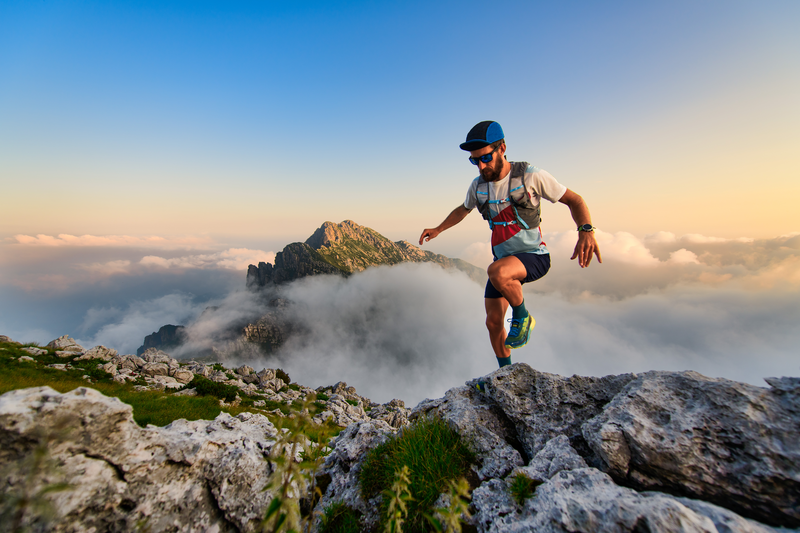
95% of researchers rate our articles as excellent or good
Learn more about the work of our research integrity team to safeguard the quality of each article we publish.
Find out more
ORIGINAL RESEARCH article
Front. Vet. Sci. , 05 February 2025
Sec. Animal Reproduction - Theriogenology
Volume 11 - 2024 | https://doi.org/10.3389/fvets.2024.1471123
The Cell division cycle 25A (CDC25A) gene has been considered as a candidate gene associated with reproductive traits for goat breeding. In this study, five truncated fragments divided at position-2285 nt to +198 nt were amplified and cloned into the luciferase reporter vectors to identify the core promoter. The luciferase reporter assay showed that the core promoter of CDC25A was located at position-663 nt to-237 nt. Afterwards, a single nucleotide polymorphism (NC_030829.1:g.51731829A > C) at the core promoter was detected using sequencing and KASP in a population of 1,016 goats and luciferase reporter vectors carrying the A allele or C allele were transfected into cells, respectively. The results displayed that the higher relative luciferase activity was observed in plasmids carrying the A allele rather than the C allele. The litter size of individuals with the AA genotype was significantly better than those with other genotypes, which corresponded to increased transcriptional activity in plasmids carrying the A allele. In short, our study provides a potential molecular genetic marker for improving reproductive efficiency in goat breeding.
For thousands of years, goats (Capra hircus) have provided humans with meat, milk, and fiber products. In today’s goat industry, improving economic returns through breeding programs remains a key focus. Reproductive traits, as critical phenotypic characteristics, have a significant impact on the economic viability of goat production. However, reproductive traits are quantitative traits with low heritability, and traditional breeding programs have difficulty to improve these traits in a short time. Marker-assisted selection (MAS) is a method based on morphological, biochemical or DNA/RNA makers to select individuals with better phenotypic traits, which is quicker and more effective than traditional breeding programs (1, 2). Using MAS to identify and choose genotypes that enhance fertility can significantly boost reproductive efficiency in goats over a short period.
