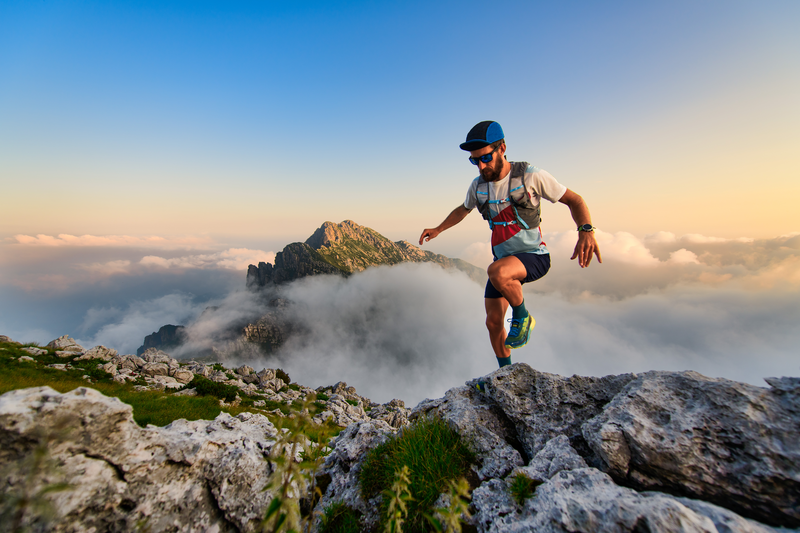
95% of researchers rate our articles as excellent or good
Learn more about the work of our research integrity team to safeguard the quality of each article we publish.
Find out more
ORIGINAL RESEARCH article
Front. Vet. Sci. , 22 October 2024
Sec. One Health
Volume 11 - 2024 | https://doi.org/10.3389/fvets.2024.1464351
This article is part of the Research Topic Mitigating Pathogen Load and AMR in Animals via Prebiotics and Probiotics View all 5 articles
A correction has been applied to this article in:
Corrigendum: Investigating Antimicrobial Resistance Genes in Probiotic Products for Companion Animals
Introduction: One of the greatest challenges of our time is antimicrobial resistance, which could become the leading cause of death globally within a few decades. In the context of One Health, it is in the common interest to mitigate the global spread of antimicrobial resistance by seeking alternative solutions, alongside appropriate drug selection and responsible use. Probiotics offer a potential avenue to reduce antibiotic usage; however, there is a scarcity of research that examines commercial products in terms of carrying antimicrobial resistance genes (ARGs) involved in resistance development through microbial vectors.
Methods: Our study investigated 10 commercially available probiotic products for cats and dogs. Initially, we conducted phenotypic testing through determination of minimum inhibitory concentration (MIC) for antibiotics important in animal and public health. Subsequently, we performed next-generation sequencing (NGS) of the products to elucidate the genetic background behind the decrease in phenotypic sensitivity.
Results: In total, 19 types of ARGs were identified, with 57.9% being found on plasmids, and in two cases, carriage as mobile genetic elements were found. One of the genes identified was the APH(3′)-Ia gene, capable of inactivating aminoglycoside antibiotics through phosphotransferase enzyme production regulation, while the other was the tetS gene, capable of conferring reduced sensitivity to tetracycline antibiotics through target protection.
Discussion: Our findings underscore the importance of approaching antimicrobial resistance investigations from a broader perspective. We suggest that further studies in this area are justified and raise questions regarding the need to extend legally required studies on probiotic products from their use in economic livestock to their use in companion animals.
Antimicrobial resistance is the property of bacteria enabling them to survive targeted treatment with antibiotics (1). Nowadays, infections caused by resistant and multi-resistant strains are spreading increasingly (2) which, according to the World Health Organization (WHO), will become one of the 10 leading threats to global health in the twenty-first century (3).
Probiotics are living microorganisms that, upon entering the body, exert beneficial effects on the entire host organism. The majority belong to bacterial strains which occur naturally in the gut. Species belonging to the Enterococcus, Lactobacillus, and Bifidobacterium genera are commonly used for this purpose, which is the maintenance or restoration of gut microbiome balance (4). Although numerous studies regarding their effects on the human body have been conducted, significantly fewer investigations have been undertaken on their effects on companion animals. Besides the intestinal effects, it is likely that probiotics exert immunomodulatory and stress-reducing effects in companion animals and assist in balancing digestion-related blood parameters (5). Certain probiotic strains also exhibit antimicrobial effects against specific pathogenic gastrointestinal bacteria (6). Existing research suggests that the effects of probiotics are most favorable for bacteria species specific to the host, especially in gastrointestinal diseases (7). For probiotic organisms, it may be desirable to have antibacterial resistance to survive in antibiotic treatment. However, bacteria can transfer resistance genes to each other vertically or horizontally, as a result they may be able to transfer resistant genes to pathogenic bacteria. The mammalian gastrointestinal tract provides favorable conditions for gene transfer. In the case of products for livestock, regulations prohibit the inclusion of resistance genes relevant from a public health perspective, but such regulation is lacking for companion animals (8).
During the examination of the Bacillus amyloliquefaciens strain used as a probiotic, it was found that although it was sensitive to fluoroquinolones, it exhibited reduced sensitivity to older antibacterial groups (9). However, another study did not detect resistance genes in this species (10). Intrinsic resistance is characteristic of Lactobacillus species, which generally show resistance to multiple antibiotic groups, such as aminoglycosides, glycopeptides, nucleic acid synthesis inhibitors, and folate synthesis inhibitors (11). Resistance genes against penicillin have been detected in various isolates in several studies (12–14). Oxacillin and cephalosporin resistance have also been detected in Lactobacillus plantarum and Lactobacillus rhamnosus (15, 16). Mater et al. demonstrated that Lactobacillus acidophilus strains are capable of acquiring vancomycin resistance genes from Enterococcus species via horizontal gene transfer (17). The most common resistance genes in Lactobacillus species confer resistance to tetracyclines and macrolides. The tetM and tetS genes can be found on both plasmids and chromosomes. However, the tetL gene is only described on plasmids. The ermB gene responsible for resistance to erythromycin is also found on plasmids (18). It has also been shown that strains with more than one tetracycline resistance gene exhibit greater resistance, as these genes have a synergistic effect (19). Feld et al. isolated a Lactobacillus plantarum strain with a tetM gene transposon capable of transferring it to other lactic acid-producing bacteria (20).
