- Laboratory of Veterinary Mycoplasmology, College of Veterinary Medicine, Southwest University, Chongqing, China
Accurate detection is a prerequisite for effective prevention and control of Mycoplasma synoviae infection. ELISA is the most popular method for the clinical detection of M. synoviae because of its convenience, low cost, and high detection rate. However, the cross-reactivity of commercially available ELISA kits with other avian pathogen-positive sera needs to be addressed. The aim of this study was to establish an ELISA method with high specificity for the detection of anti-M. synoviae antibodies in chicken serum to evaluate the M. synoviae infection status on poultry farms. The recombinant MS087 (rMS087) protein was expressed in Escherichia coli BL21 (DE3) and purified by Ni2+ affinity chromatography. An antibody against rMS087 was generated by immunizing BALB/c mice. Bioinformatic analysis revealed that MS087 was conserved among M. synoviae strains. Western blotting and indirect immunofluorescence results indicated that MS087 was not only localized in the cytoplasm and on the membrane but also secreted by the organism. For the established ELISA method based on rMS087, the optimal antigen concentration, blocking buffer, blocking duration, serum dilution, serum incubation duration, secondary antibody dilution, secondary antibody incubation duration and colorimetric reaction duration were 2 μg/mL, 1% BSA, 3 h, 1:500, 1.5 h, 1:20,000, 2 h and 5 min, respectively. Validation of the rMS087-based ELISA revealed a cut-off value of 0.5. The coefficients of variation of both the intra-batch and inter-batch methods were less than 9%. The assay was able to differentiate positive serum against M. synoviae from antisera against nine other avian pathogens and was able to recognize M. synoviae-positive sera at a dilution of 1:1,000. Compared with the commercial ELISA method, the rMS087-based ELISA has the potential to recognize more positive sera against M. synoviae. Collectively, the rMS087-based ELISA is a reproducible, specific, and sensitive serological method for detecting antibodies against M. synoviae in chicken serum and has robust potential for large-scale serological epidemiology of M. synoviae infection on poultry farms.
1 Introduction
Mycoplasma synoviae is a widespread pathogen in the poultry industry. It was first reported to be associated with the occurrence of infectious synovitis in chickens in the USA in the early 1950s (1) and was proven to be the causative organism for hemagglutination of red blood cells (2). In addition to acute/chronic respiratory disease, air sacculitis and/or articular lesions (3, 4), M. synoviae infection often results in reduced growth, production, and hatchability (5). Moreover, many studies (6–8) have described the association between the presence of M. synoviae in the oviduct and the production of eggs with eggshell apex abnormalities (EAA) by laying hens, characterized by an altered shell surface, shell thinning, increased translucency (detectable macroscopically, particularly upon candling), and the occurrence of cracks and breaks. M. synoviae is transmitted both horizontally and vertically, and its prevalence appears to be increasing worldwide (9). Since 2010, this pathogen has been widely prevalent in broiler flocks in mainland China (10, 11) and has subsequently rapidly spread to layer flocks (12).
Generally, M. synoviae infection can be controlled by three general approaches: biosecurity measures, medication with antimicrobials, and vaccination with commercial or autogenous vaccines (9). Several studies reported a temporary effect of antimicrobial treatments in EAA-affected layer flocks, with a decreased number of broken or downgraded eggs during treatment, but a disappearance of this effect 1–2 weeks after the end of treatment (6, 13) because the organism entered cells after infection (14–16). Although the live vaccine (MS-H) developed in Australia alleviates clinical symptoms and pathological damage and improves production performance in chickens (17, 18), it is used only in M. synoviae-free flocks and cannot block infection by wild-type strains (19, 20). Therefore, eradication measures, combined with biosafety regulations, constitute most cost-effective strategy for preventing and controlling M. synoviae infection.
In general, the most crucial step for the eradication of infectious disease is the use of appropriate diagnostic reagents. Serological tests are considered indispensable and cost-effective tools. Several serological tests, including rapid plate agglutination (RPA), hemagglutination inhibition (HI), and enzyme-linked immunosorbent assay (ELISA), have been developed for monitoring M. synoviae infection in chicken flocks (21–23). ELISA has been reported to have higher specificity than RPA and higher sensitivity than HI (21). Several ELISAs based on whole cells or membrane proteins have been developed to detect antibodies against M. synoviae (21, 24–26). However, the cross-reactivity and nonspecific reactions of these ELISAs with Mycoplasma gallisepticum have impeded the development of specific serodiagnostic tests (21, 25, 26).
A more specific ELISA was developed by using the MSPB protein, which is cleaved from the amino terminus of VlhA (27, 28), and the cross-reactivity of the method with sera against M. gallisepticum was overcome (28). However, the coating antigen shows a high degree of amino acid variability between strains (29) or even clonal isolates from a single strain (30), which affects the sensitivity of the established ELISA (27, 28). Recently, the membrane protein LP78, which binds to fibronectin and plasminogen, was used as the diagnostic antigen. Compared with commercial ELISA kits, although no cross-reactivity was observed with other poultry pathogen-positive sera, especially M. gallisepticum-positive sera, LP78-based ELISA demonstrated lower sensitivity in the detection of M. synoviae-positive serum samples (31). Therefore, it is necessary to develop a novel serological method with good specificity and sensitivity for the diagnosis of M. synoviae infection.
In general, membrane proteins are commonly used as targets for serological diagnoses. We found a M. synoviae protein MS087, which is predicted to be an F1-like ATPase-associated subunit (32), was localized in both the cytoplasm and membrane and is even secreted from the organism. In this study, we used MS087 as the coating antigen to develop an indirect ELISA to detect antibodies in chicken serum against M. synoviae, and provide a specific tool for the investigation of the epidemiology of M. synoviae in chicken farms.
2 Materials and methods
2.1 Bacterial strains, plasmid, sera, and culture conditions
M. synoviae strain CQTL01 was isolated from the synovial fluid of a Three-Yellow broiler in China in 2022. The strain was subsequently grown in KM2 medium (Tuopu, Zhaoyuan, Shandong, China) supplemented with 20% porcine serum (Jianglai, Shanghai, China) and 0.01% NAD (Sangon Biotech, Shanghai, China) at 37°C. The Escherichia coli strains DH5α and BL21(DE3) were grown in Luria–Bertani (LB) broth or on solid medium. The pET-30a(+) expression vector was preserved by our laboratory. Three hundred and sixty-eight serum samples were collected from five commercial poultry farms and two poultry slaughterhouses and were assessed with a M. synoviae ELISA antibody test kit (IDEXX, Westbrook, ME, USA) (Table 1). Chicken sera against other avian pathogens, including M. gallisepticum, Avibacterium paragallinarum (AP) serovars A, B and C, Salmonella Pullorum-Gallinarum (SPG), Newcastle disease virus (NDV), and avian influenza virus (AIV) subtypes H5, H7 and H9, respectively, were purchased from Harbin National Engineering Research Center of the Veterinary Biologics Corp in China.
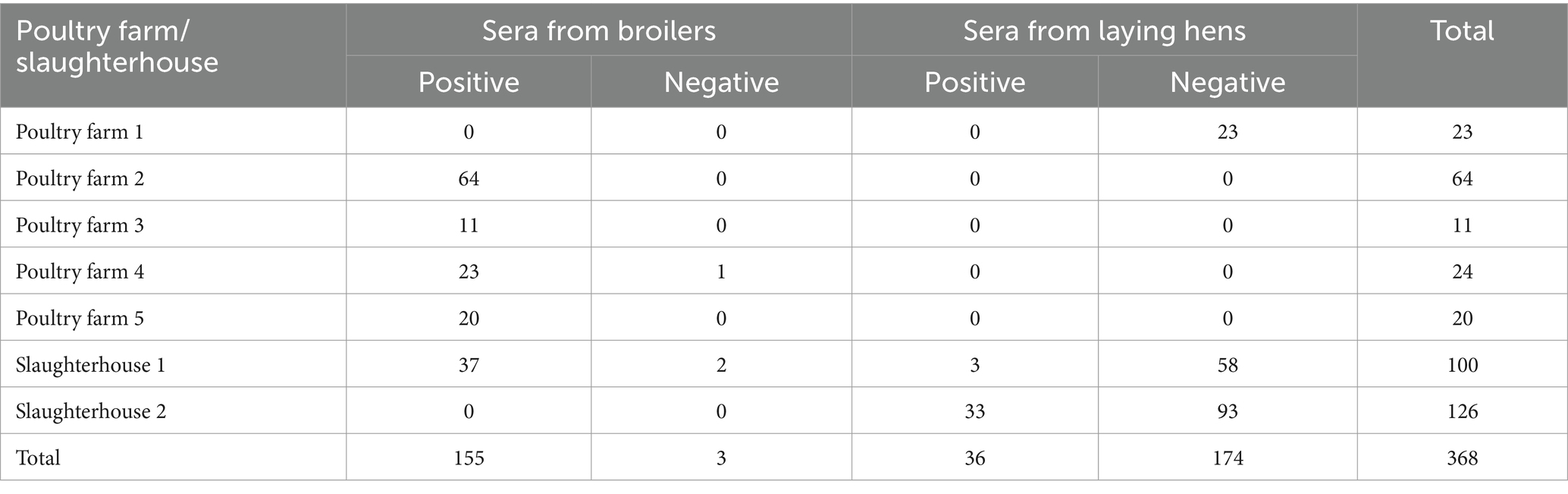
Table 1. Information on chicken sera collected from five poultry farms and two poultry slaughterhouses.
