- 1State Key Laboratory of Swine and Poultry Breeding Industry, Key Laboratory of Animal Nutrition and Feed Science in South China, Ministry of Agriculture and Rural Affairs, Guangdong Provincial Key Laboratory of Animal Breeding and Nutrition, Guangdong Engineering Technology Research Center of Animal Meat Quality and Safety Control and Evaluation, Institute of Animal Science, Guangdong Academy of Agricultural Sciences, Guangzhou, China
- 2Guangdong Laboratory for Lingnan Modern Agriculture, Heyuan Branch, Heyuan, China
- 3Longping Huangmang Ecological Agriculture Farm, Qingyuan, China
- 4Kaiping Xufeng Farming and Husbandry Co., Ltd, Jiangmen, China
Introduction: The reasonable and efficient utilization of agricultural by-products as animal feed has the capacity to not only mitigate the scarcity of conventional feedstuff but also alleviate the environmental load. This study was aimed to investigate the effects of feeding citrus pomace (CP) fermented with combined probiotics on growth performance, carcass traits, meat quality and antioxidant capacity in yellow-feathered broilers.
Methods: A cohort of 540 female yellow-feathered broilers (Qingyuan partridge chicken, 90-day-old) were randomly divided into three groups and, respectively, fed the basal diet (Control), diet containing 10% unfermented CP (UFCP) and diet containing 10% fermented CP (FCP).
Results: The results showed that dietary FCP significantly increased (p < 0.05) the final-body-weight and average-daily-gain of broilers, and the pH45 min and b*24 h values in breast muscle, while tendentiously lowering the feed-to-gain ratio (p = 0.076). The levels of inosine monophosphate (p < 0.05) and intramuscular fat (p = 0.083) in the FCP group were higher than those in the control group. Remarkably, dietary FCP and UFCP increased the levels of polyunsaturated fatty acids (PUFAs) and n-6 PUFAs (p < 0.05). Moreover, dietary FCP decreased (p < 0.05) the malondialdehyde content and increased (p < 0.05) the glutathione peroxidase content in serum. Ingestion of FCP and UFCP increased the levels of total antioxidant capacity and catalase activity in serum, and concentrations of glutathione peroxidase and catalase in breast muscle (p < 0.05). Additionally, diet containing FCP or UFCP upregulated the expression of SREBP − 1c, FAS, NRF2, GSH-Px, and CAT in breast muscle (p < 0.05).
Discussion: Overall, dietary supplementation with FCP obviously improved meat quality, enhanced the antioxidant capacity and regulated the lipid metabolism, contributing to the improvement of growth performance of yellow-feathered broilers.
1 Introduction
Citrus pomace (CP), encompassing peels, pulps, and seeds, is considered as an environmentally sustainable and economically viable agricultural by-product derived mainly from citrus processing, juicing, or canning in the citrus juice industries. It has been reported that approximately 110 to 120 million tons of CP by-products are generated per year globally (1). In addition to the abundant output, CP exhibits a comprehensive nutritional profile, featuring substantial amounts of crude protein, crude fat, and a diverse array of amino acids; moreover, it contains considerable abundant bioactive substances, such as vitamins, mineral elements, polyphenols, flavonoids, and carotenoids (2). However, owing to the dearth of rational and effective means of disposal, CP is often directly discarded or burned, leading to serious environmental pollution. The nutritional capacity and low price of CP position it as a highly promising candidate for animal feed sources, offering the potential for consumers to obtain antibiotic-free meat and egg products (3, 4).
Nowadays, microbial fermentation has garnered significant interest in the utilization of agro-industrial residues due to its capacity for large-scale treatment and enhancement of their nutritional value and functional attributes of these residues (5). It has been reported that microbial fermentation could improve the digestibility and palatability of agricultural by-products, alongside the concomitant reduction in toxic components of the by-products (5). In addition, solid-state fermentation using Candida utilis and Bacillus subtilis could remarkably increase the content of crude protein, soluble protein, and essential amino acids, while reducing the content of anti-nutritional factors (ANFs) in citrus pulp residue (6). Several studies have demonstrated that the incorporation of microbial fermented citrus peel meal as a partial substitute for corn in chicken diets can significantly enhance growth performance, reduce the feed-to-gain ratio, and exhibit potential improvements in oxidative stability of unsaturated fatty acids in meat (7–9). Additionally, a recent study has shown that supplementation of broiler diets with a methanolic extract of citrus peeling can enhance growth performance, improve immunity, and increase nutrient utilization (10). Despite this, there is currently limited research on the regulation of antioxidant capacity and meat quality in fermented citrus residue fed to broilers.
Yellow-feathered broiler, a native breed of broiler in China, is extensively raised due to its superior meat quality and unique flavor profile, which are highly favored by consumers. However, compared to the white-feathered broiler, it exhibited a slower growth rate and high feed conversion ratio (FCR), leading to elevated feeding costs (11, 12). In addition, the intensive farming of broilers, coupled with an excessive supply of energy-dense feed, has resulted in the production of meat characterized by elevated levels of saturated fat (13). Our previous studies have demonstrated that dietary supplementation with 10 mg/kg citrus extract in the diet of yellow-feather broilers can effectively enhance growth performance, exert anti-inflammatory and antioxidant effects, and improve meat quality (14). On the contrary, the utilization of citrus extract is restricted by its exorbitant cost. Citrus pomace, due to its abundant yield, cost-effectiveness, and substantial nutritional value, can potentially serve as a partial substitute for various feed components such as corn or soybean meal. In our previous in vitro study, results demonstrated that co-fermentation with Aspergillus Niger, Candida tropicalis, Lactobacillus plantarum, and Bacillus subtilis significantly enhanced the protein content while substantially reducing the crude fiber content of citrus residue (15).
Accordingly, we hypothesized that partial replacement of corn with FCP in the diet may have a positive impact on growth performance, meat quality, fatty acid profile and antioxidant capacity of yellow-feather broilers. To test the hypothesis, this study was conducted to investigate the effects of FCP on growth performance, carcass traits, meat quality, muscle fatty acid profile and antioxidant capacity of yellow-feather broilers. Our study aims to provide a theoretical foundation for the potential implementation of FCP in yellow-feather broiler production, and more broadly, in the broiler industry.
2 Materials and methods
2.1 Citrus pomace, fermentation strains, and preparation of fermented citrus pomace
The citrus pomace was purchased from Chongqing Tianbang Food Co., Ltd. (Chong-qing, China). The strain of Aspergillus niger and Candida tropicalis were obtained from the Sericulture and Agricultural Products Processing Institute of Guangdong Academy of Agricultural Sciences (Guangzhou, China). The strain of Lactobacillus plantarum and Bacillus subtilis were from our laboratory.
Preparation of FCP following our previously reported optimized fermentation method (15). In brief, citrus pomace with 60% moisture was inoculated with 20% inoculation amounts of complex probiotics of Aspergillus niger, Candida tropicalis, Bacillus subtilis, and Lactobacillus plantarum in the proportions of 1:1:1:1. The solid fermentation was processed at 30°C for 8 d after thorough mixing, FCP was then obtained. The routine nutrient levels of FCP and unfermented citrus pomace (UFCP) followed our previous study (15).
2.2 Animals, experimental design, and diets
A total of 540 ninety-day-old healthy female yellow-feathered broiler chickens (Qingyuan partridge chicken) were housed in single-layer cages (length 3.2 m × width 1.5 m × height 1.8 m) in which the floors were covered with rice husk. The broilers were raised in a sealed room with a constant temperature of 24°C and a relative humidity ranging from 50 to 60%. The light period consisted of 16 h of light and 8 h of darkness. After a 4-day adaptation period, broilers were then weighed and randomly assigned to 3 treatment groups following their body weight (BW), each group with 6 replicate cages of 30 chickens. Dietary treatment included the following: control (basal corn-soybean diet) and two other treatments in which basal diet was replaced by a 10% level of UFCP or 10% FCP (Table 1). All broilers were allowed ad libitum access to food and water throughout the trial period. The nutrient levels of UFCP and FCP, as measured in our previous studies (15), were utilized for the formulation of three isonitrogenous and isoenergetic diets. The diets (Table 1) among each group were formulated to meet the nutritional requirements of the National Research Council (16) and the Chinese Feeding Standard of Chicken (17). At the age at 94 or 124 days, the feed was cut off and the broilers in each cage were fasted. The weight of each broiler in cages was measured, and the average weight of each cage was calculated. Additionally, the daily feed intake for each cage was recorded. Subsequently, the average daily feed intake (ADFI), the average daily gain (ADG), and the feed to gain ratio (F/G) were calculated from 6 replicate cages per treatment. The F/G ratio was calculated as the total feed intake of six cage per group (kg)/(weight of all broilers in each group at 124 d [kg] – weight of all broilers in each group at 94 d [kg]).
2.3 Slaughtering and sampling
After 8 h of fasting on the last day of rearing, two broilers around the average BW from each replicate cage were electrically stunned and exsanguinated. Broilers were drawn using sterile syringes via the wing vein. The blood samples were centrifuged (at 3,000× rpm for 5 min) to obtain serum and then stored at −20°C for additional analyses. The left breast muscle from the same region was sampled and divided into three parts: one part was sealed in a polyethylene vacuum bag and refrigerated at 4°C for 45 min and 24 h for meat quality analysis, and the other two parts were immediately transferred into liquid nitrogen and stored at −80°C for subsequent chemical composition and quantitative real-time PCR (qRT-PCR) analyses.
