, A, Anderson, N, Dean, W, Ferguson-Smith, A, et al. Regulation of supply and demand for maternal nutrients in mammals by imprinted genes. J Physiol. (2003) 547:35–44. doi: 10.1113/jphysiol.2002.033274
54. Coan, PM, Burton, GJ, and Ferguson-Smith, AC. Imprinted genes in the placenta—a review. Placenta. (2005) 26 Suppl A:S10–20. doi: 10.1016/j.placenta.2004.12.009
Keywords: genistin, gestation sow, reproductive performance, serum biochemistry, placental gene
Citation: Li Y, Yu B, Liu C, Xia S, Luo Y, Zheng P, Cong G, Yu J, Luo J, Yan H and He J (2024) Effects of dietary genistin supplementation on reproductive performance, immunity and antioxidative capacity in gestating sows. Front. Vet. Sci. 11:1489227. doi: 10.3389/fvets.2024.1489227
Edited by:
Elena De Felice, University of Camerino, ItalyReviewed by:
Dagan Mao, Nanjing Agricultural University, ChinaBirendra Mishra, University of Hawaii at Manoa, United States
Yi Ma, Jiangsu University, China
Copyright © 2024 Li, Yu, Liu, Xia, Luo, Zheng, Cong, Yu, Luo, Yan and He. This is an open-access article distributed under the terms of the Creative Commons Attribution License (CC BY). The use, distribution or reproduction in other forums is permitted, provided the original author(s) and the copyright owner(s) are credited and that the original publication in this journal is cited, in accordance with accepted academic practice. No use, distribution or reproduction is permitted which does not comply with these terms.
*Correspondence: Chunxue Liu, Y3hfbGl1QGFuc2NoaW5hLmNq; Jun He, aGVqdW44MDY3QDE2My5jb20=