In a subsequent study, examining Pediococcus acidilactici strains against 21 different antimicrobial agents (penicillin, oxacillin, ampicillin, piperacillin, imipenem, vancomycin, streptomycin, gentamicin, amikacin, kanamycin, tetracycline, chloramphenicol, minocycline, doxycycline, cotrimoxazole, azithromycin, erythromycin, clindamycin, norfloxacin, ciprofloxacin, and levofloxacin), it was found that only four strains exhibited sensitivity to piperacillin, imipenem, chloramphenicol, and erythromycin. While they showed only moderate resistance to clindamycin, doxycycline, and levofloxacin, they were fully resistant to the remaining agents (21). In another investigation, a Pediococcus pentosaceus strain tested against 19 agents was only resistant to ceftazidime and sulfamethoxazole. In contrast, an Enterococcus faecalis strain was almost entirely resistant to all agents. Based on this, Pediococcus species appear to be safer in terms of resistance as probiotics compared to Enterococcus species, meaning they are less likely to contribute to the spread of antimicrobial resistance (22). However, Enterococcus faecium is the most common component of probiotic preparations for companion animals. A probiotic strain examined by Bs et al. was found to be resistant to penicillin, ampicillin, erythromycin, kanamycin, and streptomycin. However, it was sensitive to tetracycline, chloramphenicol, and rifampicin (23).
Therefore, it is particularly important to adequately regulate probiotic products marketed for companion animals in terms of antimicrobial resistance gene carriage. In our study, we investigate the most common probiotic products for companion animals available in Hungary through next-generation sequencing and compare the results with the phenotypic resistance profiles of strains isolated from these products.
We purchased 10 probiotic products available for dogs and cats from retailers selling veterinary products in Hungary. The isolation of strains indicated on the products was carried out by the Department of Microbiology and Infectious Diseases at the University of Veterinary Medicine Budapest. The isolation of Enterococcus faecium strains was successful in all nine of the products listing it (9/9). Among the other strains listed on the products, we were able to isolate Lactobacillus plantarum in one case (1/4), Pediococcus pentasaceus in two cases (2/2), and Pediococcus acidilactici in one case (1/2). Additionally, certain products indicated the presence of Pediococcus strains (one strain) and Lactococcus strains (eight strains) in deactivated form. The species identity of the strains was determined using MALDI-TOF mass spectrometry (Bruker, Mannheim, Germany). The isolates were used for minimal inhibitory concentration (MIC) testing. The properties of each product are summarized in Table 1.
Table 1. The examined products, the strains contained within them along with their strain numbers, and the colony-forming units (CFU) present in the products.
The classification of strains into categories is overseen by three main international organizations. Based on their standards, probiotic strains found in products are provided with unique identifiers. The CECT (Spanish Type Culture Collection) is a Spanish strain collection accredited with ISO 9001 standards (24). The NCIMB (National Collection of Industrial, Food and Marine Bacteria) is a privately-owned strain collection located in the United Kingdom (25). The DSM (Deutsche Sammlung von Mikroorganismen und Zellkulturen GmbH) is a German strain collection (26).
Strain solutions were prepared from the active ingredients in accordance with the recommendations of the Clinical Laboratory Standards Institute (CLSI), utilizing materials from Merck KGaA, Darmstadt, Germany (27). For the experiments, strain solutions were prepared at a concentration of 1,024 μg/mL, with adjustments made for the purity specified by the manufacturer of each active ingredient. The experiments were conducted within a 2-fold dilution range, spanning from 128 to 0.125 μg/mL.
The phenotypic expression of resistance was examined by determining the minimum inhibitory concentration (MIC) values of each bacterial strain, which was conducted using the CLSI methodology (27). The breakpoints were determined based on the guidelines of both the CLSI and the European Committee on Antimicrobial Susceptibility Testing (EUCAST) (28). The selection of antimicrobial agents was made based on international literature, aiming to cover the most frequently used antimicrobial groups.
The bacterial strains were stored at −80°C and inoculated into 3 ml of Mueller-Hinton broth (MHB) the day before the experiment, followed by incubation at 37°C for 18–24 h. The experiments were conducted using a 96-well microtiter plate (VWR International, LLC., Debrecen, Hungary). The first column of the working plates, filled with 90 μl of MHB, received a 4-fold dilution of the strain solutions, establishing an initial concentration of 128 μg/mL, from which a 2-fold dilution series was prepared, except in the last two columns of 96-well microtiter plate. The penultimate column served as a positive control (containing only bacteria), while the final column served as a negative control (containing only the drug). Bacterial suspension adjusted to 0.5 McFarland turbidity was inoculated onto the working plates up to the positive control column using a nephelometer (CheBio fejleszto Kft., Budapest, Hungary) (27). Evaluation took place after 24 h of incubation at 37°C using the SWIN automatic MIC reader (CheBio fejleszto Kft., Budapest, Hungary) and the VIZION system (CheBio fejleszto Kft., Budapest, Hungary). The reference isolate used was Escherichia coli (ATCC 25922).
Probiotic samples were prepared by using 0.1 g of probiotic powder dissolved in 1 ml PBS (1:10 dilution). Nucleic acid was extracted from the mixture using the Quick-DNA Fecal/Soil Microbe Miniprep Kit (Zymo Research, CA, USA) according to the manufacturer's instruction. Samples were disrupted by using a TissueLyzer LT Bead Mill (Qiagen, Germany). The concentration of purified DNA was measured with Qubit 2.0 equipment using the Qubit dsDNA BR Assay Kit (Thermo Scientific, Waltham, MA, USA).