The CDC25A is a dual-specificity phosphatase of the CDC25 family, which plays a crucial role in the regulation of the eukaryotic cell cycle (3, 4). In reproduction, CDC25A plays a crucial role in spermatogenesis, where its decreased expression is linked to infertility and failed sperm retrieval (5). Additionally, overexpression of miR-15b, which targets the 3’-UTR of CDC25A, negatively impacts CDC25A activity and is vital for proper spermatogenesis (6). In mouse oocytes, downregulation of CDC25A leads to the release from metaphase II arrest, demonstrating its importance in maintaining the cell cycle during meiosis (7). Among livestock, the goat CDC25A gene shares a closer evolutionary relationship with other species within the Bovidae family (Supplementary Figure S1). Dong et al. (8) found that CDC25A showed lower expression levels in cattle-yak (Bos taurus × Bos grunniens) testis than those in cattle (Bos taurus) and yak (Bos grunniens), which was considered to correlate the infertility in cattle-yak. The gene expression heat map referring to the Ruminant Genome Database1 (9) displayed that CDC25A showed relatively high expression levels in testis and ovary of goats, which may imply its potential function in reproduction (Supplementary Figure S3). For goat breeding, nucleotide polymorphisms of goat CDC25A were considered as potential molecular markers. For instance, an insertion/deletion mutation located in the intron of CDC25A has been found to be correlated with goat litter size (10) as well as growth traits (11). In a study by Wang et al. (12), CDC25A was identified as a candidate gene underlying strong selection signature with goat body size. Genes in the same family generally have similar structure and functions (13). Wang et al. (2) used whole genome sequencing (WGS) to identify a variant in CDC25C, another member of the CDC25 family, was significantly related to goat litter size. These findings stimulated our interest in exploring the relationship between CDC25A and goat reproductive traits.
Most studies in the CDC25A gene have been limited to coding region and intron variants that may affect protein function and mRNA splicing, yet polymorphisms within the promoter have been ignored. A promoter is a DNA sequence, which is needed to turn a gene on or off (14). Generally, the promoter region ranges from 2,000 bp upstream to 500 bp downstream of the transcription start site (TSS) in animals (15). The core promoter, including the RNA polymerase binding site and cis-acting elements, etc., plays a crucial role in regulating transcription of the gene (16). Mutations in the core promoter probably alter transcriptional activity and thus affect phenotypic traits. Hence, identifying the core promoter in goat CDC25A, scanning the mutation within it, and assessing the correlation of the mutation and reproductive traits are of great significance.
Herein, we identified promoter activity in different truncated fragments of the goat CDC25A gene, discovered a novel SNP in the core promoter that affected the transcriptional activity, and analyzed the correlation between the novel SNP and litter size in goat. These findings would provide a potential molecular genetic marker to improve reproductive efficiency in goat breeding.
All animal experiments were approved by the Animal Care and Use Committee of Northwest A&F University (protocol No.314020038) and conformed to the animal welfare laws and guidelines in this study.
A total of 1,016 ear samples of adult female Shaanbei White Cashmere (SBWC) were randomly collected in Yulin city, Shaanxi Province, China (17). All individuals were healthy, mated naturally and kept under the same nutritional and living conditions. In addition, the litter size data for all goats were recorded by the stockmen.
The goat genomic DNA was extracted from tissue samples using a high-salt extraction protocol and then diluted to 10 ng/μL-concentration solution, keeping at −40°C (18, 19). The purity and quality of nucleic acid were detected using NanoDrop 1,000 instrument (Thermo Fisher Scientific Inc., America).
Five truncated fragments with different length divided in the-2285 nt ~ +198 nt region (F1: position-2285 nt to +198 nt; F2: position-1176 nt to +198 nt; F3: position-663 nt to +198 nt; F4: position-237 nt to +198 nt; F5: position-115 nt to +198 nt) were amplified and cloned into the pGL3-Basic luciferase reporter vectors using seamless cloning technology, respectively (Takara Bio, Kyoto, Japan) (Figure 1A, Information of primers was shown in Table 1). According to the target transferred fragments, plasmids were named as pGL3-F1, pGL3-F2, pGL3-F3, pGL3-F4 and pGL3-F5. Besides, the luciferase reporter vectors carrying various alleles were constructed according to the protocol described above after scanning the core promoter sequences. Recombinant vectors were used for transient transfection after identification. The pRL-TK vectors were used to normalize the luciferase signal, as the internal reference.
Figure 1. Mechanism diagram. (A) The structure patterns of goat CDC25A gene. (B) The core promoter of goat CDC25A gene was located at position-663 to-237. The electrophoregram on the left displayed that the five fragments were amplified successfully. The bar plots in the middle and on the right displayed the relative luciferase activity of different fragments within CDC25A gene promoter detected in HEK293T and CHO-K1 cells, respectively. (C) One novel SNP (NC_030829.1:g.51731829A > C) within the core promoter of goat CDC25A gene was found, which might change the binding of transcription factors. (D) The novel SNP significantly affected the transcriptional activity of CDC25A gene. (E) Left: associations between the novel SNP and litter size in goat CDC25A gene and the proportion of different types of litter size in individuals with AA, AC and CC genotypes. Right: the proportion of AA, AC and CC genotypes in single-lamb and multi-lamb groups. SBWC goat, Shaanbei white cashmere goat; UTR, untranslated region; CDS, coding sequence; **p < 0.01. Bars with different letters (a, b, c, d) means p < 0.05.
