- 1College of Animal Science and Veterinary Medicine, Henan Institute of Science and Technology, Xinxiang, China
- 2Key Lab of Agricultural Animal Genetics, Breeding and Reproduction of Ministry of Education, Huazhong Agricultural University, Wuhan, China
Mastitis is a disease with frequent incidence in dairy cows, causing huge financial losses to the dairy industry globally. The identification of certain biomarkers is crucial for the early diagnosis and management of mastitis. Metabolomics technology is a useful tool to accurately and efficiently analyze the changes of metabolites in biofluids in response to internal and external stimulations. Milk is the secreted by udder, and milk metabolites can directly reflect whether the udder are in the healthy or diseased state. The milk metabolomics analysis of mastitis can reveal the physiological and pathological changes of mammary gland and screen the related biomarkers, so as to offer useful reference for the prediction, diagnosis, and management of mastitis. Therefore, the aim of the present study was to comprehensively summarize milk metabolic change caused by naturally occurring or experimentally induced mastitis in dairy cows. In addition, comparative analysis and enrichment analysis were conducted to further discover potential biomarkers of mastitis and to identify the relevant pathways differentiating the healthy and mastitic cows. Multiple milk metabolites were identified to be altered during mastitis based on different metabolomics platforms. It was noteworthy that there were 28 metabolites not only identified by at least two different studies, but also showed consistent change tendency among the different studies. By comparison with literature, we further identified 12 milk metabolites, including acetate, arginine, β-hydroxybutyrate, carnitine, citrate, isoleucine, lactate, leucine, phenylalanine, proline, riboflavin, and valine that were linked with the occurrence of mastitis, which suggested that these 12 milk metabolites could be potential biomarkers of mastitis in dairy cows. Several pathways were revealed to explain the mechanisms of the variation of milk metabolites caused by mastitis, such as phenylalanine, tyrosine and tryptophan biosynthesis, arginine and proline metabolism, riboflavin metabolism, and tricarboxylic acid (TCA) cycle. These results offer a further understanding for the alteration of milk metabolites caused by mastitis, which have a potential significance in the development of more reliable biomarkers for mastitic diagnosis in dairy cows.
1 Introduction
Mastitis, a mammary gland inflammation primarily caused by the co-infection of various pathogenic microorganisms, is a disease with frequent incidence in dairy cows. Generally, the incidence of bovine mastitis is 25%−60% globally (1, 2). Due to the decline in milk quality and production, mastitis has a negative economic effect on the dairy industry (3). The global economic losses from bovine mastitis is estimated at 35 billion dollars annually (4, 5). Even worse, mastitis is thought to be the main cause of overusing antibiotic, with many potential risks to consumers. Therefore, accurate diagnosis of mastitis and then effective treatment are crucial to the dairy industry.
The gold standard to diagnose bovine mastitis is bacteriological culturing, which aims to isolate the pathogens from infected milk samples (6). This method, however, has the disadvantage of being time-consuming. Because it entails plating milk samples on solid medium culture plates, incubating, assessing bacteria growth, and applying biochemical tests, which takes 2 to 7 days before results are available. Somatic cell count (SCC) can provide a clear numerical foundation for mastitic diagnosis. Multitudinous non-infectious factors such as parity, days in milk, and seasonal variations can also affect SCC, although these effects are always minimal (7). Therefore, additional effective diagnostic methods are desirable for bovine mastitis.
As an emerging omics technique, metabolomics is considered as the most comprehensive representation of an organism's physiological and biochemical status, and has been successfully used to investigate potential metabolite biomarkers in biofluids (8, 9). Milk is the secretion of udder, and changes of milk metabolic profiles directly reflects the mammary gland's healthy or diseased status in dairy cows (10). Furthermore, milk is available daily and milk collection is less harmful to dairy cows, and it is conductive to develop an in-line and non-invasive diagnosis technology by milk metabolomics detection for screening biomarkers. Therefore, milk metabolites are potential of interest as indicators for diagnosing mastitis in dairy cows, and many researchers put their eyes on the milk metabolic alterations by mastitis.
Currently, numerous differentially expressed milk metabolites have been identified between healthy and mastitic dairy cows. However, biomarkers that can be used for accurate diagnosis of mastitis are yet to be established. Moreover, little is known about the mechanisms by which mastitis alter milk metabolites. To address these issues, the primary objective of this study was to comprehensively review milk metabolites that were altered due to bovine mastitis. Furthermore, comparative analysis and then enrichment analysis were performed to discover potential biomarkers and to characterize relevant metabolic pathways of milk metabolic alterations caused by mastitis. The present study is crucial for further screening out reliable biomarkers that may facilitate the diagnosis of mastitis in dairy cows.
2 Differentially expressed milk metabolites between healthy and mastitic dairy cows
To date, numerous differentially expressed milk metabolites including amino acids, carbohydrates and derivates, nucleotides and analogs, nucleosides, and organic acid and derivates have been screened out between healthy and mastitic dairy cows. There are 12 previous studies that focus on identifying the differentially expressed milk metabolites according to the different definition of mastitis.
