- 1CIISA–Centro de Investigação Interdisciplinar em Sanidade Animal, Faculdade de Medicina Veterinária, Universidade de Lisboa, Lisbon, Portugal
- 2Laboratório Associado para Ciência Animal e Veterinária (AL4AnimalS), Lisbon, Portugal
- 3Instituto Superior de Agronomia, Universidade de Lisboa, Lisbon, Portugal
- 4LEAF–Linking Landscape, Environment, Agriculture and Food, Instituto Superior de Agronomia, Universidade de Lisboa, Associated Laboratory TERRA, Lisbon, Portugal
This study evaluates the effect of prolonged feeding with a high inclusion level of Spirulina, combined with peptidases, on broiler chicken’s growth performance, digesta viscosity, carcass attributes and meat quality. The experiment involved 120 male broilers divided into 40 battery brooders, each housing 3 birds. Post 7-day acclimatisation with a corn and soybean-based diet, the birds were provided with one of four diets: a corn and soybean meal-based diet (CON), a mix incorporating 15% Spirulina (SP), a Spirulina-rich mix supplemented with 0.025% of commercial VemoZyme® P (SPV), or a Spirulina-rich mix supplemented with 0.10% of porcine pancreatin (SPP). The CON group had higher body weight and weight gain (p < 0.001) and a lower feed conversion ratio (p < 0.001) from day 7–21, compared to the Spirulina-fed groups. Spirulina-fed chickens significantly increased ileum viscosity (p < 0.05). Spirulina also elevated the weight (p < 0.05) of the duodenum and the length (p < 0.001) of the entire gastrointestinal tract compared to CON. Breast and thigh muscles from Spirulina-fed broilers displayed higher values of yellowness (b*) (p < 0.001), pigments (p < 0.05), and n-3 PUFA (p < 0.01), while n-6/n-3 ratio (p < 0.001) and α-tocopherol (p < 0.001) decreased relative to the CON. In conclusion, the introduction of a high level of Spirulina into broiler diets for an extended duration, has the potential to diminish birds’ growth performance, possibly due to increased digesta viscosity. However, it does enhance the nutritional quality of the meat.
1 Introduction
The escalating demand for alternative protein sources as substitutes for conventional ingredients, like soybean (1), has stimulated interest in microalgae as a promising candidate. Notably, poultry has significant potential for integrating microalgae into their diets, indicating its potential for broader application in commercial animal feed (2). In particular, Arthrospira platensis, more commonly known as Spirulina, has gained recognition as an advantageous component of poultry nutrition (3). Spirulina’s high protein content, fluctuating between 50 and 70% (4), positions it as a viable alternative or complement to traditional protein sources (5–7).
Spirulina, an edible microalga belonging to the blue-green algae group (Cyanophyceae or cyanobacteria), is recognized for its distinct filamentous multicellular structure and resilience in harsh environments (2, 8, 9). Its cell wall, akin to that of Gram-negative bacteria (10), is predominantly comprised of peptidoglycan and lipopolysaccharides, forming a robust multi-layered barrier (11). Aside from its high protein content and the presence of all essential amino acids (12), Spirulina serves as a bountiful reservoir of carbohydrates, a variety of vitamins including pro-vitamin A, vitamin C and vitamin E, as well as a host of minerals such as iron, calcium, chromium, copper, magnesium, manganese, phosphorus, potassium, sodium and zinc (13, 14). Further enriching its nutritional profile, Spirulina also provides essential fatty acids and an array of pigments including chlorophyll a, phycocyanin, carotenes and xanthophylls (4, 15–19).
Despite the nutrient-rich profile of Spirulina, the indigestible nature of its cell wall and the resilience of its protein-pigment complexes, known as phycocyanins, which are tied to microalgal thylakoid membranes, pose challenges to its protein’s bioaccessibility and digestibility (20). To mitigate these issues, recent in vitro studies (21, 22) have investigated the potential of peptidases (EC 3.4), with or without prior treatments, in the hydrolysis of Spirulina proteins. Notably, Niccolai et al. (22) observed a remarkable increase of up to 81% in crude protein digestibility of Spirulina when applying a combination of pepsin and pancreatin. Furthermore, Costa et al. (21) confirmed the beneficial effect of pancreatin, especially when preceded by an extrusion pre-treatment, on the hydrolysis of protein potentially associated with phycocyanins. Peptidases catalyse the degradation of peptide bonds, are involved in the degradation of off-function proteins in cells and play an important role in cell homeostasis (23). However, proteins freed from Spirulina biomass could undergo a gelation process, potentially elevating the viscosity of the digesta and thereby entrapping other nutrients, which limits their absorption in monogastric animals (24, 25). This effect was demonstrated by Pestana et al. (25), who noticed a surge in digesta viscosity resulting in hindered growth performance in broilers fed a high level of Spirulina (15% of feed) supplemented with lysozyme (26). A similar observation was made when piglets were fed 10% of the microalga, where Spirulina proteins showed resistance to the activity of endogenous peptidases, consequently reducing protein digestibility (27). The application of 15% of Spirulina in broilers has been already studied, with no negative effect on production performance or meat quality (28).
Although the beneficial role of exogenous enzymes, such as carbohydrate-active enzymes (CAZymes), in enhancing the nutritional value of poultry diets is well-recognized (26, 29, 30), there remains a significant gap in our understanding of the in vivo impact of peptidases on augmenting the bioaccessibility of Spirulina proteins for monogastric animals. These enzymes are used extensively for their broad-spectrum activity against various substrates like barley, a key component of broiler diets (31, 32). CAZymes facilitate improved access to endogenous enzymes to their target substrates, by enhancing feed conversion and digestibility, mainly in young animals with immature digestive tracts (29). Similar to CAZymes, supplementing peptidases in diets that contain a high proportion of Spirulina could potentially boost broiler digestibility. One of the mixtures contains peptidase and α-amylase (VemoZyme® P) and the other encompasses pancreatic peptidase, lipase and amylase (porcine pancreatin). Despite limited information available on the function and applicability of VemoZyme® P in poultry nutrition, related enzymes from the same manufacturer, namely VemoZyme® F (a phytase) and VemoZyme® Plus (a complex enzyme preparation including carbohydrases), have been successfully utilized in laying hens (33) and broilers diets (34). Pancreatin, as a diverse enzyme mixture, is anticipated to aid broilers with an immature digestive tract in handling dietary changes, such as a transition from a corn and soybean meal-based diet to a Spirulina-enriched diet. Notably, previous studies employed pancreatin mixtures in broiler diets, demonstrating positive outcomes for fat digestibility (35). However, to our knowledge, the effects of this mixture on the degradation of algal proteins have not been explored yet.
Furthermore, while certain studies have scrutinized the influence of dietary Spirulina incorporation on broiler performance and meat quality (e.g., Pestana et al. (25), Abdelfatah et al. (36), Costa et al. (37), and Hassan et al. (38)), there exists a paucity of research probing into the combination of long-duration feeding periods with high inclusion levels of Spirulina.
Hence, the purpose of this study was to investigate the impact of incorporating 15% Spirulina in broiler diets, either alone or combined with commercial VemoZyme® P or porcine pancreatin extract, on broiler growth performance, gut viscosity, carcass traits and meat quality, spanning from day 7–35.
2 Materials and methods
2.1 Animal welfare statement
The experimental procedures conducted in this study were performed in compliance with ethical guidelines and regulations. The protocols followed were approved by the Ethics Commission of CIISA/FMV (Centre for Interdisciplinary Research in Animal Health, Faculty of Veterinary Medicine) and the Animal Care Committee of the National Veterinary Authority (Direção Geral de Alimentação e Veterinária, Lisboa, Portugal). Additionally, the study adhered to the principles and specific guidelines outlined in the European Union legislation (2010/63/EU Directive) concerning the use of animals in scientific research. The Animal Welfare Committee of the Higher Institute of Agronomy at the University of Lisbon (ORBEA/ISA) also approved the experimental procedures with animals, and the study was assigned a protocol code number 0421/000/000/2022.
2.2 Animals, management and diets
A total of 120 one-day-old male Ross 308 broiler chicks were housed in 40 wired-floor cages for 35 days, following the procedures described in Alfaia et al. (39), Pestana et al. (25), and Costa et al. (40). The birds were reared in a controlled environment room under standard brooding practices, with appropriate lighting conditions. At day 0, room temperature was maintained at 31°C, following 30°C on day 1, and from day 3 to 27, it decreased 1°C every 3 days to 20°C, after it remained constant until the end of the study. The temperature and ventilation in the room were continuously monitored throughout the study period from day 1–35. Also, the temperature at the cage level was monitored from day 1–35. Each cage measured 66 × 66 cm, with 2 drinking nipples and one feeder.
During the initial 7 days, the birds were provided with ad libitum access to a corn and soybean-based diet. From day 7–35, the birds were assigned to one of four dietary treatments: 1) a control diet based on corn and soybean meal (CON); 2) a diet containing 15% Spirulina powder (Allmicroalgae, Pataias, Portugal) (SP); 3) a diet containing 15% Spirulina powder supplemented with 0.025% of the commercial enzyme mix VemoZyme® P (VEMO, Sofia, Bulgaria) (SPV); and 4) a diet containing 15% Spirulina powder supplemented with 0.10% of porcine pancreatin extract (Merck, Darmstadt, Germany) (SPP). The VemoZyme® P enzyme had a proteolytic activity of 15,000 tyrosine units and an amylase activity of 400 units, as stated by the manufacturer. The porcine pancreatin extract contained 350 FIP-U/g protease, 6,000 FIP-U/g lipase and 7,500 FIP-U/g amylase. All diets were formulated to meet the nutrient requirements defined by the NRC (41), and the compositions and nutrient content analysis of the starter, and grower diets plus microalga powder are provided in Tables 1, 2, respectively.