2.4 Carcass traits and meat quality
After slaughtering, the left breast meat of 12 broilers per group were excised for measuring carcass traits and meat quality. The carcass traits data were collected and calculated as previously described (18). In brief, boilers were weighed after removing feathers, feet, and beak shells to obtain the carcass weight, and the dressing percentage, semi-eviscerated yield, eviscerated yield, breast muscle yield, leg muscle yield, and abdominal fat yield were calculated. Subsequently, the breast muscles, thigh muscles, and abdominal fat (including fat around the musculature and stomach) were divided and weighed to calculate the percentage of breast muscle, thigh muscle, and abdominal fat based on the carcass weight. At 45 min and 24 h postmortem, the pH and color (L*, a*, and b* values) of breast muscle were determined by an electronic pH meter (model HI 9024C HANNA Instruments, Ltd., Beijing, China) and Chroma meter (model CR-410 Konica Minolta Sensing Ins., Osaka, Japan) separately. The drip loss was measured as described by Zhang et al. (19). Briefly, about 3.0 cm length cube of the breast muscle was manually trimmed and weighed at 45 min postmortem, and suspended by a fishhook in an inflated plastic bag, sealed and stored for 24 h at 4°C, after which the sample was removed from the fishhook, blotted dry on filter paper, and reweighted. Drip loss was expressed as the weight change percentage of the sample before and after storage. To measure the cooking loss, about 25.0 g muscle was weighed, cooked at 85°C and cooled in running water at 4°C. The samples were wiped to remove the surface moisture and reweighed. Cooking loss was expressed as the weight change percentage of the sample before and after cooking. The shear force was determined as described by Yu et al. (20) The samples were cooked at 80°C (internal temperature at 70°C), and cooled in running water at 4°C. Then, the samples were cut to 1 cm × 1 cm × 3 cm sheared parallel to the muscle fiber direction and detected by using a C-LT3B digital-display muscle tenderness determination device (Tenovo, Harbin, China).
2.5 Diet and muscle chemical composition analysis
The chemical composition (metabolizable energy, crude protein, crude ash, crude fat, calcium, and total phosphorus) in experimental diets and the chemical composition (dry matter, crude protein, and IMF) in the breast muscle (12 broilers per group) were analyzed by the methods of the AOAC (21). The content of inosine monophosphate (IMP) in the breast muscle was performed with high-performance liquid chromatography (HPLC) according to the previous study (22). For the fatty acid analysis, the freeze-dried breast muscle samples were pulverized. The lipids of pulverized samples were isolated with chloroform-methanol (1,1, v/v) following Folch et al. (23). The determination of fatty acid methyl esters was carried out on an Agilent 7890B gas chromatographer system with a flame ionization detector (Agilent Technologies Inc., Santa Clara, CA, USA). The composition of fatty acids in the breast muscle was determined as previously described (24).
2.6 Measurement of serum and muscle antioxidant
Serum samples and muscle samples (12 broilers per group) were preprocessed according to the kit instructions. The total antioxidant capacity (T-AOC), total superoxide dismutase (T-SOD), catalase (CAT), and glutathione peroxidase (GSH-Px) activities and the malondialdehyde (MDA) content in serum and muscle were measured using specific kits (Nanjing Jiancheng Bioengineering Institute, Nanjing, China) according to the manufacturer’s instructions.
2.7 Total RNA extraction and quantitative real-time PCR analysis
Total RNA isolation, cDNA synthesis, and qRT-PCR were performed as the previous study (20). Primers used for selected genes are presented in Supplementary Table S1. The housekeeping gene β-actin was used to normalize each target gene expression level, and the relative expression levels of target gene were quantified by the 2−∆∆Ct method (25).
2.8 Statistical analysis
All experimental data was analyzed by the one-way ANOVA by using the SPSS software package (SPSS v. 20.0, SPSS Inc., Chicago IL, United States). The growth performance was evaluated using replicate as the experimental unit, while other experimental data were evaluated using the averages of the 2 sampled broilers per replicate. Duncan’s multiple range test was utilized for differences among groups. Data are expressed as the mean ± SEM. Statistical significance was set at p < 0.05 and statistical trends at 0.05 < p < 0.10. Means within a row with different superscript letters are significantly different (p < 0.05).
3 Results
3.1 Growth performance and carcass traits
The growth performance and carcass traits of yellow-feathered broilers fed diets with UFCP and FCP are presented in Table 2. The broilers fed with FCP significantly increased the final BW and ADG (p < 0.05), and tended to decrease the F/G (p = 0.076) compared with the broilers fed with the basal diet and UFCP, with insignificant differences in the initial BW and ADFI among all three groups (p > 0.10). Additionally, dietary inclusion of FCP and UFCP did not significantly affect the carcass traits, including dressed percentage, semi-eviscerated yield, eviscerated yield, thigh muscle yield, breast muscle yield, and abdominal fat yield (p > 0.10).
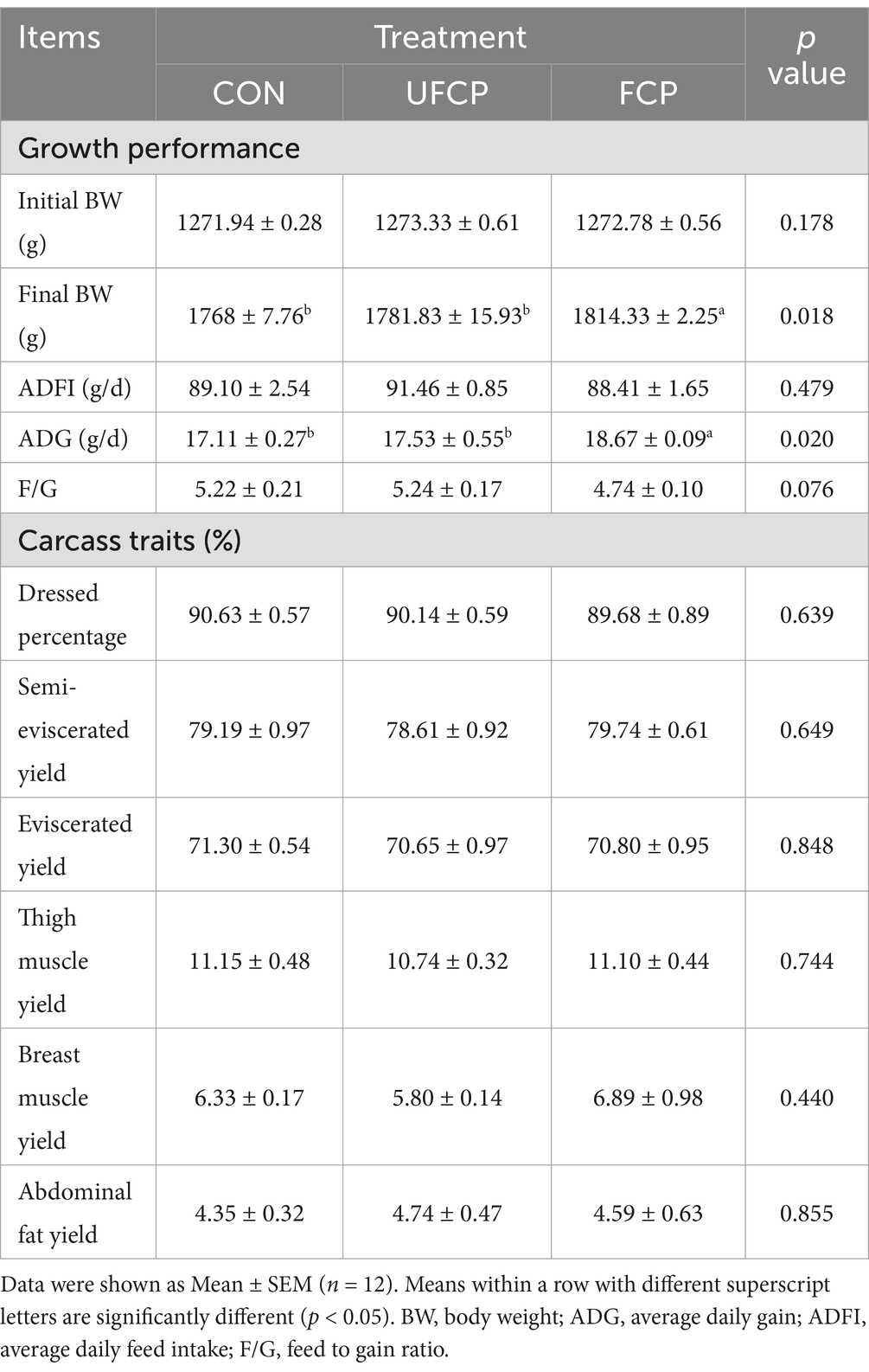
Table 2. Effects of different type of citrus pomace on the growth performance and carcass traits of Yellow-feathered broiler chickens.
3.2 Meat quality and muscle chemical compositions
The meat quality and muscle chemical composition of broilers is shown in Table 3. The broilers fed with FCP significantly increased the pH45 min, b*24 h, and IMP content in the breast muscle (p < 0.05), and tended to increase the IMF content (p = 0.083). The pH45 min and IMP content of breast muscle were increased but were insignificant in broilers treated with UFCP (p > 0.10). In addition, there was no significant difference in pH24 h, L*(45 min and 24 h), a*(45 min and 24 h), b*45 min, drip loss, cooking loss, shear force, and DM and CP contents among the three groups (p > 0.10).