2.2 Bioinformatic analysis
The full-length sequence of the gene encoding ms087 in the CQTL01 strain was obtained from the genome sequence (The data have been deposited in China National Center for Bioinformation, run accession: CRR1309196). The molecular weight (MW) of MS087 was computed with Detaibio.1 BLASTP2 was used to carry out amino acid identity matching with sequences retrieved from the NCBI database. The predictor SignalP 6.0 (33) was used to detect the presence of the signal peptide. TMHMM 2.0 (34) was applied to predict transmembrane helices. The computational online software programs CELLO v.2.5 (35) and Gpos-mPLoc (36) were used to directly predict the subcellular localization of the protein. The immunogenicity of the protein was calculated by VaxiJen V2.0 (37) using the default parameters.
2.3 Cloning, expression and purification of M. synoviae rMS087
On the basis of the ms087 gene sequence from the CQTL01 strain, two primers (ms087-F: CGCGGATCCATGAAAATAAAAAAACT TTTATCTTTTGC and ms087-R: CCGCTCGAGATCATTTGC AAAATTAGTTAAATAAGT) were designed and synthesized. The genomic DNA of the CQTL01 strain was extracted using a TIANamp bacteria DNA kit (Tiangen, Beijing, China). The ms087 gene was amplified with ApexHF HS DNA Polymerase FS Master Mix (Accurate, Changsha, Hunan, China) by using the genomic DNA of CQTL01 as the template. After purification, the PCR product was digested with BamH I and XhoI and ligated to the expression vector pET-30a(+). The recombinant plasmid was subsequently transformed into E. coli DH5α and BL21 (DE3) competent cells via the heat shock method. Recombinant MS087 (rMS087) was expressed by induction with 1 mmol/L isopropyl β-D-1-thiogalactopyranoside (IPTG) at 16°C for 20 h on a shaker at 200 r·min−1. After induction, the expression and expression form of rMS087 in the recombinant bacteria were examined via 12% sodium dodecyl sulfate–polyacrylamide gel electrophoresis (SDS–PAGE) and Western blotting with a mouse anti-His tag antibody (Bioss, Beijing, China). Soluble rMS087 was purified with Ni-NTA HisTrap™HP (Cytiva, Shanghai, China), and the concentration of purified recombinant protein was measured via a BCA protein assay kit (Epizyme Biotech, Shanghai, China) according to the manufacturer’s instructions.
2.4 Raising polyclonal antisera against rMS087
Fifty micrograms of purified rMS087 protein at a concentration of 1 mg/mL emulsified with an equal volume of Freund’s complete adjuvant (BioFROXX, Beijing, China) was used to immunize female BALB/c mice aged 7 weeks via multipoint subcutaneous injection into groins and back. Booster dose of 50 μg of emulsified rMS087 protein with an equal volume of Freund’s incomplete adjuvant were applied on days 21 and 28 after the first immunization, and additional booster dose of 50 μg of purified rMS087 protein was applied on days 35 and 42 after the first immunization, respectively. The day before each immunization, blood samples were collected from the retro-orbital sinus. On day 49 after the first immunization, blood samples were collected from the eyeballs and the mice were euthanized. A specific antibody against rMS087 in sera were evaluated via indirect ELISA. Briefly, 96-well ELISA plates (Corning Incorporated, Kennebunk, ME, USA) were coated with 100 μL of purified rMS087 protein (0.5 μg/mL) in 0.05 mol/L carbonate buffer (pH 9.6) overnight at 4°C after incubation at 37°C for 1 h. Then, the plates were blocked with 5% skim milk diluted with PBS and subsequently incubated with serially diluted sera (from 1:500 to 1:2,048,000). The produced antisera were used for identification of the distribution of MS087 in M. synoviae. The protocols were approved by the Institutional Animal Care and Use Committee of Southwest University (IACUC No. IACUC-20240322-01).
2.5 Identification of the subcellular localization of MS087 in M. synoviae
To determine the distribution of MS087 in M. synoviae, an indirect immunofluorescence assay (IFA) and Western blotting were performed. Suspension IFA was performed as previously described (38). Briefly, 50 mL of M. synoviae strain CQTL01 cultured in the late logarithmic growth phase was collected and washed three times with PBS by centrifugation. Afterward, the cells were incubated with mouse anti-rMS087 polyclonal antiserum or preimmune serum diluted at 1:10,000 with PBS containing 0.5% bovine serum albumin (BSA) overnight at 4°C with shaking at 70 r·min−1. After washing, the cells were incubated with a 1:300 dilution of CoraLite488-conjugated goat anti-mouse IgG(H + L) (Proteintech, Wuhan, Hubei, China).
The cytoplasmic and membrane fractions of M. synoviae strain CQTL01 were separated using a membrane and cytoplasmic protein extraction kit (Biosharp, Hefei, Anhui, China) according to the manufacturer’s protocol. The supernatant of the culture medium after centrifugation was also collected. The cytoplasmic fraction and the culture supernatant were concentrated with a membrane and cytoplasmic protein extraction kit before SDS–PAGE. Proteins from different fractions were loaded onto an SDS–PAGE gel and subjected to electrophoresis. The proteins were subsequently transferred to a PVDF membrane. The membrane was blocked with 5% skim milk in TBST (pH 7.2) for 2 h at room temperature (RT). The membrane was then incubated with mouse anti-rMS087 polyclonal antiserum (1:10,000) in 5% skim milk diluted with TBST overnight at 4°C to block nonspecific binding. The samples were subsequently incubated with HRP-conjugated goat anti-mouse IgG (H + L) (1:10,000) (ABclonal, Wuhan, Hubei, China) at RT for 1 h. The protein bands were visualized using an ultra-enhanced chemiluminescence (ECL) reagent (Biosharp, Hefei, Anhui, China).
2.6 Optimization of the ELISA procedure and working conditions
Three M. synoviae-negative serum samples were collected from broilers, three M. synoviae-positive serum samples were obtained from broilers, and three M. synoviae-positive serum samples were drawn from laying hens. The status of the sera was confirmed with an IDEXX M. synoviae ELISA antibody test kit. Therefore, in this assay, three serum samples were used as negative controls and six serum samples were designated positive controls according to the results from the commercial ELISA antibody test kit.
Briefly, the wells of microtiter plates (Corning Incorporated, Kennebunk, ME, USA) were coated with 100 μL of rMS087 protein at concentrations from 0.05 μg/mL to 8 μg/mL in 0.05 mol/L carbonate buffer (pH 9.6) at 37°C for 1 h and then at 4°C overnight. After the unbound antigen was discarded, the wells were washed five times with PBS containing 0.05% Tween-20 (PBST). Nonspecific binding was blocked by incubation with 200 μL of PBST, 1% BSA, 1% ovalbumin (OVA), 2.5% skim milk, or 1% gelatin at 37°C for 0.5 h to 4 h. After five washes with PBST, 100 μL of each serum sample (diluted from 1:50 to 1:8,000 in blocking buffer) was added and incubated at 37°C for 0.5 h to 3 h. After five washes, 100 μL of HRP-conjugated goat anti-chicken IgG(H + L) secondary antibody (Bioss, Beijing, China) (diluted from 1:5,000 to 1:640,000) was added and incubated at 37°C for 0.5–3 h. After washing with PBST, 50 μL of substrate A (100 mL H2O containing 2.72 g of anhydrous sodium acetate, 0.35 g of citric acid monohydrate, 0.06 mL of 30% hydrogen peroxide) and substrate B (100 mL H2O containing 0.04 g of EDTA·Na2, 0.2078 g of citric acid monohydrate, 10 mL of glycerol, 0.0391 g of TMB·2HCl) (39) were added, the mixture was incubated at RT for 5–30 min, and the reaction was ended with the addition of 50 μL of 2 mol/L H2SO4. The optical density at 450 nm (OD450) was recorded with an ELISA plate reader (Thermo Fisher Scientific, Ratastie 2, FI-01620 Vantaa, Finland). Each experiment was performed at least three times, and all samples were assayed in triplicate. The working conditions were optimized by determining the highest M. synoviae-positive (P)-to-negative (N) serum OD450 ratios.