Chinese hamster ovary K1 (CHO-K1) cells and Human embryonic kidney 293 T (HEK293T) cells were cultured in complete medium consisting of Ham’s F 12 nutrient medium (Sangon Biotech, Xi’an, China), 10% fetal bovine serum (Zeta life, America) and 1% penicillin–streptomycin (Gibco, America) at 37°C in a humidified 5% CO2 atmosphere (20–22).
Before transfection, the cells were seeded into 96-well plates at a density of 5 × 104 cells per well. 100 μL of complete medium was added to each well and transient transfection was conducted after 12 h. 100 ng of reporter plasmids and 4 ng of transfection control plasmids (pRL-TK) to normalize the luciferase signal were co-transfected into CHO-K1 and HEK293T cells with Lipofectamine 2000 (Invitrogen, America). Then cells were cultured for 24 h and a Dual-Luciferase-Assay-System (Promega, Heidelberg, Germany) was employed to monitor luciferase activity (20–22).
A 25 μL polymerase chain reaction (PCR) system and the Kang’s program (21) were used to amplify the target fragments and products were validated by electrophoresis using 1.5% agarose gels stained with GoldView (Zhonghuihecai Biotech, Xi’an, China) (Information of primers was shown in Table 1 and the primer P6 was used to detect the novel mutation). Qualified PCR products were sent to sequencing to detect mutation located in the goat CDC25A core promoter (Sangon Biotech, Xi’an, China), which were then compared with variant tables of Ensembl Database2 and Animal Omics Database (AOD)3 to search novel nucleotide polymorphisms.
Besides, genotyping was performed with the KASP™ genotyping technology (Kompetitive Allele-Specific PCR assay, FLU-ARMS, Videgene, China), and results were visualized with fluorescence ration PCR instrument (Quant Studio 5, Thermo Fisher Scientific, US). Genotype data for each animal were exported for the statistical analysis.
The frequencies of genotypes and alleles, heterozygosity (He), homozygosity (Ho), and polymorphism information content (PIC) of the mutation were calculated adopting Nei’s methods (23). Welch’s ANOVA, Post Hoc Multiple Comparisons (Tamhane’s T2 test) and χ2 test were used to analyze the correlation between the mutation and litter size. Hardy–Weinberg equilibrium was tested in SHEsis software4 (24). The following general linear model of ANOVA was used: Y ijk = μ + A i + G j + ε ijk , where Y ijk is the phenotypic data of litter size of each animal, μ is the mean of population, A i is the fixed effect of age, G j is the fixed effect of genotype, and ε ijk is the random error (25).
Promoter regions often contains CpG islands (26), which have been linked to promoter activity (27). DNA methylation at CpG islands is crucial for gene transcription and tissue-specific processes (28). Thus, we predicted the CpG islands in the CDC25A promoter using the EMBOSS Cpgplot software.5 Besides, a mutation in the core promoter may affect transcriptional activity by changing transcription factors bound with core promoters (29), and thus potential transcription factors were predicted via the PROMO software6 (factors predicted within a dissimilarity margin less or equal than 5%).
Five truncated CDC25A promoter fragments were successfully amplified (Figure 1B) and cloned into luciferase reporter vectors, which were co-transfected with pRL-TK into CHO-K1 and HEK293T cells, respectively. The dual-luciferase reporter assay displayed that the pGL3-F3 vector had significantly stronger luminescence activity than the others in both cell lines (p < 0.05) (Figure 1B), suggesting that the core promoter was located at position-663 nt to-237 nt of CDC25A gene.