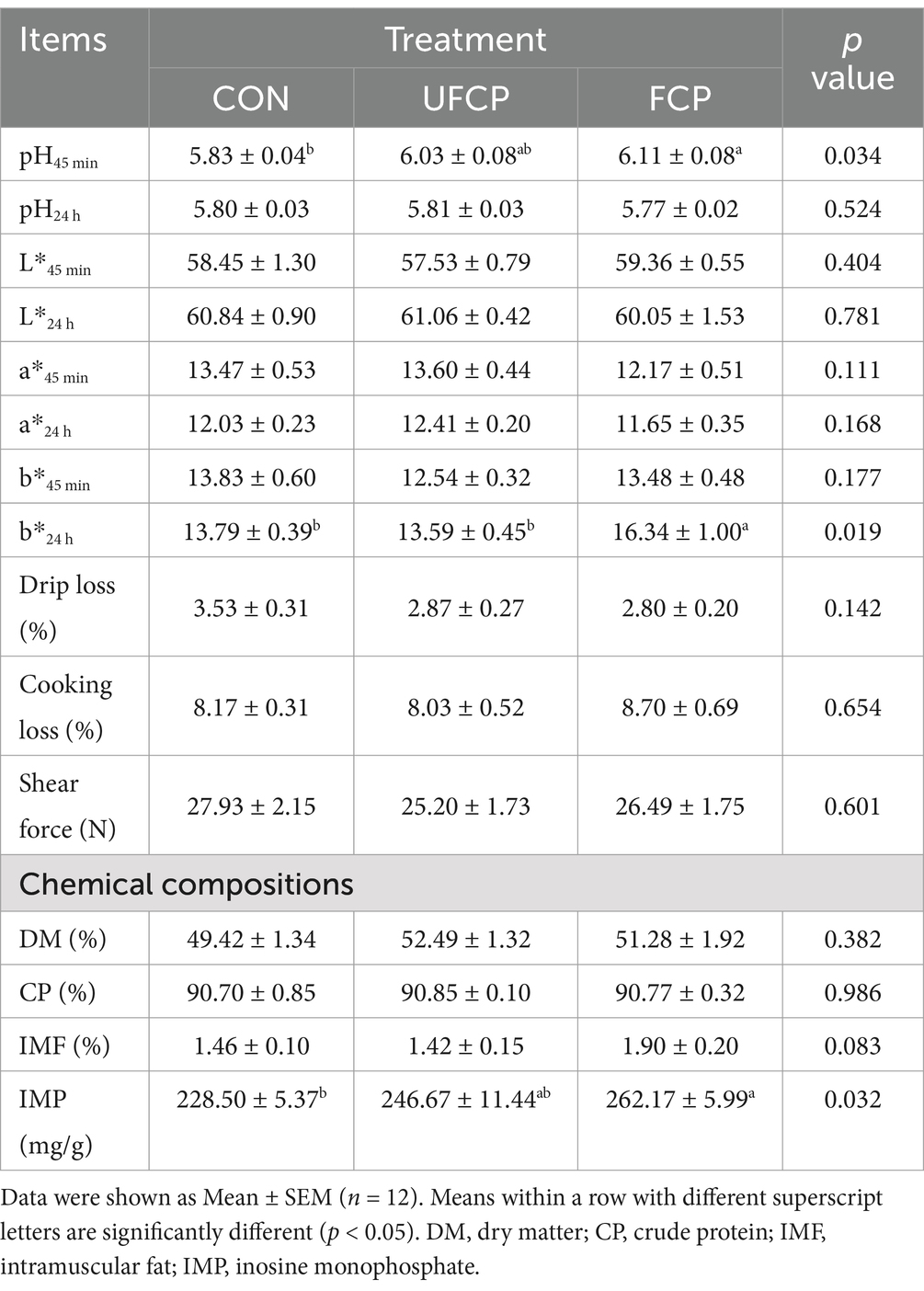
Table 3. Effects of different type of citrus pomace on the meat quality and muscle chemical compositions of Yellow-feathered broiler chickens.
3.3 Fatty acid profiles
The fatty acid profiles of the breast muscle are presented in Table 4. The broilers fed with FCP and UFCP increased the Σ PUFA and Σ n-6 PUFA contents (p < 0.05), and tended to increase the Σ n-3 PUFA content (p = 0.061) and decrease the C18:1n9 (p = 0.056) as well as Σ monounsaturated fatty acids (MUFA) (p = 0.057) contents. In addition, the content of C18:2n6 in the breast muscle was significantly increased in broilers fed with UFCP (p < 0.05). The content of C18:2n6 was increased by dietary inclusion of FCP, but not significant (p > 0.10). Moreover, results showed that FCP and UFCP had no effects on the contents of C14:0, C16:0, C16:1n7, C18:0, C18:3n3, C20:3n6, C20:4n6, C24:0, C22:6n3, Σ saturated fatty acids (SFA) and Σ unsaturated fatty acids (UFA), and the ratio of Σ n-6/n-3 PUFA (p > 0.10).
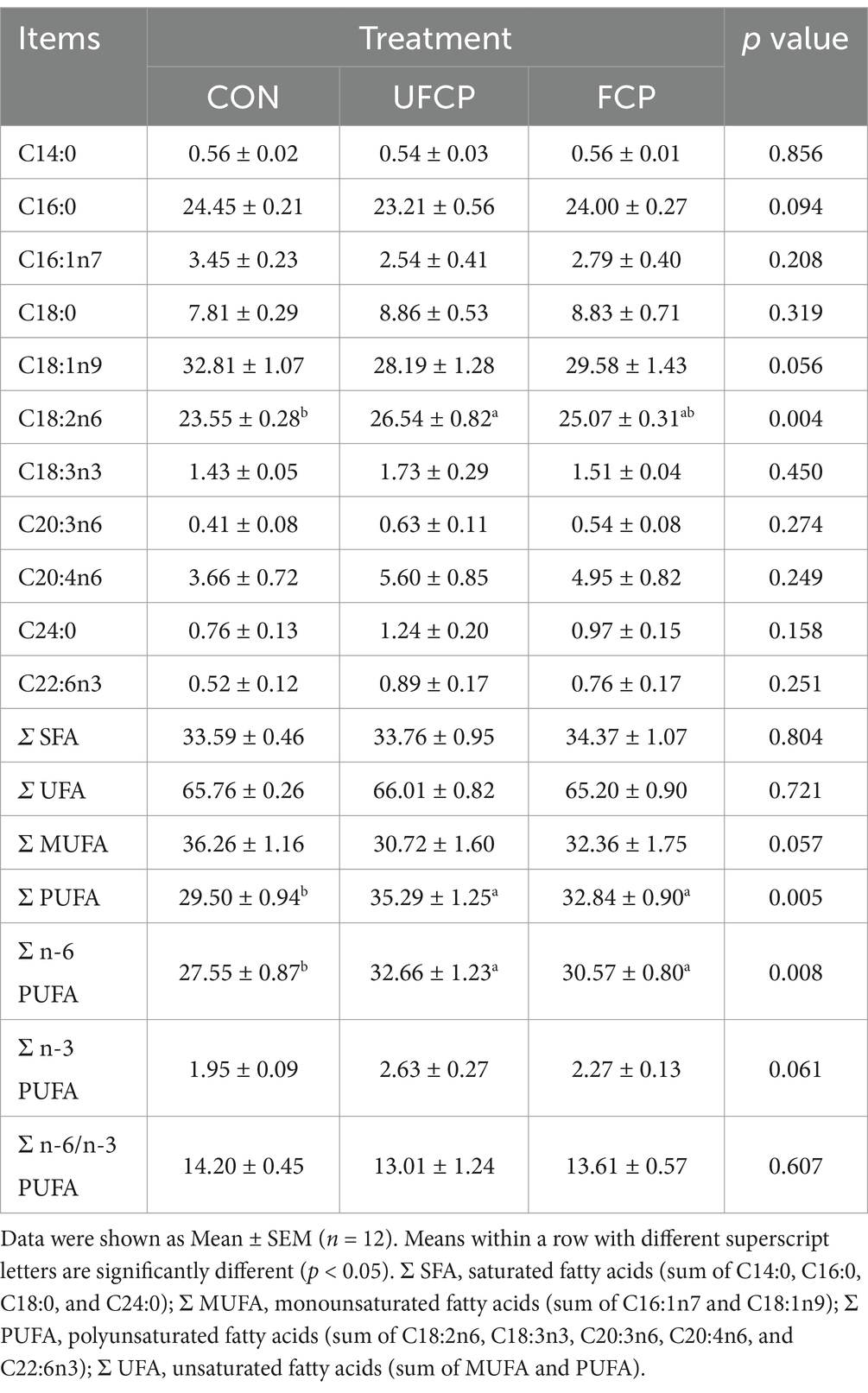
Table 4. Effects of different type of citrus pomace on fatty acid profile of breast muscle of Yellow-feathered broiler chickens (% of total fatty acids).
3.4 Antioxidant capacity
The antioxidant capacity in serum and breast muscle is shown in Table 5. Dietary supplementation with FCP and UFCP considerably increased the serum level of T-AOC, bolstered the activity of CAT in serum, and the activity of CAT and GSH-Px in breast muscle (p < 0.05). Additionally, the incorporation of FCP into the diets remarkably reduced the content of MDA and increased the GSH-Px activity in serum, respectively (p < 0.05).