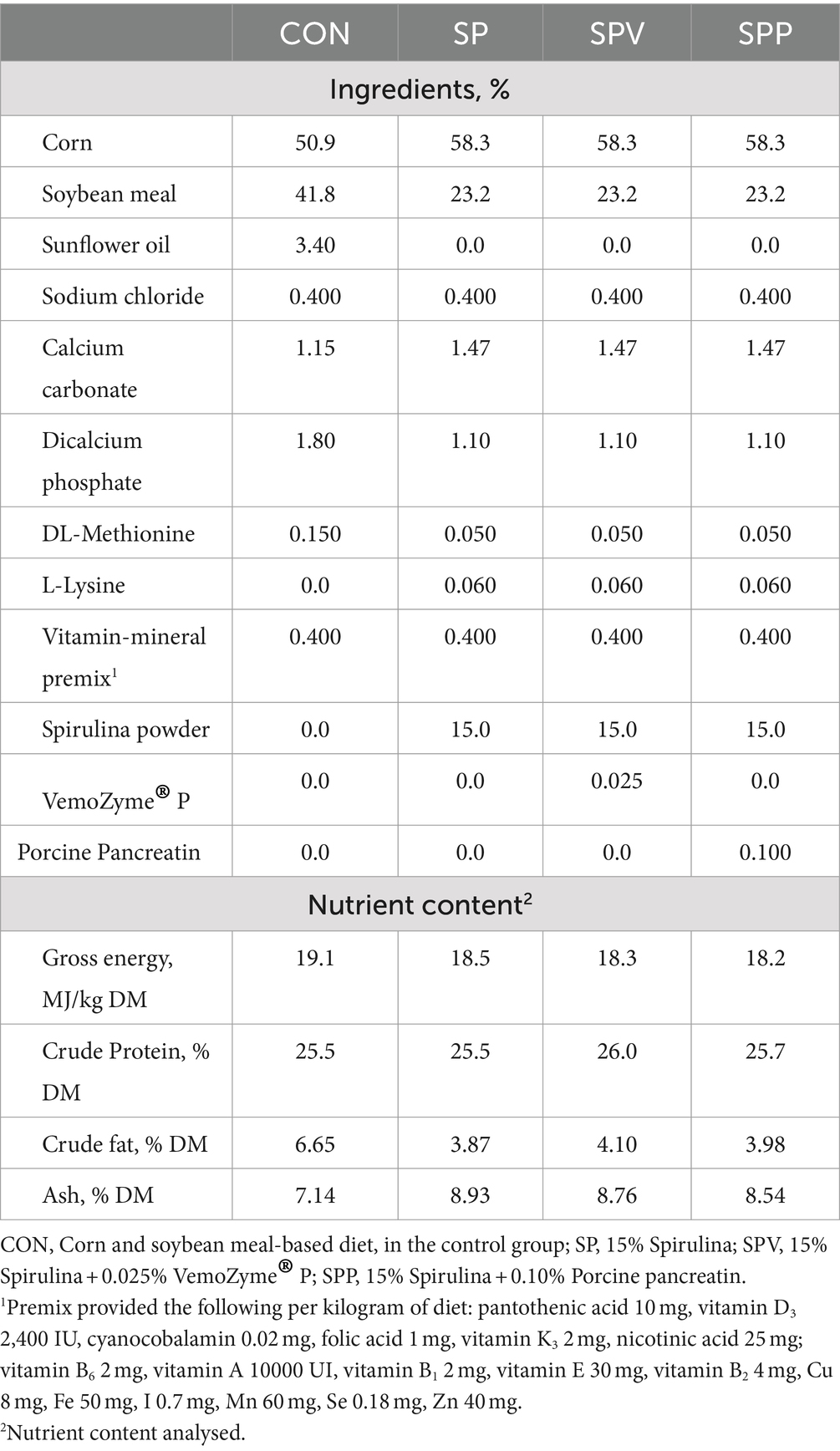
Table 1. Ingredient composition and nutrient content analysis of broiler experimental starter diets (day 7–14) (%, as-fed basis).
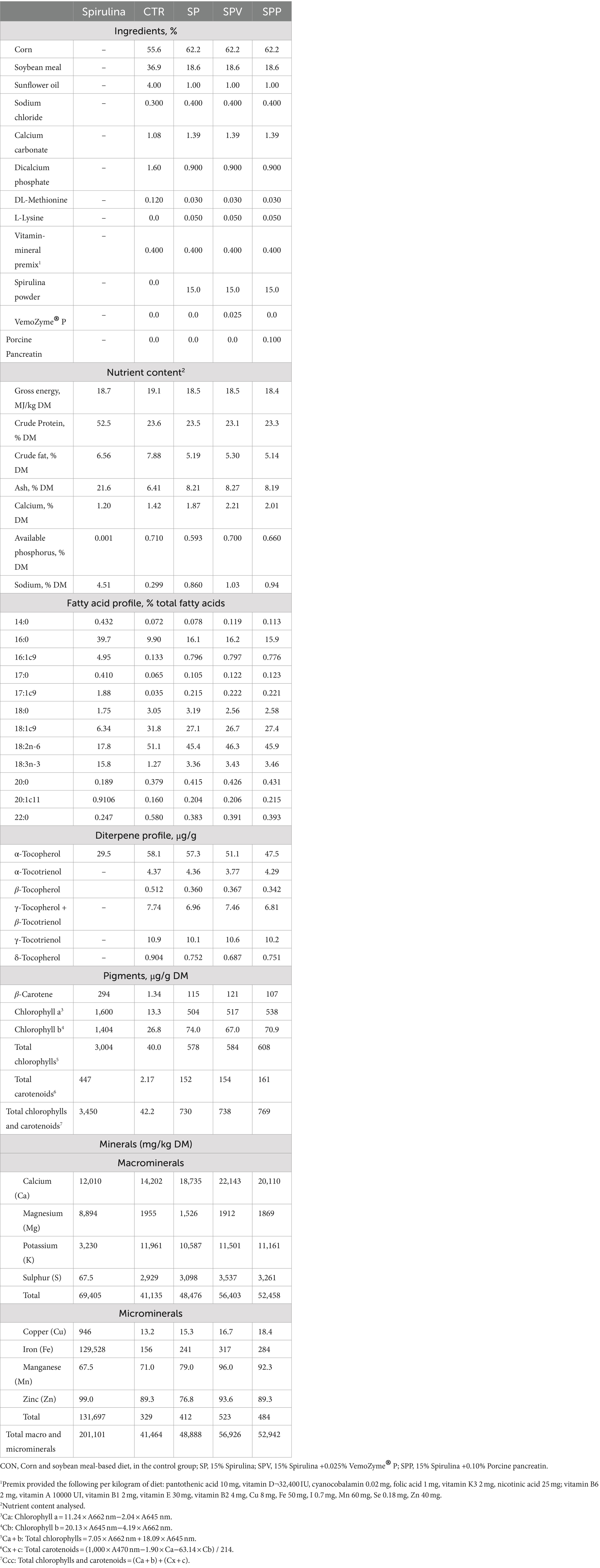
Table 2. Ingredient composition and nutrient content analysis of broiler experimental grower diets and microalga powder (day 14–35) (%, as-fed basis).
The experimental design consisted of 10 replicate cages, with 3 birds per cage. Weekly measurements of broiler and feeder weights were recorded, and feed was provided daily to calculate body weight gain (difference between body weight of two consecutive weeks, divided by 7), average daily feed intake (weekly ingestion by each cage, divided by 7) and feed conversion ratio (ratio between weekly ingestion divided by 3 and weekly body weight gain). Diet samples were analysed for dry matter (DM) by drying a sample at 103°C until a constant weight was achieved. The nitrogen (N) content of the diets was determined using the Kjeldahl method according to AOAC method 954.01 (42), and crude protein content was calculated as 6.25 times the nitrogen content. Ash content was determined using AOAC method 942.05 (42). Crude fat was determined by extracting feed samples with petroleum ether using an automatic Soxhlet extractor (Gerhardt Analytical Systems, C. Gerhardt GmbH & Co. KG, Königswinter, Germany), following a prior hydrolysis with hydrochloric acid. The gross energy of the feed was determined using adiabatic bomb calorimetry (Parr 1,261, Parr Instrument Company, Moline, IL, United States).
On day 35, one bird from each cage, with the middle weight, was subjected to electrical stunning and manual exsanguination. Blood samples were collected in Sarstedt tubes (Numbrecht, Germany) and centrifuged to obtain serum. The gastrointestinal (GI) organs (crop, gizzard, duodenum, jejunum, ileum and cecum) were removed and emptied, and the weight of the crop, gizzard, liver, pancreas, duodenum, jejunum, ileum and cecum was recorded. The length of the duodenum, jejunum, ileum, and cecum was also measured. Duodenum in the proximal part of the intestinal tract, with a “U” shape around the pancreas. The jejunum is the distal part of the duodenum. Anterior to the junction of the cecum, is the ileum. The cecum is a paired tubular structure distal along the ileum from the ileo-caecal-colic junction (43). The viscosity of the contents of the small intestine was determined following the method described by Pestana et al. (25). Briefly, samples were collected from the duodenum plus jejunum and ileum, centrifuged for 10 min at 9.000 rpm, and the viscosity of the supernatant was measured using a viscometer (Model LVDVCP-II, Brookfield Engineering Laboratories, Middleboro, MA, United States) at a room temperature maintained at 24°C. Samples of breast and thigh muscles (deboned and skinless) were chopped and kept in aluminium foil, bagged in vacuum bags and stored at −20°C until analyses.
2.3 Determination of carcass traits
The pH and colour analysis of the meat were conducted following the methods described in Pestana et al. (25) and Alfaia et al. (39). Briefly, the right breast (pectoralis major) and thigh (biceps femoris) muscles were deboned and skinned. Triplicate measurements were taken from three different spots on each muscle. The pH values were determined using a glass penetration pH electrode (HI9025, Hanna Instruments, Woonsocket, RI, United States), while colour measurements [lightness (L*), redness (a*), and yellowness (b*)] were obtained using a Minolta CR-300 Chromameter (Minolta Camera Co. Ltd., Osaka, Japan) based on the CIELAB colour space. The data were recorded after a 24-h cooling post-mortem period at 4°C, followed by 1 h of air exposure.
