- 1REQUIMTE, Network of Chemistry and Technology, LAQV, Laboratory for Green Chemistry, ICBAS, School of Medicine and Biomedical Sciences, University of Porto, Porto, Portugal
- 2Department of Immuno-Physiology and Pharmacology, Center for Pharmacological Research and Drug Innovation (MedInUP), ICBAS, School of Medicine and Biomedical Sciences, Veterinary Hospital of the University of Porto (UPVET), University of Porto, Porto, Portugal
- 3ICBAS – School of Medicine and Biomedical Sciences, University of Porto, Porto, Portugal
- 4i3S – Instituto de Investigação e Inovação em Saúde, University of Porto, Porto, Portugal
- 5HoLMiR – Hohenheim Center for Livestock Microbiome Research, University of Hohenheim, Stuttgart, Germany
- 6Institute of Animal Science, University of Hohenheim, Stuttgart, Germany
Locally produced fish hydrolysate and oil from the agrifood sector comprises a sustainable solution both to the problem of fish waste disposal and to the petfood sector with potential benefits for the animal’s health. This study evaluated the effects of the dietary replacement of mainly imported shrimp hydrolysate (5%) and salmon oil (3%; control diet) with locally produced fish hydrolysate (5%) and oil (3.2%) obtained from fish waste (experimental diet) on systemic inflammation markers, adipokines levels, cardiac function and fecal microbiota of adult dogs. Samples and measurements were taken from a feeding trial conducted according to a crossover design with two diets (control and experimental diets), six adult Beagle dogs per diet and two periods of 6 weeks each. The experimental diet, with higher docosahexaenoic (DHA) and eicosapentaenoic (EPA) acids contents, decreased plasmatic triglycerides and the activity of angiotensin converting enzyme, also tending to decrease total cholesterol. No effects of diet were observed on serum levels of the pro-inflammatory cytokines interleukin (IL)-1β, IL-8, and IL-12/IL-23 p40, and of the serum levels of the anti-inflammatory adipokine adiponectin. Blood pressure, heart rate and echocardiographic measurements were similar between diets with the only exception of left atrial to aorta diameter ratio that was higher in dogs fed the experimental diet, but without clinical relevance. Diet did not significantly affect fecal immunoglobulin A concentration. Regarding fecal microbiome, Megasphaera was the most abundant genus, followed by Bifidobacterium, Fusobacterium, and Prevotella, being the relative abundances of Fusobacterium and Ileibacterium genera positively affected by the experimental diet. Overall, results from the performed short term trial suggest that shrimp hydrolysate and salmon oil can be replaced by protein hydrolysate and oil from fish by-products without affecting systemic inflammatory markers, cardiac structure and function, but potentially benefiting bacterial genera associated with healthy microbiome. Considering the high DHA and EPA contents and the antioxidant properties of fish oil and hydrolysate, it would be worthwhile in the future to assess their long-term effects on inflammatory markers and their role in spontaneous canine cardiac diseases and to perform metabolomic and metagenomics analysis to elucidate the relevance of microbiota changes in the gut.
1 Introduction
In recent years, several studies have explored fish by-products to produce various high-value-added products such as protein concentrates, bioactive peptides, fish oils, among others (1). These products can be used as ingredients in the production of food intended for human consumption, however the development of petfood formulations has emerged as a very promising and economically viable solution. Indeed, these protein and lipid sources are an excellent option for enriching petfood in amino acids and essential fatty acids with the advantage of the absence of anti-nutritional factors or allergenic proteins and potential benefits for the animal’s health (2, 3). The main bioactivities of fish hydrolysates reported in the literature include antioxidant, antihypertensive, anti-obesity, anti-inflammatory, anticancer, antimicrobial and neuroprotective activities (4, 5).
Fish oil can be highlighted by the long-chain polyunsaturated fatty acids (PUFA) content, namely eicosapentaenoic acid (EPA, 20:5 n-3) and docosahexaenoic acid (DHA, 22:6 n-3). These long-chain omega (n)-3 PUFA are precursors of several metabolites that act as lipid mediators and are beneficial in the prevention of certain diseases, being used in therapeutic diets (e.g., cardiovascular, renal, gastrointestinal, orthopedic and dermatological) at high doses (6–10).
In addition to the economic, nutritional and bioactivity benefits associated with the valorization of fish by-products in the petfood sector, this also makes it possible to increase the environmental sustainability of the sector. In fact, the growing number of companion animals has raised concerns about their sustainability, and the pet food industry is starting to tackle this issue particularly through finding alternative protein and energy food resources (11–13).
A recent study (14) showed that the dietary inclusion of fish hydrolysate and oil locally obtained from fish waste in substitution of shrimp hydrolysate and salmon oil mainly imported from third countries was well accepted by dogs, not affecting food intake, digestibility, or fecal characteristics, but promoting blood EPA, DHA, and omega-3 index, suggesting a potential health-promoting effect. To further gain insight into the potential functional role of these upcycled resources, the present study used samples and measurements collected during the earlier experiment (14) to evaluate effects on health parameters, including immune effector molecules, fecal microbiota, and cardiac function.
2 Materials and methods
The trial was approved by the Animal Ethics Committee of School of Medicine and Biomedical Sciences, University of Porto, licensed by the Portuguese General Directorate of Food and Veterinary Medicine (Permit N° 0421/000/000/2021), and conducted by trained scientists in laboratory animal science (FELASA, category C) in line with good animal welfare practices (European Union Directive 2010/63/EU).
2.1 Animals, diets, and experimental design
Details on animals, diets and experimental design of the trial were earlier reported (14). Briefly, 12 healthy adult Beagle dogs (2 intact males, 4 neutered males, and 6 spayed females), 5.4 ± 0.57 years old, weighing 11.8 ± 2.20 kg with a body condition score of 4.3 ± 0.69 (assessed according to a 9 point-scale; 15), and housed at the kennel of the School of Medicine and Biomedical Sciences, University of Porto, were used. A commercial diet for adult medium-size dogs (Sorgal Pet Food, Ovar., Portugal) with the inclusion of 5% shrimp hydrolysate (Symrise Aqua, Equator) and 3% salmon oil (Symrise Aqua, Norway) was used as the control diet. The experimental diet comprised the same ingredients of the control diet, with slight adjustments on wheat grain (6.0 and 7.5% for the control and experimental diet), pea concentrate (7.0 and 5.0%), and poultry fat (5.0 and 5.3%), and the replacement of 5% shrimp hydrolysate with 5% fish hydrolysate, and 3% of salmon oil with 3.2% of fish oil. Fish hydrolysate was obtained by enzymatic hydrolysis with the non-specific serine endopeptidase from Bacillus licheniformis Alcalase 2.4 L (Novozymes®, Bagsvaerd, Denmark) and fish oil were obtained from fish by-products comprising heads, tails, skin, slices, and whole fish mainly of salmon, sea bream, sea bass and red fish, and provided by a company group (Empresa Transformadora de Subprodutos Animais S.A., ETSA, Loures, Portugal) dedicated to recycling in the food sector.
A detailed characterization of both diets was earlier presented (14). Briefly, comparing to the control diet, the experimental diet presented lower crude protein (27.0 vs. 29.1 g 100 g−1 dry matter, DM), but similar amino acids profile, and higher ether extract (11.6 vs. 10.4 g 100 g−1 DM) contents with a higher percentage of n-3 PUFA (6.44 vs. 3.48 g 100 g−1 total fatty acids), particularly EPA (1.25 vs. 0.355 g 100 g−1 total fatty acids), docosapentaenoic acid (C22:5 n-3; 0.360 vs. 0.116 g 100 g−1 total fatty acids), and DHA (1.96 vs. 0.494 g 100 g−1 total fatty acids), and a lower percentage of n-6 PUFA (22.1 vs. 27.0 g 100 g−1 total fatty acids).
