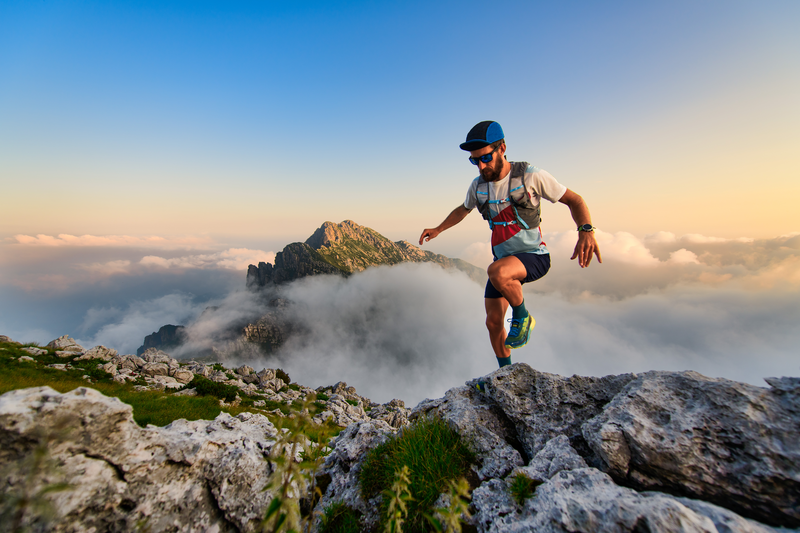
94% of researchers rate our articles as excellent or good
Learn more about the work of our research integrity team to safeguard the quality of each article we publish.
Find out more
ORIGINAL RESEARCH article
Front. Vet. Sci. , 07 November 2024
Sec. Animal Nutrition and Metabolism
Volume 11 - 2024 | https://doi.org/10.3389/fvets.2024.1474942
This article is part of the Research Topic Mitigating Pathogen Load and AMR in Animals via Prebiotics and Probiotics View all 5 articles
Necrotic enteritis caused by Clostridium perfringens (C. perfringens) is characterized by poor performance and higher mortality rates in poultry farms. Novel dietary intervention involving bioactive molecules loaded into smart magnetized nano-system with a potent antioxidant function (quercetin-loaded Fe3O4-NPs), was evaluated for their impact on growth performance, intestinal immune and antioxidant defenses, and resistance against Clostridium perfringens in a necrotic enteritis challenge model. Four experimental groups comprising a total of 200 one-day-old Ross 308 broiler chickens were fed different diets: a control basal diet, a diet supplemented with quercetin (300 mg/kg), a diet with Fe3O4-NPs (60 mg/kg), and a diet with quercetin-loaded Fe3O4-NPs (300 mg/kg). These groups were then challenged with C. perfringens during the grower period. Dietary inclusion of quercetin-loaded Fe3O4-NPs prominently reduced C. perfringens colonization and its associated virulence genes expression, which subsequently restored the impaired growth performance and intestinal histopathological changes in challenged broilers. Quercetin-loaded Fe3O4-NPs supplemented group displayed higher Lactobacillus and Bifidobacterium counts, upregulation of intestinal host defense antimicrobial peptides related genes (avian β-defensin 6 and 12) and downregulation of intestinal inflammatory regulated genes (Interleukin-1 beta, C-X-C motif chemokine ligand 8, tumor necrosis factor-α, chemokine C–C motif ligand 20, inducible nitric oxide synthase and cycloox-ygenase-2). Intestinal redox balance was boosted via upregulation of catalase, superoxide dismutase, glutathione peroxidase and heme Oxygenase 1 genes along with simultaneous decrease in hydrogen peroxide, reactive oxygen species and malondialdehyde contents in groups fed quercetin-loaded Fe3O4-NPs. Overall, new nutritional intervention with quercetin-loaded Fe3O4-NPs impacted better immune and antioxidant defenses, attenuated C. perfringens induced necrotic enteritis and contributed to better performance in the challenged birds.
Clostridium perfringens (C. perfringens) is part of the normal gut flora in poultry; however, multiple factors including contaminated environment, food contents and overcrowding can favor its intestinal colonization and lead to the bacterial overgrowth (1). Therefore, they could become pathogenic causing NE ranging from subclinical to devastating outbreaks. Necrotic enteritis caused by C. perfringens significantly impacts profitability of poultry industry, even in its subclinical form. The subclinical form is characterized by damage to the intestinal mucosal barriers, leading to impaired feed efficiency and growth performance (2). In contrast, the clinical form results in alterations in tight junction proteins, severe gastroenteritis with microbiota dysbiosis, and diarrhea, often associated with high mortality rates in birds (3). C. perfringens enteric infection gained public health concern for humans, not only as a foodborne infection transmitted through chicken meat consumption (1), but also due to the increase in its incidence because of eliminating antibiotics in poultry feed, which is aimed at combating the emergence of antibiotic-resistant bacteria (4–6). The alternative natural products from herbal, essential oils, organic acids, prebiotics and probiotics, have garnered have garnered significant research interest in the poultry industry as growth promoters and substitutes for antibiotics (7–11). However, their exact roles in driving a protective host immunity and resistance against pathogenic burdens still need to be explicitly defined (7, 12–14). Quercetin (2-(3,4-dihydroxyphenyl)-3,5,7-trihydroxy-4H-chromen-4-one) is one of the flavonoid components that can be easily obtained from natural sources like apple, broccoli, onion, pea nut and red onion etc. Several studies have highlighted its antibacterial, anti-inflammatory and antioxidant activities (15). Similarly, dietary inclusion of quercetin improved growth performance and nutrient digestibility of broiler chickens (16). Additionally, study involving broiler chickens fed diets supplemented with quercetin at doses of 0.5 and 1 g/kg of feed revealed that, although there was no increase, in the feed intake, there was a cumulative increase in feed conversion ratio (FCR) in a dose dependent manner. The study also noted an increased heart weight-to-body weight ratio and a decrease in lipid oxidation rate. The study also demonstrated improved antioxidative stability of breast meat, leading to an increased shelf life during storage (17).
It has been demonstrated that quercetin ameliorates the intestinal inflammation induced by lipopolysaccharides (LPS) in laying hens. This mitigating effect was attributed to quercetin’s immunomodulatory properties, which restore the gut microbial community and improve intestinal barrier function. The data revealed that animals fed diets supplemented with quercetin showed upregulation in the relative mRNA expression levels of tight junction associated genes, such as claudin-1 and occludin in both jejunum and ileum. Although feeding quercetin did not significantly affect TNF-α expression, it resulted in upregulation of IL-4 gene expression and downregulation of proinflammatory-related genes in the quercetin-supplemented group. There was a difference in the relative mRNA expression of the inflammatory signature between ileum and jejunum. This variation may be attributed to differences in quercetin bioavailability and absorption in different parts of the intestine (18). The uncontrolled use of antibiotics as feed additives has been reported to not only cause the emergence of antibiotics resistance bacteria, but also the presence of drug residues in meat products, which can affect human health (19). Therefore, iron oxide nanoparticles have been investigated as a source of iron in poultry feed due to their high bioavailability, which helps to reduce the cost compared to conventional inorganic sources (20). In addition to their low toxicity, iron oxide nanoparticles, particularly those that are biologically synthesized, offer further advantages (21). The antimicrobial activity of iron oxide nanoparticles against Staphylococcus aureus and Pseudomonas aeruginosa isolated from poultry feed has been reported (21). The promising antimicrobial effects of iron oxide and its role as an iron source will reduce the cost of using commercial feed additives. However, the precise mechanism by which iron oxide enhances resistance to C. perfringens infection in broiler chickens has yet to be fully explored. Therefore, our study aimed to introduce new nanoliposomes formula combining iron and quercetin as feed additive in broilers’ diets with the goal of alleviating inflammatory necrotic enteritis (NE) caused by C. perfringens. The study showed whether this ameliorative effect could be associated with a promotion of poultry’s performance, immunity, barrier defense, antioxidant system or restore gut microbiota. Such effects could, in turn, prevent systemic inflammation and promote antioxidant stability in broiler meat.
The co-precipitation method was used to prepare magnetite (Fe3O4) nanoparticles (22). Briefly, 500 g of iron salt solution containing FeCl2.4H2O (30 mmoL, Oxford, India), and FeCl3.6H2O (60 mmoL, Oxford, India) was prepared and mixed in an oxygen-free reactor and then, NaOH solution (0.8 M, Sigma-Aldrich, Germany) was added drop wise to the prepared iron salt solution. A brownish black precipitate was formed indicating Fe3O4 NPs formation. The powder was collected by centrifuging, washed with deionized water, and dried in oven at 80°C for 3 h.
To prepare quercetin-loaded Fe3O4 NPs emulsion, the emulsification-solvent technique (O/W) using a probe ultrasonicator was employed. First, 0.006 gm of the previously Fe3O4 NPs was dissolved in 20 mL of absolute ethanol (Merck, Germany NaOH, AppliChem, Panreac, ITW companies, Germany). The mixture was sonicated for 1 h in water path sonicator. Then, 0.02 gm of quercetin powder (5 mg/mL, Sigma-Aldrich, Missouri, United States), was added to 4 mL of the previously prepared Fe3O4 NPs in ethanol solution (oil phase). The resulting solution was added dropwise to 100 mL of deionized water in a clean conical flask drop by drop using a medical syringe (5 mL) over 5 min. The mixture was stirred at 1000 rpm to ensure proper mixing. The morphology of the newly formulated (quercetin-loaded Fe3O4-NPs) was carried out using transmission electron microscopy (JOEL JEM-2010) operated with a 200 kV coupled acceleration voltage to the Gatan Erlangshen ES500 digital camera model (Figures 1A,B). The surface charging behavior in contact with water-based electrolytes was assessed by measuring the zeta potential of the prepared sample using a Malvern Zetasizer (Nano ZS-90) set to 25°C (Figure 1C). High-resolution transmission electron microscopy (HR-TEM) analysis revealed that the quercetin-loaded Fe₃O₄ nanoparticles (NPs) have wire-like structures with diameters ranging from 50 to 90 nm and lengths extending into the micrometer range. Additionally, agglomeration of spherical-shaped nanoparticles with sizes less than 50 nm was observed due to the Fe3O4 NPs. The prepared nanoemulsion exhibited a negative zeta potential of −16 ± 11 mV (Figure 1C), which is sufficient to prevent nanoparticle aggregation.
