- 1Department of Pathology, University of Texas Medical Branch, Galveston, TX, United States
- 2Department of Microbiology and Immunology, University of Texas Medical Branch, Galveston, TX, United States
- 3Center for Biodefense and Emerging Infectious Diseases, University of Texas Medical Branch, Galveston, TX, United States
- 4Sealy Institute for Vaccine Sciences, University of Texas Medical Branch, Galveston, TX, United States
- 5Institute for Human Infections and Immunity, University of Texas Medical Branch, Galveston, TX, United States
Ehrlichia canis is the primary etiologic agent of canine monocytic ehrlichiosis, a serious and sometimes fatal hemorrhagic disease of dogs. Diagnosis of E. canis infection is often retrospectively confirmed by serologic detection of antibodies by immunofluorescent microscopy. Our laboratory previously identified numerous major immunoreactive proteins with species-specific linear antibody epitopes that are useful for immunodiagnosis of CME. More recently, we have defined the entire antibody-reactive immunome of E. canis, substantially increasing the number of major immunoreactive proteins known to exist. In this study, we analyzed and compared seven recently identified antibody reactive E. canis proteins with established diagnostic antigens including tandem repeat proteins TRP19, TRP36 and TRP140 and observed comparable immunoreactivity. Many of these proteins were conserved in different E. canis strains. Multiple linear antibody epitopes were mapped in a highly conserved TRP (Ecaj_0126), including within the tandem repeat domain. Temporal antibody responses were examined, and multiple proteins reacted with antibodies in sera as early as 21 days post experimental infection. Host-specific expression of the proteins was examined which revealed that some proteins exhibited higher expression in mammalian cells, while others in tick cells. This study has identified new immunodiagnostic candidates that exhibit different host expression patterns, information which may be useful for developing ultrasensitive immunodiagnostics and effective vaccines for CME.
Introduction
Canine monocytic ehrlichiosis (CME), primarily caused by Ehrlichia canis, is a tick-borne disease in dogs of global importance. E. canis is transmitted by the brown dog tick, Rhipicephalus sanguineus (1) and CME manifests as a multisystemic disease, which can manifest in multiple forms that include acute, subclinical, or chronic phases (2). The acute phase is characterized by clinical signs and hematologic abnormalities, including depression, anorexia, weight loss, fever, bleeding, thrombocytopenia, and anemia. In the subclinical phase, dogs may spontaneously clear the infection or remain infected and appear clinically healthy for months to years. Finally, some dogs may develop a severe chronic infection characterized by hypoplastic bone marrow, bleeding, and death (3).
Diagnosis of CME can be presumptively determined by visualization of intracytoplasmic E. canis-morulae within peripheral blood monocytes; however, this method is the least sensitive and specific (4). Diagnosis is most often confirmed using serologic or molecular methods such as an immunofluorescent antibody assay or PCR (5, 6). Molecular diagnostics such as PCR can be useful, but false negative and positive results are common due to low levels of circulating ehrlichiae in the blood, combined with low PCR sensitivity, contaminants that inhibit PCR, and potential for non-specific amplification (6). Diagnosis of CME by indirect fluorescent-antibody assay (IFA) is considered the serological “gold standard,” but cross-reactive antibodies can make definitive diagnosis by IFA challenging (5). Furthermore, IFA also requires expensive microscopy equipment and subjective interpretation by an experienced microscopist. More recently, molecular immunodiagnosis of E. canis infection using peptides containing linear antibody epitopes as diagnostic markers has been utilized in veterinary reference and point of care diagnostic tests (6–8).
In the last two decades, numerous E. canis immunoreactive proteins that strongly react with antibodies in sera from infected dogs have been identified and molecularly characterized (9–13). Immunomolecular characterization of these major immunoreactive proteins has revealed several tandem repeat proteins (TRPs) that contain major species-specific linear antibody epitopes located within the tandem repeats (TRs) (10, 12, 14). Three of these TRPs (TRP19, TRP36 and TRP140) have been identified as major immunoreactive proteins that are particularly useful for diagnosis of E. canis infection (15, 16). However, genetic variability (i.e., TRP36) can limit the reliability of such antigens depending on the geographic location (17, 18). Thus, the more conserved proteins (i.e., TRP19) are more reliable and preferred for immunodiagnosis of E. canis infection (18, 19).
The recently completed E. canis immunome has revealed a group of novel immunoreactive proteins (20–22). Most of these proteins are small, secreted effectors with unknown functions. In this study, we evaluated and compared seven recently identified proteins with established TRPs for immunodiagnosis of CME. To understand differences in temporal antibody reactivity and diagnostic sensitivity, we also examined the reactivity with experimentally infected dog sera and determined expression levels of these immunoreactive proteins in E. canis-infected tick (ISE6) and mammalian (DH82) cells.
Materials and methods
Gene synthesis and cell-free expression
Ehrlichia canis (Jake Strain) gene sequences used in the study are available in the Integrated Microbial Genomes (IMG).1 E. canis gene synthesis was performed (GenScript, Piscataway, NJ, USA) and the genes were cloned into a pIVEX-2.3d or pET-14b vector containing a T7 promoter/terminator and a 6× His-tag sequence. Plasmids with cloned genes were lyophilized and stored at −20°C prior to use.
Cell-free expression of the E. canis immunoreactive proteins was performed using the S30 T7 High-Yield Protein Expression System (Promega, Madison, WI, USA). Plasmids were transformed into Stellar competent cells (Takara, Mountain View, CA, USA) and plasmid was extracted and purified using QIAprep Spin Miniprep Kit (Qiagen, Germantown, MD, USA) as previously described (21). The recombinant plasmid was mixed with E. coli extract, a premix and reaction mixture and incubated at 37°C with agitation at 750 rpm for 3 h. The cell-free expressed protein was stored at 20°C until use.
Dog sera
A panel of 15 naturally infected dog sera gifted from United States, Colombia, and Brazil that were confirmed positive for E. canis antibody by IFA were used in this study. Sera collected from a dog experimentally infected with E. canis (needle inoculation) on days 0, 7, 14, 21, 28, 35, 42 and 56 post infection was used to assess temporal antibody responses to the proteins (16).