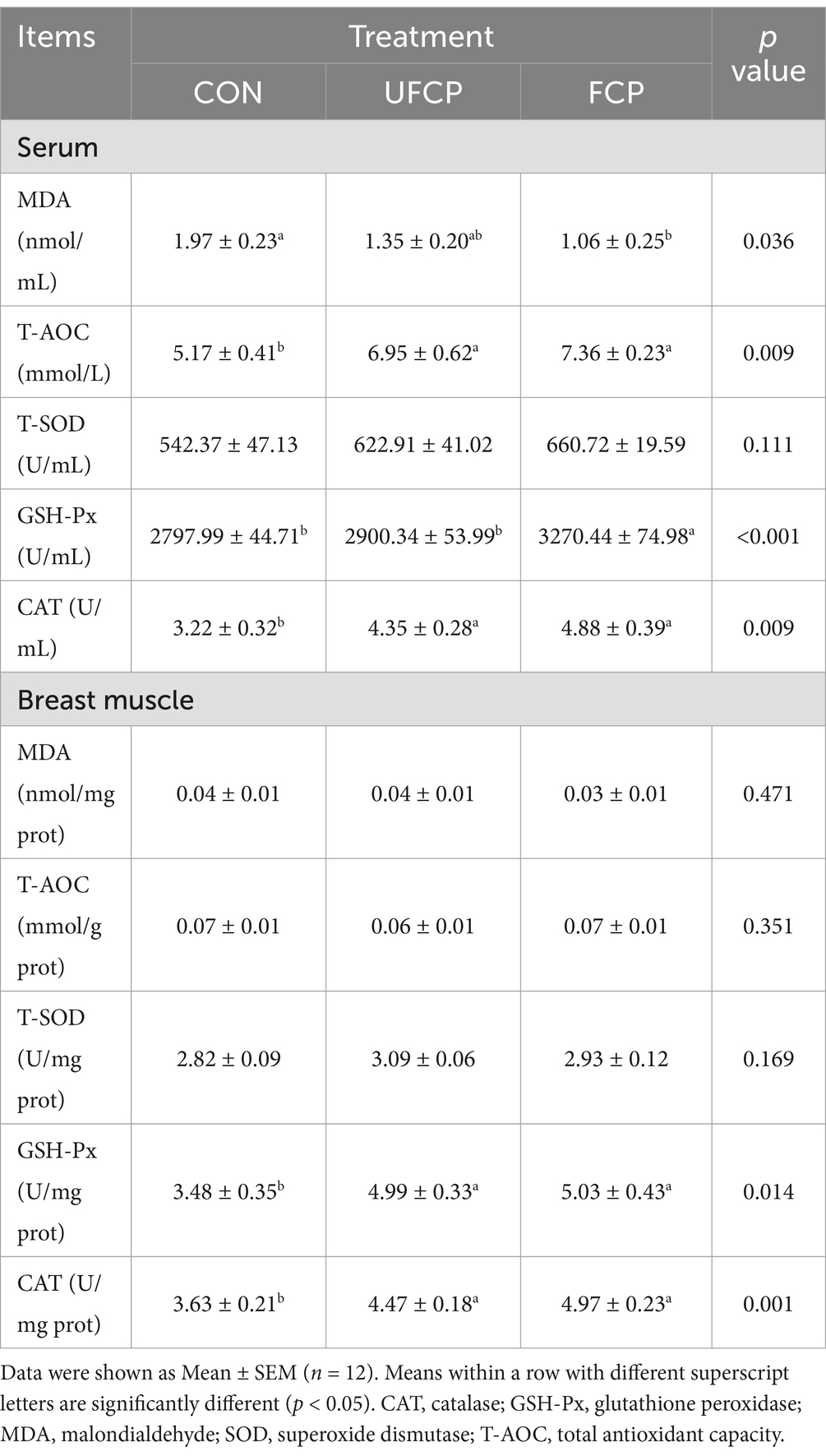
Table 5. Effects of different type of citrus pomace on antioxidant capacity of of Yellow-feathered broiler chickens.
3.5 Relative mRNA expression of genes associated with lipid metabolism and antioxidant capacity in breast muscle
To further investigate the mechanisms involved in the regulation of IMF and PUFA deposition, the relative mRNA expression of genes related to lipid metabolism in breast muscle (Figure 1) was detected by qRT-PCR. The relative mRNA expression of SREBP-1c and FAS in the breast muscle was elevated when either FCP or UFCP was incorporated into the diets (p < 0.05). In addition, dietary incorporation of FCP and UFCP was tended to decreased the relative mRNA expression of SCD1 in breast muscle (p = 0.070). No significant differences in the relative mRNA expression of PPARγ, ACC, FABP1, CPT1, and ACOX1 among the three groups (p > 0.10).
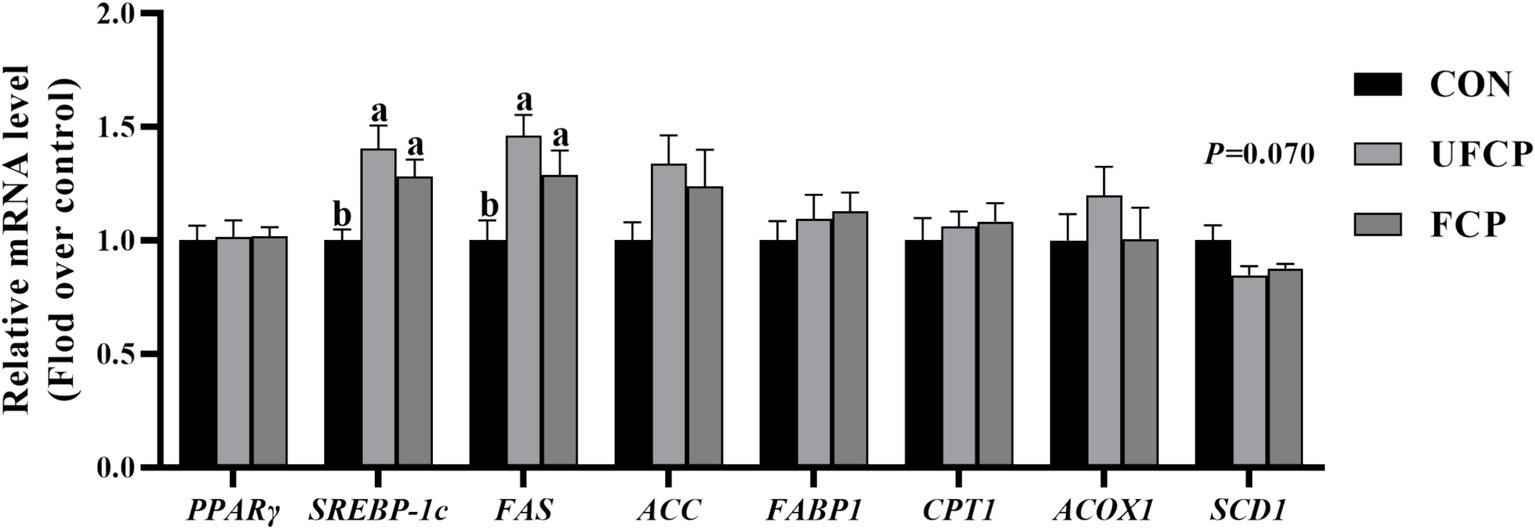
Figure 1. Effect of different type of citrus pomace on the mRNA expression levels of lipid metabolism in breast muscle of Yellow-feathered broiler chickens. Data were shown as Mean ± SEM (n = 12). Means within a row with different superscript letters are significantly different (p < 0.05). PPARγ, proliferator-activated receptors; SREBP–1c, Sterol regulatory element-binding protein 1c; FAS, fatty acid synthase; FABP1, Fatty Acid Binding Protein 1; CPT1, carnitine palmitoyltransferase 1; ACOX1, Acyl-CoA Oxidase 1; SCD1, Stearoyl-CoA Desaturase.
The relative mRNA expression of genes associated with antioxidants in breast muscle is present in Figure 2. The relative mRNA expression of nuclear factor erythroid 2–related factor 2 (NRF2) and its downstream genes, including GSH-Px and CAT, were sharply upregulated following the inclusion of FCP or UFCP in the diets (p < 0.05). However, the incorporation of neither FCP nor UFCP did not remarkably change the relative mRNA expression of HO-1 and SOD (p > 0.10).