2.1 Differentially expressed milk metabolites identified by definition of mastitis by typical inflammation signs
Clinical mastitis is often characterized by a range of typical inflammation signs such as swelling and redness of udder, along with increased SCC. Using ultra-performance liquid chromatography-quadrupole-time of flight mass spectrometry, Xi et al. (11) detected 91 differential milk metabolites between healthy and mastitic cows classifying based on presentation of clinical signs and SCC. Using liquid chromatography-mass spectrometry (LC-MS), Wang et al. (12) uncovered 673 significantly different levels of milk metabolites between healthy and mastitic cows comprehensively judged according to the clinical manifestations of the udder, milk SCC, and the result of California mastitis test (CMT), known as a fast and convenient method to detect milk SCC. Using untargeted nuclear magnetic resonance spectroscopy (1H-NMR), Luangwilai et al. (13) found that acetate, alanine, benzoate, β-hydroxybutyrate, formate, hippurate, histidine, isoleucine, lactate, leucine, N-acetylamino acid, phenylalanine, threonine, valerate, and valine contents in milk significantly increased in mastitic cows selected by the result of CMT. Through 1H-NMR metabolomic approach, Zhu et al. (14) identified 22 milk molecules that were significantly different between healthy and mastitic cows judged based on the clinical manifestations of the udder and the result of CMT.
2.2 Differentially expressed milk metabolites identified by definition of mastitis by types of bacterial pathogens
Mastitis is commonly caused by the invasion of the mammary gland by bacterial pathogens. Hence, pathogens are often detected in mastitic cows' milk (15). Using gas chromatography-mass spectrometry, Hettinga et al. (16) reported that healthy cows' milk contained lower concentrations of volatile metabolites such as acetaldehyde, 2-butanone, butyric acid, 2-methylbutanal, and ethyl acetate compared with mastitic cows caused by coagulase negative staphylococci, Escherichia coli, Staphylococcus aureus, Streptococcus dysgalactiae, and Streptococcus uberis. Moyes et al. (17) showed that free glucose, lactose, and citrate were lower, whereas β-hydroxybutyrate and glucose-6-phosphate were higher when intramammary infection with Escherichia coli compared to pre-infection. Silanikove et al. (18) showed that milk lactic acid, glucose, and glucose-6-phosphate concentrations significantly changed due to mammary gland infected by several bacteria species, such as coagulase negative staphylococci, Escherichia coli, and streptococci. With the metabolomics approach of LC-MS, Thomas et al. (19) demonstrated that the change of milk metabolites by Streptococcus uberis infection were maximal at 81 h post challenge, and a total of 490 metabolites differentially expressed compared to the pre-challenge. Using gas chromatography time-of-flight mass spectrometry, Tong et al. (20) found 22 milk metabolites to be significantly different between the healthy and Streptococcus agalactiae mastitic cows. According to Bochniarz et al. (21), milk levels of kynurenine and tryptophan were significantly lower in mastitic cows due to the infection of algae from Prototheca group, compared to healthy animals.
2.3 Differentially expressed milk metabolites identified by definition of mastitis by SCC
With the increasing herd sizes of modern dairy industry, developing large-scale tools for identification of mastitis is of great interest to ensure adequate management. For this purpose, milk SCC have been globally adopted to manage udder health. By 1H-NMR, Sundekilde et al. (22) found that acetate, β-hydroxybutyrate, butyrate, isoleucine, and lactate significantly increased, while fumarate and hippurate significantly decreased in milk with high SCC (above 7.2 × 105 cells/mL) compared to low SCC (below 1.4 × 104 cells/mL). Most recently, differential somatic cell count (DSCC), which represents the proportion of neutrophils and lymphocytes over total SCC, has been introduced as a novel indicator of under health on a wide scale. Joint DSCC and SCC offer a more comprehensive depiction of under health status, enabling more precise differentiation between healthy and mastitic cows. By 1H-NMR based metabolomics, Bobbo et al. (23) revealed lower concentrations of carnitine, dimethylsulfone, galactose, galactose-1-phosphate, glucose, hippurate, lactose, lecithin, orotate, riboflavin, and succinate, and higher levels of acetate, choline, lactate, phenylalanine, O-acetylcarnitine, valine, and 2-oxoglutarate in milk with high SCC and DSCC (SCC ≥ 200,000 cells/mL and DSCC ≥ 60%) than low SCC and DSCC (SCC <200,000 cells/mL and DSCC <60%).
3 The same milk metabolites identified by different references
Although a lot of differential milk metabolites have been identified, in the present study, the same metabolites identified by different studies are paid much attention to. That is, if a metabolite is identified by one article, when it is also identified by the other articles, then it will be used for further analysis. By comparison analysis, there were 41 milk metabolites that were identified by at least 2 different references. For example, valine was identified by Luangwilai et al. (13), Zhu et al. (14), and Bobbo et al. (23), and acetate was identified by Luangwilai et al. (13), Sundekilde et al. (22), and Bobbo et al. (23).