ELISA
Immunoreactivity and diagnostic sensitivity of cell-free expressed E. canis proteins were evaluated by ELISA as described previously (21, 22). Briefly, anti-His-antibody coated ELISA plates (GenScript) were blocked with Starting Block Blocking Buffer (Thermo Fisher) at room temperature for 20 min with agitation (300 rpm). After washing twice with PBS-Tween 20 (0.05%) (PBST), plates were coated with cell-free expression lysate containing His-tagged recombinant proteins (50 μL) diluted (1:50) in blocking buffer (TBST, 2% nonfat dry milk) and incubated overnight at 4°C. The plates were washed five times and diluted dog sera (1:200, 50 μL) were added to each well and incubated at room temperature for 1 h with agitation. Plates were washed five times and incubated with alkaline phosphatase-labeled goat anti-dog IgG (H + L) secondary antibody (100 μL; 1:5000, KPL, Gaithersburg, MD), and incubated for 1 h at room temperature with agitation. The plates were washed and BluePhos substrate (100 μL; KPL) was added and incubated for 30 min in the dark with agitation. Color development was measured at A650 on a VersaMax microplate reader (Molecular Devices) and data analyzed by Softmax Pro 7 software (Molecular Devices). The final optical density (OD650) was determined after subtracting OD650 value of the negative control (cell-free lysate from empty vector). Positive and negative dog sera were included as controls.
Epitope mapping
Linear epitopes in the E. canis TRP (Ecaj_0126) were mapped with overlapping 25 amino acid peptides (GenScript, Piscataway, NJ, USA) representing the entire open reading frame, except three 35 amino acid TRs which were synthesized separately. Lyophilized peptides were resuspended in molecular grade water (1 mg/mL) and ELISA plates (MaxiSorp; NUNC, Roskilde, Denmark) were coated (1 μg/mL) overnight at 4°C. Plates were washed three times with TBST and blocked with 10% horse serum in TBST for 1 h at room temperature with agitation. The ELISA was performed as previously described (14).
Cell culture
Ehrlichia canis (Jake strain) was propagated in DH82 cells (canine macrophage-like cells) with minimal essential medium (Gibco, Grand Island, NY, USA) supplemented with 10% fetal bovine serum (HyClone, Logan, UT, USA), 1% HEPES (Sigma Chemical Co., St. Louis, MO, USA), 1% sodium pyruvate (Sigma), and 1% nonessential amino acids (Sigma) at 37°C in a humidified 5% CO2 atmosphere. ISE6,which is a tick Ixodes scapularis embryo-derived cell line, was obtained from Dr. Ulrike Munderloh (University of Minnesota) (23) and maintained in L15B300 medium supplemented with 10% fetal bovine serum (GeminiBio, Sacramento, CA, USA), 10% tryptose phosphate broth (BD, Sparks, MD, USA) and 1% bovine lipoprotein cholesterol concentrate (MP Biomedicals, Irvine, CA, USA) at 34°C as previously described (24). ISE6 cells were infected [multiplicity of infection (MOI) = 10] with host cell-free E. canis derived from infected DH82 cells.
Ehrlichia canis antigen
Ehrlichia canis antigen for Western blot analysis was prepared as described previously (17). Briefly, infected cells (DH82 and ISE6) were collected when morulae were observed in all cells after Diff-Quik staining. Cells were then centrifuged at (5,000 × g for 15 min) and resuspended in phosphate-buffered saline (PBS). Cells were ruptured by sonication, twice (40 Hz) for 10 s, and large cell debris was pelleted by centrifugation (1,500 × g for 10 min) at 4°C. The supernatant was centrifuged (10,000 × g for 15 min) at 4°C to collect cell-free ehrlichiae. The pellet was washed in PBS, centrifuged (10,000 × g for 15 min) at 4°C, and resuspended in PBS. BCA protein assay (Pierce Biotechnology, Rockford, IL, USA) was performed to determine the protein concentration. Cell lysate prepared from the uninfected cells was used as a negative control.
Antibodies
Polyclonal rabbit antibodies were commercially generated by immunizing rabbits with peptides derived from respective E. canis immunoreactive proteins (GenScript). The antisera were used for Western blot analysis and immunofluorescence microscopy.
Gel electrophoresis and Western blotting
Purified E. canis antigen was solubilized in LDS sample buffer containing the reducing agent dithiothreitol (Invitrogen), heated at 70°C for 10 min, and separated by sodium dodecyl-sulfate polyacrylamide gel electrophoresis (SDS-PAGE) in 3-N-morpholinopropanesulfonic acid (MOPS) running buffer under reducing conditions with 4 to 20% gradient Bis-Tris acrylamide gels (NuPAGE; Invitrogen). Proteins were transferred to a nitrocellulose membrane (Protran BA85, 0.45-μm pore size; Whatman, Florham Park, NJ, USA) using a semidry transfer apparatus (Bio-Rad, Hercules, CA, USA). Membranes were blocked for 1 h with TBST blocking buffer (5% nonfat milk). E. canis antisera were diluted (1:100) in the blocking buffer and incubated for 1 h at room temperature with shaking. Membranes were washed 3 times with TBST, 5 min each and an affinity-purified alkaline phosphatase-labeled goat anti-rabbit IgG (H & L) (KPL) secondary antibody (1:5000) in blocking buffer was applied and incubated for 1 h. After washing, 5-bromo-4-chloro-3-indolyl-phosphate and nitroblue tetrazolium (BCIP-NBT) substrate (KPL) was applied to visualize bound antibody. Densitometry was performed for Western blot bands of each protein using ImageJ software and the fold-changes of E. canis proteins were determined relative to cell actin.