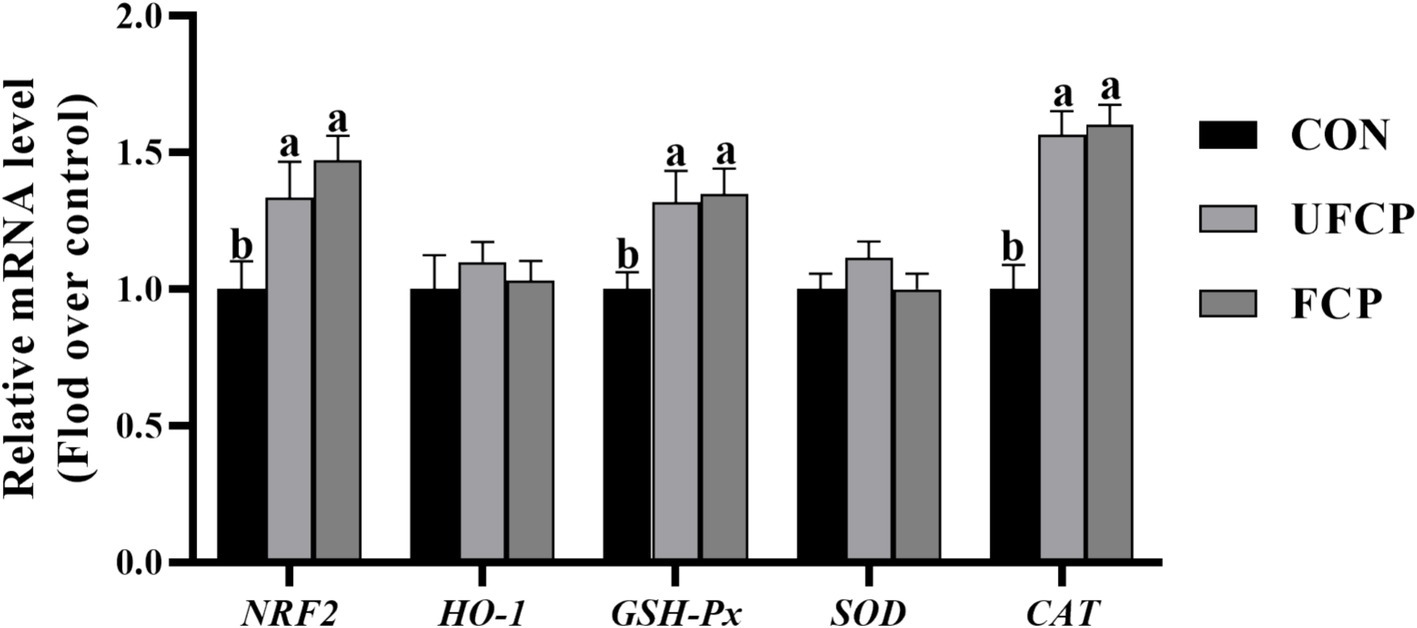
Figure 2. Effect of different type of citrus pomace on the mRNA expression levels of antioxidants in breast muscle of Yellow-feathered broiler chickens. Data were shown as Mean ± SEM (n = 12). Means within a row with different superscript letters are significantly different (p < 0.05). NRF2, nuclear factor-erythroid 2 related factor 2; HO–1, Heme Oxygenase-1; GSH–Px, glutathione peroxidase; SOD, Superoxide Dismutase; CAT, catalase.
4 Discussion
Citrus pomace, an agricultural by-product, has excellent potential for feed development in animal production due to its rich functional and nutritional components, as well as the characteristics of high yield, cost-effectiveness, and renewability (26). Unfortunately, its broad application is limited due to the presence of high fiber content and low protein content. Microbial fermentation is an effective approach that generates biological substances with health-promoting properties and ameliorates the nutritional value of plants (27). Our results showed that FCP increased the growth performance and meat quality in yellow-feathered broiler chickens. Additionally, both FCP and UFCP could improve fatty acid profile and antioxidant capacity. To sum up, FCP can be used as a good substitute for feed ingredients to improve the growth performance and antioxidant capacity of broilers and produce chicken meat that is more nutritious and meets consumer needs.
4.1 Effect of dietary FCP on growth performance of yellow-feather broilers
Previous studies have been carried out on the dietary inclusion of citrus pulp and citrus peel in poultry, but few studies are hitherto available on the dietary effect of citrus pomace. The growth performance can provide essential information for the application of citrus pomace in animal production. Herbal products with considerable antioxidative substances could ameliorate growth performance by enhancing antioxidant ability (28). In the current study, dietary inclusion of FCP at 10% enhanced the antioxidant capacity, increased BW and ADG, and decreased F/G in broilers. Similarly, Maha et al. (29) showed that dietary inclusion of orange peel increased the serum GSH level and improved the BW in broilers, suggesting that the effect on growth performance of broilers might be attributed to the antioxidant activity exhibited by orange peel. Nevertheless, dietary 10% UFCP supplementation did not alter the growth performance of broilers. Due to the isonitrogenous and isoenergetic diets employed in this study, inconsistent results regarding the growth performance of broilers in response to FCP and UFCP treatments may be attributed to the reduction of anti-nutritional factors (ANFs), such as non-starch polysaccharides (NSP), in citrus pomace after microbial fermentation. Citrus pomace contains many NSPs, which may have a negative impact on the digestion and absorption of nutrients (30). Due to the presence of considerable levels of NSPs in UFCP, it may have offset somewhat of the positive effect of enhanced antioxidant capacity on growth performance. The NSP in citrus pomace could be broken down during the process of microbial fermentation by generating diverse NSP enzymes (31, 32), which may assist in improving the availability and absorption of nutrients, thereby improving growth performance. Furthermore, the utilization of FCP offered several advantages in maintaining intestinal health, including the enhancement of intestinal microbial structure and the production of a greater quantity of beneficial bioactive metabolites. Consequently, it may potentially contribute to the promotion of broiler growth (33). These may partly account for the improvement in the growth performance of broilers by dietary FCP. However, further studies are necessary to investigate the exact mechanism by which FCP boosts growth due to its complex composition. These results indicated that FCP could be used as a good feed ingredient since replacing partially basal diets with FCP possesses a superior growth performance in yellow-feather broilers when compared with UFCP.
4.2 Effect of dietary FCP on the meat quality of yellow-feather broilers
Meat pH is an important indicator for evaluating meat quality as it is related to the shelf life, color, and water-holding capacity of meat. The value of pH was determined mainly by the degree of muscle glycolysis after animal slaughter, and a rapid pH drop can lead to protein denaturation, causing paleness and low water-holding capacity of meat, thereby reducing nutrient value (34, 35). Lu et al. (36) demonstrated that dietary 2–8% fermented pineapple residue increased the pH value at 45 min in the meat of broilers. The pineapple residue is known to contain high proportions of phenols and flavonoids, which exhibit potent antioxidant capacity (37). We obtained similar results that the pH value at 45 min in the meat of broilers was increased in both the FCP and UFCP groups. The beneficial effects of the FCP and UFCP on meat pH can be explained by polyphenols, which can reduce the activity of lactate dehydrogenase and the process and potential of anaerobic glycolysis in muscle, thereby decreasing the content of lactic acid and increasing pH value in meat (38, 39).
Meat color primarily affects consumer preferences for meat products, including L*, a*, and b* values. In this study, the b* value at 24 h of the broiler breast muscle was increased with along fed with FCP. However, dietary supplementation with UFCP was no significant in b* value at 24 h of the broiler breast muscle. Previous studies suggested that the addition to broiler feed of dried pomace of apples, cherries and strawberries showed no significant change in b* value (40). These findings suggested that the variation in b* value at 24 h of the broiler breast muscle may attributed to the retention or synthesis of specific bioactive compounds during fermentation, thereby influencing its alteration during storage. Nevertheless, further research is required to investigate the impact of FCP on variations in the b* value at 24 h in yellow-feathered broilers.
In addition, the results of this study showed that broilers fed with the FCP also increased the content of IMF and IMP in the breast muscle. IMF is one of the key indices of meat quality, positively correlated with the flavor, juiciness, and tenderness of the meat (41). IMP is considered an umami flavor enhancer, as it can affect the umami flavor of meat after cooking (20). Thus, the higher content of IMF and IMP in breast muscle indicated that FCP could partially replace corn and soybean meal in diets and may help improve meat tenderness, juiciness, and flavor. The effect of FCP and UFCP on regulating the IMF and IMP in the breast muscle of broilers has not been unambiguously investigated to date, and it is difficult to explain this phenomenon. A popular explanation for this phenomenon is that microbes and their metabolites may promote IMF accumulation and IMP synthesis in muscle (42, 43). However, there is little available information regarding the effect of FCP on meat quality, and these explanations need further verification.
The fatty acid composition of IMF serves as a vital quality attribute that consumers are concerned, it plays an important role in meat quality, and determines the flavor and nutritional value of the meat (44). Food with a higher PUFA content and a lower ratio of n-6/n-3 PUFA are readily accepted and desirable by consumers due to their nutritional value and health-enhancing properties (45, 46). Our results showed that diets supplemented with FCP and UFCP exhibit higher contents of C18:2n6, Σ PUFA, Σ n-6 PUFA, and Σ n-3 PUFA in breast muscle in comparison with the CON group. Similarly, Tayengwa et al. (47) found that diet supplementation with citrus pulp could increase the proportions of C18:2n6, Σ n-3 PUFA and Σ n-6 PUFA. Actually, many organisms, including but not limited to humans, cannot synthesize n-6 PUFA and n-3 PUFA by themselves, which are solely obtained from diets (48). Assefa et al. (49) reported that citrus exhibits significant antioxidant potential and is abundant in linoleic acid. As a result, the alteration of these above results might have been due to a certain level of PUFA, such as linoleic acid (C18:2n6) and linolenic acid (C18:3n3), present in citrus pomace (50, 51), they are the precursor of n-6 PUFA and n-3 PUFA families, respectively (52). Chicken meat possesses a considerable content of PUFA in comparison with other meats, so it is vulnerable to oxidative stress (35). For this reason, the use of antioxidants in the diet has been recommended to limit lipid peroxidation, maintain animal health, and achieve better product quality. Notably, studies have confirmed that plant extracts or dietary fermented blueberry pomace supplementation prevent lipoperoxidation (53). Therefore, we speculate that the increase in C18:2n6, Σ PUFA, Σ n-6 PUFA, and Σ n-3 PUFA in breast muscle can be partly attributed to the enhanced antioxidant properties, which could protect PUFA from oxidative stress. Indeed, enhanced antioxidant capacity in this study supports this speculation. In general, our results suggested that a diet supplemented with FCP may have a positive effect on meat quality as it contributes to increasing the values of pH and b*, the content of IMF and IMP, and improving the fatty acid composition.