Nucleotide sequences were determined by next-generation sequencing on an Illumina® NextSeq 500 sequencer (Illumina, San Diego, CA, USA) following the reference guide provided by Illumina. Illumina® Nextera XT DNA Library Preparation Kit (Illumina, San Diego, CA, USA) and Nextera XT Index Kit v2 Set A (Illumina, San Diego, CA, USA) were used to prepare Illumina specific libraries. DNA samples were diluted to 0.2 ng/μL in nuclease-free water (Promega, Madison, WI, USA) in a final volume of 2.5 μL. Reaction components were used at a reduced volume. For the tagmentation reaction, 5 μL Tagment DNA buffer with 2.5 μL AmpliconTagment Mix were used. During tagmentation, the samples were incubated at 55°C for 6 min, using the GeneAmp PCR System 9700 (Applied Biosystems/Thermo Fisher Scientific, Foster City, CA, USA). The samples were then allowed to cool to 10°C before the addition of 2.5 μL of the Neutralize Tagment buffer. Neutralization was performed for 5 min at room temperature. A total of 7.5 μL of the Nextera PCR Master Mix and 2.5 μL each of the i5 and i7 index primers were added to the tagmented DNA samples. The index primers were attached to library DNA via 12 PCR cycles (each cycle consisted of the following steps: 95°C for 10 s, 55°C for 30 s, followed by 72°C for 30 s). Following the PCR cycles, the samples were held at 72°C for 5 min and then at 10°C. Libraries were purified using Gel/PCR DNA Fragments Extraction Kit (Geneaid Biotech Ltd., Taipei, Taiwan). The concentration of the purified libraries was measured with Qubit 2.0 equipment using Qubit dsDNA HS Assay Kit (Thermo Fischer Scientific, Waltham, MA, USA). Library DNAs were pooled and denatured. The denatured library pool was loaded onto a NextSeq 500/550 Mid Output flowcell at a final concentration of 1.5 pM. Sequencing was performed using an Illumina® NextSeq 500 sequencer (Illumina, San Diego, CA, USA).
During the bioinformatic data processing, quality control of the raw sequences was performed using FastQC v0.11.9 (29), followed by the removal of low-quality sections using TrimGalore v0.6.6 (30). The reads were assembled into longer sequences (contigs) using MEGAHIT v1.2.9 (31). From these contigs, all possible open reading frames (ORFs) were determined using Prodigal v2.6.3 (32). Protein sequences were derived from these ORFs based on their nucleotide sequences. Subsequently, the protein sequences were compared to antimicrobial resistance gene (ARG) sequences in The Comprehensive Antibiotic Resistance Database (CARD) using the Resistance Gene Identifier (RGI) v5.1.0 software (33). Only results reaching the specified threshold (95%) in the CARD database were retained.
The potential mobility of identified resistance genes was assessed using the MobileElementFinder v1.0.3 program (34) which predicted genes occurring as mobile genetic elements (MGEs) on contigs. Only those ARGs found within the longest composite transposon distance specific to the species in the database within the contig were considered as potentially mobile. Additionally, plasmid encoding was examined using the PlasFlow v1.1 software, and the presence of phage genomes on contigs was determined using the VirSorter v2.2.2 (35) software.
Table 2 summarizes the results of susceptibility testing of Enterococcus faecium strains isolated from the examined products. All the products contained the same strain (NCIMB10415) except for the J-product (DSM7134). Similar MIC values were observed for all strains. All the tested strains were sensitive to penicillin, amoxicillin, amoxicillin-clavulanate, oxytetracycline, doxycycline, clindamycin, tylosin, and vancomycin. For gentamicin (100%; MIC >32 μg/mL) and potentiated sulfonamides (sulfamethoxazole and trimethoprim in a 19:1 ratio; 100%; MIC 16 μg/mL), all strains were resistant. Regarding gatifloxacin, a fourth-generation fluoroquinolone, six strains were susceptible, while three were resistant (66.7%; MIC >2 μg/mL).
Table 2. Minimum inhibitory concentration (MIC) values of Enterococcus faecium strains isolated from the products for tested antibiotics.
Table 3 summarizes the antibiotic susceptibility profiles of Lactobacillus and Pediococcus species. Among the Lactobacillus strains, one Lactobacillus plantarum strain was isolated, which showed sensitivity to penicillin, amoxicillin-clavulanate, gentamicin, oxytetracycline, doxycycline, clindamycin, tylosin, and vancomycin. It was resistant to amoxicillin (>1 μg/mL MIC), the combination of sulphonamides (>16 μg/mL MIC), and gatifloxacin (>2 μg/mL MIC). From the Pediococcus species, two Pediococcus pentasaceus and one Pediococcus acidilactici strains were isolated, all of which were susceptible only to clindamycin (< 8 μg/mL MIC), showing resistance to all other tested antibiotics.
Table 3. Minimum inhibitory concentration (MIC) values of Lactobacillus and Pediococcus strains isolated from the products for tested antibiotics.
Table 4 summarizes the results obtained from next-generation sequencing, including the isolated resistance genes from the products, their taxonomic origin, indicating the ARG family for each gene, the resistance mechanism determined by the respective gene, and the antibiotic groups against which resistance is conferred.
Table 4. The antimicrobial resistance gene (ARG) set of each product, their mechanism of action, resistance to the antibiotics group.
A total of 19 types of ARGs were identified, out of which 11 were found on plasmids, and no genes were identified on phages. Of particular concern is that in two cases, a plasmid-contained gene also acted as a mobile genetic element (MGE). One of these was the APH(3′)-Ia gene, found in the I-product. The activation of this resistance gene through enzymatic means (phosphotransferase) can inactivate aminoglycoside antibiotics (36). The other gene was the tetS gene, found in the A-product. This gene is capable of reducing sensitivity to tetracycline antibiotics through target protection (ribosomal mosaic), thereby preventing the binding of the antibiotic (37).