Immunofluorescence microscopy
Antigen slides were prepared from DH82 or ISE6 cells infected with E. canis. Infected cells were applied to 12-well Teflon coated slides, air dried and fixed with 4% paraformaldehyde (PFA) for 20 min at room temperature and washed twice in PBS. Cells were permeabilized with 0.2% Triton X-100 in PBS (BSA) for 15 min and washed. Image-It Signal Enhancer (Invitrogen) was applied to the cells for 30 min, followed by BlockAid (Invitrogen) for 30 min. Cells were then incubated with antigen-specific antisera (1:100) in blocking buffer for 1.5 h, washed three times with PBST, and incubated with goat anti-rabbit IgG (H + L) Alexa Fluorophore Plus 488 secondary antibody (1:200) for 30 min in the dark. The slides were washed thrice and mounted with ProLong Glass Antifade Reagent (Invitrogen). Immunofluorescence images were captured with an Olympus BX61 epifluorescence microscope and analyzed using Slidebook software (v.5.0; Intelligent Imaging Innovations, Denver, CO, USA).
Real time quantitative PCR (qPCR)
DH82 and ISE6 cells were cultured in T-25 flasks (Cellstar) to 90–95% confluency and incubated with cell-free E. canis at a multiplicity of infection (MOI) of 10. Samples were collected daily for DH82 (5 days) and ISE6 cells (8 days), and the absolute E. canis dsb copy number was determined by real-time qPCR and plotted against the standard curve, as previously described (25). Briefly, cells were washed with PBS and lysed in SideStep Lysis and Stabilization Buffer (Agilent Technologies, Santa Clara, CA, USA), and real-time quantitative PCR (qPCR) amplification was performed using Brilliant II SYBR Green Mastermix (Agilent), forward primer (5-GCTGCTCCACCAATAAATGTATCCCT-3), and reverse primer (5-GTTTCATTAGCCAAGAATTCCGACACT-3), using a CFX96 Touch Real Time PCR System (BioRad).
Results
Comparison of protein immunoreactivity and sensitivity
Immunodominant E. canis proteins (n = 18) were recently identified by our laboratory in addition to those (i.e., TRPs, Anks, OMP-1) that have been previously reported (9, 11–13). In this study, we selected seven proteins that exhibited the strongest immunoreactivity for further evaluation and compared them to well defined immunoreactive proteins including, TRP19, TRP36 and TRP140. Consistent with our earlier findings, TRP19, TRP36 and TRP140 reacted with 15 naturally infected CME dog sera. By ELISA, TRP36 and two newly identified proteins (Ecaj_0919 and Ecaj_0126) reacted strongly with the dog sera (mean OD650 > 2.0), and 5 others (Ecaj_0717, Ecaj_0920, Ecaj_0636, Ecaj_0073 and Ecaj_0151) were slightly lower and similar to TRP140 and TRP19 (mean OD650 > 1.7). The sera from naturally infected dogs did not react with cell-free expressed negative control protein (OD650 < 0.1) and the cell-free expressed E. canis proteins (including TRPs) did not react with sera from uninfected dog. Immunoreactivity of these proteins was determined by ELISA and ranked according to mean OD650 (Figure 1A).
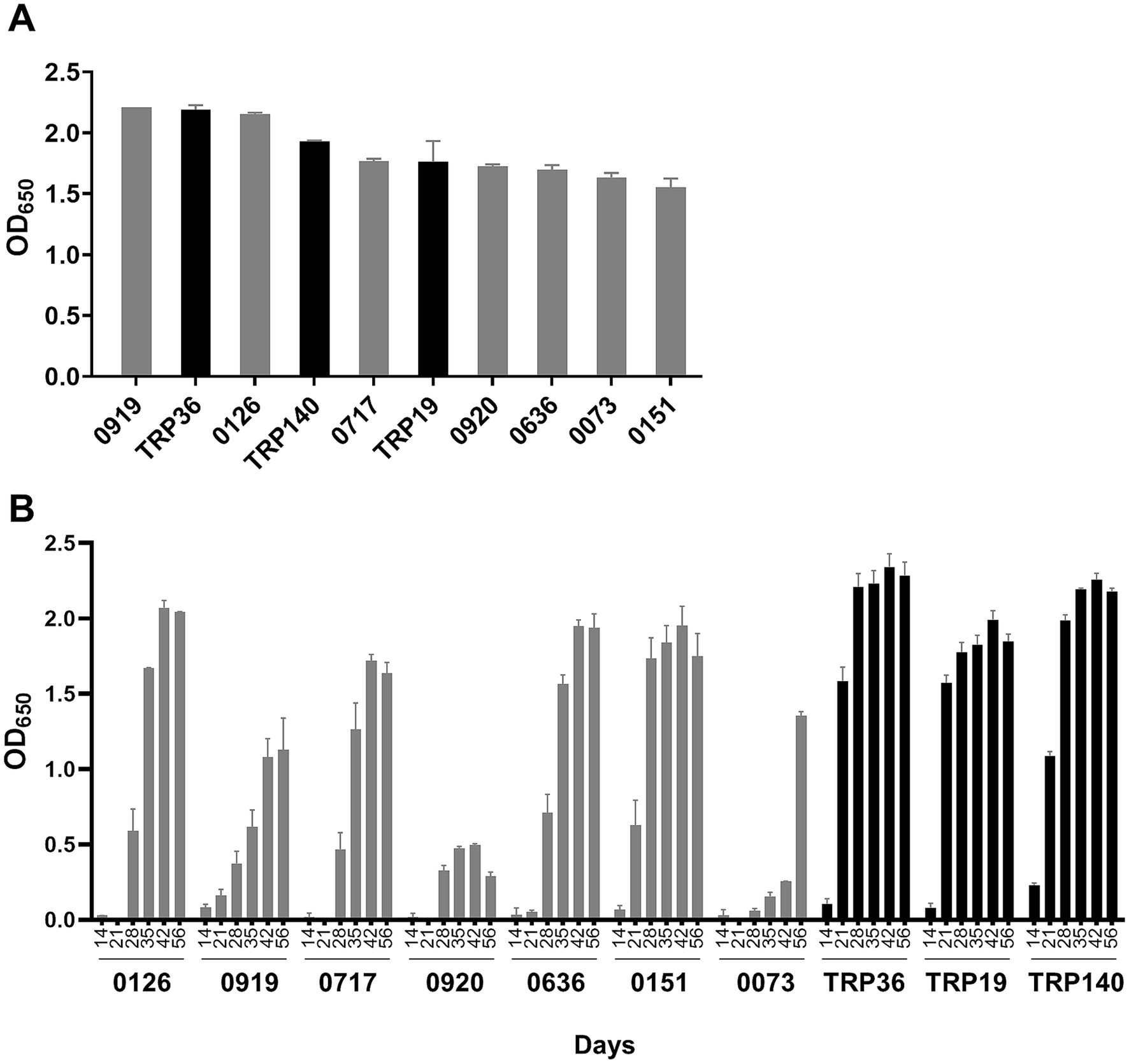
Figure 1. Comparison of E. canis major immunoreactive proteins by ELISA. (A) E. canis protein immunoreactivity. Cell-free expressed immunoreactive proteins were probed with pooled sera from 15 dogs naturally infected with E. canis. (B) Temporal antibody responses to E. canis immunoreactive proteins. Cell-free expressed proteins were probed with sera from a dog (#34) experimentally infected (needle inoculation) with E. canis collected at indicated intervals. These proteins did not react with serum from the uninfected dog (#34) as a negative control. ELISA OD values represent the mean optical density reading from triplicate wells (±SD) after background subtraction (IVTT reaction with empty plasmid template).