Before the start of the trial, the dogs were considered clinically healthy based on a comprehensive examination conducted by a veterinarian. Additionally, given the age and breed of the dogs, a baseline echocardiogram was performed, revealing thickened mitral valve leaflets in all cases, consistent with myxomatous mitral valve disease. Mild mitral valve regurgitation was observed in seven dogs, all of which showed no signs of cardiac remodeling (stage B1) (16). The severity of mitral regurgitation was assessed semiquantitatively by measuring the ratio of the mitral regurgitant maximal jet area to the left atrial area by way of color Doppler. A result of <5% was considered trace, between 5 and 20% was considered mild, between 20 and 50% was considered moderate, and >50% was considered severe (17, 18). Dogs were divided into two groups blocked for sex and received the control and experimental diets in two consecutive experimental periods of 6 weeks each following a crossover arrangement. Animals were individually fed twice a day (8:30 h and 17:00 h) with the daily ration calculated according to body condition score (15) and the ideal body weight (BW) to meet the metabolizable energy requirements (19).
2.2 Blood collection and analysis
Blood samples were collected from each dog in 1 day of the last week of each experimental period before the morning meal, from the jugular vein into EDTA BD Vacutainer (VWR International, Carnaxide, Portugal) and VACUETTE BD Vacutainer® SST™ II tubes with clot activator and separating gel (Becton, Dickinson and Company, Franklin Lakes, NJ, USA). Blood was allowed to clot for 60 min at room temperature before centrifugation at 1200⨯g for 10 min. Serum was collected, divided into aliquots, and stored at −80°C until analysis. Insulin, cholesterol LDL, cholesterol HDL, aldosterone, insulin-like growth factor (IGF-1), cytokines, leptin and adiponectin were tested in serum, whereas the other parameters analyzed were measured in EDTA plasma. A mid-volume analyzer (Cobas c501, Roche Diagnostics, Indianapolis, IN, USA) were used to analyze total protein, albumin, glucose, creatinine, urea, alanine aminotransferase, alkaline phosphatase, total cholesterol, triglycerides, and reactive C protein. Globulin concentration was determined as the difference between total protein and albumin. Troponin I, IGF-1 (Siemens Immulite 2000, Siemens Healthineers, Erlanger, Germany), insulin (Siemens Advia Centaur, Siemens Healthineers) and angiotensin II (Snibe Maglumi, Shenzhen, China) were analyzed by chemiluminescence immunoassays. Cholesterol LDL and HDL, and angiotensin converting enzyme activity were determined through molecular absorption spectrometry (Siemens Atellica, Siemens Healthineers). NT-pro-BNP was quantified by ELISA using the canine cardiopet proBNP assay (IDEXX Laboratories, Westbrook, ME, USA). Aldosterone and plasmatic renin activity were measured through radioimmunoassays (Gamma WIZARD 2470 Perkin Elmer, Waltham, MA, USA).
To evaluate the effect of diet on basal systemic inflammation, the concentrations of the pro-inflammatory cytokines tumor necrosis factor (TNF)-α, interleukin (IL)-1β, IL-8, IL-12/IL-23 p40, and interferon (IFN)-γ, and of the anti-inflammatory cytokine IL-10 were quantified in the serum using the following ELISA kits, according to the manufacturers’ instructions: IL-1β (DY3747; Canine DuoSet ELISA, R&D Systems, Oxford, UK), IL-10 (DY735; Canine DuoSet ELISA, R&D Systems), IL-12/IL-23 p40 (DY1969; Canine DuoSet ELISA, R&D Systems), TNF-α (DY1507, Canine DuoSet ELISA, R&D Systems), IFN-γ (DY781B, Canine DuoSet ELISA, R&D Systems), and IL-8 (3114-1H-20, ELISA Flex Bovine IL-8, Mabtech AB, Nacka, Sweden). The colorimetric detection was performed using a Multiskan EX microplate reader (Thermo Fisher Scientific, Carlsbad, MA, USA) using the Ascent software (Thermo Fisher Scientific). ELISA kits specific to canine adipokines were also used to analyse serum concentrations of leptin (Canine Leptin Cat. No. EZCL-31 K, Merck Millipore, Darmstadt, Germany) and adiponectin (Canine Adiponectin ELISA Kit, Cat. No. RD-ADP-c, Reddot Biotech Inc., Kelowna, Canada), following manufacturers’ instructions. For adiponectin quantification, serum samples were previously diluted 1:1000 in phosphate buffered saline (PBS) medium to be within the range of the standard curve (100–1.562 ng mL−1). For leptin quantification, 20 μL of serum samples were applied to each plate well containing 80 μL of assay buffer, as recommended by the manufacturer. A Multiskan™ FC Microplate Photometer (Thermo Fisher Scientific) with the SkanIt Software 3.1 was used to measure absorbance at 450 nm and to determine sample concentration through the four-parameter logistic curve.
2.3 Blood pressure, echocardiogram, and electrocardiogram
Measurements of blood pressure, echocardiogram and electrocardiogram were conducted on each dog on 1 day of the last week of each experimental period. All procedures were performed while the dogs were conscious and without sedation. The assessment was carried out and analyzed by a single researcher. Systolic, diastolic, and mean arterial pressures were measured using a veterinary-specific oscillometric non-invasive blood pressure system (Vet20 SunTech; SunTech Medical Inc., Morrisville, NC, USA), in accordance with the American College of Veterinary Internal Medicine (ACVIM) Consensus guidelines (20). Five readings per dog were averaged to determine the blood pressure values.
After blood pressure measurements were obtained, the dogs underwent shaving to create a window for echocardiography. Conventional echocardiography (2D, M-mode, color Doppler and spectral Doppler), with a single-lead simultaneous electrocardiogram, was conducted using an M5S phased-array transducer (1.5–4.5 MHz) and a GE Logiq S8 XDclear echocardiograph (GE HealthCare, Chicago, IL, USA), following a 15-min resting period. Data were digitally stored for subsequent measurements, adhering to the guidelines of the Echocardiography Committee of the Specialty of Cardiology of ACVIM (21). Acoustic gel was applied over the transducer and directly onto the clipped skin. To minimize variability, a single trained operator recorded three consecutive measurements for each parameter, as previously recommended (22).
For the right parasternal views, the dogs were positioned in right lateral recumbency. M-mode imaging was applied on the right parasternal short-axis view at the level of the left ventricular papillary muscles to assess the interventricular septum (IVS), left ventricle (LV) internal diameter (LVID), and LV posterior wall (LVPW) in diastole (d) and systole (s). The LVIDd was normalized to BW using the formula (23):
Fractional shortening (FS) and ejection fraction (EF) of the LV were calculated as follows (24):
The right parasternal long-axis view with 2D-guided M-mode was utilized to measure the E-point-to-septal separation interval (EPSS) in the plane of the mitral valves.
The diameters of the left atrium (LA) and aorta (Ao) were measured from the right parasternal short-axis view at the level of the Ao aligned with the commissure of the aortic non-coronary and left coronary cusps during early ventricular diastole, following established protocols which exclude the pulmonary veins (25). The LA to Ao ratio (LA/Ao) was calculated and documented.