During the determination of MIC values, a correlation can be observed between resistant values and the identified ARGs in the Enterococcus faecium strains of various products. The expression of the AAC(6′)-Ii gene enzymatically (acetyl-transferase) leads to resistance to aminoglycoside antibiotics. This gene was found in all tested products and could be one of the genes responsible for resistance (≥32 μg/mL) observed in all strains. It's typically chromosomally located, but in one case (F-product), it was found on a plasmid. The eatAv gene confers resistance to lincosamides and pleuromutilins through target protection (ABC-F type). This gene was present in all Enterococcus faecium strains from the tested products. The efmA gene determines an MFS-type efflux pump for macrolides and fluoroquinolones, potentially contributing to resistance to gatifloxacin (2 μg/mL MIC) observed in one case (J-product). The rsmA gene determines an RND-type multidrug efflux pump, which can confer resistance to phenicols, fluoroquinolones, and diaminopyrimidines. This gene was also found in all Enterococcus faecium strains and may explain the observed resistance (>128 μg/mL MIC) to potentiated sulfonamides, gatifloxacin (2 μg/mL MIC) in the H-product, I-product, and J-product cases, as well as potential florfenicol resistance (8 μg/mL MIC).
All phenotypic resistances were successfully attributed to identified ARGs. Of particular concern is the high proportion of genes found on plasmids (57.9%), with two instances where these were also MGEs. Therefore, based on our findings, it would be necessary to conduct such examinations, similar to those required for food-producing animals, before introducing probiotic preparations intended for companion animals into circulation. In product A, containing strains of Lactobacillus plantarum (NCIMB41638), Lactobacillus fermentum (NCIMB41636), and Lactobacillus rhamnosus (NCIMB41640), no ARGs were found. Therefore, the phenotypic resistance observed in this case cannot be genetically supported by detected genes.
The B-product contained Enterococcus faecium (NCIMB10415), from which we identified 15 ARGs, out of which seven were located on plasmids and one (tetS) gene was on an MGE. Four genes were specifically derived from Enterococcus species, while the rest originated from Lactococcus, Klebsiella, Pseudomonas, Hafnia, Acinetobacter, and Streptococcus species. Similarly, in product C and product H containing Enterococcus faecium (NCIMB10415), we identified 3 ARGs, all of which were chromosomal genes originating from Enterococcus spp. The D-product, E-product, and J-product containing Lactobacillus plantarum (DSM12837), Pediococcus acidilactici (DSM16243), and Enterococcus faecium (NCIMB10415) species, respectively, all showed the presence of the same four ARGs. These genes were chromosomally located, and all originated from Enterococcus spp.
The F-product contained strains of Pediococcus pentasaceus (DSM1283U), Lactobacillus brevis (DSM12835), Lactobacillus bucherii (DSM12856), Lactobacillus plantarum (DSM12836), Lactobacillus rhamnosus (NCIMB30121), Lactobacillus acidophilus (CECTU529), and Enterococcus faecium (NCIMB10415), with Pediococcus and Lactobacillus species present in deactivated form in the product. Four resistance genes matched those found in the E-product. However, in the case of the F-product, the AAC(6′)-Ii gene was located on a plasmid, while the rest were chromosomal. All identified genes were attributed to Enterococcus spp. The composition of the G-product was identical to that of the F-product, but only two chromosomally located ARGs originating from Enterococcus spp. could be identified in the tablets.
The I-product, which contained the Enterococcus faecium (NCIMB10415) strain, contained the most identified ARGs, totaling 14. Of these, eight were found on plasmids, and among these, the APH(3′)-Ia gene was also an MGE. Five of the genes were originally of Enterococcus spp. origin, while the rest may have been acquired from other species through horizontal gene transfer, including Staphylococcus, Klebsiella, Lactobacillales, Enterobacteriaceae, Enterobacterales, and Streptococcus species.
The in vitro antibiotic susceptibility of probiotic strains isolated from a total of 10 commercially available probiotic products for companion animals (dogs, cats) was investigated, and 19 different ARGs were identified during the exploration of the products through next-generation sequencing.
Gentamicin resistance was observed in all strains of Enterococcus faecium examined in our study, with MIC values >32 μg/mL. In contrast, in the study conducted by Takeuchi et al., this value exceeded 500 μg/mL in 22% of the isolated Enterococcus faecium strains (38). Extremely high values (9,000 μg/mL MIC) were detected in a strain isolated from wastewater by Xu et al. during antimicrobial susceptibility testing against sulphonamide (sulfamethoxazole-trimethoprim) (39). Significantly lower values (16 μg/mL MIC) were identified in our research. Similarly, comparable results for both gentamicin and sulphonamide susceptibility were obtained in isolates from poultry (32 μg/mL MIC, 16 μg/mL MIC) by Maasjost et al (40). Resistance to gatifloxacin was also observed in three strains, albeit at relatively low levels (>2 μg/mL MIC). This finding is supported by several previous studies. Wenzler et al. worked with clinical isolates, where the median value (2 μg/mL MIC) matched those described in our study (41).
Nine of the preparations we examined contained Enterococcus faecium, in which all 19 types of ARG were present. Xia et al. identified 18 ARGs in Enterococcus faecium strains, none of which were MGE (42), in contrast, we described the likelihood of mobility for two genes [APH(3′)-Ia, tetS]. Several of the genes we identified confer resistance to aminoglycosides through different enzymatic pathways. The AAC(6′)-Ii gene is an aminoglycoside transferase found on the chromosome, first described in Enterococcus faecium (43). The AAD(6) gene, found on a plasmid, encodes aminoglycoside nucleotide transferase (44). The APH(3′) gene family members confer aminoglycoside resistance through enzymatic inactivation. The APH(3′)-Ia gene is found on a transposon in Escherichia coli and Salmonella species (36). The APH(3′)-IIIa gene is plasmid-encoded, found in Staphylococcus aureus and Enterococcus species (45). The APH(3′)-Ib gene was identified in Escherichia coli, also found on a plasmid (46). The APH(6)-Id operates through a similar mechanism. This gene can be found on a plasmid, transposon, or chromosome (47).