3.1 The milk metabolites of inconsistent change tendency and possible reasons
Some metabolites showed inconsistent change tendency among the various references. For example, Thomas et al. (19) and Tong et al. (20) reported that the concentration of uridine in milk significantly increase in mastitic cows. Conversely, the significant decrease of milk uridine from mastitic cows was observed in the studies of Xi et al. (11) and Wang et al. (12). There are many reasons to account for this phenomenon. One of the possible reasons is the particular time point of infection over the course of mastitis. For example, compared to pre-challenge, the content of guanine in milk showed significant increase at 57 h post challenge with Streptococcus uberis, however, its concentration significantly decreased at 312 h post challenge (19). Different metabolomics platforms might be another reason to explain the phenomenon that the same milk metabolites showed inconsistent change tendency among different references. For example, in the study of Luangwilai et al. (13), 1H-NMR based metabolomics investigation was applied, whereas the metabolomics technique of LC-MS was used in the study of Wang et al. (12), which might lead to the opposite tendency of threonine between these 2 studies. Zhu et al. (14) judged mastitic cows based on the combination of clinical manifestations of the udder and CMT, whereas Luangwilai et al. (13) determined the presence of mastitis only based on CMT, which is a semiquantitative measure, and its interpretation may be subjective, leading to results that are not so accurate (24). That way, the different definitions of mastitis may contribute to the opposite change tendency of N-acetylglucosamine in these 2 studies. Besides, the differences in dairy cow breeds, number of parity, days in milk, and the varieties in bacterial types causing mastitis may also contribute to the discrepancy in results. For instance, Chinese Holstein cows with 2–4 parity and 150–195 days in milk were used in the study of Zhu et al. (14), while in the study of Bobbo et al. (23), Simmental cows with average 3.8 parities and 219 days in milk were used, which may result in the inconsistent change tendency of 2-oxoglutarate. Dairy cows with Streptococcus uberis mastitis (19) and mastitic dairy cows infected with Streptococcus agalactiae (20) could be one of the possible reasons for the opposite result of asparagine. Mastitis was naturally occurrent in the study of Wang et al. (12), whereas mastitis was experimentally induced in the study of Thomas et al. (19), which may cause the inconsistent change tendency of nonadecanoic acid in these 2 studies. Table 1 lists the information of tendency and fold change of these milk metabolites between healthy and mastitic cows, and also comprehensively summarize the possible factors contributing to this phenomenon.
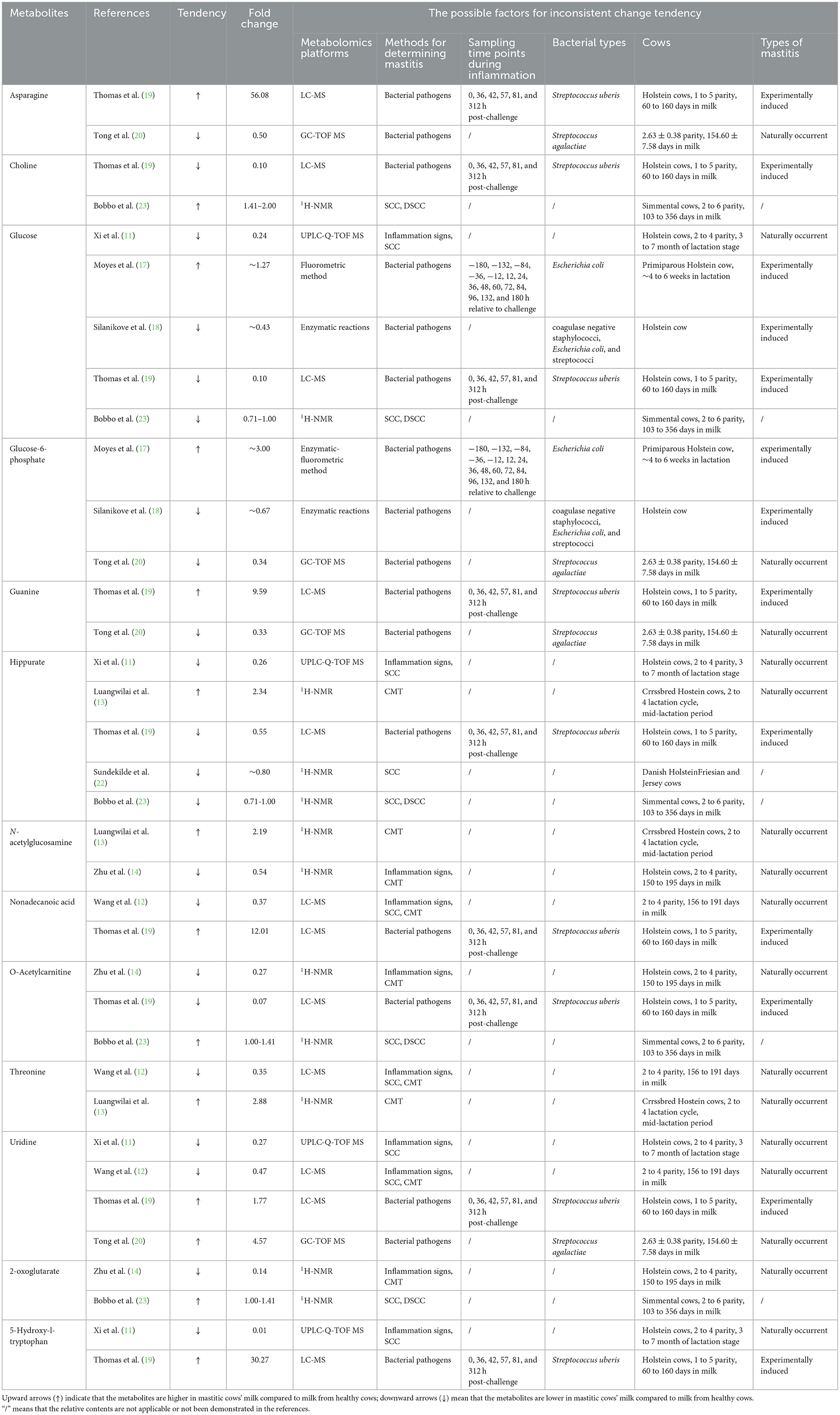
Table 1. The milk metabolites that showed inconsistent change tendency among different references and the possible reasons.