Pulmonary flow velocities were assessed using pulsed-wave Doppler imaging from the right parasternal short-axis view. Aortic and mitral flows were evaluated with pulsed-wave Doppler from the left parasternal apical 5- and 4-chamber views, respectively. For the transmitral flow, the sample volume was positioned just above the mitral valve leaflets during diastole, and peak flow velocities during early diastole (E-wave) and atrial contraction (A-wave) were measured.
Heart rate (HR) was directly calculated from the inter-beat intervals of the electrocardiographic tracing. Stroke volume (SV) was derived as the difference between EDV and ESV, where EDV represents the end diastolic volume calculated by the formula:
and ESV denotes the end systolic volume, calculated by:
Cardiac output (CO) was determined as the product of SV and HR (24).
2.4 Fecal immunoglobulin A concentration and microbiota
In 5 days of the last week of each experimental period, total feces excreted by dogs were collected. Individual samples were weighed, mixed, subsampled at different locations and immediately frozen at −20°C throughout the collection for later analysis of immunoglobulin A (IgA) concentration and fecal microbiome. Immediately before performing these analyses, feces were thawed to allow for mixing, and composited by period and dog. Samples were stored at −20°C for a period not longer than 3 months.
For IgA analysis, fecal samples were thawed, homogenized and subjected to saline extraction, according to the method described by Peters, Calvert (26), with modifications. Briefly, 1 g of fresh fecal sample was added to 10 mL of extraction buffer (PBS containing 0.5% Tween 20, Sigma-Aldrich, St. Louis, MO, USA), thoroughly homogenized using vortex and centrifuged at 1500 × g, 5°C, during 20 min. Then, 80 μL of a 25× concentrated solution of complete™ EDTA-free Protease Inhibitor Cocktail (04693132001, Roche, Basel, Switzerland) in PBS were added to 2 mL of supernatant, homogenized using vortex and centrifuged at 15000 × g, 5°C, during 15 min. The supernatants were then aliquoted and stored at −20°C until analysis. Fecal IgA concentrations were determined using a commercial ELISA kit (Dog IgA ELISA Quantitation Set, E44-104, Bethyl Laboratories Inc., Montgomery, TX, USA), according to the manufacturer’s instructions.
Samples were diluted 1:1000 or 1:2000 in dilution buffer, according to previous optimal dilution determination, and the analysis was carried out in duplicate. The colorimetric detection was performed using a Multiskan EX microplate reader (Thermo Fisher Scientific) using the Ascent software (Thermo Fisher Scientific).
For microbiome analysis, fecal DNA was extracted with E.Z.N.A.® Stool DNA Kit (Omega Bio-tek, Inc., Norcross, GA, USA), following the supplier’s protocol. After DNA extraction, primers targeting the V4 region of the 16S rRNA gene (forward: GTGYCAGCMGCCGCGGTAA, reverse: GGACTACNVGGGTWTCTAAT) with attached adapters and barcodes were used for amplification. After amplification, samples were purified and sequenced on an Illumina Novaseq 6,000 sequencer. Raw reads were imported to Qiime2 (27). Primers/adapters were removed by the cutadapt (28). Denoising, quality filtering, merging of paired reads, and chimeras removal were done by the dada2 (29). Taxonomy classification of amplicon sequence variants (ASVs) was carried out by VSEARCH-based consensus (30) and pre-fitted sklearn-based classifiers (31) against the Silva database (v138.1, 16S 99%) (32). The reference reads were preprocessed by RESCRIPt (33). Alpha diversity was assessed by Shannon’s entropy (34) and Faith’s phylogenetic diversity (35) indices, and beta diversity by Weighted UniFrac (36) distances. Alpha diversity metrics were compared by the Wilcoxon test (37) for dependent samples, and beta diversity distances by the Adonis test (999 permutations) (38). Differentially abundant genera (only for counts of genera with relative abundance ≥1% and prevalence ≥20%) were detected by Linear discriminant analysis Effect Size (LEfSe) (39). p-values obtained from LEfSe were adjusted using the Benjamini-Hochberg procedure (40). Only features with p-adj > 0.05 and LDA > 2 were considered to be differentially abundant.
2.5 Statistical analysis
Data were analyzed using the mixed procedure of the Statistical Analysis Systems software package (SAS 2021, release 3.1.0., SAS Institute, Cary, NC, USA). The model included diet, period, and dietary sequence as fixed effects and animal within dietary sequence as random effect. The level of significance was set at p < 0.05, and a trend was considered for p < 0.10. Dietary sequence did not affect any measured parameters, except for serum insulin concentration and systolic arterial pressure.
3 Results
3.1 Clinical blood chemistry
The experimental diet decreased plasmatic triglycerides (p = 0.008) and the activity of angiotensin converting enzyme (p = 0.013), also tending to decrease total cholesterol (p = 0.062; Table 1). No diet effects were observed in the other blood parameters analyzed (Table 1).
3.2 Serum cytokine and adipokine concentrations
Diet did not significantly affect serum levels of the pro-inflammatory cytokines IL-1β (p = 0.117), IL-8 (p = 0.960), and IL-12/IL-23 p40 (p = 0.332), and the serum levels of the anti-inflammatory adipokine adiponectin (p = 0.398; Table 2). Concentrations of IL-10, TNF-α, and IFN-γ were below the detection levels (31.2 pg. mL−1, 15.6 pg. mL−1, and 31.2 pg. mL−1, respectively) for most of the animals, regardless of the diet (data not shown). Moreover, serum levels of the pro-inflammatory adipokine leptin were also below detection level (0.3438 ng mL−1) for all dog samples analysed, although ELISA quality controls 1 and 2 were within the expected range (2.52 and 13.52 ng mL−1, respectively).
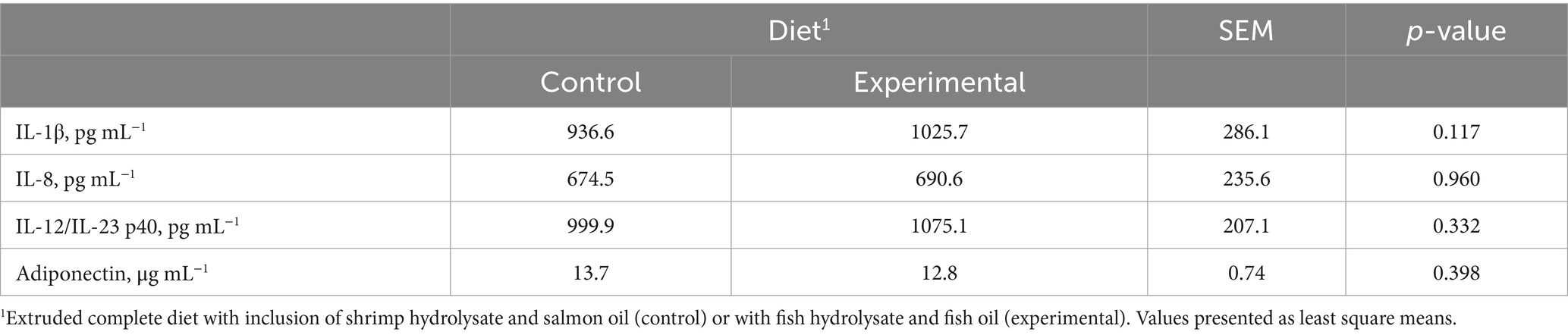
Table 2. Effect of the control and experimental diets on serum cytokine and adipokine concentrations.
3.3 Blood pressure, electrocardiogram, and echocardiogram
The electrocardiographic tracing acquired during the echocardiographic examination revealed sinus rhythm in all dogs and experimental conditions. Blood pressure values and heart rate did not exhibit any difference between the control and experimental diets with the only exception of systolic arterial pressure that tended (p = 0.097) to be higher in dogs fed the experimental diet (Table 3).