The core promoter sequences were amplified and sent to sequencing in order to scan the nucleotide polymorphisms among a population of 413 goats. One SNP (NC_030829.1:g.51731829A > C) within the core promoter was identified and three genotypes, AA, AC and CC, were detected, which had never been recorded in the Ensembl Database and AOD (Figure 1C). Then, the nucleotide diversity of the remaining 603 individuals was detected by KASP technique (Supplementary Figure S2). The frequencies of AA, AC and CC were 0.051, 0.346 and 0.603, and the A and C allele frequencies were 0.224 and 0.776, respectively. The genetic parameters were calculated as follows: Ho = 0.652, He = 0.348, Ne = 1.533, and PIC = 0.287 (Table 2). These observations indicated that the frequency of the novel SNP was high, and it had a moderate level of genetic diversity (0.25 < PIC < 0.5). Besides, the mutation is at the Hardy–Weinberg equilibrium in this population (p = 0.5318) (Table 2).
Table 2. Genetic parameters of the SNP (NC_030829.1:g.51731829A > C) within CDC25A in SBWC goats (n = 1,016).
In this study, the CpG islands in promoter and transcription factors bound with the promoter have been predicted. A same CpG island, located at position +96 to +445, and 350 bp in length, was predicted in both alleles and 43% length of the CpG island region was coincident with the core promoter using the online software EMBOSS Cpgplot (Supplementary Figure S4). However, the transcription factors binding at the SNP locus varied between different alleles. Homeobox A5 (HOXA5) was observed to bind to the C allele, and POU Class 1 Homeobox 1 (POU1F1/POU1F1a) and Nuclear Transcription Factor Y (NF-Y) were observed to bind to the A allele.
The core promoter can regulate gene expression activity via binding transcription factors. Consequently, a mutation in the core promoter can alter transcriptional activity and then affect phenotypic traits in animals. In order to confirm whether the SNP would affect the transcriptional activity of the CDC25A gene, luciferase reporter vectors were constructed. The results demonstrated that the luminescence activity of vectors carrying the A allele was significantly higher than vectors carrying the C allele in both CHO-K1 and HEK293T cells (Figure 1D).
We examined the relationship between the novel SNP and litter size using Welch’s ANOVA, followed by Tamhane’s T2 post hoc test for multiple comparisons to assess the significance of litter size differences across genotypes. Additionally, the χ2 test was applied to evaluate differences in genotype distribution among goats with varying litter sizes in the population. The association analysis revealed a significant correlation between the novel SNP and litter size in goats. Individuals with the AA genotype had a higher litter size compared to those with the CC and AC genotypes (p < 0.05). Moreover, goats with the AA genotype were more prevalent among mothers with multiple lambs than among those with single lambs (p < 0.05) (Table 3, Figure 1E).
MAS, a modern approach within the field of molecular breeding (30), offers the advantages of high efficiency and precision. It has been applied to enhance reproductive traits and overall productivity in goats (17). The CDC25A gene has been demonstrated to be essential for spermatogenesis and oocyte development (5–7, 31) and selected as a candidate gene for goat breeding (2, 10–12, 32). Previous research has demonstrated that mutations in the coding regions and introns of the CDC25A gene influence litter size in goats. However, there have been limited studies examining the relationship between promoter polymorphisms and reproductive traits in goats.
We first investigated the regional coverage of the CDC25A core promoter. Five truncated fragments with different length located from position −2285 to +198 were amplified and linked to pGL3-Basic plasmids. A dual-luciferase reporter assay was used to detect luminescence activity and the CHO-K1 and HEK293T cell lines were implied for transfection. The results displayed that maximum luminescence activity was observed at the PGL3-F3 vector in both cell lines, suggesting the core promoter of CDC25A was located at position-663 to-237 (Figure 1B).
Mutations in gene promoters were demonstrated to alter animal phenotypic traits, including litter size, milk traits, and body measurement traits, etc. (21, 33, 34). In our study, one novel SNP (NC_030829.1:g.51731829A > C) located in the CDC25A core promoter was successfully detected among 1,016 goats (Figure 1B). Genetic parameter analysis demonstrated that the frequency of the C allele was higher than the A allele in SBWC breed. Besides, the SNP had a moderate level of genetic diversity and at the Hardy–Weinberg equilibrium (p = 0.5318) in this population (Table 2) (17).