Figure 1. Transmission electron microscopy images (A,B) from different spots of the newly formulated quercetin-loaded magnetite (quercetin-loaded; Fe3O4-NPs), and C, its zeta potential.
A total of 200 one-day-old broiler chickens (Ross 308) was purchased from the commercial poultry hatchery. Birds were weighed before beginning the experimental process and divided into 4 separate groups (n = 50/group). The four groups were assigned as following; G1: supplemented with conventional basal diet defined as control group, G2: supplemented with basal diet containing Fe3O4-NPs alone (60 mg/kg diet), G3: supplemented with basal diet containing quercetin alone (300 mg/ kg diet) and G4: supplemented with basal diet containing the formulated Fe3O4-NPs loaded quercetin (300 mg/kg diet), with the iron concentration being 60 mg in the prepared nano mixture. Antibiotic free diets for starter, grower and finisher periods were prepared as stated in Table 1. All birds continued to receive these formulated diets during the whole study period (38 days) and they were raised according to Ross Broiler Management Guide (23). They were housed in hygienic conditions and had unlimited access to water and food during the study period. According to the Association of Official Analytical Chemists (24), all formulated diets underwent chemical analysis for moisture, crude protein, ether extract and crude fiber. All groups were orally challenged with 1×108 colony forming units (CFUs) of C. perfringens strain for three successive days starting at 21st day of the experimental period.
A field-isolated C. perfringens strain from NE cases from commercial broiler chickens flock in Sharkia Governorate was used in the challenge model. This strain was phenotypically identified according to conventional microbiological techniques and confirmed to be type A using a PCR assay (19). The strain was preserved at −80°C until use. Prior to injection, it was cultured on tryptose sulfite cycloserine agar (Oxoid, Basingstoke, United Kingdom) plates at 37°C for 18 h. A bacterial suspension of 1 × 108 CFU/mL was then prepared and used for oral gavage of each bird at the time of the challenge, following the described experimental protocol.
Body weight (BW) and feed intake (FI) were measured on the first day and at the end of the study period to evaluate the body weight gain (BWG), FCR [feed intake(g/bird)/weight gain (g/bird)] as previously mentioned (10) Moreover, clinical signs and mortality rates were recorded throughout the experimental period. Deceased birds were necropsied and scored for NE associated intestinal gross lesions in line with the criteria established earlier (19).
Quantification of Lactobacillus and Bifidobacterium species and Enterobacteriaceae populations at the end of experimental period and that of C. perfringens at 7 and 14 days post challenge were performed using quantitative real-time PCR (RT-PCR) technique. Briefly, DNA was extracted from intestinal contents via commercial QIAamp DNA stool kit (Qiagen, Germany) consistent with the manufacturer’s endorsements and then DNA concentration was measured via NanoDropTM 2000 spectrophotometer (Thermo Fisher Scientific Inc., United States). After that, C. perfringens populations were enumerated, in triplicates, utilizing SYBR-Green based RT-PCR method carried out on Stratagene MX3005P RT-PCR instrument via SYBR Premix Ex Taq™ kit (TaKaRa, Japan). For generating standard curves used for RT-PCR analysis, 10-fold serial dilutions of DNA extracted from pure cultures of the investigated bacterial species were performed. The copies of examined bacterial species were estimated in terms of log10 CFU per gram of the analyzed sample. The primer sequences used for quantification of bacterial species are mentioned in Table 2.
Intestinal and breast meat specimens were collected and frozen in liquid nitrogen for evaluation of antimicrobial peptide defense, inflammatory response, antioxidant status and C. perfringens virulence associated genes expression using qRT-PCR techniques. RNA isolation was performed along with the manufacturer’s directions using QIAamp RNeasy Mini kit (Qiagen GmbH, Hilden, Germany) and then RNA concentration and purification were assessed using Nanodrops 2000 spectrophotometer (Thermo Fisher Scientific Inc., Waltham, MA, United States) at optical densities of 260 and 280 nm. One-step qRT-PCR was performed as per the manufacture’s guidelines of Stratagene™ Mx3005P real-time quantitative polymerase chain reaction system (Thermo Fisher Scientific, Waltham, MA, United States) and SYBR Green based real-time PCR Kit (Qiagen, Hilden, Germany). The primer sequences used for all qRT-PCR assays are stated in Table 2.
Meat samples from all groups were collected at the end of the experimental period (38 days) for evaluation of oxidative traits of breast meat including malondialdehyde (MDA), total antioxidant capacity (T-AOC), reactive oxygen species (ROS) and hydrogen peroxide (H2O2). The reduction of H2O2 concentration was performed at 25°C at 240 nm using Halo DB-2O double beam spectrophotometer (Dynamica, Australia). The MDA as a secondary lipid oxidation product formed by hydrolysis of lipid hydroperoxides given in nMoL/g of meat tissues was measured as previously mentioned (17). In addition, meat filtrates were used for measurement of T-AOC according to manufacturer’s instruction of commercial assay kits (Sigma-Aldrich, MAK187).
Blood samples from the wing vein were collected from each replicate in two sections. One on heparinized tube to prevent clotting for estimating red blood cells (RBCs) count, hemoglobin (Hb) concentration and isolation of peripheral blood mononuclear cells for evaluating phagocytosis and killing assay. The second section was collected in a clot activator vacutainer tube to allow clotting and centrifuged for 10 min at 3,000 rpm for separation of serum for evaluation of lysozyme activity and nitric oxide production.
The count of RBCs was assessed via a Neubauer hemocytometer (Sigma-Aldrich, Germany) and Hb concentrations were calculated using the cyanomethemoglobin colorimeteric method.
For evaluation of phagocytosis and candidacidal activity, the peripheral blood mononuclear cells were separated by a modified method Sun et al. (25). Briefly, 2 mL of heparinized blood was diluted 1:1 with 1X of phosphate-buffered saline (PBS), the diluted blood was layered on the surface of 2 mL of Histopaque 1,083 (Sigma-Aldrich) and then centrifuged at 500 XG for 20 min. The interface between the Histopaque and the first layer was further isolated. After washing with 1X of PBS 3 times, the cell pellets were re-suspended in complete RPMI 1640 medium (Invitrogen, Cat#11875119) containing 10% fetal bovine serum (HyClone, Logan, UT), 100 U/mL of penicillin and 100 μg/mL of streptomycin. The total number of the cells and their viability were determined using the hemocytometer and trypan blue exclusion method.
The phagocytosis assay was performed as previously mentioned with modifications (26). Briefly, 0.5 McFarland standard of yeast suspension containing 106 cells /mL was prepared. Then, 0.5 mL of the yeast suspension was added to a tube containing 1 mL of MO/MQ cells suspension consisting of 0.5 mL of 1X PBS and 0.5 mL of RPMI 1640 media with 100 μL of activated fetal calf serum. After a 2 h incubation period, different slide smears from the cell suspensions containing yeast were prepared, stained with Giemsa stain and examined under microscope using oil emersion lens. Around 200 cells in different fields were scanned for evaluation of the percentage of phagocytic activity determined by counting the number of macrophages with ingested intracellular yeast cells, while the candidacidal activity was indicated by the number of digested or killed yeast “Ghost cells” present per 100 cells of phagocytosed candida.
Lysozyme activity in serum samples was evaluated according to the method mentioned formerly (27). Briefly, 1.9 mL of Micrococcus Lysideikticus (0.2 mg/mL) suspension in sodium phosphate buffer (pH = 6.2) was added to 100 μL of avian serum. Then, the reaction was incubated at 25° C for 0.5 and 4.5 min before measuring the absorbance on turbidimeter at 530 nm in both time intervals. The lysozyme activity was indicated by the amount of sample required to reduce the absorbance by 0.001 per minute indicating the increase in lysis of Micrococcus lysideikticus. Serum NO contents were evaluated as previously mentioned (19) using the commercial kits (Jiancheng Biotechnology Institute, Nanjing, China).
Samples were taken from intestinal tissues at the end of the experimental period and fixed in 10% neutral buffered formalin and the fixed tissue sections were then processed for routine histopathological procedures. The tissues were subsequently embedded in paraffin wax for sectioning, stained with hematoxicillin-eosin and examined under the light microscope. Died birds were necropsied and subjected to metric evaluation of histological alterations in ileal tissues consistent with the criteria established formerly (28), in which the impact factor (IF) ranged from 1 to 3 with 3 denoting sever lesions. Furthermore, the intensity of each lesion (S) was scored within the range of 0 to 3; no lesion, up to 25% alteration, 25 to 50% alteration and more than 50% alteration, respectively. Histopathological alterations were evaluated in relation to the following formula; Σ(IF*S) (28).
Statistical variance was analyzed via SPSS 27.0 software (IBM Corp., Chicago, United States) using one way analysis of variance (ANOVA). To determine statistical variations among the evaluated means, Tukey’s test was utilized, where p values lower than 0.05 implied that the experimental model terms were significant. All figures were created using GraphPad Prism (United States).
• The selected growth performance parameters are summarized in Table 3. Prior to C. perfringens infection (during starter and grower periods), BWG and FCR were significantly improved in broilers fed quercetin-loaded Fe3O4-NPs (p < 0.05), followed by those supplemented by either with quercetin or Fe3O4-NPs alone unlike the control unsupplemented birds. During the overall experimental period (38 days), necrotic enteritis infected birds showed a reduction in growth performance associated parameters and increased mortality rates (38%). In contrast, the group fed the combination of quercetin and Fe3O4-NPs exhibited the greatest improvement in BWG and FCR (p < 0.05) and had the lowest mortality rate (4%).
Table 3. Efficacy of dietary supplementation of quercetin or Fe3O4-NPs alone or their nano composite (quercetin-loaded Fe3O4-NPs) on growth performance parameters of broilers challenged with C. perfringens at grower stage.