2.4 Sensorial panel traits of breast meat
The sensory analysis was performed following the procedures outlined in Pestana et al. (25). In brief, the right skinless breast muscles were individually cooked in a water bath at 85°C using plastic bags until the internal temperature reached 78°C. For the sensory evaluation, the cooked muscle samples were trimmed of external connective tissue and cut into approximately 1 cm3 cubes. These cubes were then kept at 60°C on pre-identified heated plaques. A panel of 10 trained assessors, selected and trained according to Cross (44) at the Faculty of Veterinary Medicine (University of Lisbon, Portugal), evaluated the samples. The sensory panel sessions consisted of five sessions, with eight random samples per session. The attributes assessed were tenderness, juiciness, off-flavours and overall acceptability. A structured 8-point scale was used for the sensory evaluation, with 1 representing extremely tough, dry, weak, and negative attributes, and 8 representing extremely tender, juicy, strong, and positive attributes for tenderness, juiciness, flavour and overall acceptability, respectively.
2.5 Determination of meat lipid oxidative stability
Approximately 1.5 g of minced meat from the left breast of each bird was divided into four portions and placed in plastic bags. These portions were then exposed to air and stored in a freezer at 4°C for 0 and 8 days. The concentration of thiobarbituric acid reactive substances (TBARS), an indicator of lipid oxidation, was measured on day 0 and day 8. The spectrophotometric method described by Mercier et al. (45) was followed to analyse the ability of malondialdehyde, a product of lipid oxidation, to form a pink-coloured chromogen that absorbs 532 nm light. The measurements were taken using a UV/visible spectrophotometer (Genesys 150, ThermoScientific, Madison, United States). For TBARS quantification, a standard calibration curve was prepared using 1,1,3,3-tetraethoxypropane (Fluka, Neu Ulm, Germany) as a precursor of malondialdehyde. The results are expressed as milligrams of malondialdehyde per kilogram of meat.
2.6 Determination of total cholesterol, diterpenes, pigments and minerals in meat and experimental diets
The determination of total cholesterol, β-carotene and tocopherols in both fresh meat (750 mg) and feed (100 mg) followed the protocol described by Prates et al. (46), with specific details provided in Pestana et al. (25), Alfaia et al. (39), Costa et al. (40). The measurements were performed in duplicate, and the concentrations were calculated based on the external standard technique using a standard curve of peak area versus concentration.
For the analysis of chlorophyll a, chlorophyll b and total carotenoids, the method outlined by Teimouri et al. (47) was employed, with slight modifications described in Pestana et al. (25), Alfaia et al. (39), and Costa et al. (40). All procedures related to pigment extraction and analysis were conducted under dim light conditions to minimize the photodegradation of pigments. The contents of pigments were determined using equations described by Hynstova et al. (16).
To determine the mineral profile, including calcium (Ca), potassium (K), magnesium (Mg), sodium (Na), phosphorus (P), sulphur (S), copper (Cu), iron (Fe), manganese (Mn) and zinc (Zn), the procedures outlined in Ribeiro et al. (48) and Costa et al. (40) were followed. Inductively coupled plasma optical emission spectrometry (ICP-OES) with an iCAP 7,200 duo instrument (Thermo Scientific, Waltham, MA, United States) was utilized for the analysis. Calibration curves using multi-element standards (PlasmaQual S22, SPC Science, Baie-D’Urfé, QC, Canada) were employed to quantify the different elements.
2.7 Determination of dry matter and total lipids in meat
The dry matter content of breast and thigh meats was determined following the method described by Rosenkranz (49). Freeze-dried samples were obtained by using a freeze-drier (Labogene, CoolSafe, Lillerod, Denmark) at −60°C and 2.0 hPa. The freeze-drying process was carried out until a constant weight was achieved. After freeze-drying, the samples were stored in desiccators at room temperature until further analysis.
To determine the total lipids in the meat samples, as well as in the feed, the freeze-dried breast and thigh muscles were subjected to lipid extraction according to the procedure outlined by Folch et al. (50). The extraction was performed using a mixture of dichloromethane and methanol in a ratio of 2:1 (v/v). The extracted lipids were then evaporated to dryness, and the fatty residue obtained was weighed gravimetrically. The measurements were performed in duplicate.
2.8 Determination of fatty acid composition in meat and experimental diets
The fatty acid composition of breast and thigh muscles was determined by converting the fatty acid residue into fatty acid methyl esters (FAME). This conversion was carried out using a combined basic and acidic transesterification procedure. Firstly, the fatty acids were transesterified using NaOH in anhydrous methanol (0.5 M) at 50°C for 30 min. Subsequently, HCl/methanol (1:1 v/v) was used for a second transesterification step at 50°C for 10 min (51). The same procedure was applied for the analysis of FAME in feed samples, except that direct transesterification was performed using HCl/methanol (1:1 v/v) at 70°C for 2 h.
The analysis of FAME was conducted following the method described by Pestana et al. (25). Gas chromatography (GC System, 7890A, Agilent Technologies, California, United States) comprising a Supelcowax® 10 capillary column (30 m × 0.20 mm internal diameter, 0.20 μm film thickness; Supelco, Bellefonte, PA, United States) and flame ionization detector, was employed for the analysis, using specific parameters. The injector temperature and detector temperature were set at 250°C and 280°C, respectively. Helium gas was used as the carrier gas at a flow rate of 1.0 mL/min, with a split ratio of 1:20. The gas chromatograph oven temperature was programmed as follows: an initial temperature of 50°C was maintained for 4 min, followed by a ramp of 13°C/min to 175°C (maintained for 20 min), and then a ramp of 4°C/min to 275°C (maintained for 44 min).
Identification of FAME was based on a comparison of retention times with a standard (FAME mix 37 components, Supelco Inc. Bellefonte, PA, United States) confirmed by gas chromatography coupled to mass spectrometry using a GC–MS QP2010-Plus (Shimadzu, Kyoto, Japan). Quantification of FAME was achieved using heneicosanoic acid (21:0) methyl ester as the internal standard. The resulting fatty acid composition was expressed as g/100 g of total fatty acids.
2.9 Statistical analysis
All the data were analysed using the Generalized Linear Mixed (GLM) model of the SAS program (SAS Institute Inc., Cary, NC, United States) for most variables, except for the TBARS values, the repeated measures in time (PROCMIXED) procedure of SAS were employed. The cage served as the experimental unit for body weight, body weight gain, average daily feed intake and feed conversion ratio, while the individual bird was considered as the experimental unit for gastrointestinal organs weight and length, and content viscosity and meat quality measurements. To determine statistical differences among dietary treatments, a significant multiple comparisons test was conducted using the PDIFF option, which was adjusted with the Tukey–Kramer method. A p-value less than 0.05 was considered statistically significant to indicate meaningful differences.
3 Results
3.1 Growth performance and gastrointestinal tract parameters
Table 3 compiles the data on birds’ growth performance and gastrointestinal tract parameters in response to the different dietary conditions. Birds subjected to Spirulina diets manifested diminished body weights (BW) on day 35, in contrast to those sustained on the control diet (CON) (p < 0.001). The pattern persisted throughout the treatment span (days 14, 21, 28 and 35), wherein birds fed with Spirulina (SP, SPV and SPP) consistently showed lesser gains in body weight (BWG) and lower average daily feed intake (ADFI) relative to the control group (CON) (p < 0.001).
Similarly, the feed conversion ratio (FCR) displayed higher values for the Spirulina-fed groups (SP, SPV and SPP), as compared to the control group (CON) (p < 0.001). However, this trend was interrupted between days 21–28 and 28–35, wherein no significant FCR differences were noted. In addition, birds fed Spirulina, irrespective of enzyme supplementation, presented with increased viscosity in the ileum contents relative to their counterparts in the control diet (CON) (p < 0.001).
Despite these trends, most gastrointestinal (GI) tract organ weights remained largely unaffected by the dietary treatment (p > 0.05), as outlined in Table 4. Exceptions included the relative weights of the pancreas (p < 0.001) and duodenum (p < 0.05), which increased across all Spirulina-fed groups (SP, SPV and SPP). Moreover, the relative lengths of the duodenum, jejunum, ileum and caecum were greater in all Spirulina-fed groups (SP, SPV and SPP) (p < 0.001).
3.2 Carcass traits, meat quality and sensory evaluation
Table 5 presents a detailed analysis of the impact of dietary treatments on the carcass traits and meat quality of broiler birds. The study found no significant variance in the lightness (L*) and redness (a*) of the breast and thigh meats across the different dietary treatments (p > 0.05). However, significant differences were observed in terms of pH24h (p < 0.05) and yellowness (b*) (p < 0.001), both of which were markedly higher in birds fed Spirulina as compared to those subjected to CON.
As presented in Table 6, sensory evaluations, conducted by a trained panel for the breast meat, highlighted several significant differences between the diet groups. Birds that were fed Spirulina diets, irrespective of whether these were supplemented with enzymes, exhibited lower scores for meat tenderness, juiciness, and overall acceptability compared to those in the control group (CON) (p < 0.001). Nonetheless, the inclusion of both enzymes (SPV and SPP) appeared to counteract the decrease in meat flavour attributed to the Spirulina diets (p < 0.05). The addition of pancreatin seemed to particularly aid in reversing the decline in meat tenderness and overall acceptability. Of note, dietary treatments did not result in any significant changes in off-flavours (p > 0.05).