To understand the potential differences in diagnostic sensitivity for detection of antibodies generated early in infection, the E. canis proteins were examined using convalescent E. canis dog sera collected from an experimentally infected dog on days 0, 7, 14, 21, 28, 35, 42, and 56. Dog sera obtained prior to infection (day 0) was used as a negative control. As previously reported (15), TRP36 and TRP140 were most sensitive as both proteins reacted with antibodies in dog sera as early as day 14 (Figure 1B). TRP19 and Ecaj_0919 and Ecaj_0151 reacted with antibodies on day 21. All other immunodominant proteins reacted with antibodies at day 28 except Ecaj_0073, which reacted with antibody at day 35.
Genetic diversity of Ehrlichia canis proteins
We investigated the genetic diversity of the E. canis. Proteins (including TRPs) using a BLAST sequence analysis. Protein sequences derived from E. canis Jake strain (USA) and E. canis YZ-1 (China), and orthologous sequences from E. minasensis are shown in Table 1. E. minasensis is a relatively new Ehrlichia species that is the closest relative to E. canis (26). Four of the 7 newly identified proteins in E. canis Jake strain were identical to the homologs in the E. canis YZ-1 strain, while three proteins had minor differences in the amino acid sequence length and percent identity. TRP19 was very conserved and TRP36 and TRP140 were less conserved as previously reported (18). Compared to orthologs in E. minasensis, the E. canis TRPs varied significantly in amino acid identity (53–70%). However, E. minasensis orthologs of two newly discovered proteins (Ecaj_0073 and Ecaj_0151) were identical in length and had 88 and 94% sequence identity, respectively with E. canis. Orthologs of five remaining newly identified E. canis proteins had variations in sequence length and their amino acid identity was lower (ranging 37–80%) between strains. These data indicate that many of the recently discovered antibody reactive proteins are highly conserved among E. canis strains, but diverse in the closest E. canis relative, E. minasensis.
Tandem repeat protein (Ecaj_0126) linear epitope mapping
Our recent study defining the E. canis immunome revealed a highly immunoreactive E. canis protein (Ecaj_0126) (22). In this investigation, we determined that Ecaj_0126 contained a C-terminal TR domain (Figure 2A). Ecaj_0126 has a predicted mass of ~70 KDa (671 amino acids) and contains three nearly identical 35 amino acid repeats and a fourth partial repeat (16 amino acids). To identify linear antibody epitopes in Ecaj_0126, we screened overlapping peptides (25 amino acids; 6 amino acid overlap) and the 3 full length repeats (35 amino acids) from the TR region (Table 2). Multiple strongly immunoreactive peptides were identified with Ecaj_0126 indicating the presence of linear antibody epitopes (Figure 2B). Further investigations of these immunoreactive epitopes with additional CME dog sera (n = 10) consistently found strong antibody reactivity with the TR peptides. We then screened the Ecaj_0126 R2 peptide with 8 CME dog sera collected from different North and South American countries (USA, Colombia, and Brazil). We observed consistent antibody reactivity with all dog sera, indicating that the TR epitope is conserved among geographically dispersed E. canis strains (Figure 2C).