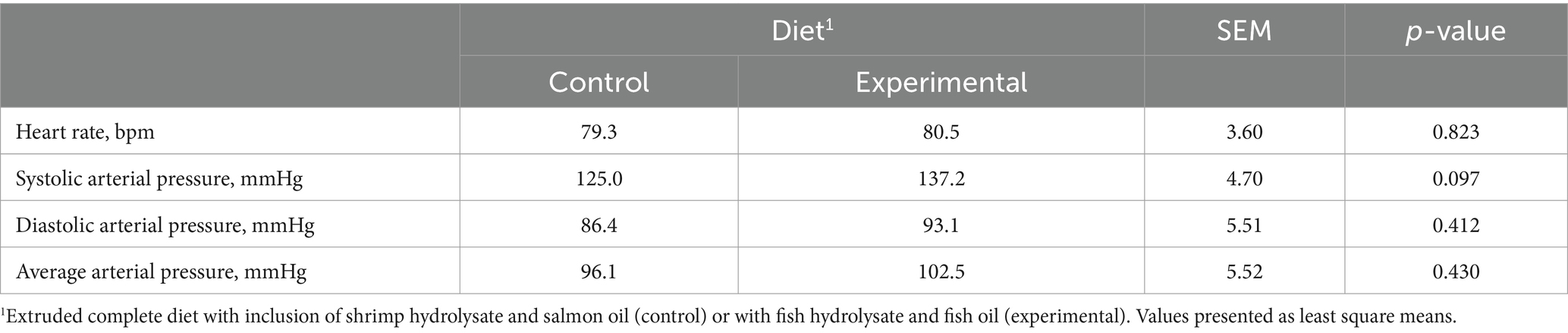
Table 3. Effect of the control and experimental diets on heart rate, systolic, diastolic, and average arterial pressures.
Regarding echocardiographic assessment, the LA/Ao ratio in dogs following the experimental diet showed a significant increase (p = 0.029) compared to the control diet, with no other significant differences being observed in the other echocardiographic measurements (Table 4).
3.4 Fecal immunoglobulin A concentration and microbiota
Diet did not significantly affect fecal IgA concentrations that averaged 5.31 and 7.37 mg g−1 of dry feces (SEM = 1.496) for the control and experimental diet, respectively (p = 0.200).
No significant differences (p = 0.155) in beta diversity (expressed by Weighted UniFrac) between the control and experimental diets were detected when tested with Adonis (Figure 1A). Similarly, diet (Figure 1B) did not significantly affect alpha diversity metrics (Shannon entropy and Faith PD) of dogs’ fecal microbiome, although the Shannon entropy had a tendency to be higher in the experimental diet (p = 0.064).
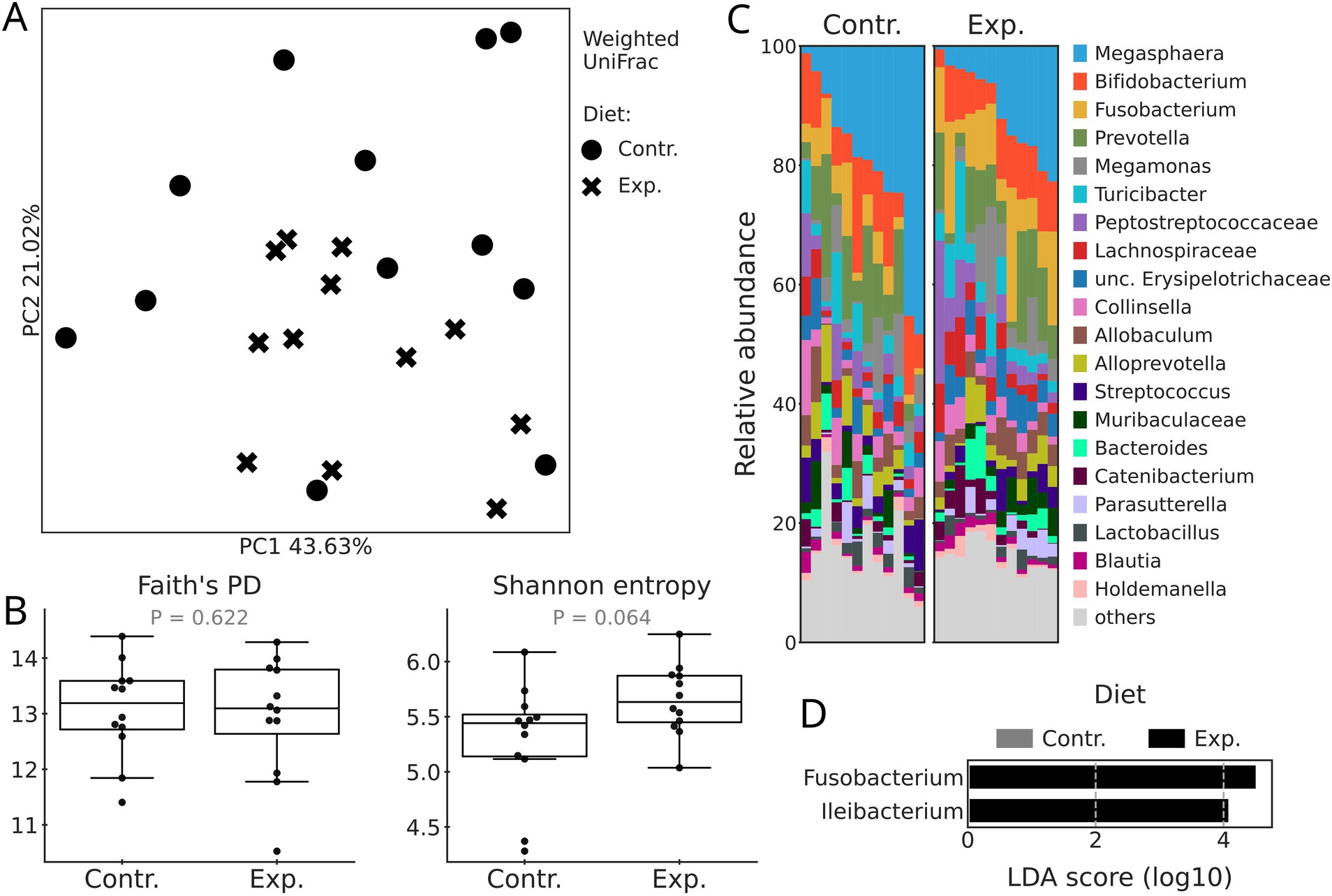
Figure 1. Microbiome diversity and composition of dogs fed with the control (Contr.) and experimental (Exp.) diets. (A) PCoA plots based on Weighted UniFrac distances. Diets are differentiated by the shape of the marker. (B) Boxplots based on Shannon entropy and Faith’s phylogenetic diversity. (C) Taxonomy barplots by diet at the genus level. If genus level was not assigned, the last available taxonomy rank was used for the label. (D) Differentially abundant genera between diets according to the LEfSe test (p-adj < 0.05, LDA > 2).
In both control and experimental diets, Megasphaera was the most abundant genus, followed by Bifidobacterium, Fusobacterium, and Prevotella (Figure 1C). A differential abundance test (LEfSe) showed that the relative abundances of only two genera, Fusobacterium and Ileibacterium were greater in the experimental diet (Figure 1D).