3.3 Oxidative stability, diterpenes and total pigments
Table 7 presents the findings on the oxidative stability of broiler breast meat across various dietary treatments over two time periods (day 0 and day 8). Oxidative stability is gauged by the measurement of TBA reactive substances, specifically MDA levels. It is worth noting that no significant interaction was found between dietary treatment and period (p > 0.05). On both day 0 and day 8, the MDA levels across all dietary treatments did not demonstrate significant differences (p > 0.05). However, a noteworthy exception was observed with the SPV treatment, which showed a marked increase in MDA levels between day 0 and day 8 (p < 0.001). This indicates that there might be an increase in the oxidation rate of broiler meat within this specific dietary group over time.
Table 8 depicts the impact of different dietary treatments on the diterpene profile and pigments in the meat of broilers. The data indicate a marked decrease in the levels of diterpenes, specifically α-tocopherol and γ-tocopherol+β-tocotrienol, in both breast and thigh meats of broilers fed with Spirulina-based diets when compared to the control group (CON) (p < 0.001). Moreover, the minor diterpenes, α-tocotrienol and β-tocopherol, were detected in breast meat but were absent in thigh meat. Conversely, the levels of pigments, including total chlorophylls, total carotenoids and their combined sum, were significantly elevated in the broiler breast and thigh meats from the Spirulina-fed groups (SP, SPV and SPP) compared to the control group (CON) (all p < 0.001, except p = 0.003 in total chlorophylls in breast meat). Among the Spirulina-fed groups, those combined with enzymes (SPV and SPP groups), exhibited higher levels of β-carotene in breast meat compared to the control group (CON) (p = 0.008), although no significant differences were found in the thigh muscle.
3.4 Meat lipids and fatty acid composition
Table 9 presents the total lipid content, total cholesterol content and fatty acid profile of both breast and thigh meats. The total lipid and total cholesterol contents of breast meat in Spirulina-fed birds were significantly lower (p < 0.001) than those fed the control diet (CON). However, the difference was not statistically significant in the thigh meat (p = 0.061 for total lipids, and p = 0.683 for total cholesterol).
For the fatty acid profile of breast meat, there was a significant increase (p < 0.001, in general) in the levels of several fatty acids (16:0, 17:0, 14:1c9, 16:1c9, 17:1c9, 18:1c11, 20:3n-6, 20:4n-6, 20:3n-3, 20:5n-3, 22:5n-3 and 22:6n-3), saturated fatty acids (SFA), and n-3 polyunsaturated fatty acids (n-3 PUFA) in Spirulina-fed birds. Additionally, Spirulina-fed groups (SP, SPV and SPP) led to decreased content of 18:2n-6 (p < 0.001), total PUFA (p = 0.001), n-6 PUFA (p < 0.001), n-6/n-3 (p < 0.001), and PUFA/SFA (p < 0.001) ratios. An interesting observation was a tendency for the content of 18:3n-6 to decrease with SP or SPP diets, and an increase with the SPV diet (p = 0.046). SPV and SPP diets also significantly increased 18:3n-3 (p = 0.033) and 20:2n-6 (p = 0.002) contents, respectively.
Similarly, for the thigh meat, Spirulina-fed birds exhibited increased contents of certain fatty acids (16:0, 17:0, 14:1c9, 16:1c9, 17:1c9, 18:1c11, 18:3n-3 and n-3 PUFA) compared to the CON group (majority p < 0.001). However, 18:2n-6 (p = 0.003), 18:3n-6 (p = 0.001) content, and n-6/n-3 ratio (p = 0.022) were significantly lower in Spirulina-fed groups (SP, SPV and SPP). There was a potential decrease in total PUFA content (p = 0.049) with the inclusion of Spirulina. The thigh meat of birds fed SP and SPV showed higher contents of 22:6n-3 (p = 0.003), SFA (p = 0.008), and a significantly lower PUFA/SFA ratio (p = 0.005) than the control (CON). The 20:5n-3 content was significantly increased by SP and SPP diets (p = 0.005), whereas SPV significantly reduced n-6 PUFA content (p = 0.022).
3.5 Mineral composition
The mineral composition of breast and thigh meats of broilers subjected to the different diets is presented in Table 10. The influence of Spirulina-enriched diets is seen to have differing effects on the mineral composition of breast and thigh meats. For the breast meat, both zinc content and overall micromineral concentration significantly decreased as a result of Spirulina integration (p < 0.001). Conversely, the copper content increased in the breast meat from broilers fed the SPP diet in comparison to the control group (CON) (p = 0.030). In the case of thigh meat, there was a significant decrease in the levels of phosphorus (p = 0.014), total macrominerals (p = 0.016), zinc (p < 0.001) and total minerals (p = 0.014) in groups fed Spirulina (SP, SPV and SPP), whereas the sodium and total micromineral content exhibited an increase (p = 0.007 and p < 0.001, respectively). A decrease in magnesium and potassium was also observed in the thigh meat of birds that were fed SPV and SPP diets (p = 0.010 and p = 0.029, respectively).
To put the above results into perspective, for instance, the zinc level in breast meat declined from 1.15 mg/100 g in the CON to 0.698 mg/100 g in the SPP group. Similarly, in the thigh meat, the phosphorus content was reduced from 246 mg/100 g in the CON to 234 mg/100 g in the SP and SPV groups. However, sodium content in the thigh meat increased from 102 mg/100 g in the CON to 111 mg/100 g in the SPP group.
4 Discussion
The presence of protein and pigment complexes attached to the thylakoid membrane (20, 52) in Spirulina, makes the hydrolysis of algal proteins difficult. This associated with the recalcitrance of microalga cell walls, compromises nutrients’ bioaccessibility and bioavailability (12). To improve the bioaccessibility of microalga nutrients in broilers, this study incorporated two commercial enzyme mixtures into the diets.
Spirulina is frequently used as a supplement or ingredient in poultry diets, with incorporation levels ranging between 0.25 and 20% (12). Notably, Valente et al. (53) suggested that microalga incorporation below 15% can augment meat quality without compromising performance. Conversely, higher incorporation levels may promote biomass gelation and escalate digesta viscosity, thereby potentially hindering nutrient digestibility and bioaccessibility (25, 54). In our study, broilers fed with Spirulina showed significantly lower BW and BWG, coupled with an increased FCR compared to the control groups. These findings echo the results of Pestana et al. (25) in a trial involving the same level of inclusion but over half the period (days 21–35). The present results can be attributed to the increased digesta viscosity in the digestive tract, particularly in the ileum, as suggested by Pestana et al. (25). This may have been due to an increase in digesta viscosity, compromising nutrient digestibility. However, interestingly, the FCR remained unaffected by the inclusion of the microalga in the diet from day 21–35. Moreover, an increase in the weight of the duodenum and the length of the duodenum, jejunum and ileum, as observed in Spirulina-fed groups (SP, SPV and SPP), could be a result of the increase in digesta viscosity. The enhanced viscosity can significantly influence nutrient digestibility, bioavailability and accessibility, leading to compromised animal growth and performance (55). In addition, it was reported that higher intakes of Spirulina (above 1.5%) may impact metabolic functions and slow growth rates in broilers (56), while smaller percentages can either improve or have no effect on poultry growth (57, 58). Further, high incorporation levels of Spirulina (up to 21%) can lead to protein gelation and increased digesta viscosity (24, 25), which in turn affects protein and amino acid digestibility and stalls animal growth (59). The discrepancies among various published studies may stem from factors like diverse Spirulina growing conditions, variable inclusion levels in diets and nutritional composition (57). Interestingly, in our study, the enzymatic supplementation in the diet did not reverse the decreased growth performance associated with microalga incorporation. However, the addition of pancreatin slightly mitigated the negative impact on body weight gain from day 28–35. The increase in digesta viscosity caused by high levels of Spirulina proteins in the diet may have trapped the enzymes, thereby inhibiting their action on feed nutrients, a phenomenon that warrants further investigation. An investigation by our group with Spirulina and combination of two types of pre-treatments, such as extrusion method or enzymatic (pancreatin and lysozyme) have shown good results in combining pre-treatments in growth performance, with similar FBW and BWG to control (37).
In the poultry industry, meat colour holds significant importance from the consumer’s perspective. The colour preferences vary widely depending on factors like geographical location, dietary preferences and environmental conditions (60). The supplementation of Spirulina, particularly in concentrations ranging from 4.0 to 15%, has been noted to enhance the redness and yellowness of poultry meat while reducing its lightness. The elevated redness may be attributed to the high iron and mineral content, and the increased yellowness could be due to the greater proportion of carotenoids, such as β–carotene and zeaxanthin present in the flesh (60–62). Our study further reinforced these findings, with Spirulina incorporation deepening the yellowness of both breast and thigh meats. This aligns with previous studies by Toyomizu et al. (60), Venkataraman et al. (63), and Altmann et al. (64). Notably, the latter demonstrated that diets supplemented with Spirulina led to more pronounced colour pigmentation of the skin, breast, and thigh meat in broilers. In a study by Moujahed et al. (65) it was observed that incorporating 2.5 and 5% Spirulina into the diet enhanced the yellowness of the breast and skin colour in Arbor Acres broilers. However, a lower level of Spirulina supplementation (0.25, 0.5, 0.75 and 1%) in the diet of Ross 308 male broilers did not significantly affect the colour of the breast meat (58). These differences are not only perceptible in instrumental analysis but also noticeable to the naked eye (64). An orange hue in poultry meat may be perceived as advantageous by consumers when Spirulina is incorporated into broilers’ diets. Meat colour is a significant quality indicator in several countries, particularly in Japan (66), the United States, and Mexico (67). Interestingly, when the breast muscles were cooked for the sensory panel, no discernible differences between samples were found. For the sensory panel, the overall acceptance of all diets was positive (scores between 5 and 6), and the absence of any significant difference in off-flavours between treatment groups, positions Spirulina-fed chicken favourably when compared to meats from birds fed on fish oil diets (68) or those rich in docosahexaenoic acid from algal products, which may affect off-flavours in meat.