A mutation within the core promoter can impact epigenetic processes and alter transcription factor binding. Thus, CpG islands in the promoter and transcription factors bound to the SNP locus were predicted. We found that the mutation did not affect the existence of CpG island but changed the binding of the transcription factors (Supplementary Figure S4). Notably, POU1F1a, a key transcription factor that influences development and growth through direct regulation of pituitary hormone secretion (35), could combine with the A allele rather than the C allele (Figure 1C). The missense mutation within POU1F1a has been reported to be strongly related to litter size in goat (25), suggesting that the novel SNP might cause binding changes of important transcription factors and affect growth or reproduction phenotypes in goat.
The changes in transcription factor binding can alter the transcriptional activity of genes (36). Therefore, to investigate whether the SNP influences gene transcriptional activity, vectors containing the A allele and C allele were constructed. The results revealed that the vector with the A allele exhibited significantly higher luminescence activity, confirming that the SNP in the CDC25A core promoter affects the gene’s transcriptional activity (Figure 1D). Notably, the up or down-regulation of the transcriptional activity of genes could alter phenotypic traits of animals. We found that the litter size of goats with the AA genotype was significantly better than those with the AC or CC genotype, which corresponded to increased transcriptional activity in plasmids carrying the A allele, and amounts of mothers with single/multi-lamb were significantly correlated with genotype frequencies. Goats with the AA genotype accounted for a higher proportion in the multi-lamb population compared with the single-lamb population. It has been indicated that CDC25A activity is crucial for both spermatogenesis (5, 6, 31) and oocyte development, particularly during the metaphase II arrest in oocytes (7). Therefore, the SNP may affect the expression of the CDC25A gene, thereby influencing downstream physiological processes, particularly reproductive performance. In summary, the novel SNP within the core promoter of the CDC25A gene changed promoter activity and was remarkably associated with litter size in goat.
Our findings would provide a potential molecular genetic marker for improving goat reproductive efficiency. However, more evidences are required to determine whether the correlation between the novel SNP and litter size is direct or indirect.
In the present study, the core promoter of the goat CDC25A gene was identified and a novel SNP in the core promoter was associated with the litter size presumably by affecting transcriptional activity. The novel SNP locus could be a potential molecular genetic marker to improve goat reproductive efficiency.
The variant data for this study have been deposited in the European Variation Archive (EVA) at EMBL-EBI under accession number PRJEB84361, https://www.ebi.ac.uk/eva/?eva-study=PRJEB84361.
The animal study was approved by Animal Care and Use Committee of Northwest A&F University. The study was conducted in accordance with the local legislation and institutional requirements.
TZ: Formal analysis, Investigation, Software, Writing – original draft, Writing – review & editing. JW: Formal analysis, Investigation, Software, Writing – original draft, Writing – review & editing. YB: Investigation, Software, Writing – review & editing. QW: Investigation, Software, Writing – review & editing. KW: Software, Writing – review & editing. HZ: Resources, Software, Writing – review & editing. LQ: Funding acquisition, Supervision, Writing – review & editing. ZG: Resources, Software, Writing – review & editing. CP: Conceptualization, Funding acquisition, Project administration, Supervision, Validation, Writing – review & editing. XL: Conceptualization, Data curation, Project administration, Resources, Writing – review & editing.
The author(s) declare that financial support was received for the research, authorship, and/or publication of this article. This work was supported by the National Key R&D Program of China (2022YFF1000100), the National Natural Science Foundation of China (No. 32060734), China Agriculture Research System of MOF and MARA and Guizhou Agricultural Production and Development Project (Qiannongmu [2024] 02).
We are grateful to the staff at the Shaanbei White Cashmere goat breeding farm for allowing us to collect samples and for the support of the Agricultural Technical Station of Veterinary and Animal Husbandry of Yulin City, Shaanxi Province, China.
The authors declare that the research was conducted in the absence of any commercial or financial relationships that could be construed as a potential conflict of interest.
All claims expressed in this article are solely those of the authors and do not necessarily represent those of their affiliated organizations, or those of the publisher, the editors and the reviewers. Any product that may be evaluated in this article, or claim that may be made by its manufacturer, is not guaranteed or endorsed by the publisher.