• Results of C. perfringens quantification using real-time PCR are presented in Figure 2. The intestinal contents of infected broilers received a basal diet supplemented with Fe3O4-NPs or quercetin, whether combined or separately, exhibited significant decreases in C. perfringens abundance compared to infected birds received control diet at 7 and 14 days post-challenge (p < 0.05). Specifically, birds receiving the combination of quercetin and Fe3O4-NPs, followed by those fed Fe3O4-NPs alone, displayed lower C. perfringens populations comparing with infected birds (1.64, 1.87 and 3.85 log10 CFU per gram, respectively) at 14 days post-challenge (p < 0.05; Figure 2A). Consistent with the C. perfringens count findings, intestinal lesion scores were lower in groups supplemented with Fe3O4-NPs, quercetin, or the quercetin-loaded Fe3O4-NPs unlike the C. perfringens infected group. The most notable decreases in intestinal scores were detected in the quercetin-loaded Fe3O4-NPs group, followed by the Fe3O4-NPs alone group (Figure 2B). Additionally, expression data for C. perfringens nanJ, nanI, nagH, netB, cpe and cnaA virulence genes demonstrated noteworthy downregulatory roles for either Fe3O4-NPs, quercetin, or their combination (p < 0.05; Figure 2C). Interestingly, dietary inclusion of quercetin-loaded Fe3O4-NPs resulted in the greatest downregulation in C. perfringens netB, cpe and cnaA virulence genes (0.21, 0.05 and 0.37- fold change, respectively).
Figure 2. Efficacy of dietary supplementation of quercetin or Fe3O4-NPs alone or their nano composite (quercetin-loaded Fe3O4-NPs) on C. perfringens population in intestinal contents of broiler chickens at 7- and 14-days post challenge with C. perfringens (A), intestinal lesion score (B) and the expression of C. perfringens virulence genes (C) including nanJ, nanI, nagH, netB, cpe and cnaA. Control: birds fed basal diet and challenged with C. perfringens at the grower period, Fe3O4-NPs: birds fed basal diet supplemented with 60 mg/kg diet of Fe3O4-NPs, quercetin: birds fed basal diet supplemented with 300 mg/kg diet of quercetin, quercetin-loaded Fe3O4-NPs: birds fed basal diet supplemented with a nanocomposite of quercetin and Fe3O4-NPs (300 mg/kg diet). a–d Means within the same column carrying different superscripts are significantly different at p < 0.05.
Data presented in Figure 3 showed varying expression levels of intestinal innate defense and inflammatory associated markers following C. perfringens challenge, in response to supplementation of Fe3O4-NPs, quercetin, or their combination. Notably, the most marked upregulation levels of intestinal innate antimicrobial defense encoded genes; AVBD6 and AVBD12 as well as the anti-inflammatory IL-10 gene were noted in group receiving quercetin-loaded Fe3O4-NPs post C. perfringens challenge (3.23, 1.98 and 3.78-fold change, respectively).
Figure 3. Efficacy of dietary supplementation of quercetin or Fe3O4-NPs alone or their nano composite (quercetin-loaded Fe3O4-NPs) on the mRNA expression of host defense antimicrobial peptides (Avian β-defensin 6 and 12; AvBD6, B and AvBD12, C), inflammatory regulators genes [interleukin, IL-10, (A), (IL)-1β, (D), C-X-C motif chemokine ligand 8; CXCL8, E], tumor necrosis factor-α (TNF-α, F), chemokine C–C motif ligand 20 or also known as macrophage inflammatory proteins −3 α (CCL20, G), inducible nitric oxide synthase (iNOS, H) cycloox-ygenase-2 (COX-2, I), caspase-1, (J), caspase-3, K and cytochrome P450, family 17, subfamily A, polypeptide 1 (CYP17A1, L) in intestinal tissues of broiler chickens challenged with C. perfringens. Control: birds fed basal diet and challenged with C. perfringens at the grower period, Fe3O4-NPs: birds fed basal diet supplemented with 60 mg/kg diet of Fe3O4-NPs, quercetin: birds fed basal diet supplemented with 300 mg/kg diet of quercetin, quercetin-loaded Fe3O4-NPs: birds fed basal diet supplemented with a nanocomposite of quercetin and Fe3O4-NPs (300 mg/kg diet). a–d Means within the same column carrying different superscripts are significantly different at p < 0.05.
Relative mRNA expression levels of IL-1β and CXCL8 genes were significantly downregulated (p < 0.05) in birds fed quercetin-loaded Fe3O4-NPs, followed by those fed quercetin or Fe3O4-NPs alone, unlike the infected control group. Birds supplemented with the combination of quercetin and Fe3O4-NPs also showed the most pronounced downregulation of TNF-α and iNOS genes (0.51 and 0.48-fold change, respectively). Additionally, the most notable downregulation of COX-2 gene expression was observed in the groups fed quercetin and quercetin-loaded Fe3O4-NPs (0.80 and 0.77-fold change, respectively). For the CCL20 gene, dietary inclusion of quercetin-loaded Fe3O4-NPs resulted in detectable downregulation (0.49-fold change) unlike the infected control group (p < 0.05).
The relative mRNA expression levels of CYP17A1, Caspase-1 and Caspase-3 genes were significantly downregulated (p < 0.05) in birds fed quercetin-loaded Fe3O4-NPs, quercetin, and Fe3O4-NPs alone, compared to the infected group. Interestingly, CYP17A1 and Caspase-1 genes achieved their significant lowest expression levels (p < 0.05) in birds fed quercetin-loaded Fe3O4-NPs. Meanwhile, dietary inclusion of quercetin and quercetin-loaded Fe3O4-NPs resulted in prominent downregulation of the Caspase-3 gene unlike the infected control group (p < 0.05), with no significant variations between the quercetin and quercetin-loaded Fe3O4-NPs groups (p > 0.05).
Quercetin-loaded Fe3O4-NPs upregulated antioxidant encoded genes, which consequently mitigated oxidative stress associated with C. perfringens infection, as illustrated in Figure 4. The relative mRNA expression levels of antioxidant related genes, including CAT, SOD, HO-1, GSH-Px and NQO1 were significantly increased in birds fed Fe3O4-NPs, quercetin, or their combination in comparison with the control group. Of note, the most significant increases (p < 0.05) in the relative mRNA expression levels of CAT, GSH-Px and NQO1 genes were noticed in birds supplemented with quercetin-loaded Fe3O4-NPs (1.65, 1.45 and 1.59-fold change, respectively). Meanwhile, there was no significant variations (p > 0.05) in the transcription levels of the SOD gene between the quercetin and quercetin-loaded Fe3O4-NPs groups (1.41 and 1.44-fold change, respectively). Moreover, the quercetin-loaded Fe3O4-NPs group showed significant upregulation (p < 0.05) of the HO-1 gene expression level (1.37-fold change), followed by the quercetin (1.21-fold change) and Fe3O4-NPs (1.19-fold change) groups, compared to the control group. There were no significant differences (p > 0.05) between the quercetin and Fe3O4-NPs groups in terms of HO-1 expression.
Figure 4. Efficacy of dietary supplementation of quercetin or Fe3O4-NPs alone or their nano composite (quercetin-loaded Fe3O4-NPs) antioxidant related genes including catalase (A), superoxide dismutase (SOD, B), glutathione peroxidase (GSH-Px, C), heme oxygenase-1 (HO-1, D), NAD(P)H dehydrogenase quinone 1 (NQO1, E) in intestinal tissues of broiler chickens challenged with C. perfringens. Control: birds fed basal diet and challenged with C. perfringens at the grower period, Fe3O4-NPs: birds fed basal diet supplemented with 60 mg/kg diet of Fe3O4-NPs, quercetin: birds fed basal diet supplemented with 300 mg/kg diet of quercetin, quercetin-loaded Fe3O4-NPs: birds fed basal diet supplemented with a nanocomposite of quercetin and Fe3O4-NPs (300 mg/kg diet). a–d Means within the same column carrying different superscripts are significantly different at p < 0.05.
Biochemical analysis of broiler breast muscles for oxidative stress-associated enzymes showed a significant reduction in ROS, H2O2 and MDA levels, with a prominent increase in T-AOC levels in all supplemented broiler groups compared to the control group fed the basal diet (p < 0.05; Table 4). Notably, the T-AOC level reached its peak (3.39 U/mg of protein), while ROS, H2O2, and MDA levels attained their lowest values (32.11 μL/ g tissue, 16.24 μmoL/g tissue and 1.82 nmoL/g tissue, respectively) in the quercetin-loaded Fe3O4-NPs fed group. Concerning the expression data of antioxidant-related genes in the breast meat of broilers, as shown in Figure 5, there were noticeable increases in the relative mRNA expression levels of antioxidant related genes, including CAT, SOD, HO-1, GSH-Px and NQO1, in the breast muscles of broilers in response to supplementation of Fe3O4-NPs, quercetin, or their combination, compared with the control group (p < 0.05). Remarkably, the highest transcription levels of SOD and GSH-Px genes were observed in the quercetin and quercetin-loaded Fe3O4-NPs fed groups, with no significant differences between them. Moreover, the maximum significant upregulation (p < 0.05) of CAT, HO-1 and NQO1 genes was attained in the quercetin-loaded Fe3O4-NPs supplemented group with fold changes of 4.79, 2.32 and 2.48-, respectively.
Table 4. Efficacy of dietary supplementation of quercetin or Fe3O4-NPs alone or their nano composite (quercetin-loaded Fe3O4-NPs) on antioxidant related biomarkers muscle tissues of broilers challenged with C. perfringens at grower stage.
Figure 5. Efficacy of dietary supplementation of quercetin or Fe3O4-NPs alone or their nano composite (quercetin-loaded Fe3O4-NPs) antioxidant related genes Catalase (A), superoxide dismutase (SOD B), glutathione peroxidase (GSH-Px, C), heme oxygenase-1 (HO-1, D), NAD(P)H dehydrogenase quinone 1 (NQO1, E) in breast muscle tissues of broiler chickens challenged with C. perfringens. Control: birds fed basal diet and challenged with C. perfringens at the grower period, Fe3O4-NPs: birds fed basal diet supplemented with 60 mg/kg diet of Fe3O4-NPs, quercetin: birds fed basal diet supplemented with 300 mg/kg diet of quercetin, quercetin-loaded Fe3O4-NPs: birds fed basal diet supplemented with a nanocomposite of quercetin and Fe3O4-NPs (300 mg/kg diet). a–d Means within the same column carrying different superscripts are significantly different at p < 0.05.