Substituting corn-based diets with Spirulina necessitates careful analysis. Spirulina is a novel ingredient incorporated in diets, so it is important to do randomized, double-blind and placebo clinical trials to test the microalga’s functionality (13). The latter’s higher PUFA content could contribute to lipid oxidation, thereby negatively impacting meat quality (69, 70). The PUFA content directly influences lipid oxidation (71), rendering thigh meat. This portion contains more lipids than breast meat, more prone to lipid oxidation (72, 73). Despite the greater lipid and PUFA content in thigh meat, we focused our analysis on TBARS in breast meat due to its prominence in consumers’ diets. Levels of TBARS exceeding 0.8 mg/kg can indicate rancidity and adversely affect meat acceptability because of the resultant oxidized flavour, which is not acceptable to consumers (57, 74). In our study, TBARS levels in breast meat ranged from 0.14 to 0.56 mg/kg, significantly below this threshold.
Spirulina is a microalga renowned for its high content of antioxidants such as carotenoids, β-carotene, and tocopherols (75), which can protect lipids from oxidation (76). Our research indicates that the high levels of total carotenoids in both types of poultry meat may reflect Spirulina’s antioxidant capabilities and its ability to enhance the oxidative stability of broiler meat. The high carotenoid content in chickens fed Spirulina could explain the increase in meat yellowness, as a greater proportion of carotenoids in the meat can result in heightened yellowness. In the thigh meat of Spirulina-fed groups (SP, SPV, and SPP), the α-tocopherol and γ-tocopherol+β-tocotrienol content was higher than in the breast meat, likely due to the thigh’s higher fat content (77). Nevertheless, the decreased value of α-tocopherol in Spirulina-fed groups compared to the control might be attributed to several factors. Firstly, the oxidative stress induced by the higher polyunsaturated fatty acids (PUFAs) in Spirulina could increase the consumption of α-tocopherol as an antioxidant to counteract lipid peroxidation. Secondly, Spirulina’s high phycocyanin content may provide an additional antioxidant effect, thereby reducing the need for α-tocopherol and causing its levels to decrease as it is utilized more rapidly in oxidative processes. Despite the reduction, the α-tocopherol levels in Spirulina-fed groups remain within the typical range found in chickens (77, 78). Of the vitamin E diterpenes, α-tocopherol was found in the highest concentrations, while other homologues were present at lower levels, consistent with typical values in broilers (77, 78). Therefore, our results indicate that incorporating 15% Spirulina into the diet can enhance the antioxidant function of broiler meat through its high carotenoid content and possibly through synergistic effects with its other antioxidant compounds.
Breast and thigh meats are recognized as lean meats due to their low-fat content, which is generally less than 5% (79). In this study, these criteria were met as the total lipid content ranged from 0.82 to 1.35% in the breast and 2.32 to 2.90% in the thigh. The thigh meat exhibited a higher cholesterol content than the breast, surpassing values reported by Pestana et al. (25). However, the introduction of Spirulina led to a decrease in the cholesterol values of breast meat.
The fatty acid profile was found to be affected by Spirulina in both types of meat, corroborating previous findings by Cortinas et al. (80) and Botsoglou et al. (81). The most abundant fatty acids across all diets were SFA, such as palmitic (16:0) and stearic (18:0) acids, monounsaturated fatty acids (MUFA), like palmitoleic (16:1c9) and oleic acid (18:1c9), n-3 PUFA α-linolenic acid (18:3n-3), and n-6 PUFA, such as linoleic acid (18:2n-6) and arachidonic acid (20:4n-6). These fatty acids are also the most prevalent in Spirulina. The incorporation of Spirulina in the diet increased the SFA content in both types of meat, with palmitic (16:0) and stearic (18:0) acids being predominant. SFA are more resistant to oxidation and are more readily deposited in meats (76). The arachidonic acid content was higher in breast meat that included Spirulina, which could be due to the conversion of linoleic acid (82). Furthermore, the linoleic acid content was reduced in the breast and thigh of broilers fed diets containing Spirulina, and arachidonic acid was not detected in the microalga powder or diets. Both cereal-based feed and Spirulina are weak sources of α-linolenic acid, with the latter containing less than 1% (83). However, the SPV for the breast and SP, SPV and SPP for the thigh, led to an increase in α-linolenic acid, thereby improving the n-3 PUFA content. Due to the competition for desaturase enzymes, which are the same for both n-6 and n-3 pathways, the conversion of essential fatty acids, mainly α-linolenic acid, along the entire n-3 pathway is a limited mechanism (84, 85). Thus, it is crucial to ensure an appropriate dietary linoleic acid/α-linolenic acid ratio to obtain an efficient conversion of α-linolenic acid into beneficial eicosapentaenoic acid (20:5n-3) and docosahexaenoic acid (22:6n-3) (86). In this study, an increase in eicosapentaenoic and docosahexaenoic acids was observed in the breast and thigh meat of broilers fed Spirulina. This suggests a possible conversion of α-linolenic acid into these long-chain PUFA (LC-PUFA), as they were not detected in the microalga. Additionally, the decrease in total PUFA in breast meat, and a trend towards a decrease in the thigh, may suggest an increase in the de novo synthesis of fatty acids, facilitated by Δ6 and Δ5 desaturases and elongases (87). Aside from the LC-PUFA content, the fatty acid profile from the breast and thigh meats was consistent with the diets, as reported by other studies (77, 88). The addition of VemoZyme® P and porcine pancreatin did not alter these fatty acid profiles.
The changes in meat fatty acid profile, particularly the increase in n-3 PUFA and the decrease in n-6/n-3 ratio, can be attributed to the composition of Spirulina. Spirulina is a rich source of n-3 fatty acids, including alpha-linolenic acid, which can be converted into longer-chain n-3 PUFA such as eicosapentaenoic acid and docosahexaenoic acid in broilers. The dietary inclusion of Spirulina likely enhanced the deposition of these fatty acids in the meat (4, 89). PUFA play essential roles in functions such as the production and metabolism of prostaglandins, thromboxanes and leukotrienes (89, 90). Dietary guidelines recommend a PUFA/SFA ratio in human diets above 0.45 and an n-6/n-3 ratio not exceeding 4.0 (91). In this study, the guideline for the PUFA/SFA ratio was met, but the n-6/n-3 ratio in breast and thigh meats across dietary treatments significantly exceeded the recommended levels.
Minerals play a significant role in various physiological processes, including maintaining normal body fluids, regulating absorption, secretion and excretion, as well as contributing to skeletal structure and soft tissues (92). Although the integration of Spirulina into the diet did not influence the macro-mineral composition in the breast meat, in the thigh meat, there was a noticeable decrease in magnesium, potassium and total content among groups fed Spirulina (SP, SPV and SPP). Magnesium is a critical player in cellular functions, acting as a cofactor in all major metabolic pathways (93, 94). In contrast, sodium content was higher in groups fed Spirulina (SP, SPV and SPP) compared to the control diet (CON). Sodium and potassium play a crucial role in maintaining water balance, regulating muscle and heart activities, as well as hydrogen ion regulation, and gastric and kidney secretions. They are also integral constituents of blood and body fluids (92).
Regarding micro-minerals, zinc and total content were found to be lower in both breast and thigh meats of the Spirulina-fed groups (SP, SPV and SPP). Zinc, a vital micromineral, is important for the growth and development of broilers, as it influences DNA synthesis and the metabolism of lipids and carbohydrates (95–97). The decrease in zinc levels in the treatment groups may be explained by the high phytic acid content in Spirulina, which is known to bind zinc and reduce its bioavailability. Furthermore, the increased gastrointestinal viscosity due to Spirulina could impair the absorption of minerals, including zinc. These factors likely contributed to the reduced zinc levels observed in the Spirulina-fed groups. Nevertheless, this decreased should be considered if it is higher, because can me associated with severe physical and pathological changes, that influences growth, immune response and reproductive failure (97). Therefore, it is essential to consider the balance of minerals in the diets when incorporating Spirulina to ensure the optimal growth and development of broilers.
5 Conclusion
This study demonstrates that incorporating a high level of Spirulina (15%) into broiler diets for an extended period (from day 7–35), with or without enzyme supplementation, negatively impacts broiler growth performance, particularly body weight and body weight gain. The FCR remained unaffected by the inclusion of Spirulina from day 21–35. The increased digesta viscosity observed in Spirulina-fed groups appears to hinder nutrient digestibility, which could explain the compromised growth performance.
In addition, Spirulina integration enhances meat quality by reducing cholesterol content in breast meat and improving the antioxidant profile and n-3 PUFA levels in both breast and thigh meats. These changes potentially make the meat more nutritious and desirable for health-conscious consumers. The addition of commercial enzyme mixtures in this study was not effective in mitigating the increased viscosity in the digestive tract, suggesting that a combination of enzymatic and mechanical or physical pre-treatments may be necessary.
Future research should focus on exploring alternative strategies to improve the digestibility of Spirulina in broiler diets. This could include the use of different enzymatic formulations with specific activity against Spirulina proteins or combined mechanical and enzymatic pre-treatments. Additionally, long-term studies should investigate the potential health benefits of consuming meat enriched with n-3 PUFAs and antioxidants from Spirulina-fed broilers, providing a comprehensive understanding of its implications for both poultry production and human health.
Data availability statement
The raw data supporting the conclusions of this article will be made available by the authors, without undue reservation.
Ethics statement
The animal study was approved by ORBEA/ISA (approval code: 0421/000/000/2022), Instituto Superior de Agronomia, Universidade de Lisboa, Portugal. The study was conducted in accordance with the local legislation and institutional requirements.