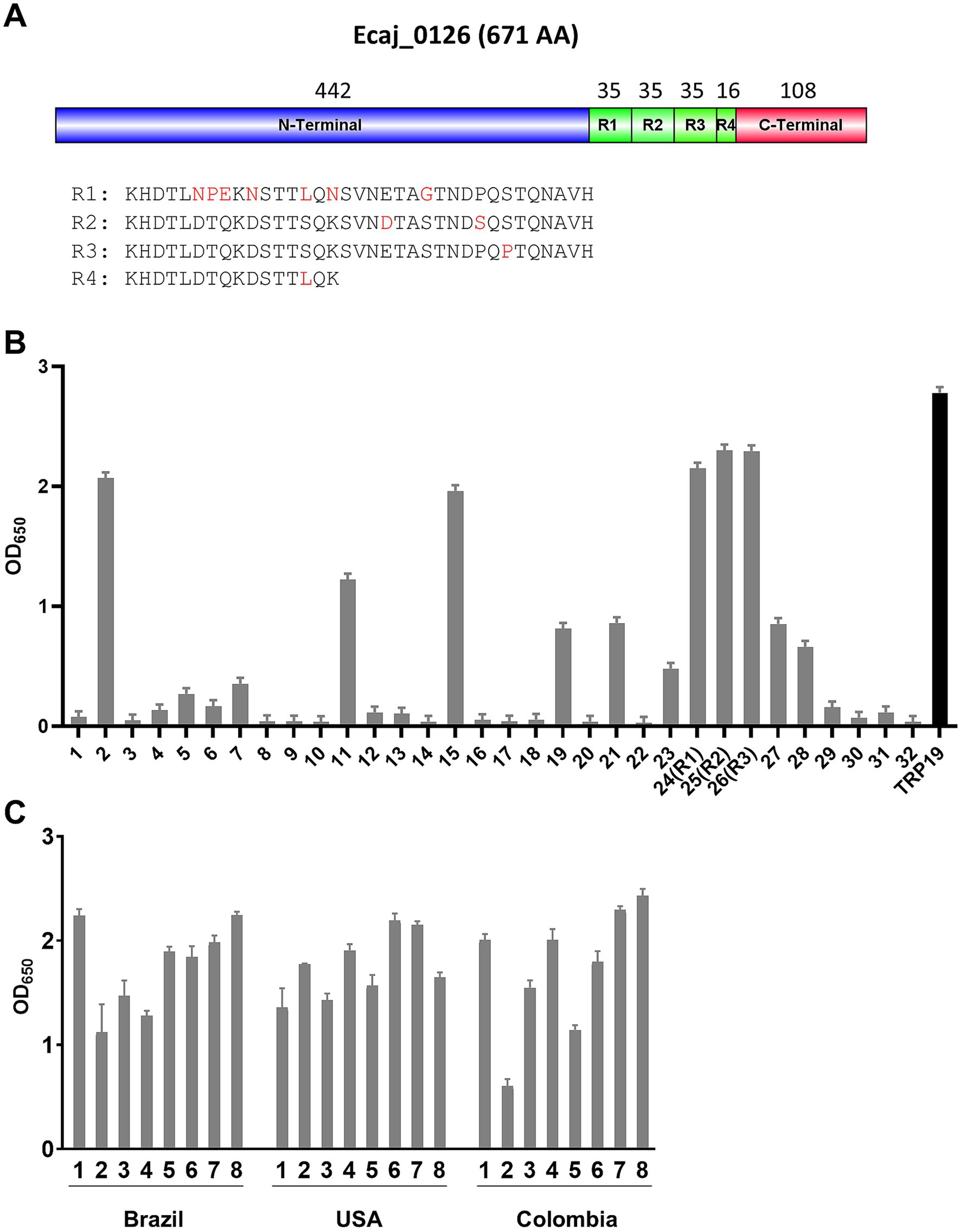
Figure 2. Linear epitope mapping of Ecaj_0126. (A) Schematic of the E. canis TRP (Ecaj_1026) showing domains and location of TRs. Number of amino acids is shown above each domain. Alignment of amino acid sequences of four TRs is shown below and heterogenous residues are showed in red. R = repeat. (B) Immunoreactivity of overlapping synthetic peptides as determined by ELISA with pooled CME dog sera from USA, Colombia and Brazil. TRP19 peptide was used as a positive control. ELISA OD values represent the mean optical density from triplicate wells (±SD) after background subtraction. (C) Immunoreactivity of Ecaj_0126 R2 peptide with 24 CME dog sera from USA, Columbia and Brazil.
Temporal expression of Ehrlichia canis proteins in mammalian and tick cells
By real time qPCR, E. canis replication increased more rapidly in DH82 cells, peaking at day 4 compared to ISE6 cells, which exhibited a slower (peak at day 7) more linear growth curve (Figure 3A). To examine differences in the protein expression levels of the E. canis TRPs and newly identified immunoreactive proteins, IFA and Western blot analyses were performed using E. canis-infected DH82 cells and ISE6 cells (Figure 3B). After determining the growth curve in both cell lines, we prepared E. canis antigen slides using DH82 cells (day 4) and ISE6 cells (day 7). By immunoblot, four proteins (TRP140, Ecaj_0126, 0920 and 0073) exhibited higher overall expression levels in ISE6 cells compared to DH82. In contrast, TRP19, TRP36, Ecaj_0636 and 0919 exhibited higher overall expression in DH82 cells. IFA images were consistent with Western immunoblot densitometry on days 4 (DH82) and 7 (ISE6) post infection.
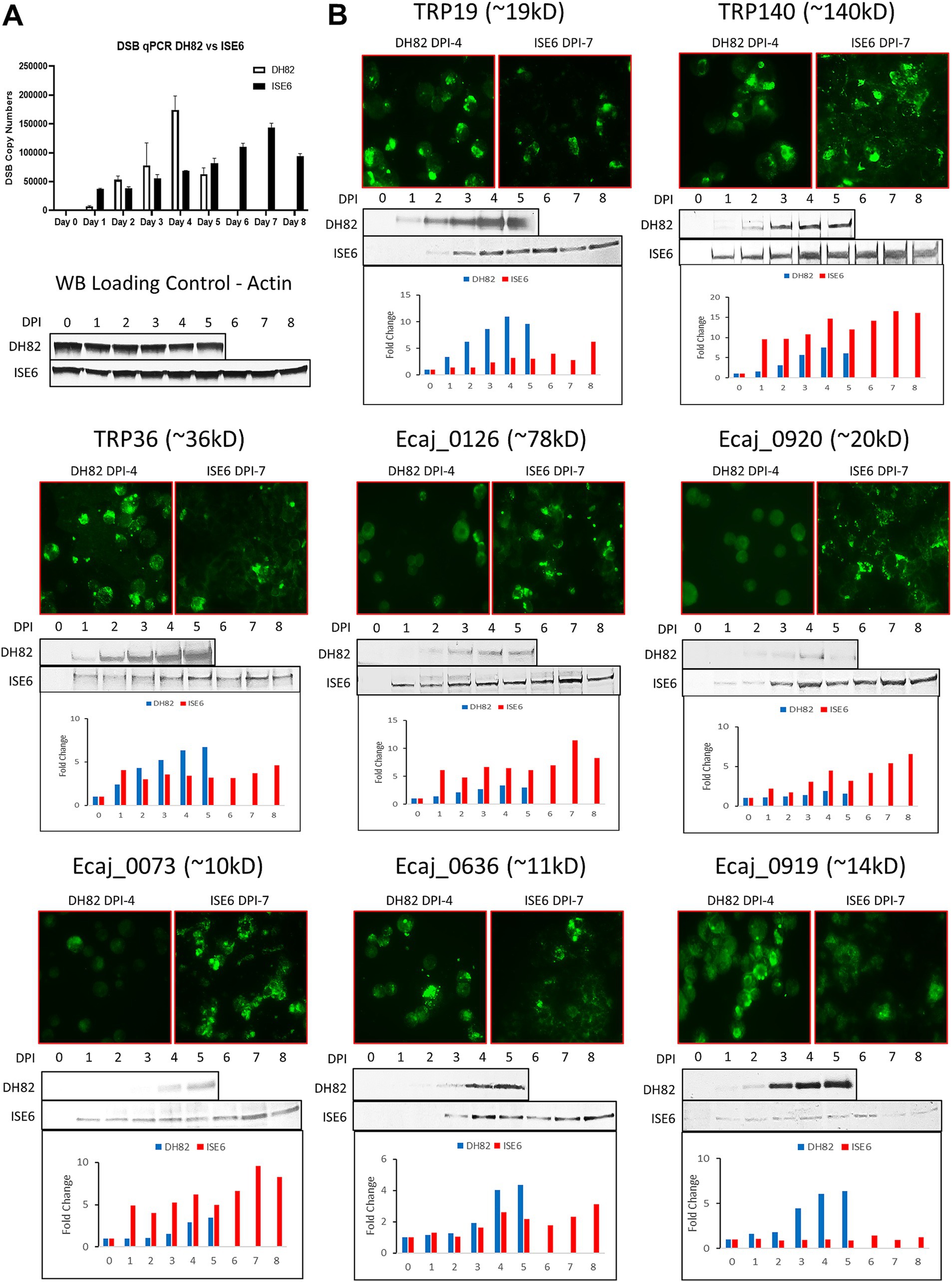
Figure 3. Host-specific and temporal expression of E. canis immunoreactive proteins. (A) Real time qPCR quantification of E. canis temporal growth in DH82 and ISE6 cells. (B) Immunofluorescent microscopy and Western immunoblot analysis of E. canis immunoreactive protein expression in DH82 and ISE6 cells. Immunofluorescent micrographs correspond to day 4 (DH82) and day 7 (ISE6) of infection. Antibodies used in the analysis were produced in rabbits against protein-specific peptides. Densitometry was performed for Western blot bands of each E. canis protein and cell actin using ImageJ software and the chart bars show the fold-changes of each protein relative to actin.
Discussion
Defining the antibody-reactive antigens of Ehrlichia spp. and their molecular characteristics is important for developing the most sensitive and specific immunodiagnostics and perhaps more importantly, protective vaccines. Defining Ehrlichia immunoreactive proteins has progressed over the last 25 years culminating in the full characterization of the antibody-reactive immunomes of E. chaffeensis and E. canis (20–22). A total of 18 new proteins were identified in E. canis that were previously unknown (22). Prior to completing the immunome, a small subset of E. canis proteins that react strongly with antibodies in sera from infected dogs were defined and considered major immunoreactive proteins, including three E. canis TRPs (TRP140, TRP36 and TRP19) and corresponding orthologs in E. chaffeensis (TRP120, TRP47 and TRP32). A more detailed immunomolecular analysis revealed major linear species-specific epitopes within the TR units of these proteins. Notably, peptides representing these epitopes are sensitive and specific for detection of antibodies, some of which are currently used as diagnostic markers in veterinary reference and point of care tests (7, 8).