4.3 Effect of dietary FCP on the mRNA expression levels of genes involved in the lipid metabolism of yellow-feather broilers
To investigate the molecular mechanism of IMF and PUFA changes in breast muscle, we measured the related-gene expression involved in lipid metabolism in the breast muscle, and an upregulated relative mRNA expression of lipid synthesis-related factors SREBP1-c and FAS was observed from broilers fed FCP and UFCP. SREBP1-c is a transcription factor that can regulate the expression of genes involved in the de novo fatty acid synthesis, including fatty acid synthesis, elongation, and desaturation (54). FAS, a well-known target of SREBP1-c, is mainly involved in the synthesis of long-chain fatty acids (55). Therefore, in this study, the IMF content in the FCP group and the proportion of PUFA, n-6 PUFA, and n-3 PUFA in FCP and UFCP groups were increased, possibly due to the upregulation of relative mRNA expression of SREBP1-c and FAS. Although dietary incorporation of UFCP upregulated the relative mRNA expression of SREBP1-c and FAS, it did not alter the IMF content in chicken meat. A previous study reported that the IMF content in the breast and thigh muscles of broiler chicks decreased with an increased NSP content from the wheat diet (56). Accordingly, the high content of NSPs in UFCP may be responsible for this phenomenon. Simultaneously, we also observed a reduction in the relative mRNA expression of SCD1 in response to FCP and UFCP treatment. SCD1 is the rate-limiting enzyme that can convert SFA into MUFA (57). This may explain why the MUFA in chicken meat was decreased in broilers fed with FCP and UFCP.
4.4 Effect of dietary FCP on the antioxidant capacity of yellow-feather broilers
The antioxidant ability is positively correlated with a healthy body and meat quality (58). MDA, T-AOC, SOD, CAT, and GSH-PX are the primary indicators reflecting the degree of oxidative stress in the body. Our results showed that the dietary inclusion of FCP and UFCP increased the activities of the antioxidant enzymes GSH-Px and CAT in serum and breast muscle, and T-AOC in serum, whereas decreased the serum content of MDA. The study conducted by Gungor et al. (59) reported an increase in the activity of GSH-Px in the serum of broiler chicks following the dietary inclusion of fermented and unfermented grape pomace. Similarly, the dietary inclusion of fermented and unfermented pomegranate pomace has been found to decrease the MDA content in the breast muscle of broiler chickens (60). The activity of antioxidant enzymes depends in part on their expression levels of the antioxidant gene, which is controlled by NRF2 (61). In the present study, we further observed that the gene expression levels of NRF2, GSH-Px, and CAT in breast muscle were elevated by both FCP and UFCP supplementation. Huang et al. (62) reported that apple polyphenols improve intestinal antioxidant capacity by activating the NRF2/Keap1 Signaling and improving the mRNA expression of related antioxidant genes. Consequently, we have reason to believe that dietary inclusion of FCP and UFCP augments the health of the host and oxidative stability of chicken meat by promoting the gene expression of enzymes related to antioxidants through activating the NRF2 signaling pathway. The presence of phenolic compounds and flavonoid compounds in citrus pomace may account for the enhancement in broilers’ antioxidant capacity when consuming FCP and UFCP. These bioactive substances supply a powerful antioxidant capacity to the organism by facilitating the antioxidant enzyme systems with a good radical scavenging ability (63). Collectively, these results suggested that supplementation of FCP and UFCP at 10% inclusion levels in the diets of broilers could bolster antioxidant capacity and thereby contribute to ameliorating the health and meat quality of chickens.
5 Conclusion
Collectively, our study revealed that the incorporation of FCP into the diet could improve the growth performance and meat quality in yellow-feather broilers. Additionally, the dietary inclusion of either 10% FCP or 10% UFCP exhibited a beneficial effect on the fatty acid profile and antioxidant capacity in yellow-feather broilers. Moreover, alteration of expression of genes related to lipid metabolism and antioxidants might support these benefits. These findings provide a promising outlook for FCP and UFCP as viable and nutrient-rich components for inclusion in broiler chicken feed formulations. Given these results above, the inclusion of FCP was recommended when added to the diet of broiler chickens. However, the optimum inclusion level of FCP in the diets of broiler chickens should be further investigated.
Data availability statement
The datasets presented in this study can be found in online repositories. The names of the repository/repositories and accession number(s) can be found in the article/Supplementary material.
Ethics statement
The animal study was approved by Animal Ethics of the Institute of Animal Science, Guangdong Academy of Agriculture Science, Guangzhou, China (authorization number GAASIAS-2016-017). The study was conducted in accordance with the local legislation and institutional requirements.
Author contributions
YCL: Software, Visualization, Writing – original draft. YT: Data curation, Formal analysis, Investigation, Writing – review & editing. HM: Formal analysis, Investigation, Software, Writing – review & editing. ZCL: Formal analysis, Project administration, Writing – review & editing. ZML: Formal analysis, Writing – review & editing. XM: Writing – review & editing. ZHL: Resources, Writing – review & editing. WH: Resources, Writing – review & editing. YFL: Conceptualization, Validation, Writing – review & editing. MY: Conceptualization, Supervision, Writing – review & editing.
Funding
The author(s) declare financial support was received for the research, authorship, and/or publication of this article. This research was funded by, State Key Laboratory of Swine and Poultry Breeding Industry (2023QZ-NK09, ZQQZ-03, GDNKY-ZQQZ-K2), Special Fund for Scientific Innovation Strategy-Construction of High-Level Academy of Agriculture Science-Distinguished Scholar (R2020YJ-YB2002, R2023PY-JX015), Science and Technology Program of Guangdong Province (2023B0202010020), Science and Technology Program of Qingyuan City (2021DZX018), Science and Technology Program of Jiangmen City (2021660200370006997), Modern Agricultural Industrial Park Project of Qingcheng District, Qingyuan City, Guangdong Province, and the Science and Technology Research Project of Education Department of Jiangxi Province (GJJ191140).
Acknowledgments
The authors would like to thank Zhimei Tian, Min Song, Dun Deng, Ting Rong, Yiyan Cui for their help.
Conflict of interest
WH was employed by Kaiping Xufeng Farming and Husbandry Co., Ltd.
The remaining authors declare that the research was conducted in the absence of any commercial or financial relationships that could be construed as a potential conflict of interest.
Publisher’s note
All claims expressed in this article are solely those of the authors and do not necessarily represent those of their affiliated organizations, or those of the publisher, the editors and the reviewers. Any product that may be evaluated in this article, or claim that may be made by its manufacturer, is not guaranteed or endorsed by the publisher.
Supplementary material
The Supplementary material for this article can be found online at: https://www.frontiersin.org/articles/10.3389/fvets.2024.1469947/full#supplementary-material
Abbreviations
ADFI, average daily feed intake; ADG, average daily gain; ANFs, anti-nutritional factors; AOAC, Association of Official Analytical Chemists; BW, body weight; CAT, catalase; FCP, fermented citrus pomace; F/G, feed to gain ratio; GSH-Px, glutathione peroxidase; HPLC, high-performance liquid chromatography; IMF, intramuscular fat; IMP, inosine monophosphate; MDA, malondialdehyde; MUFA, monounsaturated fatty acid; NRF2, nuclear factor erythroid 2–related factor 2; NSP, non-starch polysaccharide; PUFA, polyunsaturated fatty acid; qRT-PCR, quantitative real-time PCR; SFA, saturated fatty acid; T-AOC, total antioxidant capacity; T-SOD, total superoxide dismutase; UFA, unsaturated fatty acid; UFCP, unfermented citrus pomace.