Author contributions
MS: Formal analysis, Investigation, Writing – original draft, Writing – review & editing. MC: Formal analysis, Investigation, Methodology, Validation, Writing – original draft, Writing – review & editing. BT: Formal Analysis, Investigation, Methodology, Writing – original draft, Writing – review & editing. JPe: Formal analysis, Investigation, Methodology, Writing – original draft, Writing – review & editing. JT: Formal analysis, Investigation, Methodology, Writing – original draft, Writing – review & editing. CM: Formal analysis, Investigation, Methodology, Writing – original draft, Writing – review & editing. CA: Formal analysis, Investigation, Methodology, Writing – original draft, Writing – review & editing. VM: Formal analysis, Investigation, Methodology, Writing – original draft, Writing – review & editing. DC: Formal analysis, Investigation, Methodology, Writing – original draft, Writing – review & editing. MM: Formal analysis, Writing – original draft, Writing – review & editing. ML: Resources, Writing – original draft, Writing – review & editing. JPr: Conceptualization, Funding acquisition, Methodology, Project administration, Resources, Supervision, Validation, Writing – original draft, Writing – review & editing.
Funding
The author(s) declare that financial support was received for the research, authorship, and/or publication of this article. This research was financially supported by grants awarded by the Fundação para a Ciência e a Tecnologia (FCT, Lisbon, Portugal) to CIISA (UIDB/00276/2020), AL4AnimalS (LA/P/0059/2020), LEAF (UIDB/04129/2020), to JPe (SFRH/BPD/116816/2016), and PhD grant to MS (UI/BD/153071/2022). The project also received funding from the Portugal 2020 project (P2020/17/SI/70114/2019) and an associated researcher contract for MC.
Conflict of interest
The authors declare that the research was conducted in the absence of any commercial or financial relationships that could be construed as a potential conflict of interest.
Publisher’s note
All claims expressed in this article are solely those of the authors and do not necessarily represent those of their affiliated organizations, or those of the publisher, the editors and the reviewers. Any product that may be evaluated in this article, or claim that may be made by its manufacturer, is not guaranteed or endorsed by the publisher.
References
1. Chaves, AAM, Martins, CF, Carvalho, DFP, Ribeiro, DM, Lordelo, M, Freire, JPB, et al. A viewpoint on the use of microalgae as an alternative feedstuff in the context of pig and poultry feeding-a special emphasis on tropical regions. Trop Anim Health Prod. (2021) 53:396. doi: 10.1007/s11250-021-02800-5
2. Becker, EW . Micro-algae as a source of protein. Biotechnol Adv. (2007) 25:207–10. doi: 10.1016/j.biotechadv.2006.11.002
3. Holman, BWB, and Malau-Aduli, AEO. Spirulina as a livestock supplement and animal feed. J Anim Physiol Anim Nutr (Berl). (2013) 97:615–23. doi: 10.1111/j.1439-0396.2012.01328.x
4. Soni, RA, Sudhakar, K, and Rana, RS. Spirulina – from growth to nutritional product: a review. Trends Food Sci Technol. (2017) 69:157–71. doi: 10.1016/j.tifs.2017.09.010
5. Austic, RE, Mustafa, A, Jung, B, Gatrell, S, and Lei, XG. Potential and limitation of a new defatted diatom microalgal biomass in replacing soybean meal and corn in diets for broiler chickens. J Agric Food Chem. (2013) 61:7341–8. doi: 10.1021/jf401957z
6. Spolaore, P, Joannis-Cassan, C, Duran, E, and Isambert, A. Commercial applications of microalgae. J Biosci Bioeng. (2006) 101:87–96. doi: 10.1263/jbb.101.87
7. Swiatkiewicz, S, Arczewska-Wlosek, A, and Józefiak, D. Application of microalgae biomass in poultry nutrition. Worlds Poult Sci J. (2015) 71:663–72. doi: 10.1017/S0043933915002457
8. Madkour, FF, Kamil, AE-W, and Nasr, HS. Production and nutritive value of Spirulina platensis in reduced cost media. Egypti J Aquat Res. (2012) 38:51–7. doi: 10.1016/j.ejar.2012.09.003
9. Seyidoglu, N, Inan, S, and Aydin, C. A prominent superfood: Spirulina platensis In: N Shiomi and VY Waisundara, editors. Superfood and functional food - the development of superfoods and their roles as medicine. London: Intech Open (2017)
10. Drews, G . Fine structure and chemical composition of the cell envelopes In: NG Carr and BA Whitton, editors. The biology of blue-green algae. Los Angeles, CA: University of California (1973)
11. The, CZ . Chemicals of Spirulina In: A Vonshak , editor. Spirulina platensis Arthrospira: Physiology, cell-biology and biotechnology. Oxford: Taylor & Francis (1997)
12. Spínola, MP, Costa, MM, and Prates, JAM. Digestive constraints of Arthrospira Platensis in poultry and swine feeding. Food Secur. (2022) 11:2984. doi: 10.3390/foods11192984
13. Bortolini, DG, Maciel, GM, Fernandes, IAA, Pedro, AC, Rubio, FTV, Branco, IG, et al. Functional properties of bioactive compounds from Spirulina Spp.: Current status and future trends. Food Chem: Mol Sci. (2022) 5:100134. doi: 10.1016/j.fochms.2022.100134
14. Tounsi, L, Hentati, F, Ben Hlima, H, Barkallah, M, Smaoui, S, Fendri, I, et al. Microalgae as feedstock for bioactive polysaccharides. Int J Biol Macromol. (2022) 221:1238–50. doi: 10.1016/j.ijbiomac.2022.08.206
15. Babadzhanov, A, Abdusamatova, N, Yusupova, F, Faizullaeva, N, Mezhlumyan, L, and Malikova, M. Chemical composition of Spirulina platensis cultivated in Uzbekistan. Chem Nat Compd. (2004) 40:276–9. doi: 10.1023/B:CONC.0000039141.98247.e8
16. Howe, P, Meyer, B, Record, S, and Baghurst, K. Dietary intake of Long-chain Omega-3 polyunsaturated fatty acids: contribution of meat sources. Nutrition. (2006) 22:47–53. doi: 10.1016/j.nut.2005.05.009
17. Hynstova, V, Sterbova, D, Klejdus, B, Hedbavny, J, Huska, D, and Adam, V. Separation, identification and quantification of carotenoids and chlorophylls in dietary supplements containing chlorella vulgaris and Spirulina platensis using high performance thin layer chromatography. J Pharm Biomed Anal. (2018) 148:108–18. doi: 10.1016/j.jpba.2017.09.018
18. Wang, L, Pan, B, Sheng, J, Xu, J, and Hu, Q. Antioxidant activity of Spirulina platensis extracts by supercritical carbon dioxide extraction. Food Chem. (2007) 105:36–41. doi: 10.1016/j.foodchem.2007.03.054
19. Omar, AE, Al-Khalaifah, H, Osman, A, Gouda, A, Shalaby, S, Roushdy, EM, et al. Modulating the growth, antioxidant activity, and Immunoexpression of Proinflammatory cytokines and apoptotic proteins in broiler chickens by adding dietary Spirulina platensis Phycocyanin. Antioxidants. (2022) 11:991. doi: 10.3390/antiox11050991
20. Böcker, L, Hostettler, T, Diener, M, Eder, S, Demuth, T, Adamcik, J, et al. Time-temperature-resolved functional and structural changes of Phycocyanin extracted from Arthrospira Platensis/Spirulina. Food Chem. (2020) 316:126374. doi: 10.1016/j.foodchem.2020.126374
21. Costa, MM, Spínola, MP, and Prates, JAM. Combination of mechanical/physical pretreatments with trypsin or Pancreatin on Arthrospira Platensis protein degradation. Agriculture. (2023) 13:198. doi: 10.3390/agriculture13010198
22. Niccolai, A, Chini Zittelli, G, Rodolfi, L, Biondi, N, and Tredici, MR. Microalgae of interest as food source: biochemical composition and digestibility. Algal Res. (2019) 42:101617. doi: 10.1016/j.algal.2019.101617
23. Kos, J . Peptidases: role and function in health and disease. Int J Mol Sci. (2023) 24:7823. doi: 10.3390/ijms24097823
24. Evans, AM, Smith, DL, and Moritz, JS. Effects of algae incorporation into broiler starter diet formulations on nutrient digestibility and 3 to 21 D bird performance. J Appl Poult Res. (2015) 24:206–14. doi: 10.3382/japr/pfv027
25. Pestana, JM, Puerta, B, Santos, H, Madeira, MS, Alfaia, CM, Lopes, PA, et al. Impact of dietary incorporation of Spirulina (Arthrospira Platensis) and exogenous enzymes on broiler performance, carcass traits, and meat quality. Poult Sci. (2020) 99:2519–32. doi: 10.1016/j.psj.2019.11.069
26. Coelho, D, Lopes, PA, Cardoso, V, Ponte, P, Brás, J, Madeira, MS, et al. A two-enzyme constituted mixture to improve the degradation of Arthrospira Platensis microalga Cell Wall for Monogastric diets. J Anim Physiol Anim Nutr (Berl). (2020) 104:310–21. doi: 10.1111/jpn.13239
27. Martins, CF, Pestana Assunção, J, Ribeiro Santos, DM, Madeira, M, Alfaia, C, Lopes, P, et al. Effect of dietary inclusion of Spirulina on production performance, nutrient digestibility and meat quality traits in post-weaning piglets. J Anim Physiol Anim Nutr (Berl). (2021) 105:247–59. doi: 10.1111/jpn.13470
28. Moustafa, ES, Alsanie, W, Gaber, A, Kamel, N, Alaqil, A, and Abbas, AO. Blue-green algae (Spirulina platensis) alleviates the negative impact of heat stress on broiler production performance and redox status. Animals (Basel). (2021) 11:1243. doi: 10.3390/ani11051243
29. Choct, M . Enzymes for the feed industry: past, present and future. Worlds Poult Sci J. (2006) 62:5–16. doi: 10.1079/WPS200480
30. Slominski, BA . Recent advances in research on enzymes for poultry diets. Poult Sci. (2011) 90:2013–23. doi: 10.3382/ps.2011-01372
31. Costa, M, Fernandes, VO, Ribeiro, T, Serrano, L, Cardoso, V, Santos, H, et al. Construction of Gh16 Β-Glucanase Mini-Cellulosomes to improve the nutritive value of barley-based diets for broilers. J Agric Food Chem. (2014) 62:7496–506. doi: 10.1021/jf502157y
32. Fernandes, VO, Costa, M, Ribeiro, T, Serrano, L, Cardoso, V, Santos, H, et al. 1,3-1,4-Β-Glucanases and not 1,4-Β-Glucanases improve the nutritive value of barley-based diets for broilers. Anim Feed Sci Technol. (2016) 211:153–63. doi: 10.1016/j.anifeedsci.2015.11.007
33. Saleh, AA, Elsawee, M, Soliman, MM, Elkon, RYN, Alzawqari, MH, Shukry, M, et al. Effect of bacterial or fungal Phytase supplementation on the performance, egg quality, plasma biochemical parameters, and reproductive morphology of laying hens. Animals. (2021) 11:540. doi: 10.3390/ani11020540
34. Georgieva, V, Chobanova, S, Ganchev, G, Manolov, I, Jarkov, D, Lalev, M, et al. Effect of addition of multienzyme preparation Vemozyme®plus on productive and slaughter parameters and meat composition of broiler chickens, fed wheat-corn-soybean meal diets. Agric Sci Technol. (2010) 2:197–201.