E. canis is a globally distributed disease and many studies have identified genetic variation in major immunoreactive proteins such as TRP36 (18, 19). Conversely, TRP19 appears to be relatively conserved which is beneficial for developing diagnostic tests that are reliable worldwide (19). Notably, many of the new major immunoreactive proteins (Ecaj_0126, 0636, 0073, 0151) examined in this study appear to be highly conserved, whereas others exhibit some genetic diversity. This suggests that many of these conserved proteins would be particularly useful for reliable diagnosis of CME regardless of geographic location.
We previously reported that TRP36 and TRP19 elicited early antibody responses in dogs experimentally infected with E. canis (15, 16). Similarly, we found that several of the new major immunoreactive proteins elicit antibodies 3 weeks post infection. Using sera from an experimentally infected dog, we observed lower antibody responses to some proteins (Ecaj_0073 and Ecaj_0920) than was observed with naturally infected dog sera (Figure 1). The difference in the response we observed with sera from naturally infected dogs may be related to factors associated with tick transmission compared to experimental needle inoculation, the route used to experimentally infect dogs. It is notable that these two proteins exhibited higher expression levels in tick cells compared to mammalian cells in this study which supports this possibility. Nevertheless, all the new major immunoreactive proteins elicit a robust antibody response in naturally infected dogs and suggests that these proteins could be valuable diagnostic and/or vaccine candidates.
Since changes in Ehrlichia phenotype in different hosts is not well understood, we examined the expression of these immunoreactive proteins in tick and mammalian host cells. In a previous study we reported Ehrlichia gene transcription profiles in tick and mammalian cells and found that E. chaffeensis transcriptome expression is higher in tick cells compared to mammalian cells (24). However, TRPs were found to be some of the most highly expressed genes in mammalian cells, which was consistent with the expression data we observed in the current study. Notably, half of the proteins examined in this study demonstrated higher expression levels in tick cells suggesting they may be important for tick infection and transmission. These differences in host expression also illustrate how studies using only ehrlichiae produced in mammalian cells could be biased. Many strongly immunoreactive proteins are more highly expressed in ticks and likely to be important targets for transmission blocking vaccines or elicit earlier antibody responses that would be useful for improving diagnostic sensitivity.
E. chaffeensis and E. canis TRPs were identified more than a decade ago based on strong antibody reactivity by Western immunoblot (16). Subsequently, immunomolecular analysis and epitope mapping identified species-specific linear antibody epitopes in TRPs that defined the molecular basis of antibody reactivity (12, 14, 27). Notably, TRPs are now known to be secreted effector proteins that have multiple functions and interactions with the host cell including acting as nucleomodulins, ubiquitin ligases and eukaryotic signaling pathway ligand mimics (28). Similarly, a new TRP was identified (Ecaj_0126) that is 671 amino acids in length and has three (35 amino acids) TRs. Like other well-known TRPs, Ecaj_0126 exhibited strong immunoreactivity, and in this study, we identified a linear antibody epitope in the TR of Ecaj_0126. Moreover, we also identified at least 3 major linear epitopes outside the TR domain that exhibited immunoreactivity similar to the TR epitope. Notably, Ecaj_0126 is highly conserved (100%) among dispersed E. canis strains, suggesting it could be a very reliable diagnostic antigen regardless of genetic and phenotypic variation among strains. This is further supported by the strong and consistent reactivity of Ecaj_0126 peptides with dog sera from North and South America.