The catA8 gene encodes a chloramphenicol acetyltransferase enzyme. Through enzymatic inactivation, it neutralizes phenicols, primarily chloramphenicol (48). Metagenomic analysis of the catA8 gene identified by us supported its origin in Lactobacillus and its presence on a plasmid. The eatAv gene was first identified in Enterococcus faecium bacteria. Its mechanism involves target protection, conferring resistance to pleuromutilins and lincosamides (49). The expression of ermB is induced by the erythromycin agent. As a result, resistance is developed against macrolides and lincosamides through modification of the target (50).
The msrC gene, typically found in Enterococcus faecium strains, is a chromosomally encoded gene that confers resistance primarily to macrolides, specifically erythromycin. Through target protection, it prevents the action of antibiotics (51). We observed phenotypic expression of this gene in most cases based on MIC values, and in one preparation, we identified it on a plasmid. Urshev et al. detected the msrC gene in an Enterococcus faecium strain (52). Thumu et al. first identified the msrC gene in Pediococcus pentasaceus species, but they also detected the presence of the ermB gene (53). In our investigations, we identified both genes in several preparations, but based on metagenomic analysis, we found that these genes were of Enterococcus spp. origin. However, phenotypically, we observed the expression of resistance as defined by them. The rsmA gene encodes a small RNA-binding protein that plays a post-transcriptional regulatory role in shaping the virulence genes of Pseudomonas aeruginosa. It confers resistance to diaminopyrimidines, phenicols, and fluoroquinolones through an efflux pump mechanism (54). The SAT-4 gene, derived from Campylobacter coli bacteria, is a plasmid-mediated streptothricin acetyltransferase (55).
The tetC gene is responsible for conferring resistance to tetracycline antibiotics, primarily in Gram-negative bacteria, as it regulates the expression of an efflux pump gene, usually found on plasmids. The tetD gene is similar but is exclusively present in Gram-negative species. Resistance is developed through target protection mechanisms (56). The tetM and tetS genes are responsible for ribosome protection proteins and are located on mobile genetic elements, found in both Gram-negative and Gram-positive bacteria. Similar to Pan et al. (57) we also identified the tetM gene on a plasmid, but during this study, it was identified as originating from Enterococcus. The tetS gene we detected proved to be of Streptococcus spp. origin, found on a plasmid, and was mobile in the case of B-product. Nawaz et al. identified these genes during their study of Lactobacillus plantarum, successfully transferring the genes into Enterococcus isolates under experimental conditions (13). In our investigations, the ermB gene identified in preparations containing Lactobacillus plantarum always proved to be of Enterococcus spp. origin.
Based on the results, it is recommended in practice to consider introducing stricter regulations during the production and marketing authorization of probiotics, ensuring the reduction of the ARG pool in products to a minimal level, with particular attention to the exclusion of genes that may have significant public health importance. Preliminary resistance profile assessments during the selection of probiotic strains reduce the likelihood of spreading resistances that can be easily transferred, creating a bridge between animal and public health, and posing a significant risk through their use. In every case of probiotics, it would be necessary to compile a panel of antibiotic active substances based on phenotypic test results, upon which genetic background exploration would be conducted using next-generation sequencing, with particular attention to the carriage of ARGs on plasmids or phages, as well as their encoding as MGEs. The exclusion of these is essential for minimizing the chances of horizontal gene transfer. The limitations of our studies include that they were conducted with only a few products involved. In the future, it is definitely worthwhile to involve a broader range of products in the studies and to conduct more parallel investigations to enable statistical analyses.
We can conclude that among the probiotic strains we studied, Enterococcus faecium bacteria carry the most resistance genes, in accordance with the existing literature. Among Lactobacillus and Pediococcus species, we identified resistance genes of Enterococcus strains in most cases, also consistent with literature. We detected a Lactobacillus plantarum-derived gene (efmA) responsible for resistance, which has not been previously described. The majority of identified ARGs (57.9%) were found on plasmids; in two preparations, B-product (tetS gene) and I-product [APH(3′)-Ia gene], we identified plasmid-borne ARGs, also serving as MGE genes. The literature on this topic is relatively scarce, particularly regarding studies exploring the ARG repertoire in probiotic preparations intended for companion animals. Our results underscore the importance of conducting such studies, and it may be worth considering legally mandating such investigations as a condition for distribution, similar to legislation implemented for products intended for livestock.
The datasets presented in this study can be found in online repositories. The names of the repository/repositories and accession number(s) can be found in the article/Supplementary material.
AK: Conceptualization, Methodology, Project administration, Supervision, Visualization, Writing – original draft. ES: Data curation, Investigation, Writing – original draft. ÁS: Resources, Writing – original draft. MP: Formal analysis, Software, Writing – review & editing. KBán: Validation, Writing – review & editing. GK: Validation, Writing – review & editing. EK: Methodology, Writing – review & editing. KBal: Methodology, Writing – review & editing. ÁJ: Conceptualization, Funding acquisition, Validation, Writing – review & editing.
The author(s) declare financial support was received for the research, authorship, and/or publication of this article. This research was supported by Project No. RRF-2.3.1-21-2022-00001 was implemented with the support provided by the Recovery and Resilience Facility (RRF), financed under the National Recovery Fund budget estimate, RRF-2.3.1-21 funding scheme. Prepared with the professional support of the Doctoral Student Scholarship Program of the Co-operative Doctoral Program of the Ministry of Innovation and Technology financed from the National Research, Development and Innovation Fund.