2.7 Calculation of the cut-off value
Three hundred and sixty-eight chicken serum samples were tested via the ELISA method established in this study, with three replicates for each serum sample. A receiver operating characteristic (ROC) curve was generated via GraphPad Prism 8.0 software on the basis of the average OD450 values determined for the serum samples, with the value generated at the maximum value of the Youden index was set as the cut-off value (40, 41).
2.8 Evaluation of reproducibility
Reproducibility, i.e., intra- and inter-assay variation, between runs was determined as described previously (39), with minor modifications. In brief, four M. synoviae-negative and four M. synoviae-positive serum samples identified by both the commercial ELISA antibody test kit and the rMS087-based ELISA antibody test method were selected randomly for reproducibility experiments. Five replicates of each sample in the same batch were chosen for the intra-assay (within plate) variation assessment, and three plates from different batches were chosen for the inter-assay (between runs) variation assessment. The coefficient of variations (CVs) were calculated.
2.9 Cross-reactivity with positive sera against other avian pathogens
The cross-reactivity of the established ELISA method with other avian pathogen-positive sera was investigated by using positive sera against M. gallisepticum, Salmonella Pullorum-Gallinarum, A. paragallinarum serovars A, B, and C, Newcastle disease virus, and avian influenza virus subtypes H5, H7 and H9. Two M. synoviae-negative and two M. synoviae-positive serum samples confirmed by both the IDEXX M. synoviae ELISA antibody test kit and the ELISA antibody test method established in this study were used as controls.
2.10 Maximum dilution of sera
Five M. synoviae-positive serum samples were diluted with blocking buffer as follows: 1:500, 1:1,000, 1:2,000, 1:4,000, 1:8,000, 1:16,000, 1:32,000, and 1:64,000. Then, ELISA was carried out with the optimal working conditions except for the optimal dilution of the serum. The maximum dilution of sera for the ELISA was assessed according to the cut-off value.
2.11 Serum test
Anti-M. synoviae antibodies were tested in 368 chicken serum samples via both a commercial ELISA antibody test kit and the rMS087-based ELISA antibody test method, with three replicates for each serum sample.
3 Results
3.1 Bioinformatic analysis
The full length of the ms087 gene in the M. synoviae CQTL01 strain was 606 bp, and the gene did not contain a TGA codon, encoding tryptophan in Mycoplasma. The amino acid sequence shared over 97% homology with proteins of M. synoviae strains deposited in the GenBank database and had no more than 40% homology with the proteins of other Mycoplasma species according to the BLAST analysis (Supplementary Figure S1). For example, the amino acid sequence of M. synoviae had no more than 32% homology with the amino acid sequence of M. meleagridis and had no more than 21% homology with the amino acid sequence of M. canis. The ORF was predicted to encode a protein containing 201 amino acids with a MW of 23 kDa. The amino acid sequence of the MS087 protein was predicted to possess a signal peptide cleaved by signal peptidase I of the Sec translocator (the cleavage site is between amino acid positions 24 and 25) but lacked a transmembrane domain. Both CELLO v.2.5 and Gpos-mPLoc calculated that MS087 could be secreted by the organism as an extracellular protein. However, MS087 was predicted to have an antigenicity score of 0.3061 (the threshold score for antigenicity is 0.4).
3.2 Expression and purification of the rMS087
The full-length ms087 gene was amplified from the CQTL01 strain via PCR (Figure 1A) and then cloned and inserted into the pET-30a(+) vector, which was subsequently successfully transformed into E. coli BL21(DE3) competent cells. After IPTG induction of the transformed cells, His-tagged rMS087 was expressed in soluble and inclusion body forms by the recombinant bacteria harboring the ms087 gene (Figures 1B,C) and the purity of soluble rMS087 after purification was confirmed by SDS–PAGE (Figure 1D).
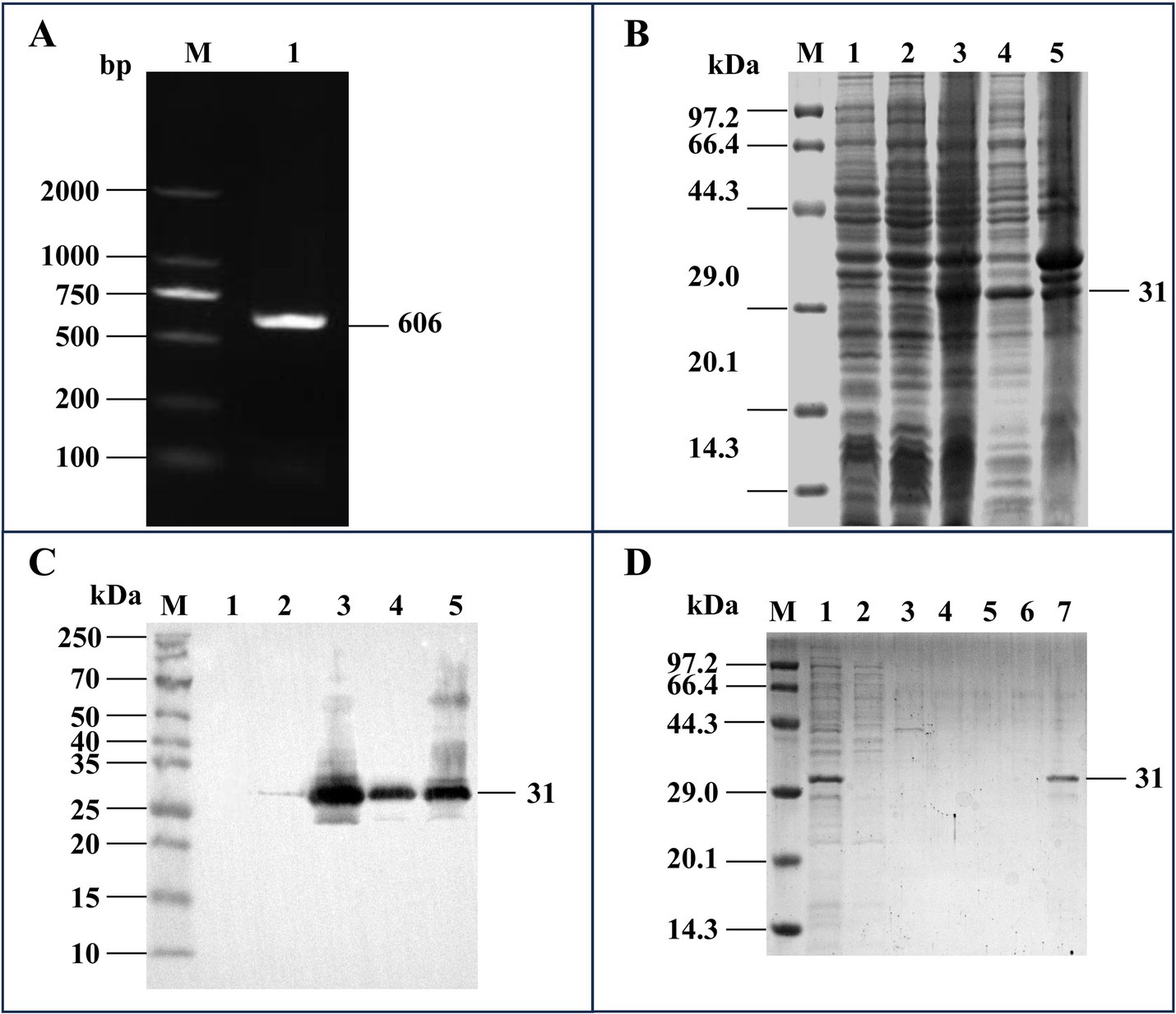
Figure 1. Cloning of the ms087 gene and expression and purification of the rMS087 protein. (A) PCR amplification of the ms087 gene by using the genomic DNA of the M. synoviae CQTL01 strain as the template. The electrophoresis was performed via an agarose gel. Lane M: DNA marker; lane 1: ms087 gene. (B) Identification of the expression form of rMS087 in recombinant bacteria by SDS–PAGE. (C) Identification of the expression form of rMS087 in recombinant bacteria by Western blotting with a mouse anti-His tag antibody. (B,C) Lane M: protein marker; lane 1: E. coli BL21 (DE3) containing the pET-30a(+) plasmid; lane 2: total cell lysate of the recombinant bacteria without IPTG induction; lane 3: total cell lysate of the recombinant bacteria induced by IPTG; lane 4: the supernatant of the cell lysate of the recombinant bacteria induced by IPTG; lane 5: the precipitate of the cell lysate of the recombinant bacteria induced by IPTG. (D) Recombinant MS087 protein purified by Ni2+ affinity chromatography. The supernatant of the cell lysate was loaded onto (Lane 1) and flowed through (Lane 2) the column. The column was subsequently washed with a linear imidazole gradient of 0.1 mol/L (Lane 3), 0.2 mol/L (Lane 4) and 0.3 mol/L (Lane 5), and the purified protein was collected (Lane 6). (B–D) Five microliters of sample (1 mg/mL) was loaded into each lane of the SDS–PAGE gel, and the proteins in the SDS–PAGE gel were stained with Coomassie Brilliant Blue staining (B,D).