3.2 The milk metabolites of consistent change tendency
Of the aforementioned 41 milk metabolites, there were 28 metabolites that showed consistent change tendency among the various references, including acetate, adenine, arginine, β-hydroxybutyrate, capryloylcholine, carnitine, cis-aconitate, citrate, creatine, creatinine, DL-2-aminooctanoic acid, girgensonine, glycerol, indoleacrylic acid, isoleucine, lactate, leucine, orotate, phenylalanine, piperidine, proline, puromycin, riboflavin, sn-glycero-3-phosphocholine, sn-glycero-3-phosphoethanolamine, valine, 6-Hydroxy-5-methoxyindole glucuronide, and 8-Hydroxy-7-methylguanine. Table 2 lists the information of tendency and fold change of these milk metabolites between healthy and mastitic cows.
All these milk metabolites were only identified by the method of metabolomics. To further illuminate the relationships between these metabolites and mastitis, literature search was conducted, and 12 milk metabolites, including acetate, arginine, β-hydroxybutyrate, carnitine, citrate, isoleucine, lactate, leucine, phenylalanine, proline, riboflavin, and valine were confirmed to be closely associated with immune function and mammary infection.
The correlation between Staphylococcus aureus load and the contents of milk isoleucine and leucine was strong. In addition, a high sensitivity and specificity of these two amino acids, at a threshold of 100 μg/ml, to discriminate Staphylococcus aureus positive and Staphylococcus aureus negative milk samples was observed (25). Staphylococcus aureus requires isoleucine, leucine, and valine to synthesize protein for nutrients requirements, which is vital to Staphylococcus aureus's development and virulence (26). Isoleucine levels affected the expression of genes linked to the capacity of Staphylococcus aureus to cause disease (27). Accoridng to Konashi et al. (28), phenylalanine deficiency in chickens impaired their immunological responses, while dietary supplementation with this amino acid could reverse the problem. In addition, valine, phenylalanine, isoleucine, leucine, and proline in the serum showed high predictive abilities for discriminating the mastitis from healthy cows (1, 29). Carnitine transports long-chain fatty acids from the cytosol to the mitochondrial matrix, which in turn, further regulates the energy metabolism. Early lactation excessive energy deficit impairs immune function, which has been linked to a higher risk of infectious conditions such as mastitis (30). Carnitine and proline in serum pre-calving could correctly classified cows for their future mastitic status (31). Arginine is essential for the maintenance of immune function, and this amino acid exerts anti-inflammatory functions. In primary bovine mammary epithelial cells, arginine supply reduced the proinflammatory responses induced by lipopolysaccharide (LPS) via regulating the amount of proinflammatory cytokines and chemokines as well as the activity of NF-κB (32).
Riboflavin, also known as vitamin B2, has a variety of immune modulatory effects. It has been demonstrated that intramuscular administration of riboflavin in cows stimulates neutrophil function such as phagocytic bactericidal activity and nitroblue tetrazolium reductivity. Besides, riboflavin injection intravenously to cows with high SCC caused a rapid reduction of SCC in udder quarters infected by Staphylococcus aureus (33, 34). Previous studies showed a correlation between blood β-hydroxybutyrate and the likelihood of mastitis in early lactation (35). Increased β-hydroxybutyrate levels are linked to oxidative stress, which impairs the immune system and intensifies the inflammation. Besides, increased β-hydroxybutyrate levels inhibit bovine peripheral blood mononuclear cells, and impair polymorphonuclear leukocytes activity, exposing dairy cows to mastitis in the periparturient phase (36). Recent investigations reveal that β-hydroxybutyrate is metabolized in reaction to immune challenges. According to Gross et al. (37), the level of β-hydroxybutyrate in cows' blood was linked to their metabolic responses to an LPS challenge and to their subsequent recovery of udder health and function. The major end products of carbohydrate metabolism are acetate and lactate, which are created by milk bacteria, or by anaerobic epithelium respiration in an oxygen-deprived environment following mastitis. The presence of bacteria in milk will produce a distinct metabolic fingerprint, expressed as an increase in lactate concentration (22, 23). Citrate is regarded as a biomarker of mitochondrial metabolism in the mammary gland. Citrate is secreted into milk by the mammary epithelial cells, and the concentration of this substance indicates mammary activity (17, 38).