4 Discussion
Fish hydrolysate and oil obtained from the local agrifood sector contributes both for the problem of fish waste disposal and to the sustainability of the petfood sector. The present study evaluated the effects of the dietary replacement of shrimp hydrolysate and salmon oil with fish hydrolysate and oil on systemic inflammation markers, adipokine levels, cardiac function and fecal microbiota of adult dogs from a feeding trial earlier reported (14).
The clinical blood chemistry parameters evaluated were all within the normal ranges for healthy canines. The tendency for a decreased total cholesterol and a significant decrease of triglycerides observed with the experimental diet could be mainly attributed to its higher content of DHA and EPA as verified in previous studies (41, 42). Although not clearly understood, the mechanisms of action of omega-3 PUFA might be attributed, among others, to the regulation of transcription factors that reduce lipogenesis, increased ß-oxidation and stimulation of the activity of the lipoprotein lipase (43–45).
Leptin, a pro-inflammatory adipokine mainly secreted by adipose tissue and that positively correlates with body fat content (46, 47), was below detection level in all dog samples analysed, although ELISA quality controls were within the expected range. Similarly, it was shown by others using the same commercial ELISA kit used here that in dogs with ideal weight the levels of leptin were undetectable in the majority of the animals, in contrast with obese animals in which leptin levels were detectable (48). The levels of the adipokine adiponectin, that can have anti-inflammatory properties (49), were detectable in all animals within the range reported by others for healthy dogs (50). Decreased adiponectin serum levels in dogs have been associated with obesity (51, 52). During this study no changes in adiponectin levels were observed, which is in accordance with no detected changes in BW.
Protein hydrolysate and oil from fish by-products did not significantly influence basal levels of inflammation, which is in accordance with the similar levels of C-reactive protein detected in the serum of dogs under control and experimental diets. A high variability in the serum levels of the pro-inflammatory cytokines IL-1β, IL-8 and IL-12/IL-23 p40 was observed among the different dogs, regardless of diet, while IL-10, TNF-α and IFN-γ were below the detection limits for most animals. Previous research has reported variations in the levels of different cytokines among individuals, irrespective of breed, age and sex, being the serum levels of the cytokines IL-10, TNF-α and IFN-γ usually reported to be low or undetectable (53–56). Increased serum levels of IL-8 and IL-1β have been associated with both acute and chronic inflammation originated from diverse pathological conditions (53, 57–59), including heart disease (52, 60). The lack of differences in the levels of these particular cytokines in the present study are in line with the absence of significant alterations in hematological and cardiac parameters.
Hydrolysed proteins have been used in diets for dogs with adverse food reactions or gastrointestinal disorders (61). In several studies, the inclusion of protein hydrolysates did not significantly affect serum or plasma cytokine levels (62, 63). However, inclusion of black soldier fly larvae and microalgae-like Schizochytrium protein hydrolysates in the diet decreased serum IL-8, suggesting a potential anti-inflammatory role for these particular hydrolysates in dogs (64). Discrepant reported results on dietary inclusion of protein hydrolysates in dogs may be explained by many variables, including the hydrolysate origin, the percentage of inclusion and the duration of the feeding trial.
In the present study, both diets were fed to healthy dogs and contain oil from fish sources, rich in EPA and DHA (14). The role of EPA and DHA in inflammation is pleiotropic by influencing several biological processes, such as cell membrane composition and fluidity, production of prostaglandins E (PGE), leukotrienes B (LTB), resolvins and protectins, and signaling pathways involved in the expression of pro-inflammatory genes (65, 66). The replacement of shrimp hydrolysate and salmon oil by fish hydrolysate and oil led to an increase of EPA and DHA contents resulting in significantly higher concentrations of these PUFA in the red blood cells of dogs fed the experimental diet (2.02 vs. 1.41 g 100 g−1 of EPA and 1.75 vs. 1.17 g 100 g−1 of DHA for experimental and control diet, respectively) (14). In a large human community-based cohort, the EPA and DHA concentrations of red blood cells were inversely correlated with several markers of inflammation (67). In that line, several authors have suggested that EPA and DHA-enriched diets could be used as part of anti-inflammatory treatments in dogs with chronic inflammatory diseases. As such, the introduction of fish oil in diets for dogs of different breeds led to a decrease in the cellular production of the pro-inflammatory mediators PGE2 or LTB4 (68, 69) and in decreased production of IL-1β by mononuclear blood cells (6). The present study shows that shrimp hydrolysate and salmon oil can be replaced by protein hydrolysate and oil from fish by-products without affecting systemic inflammatory markers. Still, as differences were observed in EPA and DHA content of red blood cells of dogs fed the experimental diet, further studies using longer periods of dietary supplementation are necessary to assess long-term effects of these food resources.
The present study demonstrates that an experimental diet containing fish hydrolysate and oil has no significant impact in most of the parameters related to cardiac structure and function, as indicated by the results obtained from cardiac biomarkers (NT-proBNP and troponin I), blood pressure, and echocardiographic assessments; also, it is important to refer that all parameters felt within the reference range for a healthy adult dog (20, 70, 71). While a significant increase in the echocardiographic LA/Ao ratio, a marker of left atrial enlargement, was observed in the experimental diet group, all values remained within the reference range for dogs (72). These findings suggest that the diet under investigation does not impact cardiac remodeling; however, it is important to note that the assessment was conducted over a short duration. Therefore, it is crucial to evaluate the diet’s long-term effects on cardiac health. In myxomatous mitral valve disease, the most prevalent naturally occurring heart condition in dogs, left atrial enlargement serves as the most reliable independent indicator of cardiac disease progression. This disease is associated with changes in energy metabolism, oxidative stress, and inflammation. Interestingly, a prior study demonstrated that a diet supplemented with a blend including medium-chain triglycerides, EPA and DHA, magnesium, taurine and vitamin E, reduced systolic arterial blood pressure and left atrial enlargement in dogs with early preclinical myxomatous mitral valve disease (stage B2 and C), attributed to various properties, including its antioxidant and anti-inflammatory effects (73). Considering the antioxidant properties of fish hydrolysate and oil, it would be worthwhile in the future to assess its role in advanced stages of heart disease, with secondary cardiac remodeling and/or heart failure. Lower levels of serum adiponectin have been reported in dogs with valve disease (74, 75), in accordance with studies in mice and humans that show a role of this adipokine in the cardiovascular system (76, 77). According to no major changes observed in cardiac parameters we did not observe significantly changes in the adiponectin serum levels in animals fed the experimental diet.
In addition, the experimental diet decreased the angiotensin converting enzyme activity, suggesting a potential role of this diet on mitigating the renin-angiotensin-aldosterone system, a neurohormonal system that function to preserve intravascular volume and perfusion pressure in situations of decreased cardiac output (78). Moreover, considering the recommended dose of 40 mg kg−1 BW of EPA and 25 mg kg−1 DHA to achieve a cardioprotective effect (6), the intake of EPA and particularly of DHA with the experimental diet (18 mg kg−1 BW of EPA and 28 mg kg−1 BW of DHA) might benefit the cardiac function of dogs.