35. Al-Marzooqi, W, and Leeson, S. Evaluation of dietary supplements of lipase, detergent, and crude porcine pancreas on fat utilization by young broiler chicks. Poult Sci. (1999) 78:1561–6. doi: 10.1093/ps/78.11.1561
36. Abdelfatah, SH, Yassin, AM, Khattab, MS, Abdel-Razek, AS, and Saad, AH. Spirulina platensis as a growth booster for broiler; insights into their nutritional, molecular, immunohistopathological, and microbiota modulating effects. BMC Vet Res. (2024) 20:11. doi: 10.1186/s12917-023-03858-z
37. Costa, MM, Spínola, MP, Tavares, B, Pestana, JM, Tavares, JC, Martins, CF, et al. Effects of high dietary inclusion of Arthrospira platensis, either extruded or supplemented with a super-dosing multi-enzyme mixture, on broiler growth performance and major meat quality parameters. BMC Vet Res. (2024) 20:176. doi: 10.1186/s12917-024-04027-6
38. Hassan, RI, Refaie, MS, RD, EL-S, Rehan, I, Zigo, F, Karaffov, V, et al. Effect of dietary microalgae (Spirulina platensis) on growth performance, ingestive behavior, hemato-biochemical parameters, and economic efficiency of Fayoumi broilers. Life. (2022) 12:1892. doi: 10.3390/life12111892
39. Alfaia, CM, Pestana, JM, Rodrigues, M, Coelho, D, Aires, MJ, Ribeiro, DM, et al. Influence of dietary chlorella vulgaris and carbohydrate-active enzymes on growth performance, meat quality and lipid composition of broiler chickens. Poult Sci. (2021) 100:926–37. doi: 10.1016/j.psj.2020.11.034
40. Costa, MM, Pestana, JM, Osório, D, Alfaia, CM, Martins, CF, Mourato, M, et al. Effect of dietary Laminaria Digitata with Carbohydrases on broiler production performance and meat quality, lipid profile, and mineral composition. Animals. (2022) 12:1007. doi: 10.3390/ani12081007
41. Council NR . Nutrient requirements of poultry. 9th ed. Washington, DC: National Academies Press (1994).
42. AOAC . Official methods of analysis. 17th ed. Washington DC: Association of Official Analytical Chemists (2000).
43. Turk, DE . The anatomy of the avian digestive tract as related to feed utilization. Poult Sci. (1982) 61:1225–44. doi: 10.3382/ps.0611225
44. Cross, RH . Training and testing of judges for sensory analysis of meat quality. Food Technol. (1978) 36:48–54.
45. Mercier, Y, Gatellier, P, and Renerre, M. Lipid and protein oxidation in vitro, and antioxidant potential in meat from Charolais cows finished on pasture or mixed diet. Meat Sci. (2004) 66:467–73. doi: 10.1016/S0309-1740(03)00135-9
46. Mestre Prates, JA, Gonçalves Quaresma, MA, Branquinho Bessa, RJ, Andrade Fontes, CMG, and Mateus Alfaia, CMP. Simultaneous Hplc quantification of Total cholesterol, tocopherols and Β-carotene in Barrosã-Pdo veal. Food Chem. (2006) 94:469–77. doi: 10.1016/j.foodchem.2005.01.021
47. Teimouri, M, Amirkolaie, AK, and Yeganeh, S. The effects of Spirulina platensis meal as a feed supplement on growth performance and pigmentation of rainbow trout (Oncorhynchus Mykiss). Aquaculture. (2013) 396-399:14–9. doi: 10.1016/j.aquaculture.2013.02.009
48. Ribeiro, DM, Scanlon, T, Kilminster, T, Martins, CF, Greeff, J, Milton, J, et al. Mineral profiling of muscle and hepatic tissues of Australian merino, Damara and Dorper lambs: effect of weight loss. J Anim Physiol Anim Nutr (Berl). (2020) 104:823–30. doi: 10.1111/jpn.13339
49. Rosenkranz, FD . Freeze Drying. West Sussex: Vacuum Products Edwards High Vacuum International (1993).
50. Folch, J, Lees, M, and Sloane Stanley, GH. A simple method for the isolation and purification of Total Lipides from animal tissues. J Biol Chem. (1957) 226:497–509. doi: 10.1016/S0021-9258(18)64849-5
51. Valente, LMP, Cabrita, ARJ, Maia, MRG, Valente, IM, Engrola, S, Fonseca, AJM, et al. Effect of double-muscling in Belgian blue young bulls on the intramuscular fatty acid composition with emphasis on conjugated linoleic acid and polyunsaturated fatty acids. Anim Sci. (2001) 73:253–60. doi: 10.1017/S1357729800058227
52. Buecker, S, Grossmann, L, Loeffler, M, Leeb, E, and Weiss, J. Thermal and acidic denaturation of Phycocyanin from Arthrospira Platensis: effects of complexation with Λ-carrageenan on blue color stability. Food Chem. (2022) 380:132157. doi: 10.1016/j.foodchem.2022.132157
53. Valente, LM, Cabrita, A, Maia, MR, Valente, I, Engrola, S, Fonseca, AJ, et al. Microalgae as feed ingredients for livestock production and aquaculture In: CM Galanakis , editor. Microalgae: Cultivation, recovery of compounds and applications. Amsterdam: Academic Press, Elvesier (2021)
54. Becker, EW . Microalgae for human and animal nutrition In: A Richmond , editor. Handbook of Microalgal Culture. Hoboken, NJ: Wiley-Blackwell (2013)
55. Johnson, IT, and Gee, JM. Gastrointestinal adaptation in response to soluble non-available polysaccharides in the rat. Br J Nutr. (1986) 55:497–505. doi: 10.1079/BJN19860057
56. Shanmugapriya, B, Babu, SS, Hariharan, T, Sivaneswaran, S, and Anusha, M. Dietary Administration of Spirulina platensis as probiotics on growth performance and histopathology in broiler chicks. Int J Recent Sci Res. (2015) 6:2650–3.
57. Bonos, E, Kasapidou, E, Kargopoulos, A, Karampampas, A, Nikolakakis, I, Christaki, E, et al. Spirulina as a functional ingredient in broiler chicken diets. S Afr J Anim Sci. (2016) 46:94–102. doi: 10.4314/sajas.v46i1.12
58. Park, J, Lee, S, and Kim, I-S. Effect of dietary Spirulina (Arthrospira) platensis on the growth performance, antioxidant enzyme activity, nutrient digestibility, Cecal microflora, excreta noxious gas emission, and breast meat quality of broiler chickens. Poult Sci. (2018) 97:2451–9. doi: 10.3382/ps/pey093
59. Ross, E, and Dominy, W. The nutritional value of dehydrated, blue-green algae (Spirulina Plantensis) for poultry. Poult Sci. (1990) 69:794–800. doi: 10.3382/ps.0690794
60. Toyomizu, M, Sato, K, Taroda, H, Kato, T, and Akiba, Y. Effects of dietary Spirulina on meat colour in muscle of broiler chickens. Br Poult Sci. (2001) 42:197–202. doi: 10.1080/00071660120048447
61. Homma, N, Nakayama, T, and Sato, A. Carotenoid pigments in blue-green algae, Spirulina platensis. Bull Nigata Prefec women college. (1973) 10:135–44.
62. Kalia, S, and Lei, XG. Dietary microalgae on poultry meat and eggs: explained versus unexplained effects. Curr Opin Biotechnol. (2022) 75:102689. doi: 10.1016/j.copbio.2022.102689
63. Venkataraman, LV, Somasekaran, T, and Becker, EW. Replacement value of blue-green alga (Spirulina platensis) for fishmeal and a vitamin-mineral premix for broiler chicks. Br Poult Sci. (1994) 35:373–81. doi: 10.1080/00071669408417702
64. Altmann, BA, Neumann, C, Velten, S, Liebert, F, and Mörlein, D. Meat quality derived from high inclusion of a Micro-alga or insect meal as an alternative protein source in poultry diets: a pilot study. Food Secur. (2018) 7:34. doi: 10.3390/foods7030034
65. Moujahed, A, Hassani, S, Zairi, S, Mahdi, B, Darej, C, and Damergi, C. Effect of dehydrated Spirulina platensis on performances and meat quality of broilers. Res Opin Anim Vet Sci. (2011) 1:505–9.