3.3 Production of polyclonal antiserum against rMS087
Purified rMS087 was used to generate rMS087 antiserum by immunization of BALB/c mice. The titers of the anti-rMS087 antibody in the sera of 5 mice were 1:1,024,000, 1:512,000, 1:256,000, 1:25,600, and 1:512,000. Antiserum with an antibody titer of 1:1,024,000 was used randomly for the subsequent experiments, and this antiserum could react with rMS087 of the recombinant bacteria (Figure 2A).
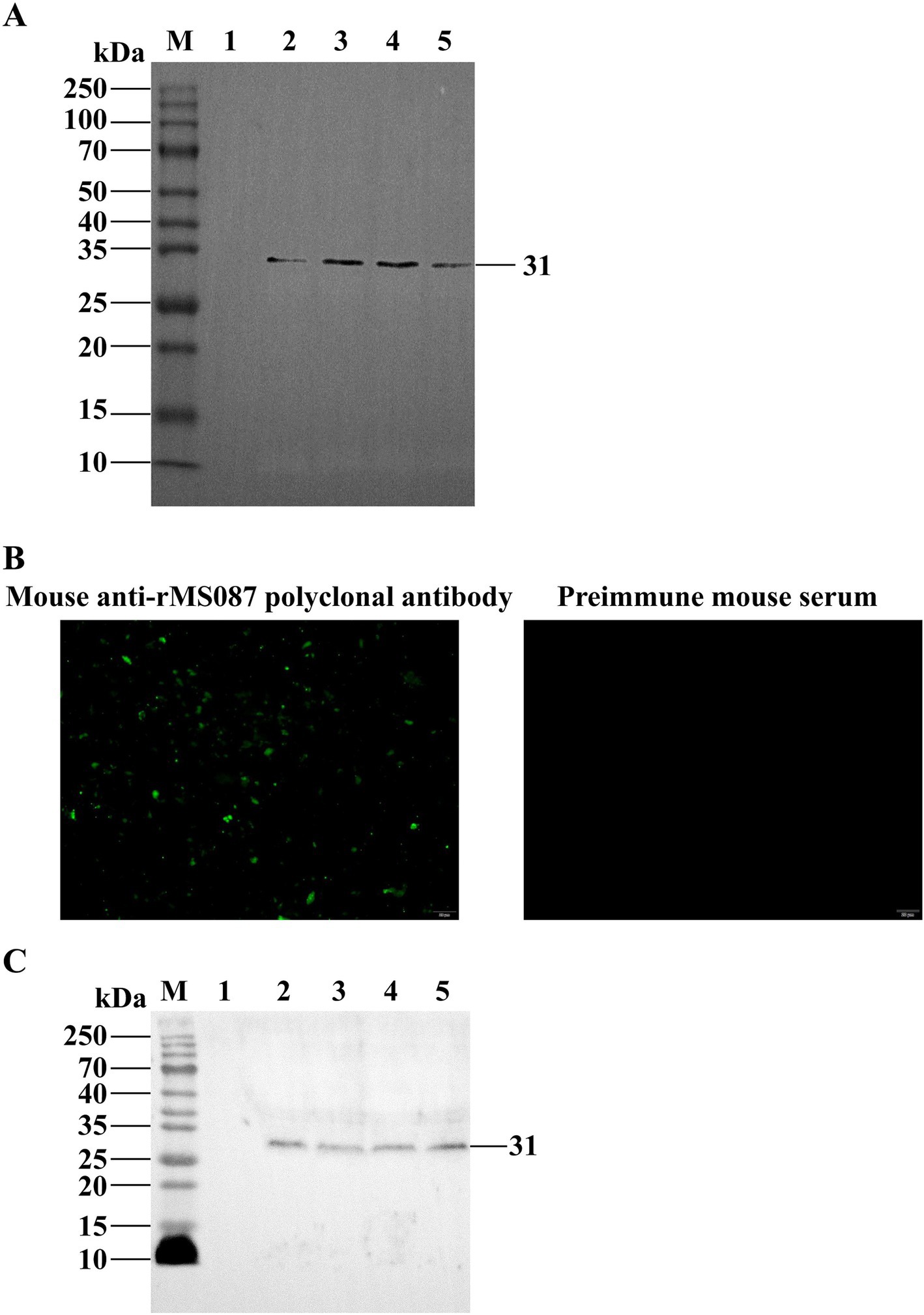
Figure 2. Identification of the subcellular localization of MS087 in M. synoviae by IFA and Western blotting. (A) Identification of the expression form of rMS087 in recombinant bacteria by Western blotting with a mouse anti-rMS087 antibody. Lane M: protein marker; lane 1: E. coli BL21 (DE3) containing the pET-30a(+) plasmid; lane 2: total cell lysate of the recombinant bacteria without IPTG induction; lane 3: total cell lysate of the recombinant bacteria induced by IPTG; lane 4: the supernatant of the cell lysate of the recombinant bacteria induced by IPTG; lane 5: the precipitate of the cell lysate of the recombinant bacteria induced by IPTG. (B) MS087 was localized on the surface of the M. synoviae strain CQTL01. (C) Analysis of the localization of MS087 by Western blotting. Lane M: protein marker; lane 1: whole cells; lane 2: cytoplasmic fraction; lane 3: membrane fraction; lane 4: culture medium supernatant. (A,C) Five microliters of sample (1 mg/mL) was loaded into each lane of the SDS–PAGE gel.
3.4 Distribution of MS087 in M. synoviae
IFA and Western blotting were performed to determine the distribution of MS087 in M. synoviae via the mouse anti-rMS087 polyclonal antiserum. In the suspension IFA, green fluorescent puncta were observed on the surface of the CQTL01 strain after incubation with mouse anti-rMS087 serum, whereas no fluorescent signal was observed after incubation with preimmune mouse serum (Figure 2B). These results implied that MS087 was localized on the surface of M. synoviae. The distribution of MS087 in M. synoviae was subsequently analyzed via Western blotting (Figure 2C). The mouse anti-rMS087 serum reacted with whole cell proteins, as well as membrane, cytoplasmic and extracellular proteins, at approximately 31 kDa, suggesting that MS087 is present in both the membrane and cytoplasm of M. synoviae and can even be secreted from the organism.
3.5 Optimization of the working conditions of the rMS087-based ELISA method for the detection of antiserum against M. synoviae
Optimization of the working conditions for the detection of anti-M. synoviae antibodies in chicken sera involves the optimal antigen concentration, blocking buffer, blocking duration, serum dilution, serum incubation duration, secondary antibody dilution, secondary antibody incubation duration, and colorimetric reaction duration. As shown in Figure 3A, 100 μL of purified rMS087, adjusted to a concentration of 2 μg/mL with 0.05 mol/L carbonate buffer, was added to each well. After the unbound antigen was discarded, 200 μL of 1% BSA diluted with PBS (Figure 3B) was used to block nonspecific binding at 37°C for 3 h (Figure 3C). The optimal dilution of serum with 1% BSA was 1:500 (Figure 3D), and the optimal incubation time for the serum samples was 1.5 h (Figure 3E). As shown in Figure 3F, the P/N ratio increased with increasing HRP-conjugated goat anti-chicken IgG (H + L) secondary antibody dilution, reached a maximum at 1:20,000 and subsequently decreased with increasing serum dilution. Moreover, the highest P/N ratio was obtained after incubation with the secondary antibody for 2 h (Figure 3G). Finally, the highest P/N value was obtained when the enzyme reacted with the substrate for 5 min (Figure 3H).