The Supplementary material for this article can be found online at: https://www.frontiersin.org/articles/10.3389/fvets.2024.1471123/full#supplementary-material
AOD, Animal Omics Database; CDC25A, Cell division cycle 25A; CDS, coding sequence; CHO-K1 cell, Chinese hamster ovary K1 cell; He, heterozygosity; HEK293T cell, human embryonic kidney 293T cell; Ho, homozygosity; HOXA5, Homeobox A5; MAS, marker-assisted selection; NF-Y, Nuclear Transcription Factor Y; PCR, polymerase chain reaction; PIC, polymorphism information content; POU1F1/POU1F1a, POU Class 1 Homeobox 1/POU Class 1 Homeobox 1a; SBWC goat, Shaanbei white cashmere goat; SNP, single nucleotide polymorphism; TSS, transcription start site; UTR, untranslated region; WGS, whole genome sequencing; KASP, kompetitive allele-specific PCR.
1. ^http://animal.nwsuaf.edu.cn/code/index.php/RGD
2. ^http://asia.ensembl.org/index.html
3. ^http://animal.nwsuaf.edu.cn/
5. ^https://www.ebi.ac.uk/Tools/seqstats/emboss_cpgplot/
6. ^http://alggen.lsi.upc.es/cgi-bin/promo_v3/promo/promoinit.cgi?dirDB=TF_8.3
1. Li, W, Liu, D, Tang, S, Li, D, Han, R, Tian, Y, et al. A multiallelic indel in the promoter region of the cyclin-dependent kinase inhibitor 3 gene is significantly associated with body weight and carcass traits in chickens. Poult Sci. (2019) 98:556–65. doi: 10.3382/ps/pey404
2. Wang, Z, Zhang, X, Jiang, E, Yan, H, Zhu, H, Chen, H, et al. InDels within caprine IGF2BP1 intron 2 and the 3′-untranslated regions are associated with goat growth traits. Anim Genet. (2020) 51:117–21. doi: 10.1111/age.12871
3. Lavecchia, A, Di Giovanni, C, and Novellino, E. CDC25 phosphatase inhibitors: an update. Mini Rev Med Chem. (2012) 12:62–73. doi: 10.2174/138955712798868940
4. Rudolph, J. Cdc25 phosphatases: structure, specificity, and mechanism. Biochemistry. (2007) 46:3595–604. doi: 10.1021/bi700026j
5. Chen, Y, Li, X, Liao, H, Leung, X, He, J, Wang, X, et al. CFTR mutation compromises spermatogenesis by enhancing miR-15b maturation and suppressing its regulatory target CDC25A. Biol Reprod. (2019) 101:50–62. doi: 10.1093/biolre/ioz062
6. Suryandari, DA, Midoen, YH, Yunaini, L, Setyaningsih, S, and Freisleben, HJ. Decreased expression of CDC25A in azoospermia as the etiology of spermatogenesis failure. J Reprod Infert. (2018) 19:100–8.
7. Oh, JS, Susor, A, Schindler, K, Schultz, RM, and Conti, M. Cdc25A activity is required for the metaphase II arrest in mouse oocytes. J Cell Sci. (2013) 126:1081–5. doi: 10.1242/jcs.115592
8. Dong, LY, Li, QF, Qu, XG, Li, YX, Li, XF, Hu, HT, et al. Hereditas. (2009) 31:495–9. doi: 10.3724/sp.j.1005.2009.00495
9. Fu, W, Wang, R, Nanaei, HA, Wang, J, Hu, D, and Jiang, Y. RGD v2.0: a major update of the ruminant functional and evolutionary genomics database. Nucleic Acids Res. (2022) 50:D1091–9. doi: 10.1093/nar/gkab887
10. Yang, Y, Sun, X, Cui, W, Liu, N, Wang, K, Qu, L, et al. The detection of mutation within goat cell division cycle 25 a and its effect on kidding number. Anim Biotechnol. (2021) 33. Advance online publication.:1504–9. doi: 10.1080/10495398.2021.1910519
11. Cui, W, Liu, N, Zhang, X, Zhang, Y, Qu, L, Yan, H, et al. A 20-bp insertion/deletion (indel) polymorphism within the CDC25A gene and its associations with growth traits in goat. Arch Anim Breed. (2019) 62:353–60. doi: 10.5194/aab-62-353-2019
12. Wang, X, Liu, J, Zhou, G, Guo, J, Yan, H, Niu, Y, et al. Whole-genome sequencing of eight goat populations for the detection of selection signatures underlying production and adaptive traits. Sci Rep. (2016) 6:38932. doi: 10.1038/srep38932