All these evidences confirm the changes of acetate, arginine, β-hydroxybutyrate, carnitine, citrate, isoleucine, lactate, leucine, phenylalanine, proline, riboflavin, and valine are linked to mammary infection, suggesting these 12 milk metabolites may be potential biomarkers for diagnosing mastitis in cows.
4 Metabolic pathways analysis of milk metabolites affected by mastitis
To gain a deeper understanding of how multiple pathways distinguished the healthy and mastitic milk, the 28 metabolites which showed consistent change tendency were used as a basis for a Kyoto Encyclopedia of Genes and Genomes (KEGG) enrichment analysis by using MetaboAnalyst 5.0. Pathway topology analysis with a criterion of p < 0.05 and impactor value >0.1 revealed 4 indicated pathways, namely phenylalanine, tyrosine and tryptophan biosynthesis, arginine and proline metabolism, riboflavin metabolism, and tricarboxylic (TCA) cycle (Table 3).

Table 3. Metabolic pathways revealed by enrichment analysis on the milk metabolites significantly differing the healthy dairy cows from the mastitic ones (p value <0.05 and impact value >0.1).
Phenylalanine is needed to maintain an enough provision of tetrahydrobiopterin to produce nitric oxide (NO) by inducible nitric oxide synthase in activated macrophages and other leukocytes, whereas NO is a pro-inflammatory cytokine with important regulatory functions in the inflammatory process when bacteria invade the mammary gland (39). Arginine is the main amino acid to synthesize creatine in the liver. Since creatine is an intermediary molecule in energy processes, it has significant importance on regulating dairy cows' negative energy balance (40, 41). During the periparturient stage, NEB is a major contributor to oxidative stress, which may impair immune and anti-inflammatory responses. According to a recent study, oxidative stress is the main cause of periparturient diseases in dairy cows, including mastitis (42). Proline is a crucial amino acid that protects lymphocytes from apoptosis, stimulates cell growth, and promotes antibody generation (43). The increase of free amino acids including arginine, proline, and phenylalanine in mastitic milk may be linked to increase of pathogen-specific fermentative processes and protein degradation activities.
It has been discovered that riboflavin increases the non-specific host defense mechanisms in mice against a range of bacterial infections by inducing neutrophil production and improving macrophage activity. In mastitic cows, the riboflavin metabolism may be linked to the improvement of peripheral blood neutrophils' nitroblue tetrazolium reductivity and the activation of host-defense mechanisms against bacterial infection in the mammary gland (33, 34). Neutrophils are the main cells of defense involved in bacterial clearance after mammary gland infection. During the peripartum stage, impairment of neutrophil activity is a key contributor to the high incidence of mastitis, and postpartum mastitis also have been associated with decreased neutrophil function (44). The TCA cycle, which is the fundamental metabolic pathway in mitochondria, is vital for dairy cows' health. By disturbing the TCA cycle in the mammary gland, mastitis alters milk metabolites, and the pathogens are expected to divert these for their own metabolic processes and development as the mastitis advances. The levels of some TCA cycle intermediates such as cis-aconitate and citrate decreased significantly in the mastitic milk, suggesting that the TCA cycle is downregulated in bovine mastitis. Besides, citrate generates a citrate-iron complex that is accessible to bacteria by competing with lactoferrin for iron. The notable decrease of citrate in mastitic cows may suggest that lactoferrin's ability to bind iron has increased, contributing to host defense and limiting availability of iron to bacterial invaders (17, 38).
As a result, our results offer possible molecular mechanisms for the observed variations in milk metabolomes between the healthy and mastitic dairy cows.
5 Conclusions
In dairy cows, mastitis is a complicated and serious syndrome causing large financial losses in the dairy industry. Thus, the development of diagnostic biomarkers for mastitis is highly desired. This study conducted a comprehensive overview of the application of metabolomics technology on screening the milk metabolic change in response to mastitis. Numerous metabolites in milk were altered due to mastitis. By comparative analysis, we found 28 metabolites with consistent change tendency among the different references. These metabolites were mainly involved in phenylalanine, tyrosine and tryptophan biosynthesis, arginine and proline metabolism, riboflavin metabolism, and TCA cycle. By further comparison with literature, several metabolites including acetate, arginine, β-hydroxybutyrate, carnitine, citrate, isoleucine, lactate, leucine, phenylalanine, proline, riboflavin, and valine were confirmed to be involved in inflammation. Therefore, the present study highlights that these 12 milk metabolites may be used as biomarkers to diagnose mastitis in dairy cows (Figure 1). It merits further experiments to investigate how useful of these metabolites as biomarkers for mastitis diagnosis, and also its biochemical and physiological mechanisms in the occurrence of mastitis.