Data availability statement
The original contributions presented in the study are included in the article/Supplementary material, further inquiries can be directed to the corresponding author.
Ethics statement
Ethical approval was not required for the study involving animals in accordance with the local legislation and institutional requirements because all cell strains and animal sera were purchased from commercial companies, gifted from other research groups, or isolated as part of our previous approved study.
Author contributions
JP: Data curation, Formal analysis, Investigation, Methodology, Software, Validation, Writing – original draft. TL: Data curation, Formal analysis, Investigation, Software, Validation, Writing – review & editing. XZ: Data curation, Formal analysis, Methodology, Writing – review & editing. JM: Conceptualization, Data curation, Formal analysis, Funding acquisition, Investigation, Methodology, Project administration, Resources, Supervision, Validation, Writing – original draft, Writing – review & editing.
Funding
The author(s) declare financial support was received for the research, authorship, and/or publication of this article. This study was supported by the Clayton Foundation for Research.
Conflict of interest
The authors declare that the research was conducted in the absence of any commercial or financial relationships that could be construed as a potential conflict of interest.
Publisher’s note
All claims expressed in this article are solely those of the authors and do not necessarily represent those of their affiliated organizations, or those of the publisher, the editors and the reviewers. Any product that may be evaluated in this article, or claim that may be made by its manufacturer, is not guaranteed or endorsed by the publisher.
Supplementary material
The Supplementary material for this article can be found online at: https://www.frontiersin.org/articles/10.3389/fvets.2024.1481934/full#supplementary-material
Footnotes
References
1. Groves, MG, Dennis, GL, Amyx, HL, and Huxsoll, DL. Transmission of Ehrlichia canis to dogs by ticks (Rhipicephalus sanguineus). Am J Vet Res. (1975) 36:937–40.
2. Mylonakis, ME, Harrus, S, and Breitschwerdt, EB. An update on the treatment of canine monocytic ehrlichiosis (Ehrlichia canis). Vet J. (2019) 246:45–53. doi: 10.1016/j.tvjl.2019.01.015
3. Mylonakis, ME, Koutinas, AF, Breitschwerdt, EB, Hegarty, BC, Billinis, CD, Leontides, LS, et al. Chronic canine ehrlichiosis (Ehrlichia canis): a retrospective study of 19 natural cases. J Am Anim Hosp Assoc. (2004) 40:174–84. doi: 10.5326/0400174
4. Mylonakis, ME, Koutinas, AF, Billinis, C, Leontides, LS, Kontos, V, Papadopoulos, O, et al. Evaluation of cytology in the diagnosis of acute canine monocytic ehrlichiosis (Ehrlichia canis): a comparison between five methods. Vet Microbiol. (2003) 91:197–204. doi: 10.1016/S0378-1135(02)00298-5
5. Waner, T, Harrus, S, Jongejan, F, Bark, H, Keysary, A, and Cornelissen, AW. Significance of serological testing for ehrlichial diseases in dogs with special emphasis on the diagnosis of canine monocytic ehrlichiosis caused by Ehrlichia canis. Vet Parasitol. (2001) 95:1–15. doi: 10.1016/S0304-4017(00)00407-6
6. Harrus, S, and Waner, T. Diagnosis of canine monocytotropic ehrlichiosis (Ehrlichia canis): an overview. Vet J. (2011) 187:292–6. doi: 10.1016/j.tvjl.2010.02.001
7. Beall, MJ, Mainville, CA, Arguello-Marin, A, Clark, G, Lemieux, C, Saucier, J, et al. An improved point-of-care ELISA for the diagnosis of Anaplasmosis and Ehrlichiosis during the acute phase of tick-borne infections in dogs. Top Companion Anim Med. (2022) 51:100735. doi: 10.1016/j.tcam.2022.100735
8. Qurollo, BA, Stillman, BA, Beall, MJ, Foster, P, Hegarty, BC, Breitschwerdt, EB, et al. Comparison of Anaplasma and Ehrlichia species-specific peptide ELISAs with whole organism-based immunofluorescent assays for serologic diagnosis of anaplasmosis and ehrlichiosis in dogs. Am J Vet Res. (2021) 82:71–80. doi: 10.2460/ajvr.82.1.71
9. Yu, XJ, McBride, JW, Diaz, CM, and Walker, DH. Molecular cloning and characterization of the 120-kilodalton protein gene of Ehrlichia canis and application of the recombinant 120-kilodalton protein for serodiagnosis of canine ehrlichiosis. J Clin Microbiol. (2000) 38:369–74. doi: 10.1128/JCM.38.1.369-374.2000
10. Doyle, CK, Cardenas, AM, Aguiar, DM, Labruna, MB, Ndip, LM, Yu, XJ, et al. Molecular characterization of E. canis gp36 and E. chaffeensis gp47 tandem repeats among isolates from different geographic locations. Ann N Y Acad Sci. (2005) 1063:433–5. doi: 10.1196/annals.1355.079
11. Nethery, KA, Doyle, CK, Zhang, X, and McBride, JW. Ehrlichia canis gp200 contains dominant species-specific antibody epitopes in terminal acidic domains. Infect Immun. (2007) 75:4900–8. doi: 10.1128/IAI.00041-07
12. McBride, JW, Doyle, CK, Zhang, X, Cardenas, AM, Popov, VL, Nethery, KA, et al. Identification of a glycosylated Ehrlichia canis 19-kilodalton major immunoreactive protein with a species-specific serine-rich glycopeptide epitope. Infect Immun. (2007) 75:74–82. doi: 10.1128/IAI.01494-06