13. Uehara, S, Uno, Y, and Yamazaki, H. The marmoset cytochrome P450 superfamily: sequence/phylogenetic analyses, genomic structure, and catalytic function. Biochem Pharmacol. (2020) 171:113721. doi: 10.1016/j.bcp.2019.113721
14. Mishal, R, and Luna-Arias, JP. Role of the TATA-box binding protein (TBP) and associated family members in transcription regulation. Gene. (2022) 833:146581. doi: 10.1016/j.gene.2022.146581
15. van Arensbergen, J, FitzPatrick, VD, de Haas, M, Pagie, L, Sluimer, J, Bussemaker, HJ, et al. Genome-wide mapping of autonomous promoter activity in human cells. Nat Biotechnol. (2017) 35:145–53. doi: 10.1038/nbt.3754
16. Hernandez-Garcia, CM, and Finer, JJ. Identification and validation of promoters and cis-acting regulatory elements. Plant Sci. (2014) 218:109–19. doi: 10.1016/j.plantsci.2013.12.007
17. Zhang, T, Liu, M, Yang, Y, Wang, K, Zhao, H, and Pan, C. An upstream deletion polymorphism within the goat period circadian regulator 1 (PER1) gene was associated with growth traits. Anim Biotechnol. (2021) 34. Advance online publication.:819–24. doi: 10.1080/10495398.2021.1998091
18. Aljanabi, SM, and Martinez, I. Universal and rapid salt-extraction of high quality genomic DNA for PCR-based techniques. Nucleic Acids Res. (1997) 25:4692–3. doi: 10.1093/nar/25.22.4692
19. Lan, XY, Pan, CY, Chen, H, Zhang, CL, Li, JY, Zhao, M, et al. An AluI PCR-RFLP detecting a silent allele at the goat POU1F1 locus and its association with production traits. Small Rumin Res. (2007) 73:8–12. doi: 10.1016/j.smallrumres.2006.10.009
20. Chen, R, Cui, Y, Zhang, X, Zhang, Y, Chen, M, Zhou, T, et al. Chlorpyrifos induction of testicular-cell apoptosis through generation of reactive oxygen species and phosphorylation of AMPK. J Agric Food Chem. (2018) 66:12455–70. doi: 10.1021/acs.jafc.8b03407
21. Kang, Z, Zhang, S, He, L, Zhu, H, Wang, Z, Yan, H, et al. A 14-bp functional deletion within the CMTM2 gene is significantly associated with litter size in goat. Theriogenology. (2019) 139:49–57. doi: 10.1016/j.theriogenology.2019.07.026
22. Yang, Q, Han, L, Li, J, Xu, H, Liu, X, Wang, X, et al. Activation of Nrf2 by Phloretin attenuates palmitic acid-induced endothelial cell oxidative stress via AMPK-dependent signaling. J Agric Food Chem. (2019) 67:120–31. doi: 10.1021/acs.jafc.8b05025
23. Nei, M. Analysis of gene diversity in subdivided populations. Proc Natl Acad Sci USA. (1973) 70:3321–3. doi: 10.1073/pnas.70.12.3321
24. Li, Z, Zhang, Z, He, Z, Tang, W, Li, T, Zeng, Z, et al. A partition-ligation-combination-subdivision EM algorithm for haplotype inference with multiallelic markers: update of the SHEsis. Cell Res. (2009) 19:519–23. doi: 10.1038/cr.2009.33
25. Zhang, Y, Cui, W, Yang, H, Wang, M, Yan, H, Zhu, H, et al. A novel missense mutation (L280V) within POU1F1 gene strongly affects litter size and growth traits in goat. Theriogenology. (2019) 135:198–203. doi: 10.1016/j.theriogenology.2019.06.021
26. Gardiner-Garden, M, and Frommer, M. CpG islands in vertebrate genomes. J Mol Biol. (1987) 196:261–82. doi: 10.1016/0022-2836(87)90689-9
27. Nguyen, TA, Jones, RD, Snavely, AR, Pfenning, AR, Kirchner, R, Hemberg, M, et al. High-throughput functional comparison of promoter and enhancer activities. Genome Res. (2016) 26:1023–33. doi: 10.1101/gr.204834.116
28. Dong, E, Chen, Y, Gavin, DP, Grayson, DR, and Guidotti, A. Valproate induces DNA demethylation in nuclear extracts from adult mouse brain. Epigenetics. (2010) 5:730–5. doi: 10.4161/epi.5.8.13053