We are grateful to the staff of the Department of Microbiology and Infectious Diseases for their assistance in isolating the strains from the preparations. Additionally, we extend our thanks to the assistants at the Microbiology Laboratory of the Department of Pharmacology and Toxicology for their support with preparatory work in our investigations.
The authors declare that the research was conducted in the absence of any commercial or financial relationships that could be construed as a potential conflict of interest.
All claims expressed in this article are solely those of the authors and do not necessarily represent those of their affiliated organizations, or those of the publisher, the editors and the reviewers. Any product that may be evaluated in this article, or claim that may be made by its manufacturer, is not guaranteed or endorsed by the publisher.
The Supplementary Material for this article can be found online at: https://www.frontiersin.org/articles/10.3389/fvets.2024.1464351/full#supplementary-material
1. Alós J-I. Resistencia bacteriana a los antibióticos: una crisis global. Enfermedades Infecciosas y Microbiología Clínica. (2015) 33:692–9. doi: 10.1016/j.eimc.2014.10.004
2. Munita JM, Arias CA. Mechanisms of antibiotic resistance. Microbiol Spectr. (2016) 4:15. doi: 10.1128/microbiolspec.VMBF-0016-2015
3. WHO. Global Antimicrobial Resistance Forum Launched to Help Tackle Common Threat to Planetary Health. Available at: https://www.who.int/news-room/articles-detail/global-antimicrobial-resistance-forum-launched-to-help-tackle-common-threat-to-planetary-health (accessed May 14, 2023).
4. Wilkins T, Sequoia J. Probiotics for gastrointestinal conditions: a summary of the evidence. Am Fam Physician. (2017) 96:170–8.
5. Grześkowiak Ł, Endo A, Beasley S, Salminen S. Microbiota and probiotics in canine and feline welfare. Anaerobe. (2015) 34:14–23. doi: 10.1016/j.anaerobe.2015.04.002
6. He Y, Li F, Zhang W, An M, Li A, Wang Y, et al. Probiotic potential of Bacillus amyloliquefaciens isolated from Tibetan Yaks. Probiot Antimicr Prot. (2022) 22:5. doi: 10.1007/s12602-022-10027-5
7. Kumar S, Pattanaik AK, Sharma S, Jadhav SE, Dutta N, Kumar A. Probiotic potential of a Lactobacillus bacterium of canine faecal-origin and its impact on select gut health indices and immune response of dogs. Probiotics Antimicrob Proteins. (2017) 9:262–77. doi: 10.1007/s12602-017-9256-z
8. Osmanagaoglu O, Kiran F, Ataoglu H. Evaluation of in vitro probiotic potential of Pediococcus pentosaceus OZF isolated from human breast milk. Probiot Antimicr Prot. (2010) 2:162–74. doi: 10.1007/s12602-010-9050-7
9. Du H, Yao W, Kulyar MF-A, Ding Y, Zhu H, Pan H, et al. Effects of Bacillus amyloliquefaciens TL106 isolated from Tibetan pigs on probiotic potential and intestinal microbes in weaned piglets. Microbiol Spectr. (2021) 10:e01205–21. doi: 10.1128/spectrum.01205-21
10. Islam MI, Seo H, Redwan A, Kim S, Lee S, Siddiquee M, et al. In vitro and in vivo anti-Clostridioides difficile effect of a probiotic Bacillus amyloliquefaciens strain. J Microbiol Biotechnol. (2022) 32:46–55. doi: 10.4014/jmb.2107.07057
11. Charteris WP, Kelly PM, Morelli L, Collins JK. Development and application of an in vitro methodology to determine the transit tolerance of potentially probiotic Lactobacillus and Bifidobacterium species in the upper human gastrointestinal tract. J Appl Microbiol. (1998) 84:759–68. doi: 10.1046/j.1365-2672.1998.00407.x
12. Charteris WP, Kelly PM, Morelli L, Collins JK. Gradient diffusion antibiotic susceptibility testing of potentially probiotic lactobacilli. J Food Prot. (2001) 64:2007–14. doi: 10.4315/0362-028X-64.12.2007
13. Nawaz M, Wang J, Zhou A, Ma C, Wu X, Moore JE, et al. Characterization and transfer of antibiotic resistance in lactic acid bacteria from fermented food products. Curr Microbiol. (2011) 62:1081–9. doi: 10.1007/s00284-010-9856-2
14. Belletti N, Gatti M, Bottari B, Neviani E, Tabanelli G, Gardini F. Antibiotic resistance of lactobacilli isolated from two Italian hard cheeses. J Food Prot. (2009) 72:2162–9. doi: 10.4315/0362-028X-72.10.2162
15. Coppola R, Succi M, Tremonte P, Reale A, Salzano G, Sorrentino E. Antibiotic susceptibility of Lactobacillus rhamnosus strains isolated from Parmigiano Reggiano cheese. Lait. (2005) 85:193–204. doi: 10.1051/lait:2005007
16. Zdolec N, Filipović I, Fleck ZC, Marić A, Jankuloski D, Kozačinski L, et al. Antimicrobial susceptibility of lactic acid bacteria isolated from fermented sausages and raw cheese. Vet Arh. (2011) 81:133–41.