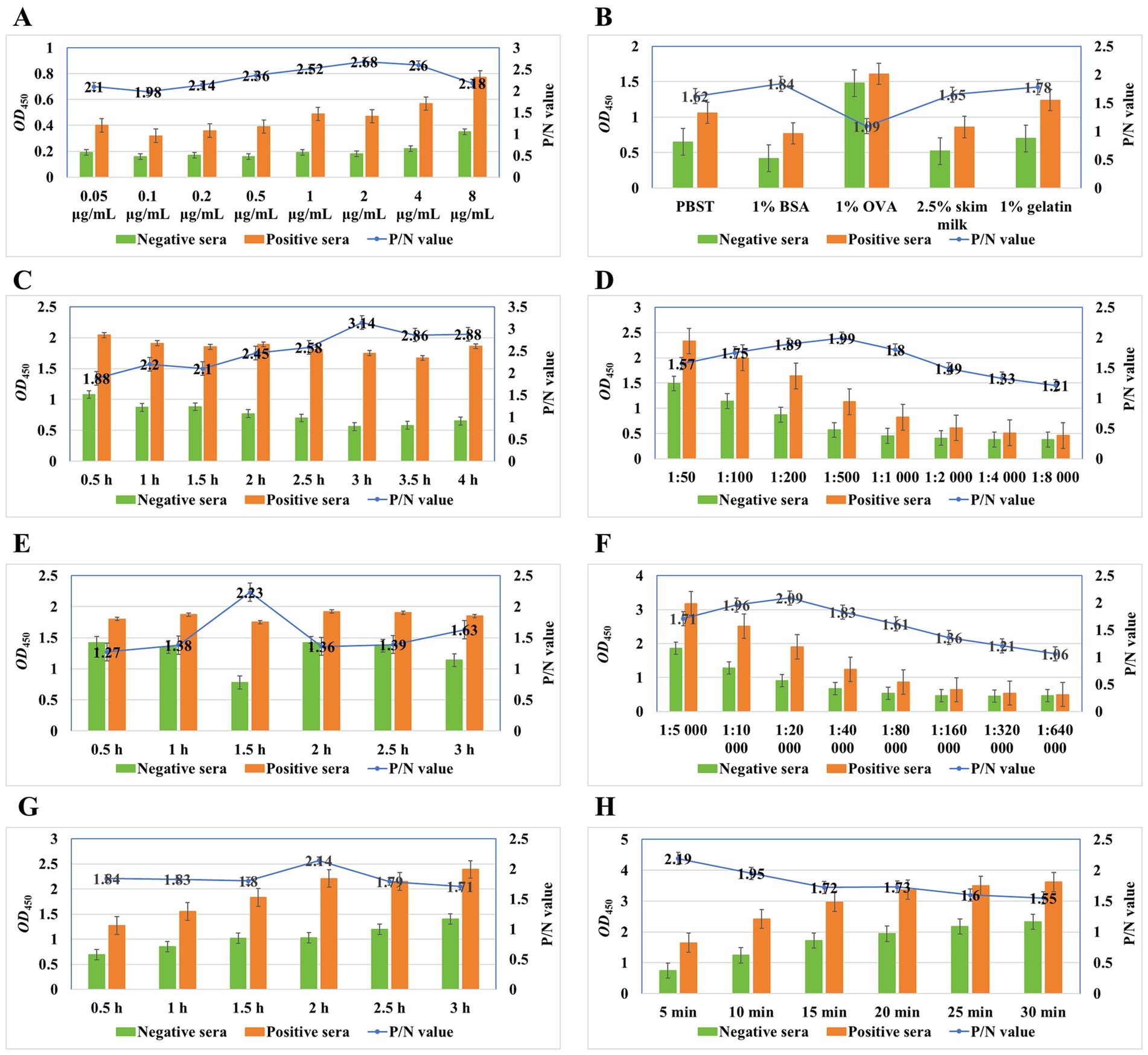
Figure 3. Optimal working conditions of the ELISA method for detection of anti-M. synoviae antibodies on the basis of the rMS087 protein. The optimal antigen concentration was 2 μg/mL in the coating buffer (A). The optimal blocking buffer was 1% BSA dissolved in PBS (B), and the optimal incubation duration for the blocking step was 3 h (C). The optimal dilutions of serum and secondary antibodies were 1:500 (D) and 1:20,000 (F) diluted in blocking buffer. The optimal incubation duration for the serum and secondary antibodies were 1.5 h (E) and 2 h (G), respectively. The optimal colorimetric reaction duration was determined after exposure to the substrate solution for 5 min (H).
3.6 Cut-off values for scoring ELISA-tested chicken sera
One hundred and fifty-eight serum samples from broilers and 210 serum samples from layers were assessed via the M. synoviae ELISA antibody method based on rMS087. The ROC curve was generated on the basis of the values obtained from 368 serum samples, with the maximum value of the Youden index as the cut-off value. The maximum Youden index was 0.958, and the cut-off value was 0.5, indicating that when the OD450 value of the serum to be tested was ≥0.50, the sample was considered as positive for anti-M. synoviae antibodies; when the OD450 value was <0.50, the sample was considered as negative for anti-M. synoviae antibodies.
3.7 Reproducibility, cross reactivity and maximum serum dilution of the M. synoviae ELISA antibody test method
The reproducibility of the rMS087-based ELISA was assessed by determining the intra- and inter-assay variation. The intra-assay CVs of 4 negative serum samples and four positive serum samples ranged from 1.32 to 5.50%, and the inter-assay CVs of these samples ranged from 4.01 to 8.77%, suggesting that the ELISA results are reproducible, yielding low and acceptable variation.
The ELISA exhibited no cross-reactivity with sera containing antibodies against nine avian pathogens (Figure 4). These data indicated that the ELISA was specific to anti-M. synoviae antibodies induced by natural infection and that there was no cross-reaction with antisera against other avian pathogens.
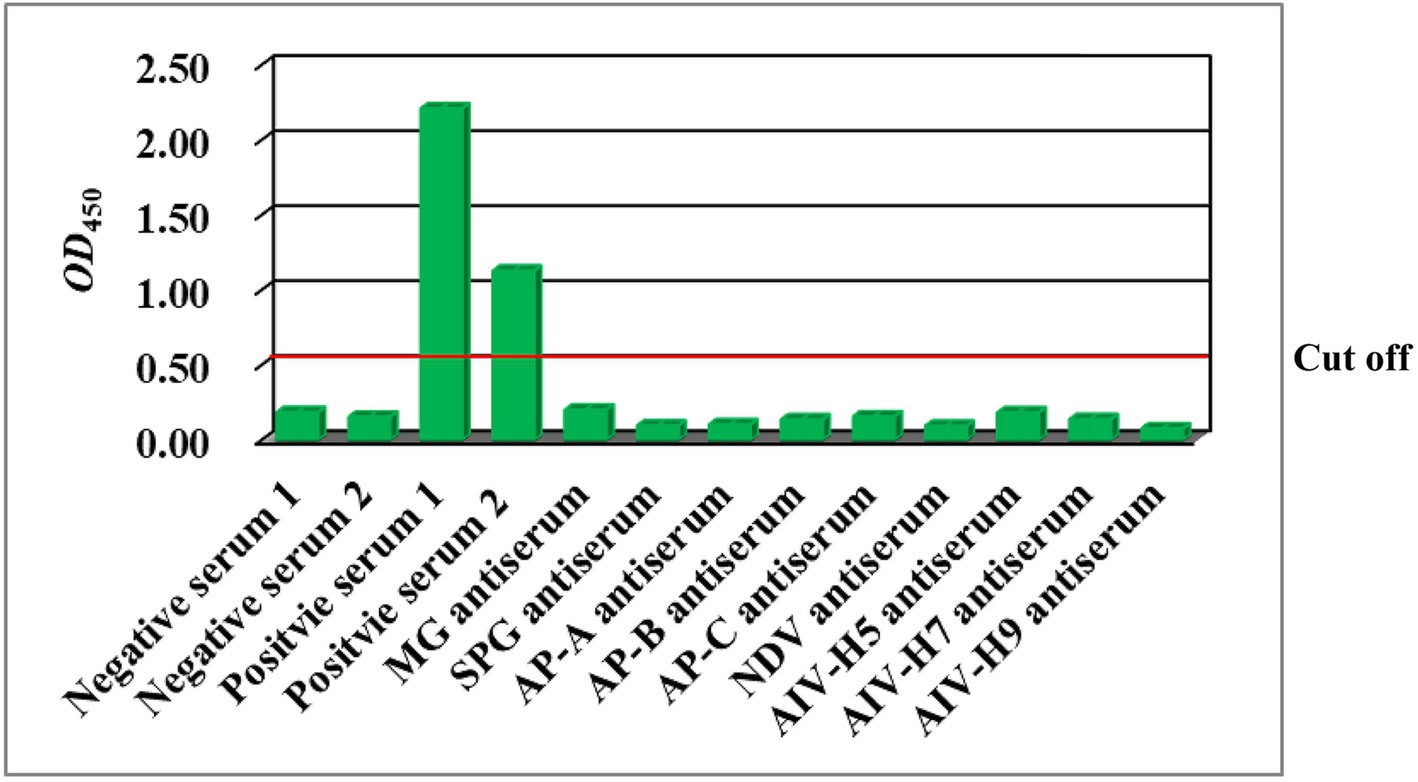
Figure 4. Results of the detection of positive sera against avian pathogens via the rMS087-based ELISA antibody test method. MG: M. gallisepticum; SPG: Salmonella Pullorum-Gallinarum; AP-A: A. paragallinarum serovar A; AP-B: A. paragallinarum serovar B; AP-C: A. paragallinarum serovar C; NDV: Newcastle disease virus; AIV-H5: avian influenza virus subtype H5; AIV-H7: avian influenza virus subtype H7; AIV-H9: avian influenza virus subtype H9.