Compared with C. perfringens challenged group, significant (p < 0.05) increases in RBCs counts and hemoglobin (Hb) concentrations were noted in groups supplemented with quercetin-loaded Fe3O4-NPs followed by Fe3O4-NPs (Table 5). Our data indicated that feeding broilers Fe3O4-NPs, quercetin, or quercetin-loaded Fe3O4-NPs had an immunomodulatory effect on phagocytes derived from peripheral blood mononuclear cells (Table 5). The most significant enhancements in the phagocytic and killing activities of phagocytes, along with highest lysozyme activity and lowest production of inflammatory serum NO, were noticed in the group of chickens fed quercetin-loaded Fe3O4-NPs (p < 0.05).
Table 5. Efficacy of dietary supplementation of quercetin or Fe3O4-NPs alone or their nano composite (quercetin-loaded Fe3O4-NPs) on hematological picture and innate immune defense of broilers challenged with C. perfringens at grower stage.
Notably, C. perfringens intestinal colonization during infection in control infected group was associated with microbial disruption, as reflected by a notable decrease in the populations of Lactobacillus and Bifidobacterium species, alongside an increase in the Enterobacteriaceae community. Meanwhile, supplementation with quercetin-loaded Fe3O4-NPs restored this microbial balance by favoring the growth of beneficial Lactobacillus and Bifidobacterium species while reducing the abundance of harmful Enterobacteriaceae (Figure 6).
Figure 6. Efficacy of dietary supplementation of quercetin or Fe3O4-NPs alone or their nano composite (quercetin-loaded Fe3O4-NPs) on intestinal Lactobacillus spp. (A), Bifidobacterium spp. (B) and Enterobacteriaceae (C) populations of broiler chickens challenged with C. perfringens. Control: birds fed basal diet and challenged with C. perfringens at the grower period, Fe3O4-NPs: birds fed basal diet supplemented with 60 mg/kg diet of Fe3O4-NPs, quercetin: birds fed basal diet supplemented with 300 mg/kg diet of quercetin, quercetin-loaded Fe3O4-NPs: birds fed basal diet supplemented with a nanocomposite of quercetin and Fe3O4-NPs (300 mg/kg diet). a–d Means within the same column carrying different superscripts are significantly different at p < 0.05.
Histopathological pictures of intestinal tissues from broilers experimentally infected with C. perfringens, in response to supplementation of Fe3O4-NPs, or quercetin, or formulated quercetin-loaded Fe3O4-NPs, are illustrated in Figure 7. Infected control birds revealed sloughing of some mucosal epithelium and necrotic sheets of lining epithelium with pyknotic nuclei. Furthermore, there were marked infiltrations of inflammatory cells within the lamina propria and submucosa adjacent to areas of lytic necrosis, as well as the presence of dilated blood vessels. Moreover, birds supplemented with Fe3O4-NPs displayed mild aggregations of inflammatory cells within the mucosal and submucosal layers. Quercetin-fed birds exhibited necrotic and sloughed areas on some mucosal villi, with moderate infiltration of inflammatory cells in the lamina propria and submucosa. Notably, the inclusion of quercetin-loaded Fe3O4-NPs restored the intestinal histopathological architecture, preserving mucosal villi, lamina propria, mucosal crypts, and musculosa. C. perfringens infected birds had the highest ileal total scores due to increased epithelial and lamina propria thickness from inflammatory cell infiltration, congestion, and excessive enterocyte proliferation. Conversely, supplementation with quercetin-loaded Fe3O4-NPs subsided these exaggerated ileal scores, as reflected by improvements in the measured metrics (Figure 8).
Figure 7. Photomicrograph of H&E stained intestinal sections showing sloughing of some mucosal epithelium and necrotic sheets of lining epithelium with pyknotic nuclei (arrow), marked inflammatory cells’ infiltrations within lamina propria and submucosa (star) adjacent to the area of lytic necrosis (curved arrow) besides the presence of dilated blood vessels (arrowheads) in control infected birds (A,B), mild aggregations of inflammatory cells within mucosal and submucosal layers (star) in Fe3O4-NPs supplemented birds (C), necrotic and sloughed area at some mucosal villi (arrow) with moderate numbers of inflammatory cells’ infiltration within lamina propria and submucosa (star) (D) and maintenance of mucosal villi, lamina propria, mucosal crypts and musculosa in quercetin-loaded Fe3O4-NPs fed birds (E), scale bar 200,100 and 20 μm. Control: birds fed basal diet and challenged with C. perfringens at the grower period, Fe3O4-NPs: birds fed basal diet supplemented with 60 mg/kg diet of Fe3O4-NPs, quercetin: birds fed basal diet supplemented with 300 mg/kg diet of quercetin, quercetin-loaded Fe3O4-NPs: birds fed basal diet supplemented with a nanocomposite of quercetin and Fe3O4-NPs (300 mg/kg diet).
Figure 8. Efficacy of dietary supplementation of quercetin or Fe3O4-NPs alone or their nano composite (quercetin-loaded Fe3O4-NPs) on metric evaluation of the intestinal tissues alterations (A–E) of broilers challenged with challenged with C. perfringens at grower stage. Control: birds fed basal diet and challenged with C. perfringens at the grower period, quercetin: birds fed basal diet supplemented with (300 mg/kg diet of quercetin, Fe3O4-NPs: birds fed basal diet supplemented with 60 mg/kg diet of Fe3O4-NPs, quercetin-loaded Fe3O4-NPs: birds fed basal diet supplemented with a nanocomposite of quercetin and Fe3O4-NPs; 300 mg/kg diet). a–dMeans within the same column carrying different superscripts are significantly different at p < 0.05.
Necrotic enteritis caused by C. perfringens infection in broilers is a multifactorial disease influenced by various predisposing factors (29). This disease poses a significant threat not only to the health of broilers but also to the profitability of the poultry industry, particularly due to the short rearing period of broilers (6). The disease is also a public health concern due to its potential as a foodborne infection (1). The ban forbidding antibiotic use in poultry to combat bacterial resistance caused a spike in the occurrence of NE in broiler populations. The use of novel generations of nanocomposite based on natural products in poultry diet seems to be promising (30, 31). Quercetin is a natural flavonoid with pleotropic biological effects. It has been demonstrated that quercetin supplementation mitigates intestinal inflammation and maintains intestinal immune barrier integrity in aged laying hens (15). Iron oxide nanoparticles, particularly magnetite NPs, have revolutionized various fields due to their high surface area, availability, cost-effectiveness, rapid adsorption kinetics, and ease of dispersibility in solutions (32). Additionally, their superparamagnetic propderties allow for easy separation from reaction mixtures using a simple magnet. In this context, incorporation of quercetin into the magnetite NPs in a suitable pharmaceutical formula, that overcomes their low bioavailability and maintains their action, would be required. Moreover, the exact mechanism of their targeted action and their effectiveness in enhancing broiler resistance to C. perfringens infection needs to be further explored. Therefore, our study explored the immunomodulatory and protective effect of magnetite NPs enclosed quercetin on broiler chickens experimentally infected with C. perfringens.
The data in this study indicated that broilers challenged with C. perfringens and received quercetin-loaded Fe3O4-NPs showed a restored BWG and FCR over the whole experimental period. In consistence with these results, Goliomytis, Tsoureki, Simitzis, Charismiadou, Hager-Theodorides and Deligeorgis (17) demonstrated that dietary levels of free dietary quercetin (0.5 g/kg of feed) enhanced FCR of broiler chickens. Additionally, the production efficiency of broiler chickens was enhanced by supplemental quercetin at a concentration of 200 ppm (33). The augmented growth performance of broilers post dietary supplementation of nanosized particles of quercetin at lower levels (100 ppm/kg) is attributed to their enhanced bioavailability and solubility (34). Additionally, quercetin supplementation positively effect on growth performance and nutrient digestibility of broilers (16). Moreover, supplementing nano sized magnetite iron exerted a notable growth promoting role during the entire production cycle, as evidenced by increased body weight gain and improved FCR in broilers. These favorable findings can be attributed to the critical role of iron in oxygen transport, the regulation of various proteins and enzymes involved in cell development and differentiation, and overall health maintenance (32). Besides, it participate pointedly to the tricarboxylic acid cycle via supporting many enzymes, which assist in the elimination of harmful metabolites by peroxidases and catalases within iron (35). Furthermore, iron nanoparticles at the level of 40 mg/kg increased broiler performance (36). This may be due to the ability of nanosized particles to cross biological barriers more effectively, be rapidly absorbed by cells, and thus demonstrate better bioavailability compared to other mineral forms (37). Specifically, encapsulating potent antioxidant bioactive components such as quercetin within Fe₃O₄-NPs in broiler feed provides additional protection and allows for synergistic effects that enhance bird performance. Furthermore, conjugating quercetin with superparamagnetic iron oxide nanoparticles improves its bioavailability compared to free quercetin (38).
In addition, our pathological images showed that C. perfringens infection in broilers caused multifocal areas of necrosis, marked inflammatory cells’ infiltrations within lamina propria and submucosa adjacent to the area of lytic necrosis besides the presence of dilated blood vessels in intestinal tissues with high scored histological alterations. However, the severity of intestinal lesions and histological scores improved following supplementation with quercetin-loaded iron nanocomposites, as indicated by a significant reduction in the mRNA expression of netB and cpe toxin-related gene. In this regard, NetB toxin is considered a pore forming toxin regulated by netB gene and played a role in progression of the histopathological lesions of NE infected chickens (39). This inflammatory necrosis induced by C. perfringens toxin occurs through the formation of mushroom-like transmembrane pores in enterocytes. This process is mediated by bacterial adhesion to the intestinal mucosa via fimbrial adhesins (40). Consequently, these transmembrane pores disrupt the cell membrane’s osmotic balance, leading to osmotic cell lysis and necrosis (41). Similar to NetB toxin, Cpe enterotoxin also induces multiple pore formation in the plasma membrane of epithelial cells by binding to the claudin receptor. This leads to calcium influx, activation of calpain and caspase-3, and ultimately, cell death (41). Accordingly, Kumar and his colleagues studied the antimicrobial effect of microencapsulated formula containing bioactive natural molecules on Cobb birds infected with both Eimeria and C. perfringens. They demonstrated restoration in duodenal histopathological architecture in birds that were fed this microencapsulated formula (6).