17. Mater DDG, Langella P, Corthier G, Flores M-J. A probiotic Lactobacillus strain can acquire vancomycin resistance during digestive transit in mice. J Mol Microbiol Biotechnol. (2007) 14:123–7. doi: 10.1159/000106091
18. Çataloluk O, Gogebakan B. Presence of drug resistance in intestinal lactobacilli of dairy and human origin in Turkey. FEMS Microbiol Lett. (2004) 236:7–12. doi: 10.1111/j.1574-6968.2004.tb09620.x
19. Ammor MS, Flórez AB, van Hoek AHAM, de los Reyes-Gavilán CG, Aarts HJM, Margolles A, et al. Molecular characterization of intrinsic and acquired antibiotic resistance in lactic acid bacteria and bifidobacteria. J Mol Microbiol Biotechnol. (2007) 14:6–15. doi: 10.1159/000106077
20. Feld L, Bielak E, Hammer K, Wilcks A. Characterization of a small erythromycin resistance plasmid pLFE1 from the food-isolate Lactobacillus plantarum M345. Plasmid. (2009) 61:159–70. doi: 10.1016/j.plasmid.2009.01.002
21. Zhao M, Liu K, Zhang Y, Li Y, Zhou N, Li G. Probiotic characteristics and whole-genome sequence analysis of Pediococcus acidilactici isolated from the feces of adult beagles. Front Microbiol. (2023) 14:1179953. doi: 10.3389/fmicb.2023.1179953
22. Yang Q, Lü Y, Zhang M, Gong Y, Li Z, Tran NT, et al. Lactic acid bacteria, Enterococcus faecalis Y17 and Pediococcus pentosaceus G11, improved growth performance, and immunity of mud crab (Scylla paramamosain). Fish Shellfish Immunol. (2019) 93:135–43. doi: 10.1016/j.fsi.2019.07.050
23. Bs S, Thankappan B, Mahendran R, Muthusamy G, Femil selta DR, Angayarkanni J. Evaluation of GABA production and probiotic activities of Enterococcus faecium BS5. Probiot Antimicr Prot. (2021) 13:993–1004. doi: 10.1007/s12602-021-09759-7
24. UV. Spanish Type Culture Collection (2023). Available at: https://www.uv.es/uvweb/spanish-type-culture-collection/en/spanish-type-culture-collection-1285872233521.html (accessed August 1, 2023).
25. NCIMB. Microbiology. Analytical Services. Biomaterial Storage (2023). Available at: https://www.ncimb.com/ (accessed August 1, 2023).
26. German Collection of Microorganisms and Cell Cultures GmbH. Catalogue (2023). Available at: https://www.dsmz.de/collection/catalogue/microorganisms/catalogue (accessed August 1, 2023).
27. Clinical and Laboratory Standards Institute. Methods for Dilution Antimicrobial Susceptibility Tests for Bacteria That Grow Aerobically. 11th ed. Wayne, PA: Clinical and Laboratory Standards Institute (2018).
28. EUCAST. Breakpoint Tables for Interpretation of MICs and Zone Diameters. The European Committee on Antimicrobial Susceptibility Testing (2023).
29. Andrews S. FastQC A Quality Control Tool for High Throughput Sequence Data (2012). Available at: https://www.bioinformatics.babraham.ac.uk/projects/fastqc/ (accessed April 25, 2022).
30. Krueger F, James F, Ewels P, Afyounian E, Schuster-Boeckler B. FelixKrueger/TrimGalore: v0.6.7—DOI via Zenodo. GitHub (2021).
31. Dinghua. MEGAHIT: an ultra-fast single-node solution for large and complex metagenomics assembly via succinct de Bruijn graph. Bioinformatics. (2022) 2022:btv033. doi: 10.1093/bioinformatics/btv033
32. Hyatt D, Chen G-L, Locascio PF, Land ML, Larimer FW, Hauser LJ. Prodigal: prokaryotic gene recognition and translation initiation site identification. BMC Bioinformat. (2010) 11:119. doi: 10.1186/1471-2105-11-119
33. Alcock BP, Raphenya AR, Lau TTY, Tsang KK, Bouchard M, Edalatmand A, et al. CARD 2020: antibiotic resistome surveillance with the comprehensive antibiotic resistance database. Nucleic Acids Res. (2020) 48:D517–25. doi: 10.1093/nar/gkz935
34. Johansson MHK, Bortolaia V, Tansirichaiya S, Aarestrup FM, Roberts AP, Petersen TN. Detection of mobile genetic elements associated with antibiotic resistance in Salmonella enterica using a newly developed web tool: MobileElementFinder. J Antimicrob Chemother. (2021) 76:101–9. doi: 10.1093/jac/dkaa390
35. Roux S, Enault F, Hurwitz BL, Sullivan MB. VirSorter: mining viral signal from microbial genomic data. PeerJ. (2015) 3:e985. doi: 10.7717/peerj.985
36. Tauch A, Krieft S, Kalinowski J, Pühler A. The 51,409-bp R-plasmid pTP10 from the multiresistant clinical isolate Corynebacterium striatum M82B is composed of DNA segments initially identified in soil bacteria and in plant, animal, and human pathogens. Mol Gen Genet. (2000) 263:1–11. doi: 10.1007/PL00008668
37. Charpentier E, Gerbaud G, Courvalin P. Presence of the Listeria tetracycline resistance gene tet(S) in Enterococcus faecalis. Antimicrob Agents Chemother. (1994) 38:2330–5. doi: 10.1128/AAC.38.10.2330
38. Takeuchi K, Tomita H, Fujimoto S, Kudo M, Kuwano H, Ike Y. Drug resistance of Enterococcus faecium clinical isolates and the conjugative transfer of gentamicin and erythromycin resistance traits. FEMS Microbiol Lett. (2005) 243:347–54. doi: 10.1016/j.femsle.2004.12.022
39. Xu J, Gallert C, Winter J. Multiple antibiotic resistances of Enterococcus isolates from raw or sand-filtered sewage. Appl Microbiol Biotechnol. (2007) 74:493–500. doi: 10.1007/s00253-006-0668-z
40. Maasjost J, Mühldorfer K, Jäckel SC de, Hafez HM. Antimicrobial susceptibility patterns of Enterococcus faecalis and Enterococcus faecium isolated from poultry flocks in Germany. avdi. (2015) 59:143–8. doi: 10.1637/10928-090314-RegR