As is well documented that various bacterial species that cause mastitis may influence a distinct metabolomic profile. However, the present study only generally summarized the milk metabolites alteration response to mastitis, and did not classify according to the types of bacterial pathogens. In the future, the study of milk metabolomics should focus more on the basis of etiologic agent, which will help for identifying biomarkers of mastitis against the specific bacterial types.
Author contributions
CD: Writing – original draft. XZhao: Data curation, Writing – review & editing. SZ: Writing – review & editing, Supervision, Visualization. CC: Writing – review & editing, Data curation. XZhang: Writing – review & editing, Supervision. ZT: Supervision, Writing – review & editing.
Funding
The author(s) declare financial support was received for the research, authorship, and/or publication of this article. This work were funded by the national natural science foundation of China (32302798) and Key R&D and extension projects (scientific and technological project) of Henan Province (232102110082 and 232102111049).
Conflict of interest
The authors declare that the research was conducted in the absence of any commercial or financial relationships that could be construed as a potential conflict of interest.
Publisher's note
All claims expressed in this article are solely those of the authors and do not necessarily represent those of their affiliated organizations, or those of the publisher, the editors and the reviewers. Any product that may be evaluated in this article, or claim that may be made by its manufacturer, is not guaranteed or endorsed by the publisher.
References
1. Dervishi E, Zhang G, Dunn SM, Mandal R, Wishart DS, Ametaj BN, et al. Metabolomics identifies metabolite alterations that precede subclinical mastitis in the blood of transition dairy cows. J Proteome Res. (2017) 16:433–46. doi: 10.1021/acs.jproteome.6b00538
2. Ruegg PL. A 100-Year Review: mastitis detection, management, and prevention. J Dairy Sci. (2017) 100:10381–97. doi: 10.3168/jds.2017-13023
3. Hu H, Fang Z, Mu T, Wang Z, Ma Y, Ma Y, et al. Application of metabolomics in diagnosis of cow mastitis: a review. Front Vet Sci. (2021) 8:747519. doi: 10.3389/fvets.2021.747519
4. Petersson-Wolfe CS, Leslie KE, Swartz TH. An update on the effect of clinical mastitis on the welfare of dairy cows and potential therapies. Vet Clin North Am Food Anim Pract. (2018) 34:525–35. doi: 10.1016/j.cvfa.2018.07.006
5. Turk R, Rošić N, Kuleš J, Horvatić A, Gelemanovic A, Galen A, et al. Milk and serum proteomes in subclinical and clinical mastitis in Simmental cows. J Proteomics. (2021) 244:104277. doi: 10.1016/j.jprot.2021.104277
6. Ashraf A, Imran M. Diagnosis of bovine mastitis: from laboratory to farm. Trop Anim Health Prod. (2018) 50:1193–202. doi: 10.1007/s11250-018-1629-0
7. Sumon SMMR, Parvin MS, Ehsan MA, Islam MT. Dynamics of somatic cell count and intramammary infection in lactating dairy cows. J Adv Vet Anim Res. (2020) 7:314–9. doi: 10.5455/javar.2020.g423
8. Zhu C, Zhang Q, Zhao X, Yang Z, Yang F, Yang Y, et al. Metabolomic analysis of multiple biological specimens (feces, serum, and urine) by 1H-NMR spectroscopy from dairy cows with clinical mastitis. Animals. (2023) 13:741. doi: 10.3390/ani13040741
9. Yue S, Ding S, Zhou J, Yang C, Hu X, Zhao X, et al. Metabolomics approach explore diagnostic biomarkers and metabolic changes in heat-stressed dairy cows. Animals. (2020) 10:1741. doi: 10.3390/ani10101741
10. Wang Y, Nan X, Zhao Y, Jiang L, Wang H, Zhang F, et al. Consumption of supplementary inulin modulates milk microbiota and metabolites in dairy cows with subclinical mastitis. Appl Environ Microbiol. (2022) 88:e0205921. doi: 10.1128/aem.02059-21
11. Xi X, Kwok LY, Wang Y, Ma C, Mi Z, Zhang H. Ultra-performance liquid chromatography-quadrupole-time of flight mass spectrometry MSE-based untargeted milk metabolomics in dairy cows with subclinical or clinical mastitis. J Dairy Sci. (2017) 100:4884–96. doi: 10.3168/jds.2016-11939