Enterocytes are known to play a key role in regulating iron absorption and its transport into circulation (41, 42). The current study suggested that necrotic damage to enterocytes impairs absorption of iron. This, in turn, induced an oxidative stress response due to the accumulation of free radicals. Therefore, supplementing poultry diets with nano-iron combined with natural flavonoids such as quercetin enhances iron absorption and bioavailability, helping enterocytes better tolerate oxidative damage induced by C. perfringens infection. Our data indicated that the improvement in growth performance was associated with a reduction in the severity of intestinal inflammation and necrosis caused by C. perfringens infection in broilers.
Although it has been reported that the CFU of C. perfringens can reach 105 in healthy chickens, predisposing factors affecting the gut microenvironment and host immunity can lead to higher colonization and increased toxin production, contributing to necrotic enteritis (NE) in birds (3). A comparative study of biologically and chemically synthesized iron oxide nanoparticles showed that biologically synthesized nanoparticles, which contained natural plant extracts, enhanced the microbicidal activity of the nanoparticles against S. aureus and P. aeruginosa strains isolated from poultry feed (21). However, the direct antimicrobial activity of quercetin-loaded iron nanocomposite against C. perfringens isolated from broilers, which consequently hinders its colonization within chicken’s intestine is not deeply investigated until yet. Our data reported that infected control birds expressed higher intestinal C. perfringens populations with exalted C. perfringens virulence. Conversely, broilers fed quercetin-loaded Fe3O4-NPs exhibited a reduction in intestinal C. perfringens abundance along with diminishing in the mRNA expression of nanJ, nanI, nagH and cnaA virulence genes. Previous studies have proven the antimicrobial activities of magnetic iron against many bacterial and parasitic strains (43, 44). Additionally, incorporating an optimal amount of the powerful antioxidant quercetin into Fe₃O₄-NPs enhanced the host’s endogenous defense mechanisms against microbial infections and external stressors (45).
Likewise, the majority of magnetic NPs exerts a bactericidal character as applied against both Gram positive and Gram negative bacteria (46). Moreover, iron oxide magnetic nanoparticles can be used as antimicrobial delivery systems due to their enhanced ability to deliver antimicrobials specifically to targeted site (47) without using of antibiotics (48). Furthermore, magnetite iron oxide NPs were proven to have a strong antibacterial activity through numerous theories as following: iron NPs small size enables them to penetrate the bacterial cell wall triggering cell membrane disruption (49) and oxidative cytotoxic damage to bacterial cell via elevating the bacterial cells reactive hydroxyl radicals (50) g (70). Additionally, they can bind to bacterial DNA and proteins, thereby initiating a bactericidal effect (51) besides the high magnetite role of small sized nanoparticles on bacterial cells causing clogging between bacterial cells instigating their death (52). Altogether, proteolytic, hydrolytic and collagenolytic enzymes like NagH, NanI and NanJ secreted from C. perfringens promote extracellular matrix and intercellular damage, mucosal permeability and cell death (1). Moreover, upregulation of cnaA gene associated with collagen adhesion protein was reported to facilitate C. perfringens adhesion to extracellular matrix and enhance their colonization within ileal mucosa (71). In this context, iron oxide nanoparticles exhibit exceptional paramagnetic activity, generating magnetic fields with strong adhesion properties and further cause biofilm destruction and cell death through mechanisms such as vibration damage, local hyperthermia, cell fusion, membrane rupture, and ultimately, cell death (53). Furthermore, iron oxide nanocrystals can prohibit clostridial spore germination and diminish associated inflammation (54).
In addition, quercetin can inhibit the exaggerated inflammatory response following infection via subsiding the expression of TNF-α, IL-1β, and IL-6 in macrophages (55). Moreover, dietary supplementation of quercetin improved immune function by increasing the secretion of immune molecules to protect against infection (56). Also, quercetin exerts broad-spectrum antibacterial properties via disrupting cell walls of bacteria, changing the bacterial cells permeability; impeding the synthesis of nucleic acids, and in that way impacting the synthesis and expression of proproteins; and decreasing enzyme and toxin activities (57). Collectively, the prominent antibacterial effect proved for iron oxide nanoparticles combined with quercetin was attributed to their destructive effect on bacterial cell wall and subsequently losing their different virulence factors enabling them to establish an infection and damage the host and interfering with host immunity (58). These previous findings further supported the superior role of combining both quercetin and iron nanoparticles on broilers performance and fight against C. perfringens infection.
Besides the above-mentioned issues, quercetin-loaded Fe3O4-NPs improved antioxidant stability in broilers breast muscles through regulation of antioxidant enzymes encoded genes. Similar findings reported by Goliomytis and his co-workers, where inclusion of quercetin (0.5 and 1 g/kg of feed) to broiler diets for 42 days indicated improvement in growth performance and oxidative stability of broilers breast muscles (17). Coordinated with previously published data, a study reported that using nano-Fe (40 mg/kg) either alone or combined with a natural extract in broilers’ diets improved their resistance to heat stress. The study proposed that nano-Fe enhanced broiler growth performance and meat quality associated with low mortality under hot conditions (36). Similarly, quercetin-loaded Fe3O4-NPs reduced oxidative stress associated with the effect of C. perfringens secreted toxins by increasing the mRNA expression of antioxidant-related genes (CAT, SOD1, GSH-Px, HO-1, and NQO1), compared to the control non-supplemented group. In the same line, upregulation of HO-1 is a protective feedback mechanism of cells against oxidative stress associated damage (31). On the other hand, the increased production of intestinal avian defensins, due to the upregulation of their related genes (Avβd6 and Avβd12), in C. perfringens-infected broilers fed a diet supplemented with quercetin-loaded Fe3O4-NPs, suggests an indirect bactericidal effect on C. perfringens. The increased production of defensins also indicates that quercetin-loaded Fe3O4-NPs improve epithelial cell function and mucosal integrity (72) reported the ability of quercetin supplementation in boosting gut integrity as evidenced by increases in mRNA expression of genes encoding intestinal tight junction protein; zona occludens-1 (ZO-1) and occludin in broilers challenged model compared to control group fed basal diet only Additionally, quercetin represents one of the natural flavonoids, which could protect intestinal tight junction integrity from oxidative stress through its antioxidant effect. Moreover, quercetin ameliorated intestinal inflammation in broiler chickens through restoring intestinal barrier functions and microbiota community, which consequently reduced inflammatory mediators and mucosal damage (72). Given that CXCL8 is an inflammatory mediator that has a role in activation and recruitment of neutrophils (73), pro-inflammatory cytokines stimulate CCL20 production, which has a role in T-cell infiltration (74). These inflammatory mediators exaggerate mucosal damage, vascular permeability, oxygen species production and recruitment of heterophils, monocytes and lymphocytes to inflamed bird’s gut (1). Indeed, the increase in the production of antioxidant enzymes post supplementation of quercetin-loaded Fe3O4-NPs could reduce COX-2 and iNos production, which consequently reduce inflammation. These findings were supported by downregulation of TiNF-α, IL-1β, Caspase-1 and CXCL8 genes in groups of bird fed quercetin-loaded Fe3O4-NPs suggesting the neglected effect of NE derived C. perfringens virulence on epithelial cells. Furthermore, upregulation of anti-inflammatory mediators; IL-10 and HO-1 suggested the ability of quercetin-loaded Fe3O4-NPs to induce polarization of anti-inflammatory macrophages phenotypes promoting the homeostatic response within intestinal barriers, which come concurrently with (75).
Clostridium perfringens α-toxin induces hemolysis of erythrocytes in many species involving poultry (59). In the current study, the RBCs counts and hemoglobin concentration in groups supplemented with Fe3O4-NPs post C. perfringens challenge exhibited higher values unlike un supplemented control challenged group. These outcomes could be attributed to their bactericidal role against experimental challenge beside their impact on augmenting RBCs synthesis (60) which was further boosted following formulation of iron into nano sized molecule (32). We further investigated whether quercetin-loaded Fe₃O₄-NPs, as feed additives in broiler diets, have a systemic effect in preventing microbial spreading or its products, thereby reducing endotoxemia and systemic inflammation. This study demonstrated that broilers fed quercetin-loaded Fe₃O₄-NPs showed enhanced phagocytic capacity and killing ability of phagocytes derived from peripheral blood mononuclear cells, compared to the infected, non-supplemented control group. This activation was associated with increased lysozyme activity and reduced nitric oxide production, suggesting that iron-quercetin may mitigate systemic inflammation caused by C. perfringens in peripheral blood. Indeed, Zanella and colleagues proposed that iron oxide nanoparticles can cross lipid bilayers directly, creating transient conductance without adversely affecting membrane integrity (76). This effect may explain the enhanced immunity and antioxidant activity observed in the current study from combining iron nanoparticles with quercetin, particularly in combating inflammatory necrotic enteritis caused by C. perfringens infection in broilers.
Mounting evidence indicated the relation between microbiota abundance and NE in chickens (77). For instance, the relation between low abundance of Ruminococcus species and NE derived C. perfringens was reported (3). Herein, there was an increase in the abundance of Lactobacillus and bifidobacterium species in quercetin-loaded Fe3O4-NPs supplemented groups compared to the control one. Accordingly, lactobacillus counts were boosted (p < 0.05), due to enhancement of the gut microbiota environment in quercetin-supplemented groups (33).
Lactobacillus and bifidobacteria produce short-chain fatty acids that serve as energy sources for intestinal bacteria, enhance nutrient utilization, improve immune defense, and aid in pathogen clearance from the gut (77, 78). In this context, quercetin alleviated microbiota dysbiosis caused by antibiotics in an animal model of liver damage by promoting the growth of Lactobacillus, Bifidobacterium, and Bacteroides, while reducing the abundance of Enterobacteriaceae and Clostridium species (79). Moreover, dietary inclusion of optimum level of iron plays an important role in increasing the abundance of beneficial microbiota; however iron deficiency can reduce its load and its overload can augment the growth of harmful ones like E. coli and Bacteroides (80). Another study reported that feeding broilers on Lactobacillus probiotics enhanced broiler resistance against C. perfringens through the competitive exclusion mechanisms experimental infection and mitigated inflammatory NE associated with its infection (73).