66. CAICEDO, RE, KAWASHIMA, M, and KAMIYOSHI, M. Branded quality chicken booms in Japan. Nippon Kakin Gakkaishi. (1997) 34:36–44. doi: 10.2141/jpsa.34.36
67. Castañeda, MP, Hirschler, EM, and Sams, AR. Skin pigmentation evaluation in broilers fed natural and synthetic pigments. Poult Sci. (2005) 84:143–7. doi: 10.1093/ps/84.1.143
68. Wood, JD, Enser, M, Fisher, AV, Nute, GR, Sheard, PR, Richardson, RI, et al. Fat deposition, fatty acid composition and meat quality: a review. Meat Sci. (2008) 78:343–58. doi: 10.1016/j.meatsci.2007.07.019
69. Gkarane, V, Ciulu, M, Altmann, BA, Schmitt, AO, and Mörlein, D. The effect of algae or insect supplementation as alternative protein sources on the volatile profile of chicken meat. Food Secur. (2020) 9:1235. doi: 10.3390/foods9091235
70. Neumann, C, Rothstein Née Velten, S, and Liebert, F. Improving the dietary protein quality by amino acid fortification with a high inclusion level of Micro algae (Spirulina platensis) or insect meal (Hermetia Illucens) in meat type chicken diets. Open J Anim Sci. (2018) 8:12–26. doi: 10.4236/ojas.2018.81002
71. Cortinas, L, Barroeta, A, Villaverde, C, Galobart, J, Guardiola, F, and Baucells, MD. Influence of the dietary Polyunsaturation level on chicken meat quality: lipid oxidation. Poult Sci. (2005) 84:48–55. doi: 10.1093/ps/84.1.48
72. Betti, M, Schneider, BL, Wismer, WV, Carney, VL, Zuidhof, MJ, and Renema, RA. Omega-3-enriched broiler meat: 2. Functional properties, oxidative stability, and consumer acceptance. Poult Sci. (2009) 88:1085–95. doi: 10.3382/ps.2008-00158
73. Leskanich, C, and Noble, R. Manipulation of the N-3 polyunsaturated fatty acid composition of avian eggs and meat. Worlds Poult Sci J. (1997) 53:155–83. doi: 10.1079/WPS19970015
74. O'Neill, LM, Galvin, K, Morrissey, PA, and Buckley, DJ. Comparison of effects of dietary olive oil, tallow and vitamin E on the quality of broiler meat and meat products. Br Poult Sci. (1998) 39:365–71. doi: 10.1080/00071669888917
75. Abdel-Moneim, AE, Shehata, AM, Mohamed, NG, and Elbaz, A. & Ibrahim NSSynergistic effect of Spirulina platensis and selenium nanoparticles on growth performance, serum metabolites, immune responses, and antioxidant capacity of heat-stressed broiler chickens. Biol Trace Elem Res. (2021) 200:768–79. doi: 10.1007/s12011-021-02662-w
76. Long, SF, Kang, S, Wang, QQ, Xu, YT, Pan, L, Hu, JX, et al. Dietary supplementation with Dha-rich microalgae improves performance, serum composition, carcass trait, antioxidant status, and fatty acid profile of broilers. Poult Sci. (2018) 97:1881–90. doi: 10.3382/ps/pey027
77. Ribeiro, T, Lordelo, MM, Alves, SP, Bessa, RJ, Costa, P, Lemos, JP, et al. Direct supplementation of diet is the Most efficient way of enriching broiler meat with N-3 Long-chain polyunsaturated fatty acids. Br Poult Sci. (2013) 54:753–65. doi: 10.1080/00071668.2013.841861
78. Ponte, PI, Alves, SP, Bessa, RJ, Ferreira, LM, Gama, LT, Brás, JL, et al. Influence of pasture intake on the fatty acid composition, and cholesterol, tocopherols, and Tocotrienols content in meat from free-range broilers. Poult Sci. (2008) 87:80–8. doi: 10.3382/ps.2007-00148
79. Committee, FA . Report on review of food labelling and advertising. London: Her Majesty’s Stationery Office (1990).
80. Cortinas, L, Villaverde, C, Galobart, J, Baucells, MD, Codony, R, and Barroeta, AC. Fatty acid content in chicken thigh and breast as affected by dietary Polyunsaturation level. Poult Sci. (2004) 83:1155–64. doi: 10.1093/ps/83.7.1155
81. Botsoglou, NA, Florou-Paneri, P, Christaki, E, Fletouris, DJ, and Spais, AB. Effect of dietary oregano essential oil on performance of chickens and on Iron-induced lipid oxidation of breast, thigh and abdominal fat tissues. Br Poult Sci. (2002) 43:223–30. doi: 10.1080/00071660120121436
82. Martins, S, Alfaia, C, Lopes, P, and Prates, J. Dietary sources and health implications of arachidonic acid In: JM O'Keefe , editor. Arachidonic acid-sources, biosynthesis and health effects. New York: Nova Science Publishers Inc. (2014)
83. Tokuşoglu, Ö, and üUnal, MK. Biomass nutrient profiles of three microalgae: Spirulina platensis, chlorella vulgaris, and Isochrisis Galbana. J Food Sci. (2003) 68:1144–8. doi: 10.1111/j.1365-2621.2003.tb09615.x
84. Emken, EA, Adlof, RO, and Gulley, RM. Dietary linoleic acid influences desaturation and acylation of deuterium-labeled linoleic and linolenic acids in young adult males. Biochim Biophys Acta Mol Cell Biol Lipids. (1994) 1213:277–88. doi: 10.1016/0005-2760(94)00054-9
85. López-Ferrer, S, Baucells, MD, Barroeta, AC, Galobart, J, and Grashorn, MA. N-3 enrichment of chicken meat. 2. Use of precursors of Long-chain polyunsaturated fatty acids: linseed oil. Poult Sci. (2001) 80:753–61. doi: 10.1093/ps/80.6.753
86. Griffin, BA . How relevant is the ratio of dietary N-6 to N-3 polyunsaturated fatty acids to cardiovascular disease risk? Evidence from the Optilip study. Curr Opin Lipidol. (2008) 19:57–62. doi: 10.1097/MOL.0b013e3282f2e2a8
87. Barceló-Coblijn, G, and Murphy, EJ. Alpha-linolenic acid and its conversion to longer chain N−3 fatty acids: benefits for human health and a role in maintaining tissue N−3 fatty acid levels. Prog Lipid Res. (2009) 48:355–74. doi: 10.1016/j.plipres.2009.07.002
88. Ribeiro, T, Lordelo, MM, Costa, P, Alves, SP, Benevides, WS, Bessa, RJ, et al. Effect of reduced dietary protein and supplementation with a docosahexaenoic acid product on broiler performance and meat quality. Br Poult Sci. (2014) 55:752–65. doi: 10.1080/00071668.2014.971222
89. FAO . Fats and fatty acids in human nutrition. Report of an expert consultation. Geneva: FAO Food and Nutrition Paper (2008).
90. Simopoulos, AP . The importance of the Omega-6/Omega-3 fatty acid ratio in cardiovascular disease and other chronic diseases. Exp Biol Med (Maywood). (2008) 233:674–88. doi: 10.3181/0711-MR-311
91. Burghardt, PR, Kemmerer, ES, Buck, BJ, Osetek, AJ, Yan, C, Koch, LG, et al. Dietary N-3: N-6 fatty acid ratios differentially influence hormonal signature in a rodent model of metabolic syndrome relative to healthy controls. Nutr Metab. (2010) 7:1–6. doi: 10.1186/1743-7075-7-53
92. Schaible, PJ . The minerals in poultry nutrition—a review. Poult Sci. (1941) 20:278–88. doi: 10.3382/ps.0200278
93. Liu, Y, Guo, Y, Wang, Z, and Nie, W. Effects of source and level of magnesium on catalase activity and its gene expression in livers of broiler chickens. Arch Anim Nutr. (2007) 61:292–300. doi: 10.1080/17450390701432019
94. Saris, N-EL, Mervaala, E, Karppanen, H, Khawaja, JA, and Lewenstam, A. Magnesium: an update on physiological, clinical and analytical aspects. Clin Chim Acta. (2000) 294:1–26. doi: 10.1016/S0009-8981(99)00258-2
95. Baltaci, AK, Yuce, K, and Mogulkoc, R. Zinc metabolism and Metallothioneins. Biol Trace Elem Res. (2018) 183:22–31. doi: 10.1007/s12011-017-1119-7
96. Chen, X, He, C, Zhang, K, Wang, J, Ding, X, Zeng, Q, et al. Comparison of zinc bioavailability in zinc-Glycine and zinc-methionine chelates for broilers fed with a corn-soybean meal diet. Front Physiol. (2022) 13:983954. doi: 10.3389/fphys.2022.983954
Keywords: Spirulina, peptidase, broiler chicken, growth performance, meat quality
Citation: Spínola MP, Costa MM, Tavares B, Pestana JM, Tavares JC, Martins CF, Alfaia CM, Maciel V, Carvalho DFP, Mourato MP, Lordelo MM and Prates JAM (2024) Impact of long-term feeding a high level of Spirulina combined with enzymes on growth performance, carcass traits and meat quality in broiler chickens. Front. Vet. Sci. 11:1451516. doi: 10.3389/fvets.2024.1451516
Edited by:
Valiollah Palangi, Ege University, TürkiyeReviewed by:
Ilias Giannenas, Aristotle University of Thessaloniki, GreeceCanan Bölükbaşı Aktaş, Atatürk University, Türkiye
Copyright © 2024 Spínola, Costa, Tavares, Pestana, Tavares, Martins, Alfaia, Maciel, Carvalho, Mourato, Lordelo and Prates. This is an open-access article distributed under the terms of the Creative Commons Attribution License (CC BY). The use, distribution or reproduction in other forums is permitted, provided the original author(s) and the copyright owner(s) are credited and that the original publication in this journal is cited, in accordance with accepted academic practice. No use, distribution or reproduction is permitted which does not comply with these terms.
*Correspondence: José A. M. Prates, amFwcmF0ZXNAZm12LnVsaXNib2EucHQ=
†These authors have contributed equally to this work and share first authorship