12. Wang Y, Nan X, Zhao Y, Wang H, Wang M, Jiang L, et al. Coupling 16S rDNA sequencing and untargeted mass spectrometry for milk microbial composition and metabolites from dairy cows with clinical and subclinical mastitis. J Agric Food Chem. (2020) 68:8496–508. doi: 10.1021/acs.jafc.0c03738
13. Luangwilai M, Duangmal K, Chantaprasarn N, Settachaimongkon S. Comparative metabolite profiling of raw milk from subclinical and clinical mastitis cows using 1H-NMR combined with chemometric analysis. Int J Food Sci Tech. (2021) 56:493–503. doi: 10.1111/ijfs.14665
14. Zhu C, Tang K, Lu X, Tang J, Laghi L. An untargeted metabolomics investigation of milk from dairy cows with clinical mastitis by 1H-NMR. Foods. (2021) 10:1707. doi: 10.3390/foods10081707
15. Wall SK, Wellnitz O, Bruckmaier RM, Schwarz D. Differential somatic cell count in milk before, during, and after lipopolysaccharide- and lipoteichoic-acid-induced mastitis in dairy cows. J Dairy Sci. (2018) 101:5362–73. doi: 10.3168/jds.2017-14152
16. Hettinga KA, van Valenberg HJ, Lam TJ, van Hooijdonk AC. Detection of mastitis pathogens by analysis of volatile bacterial metabolites. J Dairy Sci. (2008) 91:3834–9. doi: 10.3168/jds.2007-0941
17. Moyes KM, Larsen T, Sørensen P, Ingvartsen KL. Changes in various metabolic parameters in blood and milk during experimental Escherichia coli mastitis for primiparous Holstein dairy cows during early lactation. J Anim Sci Biotechnol. (2014) 5:47. doi: 10.1186/2049-1891-5-47
18. Silanikove N, Merin U, Shapiro F, Leitner G. Milk metabolites as indicators of mammary gland functions and milk quality. J Dairy Res. (2014) 81:358–63. doi: 10.1017/S0022029914000260
19. Thomas FC, Mudaliar M, Tassi R, McNeilly TN, Burchmore R, Burgess K, et al. Mastitomics, the integrated omics of bovine milk in an experimental model of Streptococcus uberis mastitis: 3. Untargeted metabolomics Mol Biosyst. (2016) 12:2762–9. doi: 10.1039/C6MB00289G
20. Tong J, Zhang H, Zhang Y, Xiong B, Jiang L. Microbiome and metabolome analyses of milk from dairy cows with subclinical Streptococcus agalactiae mastitis-potential biomarkers. Front Microbiol. (2019) 10:2547. doi: 10.3389/fmicb.2019.02547
21. Bochniarz M, Piech T, Kocki T, Iskra M, Krukowski H, Jagielski T. Tryptophan, kynurenine and kynurenic acid concentrations in milk and serum of dairy cows with Prototheca mastitis. Animals. (2021) 11:3608. doi: 10.3390/ani11123608
22. Sundekilde UK, Poulsen NA, Larsen LB, Bertram HC. Nuclear magnetic resonance metabonomics reveals strong association between milk metabolites and somatic cell count in bovine milk. J Dairy Sci. (2013) 96:290–9. doi: 10.3168/jds.2012-5819
23. Bobbo T, Meoni G, Niero G, Tenori L, Luchinat C, Cassandro M, et al. Nuclear magnetic resonance spectroscopy to investigate the association between milk metabolites and udder quarter health status in dairy cows. J Dairy Sci. (2022) 105:535–48. doi: 10.3168/jds.2021-20906
24. Wollowski L, Heuwieser W, Kossatz A, Addis MF, Puggioni GMG, Meriaux L, et al. The value of the biomarkers cathelicidin, milk amyloid A, and haptoglobin to diagnose and classify clinical and subclinical mastitis. J Dairy Sci. (2021) 104:2106–22. doi: 10.3168/jds.2020-18539
25. Grispoldi L, Karama M, Ianni F, La Mantia A, Pucciarini L, Camaioni E, et al. The relationship between S. aureus and branched-chain amino acids content in composite cow milk. Animals. (2019) 9:981. doi: 10.3390/ani9110981
26. Kaiser JC, Sen S, Sinha A, Wilkinson BJ, Heinrichs DE. The role of two branched-chain amino acid transporters in Staphylococcus aureus growth, membrane fatty acid composition and virulence. Mol Microbiol. (2016) 102:850–64. doi: 10.1111/mmi.13495
27. Kaiser JC, King AN, Grigg JC, Sheldon JR, Edgell DR, Murphy MEP, et al. Repression of branched-chain amino acid synthesis in Staphylococcus aureus is mediated by isoleucine via CodY, and by a leucine-rich attenuator peptide. PLoS Genet. (2018) 14:e1007159. doi: 10.1371/journal.pgen.1007159
28. Konashi S, Takahashi K, Akiba Y. Effects of dietary essential amino acid deficiencies on immunological variables in broiler chickens. Br J Nutr. (2000) 83:449–56.