The use of quercetin-loaded Fe3O4-NPs represents a promising new feed additive for broilers’ diets, aligning with the global goal of forbidding the uncontrolled antibiotics use while ensuring efficient and sustainable poultry production. Herein, the newly formulated nanocomposite strengthens intestinal and antioxidant barriers supporting maximized performance during NE experimental model. The association between reduction in C. perfringens abundance and its virulence suggests the direct antimicrobial effect of quercetin-loaded iron nanocomposite in potentiating chicken intestinal microenvironment, barrier functions and immunity, thereby hindering C. perfringens colonization and its effect on intestinal mucosal damage. Finally, this study strongly directs future researches for expanding the application of other nanomaterials to trigger advances in nanotechnology development particularly, in poultry feed industry.
The original contributions presented in the study are included in the article/supplementary material. Further inquiries can be directed to the corresponding author.
The animal study was approved by All experiments were performed at Faculty of Veterinary Medicine, Zagazig University, Egypt according to the Institutional Animal Care and Use Committee (IACUC) guidelines with approval number of ZUIACUC/2/F/192/2022. The study was conducted in accordance with the local legislation and institutional requirements.
AA-N: Conceptualization, Formal analysis, Visualization, Writing – original draft. AE-D: Methodology, Software, Visualization, Writing – original draft. DI: Conceptualization, Formal analysis, Funding acquisition, Investigation, Methodology, Supervision, Visualization, Writing – original draft, Writing – review & editing. MAE-H: Conceptualization, Funding acquisition, Investigation, Methodology, Project administration, Supervision, Visualization, Writing – original draft, Writing – review & editing. HA-K: Conceptualization, Funding acquisition, Writing – original draft. OE-b: Formal Analysis, Investigation, Methodology, Visualization, Writing – original draft. ES: Conceptualization, Methodology, Validation, Visualization, Writing – original draft. ME-A: Resources, Software, Supervision, Validation, Writing – original draft. MI: Project administration, Software, Supervision, Writing – original draft. SB: Investigation, Methodology, Writing – review & editing. NE: Methodology, Writing – original draft. TI: Conceptualization, Data curation, Formal analysis, Resources, Writing – original draft. SE: Funding acquisition, Investigation, Methodology, Project administration, Writing – original draft.
The author(s) declare financial support was received for the research, authorship, and/or publication of this article. This research was funded by Taif University, Saudi Arabia, Project No. (TU-DSPP-2024-192).
The authors extend their appreciation to Taif University, Saudi Arabia for supporting this work through project number (TU-DSPP-2024-192).
The authors declare that the research was conducted in the absence of any commercial or financial relationships that could be construed as a potential conflict of interest.
All claims expressed in this article are solely those of the authors and do not necessarily represent those of their affiliated organizations, or those of the publisher, the editors and the reviewers. Any product that may be evaluated in this article, or claim that may be made by its manufacturer, is not guaranteed or endorsed by the publisher.
1. Mora, ZV, Macías-Rodríguez, ME, Arratia-Quijada, J, Gonzalez-Torres, YS, Nuño, K, and Villarruel-López, A. Clostridium perfringens as foodborne pathogen in broiler production: pathophysiology and potential strategies for controlling necrotic enteritis. Animals. (2020) 10:1718. doi: 10.3390/ani10091718
2. Ibrahim, D, Ismail, TA, Khalifa, E, El-Kader, A, Shaimaa, A, Mohamed, DI, et al. Supplementing Garlic Nanohydrogel Optimized Growth, Gastrointestinal Integrity and Economics and Ameliorated Necrotic Enteritis in Broiler Chickens Using a Clostridium perfringens Challenge Model. Animals. (2021) 11:2027. doi: 10.3390/ani11072027
3. Gharib-Naseri, K, Kheravii, S, Keerqin, C, Morgan, N, Swick, R, Choct, M, et al. Two different Clostridium perfringens strains produce different levels of necrotic enteritis in broiler chickens. Poult Sci. (2019) 98:6422–32. doi: 10.3382/ps/pez480
4. Ammar, AM, El-Naenaeey, E-SY, Abd El-Hamid, MI, El-Gedawy, AA, and Elmalt, RM. Campylobacter as a major foodborne pathogen: A review of its characteristics, pathogenesis, antimicrobial resistance and control. J Microbiol Biotechnol Food Sci. (2021) 10:609–19. doi: 10.15414/jmbfs.2021.10.4.609-619
5. Elfaky, MA, Abdel-Hamid, MI, Khalifa, E, Alshareef, WA, Mosbah, RA, Elazab, ST, et al. Innovative next-generation therapies in combating multi-drug-resistant and multi-virulent Escherichia coli isolates: Insights from in vitro, in vivo, and molecular docking studies. Appl Microbiol Biotechnol. (2022) 106:1691–703. doi: 10.1007/s00253-022-11781-w
6. Kumar, M, Ratwan, P, Dahiya, S, and Nehra, AK. Climate change and heat stress: Impact on production, reproduction and growth performance of poultry and its mitigation using genetic strategies. J Therm Biol. (2021) 97:102867. doi: 10.1016/j.jtherbio.2021.102867
7. Abd el-Hamid, MI, el-Azzouny, MM, el-Malt, RMS, Elkenawy, ME, Abdelwarith, AA, Younis, EM, et al. Future impact of thymoquinone-loaded nanoemulsion in rabbits: prospects for enhancing growth, immunity, antioxidant potential and resistance against Pasteurella multocida. Front Vet Sci. (2024) 10:1340964. doi: 10.3389/fvets.2023.1340964
8. Aljazzar, A, Abd El-Hamid, MI, El-Malt, RM, El-Gharreb, WR, Abdel-Raheem, SM, Ibrahim, AM, et al. Prevalence and antimicrobial susceptibility of Campylobacter species with particular focus on the growth promoting, immunostimulant and anti-Campylobacter jejuni activities of eugenol and trans-cinnamaldehyde mixture in broiler chickens. Animals. (2022) 12:905. doi: 10.3390/ani12070905
9. Elmowalid, GAE, Ahmad, AAM, El-Hamid, MIA, Ibrahim, D, Wahdan, A, El Oksh, AS, et al. Nigella sativa Extract Potentially Inhibited Methicillin Resistant Staphylococcus aureus Induced Infection in Rabbits: Potential Immunomodulatory and Growth Promoting Properties. Animals. (2022) 12:2635. doi: 10.3390/ani12192635
10. Ibrahim, D, Sewid, AH, Arisha, AH, Abd El-Fattah, AH, Abdelaziz, AM, Al-Jabr, OA, et al. Influence of Glycyrrhiza glabra Extract on Growth, Gene Expression of Gut Integrity, and Campylobacter jejuni Colonization in Broiler Chickens. Front vet sci. (2020):7. doi: 10.3389/fvets.2020.612063
11. Kishawy, AT, Al-Khalaifah, HS, Nada, HS, Roushdy, EM, Zaglool, AW, Ahmed Ismail, T, et al. Black Pepper or Radish Seed Oils in a New Combination of Essential Oils Modulated Broiler Chickens’ Performance and Expression of Digestive Enzymes, Lipogenesis, Immunity, and Autophagy-Related Genes. Vet Sci. (2022) 9:43. doi: 10.3390/vetsci9020043
12. Abd El-Hamid, MI, Ibrahim, D, Hamed, RI, Nossieur, HH, Elbanna, MH, Baz, H, et al. Modulatory Impacts of Multi-Strain Probiotics on Rabbits’ Growth, Nutrient Transporters, Tight Junctions and Immune System to Fight against Listeria monocytogenes Infection. Animals. (2022) 12:2082. doi: 10.3390/ani12162082
13. El-Ghareeb, WR, Kishawy, AT, Anter, RG, Aboelabbas Gouda, A, Abdelaziz, WS, Alhawas, B, et al. Novel Antioxidant Insights of Myricetin on the Performance of Broiler Chickens and Alleviating Experimental Infection with Eimeria spp.: Crosstalk between Oxidative Stress and Inflammation. Antioxidants. (2023) 12:1026. doi: 10.3390/antiox12051026
14. Ibrahim, D, Eldemery, F, Metwally, AS, Abd-Allah, EM, Mohamed, DT, Ismail, TA, et al. Dietary Eugenol Nanoemulsion Potentiated Performance of Broiler Chickens: Orchestration of Digestive Enzymes, Intestinal Barrier Functions and Cytokines Related Gene Expression With a Consequence of Attenuating the Severity of E. coli O78 Infection. Front Vet Sci. (2022) 540:847850. doi: 10.3389/fvets.2022.847580
15. Feng, J, Li, Z, Ma, H, Yue, Y, Hao, K, Li, J, et al. Quercetin alleviates intestinal inflammation and improves intestinal functions via modulating gut microbiota composition in LPS-challenged laying hens. Poult Sci. (2023) 102:102433. doi: 10.1016/j.psj.2022.102433
16. Hoque, MR, and Kim, IH. Effect of quercetin supplementation on growth performance, nutrient digestibility, excreta bacterial count, noxious gas emission, and meat quality of broilers when fed different protein diets. Can J Anim Sci. (2023) 103:185–91. doi: 10.1139/cjas-2021-0122
17. Goliomytis, M, Tsoureki, D, Simitzis, P, Charismiadou, M, Hager-Theodorides, A, and Deligeorgis, S. The effects of quercetin dietary supplementation on broiler growth performance, meat quality, and oxidative stability. Poult Sci. (2014) 93:1957–62. doi: 10.3382/ps.2013-03585
18. Alhawas, B, Abd El-Hamid, MI, Hassan, Z, Ibrahim, GA, Neamat-Allah, AN, El-Ghareeb, WR, et al. Curcumin loaded liposome formulation: Enhanced efficacy on performance, flesh quality, immune response with defense against Streptococcus agalactiae in Nile tilapia (Orechromis niloticus). Fish Shellfish Immunol. (2023) 138:108776. doi: 10.1016/j.fsi.2023.108776
19. Ibrahim, D, Al-Khalaifah, HS, Abdelfattah-Hassan, A, Eldoumani, H, Khater, SI, Arisha, AH, et al. Promising Role of Growth Hormone-Boosting Peptide in Regulating the Expression of Muscle-Specific Genes and Related MicroRNAs in Broiler Chickens. Animals. (2021) 11:1906. doi: 10.3390/ani11071906
20. Basharat, S, Tahir, SK, Majeed, KA, Yousaf, MS, Hussain, KK, Rashid, MA, et al. Effects of Iron Oxide Nanoparticle Supplementation on the Growth Performance, Serum Metabolites, Meat Quality, and Jejunal Basal Morphology in Broilers. Animals. (2023) 14:99. doi: 10.3390/ani14010099
21. Mohan, P, and Mala, R. Comparative antibacterial activity of magnetic iron oxide nanoparticles synthesized by biological and chemical methods against poultry feed pathogens. Materials Res Express. (2019) 6:115077. doi: 10.1088/2053-1591/ab4964
22. Ahn, T, Kim, JH, Yang, H-M, Lee, JW, and Kim, J-D. Formation pathways of magnetite nanoparticles by coprecipitation method. J Phys Chem C. (2012) 116:6069–76. doi: 10.1021/jp211843g
23. Aviagen, WR. 308: Broiler’s Management and Nutrition Specification. ROSS. AOAC International Aviagen Inc: An Aviagen Brand (2018).