As shown in Figure 5, with increasing dilution ratio of the serum, the OD450 value decreased gradually. Five serum samples at a dilution of 1:1,000, three at a dilution of 1:2,000, and one at a dilution of 1:4,000 were considered positive, whereas no serum sample at a dilution of 1:8,000 or more were considered positive, suggesting that the serum can be diluted up to 1,000 times when using this method.
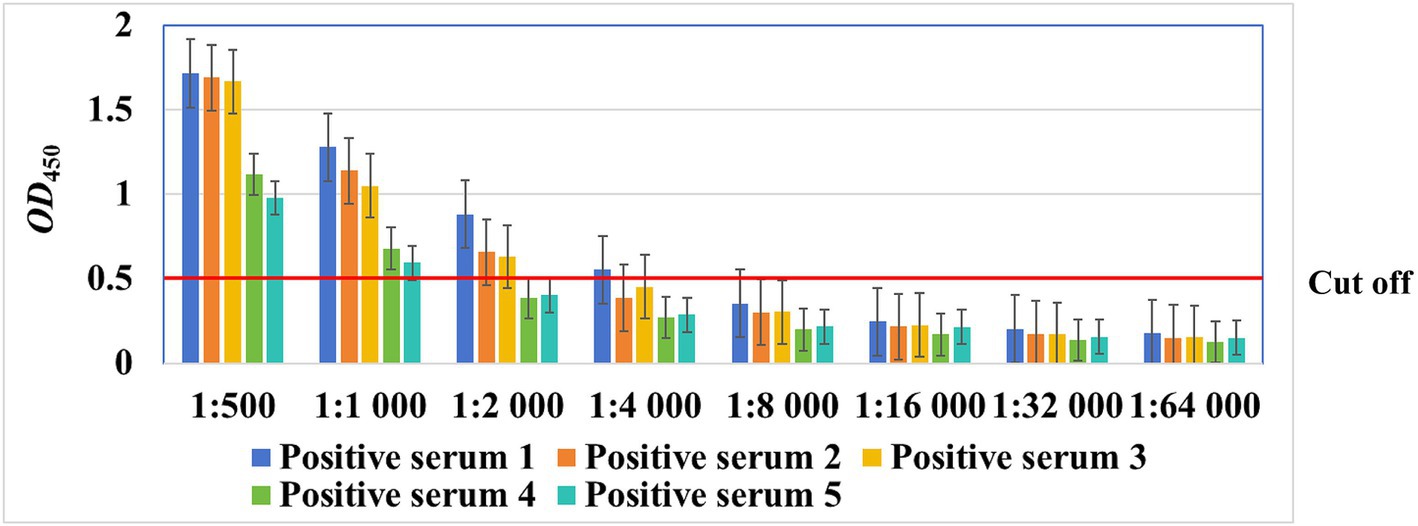
Figure 5. The maximum dilution ratio of chicken sera for detection by the rMS087-based ELISA antibody test method.
3.8 Comparisons with different detection methods
Serum samples collected from two slaughterhouses and five poultry farms were tested via a commercial ELISA kit and the rMS087-based ELISA method. The results are shown in Table 2. The results of the commercial ELISA kit and the rMS087-based ELISA were inconsistent in 28 samples. The total agreement between the commercial ELISA kit and the rMS087-based ELISA was 92.4%, with the sensitivity and specificity of the rMS087-based ELISA being 93.2% and 91.5% compared to those of the commercial ELISA kit, respectively. However, the rMS087-based ELISA method (52.4%, 193/368) could detect more M. synoviae-positive serum samples than commercial ELISA kit (51.9%, 191/368).
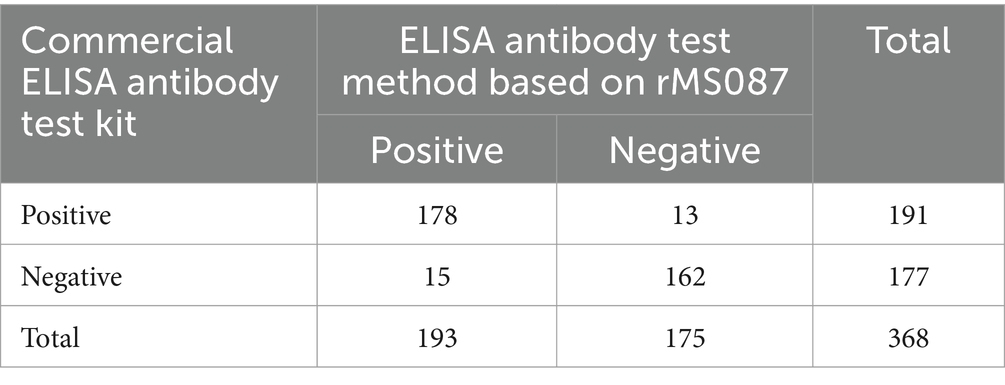
Table 2. Detection of 368 chicken serum samples via a M. synoviae commercial ELISA antibody test kit and the rMS087-based ELISA antibody test method.
4 Discussion
Accurate detection is a prerequisite for the effective prevention and control of M. synoviae infection. In modern poultry production, molecular detection and serological investigations are the most commonly used methods for infectious disease diagnosis. Owing to the sampling sites (upper respiratory tract) used in live poultry (42, 43), the sensitivity of molecular methods for detecting M. synoviae is limited. Serological methods, especially ELISA, make the detection of M. synoviae infection more convenient and inexpensive and have a higher detection rate than real-time PCR does (42), even if antibodies cannot be detected in the early stage of infection (31).
The ELISA methods established previously have shown cross-reactivity with antisera against other avian Mycoplasmas due to the use of whole bacterial cells or membrane proteins (21, 24–26) or have shown reduced sensitivity because of variable amino acid sequences in selected proteins (27, 28).
MS087, which contains 201 amino acids, was predicted to be 23 kDa even if its signal peptide was not cleaved. However, the MW of MS087 in M. synoviae (Figure 2C) or recombinant bacteria (Figure 1) is 31 kDa. The actual MW of MS087 is larger than the predicted MW. One possible reason might be due to inaccurate prediction of online bioinformatics. The second possible reason was that the protein undergone posttranslational modification, such as acetylation (44), lysine methylation (45), etc.
Through BLAST analysis, we showed that MS087 was predicted to be an F1-like ATPase-associated subunit localized on the cell membrane (32), widely expressed in M. synoviae and had a highly conserved amino acid sequence. Our study showed that the protein was localized in both the cytoplasm and the membrane and was even secreted from the organism in our study. The data mentioned above indicate that the MS087 protein has the potential to serve as a good coating antigen for the development of an ELISA method to detect M. synoviae infection in chicken flocks.
We visited several layer farms. The breeders reported that laying hens that produced eggs with EAA did not develop arthritis in either layer pullet flocks or laying hen flocks. These findings suggest that different strains of M. synoviae, which cause arthritis in broilers and the production of eggs with EAA in laying hens, may have different tissue tropisms, although this speculation has not been proven. Therefore, in the process of establishing this method, we used positive control sera against M. synoviae derived from broilers and laying hens.