References
1. Šafranko, S, Stanković, A, Hajra, S, Kim, H-J, Strelec, I, Dutour-Sikirić, M, et al. Preparation of multifunctional N-doped carbon quantum dots from Citrus Clementina Peel: investigating targeted pharmacological activities and the potential application for Fe3+ sensing. Pharmaceuticals. (2021) 14:857. doi: 10.3390/ph14090857
2. Kesbiç, OS, Acar, Ü, Mohammady, EY, Salem, SM, Ragaza, JA, El-Haroun, E, et al. The beneficial effects of Citrus Peel waste and its extract on fish performance and health status: a review. Aquac Res. (2022) 53:4217–32. doi: 10.1111/are.15945
3. Vlaicu, PA, Untea, AE, Panaite, TD, and Turcu, RP. Effect of dietary Orange and grapefruit Peel on growth performance, health status, meat quality and intestinal microflora of broiler chickens. Ital J Anim Sci. (2020) 19:1394–405. doi: 10.1080/1828051X.2020.1845576
4. Nazok, A, Rezaei, M, and Sayyahzadeh, H. Effect of different levels of dried Citrus pulp on performance, egg quality, and blood parameters of laying hens in early phase of production. Trop Anim Health Prod. (2010) 42:737–42. doi: 10.1007/s11250-009-9481-x
5. Munekata, PES, Domínguez, R, Pateiro, M, Nawaz, A, Hano, C, Walayat, N, et al. Strategies to increase the value of pomaces with fermentation. Fermentation. (2021) 7:299. doi: 10.3390/fermentation7040299
6. Mnisi, CM, Mlambo, V, Kumanda, C, and Crafford, A. Effect of graded levels of red grape pomace (Vitis Vinifera L.) powder on physiological and meat quality responses of Japanese quail. Acta Agriculturae Scandinavica, section A. Anim Sci. (2021) 70:100–6. doi: 10.1080/09064702.2021.1923796
7. Ahmed, ST, Mun, HS, Islam, MM, Kim, SS, Hwang, JA, Kim, YJ, et al. Effects of Citrus Junos by-products fermented with multistrain probiotics on growth performance, immunity, Caecal microbiology and meat oxidative stability in broilers. Br Poult Sci. (2014) 55:540–7. doi: 10.1080/00071668.2014.938021
8. Odunlade, TA, Oluremi, OIA, and Abang, FBP. Effect of the nutrient composition of biodegraded sweet Orange (Citrus Sinensis) fruit Peel on the growth performance of starter broiler chicks. Anim Vet Sci. (2020) 8:104–9. doi: 10.11648/j.avs.20200805.13
9. Oyewole, B, Oluremi, O, Aribido, S, and Ayoade, J. Comparative chemical composition of 24-hour fermented sweet Orange fruit (Citrus Sinensis) Peel meal and maize and effect on performance response of starting pullet chicks. Niger J Anim Prod. (2012) 39:57–65. doi: 10.51791/njap.v39i2.831
10. Ahmad, F, Sultan, A, Khan, S, Ali, M, Ali, I, Abdullah, H, et al. Effect of Citrus peeling (Citrus Sinensis) on production performance, humoral immunity, nutrients, and energy utilization of broiler quails. Poult Sci. (2024) 103:103207. doi: 10.1016/j.psj.2023.103207
11. Wang, L-d, Zhang, Y, Kong, L-l, Wang, Z-x, Bai, H, Jiang, Y, et al. Effects of rearing system (floor vs. cage) and sex on performance, meat quality and enteric microorganism of yellow feather broilers. J Integr Agric. (2021) 20:1907–20. doi: 10.1016/S2095-3119(20)63420-7
12. Zhang, Q, Zhang, H, Jiang, Y, Wang, J, Wu, D, Wu, C, et al. Chromium propionate supplementation to energy-and protein-reduced diets reduces feed consumption but improves feed conversion ratio of yellow feathered male broilers in the early period and improves meat quality. Poult Sci. (2023) 103:103260. doi: 10.1016/j.psj.2023.103260
13. Ebrahimi, A, Qotbi, AAA, Seidavi, A, and Bahar, B. The effects of dietary supplementation of Citrus Sinensis Peel extract on production and quality parameters of broiler chicken. J Appl Anim Res. (2014) 42:445–50. doi: 10.1080/09712119.2013.875916
14. Yu, M, Li, Z, Wang, G, Cui, Y, Rong, T, Tian, Z, et al. Dietary supplementation with Citrus extract alters the plasma parameters, circulating amino acid profiles and gene expression of small intestinal nutrient transporters in Chinese yellow-feathered broilers. J Sci Food Agric. (2020) 100:5126–35. doi: 10.1002/jsfa.10525
15. Mei, H, Tang, Y, Ma, X, Li, Y, Luo, Z, Huang, W, et al. Screening of fermentation Technology for Producing Protein Feed from Citrus pomace. Feed Res. (2022) 45:60–3. doi: 10.13557/j.cnki.issn1002-2813.2022.14.014
16. National Academies Press. Nutrient requirements of poultry National Academies Press (1994). Available at: https://nap.nationalacademies.org/catalog/2114/nutrient-requirements-of-poultry-ninth-revised-edition-1994
18. Ye, J, Jiang, S, Cheng, Z, Ding, F, Fan, Q, Lin, X, et al. Feed restriction improves lipid metabolism by changing the structure of the Cecal microbial community and enhances the meat quality and flavor of bearded chickens. Animals. (2022) 12:970. doi: 10.3390/ani12080970
19. Zhang, C, Yang, L, Zhao, X, Chen, X, Wang, L, and Geng, Z. Effect of dietary resveratrol supplementation on meat quality, muscle Antioxidative capacity and mitochondrial biogenesis of broilers. J Sci Food Agric. (2018) 98:1216–21. doi: 10.1002/jsfa.8576
20. Yu, M, Li, Z, Rong, T, Wang, G, Liu, Z, Chen, W, et al. Different dietary starch sources Alter the carcass traits, meat quality, and the profile of muscle amino acid and fatty acid in finishing pigs. J Anim Sci Biotechnol. (2020) 11:78. doi: 10.1186/s40104-020-00484-9
21. AOAC. Official methods of analysis association of official analytical chemists. Gaithersburg, Ed. George W. MD: William Horwitz (2007).
22. Li, X, He, L, Yang, Y, Liu, F, Cao, Y, and Zuo, J. Effects of extracellular polysaccharides of Ganoderma Lucidum supplementation on the growth performance, blood profile, and meat quality in finisher pigs. Livest Sci. (2015) 178:187–94. doi: 10.1016/j.livsci.2015.04.001
23. Folch, J, Lees, M, and Stanley, GHS. A simple method for the isolation and purification of Total Lipides from animal tissues. J Biol Chem. (1957) 226:497–509. doi: 10.1016/S0021-9258(18)64849-5
24. Yu, M, Li, Z, Chen, W, Rong, T, Wang, G, Li, J, et al. Use of Hermetia Illucens larvae as a dietary protein source: effects on growth performance, carcass traits, and meat quality in finishing pigs. Meat Sci. (2019) 158:107837. doi: 10.1016/j.meatsci.2019.05.008
25. Yu, M, Mu, C, Yang, Y, Zhang, C, Su, Y, Huang, Z, et al. Increases in circulating amino acids with in-feed antibiotics correlated with gene expression of intestinal amino acid transporters in piglets. Amino Acids. (2017) 49:1587–99. doi: 10.1007/s00726-017-2451-0
26. Azizi, M, Seidavi, A, Ragni, M, Laudadio, V, and Tufarelli, V. Practical applications of agricultural wastes in poultry feeding in Mediterranean and Middle East regions. Part 1: Citrus, grape, pomegranate and apple wastes. Worlds Poult Sci J. (2018) 74:489–98. doi: 10.1017/S0043933918000478
27. Zannini, D, Dal Poggetto, G, Malinconico, M, Santagata, G, and Immirzi, B. Citrus pomace biomass as a source of pectin and lignocellulose fibers: from waste to upgraded biocomposites for mulching applications. Polymers. (2021) 13:1280. doi: 10.3390/polym13081280
28. Rajput, SA, Sun, L, Zhang, N, Khalil, MM, Gao, X, Ling, Z, et al. Ameliorative effects of grape seed Proanthocyanidin extract on growth performance, immune function, antioxidant capacity, biochemical constituents, liver histopathology and aflatoxin residues in broilers exposed to aflatoxin B1. Toxins. (2017) 9:371. doi: 10.3390/toxins9110371
29. Abd El Latif, MA, Abdel-Wareth, AAA, Daley, M, and Lohakare, J. Effect of dietary Orange Peel meal and multi-enzymes on productive, physiological and nutritional responses of broiler chickens. Animals. (2023) 13:473. doi: 10.3390/ani13152473
30. Mourão, JL, Pinheiro, V, Prates, J, Bessa, R, Ferreira, L, Fontes, C, et al. Effect of dietary dehydrated pasture and Citrus pulp on the performance and meat quality of broiler chickens. Poult Sci. (2008) 87:733–43. doi: 10.3382/ps.2007-00411
31. Hu, X, Zeng, J, Shen, F, Xia, X, Tian, X, and Wu, Z. Citrus pomace fermentation with autochthonous probiotics improves its nutrient composition and antioxidant activities. Lwt. (2022) 157:113076. doi: 10.1016/j.lwt.2022.113076
32. Huang, W-Q, Hu, X, Zeng, J-R, Tian, X-F, and Wu, Z-Q. Changing the nutrient composition and enhancing the hydrolytic enzyme activity of Citrus pulp residue by Cofermentation with Candida Utilis and Bacillus Subtilis. Process Biochem. (2021) 107:83–90. doi: 10.1016/j.procbio.2021.05.005
33. Uerlings, J, Arévalo Sureda, E, Schroyen, M, Kroeske, K, Tanghe, S, De Vos, M, et al. Impact of Citrus pulp or inulin on intestinal microbiota and metabolites, barrier, and immune function of weaned piglets. Front Nutr. (2021) 8:8. doi: 10.3389/fnut.2021.650211