24. AOAC. (2012). Official Method of Analysis: Association of Analytical Chemists. 19th Edition, Washington: Scientific research, 121–130.
25. Sun, S, Li, Y, Guo, Q, Shi, C, Yu, J, and Ma, L. In vitro interactions between tacrolimus and azoles against Candida albicans determined by different methods. Antimicrob Agents Chemother. (2008) 52:409–17. doi: 10.1128/AAC.01070-07
26. Rege, N, and Dahanukar, S. Quantitation of microbicidal activity of mononuclear phagocytes: an in vitro technique. J Postgrad Med. (1993) 39:22–5.
27. Lin, S, Pan, Y, Luo, L, and Luo, L. Effects of dietary β-1, 3-glucan, chitosan or raffinose on the growth, innate immunity and resistance of koi (Cyprinus carpio koi). Fish Shellfish Immunol. (2011) 31:788–94. doi: 10.1016/j.fsi.2011.07.013
28. Belote, B, Tujimoto-Silva, A, Hümmelgen, P, Sanches, A, Wammes, J, Hayashi, R, et al. Histological parameters to evaluate intestinal health on broilers challenged with Eimeria and Clostridium perfringens with or without enramycin as growth promoter. Poult Sci. (2018) 97:2287–94. doi: 10.3382/ps/pey064
29. Immerseel, FV, Buck, JD, Pasmans, F, Huyghebaert, G, Haesebrouck, F, and Ducatelle, R. Clostridium perfringens in poultry: an emerging threat for animal and public health. Avian Pathol. (2004) 33:537–49. doi: 10.1080/03079450400013162
30. Abd el-Hamid, MI, Ibrahim, D, Elazab, ST, Gad, WM, Shalaby, M, el-Neshwy, WM, et al. Tackling strong biofilm and multi-virulent vancomycin-resistant Staphylococcus aureus via natural alkaloid-based porous nanoparticles: perspective towards near future eradication. Front Cell Infect Microbiol. (2024) 13:1287426. doi: 10.3389/fcimb.2023.1287426
31. Abd El-Hamid, MI, El-Malt, RMS, Khater, SI, Abdelwarith, AA, Khamis, T, Abd El-Wahab, RA, et al. Impact of Liposomal Hesperetin in Broilers: Prospects for Improving Performance, Antioxidant Potential, Immunity, and Resistance against Listeria monocytogenes. Avian Pathol. (2024) 1–57. doi: 10.1080/03079457.2024.2395357
32. Abdel-Rahman, HG, Alian, HA, and Mahmoud, MM. Impacts of dietary supplementation with nano-iron and methionine on growth, blood chemistry, liver biomarkers, and tissue histology of heat-stressed broiler chickens. Trop Anim Health Prod. (2022) 54:126. doi: 10.1007/s11250-022-03130-w
33. Abdel-Latif, MA, Elbestawy, AR, El-Far, AH, Noreldin, AE, Emam, M, Baty, RS, et al. Quercetin dietary supplementation advances growth performance, gut microbiota, and intestinal mrna expression genes in broiler chickens. Animals. (2021) 11:2302. doi: 10.3390/ani11082302
34. Minaei, A, Sabzichi, M, Ramezani, F, Hamishehkar, H, and Samadi, N. Co-delivery with nano-quercetin enhances doxorubicin-mediated cytotoxicity against MCF-7 cells. Mol Biol Rep. (2016) 43:99–105. doi: 10.1007/s11033-016-3942-x
35. Nikonov, I, Folmanis, YG, Folmanis, G, Kovalenko, L, Laptev, GY, Egorov, I, et al. Iron nanoparticles as a food additive for poultry. Proceed Dokl Biol Sci. (2011) 440:328–31. doi: 10.1134/S0012496611050188
36. Almeldin, YA, Eldlebshany, AE, Elkhalek, EA, Abdel-Wareth, AA, and Lohakare, J. The effect of combining green iron nanoparticles and algae on the sustainability of broiler production under heat stress conditions. Front Vet Sci. (2024) 11:1359213. doi: 10.3389/fvets.2024.1359213
37. Ghasemi, Z, Abdi, V, and Sourinejad, I. Green fabrication of Ag/AgCl@ TiO2 superior plasmonic nanocomposite: Biosynthesis, characterization and photocatalytic activity under sunlight. J Alloys Compd. (2020) 841:155593. doi: 10.1016/j.jallcom.2020.155593
38. Amanzadeh, E, Esmaeili, A, Abadi, REN, Kazemipour, N, Pahlevanneshan, Z, and Beheshti, S. Quercetin conjugated with superparamagnetic iron oxide nanoparticles improves learning and memory better than free quercetin via interacting with proteins involved in LTP. Sci Rep. (2019) 9:6876. doi: 10.1038/s41598-019-43345-w
39. Rood, JI, Keyburn, AL, and Moore, RJ. NetB and necrotic enteritis: the hole movable story. Avian Pathol. (2016) 45:295–301. doi: 10.1080/03079457.2016.1158781
40. Savva, CG, Clark, AR, Naylor, CE, Popoff, MR, Moss, DS, Basak, AK, et al. The pore structure of Clostridium perfringens epsilon toxin. Nat Commun. (2019) 10:2641. doi: 10.1038/s41467-019-10645-8
41. Al-Khalaifah, HS, Shahin, SE, Omar, AE, Mohammed, HA, Mahmoud, HI, and Ibrahim, D. Effects of graded levels of microbial fermented or enzymatically treated dried brewer's grains on growth, digestive and nutrient transporter genes expression and cost effectiveness in broiler chickens. BMC Vet Res. (2020) 16:1–15. doi: 10.1186/s12917-020-02603-0
42. Omar, AE, Al-Khalaifah, HS, Ismail, TA, Abd El-Aziz, RM, El-Mandrawy, SA, Shalaby, SI, et al. Performance, serum biochemical and immunological parameters, and digestive enzyme and intestinal barrier-related gene expression of broiler chickens fed fermented fava bean by-products as a substitute for conventional feed. Front Vet Sci. (2021) 8:696841. doi: 10.3389/fvets.2021.696841
43. Gabrielyan, L, Hovhannisyan, A, Gevorgyan, V, Ananyan, M, and Trchounian, A. Antibacterial effects of iron oxide (Fe3O4) nanoparticles: distinguishing concentration-dependent effects with different bacterial cells growth and membrane-associated mechanisms. Appl Microbiol Biotechnol. (2019) 103:2773–82. doi: 10.1007/s00253-019-09653-x
44. Khatami, M, Alijani, H, Sharifi, I, Sharifi, F, Pourseyedi, S, Kharazi, S, et al. Leishmanicidal activity of biogenic Fe3O4 nanoparticles. Sci Pharm. (2017) 85:36. doi: 10.3390/scipharm85040036
45. Besednova, NN, Andryukov, BG, Zaporozhets, TS, Kryzhanovsky, SP, Kuznetsova, TA, Fedyanina, LN, et al. Algae polyphenolic compounds and modern antibacterial strategies: Current achievements and immediate prospects. Biomedicine. (2020) 8:342. doi: 10.3390/biomedicines8090342
46. Wang, L, Hu, C, and Shao, L. The antimicrobial activity of nanoparticles: present situation and prospects for the future. Int J Nanomedicine. (2017) 14:1227–49. doi: 10.2147/IJN.S121956
47. Hasany, S, Abdurahman, N, Sunarti, A, and Jose, R. Magnetic iron oxide nanoparticles: chemical synthesis and applications review. Curr Nanosci. (2013) 9:561–75. doi: 10.2174/15734137113099990085
48. Xu, C, Akakuru, OU, Zheng, J, and Wu, A. Applications of iron oxide-based magnetic nanoparticles in the diagnosis and treatment of bacterial infections. Front Bioeng Biotechnol. (2019) 7:141. doi: 10.3389/fbioe.2019.00141
49. Lee, C, Kim, JY, Lee, WI, Nelson, KL, Yoon, J, and Sedlak, DL. Bactericidal effect of zero-valent iron nanoparticles on Escherichia coli. Environ Sci Technol. (2008) 42:4927–33. doi: 10.1021/es800408u
50. Taylor, EN, and Webster, TJ. The use of superparamagnetic nanoparticles for prosthetic biofilm prevention. Int J Nanomedicine. (2009) 4:145–52. doi: 10.2147/IJN.S5976
51. Arokiyaraj, S, Saravanan, M, Prakash, NU, Arasu, MV, Vijayakumar, B, and Vincent, S. Enhanced antibacterial activity of iron oxide magnetic nanoparticles treated with Argemone mexicana L. leaf extract: an in vitro study. Mater Res Bull. (2013) 48:3323–7. doi: 10.1016/j.materresbull.2013.05.059
52. Alangari, A, Alqahtani, MS, Mateen, A, Kalam, MA, Alshememry, A, Ali, R, et al. Iron Oxide Nanoparticles: Preparation, Characterization, and Assessment of Antimicrobial and Anticancer Activity. Adsorpt Sci Technol. (2022) 2022:1562051. doi: 10.1155/2022/1562051
53. Li, W, Wei, W, Wu, X, Zhao, Y, and Dai, H. The antibacterial and antibiofilm activities of mesoporous hollow Fe 3 O 4 nanoparticles in an alternating magnetic field. Biomater Sci. (2020) 8:4492–507. doi: 10.1039/D0BM00673D
54. Lee, W-T, Wu, Y-N, Chen, Y-H, Wu, S-R, Shih, T-M, Li, T-J, et al. Octahedron iron oxide nanocrystals prohibited Clostridium difficile spore germination and attenuated local and systemic inflammation. Sci Rep. (2017) 7:8124. doi: 10.1038/s41598-017-08387-y
55. Endale, M, Park, S-C, Kim, S, Kim, S-H, Yang, Y, Cho, JY, et al. Quercetin disrupts tyrosine-phosphorylated phosphatidylinositol 3-kinase and myeloid differentiation factor-88 association, and inhibits MAPK/AP-1 and IKK/NF-κB-induced inflammatory mediators production in RAW 264.7 cells. Immunobiology. (2013) 218:1452–67. doi: 10.1016/j.imbio.2013.04.019