The experimental diet containing fish hydrolysate and oil had no significant effect on the fecal IgA and the composition of the canine fecal microbiome compared to a commonly used commercial diet including shrimp hydrolysate and salmon oil. Secretory IgA, the most abundant immunoglobulin in mucous secretions, plays a significant role in mucosal immunity and promotes gut health (79). Gut secretory IgA is an important modulator of gut microbiota (80), and the reverse also occurs, being well documented in how gut microbiota drives IgA production (81, 82). Only two genera, Fusobacterium and Ileibacterium increased their relative abundance when the experimental diet was applied. Care should be taken in driving strong conclusions of these results as the authors are aware of the limitations of using LEfSe in such analysis (83). Being Fusobacterium a butyrate producer that utilizes lysine degradation pathways to produce butyrate from protein sources, an increase in its abundance has been reported in dogs fed high protein diets (84–86). However, in the present study, the experimental diet presented slightly lower protein and lysine content than the control diet, with no differences being observed on butyrate concentration among diets (14). Fusobacterium, despite being associated with inflammatory bowel disease (87) and colorectal cancer (88) in humans, is attributed to the healthy microbiome in dogs (89). Not much information is available on the role that Ileibacterium plays in dogs’ health but in mice, this genus was associated with reduced risks of inflammation and dysbiosis (90, 91). The results demonstrate that the diet containing fish hydrolysate and oil can be used as the substitution for shrimp hydrolysate without drastic changes in dogs’ fecal microbiome, while potentially propagating bacterial genera associated with the healthy canine microbiome. It is known that the preprocessing storage temperatures may affect the gut microbiome composition in dogs (92). However, despite the samples in this study were not stored at the optimal temperature, they follow optimized standardized protocols from sample collection, storage and processing, providing that all samples were treated in the same conditions, the findings were not affected by it. However, additional metabolomic and metagenomics analysis could be useful in elucidating the relevance and role of these microbiota changes in the gut.
5 Conclusion
The present short term study shows that replacing imported shrimp hydrolysate and salmon oil with locally produced fish oil and hydrolysate from the agrifood industry did not affect systemic inflammatory markers, cardiac structure and function, but potentially benefit bacterial genera associated with healthy canine microbiome. Future studies should address the long-term effects of these food resources and their role in spontaneous canine cardiac diseases.
Data availability statement
The datasets presented in this study can be found in online repositories. The names of the repository/repositories and accession number(s) can be found at: https://www.ebi.ac.uk/ena, PRJEB74498.
Ethics statement
The animal study was approved by the Animal Ethics Committee of School of Medicine and Biomedical Sciences, University of Porto, licensed by the Portuguese General Directorate of Food and Veterinary Medicine (Permit Nº 0421/000/000/2021). The study was conducted in accordance with the local legislation and institutional requirements.
Author contributions
ARJC: Conceptualization, Formal analysis, Funding acquisition, Investigation, Project administration, Resources, Supervision, Writing – original draft, Writing – review & editing. CB: Formal analysis, Investigation, Writing – review & editing. APF-S: Investigation, Writing – review & editing. AC: Formal analysis, Investigation, Writing – review & editing. LT: Formal analysis, Investigation, Writing – review & editing. MM: Writing – review & editing. MV: Writing – review & editing. TY: Formal analysis, Investigation, Writing – review & editing. AC-S: Formal analysis, Investigation, Writing – review & editing. AF: Conceptualization, Formal analysis, Funding acquisition, Investigation, Resources, Supervision, Writing – original draft, Writing – review & editing.
Funding
The author(s) declare that financial support was received for the research, authorship, and/or publication of this article. This work received financial support from the Portuguese Foundation for Science and Technology (FCT/MCTES; UIDB/50006/2020 DOI: 10.54499/UIDB/50006/2020) through national funds. The authors acknowledge project HealthyPetFood (POCI-01-0247-FEDER-047073) supported by Portugal 2020 program through the European Regional Development Fund. The contract of MRGM was funded through DL 57/2016–Norma transitória (SFRH/BPD/70176/2010) by FCT. The authors also acknowledge the support of the HighPerformance and Cloud Computing Group at the Zentrum für Datenverarbeitung of the University of Tübingen, the state of Baden-Württemberg through bwHPC, and the German Research Foundation (DFG) (Grant no. INST 37/935-1FUGG).
Acknowledgments
The authors acknowledge, from the School of Medicine and Biomedical Sciences, University of Porto, Portugal, Sílvia Azevedo for the technical assistance and analytical determinations, Margarida Guedes and Patrícia Alves for the technical assistance throughout in vivo trials collection, and the staff of the kennel for assistance and maintenance of the dogs. The authors are thankful to Tiago Aires from Sorgal, Sociedade de Óleos e Rações, S.A., for supplying the diets and to André Almeida and Ana Rosa from Indústria Transformadora de Subprodutos, S.A. for supplying the fish hydrolysate and oil.
Conflict of interest
The authors declare that the research was conducted in the absence of any commercial or financial relationships that could be construed as a potential conflict of interest.
The author(s) declared that they were an editorial board member of Frontiers, at the time of submission. This had no impact on the peer review process and the final decision.
Publisher’s note
All claims expressed in this article are solely those of the authors and do not necessarily represent those of their affiliated organizations, or those of the publisher, the editors and the reviewers. Any product that may be evaluated in this article, or claim that may be made by its manufacturer, is not guaranteed or endorsed by the publisher.
References
1. Ghaly, AE, Ramakrishnan, VV, Brooks, MS, Budge, SM, and Dave, D. Fish processing wastes as a potential source of proteins, amino acids and oils: a critical review. J Microb Bioch Tech. (2013) 5:107–29. doi: 10.4172/1948-5948.1000110
2. Araujo, J, Sica, P, Costa, C, and Márquez, MC. Enzymatic hydrolysis of fish waste as an alternative to produce high value-added products. Waste Biomass Valor. (2021) 12:847–55. doi: 10.1007/s12649-020-01029-x
3. Rodrigues, M, Rosa, A, Almeida, A, Martins, R, Ribeiro, T, Pintado, M, et al. Omega-3 fatty acids from fish by-products: innovative extraction and application in food and feed. Food Bioprod Process. (2024) 145:32–41. doi: 10.1016/j.fbp.2024.02.007
4. Gao, R, Yu, Q, Shen, Y, Chu, Q, Chen, G, Fen, S, et al. Production, bioactive properties, and potential applications of fish protein hydrolysates: developments and challenges. Trends Food Sci Technol. (2021) 110:687–99. doi: 10.1016/j.tifs.2021.02.031
5. Heffernan, S, Giblin, L, and O’Brien, N. Assessment of the biological activity of fish muscle protein hydrolysates using in vitro model systems. Food Chem. (2021) 359:129852. doi: 10.1016/j.foodchem.2021.129852
6. Freeman, LM, Rush, JE, Kehayias, JJ, Ross, JN Jr, Meydani, SN, Brown, DJ, et al. Nutritional alterations and the effect of fish oil supplementation in dogs with heart failure. J Vet Intern Med. (1998) 12:440–8. doi: 10.1111/j.1939-1676.1998.tb02148.x
7. Neumayer, HH, Heinrich, M, Schmissas, M, Haller, H, Wagner, K, and Luft, FC. Amelioration of ischemic acute renal failure by dietary fish oil administration in conscious dogs. J Am Soc Nephrol. (1992) 3:1312–20. doi: 10.1681/ASN.V361312
8. Roush, JK, Cross, AR, Renberg, WC, Dodd, CE, Sixby, KA, Fritsch, DA, et al. Evaluation of the effects of dietary supplementation with fish oil omega-3 fatty acids on weight bearing in dogs with osteoarthritis. J Am Vet Med Assoc. (2010) 236:67–73. doi: 10.2460/javma.236.1.67
9. Blaskovic, M, Rosenkrantz, W, Neuber, A, Sauter-Louis, C, and Mueller, RS. The effect of a spot-on formulation containing polyunsaturated fatty acids and essential oils on dogs with atopic dermatitis. Vet J. (2014) 199:39–43. doi: 10.1016/j.tvjl.2013.10.024
10. Simpson, KW, Miller, ML, Loftus, JP, Rishniw, M, Frederick, CE, and Wakshlag, JJ. Randomized controlled trial of hydrolyzed fish diets in dogs with chronic enteropathy. J Vet Intern Med. (2023) 37:2334–43. doi: 10.1111/jvim.16844
11. Okin, GS. Environmental impacts of food consumption by dogs and cats. PLoS One. (2017) 12:e0181301. doi: 10.1371/journal.pone.0181301
12. Swanson, KS, Carter, RA, Yount, TP, Aretz, J, and Buff, PR. Nutritional sustainability of pet foods. Adv Nutr. (2013) 4:141–50. doi: 10.3945/an.112.003335
13. Pedrinelli, V, Teixeira, FA, Queiroz, MR, and Brunetto, MA. Environmental impact of diets for dogs and cats. Sci Rep. (2022) 12:18510. doi: 10.1038/s41598-022-22631-0
14. Cabrita, ARJ, Maia, MRG, Alves, AP, Aires, T, Rosa, A, Almeida, A, et al. Protein hydrolysate and oil from fish waste reveal potential as dog food ingredients. Front Vet Sci. (2024) 11:1372023. doi: 10.3389/fvets.2024.1372023