13. McBride, JW, Yu, X, and Walker, DH. Molecular cloning of the gene for a conserved major immunoreactive 28-kilodalton protein of Ehrlichia canis: a potential serodiagnostic antigen. Clin Diagn Lab Immunol. (1999) 6:392–9. doi: 10.1128/CDLI.6.3.392-399.1999
14. Luo, T, Zhang, X, and McBride, JW. Major species-specific antibody epitopes of the Ehrlichia chaffeensis p120 and E. canis p140 orthologs in surface-exposed tandem repeat regions. Clin Vacc Immunol. (2009) 16:982–90. doi: 10.1128/CVI.00048-09
15. Cardenas, AM, Doyle, CK, Zhang, X, Nethery, K, Corstvet, RE, Walker, DH, et al. Enzyme-linked immunosorbent assay with conserved immunoreactive glycoproteins gp36 and gp19 has enhanced sensitivity and provides species-specific immunodiagnosis of Ehrlichia canis infection. Clin Vacc Immunol. (2007) 14:123–8. doi: 10.1128/CVI.00361-06
16. McBride, JW, Corstvet, RE, Gaunt, SD, Boudreaux, C, Guedry, T, and Walker, DH. Kinetics of antibody response to Ehrlichia canis immunoreactive proteins. Infect Immun. (2003) 71:2516–24. doi: 10.1128/IAI.71.5.2516-2524.2003
17. Zhang, X, Luo, T, Keysary, A, Baneth, G, Miyashiro, S, Strenger, C, et al. Genetic and antigenic diversities of major immunoreactive proteins in globally distributed Ehrlichia canis strains. Clin Vacc Immunol. (2008) 15:1080–8. doi: 10.1128/CVI.00482-07
18. Aguiar, DM, Zhang, X, Melo, AL, Pacheco, TA, Meneses, AM, Zanutto, MS, et al. Genetic diversity of Ehrlichia canis in Brazil. Vet Microbiol. (2013) 164:315–21. doi: 10.1016/j.vetmic.2013.02.015
19. Nambooppha, B, Rittipornlertrak, A, Tattiyapong, M, Tangtrongsup, S, Tiwananthagorn, S, Chung, YT, et al. Two different genogroups of Ehrlichia canis from dogs in Thailand using immunodominant protein genes. Infection Genet Evol. (2018) 63:116–25. doi: 10.1016/j.meegid.2018.05.027
20. Luo, T, Patel, JG, Zhang, X, Walker, DH, and McBride, JW. Ehrlichia chaffeensis and E. canis hypothetical protein immunoanalysis reveals small secreted immunodominant proteins and conformation-dependent antibody epitopes. NPJ Vacc. (2020) 5:85. doi: 10.1038/s41541-020-00231-1
21. Luo, T, Patel, JG, Zhang, X, Walker, DH, and McBride, JW. Immunoreactive protein repertoires of Ehrlichia chaffeensis and E. canis reveal the dominance of hypothetical proteins and conformation-dependent antibody epitopes. Infect Immun. (2021) 89:e0022421. doi: 10.1128/IAI.00224-21
22. Luo, T, Patel, JG, Zhang, X, and McBride, JW. Antibody reactive immunomes of Ehrlichia chaffeensis and E. canis are diverse and defined by conformational antigenic determinants. Front Cell Infect Microbiol. (2023) 13:1321291. doi: 10.3389/fcimb.2023.1321291
23. Munderloh, UG, Liu, Y, Wang, M, Chen, C, and Kurtti, TJ. Establishment, maintenance and description of cell lines from the tick Ixodes scapularis. J Parasitol. (1994) 80:533–43. doi: 10.2307/3283188
24. Kuriakose, JA, Miyashiro, S, Luo, T, Zhu, B, and McBride, JW. Ehrlichia chaffeensis transcriptome in mammalian and arthropod hosts reveals differential gene expression and post transcriptional regulation. PLoS One. (2011) 6:e24136. doi: 10.1371/journal.pone.0024136
25. Dunphy, PS, Luo, T, and McBride, JW. Ehrlichia chaffeensis exploits host SUMOylation pathways to mediate effector-host interactions and promote intracellular survival. Infect Immun. (2014) 82:4154–68. doi: 10.1128/IAI.01984-14
26. Cabezas-Cruz, A, Valdes, JJ, and de la Fuente, J. The glycoprotein TRP36 of Ehrlichia sp. UFMG-EV and related cattle pathogen Ehrlichia sp. UFMT-BV evolved from a highly variable clade of E. canis under adaptive diversifying selection. Parasit Vectors. (2014) 7:584. doi: 10.1186/s13071-014-0584-5
27. Doyle, CK, Nethery, KA, Popov, VL, and McBride, JW. Differentially expressed and secreted major immunoreactive protein orthologs of Ehrlichia canis and E. chaffeensis elicit early antibody responses to epitopes on glycosylated tandem repeats. Infect Immun. (2006) 74:711–20. doi: 10.1128/IAI.74.1.711-720.2006
Keywords: Ehrlichia, E. canis, immunoreactive protein, Canine monocytic ehrlichiosis (CME), immunodiagnosis, vaccine, tandem repeat proteins (TRPs)
Citation: Patel JG, Luo T, Zhang X and McBride JW (2024) Immuno- and expression analysis of Ehrlichia canis immunoreactive proteins. Front. Vet. Sci. 11:1481934. doi: 10.3389/fvets.2024.1481934
Edited by:
Eric M. Nicholson, Agricultural Research Service (USDA), United StatesReviewed by:
Jifeng Bian, Agricultural Research Service (USDA), United StatesBenjamin Cull, University of Minnesota Twin Cities, United States
Copyright © 2024 Patel, Luo, Zhang and McBride. This is an open-access article distributed under the terms of the Creative Commons Attribution License (CC BY). The use, distribution or reproduction in other forums is permitted, provided the original author(s) and the copyright owner(s) are credited and that the original publication in this journal is cited, in accordance with accepted academic practice. No use, distribution or reproduction is permitted which does not comply with these terms.
*Correspondence: Jere W. McBride, jemcbrid@utmb.edu