29. Wang, X, Tomso, DJ, Liu, X, and Bell, DA. Single nucleotide polymorphism in transcriptional regulatory regions and expression of environmentally responsive genes. Toxicol Appl Pharmacol. (2005) 207:84–90. doi: 10.1016/j.taap.2004.09.024
30. Collard, BC, and Mackill, DJ. Marker-assisted selection: an approach for precision plant breeding in the twenty-first century. Philos Trans R Soc Lond Ser B Biol Sci. (2008) 363:557–72. doi: 10.1098/rstb.2007.2170
31. Carrell, DT, Emery, BR, and Hammoud, S. Altered protamine expression and diminished spermatogenesis: what is the link? Hum Reprod Update. (2007) 13:313–27. doi: 10.1093/humupd/dml057
32. Wang, K, Liu, X, Qi, T, Hui, Y, Yan, H, Qu, L, et al. Whole-genome sequencing to identify candidate genes for litter size and to uncover the variant function in goats (Capra hircus). Genomics. (2021) 113:142–50. doi: 10.1016/j.ygeno.2020.11.024
33. Liu, T, Ju, X, Zhang, M, Wei, C, Wang, D, Wang, Z, et al. A 67-bp variable duplication in the promoter region of the ADIPOQ is associated with milk traits in Xinjiang brown cattle. Anim Biotechnol. (2021) 33:1738–45. Advance online publication. doi: 10.1080/10495398.2020.1868487
34. Shishay, G, Liu, G, Jiang, X, Yu, Y, Teketay, W, Du, D, et al. Variation in the promoter region of the MC4R gene elucidates the Association of Body Measurement Traits in Hu sheep. Int J Mol Sci. (2019) 20:240. doi: 10.3390/ijms20020240
35. Sobrier, ML, Tsai, YC, Pérez, C, Leheup, B, Bouceba, T, Duquesnoy, P, et al. Functional characterization of a human POU1F1 mutation associated with isolated growth hormone deficiency: a novel etiology for IGHD. Hum Mol Genet. (2016) 25:472–83. doi: 10.1093/hmg/ddv486
Keywords: goat, litter size, CDC25A gene, promoter activity, SNP, KASP
Citation: Zhang T, Wang J, Bai Y, Wang Q, Wang K, Zhu H, Qu L, Guo Z, Pan C and Lan X (2025) A functional SNP of the core promoter region within goat CDC25A gene affects litter size. Front. Vet. Sci. 11:1471123. doi: 10.3389/fvets.2024.1471123
Received: 26 July 2024; Accepted: 22 October 2024;
Published: 05 February 2025.
Edited by:
Kangfeng Jiang, Yunnan Agricultural University, ChinaReviewed by:
Ran Di, Chinese Academy of Agricultural Sciences, ChinaCopyright © 2025 Zhang, Wang, Bai, Wang, Wang, Zhu, Qu, Guo, Pan and Lan. This is an open-access article distributed under the terms of the Creative Commons Attribution License (CC BY). The use, distribution or reproduction in other forums is permitted, provided the original author(s) and the copyright owner(s) are credited and that the original publication in this journal is cited, in accordance with accepted academic practice. No use, distribution or reproduction is permitted which does not comply with these terms.
*Correspondence: Xianyong Lan, bGFueGlhbnlvbmc3OUAxMjYuY29t; Chuanying Pan, Y2h1YW55aW5ncGFuQDEyNi5jb20=
†These authors have contributed equally to this work
Disclaimer: All claims expressed in this article are solely those of the authors and do not necessarily represent those of their affiliated organizations, or those of the publisher, the editors and the reviewers. Any product that may be evaluated in this article or claim that may be made by its manufacturer is not guaranteed or endorsed by the publisher.
Research integrity at Frontiers
Learn more about the work of our research integrity team to safeguard the quality of each article we publish.