41. Wenzler S, Schmidt-Eisenlohr E, Daschner F. Comparative in vitro activities of three new quinolones and azithromycin against aerobic pathogens causing respiratory tract and abdominal wound infections. Chemotherapy. (2004) 50:40–2. doi: 10.1159/000077284
42. Xia M, Mu S, Fang Y, Zhang X, Yang G, Hou X, et al. Genetic and probiotic characteristics of urolithin A producing Enterococcus faecium FUA027. Foods. (2023) 12:1021. doi: 10.3390/foods12051021
43. Costa Y, Galimand M, Leclercq R, Duval J, Courvalin P. Characterization of the chromosomal aac(6′)-Ii gene specific for Enterococcus faecium. Antimicrob Agents Chemother. (1993) 37:1896–903. doi: 10.1128/AAC.37.9.1896
44. Cerdá P, Goñi P, Millán L, Rubio C, Gómez-Lus R. Detection of the aminoglycosidestreptothricin resistance gene cluster ant(6)-sat4-aph(3 ')-III in commensal viridans group streptococci. Int Microbiol. (2007) 10:57–60. doi: 10.2436/20.1501.01.8
45. Trieu-Cuot P, Courvalin P. Nucleotide sequence of the Streptococcus faecalis plasmid gene encoding the 3′5′'-aminoglycoside phosphotransferase type III. Gene. (1983) 23:331–41. doi: 10.1016/0378-1119(83)90022-7
46. Pansegrau W, Miele L, Lurz R, Lanka E. Nucleotide sequence of the kanamycin resistance determinant of plasmid RP4: homology to other aminoglycoside 3′-phosphotransferases. Plasmid. (1987) 18:193–204. doi: 10.1016/0147-619X(87)90062-X
47. Daly M, Villa L, Pezzella C, Fanning S, Carattoli A. Comparison of multidrug resistance gene regions between two geographically unrelated Salmonella serotypes. J Antimicr Chemother. (2005) 55:558–61. doi: 10.1093/jac/dki015
48. Perreten V, Schwarz FV, Teuber M, Levy SB. Mdt(A), a new efflux protein conferring multiple antibiotic resistance in Lactococcus lactis and Escherichia coli. Antimicrob Agents Chemother. (2001) 45:1109–14. doi: 10.1128/AAC.45.4.1109-1114.2001
49. Isnard C, Malbruny B, Leclercq R, Cattoir V. Genetic basis for in vitro and in vivo resistance to Lincosamides, Streptogramins A, and Pleuromutilins (LSAP phenotype) in Enterococcus faecium. Antimicrob Agents Chemother. (2013) 57:4463–9. doi: 10.1128/AAC.01030-13
50. Min Y-H, Kwon A-R, Yoon E-J, Shim M-J, Choi E-C. Translational attenuation and mRNA stabilization as mechanisms of erm(B) induction by erythromycin. Antimicrob Agents Chemother. (2008) 52:1782–9. doi: 10.1128/AAC.01376-07
51. Singh KV, Malathum K, Murray BE. Disruption of an Enterococcus faecium species-specific gene, a homologue of acquired macrolide resistance genes of Staphylococci, is associated with an increase in macrolide susceptibility. Antimicrob Agents Chemother. (2001) 45:263–6. doi: 10.1128/AAC.45.1.263-266.2001
52. Urshev Z, Yungareva T. Initial safety evaluation of Enterococcus faecium LBBE81. Biotechnol Biotechnol Equip. (2021) 35:11–7. doi: 10.1080/13102818.2020.1840438
53. Thumu SCR, Halami PM. Presence of erythromycin and tetracycline resistance genes in lactic acid bacteria from fermented foods of Indian origin. Antonie Van Leeuwenhoek. (2012) 102:541–51. doi: 10.1007/s10482-012-9749-4
54. Mulcahy H, O'Callaghan J, O'Grady EP, Adams C, O'Gara F. The posttranscriptional regulator RsmA plays a role in the interaction between Pseudomonas aeruginosa and human airway epithelial cells by positively regulating the type III secretion system. Infect Immun. (2006) 74:3012–5. doi: 10.1128/IAI.74.5.3012-3015.2006
55. Jacob J, Evers S, Bischoff K, Carlier C, Courvalin P. Characterization of the sat 4 gene encoding a streptothricin acetyltransferase in Campylobacter coli BE/G4. FEMS Microbiol Lett. (1994) 120:13–7. doi: 10.1111/j.1574-6968.1994.tb07000.x
56. Roberts MC. Update on acquired tetracycline resistance genes. FEMS Microbiol Lett. (2005) 245:195–203. doi: 10.1016/j.femsle.2005.02.034
Keywords: probiotics, ARG, NGS, companion animals, antimicrobial resistance
Citation: Kerek A, Szabó E, Szabó Á, Papp M, Bányai K, Kardos G, Kaszab E, Bali K and Jerzsele Á (2024) Investigating antimicrobial resistance genes in probiotic products for companion animals. Front. Vet. Sci. 11:1464351. doi: 10.3389/fvets.2024.1464351
Received: 13 July 2024; Accepted: 07 October 2024;
Published: 22 October 2024.
Edited by:
Iram Liaqat, Government College University, Lahore, PakistanReviewed by:
Riffat Iqbal, Government College, PakistanCopyright © 2024 Kerek, Szabó, Szabó, Papp, Bányai, Kardos, Kaszab, Bali and Jerzsele. This is an open-access article distributed under the terms of the Creative Commons Attribution License (CC BY). The use, distribution or reproduction in other forums is permitted, provided the original author(s) and the copyright owner(s) are credited and that the original publication in this journal is cited, in accordance with accepted academic practice. No use, distribution or reproduction is permitted which does not comply with these terms.
*Correspondence: Adam Kerek, a2VyZWsuYWRhbUB1bml2ZXQuaHU=
Disclaimer: All claims expressed in this article are solely those of the authors and do not necessarily represent those of their affiliated organizations, or those of the publisher, the editors and the reviewers. Any product that may be evaluated in this article or claim that may be made by its manufacturer is not guaranteed or endorsed by the publisher.
Research integrity at Frontiers
Learn more about the work of our research integrity team to safeguard the quality of each article we publish.