15. Laflamme, D. Development and validation of a body condition score system for dogs. Canine Practice. (1997) 22:10–5.
16. Keene, BW, Atkins, CE, Bonagura, JD, Fox, PR, Häggström, J, Fuentes, VL, et al. ACVIM consensus guidelines for the diagnosis and treatment of myxomatous mitral valve disease in dogs. J Vet Intern Med. (2019) 33:1127–40. doi: 10.1111/jvim.15488
17. Liu, B, Leach, SB, Pan, W, Zheng, F, Jia, L, Zhou, X, et al. Preliminary outcome of a novel edge-to-edge closure device to manage mitral regurgitation in dogs. Front Vet Sci. (2020) 7:597879. doi: 10.3389/fvets.2020.597879
18. Chetboul, V, and Tissier, R. Echocardiographic assessment of canine degenerative mitral valve disease. J Vet Cardiol Off J Eur Soc. (2012) 14:127–48. doi: 10.1016/j.jvc.2011.11.005
19. FEDIAF. Nutritional guidelines for complete and complementary pet food for cats and dogs. Bruxelles: FEDIAF (2021).
20. Acierno, MJ, Brown, S, Coleman, AE, Jepson, RE, Papich, M, Stepien, RL, et al. ACVIM consensus statement: guidelines for the identification, evaluation, and management of systemic hypertension in dogs and cats. J Vet Intern Med. (2018) 32:1803–22. doi: 10.1111/jvim.15331
21. Thomas, WP, Gaber, CE, Jacobs, GJ, Kaplan, PM, Lombard, CW, Moise, NS, et al. Recommendations for standards in transthoracic two-dimensional echocardiography in the dog and cat. Echocardiography Committee of the Specialty of cardiology, American College of Veterinary Internal Medicine. J Vet Intern Med. (1993) 7:247–52. doi: 10.1111/j.1939-1676.1993.tb01015.x
22. Gottdiener, JS, Bednarz, J, Devereux, R, Gardin, J, Klein, A, Manning, WJ, et al. American Society of Echocardiography recommendations for use of echocardiography in clinical trials. J Am Soc Echocardiogr. (2004) 17:1086–119. doi: 10.1016/j.echo.2004.07.013
23. Cornell, CC, Kittleson, MD, Della Torre, P, Häggström, J, Lombard, CW, Pedersen, HD, et al. Allometric scaling of M-mode cardiac measurements in normal adult dogs. J Vet Intern Med. (2004) 18:311–21. doi: 10.1111/j.1939-1676.2004.tb02551.x
24. Chetboul, V, Bussadori, C, and Madron, E. Global left ventricle systolic function assessment In: E Madron, editor. Clinical echocardiography of the dog and cat. Amsterdam: Elsevier (2012). 111–25.
25. Hansson, K, Häggström, J, Kvart, C, and Lord, P. Left atrial to aortic root indices using two-dimensional and M-mode echocardiography in cavalier king Charles spaniels with and without left atrial enlargement. Vet Radiol Ultrasound. (2002) 43:568–75. doi: 10.1111/j.1740-8261.2002.tb01051.x
26. Peters, IR, Calvert, EL, Hall, EJ, and Day, MJ. Measurement of immunoglobulin concentrations in the feces of healthy dogs. Clin Diagn Lab Immunol. (2004) 11:841–8. doi: 10.1128/CDLI.11.5.841-848.2004
27. Bolyen, E, Rideout, JR, Dillon, MR, Bokulich, NA, Abnet, CC, Al-Ghalith, GA, et al. Reproducible, interactive, scalable and extensible microbiome data science using QIIME 2. Nat Biotechnol. (2019) 37:852–7. doi: 10.1038/s41587-019-0209-9
28. Martin, M. Cutadapt removes adapter sequences from high-throughput sequencing reads. EMBnet J. (2011) 17:200. doi: 10.14806/ej.17.1.200
29. Callahan, BJ, McMurdie, PJ, Rosen, MJ, Han, AW, Johnson, AJA, and Holmes, SP. DADA2: high-resolution sample inference from Illumina amplicon data. Nat Methods. (2016) 13:581–3. doi: 10.1038/nmeth.3869
30. Rognes, T, Flouri, T, Nichols, B, Quince, C, and Mahé, F. VSEARCH: a versatile open source tool for metagenomics. PeerJ. (2016) 4:e2584. doi: 10.7717/peerj.2584
31. Pedregosa, F, Varoquaux, G, Gramfort, A, Michel, V, Thirion, B, Grisel, O, et al. Scikit-learn: machine learning in Python. J Mach Learn Res. (2011) 12:2825–30.