A cross-reactivity test revealed that the ELISA method based on rMS087 did not react with antisera against other major avian pathogens. Both the specificity (91.5%) and the sensitivity (93.2%) of the rMS087-based ELISA were greater than 90%. Whereas, the specificity and the sensitivity of the LP78-based ELISA were 94.1% and 85.7%, respectively (31). Moreover, the ELISA method based on rMS087 (52.4%, 193/368) detected more positive sera against M. synoviae compared to the commercial kit (51.9%, 191/368), and the rLP78-based ELISA (61.8%, 105/170) detected fewer positive sera against M. synoviae than the commercial kit (70.0%, 119/170) (31). This difference may be because the LP78 protein is a membrane protein, while the MS087 protein not only exists on the membrane but can also be secreted out of cells, continuously stimulating the Th2 cell response and inducing the production of more antibodies against MS087.
There are four main types of Mycoplasmas that cause diseases in poultry, namely M. gallisepticum, M. synoviae, M. meleagridis, and M. iowae. M. meleagridis and M. iowae are the turkey pathogens. The purpose of this study was to establish an ELISA for the detection of anti-M. synoviae antibodies in chicken sera. Therefore, when determining whether there is cross-reactivity in the established detection method, only M. gallisepticum antibodies were used.
In subsequent work, we will use different M. synoviae strains to infect specific pathogen-free (SPF) white Leghorn-type chickens and M. synoviae-free layers to determine when the rMS087-based ELISA can detect anti-M. synoviae antibodies after infection. These findings can provide data to support the early diagnosis of M. synoviae infection. On this basis, the sensitivities of the rMS087-based ELISA and real-time fluorescence quantitative PCR will be compared.
5 Conclusion
In summary, this study established an ELISA method based on the secretory protein MS087 of M. synoviae, which has good specificity and sensitivity, and MS087could be used as a coating antigen for the detection of serum antibodies against M. synoviae in chicken flocks.
Data availability statement
The datasets presented in this study can be found in online repositories. The names of the repository/repositories and accession number(s) can be found at: https://ngdc.cncb.ac.cn/gsa/search?searchTerm=CRR1309196.
Ethics statement
The animal study was approved by Institutional Animal Care and Use Committee of Southwest University. The study was conducted in accordance with the local legislation and institutional requirements.
Author contributions
YZ: Methodology, Writing – review & editing. YW: Data curation, Methodology, Writing – review & editing. JH: Methodology, Writing – review & editing. JL: Methodology, Writing – review & editing. HD: Conceptualization, Funding acquisition, Supervision, Writing – original draft, Writing – review & editing.
Funding
The author(s) declare that financial support was received for the research, authorship, and/or publication of this article. This work was supported by the Lhasa Science and Technology Plan Project (LSKJ202412).
Acknowledgments
The authors would like to acknowledge all the study participants and individuals who contributed to this study.
Conflict of interest
The authors declare that the research was conducted in the absence of any commercial or financial relationships that could be construed as a potential conflict of interest.
Publisher’s note
All claims expressed in this article are solely those of the authors and do not necessarily represent those of their affiliated organizations, or those of the publisher, the editors and the reviewers. Any product that may be evaluated in this article, or claim that may be made by its manufacturer, is not guaranteed or endorsed by the publisher.
Supplementary material
The Supplementary material for this article can be found online at: https://www.frontiersin.org/articles/10.3389/fvets.2024.1472979/full#supplementary-material
Footnotes
References
1. Anderson, GC, Bletner, JK, Munro, DA, Olson, NO, and Shelton, DC. Studies of infectious synovitis in chickens. Am J Vet Res. (1956) 17:747–54.
2. Olson, NO, Adler, HE, DaMassa, AJ, and Corstvet, RE. The effect of intranasal exposure to Mycoplasma synoviae and infectious bronchitis on development of lesions and agglutinins. Avian Dis. (1964) 8:623–31. doi: 10.2307/1587950
3. Lockaby, SB, Hoerr, FJ, Lauerman, LH, and Kleven, SH. Pathogenicity of Mycoplasma synoviae in broiler chickens. Vet Pathol. (1998) 35:178–90. doi: 10.1177/030098589803500303
4. Wei, X, Chen, W, Sun, Q, Zhong, Q, Yan, Z, Zhou, Q, et al. Epidemiological investigations and multilocus sequence typing of Mycoplasma synoviae isolates from chicken farms in China. Poult Sci. (2023) 102:102006. doi: 10.1016/j.psj.2022.102006
5. Moreira, FA, Cardoso, L, and Coelho, AC. Epidemiological survey on Mycoplasma synoviae infection in Portuguese broiler breeder flocks. Vet Ital. (2015) 51:93–8. doi: 10.12834/VetIt.116.329
6. Catania, S, Bilato, D, Gobbo, F, Granato, A, Terregino, C, Iob, L, et al. Treatment of eggshell abnormalities and reduced egg production caused by Mycoplasma synoviae infection. Avian Dis. (2010) 54:961–4. doi: 10.1637/9121-110309-Case.1
7. Cisneros-Tamayo, M, Kempf, I, Coton, J, Michel, V, Bougeard, S, de Boisséson, C, et al. Investigation on eggshell apex abnormality (EAA) syndrome in France: isolation of Mycoplasma synoviae is frequently associated with Mycoplasma pullorum. BMC Vet Res. (2020) 16:271. doi: 10.1186/s12917-020-02487-0
8. Feberwee, A, de Wit, JJ, and Landman, WJ. Induction of eggshell apex abnormalities by Mycoplasma synoviae: field and experimental studies. Avian Pathol. (2009) 38:77–85. doi: 10.1080/03079450802662772
9. Yadav, JP, Tomar, P, Singh, Y, and Khurana, SK. Insights on Mycoplasma gallisepticum and Mycoplasma synoviae infection in poultry: a systematic review. Anim Biotechnol. (2022) 33:1711–20. doi: 10.1080/10495398.2021.1908316
10. Sun, SK, Lin, X, Chen, F, Wang, DA, Lu, JP, Qin, JP, et al. Epidemiological investigation of Mycoplasma synoviae in native chicken breeds in China. BMC Vet Res. (2017) 13:115. doi: 10.1186/s12917-017-1029-0
11. Xue, J, Xu, MY, Ma, ZJ, Zhao, J, Jin, N, and Zhang, GZ. Serological investigation of Mycoplasma synoviae infection in China from 2010 to 2015. Poult Sci. (2017) 96:3109–12. doi: 10.3382/ps/pex134
12. Sui, C, Cui, H, Ji, J, Xu, X, Kan, Y, Yao, L, et al. Epidemiological investigations and locally determined genotype diversity of Mycoplasma synoviae in Central China from 2017 to 2019. Poult Sci. (2022) 101:101522. doi: 10.1016/j.psj.2021.101522
13. Le Carrou, J, Reinhardt, AK, Kempf, I, and Gautier-Bouchardon, AV. Persistence of Mycoplasma synoviae in hens after two enrofloxacin treatments and detection of mutations in the parC gene. Vet Res. (2006) 37:145–54. doi: 10.1051/vetres:2005046
14. Buim, MR, Buzinhani, M, Yamaguti, M, Oliveira, RC, Mettifogo, E, Ueno, PM, et al. Mycoplasma synoviae cell invasion: elucidation of the Mycoplasma pathogenesis in chicken. Comp Immunol Microbiol Infect Dis. (2011) 34:41–7. doi: 10.1016/j.cimid.2009.11.001
15. Dusanić, D, Bercic, RL, Cizelj, I, Salmic, S, Narat, M, and Bencina, D. Mycoplasma synoviae invades non-phagocytic chicken cells in vitro. Vet Microbiol. (2009) 138:114–9. doi: 10.1016/j.vetmic.2009.02.014
16. Xu, B, Liu, R, Ding, M, Zhang, J, Sun, H, Liu, C, et al. Interaction of Mycoplasma synoviae with chicken synovial sheath cells contributes to macrophage recruitment and inflammation. Poult Sci. (2020) 99:5366–77. doi: 10.1016/j.psj.2020.08.003