29. Zhang G, Tobolski D, Zwierzchowski G, Mandal R, Wishart DS, Ametaj BN. Identification of serum-predictive biomarkers for subclinical mastitis in dairy cows and new insights into the pathobiology of the disease. J Agric Food Chem. (2022) 70:1724–46. doi: 10.1021/acs.jafc.1c07281
30. Bach KD, Barbano DM, McArt JAA. The relationship of excessive energy deficit with milk somatic cell score and clinical mastitis. J Dairy Sci. (2021) 104:715–27. doi: 10.3168/jds.2020-18432
31. Zandkarimi F, Vanegas J, Fern X, Maier CS, Bobe G. Metabotypes with elevated protein and lipid catabolism and inflammation precede clinical mastitis in prepartal transition dairy cows. J Dairy Sci. (2018) 101:5531–48. doi: 10.3168/jds.2017-13977
32. Dai H, Coleman DN, Hu L, Martinez-Cortés I, Wang M, Parys C, et al. Methionine and arginine supplementation alter inflammatory and oxidative stress responses during lipopolysaccharide challenge in bovine mammary epithelial cells in vitro. J Dairy Sci. (2020) 103:676–89. doi: 10.3168/jds.2019-16631
33. Osame S, Araki S, Kimura M. Effects of vitamin B2 on neutrophil functions in cattle. J Vet Med Sci. (1995) 57:493–5. doi: 10.1292/jvms.57.493
34. Sato S, Hori H, Okada K. Effect of vitamin B2 on somatic cell counts in milk of clinical Staphylococcus aureus mastitis. J Vet Med Sci. (1999) 61:569–71. doi: 10.1292/jvms.61.569
35. Bouvier-Muller J, Allain C, Tabouret G, Enjalbert F, Portes D, Noirot C, et al. Whole blood transcriptome analysis reveals potential competition in metabolic pathways between negative energy balance and response to inflammatory challenge. Sci Rep. (2017) 7:2379. doi: 10.1038/s41598-017-02391-y
36. Khan MZ, Ma Y, Xiao J, Chen T, Ma J, Liu S, et al. Role of selenium and vitamins E and B9 in the alleviation of bovine mastitis during the periparturient period. Antioxidants. (2022) 11:657. doi: 10.3390/antiox11040657
37. Gross JJ, Grossen-Rösti L, Wall SK, Wellnitz O, Bruckmaier RM. Metabolic status is associated with the recovery of milk somatic cell count and milk secretion after lipopolysaccharide-induced mastitis in dairy cows. J Dairy Sci. (2020) 103:5604–15. doi: 10.3168/jds.2019-18032
38. Hyvönen P, Haarahiltunen T, Lehtolainen T, Heikkinen J, Isomäki R, Pyörälä S. Concentrations of bovine lactoferrin and citrate in milk during experimental endotoxin mastitis in early-versus late-lactating dairy cows. J Dairy Res. (2010) 77:474–80. doi: 10.1017/S0022029910000579
39. Srichok J, Yingbun N, Kowawisetsut T, Kornmatitsuk S, Suttisansanee U, Temviriyanukul P, et al. Synergistic antibacterial and anti-inflammatory activities of Ocimum tenuiflorum Ethanolic extract against major bacterial mastitis pathogens. Antibiotics. (2022) 11:510. doi: 10.3390/antibiotics11040510
40. Poortmans JR, Kumps A, Duez P, Fofonka A, Carpentier A, Francaux M. Effect of oral creatine supplementation on urinary methylamine, formaldehyde, and formate. Med Sci Sports Exerc. (2005) 37:1717–20. doi: 10.1249/01.mss.0000176398.64189.e6
41. Wang Y, Yang G, Cheng X, Zhang H, Qian W, Yu C. Pathway analysis of plasma different metabolites for dairy cow ketosis. Ital J Anim Sci. (2016) 15:1–7. doi: 10.1080/1828051X.2016.1180643
42. Ayemele AG, Tilahun M, Lingling S, Elsaadawy SA, Guo Z, Zhao G, et al. Oxidative Stress in Dairy Cows: Insights into the Mechanistic Mode of Actions and Mitigating Strategies. Antioxidants. (2021) 10:1918. doi: 10.3390/antiox10121918
43. Duval D, Demangel C, Munier-Jolain K, Miossec S, Geahel I. Factors controlling cell proliferation and antibody production in mouse hybridoma cells: I. Influence of the amino acid supply. Biotechnol Bioeng. (1991) 38:561–70. doi: 10.1002/bit.260380602
Keywords: mastitis, dairy cows, milk, metabolites, pathway analysis
Citation: Du C, Zhao X, Zhang S, Chu C, Zhang X and Teng Z (2024) Milk metabolite profiling of dairy cows as influenced by mastitis. Front. Vet. Sci. 11:1475397. doi: 10.3389/fvets.2024.1475397
Received: 03 August 2024; Accepted: 22 October 2024;
Published: 13 November 2024.
Edited by:
Michael Kogut, United States Department of Agriculture, United StatesReviewed by:
Shu-Cheng Huang, Henan Agricultural University, ChinaJie Cai, Zhejiang University, China
Copyright © 2024 Du, Zhao, Zhang, Chu, Zhang and Teng. This is an open-access article distributed under the terms of the Creative Commons Attribution License (CC BY). The use, distribution or reproduction in other forums is permitted, provided the original author(s) and the copyright owner(s) are credited and that the original publication in this journal is cited, in accordance with accepted academic practice. No use, distribution or reproduction is permitted which does not comply with these terms.
*Correspondence: Xiaojian Zhang, enhqOTEwOUBoaXN0LmVkdS5jbg==; Zhanwei Teng, dGVuZ3poYW53ZWlAaGlzdC5lZHUuY24=