56. Yang, J, Maria, T, Zhou, B, Xiao, F, Wang, M, Mao, Y, et al. Quercetin improves immune function in Arbor Acre broilers through activation of NF-κB signaling pathway. Poult Sci. (2020) 99:906–13. doi: 10.1016/j.psj.2019.12.021
57. Qi, W, Qi, W, Xiong, D, and Long, M. Quercetin: its antioxidant mechanism, antibacterial properties and potential application in prevention and control of toxipathy. Molecules. (2022) 27:6545. doi: 10.3390/molecules27196545
58. Nikolic, P, and Mudgil, P. The cell wall, cell membrane and virulence factors of Staphylococcus aureus and their role in antibiotic resistance. Microorganisms. (2023) 11:259. doi: 10.3390/microorganisms11020259
59. Lee, K-W, and Lillehoj, HS. Role of Clostridium perfringens necrotic enteritis B-like toxin in disease pathogenesis. Vaccine. (2021) 10:61. doi: 10.3390/vaccines10010061
60. Feijo, JC, Vieira, SL, Horn, RM, Altevogt, WE, and Tormes, G. Iron requirements of broiler chickens as affected by supplemental phytase. J Anim Sci. (2023) 101:skad265. doi: 10.1093/jas/skad265
61. Brady, K, Porter, TE, Liu, H-C, and Long, JA. Characterization of gene expression in the hypothalamo-pituitary-gonadal axis during the preovulatory surge in the turkey hen. Poult Sci. (2019) 98:7041–9. doi: 10.3382/ps/pez437
62. Hassanpour, H, Yazdani, A, Khabir Soreshjani, K, and Asgharzadeh, S. Evaluation of endothelial and inducible nitric oxide synthase genes expression in the heart of broiler chickens with experimental pulmonary hypertension. Br Poult Sci. (2009) 50:725–32. doi: 10.1080/00071660903141005
63. Gharib-Naseri, K, Dorigam, JC, Doranalli, K, Morgan, N, Swick, RA, Choct, M, et al. Bacillus amyloliquefaciens CECT 5940 improves performance and gut function in broilers fed different levels of protein and/or under necrotic enteritis challenge. Animal Nutrit. (2021) 7:185–97. doi: 10.1016/j.aninu.2020.05.004
64. Mamun, M, Parvej, M, Ahamed, S, Hassan, J, Nazir, K, Nishikawa, Y, et al. Prevalence and characterization of shigatoxigenic Escherichia coli in broiler birds in Mymensingh. Bangladesh J Vet Med. (2016) 14:5–8. doi: 10.3329/bjvm.v14i1.28809
65. Rinttilä, T, Kassinen, A, Malinen, E, Krogius, L, and Palva, A. Development of an extensive set of 16S rDNA-targeted primers for quantification of pathogenic and indigenous bacteria in faecal samples by real-time PCR. J Appl Microbiol. (2004) 97:1166–77. doi: 10.1111/j.1365-2672.2004.02409.x
66. Bartosch, S, Fite, A, Macfarlane, GT, and McMurdo, ME. Characterization of bacterial communities in feces from healthy elderly volunteers and hospitalized elderly patients by using real-time PCR and effects of antibiotic treatment on the fecal microbiota. Appl Environ Microbiol. (2004) 70:3575–81. doi: 10.1128/AEM.70.6.3575-3581.2004
67. Walter, J, Hertel, C, Tannock, GW, Lis, CM, Munro, K, and Hammes, WP. Detection of Lactobacillus, Pediococcus, Leuconostoc, and Weissella species in human feces by using group-specific PCR primers and denaturing gradient gel electrophoresis. Appl Environ Microbiol. (2001) 67:2578–85. doi: 10.1128/AEM.67.6.2578-2585.2001
68. Mahamat Abdelrahim, A, Radomski, N, Delannoy, S, Djellal, S, Le Négrate, M, Hadjab, K, et al. Large-scale genomic analyses and toxinotyping of Clostridium perfringens implicated in foodborne outbreaks in France. Front Microbiol. (2019) 10:777. doi: 10.3389/fmicb.2019.00777
69. Sun, Z, Lu, M, Lillehoj, H, Lee, Y, Goo, D, Yuan, B, et al. Characterization of Collagen Binding Activity of Clostridium perfringens Strains Isolated from Broiler Chickens. Pathogens. (2023) 12:778. doi: 10.3390/pathogens12060778
70. Ibrahim, D, Rahman, MMIA, Abd El-Ghany, AM, Hassanen, EA, Al-Jabr, OA, Abd El-Wahab, RA, et al. (2024). Chlorella vulgaris extract conjugated magnetic iron nanoparticles in nile tilapia (Oreochromis niloticus): Growth promoting, immunostimulant and antioxidant role and combating against the synergistic infection with Ichthyophthirius multifiliis and Aeromonas hydrophila. Fish & Shellfish Immunology. 145, 109352.
71. Wade, B, Keyburn, AL, Haring, V, Ford, M, Rood, JI, and Moore, RJ. The adherent abilities of Clostridium perfringens strains are critical for the pathogenesis of avian necrotic enteritis. Vet. Microbiol. (2016) 197:53–61. doi: 10.1016/j.vetmic.2016.10.028
72. Sun, D, Li, C, Chen, S, and Zhang, X. Emerging role of dendritic cell intervention in the treatment of inflammatory bowel disease. BioMed Research International. (2022) 7025634.
73. Alizadeh-Ghamsari, AH, Shaviklo, AR, and Hosseini, SA. Effects of a new generation of fish protein hydrolysate on performance, intestinal microbiology, and immunity of broiler chickens. Journal of Animal Science and Technology. (2023) 65:804.
74. Dal Pont, GC, Lee, A, Bortoluzzi, C, Junior, NR, Farnell, YZ, Pilla, R, et al. Distillers dried grains with soluble and enzyme inclusion in the diet effects broilers performance, intestinal health, and microbiota composition. Poultry Science. (2023) 102:102981.
75. Naito, Y, Takagi, T, and Higashimura, Y. Heme oxygenase-1 and anti-inflammatory M2 macrophages. Archives of biochemistry and biophysics. (2014) 564:83–88.
76. Zanella, D, Bossi, E, Gornati, R, Bastos, C, Faria, N, and Bernardini, G. Iron oxide nanoparticles can cross plasma membranes. Scientific Reports. (2017) 7:11413.
77. Sun, X, and Jia, Z. Microbiome modulates intestinal homeostasis against inflammatory diseases. Vet. Immunol. Immunopathol. (2018) 205:97–105.
78. Ismail, H, Ibrahim, D, El Sayed, S, Wahdan, A, El-Tarabili, RM, Rizk El-Ghareeb, W, et al. “Prospective application of nanoencapsulated Bacillus amyloliquefaciens on broiler chickens’ performance and gut health with efficacy against Campylobacter jejuni colonization”. Animals. (2023) 13:775.
79. Abd El-Emam, MM, Mostafa, M, Farag, AA, Youssef, HS, El-Demerdash, AS, Bayoumi, H, et al. “The potential effects of quercetin-loaded nanoliposomes on amoxicillin/clavulanate-induced hepatic damage: targeting the Sirt1/Nrf2/Nf-κB signaling pathway and microbiota modulation”. Antioxidants. (2023) 12:1487.
Keywords: quercetin, magnetite, necrotic enteritis, antimicrobial peptide, intestinal antioxidant defense, inflammation
Citation: Al-Nasser A, El-Demerdash AS, Ibrahim D, Abd El-Hamid MI, Al-Khalaifah HS, El-borady OM, Shukry E, El-Azzouny MM, Ibrahim MS, Badr S, Elshater NS, Ismail TA and El Sayed S (2024) Innovative unified impact of magnetite iron nanoparticles and quercetin on broiler chickens: performance, antioxidant and immune defense and controlling of Clostridium perfringens infection. Front. Vet. Sci. 11:1474942. doi: 10.3389/fvets.2024.1474942
Received: 02 August 2024; Accepted: 25 September 2024;
Published: 07 November 2024.
Edited by:
Iram Liaqat, Government College University, PakistanReviewed by:
Awais Khalid, Hazara University, PakistanCopyright © 2024 Al-Nasser, El-Demerdash, Ibrahim, Abd El-Hamid, Al-Khalaifah, El-borady, Shukry, El-Azzouny, Ibrahim, Badr, Elshater, Ismail and El Sayed. This is an open-access article distributed under the terms of the Creative Commons Attribution License (CC BY). The use, distribution or reproduction in other forums is permitted, provided the original author(s) and the copyright owner(s) are credited and that the original publication in this journal is cited, in accordance with accepted academic practice. No use, distribution or reproduction is permitted which does not comply with these terms.
*Correspondence: Doaa Ibrahim, ZG9pYnJhaGltQHZldC56dS5lZHUuZWc=
Disclaimer: All claims expressed in this article are solely those of the authors and do not necessarily represent those of their affiliated organizations, or those of the publisher, the editors and the reviewers. Any product that may be evaluated in this article or claim that may be made by its manufacturer is not guaranteed or endorsed by the publisher.
Research integrity at Frontiers
Learn more about the work of our research integrity team to safeguard the quality of each article we publish.