17. Feberwee, A, Morrow, CJ, Ghorashi, SA, Noormohammadi, AH, and Landman, WJ. Effect of a live Mycoplasma synoviae vaccine on the production of eggshell apex abnormalities induced by a M. synoviae infection preceded by an infection with infectious bronchitis virus D1466. Avian Pathol. (2009) 38:333–40. doi: 10.1080/03079450903183652
18. Markham, JF, Morrow, CJ, and Whithear, KG. Efficacy of a temperature-sensitive Mycoplasma synoviae live vaccine. Avian Dis. (1998) 42:671–6. doi: 10.2307/1592701
19. Feberwee, A, Dijkman, R, Klinkenberg, D, and Landman, WJM. Quantification of the horizontal transmission of Mycoplasma synoviae in non-vaccinated and MS-H-vaccinated layers. Avian Pathol. (2017) 46:346–58. doi: 10.1080/03079457.2017.1282602
20. Han, S, Wang, Y, Chang, W, Wang, L, Fang, J, Han, J, et al. Evaluation of the protective efficacy of six major immunogenic proteins of Mycoplasma synoviae. Front Vet Sci. (2024) 10:1334638. doi: 10.3389/fvets.2023.1334638
21. Opitz, HM, Duplessis, JB, and Cyr, MJ. Indirect micro-enzyme-linked immunosorbent assay for the detection of antibodies to Mycoplasma synoviae and M. gallisepticum. Avian Dis. (1983) 27:773–86. doi: 10.2307/1590321
22. Thornton, GA. A comparison of three Mycoplasma synoviae rapid plate agglutination antigens in experimental M. synoviae infection in chickens. Avian Pathol. (1978) 7:123–30. doi: 10.1080/03079457808418264
23. Vardaman, TH, and Yoder, HW Jr. Preparation of Mycoplasma synoviae hemagglutinating antigen and its use in the hemagglutination-inhibition test. Avian Dis. (1969) 13:654–61. doi: 10.2307/1588539
24. Feberwee, A, Mekkes, DR, de Wit, JJ, Hartman, EG, and Pijpers, A. Comparison of culture, PCR, and different serologic tests for detection of Mycoplasma gallisepticum and Mycoplasma synoviae infections. Avian Dis. (2005) 49:260–8. doi: 10.1637/7274-090804R
25. Higgins, PA, and Whithear, KG. Detection and differentiation of Mycoplasma gallisepticum and M. synoviae antibodies in chicken serum using enzyme-linked immunosorbent assay. Avian Dis. (1986) 30:160–8. doi: 10.2307/1590628
26. Opitz, HM, and Cyr, MJ. Triton X-100-solubilized Mycoplasma gallisepticum and M. synoviae ELISA antigens. Avian Dis. (1986) 30:213–5. doi: 10.2307/1590636
27. Noormohammadi, AH, Browning, GF, Jones, J, and Whithear, KG. Improved detection of antibodies to Mycoplasma synoviae vaccine MS-H using an autologous recombinant MSPB enzyme-linked immunosorbent assay. Avian Pathol. (2002) 31:611–7. doi: 10.1080/0307945021000024553
28. Noormohammadi, AH, Markham, PF, Markham, JF, Whithear, KG, and Browning, GF. Mycoplasma synoviae surface protein MSPB as a recombinant antigen in an indirect ELISA. Microbiology (Reading). (1999) 145:2087–94. doi: 10.1099/13500872-145-8-2087
29. Noormohammadi, AH, Markham, PF, Whithear, KG, Walker, ID, Gurevich, VA, Ley, DH, et al. Mycoplasma synoviae has two distinct phase-variable major membrane antigens, one of which is a putative hemagglutinin. Infect Immun. (1997) 65:2542–7. doi: 10.1128/iai.65.7.2542-2547.1997
30. Noormohammadi, AH, Markham, PF, Kanci, A, Whithear, KG, and Browning, GF. A novel mechanism for control of antigenic variation in the haemagglutinin gene family of Mycoplasma synoviae. Mol Microbiol. (2000) 35:911–23. doi: 10.1046/j.1365-2958.2000.01766.x
31. Han, S, Wang, Y, Wang, L, Chang, W, Wen, B, Fang, J, et al. Mycoplasma synoviae LP78 is a fibronectin/plasminogen binding protein, putative adhesion, and potential diagnostic antigen. Front Microbiol. (2024) 14:1335658. doi: 10.3389/fmicb.2023.1335658
32. Béven, L, Charenton, C, Dautant, A, Bouyssou, G, Labroussaa, F, Sköllermo, A, et al. Specific evolution of F1-like ATPases in mycoplasmas. PLoS One. (2012) 7:e38793. doi: 10.1371/journal.pone.0038793
33. Teufel, F, Almagro Armenteros, JJ, Johansen, AR, Gíslason, MH, Pihl, SI, Tsirigos, KD, et al. SignalP 6.0 predicts all five types of signal peptides using protein language models. Nat Biotechnol. (2022) 40:1023–5. doi: 10.1038/s41587-021-01156-3
34. Krogh, A, Larsson, B, von Heijne, G, and Sonnhammer, EL. Predicting transmembrane protein topology with a hidden Markov model: application to complete genomes. J Mol Biol. (2001) 305:567–80. doi: 10.1006/jmbi.2000.4315
35. Yu, CS, Lin, CJ, and Hwang, JK. Predicting subcellular localization of proteins for gram-negative bacteria by support vector machines based on n-peptide compositions. Protein Sci. (2004) 13:1402–6. doi: 10.1110/ps.03479604
36. Shen, HB, and Chou, KC. Gpos-mPLoc: a top-down approach to improve the quality of predicting subcellular localization of gram-positive bacterial proteins. Protein Pept Lett. (2009) 16:1478–84. doi: 10.2174/092986609789839322
37. Doytchinova, IA, and Flower, DR. VaxiJen: a server for prediction of protective antigens, tumour antigens and subunit vaccines. BMC Bioinformatics. (2007) 8:4. doi: 10.1186/1471-2105-8-4
38. Qi, J, Wang, Y, Li, H, Shang, Y, Gao, S, Ding, C, et al. Mycoplasma synoviae dihydrolipoamide dehydrogenase is an immunogenic fibronectin/plasminogen binding protein and a putative adhesin. Vet Microbiol. (2022) 265:109328. doi: 10.1016/j.vetmic.2021.109328
39. Tian, Y, Xu, Z, Wen, Y, Yang, M, Ning, Y, Wang, Z, et al. Development of an indirect ELISA for detection of anti-Mycoplasma hyopneumoniae IgG in naturally infected pathogen-induced convalescent sera. BMC Vet Res. (2021) 17:123. doi: 10.1186/s12917-021-02828-7
40. Garg, K, and Campolonghi, S. A step-by-step guide to selecting an optimal cut-off value based on the receiver operating characteristic and Youden index in methods designed to diagnose Lyme disease. Methods Mol Biol. (2024) 2742:69–76. doi: 10.1007/978-1-0716-3561-2_5
41. Xu, H, Lohr, J, and Greiner, M. The selection of ELISA cut-off points for testing antibody to Newcastle disease by two-graph receiver operating characteristic (TG-ROC) analysis. J Immunol Methods. (1997) 208:61–4. doi: 10.1016/s0022-1759(97)00128-2
42. Cortés, V, Sevilla-Navarro, S, García, C, Tudón, A, Marín, C, and Catalá-Gregori, P. Seroprevalence and prevalence of Mycoplasma synoviae in laying hens and broiler breeders in Spain. Poult Sci. (2021) 100:100911. doi: 10.1016/j.psj.2020.11.076
43. Moronato, ML, Cecchinato, M, Facchetti, G, Mainenti, M, Gobbo, F, and Catania, S. Application of different laboratory techniques to monitor the behaviour of a Mycoplasma synoviae vaccine (MS-H) in broiler breeders. BMC Vet Res. (2018) 14:357. doi: 10.1186/s12917-018-1669-8
44. Liu, M, Guo, L, Fu, Y, Huo, M, Qi, Q, and Zhao, G. Bacterial protein acetylation and its role in cellular physiology and metabolic regulation. Biotechnol Adv. (2021) 53:107842. doi: 10.1016/j.biotechadv.2021.107842
Keywords: Mycoplasma synoviae, subcellular localization, working condition, reproducibility, cross-reactivity, sensitivity, specificity
Citation: Zhang Y, Wu Y, He J, Lai J and Ding H (2024) Recombinant MS087-based indirect ELISA for the diagnosis of Mycoplasma synoviae. Front. Vet. Sci. 11:1472979. doi: 10.3389/fvets.2024.1472979
Edited by:
David C. B. Taras, Boehringer Ingelheim, GermanyReviewed by:
Tesfaye Gelanew, Armauer Hansen Research Institute (AHRI), EthiopiaMichael Gassel, Boehringer Ingelheim, Germany
Copyright © 2024 Zhang, Wu, He, Lai and Ding. This is an open-access article distributed under the terms of the Creative Commons Attribution License (CC BY). The use, distribution or reproduction in other forums is permitted, provided the original author(s) and the copyright owner(s) are credited and that the original publication in this journal is cited, in accordance with accepted academic practice. No use, distribution or reproduction is permitted which does not comply with these terms.
*Correspondence: Honglei Ding, aG9uZ2xlaWRpbmdAc3d1LmVkdS5jbg==
†These authors have contributed equally to this work and share first authorship