34. Liu, X, Cao, G, Zhou, J, Yao, X, and Fang, B. The effects of Bacillus Coagulans-fermented and non-fermented Ginkgo Biloba on abdominal fat deposition and meat quality of Peking duck. Poult Sci. (2017) 96:2264–73. doi: 10.3382/ps/pex017
35. Cao, F, Zhang, X, Yu, W, Zhao, L, and Wang, T. Effect of feeding fermented Ginkgo Biloba leaves on growth performance, meat quality, and lipid metabolism in broilers. Poult Sci. (2012) 91:1210–21. doi: 10.3382/ps.2011-01886
36. Lu, P, Guo, R, Zou, C, Chen, H, Chen, D, Yang, L, et al. Insight into the chemical composition, antioxidant capacity, meat quality, fatty acid profile, and volatile compounds of yellow-feathered chickens fed with fermented pineapple residue. Food Chem. (2024) 24:101874. doi: 10.1016/j.fochx.2024.101874
37. Aziz, M, and Karboune, S. Natural antimicrobial/antioxidant agents in meat and poultry products as well as fruits and vegetables: a review. Crit Rev Food Sci Nutr. (2018) 58:486–511. doi: 10.1080/10408398.2016.1194256
38. Zhang, C, Luo, J, Yu, B, Zheng, P, Huang, Z, Mao, X, et al. Dietary resveratrol supplementation improves meat quality of finishing pigs through changing muscle Fiber characteristics and Antioxidative status. Meat Sci. (2015) 102:15–21. doi: 10.1016/j.meatsci.2014.11.014
39. Meng, Q, Sun, S, Bai, Y, Luo, Z, Li, Z, Shi, B, et al. Effects of dietary resveratrol supplementation in sows on Antioxidative status, Myofiber characteristic and meat quality of offspring. Meat Sci. (2020) 167:108176. doi: 10.1016/j.meatsci.2020.108176
40. Sosnówka-Czajka, E, Skomorucha, I, Obremski, K, and Wojtacha, P. Performance and meat quality of broiler chickens fed with the addition of dried fruit pomace. Poult Sci. (2023) 102:102631. doi: 10.1016/j.psj.2023.102631
41. Sun, G, Li, F, Ma, X, Sun, J, Jiang, R, Tian, Y, et al. Gga-Mirna-18b-3p inhibits intramuscular adipocytes differentiation in chicken by targeting the Acot13 gene. Cells. (2019) 8:556. doi: 10.3390/cells8060556
42. Liu, S, Du, M, Tu, Y, You, W, Chen, W, Liu, G, et al. Fermented mixed feed alters growth performance, carcass traits, meat quality and muscle fatty acid and amino acid profiles in finishing pigs. Anim Nutr. (2023) 12:87–95. doi: 10.1016/j.aninu.2022.09.003
43. Liu, S, Tu, Y, Sun, J, Cai, P, Zhou, Y, Huang, Y, et al. Fermented mixed feed regulates intestinal microbial community and metabolism and alters pork flavor and umami. Meat Sci. (2023) 201:109177. doi: 10.1016/j.meatsci.2023.109177
44. Kouba, M, and Mourot, J. A review of nutritional effects on fat composition of animal products with special emphasis on N-3 polyunsaturated fatty acids. Biochimie. (2011) 93:13–7. doi: 10.1016/j.biochi.2010.02.027
45. Kilar, J, and Kasprzyk, A. Fatty acids and nutraceutical properties of lipids in fallow deer (Dama Dama) meat produced in organic and conventional farming systems. Food Secur. (2021) 10:2290. doi: 10.3390/foods10102290
46. Ahmed, ST, Islam, MM, Bostami, AR, Mun, H-S, Kim, Y-J, and Yang, C-J. Meat composition, fatty acid profile and oxidative stability of meat from broilers supplemented with pomegranate (Punica Granatum L.) by-products. Food Chem. (2015) 188:481–8. doi: 10.1016/j.foodchem.2015.04.140
47. Tayengwa, T, Chikwanha, OC, Neethling, J, Dugan, MER, Mutsvangwa, T, and Mapiye, C. Polyunsaturated fatty acid, volatile and sensory profiles of beef from steers fed Citrus pulp or grape pomace. Food Res Int. (2021) 139:109923. doi: 10.1016/j.foodres.2020.109923
48. Hawkey, E, and Nigg, JT. Omega− 3 fatty acid and Adhd: blood level analysis and Meta-analytic extension of supplementation trials. Clin Psychol Rev. (2014) 34:496–505. doi: 10.1016/j.cpr.2014.05.005
49. Assefa, AD, Saini, RK, and Keum, Y-S. Fatty acids, tocopherols, phenolic and antioxidant properties of six Citrus fruit species: a comparative study. J Food Meas Charact. (2017) 11:1665–75. doi: 10.1007/s11694-017-9546-x
50. Russo, C, Maugeri, A, Lombardo, GE, Musumeci, L, Barreca, D, Rapisarda, A, et al. The second life of Citrus fruit waste: a valuable source of bioactive compounds. Molecules. (2021) 26:5991. doi: 10.3390/molecules26195991
51. Carullo, G, Ramunno, A, Sommella, EM, De Luca, M, Belsito, EL, Frattaruolo, L, et al. Ultrasound-assisted extraction, chemical characterization, and impact on cell viability of food wastes derived from southern Italy autochthonous Citrus fruits. Antioxidants. (2022) 11:285. doi: 10.3390/antiox11020285
52. Salvador, AM, García-Maldonado, E, Gallego-Narbón, A, Zapatera, B, and Vaquero, MP. Fatty acid profile and Cardiometabolic markers in relation with diet type and Omega-3 supplementation in Spanish vegetarians. Nutrients. (2019) 11:1659. doi: 10.3390/nu11071659
53. Bešlo, D, Golubić, N, Rastija, V, Agić, D, Karnaš, M, Šubarić, D, et al. Antioxidant activity, metabolism, and bioavailability of polyphenols in the diet of animals. Antioxidants. (2023) 12:1141. doi: 10.3390/antiox12061141
54. Lee, JH, Jeon, YG, Lee, K-H, Lee, HW, Park, J, Jang, H, et al. Rnf20 suppresses tumorigenesis by inhibiting the Srebp1c-Pttg1 Axis in kidney Cancer. Mol Cell Biol. (2017) 37:e00265–17. doi: 10.1128/MCB.00265-17
55. Chen, J, Yang, XJ, Tong, H, et al. Expressions of Fas and Hsl Mrna in longissimus Dorsi muscle and their relation to intramuscular fat contents in pig. J Agric Biotechnol. (2004) 12, 422–426. Available at: https://kns.cnki.net/kcms2/article/abstract?v=NK8hpUzgeRW4L0AcZe3T3_9DW6ANMgdL-oXZrZF-bI-G2eXFbKybiN8oLFNmE4yejjf7nFXVm_SuW80c8M5Ex7rMCIa1Vj4Gj9nXmqxlEHUEwX3uvKREjv14kkVQyGsrjFWsXnPAg0add8i23zr-IYUEdRBMMlFd93yB2i96ihj2giZf6t1v3nqOHVsYZBAu&uniplatform=NZKPT&language=CHS
56. Chen, Z, and Shan, A, Effect of S-Nsps level in white diet on meat quality of broilers. The 5th National Conference on Feed and Nutrition; (2006).
57. Liu, K, Lin, L, Li, Q, Xue, Y, Zheng, F, Wang, G, et al. Scd1 controls De novo beige fat biogenesis through succinate-dependent regulation of mitochondrial complex ii. Proc Natl Acad Sci. (2020) 117:2462–72. doi: 10.1073/pnas.1914553117
58. Grabowska, M, Wawrzyniak, D, Rolle, K, Chomczyński, P, Oziewicz, S, Jurga, S, et al. Let food be your medicine: nutraceutical properties of lycopene. Food Funct. (2019) 10:3090–102. doi: 10.1039/C9FO00580C
59. Gungor, E, Altop, A, and Erener, G. Effect of raw and fermented grape pomace on the growth performance, antioxidant status, intestinal morphology, and selected bacterial species in broiler chicks. Animals. (2021) 11:364. doi: 10.3390/ani11020364
60. Gungor, E, Altop, A, Erener, G, and Coskun, I. Effect of raw and fermented pomegranate pomace on performance, antioxidant activity, intestinal microbiota and morphology in broiler chickens. Arch Anim Nutr. (2021) 75:137–52. doi: 10.1080/1745039X.2021.1894065
61. Wen, W, Chen, X, Huang, Z, Chen, D, Yu, B, He, J, et al. Dietary lycopene supplementation improves meat quality, antioxidant capacity and skeletal muscle Fiber type transformation in finishing pigs. Anim Nutr. (2022) 8:256–64. doi: 10.1016/j.aninu.2021.06.012
62. Huang, T, Che, Q, Chen, X, Chen, D, Yu, B, He, J, et al. Apple polyphenols improve intestinal antioxidant capacity and barrier function by activating the Nrf2/Keap1 signaling pathway in a pig model. J Agric Food Chem. (2022) 70:7576–85. doi: 10.1021/acs.jafc.2c02495
Keywords: fermented citrus pomace, yellow-feathered broilers, growth performance, meat quality, antioxidant capacity, fatty acid composition, lipid metabolism
Citation: Liu Y, Tang Y, Mei H, Liu Z, Li Z, Ma X, Luo Z, Huang W, Li Y and Yu M (2024) Feeding citrus pomace fermented with combined probiotics improves growth performance, meat quality, fatty acid profile, and antioxidant capacity in yellow-feathered broilers. Front. Vet. Sci. 11:1469947. doi: 10.3389/fvets.2024.1469947
Edited by:
Massimo Trabalza-Marinucci, University of Perugia, ItalyReviewed by:
Yang Zhi, Yangzhou University, ChinaPetru Alexandru Vlaicu, National Research Development Institute for Animal Biology and Nutrition, Romania
Copyright © 2024 Liu, Tang, Mei, Liu, Li, Ma, Luo, Huang, Li and Yu. This is an open-access article distributed under the terms of the Creative Commons Attribution License (CC BY). The use, distribution or reproduction in other forums is permitted, provided the original author(s) and the copyright owner(s) are credited and that the original publication in this journal is cited, in accordance with accepted academic practice. No use, distribution or reproduction is permitted which does not comply with these terms.
*Correspondence: Yuanfei Li, bGkteXVhbi1mZWlAb3V0bG9vay5jb20=; Miao Yu, eXVtaWFvQGdkYWFzLmNu
†These authors have contributed equally to this work