32. Quast, C, Pruesse, E, Yilmaz, P, Gerken, J, Schweer, T, Yarza, P, et al. The SILVA ribosomal RNA gene database project: improved data processing and web-based tools. Nucleic Acids Res. (2013) 41:D590–6. doi: 10.1093/nar/gks1219
33. Robeson, MS II, O’Rourke, DR, Kaehler, BD, Ziemski, M, Dillon, MR, Foster, JT, et al. RESCRIPt: Reproducible sequence taxonomy reference database management. PLoS Comput Biol. (2021) 17:e1009581. doi: 10.1371/journal.pcbi.1009581
34. Shannon, CE. A mathematical theory of communication. Bell Syst Tech J. (1948) 27:379–423. doi: 10.1002/j.1538-7305.1948.tb01338.x
35. Faith, D. Conservation evaluation and phylogenetic diversity. Biol Conserv. (1992) 61:1–10. doi: 10.1016/0006-3207(92)91201-3
36. Lozupone, C, Lladser, M, Knights, D, Stombaugh, J, and Knight, R. UniFrac: an effective distance metric for microbial community comparison. ISME J. (2011) 5:169–72. doi: 10.1038/ismej.2010.133
37. Wilcoxon, F. Individual comparisons by ranking methods. Biom Bull. (1945) 1:80. doi: 10.2307/3001968
38. Anderson, MJ. A new method for non-parametric multivariate analysis of variance. Austral Ecol. (2001) 26:32–46. doi: 10.1111/j.1442-9993.2001.01070.pp.x
39. Segata, N, Izard, J, Waldron, L, Gevers, D, Miropolsky, L, Garrett, WS, et al. Metagenomic biomarker discovery and explanation. Genome Biol. (2011) 12:R60. doi: 10.1186/gb-2011-12-6-r60
40. Benjamini, Y, and Hochberg, Y. Controlling the false discovery rate: a practical and powerful approach to multiple testing. J Royal Stat Soc. (1995) 57:289–300. doi: 10.1111/j.2517-6161.1995.tb02031.x
41. de Albuquerque, P, De Marco, V, Vendramini, THA, Amaral, AR, Catanozi, S, Santana, KG, et al. Supplementation of omega-3 and dietary factors can influence the cholesterolemia and triglyceridemia in hyperlipidemic schnauzer dogs: a preliminary report. PLoS One. (2021) 16:e0258058. doi: 10.1371/journal.pone.0258058
42. Jackson, MI, and Jewell, DE. Feeding of fish oil and medium-chain triglycerides to canines impacts circulating structural and energetic lipids, endocannabinoids, and non-lipid metabolite profiles. Frontiers in veterinary. Front Vet Sci. (2023) 10:1168703. doi: 10.3389/fvets.2023.1168703
43. Toth, PP, Dayspring, TD, and Pokrywka, GS. Drug therapy for hypertriglyceridemia: fibrates and omega-3 fatty acids. Curr Atheroscler Rep. (2009) 11:71–9. doi: 10.1007/s11883-009-0012-z
44. Watts, GF, and Karpe, F. Triglycerides and atherogenic dyslipidaemia: extending treatment beyond statins in the high-risk cardiovascular patient. Heart. (2011) 97:350–6. doi: 10.1136/hrt.2010.204990
45. Bornfeldt, KE. Triglyceride lowering by omega-3 fatty acids: a mechanism mediated by N-acyl taurines. J Clin Invest. (2021) 131:558. doi: 10.1172/JCI147558
46. Cortese, L, Terrazzano, G, and Pelagalli, A. Leptin and immunological profile in obesity and its associated diseases in dogs. Int J Mol Sci. (2019) 20:392. doi: 10.3390/ijms20102392
47. Kiernan, K, and MacIver, NJ. The role of the adipokine leptin in immune cell function in health and disease. Front Immunol. (2020) 11:622468. doi: 10.3389/fimmu.2020.622468
48. Tvarijonaviciute, A, Ceron, JJ, and Martínez-Subiela, S. Assessment of five ELISAs for measurement of leptin concentrations in dogs. Am J Vet Res. (2011) 72:169–73. doi: 10.2460/ajvr.72.2.169
49. Feijóo-Bandín, S, Aragón-Herrera, A, Moraña-Fernández, S, Anido-Varela, L, Tarazón, E, Roselló-Lletí, E, et al. Adipokines and inflammation: focus on cardiovascular diseases. Int J Mol Sci. (2020) 21:711. doi: 10.3390/ijms21207711
50. Damoiseaux, C, Merveille, AC, Krafft, E, Da Costa, AM, Gomart, S, Jespers, P, et al. Effect of physiological determinants and cardiac disease on plasma adiponectin concentrations in dogs. J Vet Intern Med. (2014) 28:1738–45. doi: 10.1111/jvim.12433
51. Muñoz-Prieto, A, Cerón, JJ, Martínez-Subiela, S, Mrljak, V, and Tvarijonaviciute, A. A systematic review and meta-analysis of serum adiponectin measurements in the framework of dog obesity. Animals. (2020) 10:1650. doi: 10.3390/ani10091650
52. Tropf, M, Nelson, OL, Lee, PM, and Weng, HY. Cardiac and metabolic variables in obese dogs. J Vet Intern Med. (2017) 31:1000–7. doi: 10.1111/jvim.14775
53. Franco-Martinez, L, Muñoz-Prieto, A, Busato, F, Karveliene, B, Stadaliene, I, Ceron, JJ, et al. Evaluation of the presence of gingivitis as confounding factor in assessing inflammatory status in serum and saliva of dogs with diabetes mellitus. BMC Vet Res. (2024) 20:116. doi: 10.1186/s12917-024-03962-8
54. Barthélemy, A, Rannou, B, Forterre, M, Verwaerde, P, Bonnet-Garin, JM, Pouzot-Nevoret, C, et al. Differences between coagulation and cytokine profiles in dogs of different ages. Vet J. (2015) 205:410–2. doi: 10.1016/j.tvjl.2015.05.012
55. Zois, NE, Moesgaard, SG, Kjelgaard-Hansen, M, Rasmussen, CE, Falk, T, Fossing, C, et al. Circulating cytokine concentrations in dogs with different degrees of myxomatous mitral valve disease. Vet J. (2012) 192:106–11. doi: 10.1016/j.tvjl.2011.05.009
56. Calvalido, J, Wood, GA, Mutsaers, AJ, Wood, D, Sears, W, and Woods, JP. Comparison of serum cytokine levels between dogs with multicentric lymphoma and healthy dogs. Vet Immunol Immunopathol. (2016) 182:106–14. doi: 10.1016/j.vetimm.2016.10.009
57. Prachar, C, Kaup, F, and Neumann, S. Interleukin-1 beta (IL-1β) in the peripheral blood of dogs as a possible marker for the detection of early stages of inflammation. Open J Vet Med. (2013) 3:302–8. doi: 10.4236/ojvm.2013.37049
58. Choi, SW, Kim, YH, Kang, MS, Jeong, Y, Ahn, JO, Choi, JH, et al. Serum concentration of inflammatory cytokines in dogs with suspected acute pancreatitis. Vet Sci. (2021) 8:051. doi: 10.3390/vetsci8030051
59. Safra, N, Hitchens, PL, Maverakis, E, Mitra, A, Korff, C, Johnson, E, et al. Serum levels of innate immunity cytokines are elevated in dogs with metaphyseal osteopathy (hypertrophic osteodytrophy) during active disease and remission. Vet Immunol Immunopathol. (2016) 179:32–5. doi: 10.1016/j.vetimm.2016.08.003
60. Piantedosi, D, Musco, N, Palatucci, AT, Carriero, F, Rubino, V, Pizzo, F, et al. Pro-inflammatory and immunological profile of dogs with myxomatous mitral valve disease. Vet Sci. (2022) 9:326. doi: 10.3390/vetsci9070326
61. Cave, NJ. Hydrolyzed protein diets for dogs and cats. Vet Clin Small Anim Prac. (2006) 36:1251–68. doi: 10.1016/j.cvsm.2006.08.008
62. Zinn, KE, Hernot, DC, Fastinger, ND, Karr-Lilienthal, LK, Bechtel, PJ, Swanson, KS, et al. Fish protein substrates can substitute effectively for poultry by-product meal when incorporated in high-quality senior dog diets. J Anim Physiol Anim Nutr. (2009) 93:447–55. doi: 10.1111/j.1439-0396.2008.00826.x
63. Pinto, CFD, de Oliveira, BB, Bortolo, M, Guldenpfennig, R, Marx, FR, and Trevizan, L. Hydrolyzed chicken liver used as single source of animal protein in diet and its effect on cytokines, immunoglobulins, and fecal microbiota profile of adult dogs. PLoS One. (2022) 17:e0271932. doi: 10.1371/journal.pone.0271932
64. Wei, Y, Xue, L, Ma, D, Weng, Y, Liu, M, Li, L, et al. The effect of dietary protein hydrolysate from black soldier fly larvae and Schizochytrium on palatability, nutrient metabolites and health status in beagle dogs. Meta. (2024) 14:165. doi: 10.3390/metabo14030165
65. Innes, JK, and Calder, PC. Omega-6 fatty acids and inflammation. Prostaglandins Leukot Essent Fatty Acids. (2018) 132:41–8. doi: 10.1016/j.plefa.2018.03.004
66. Serhan, CN, and Levy, BD. Resolvins in inflammation: emergence of the pro-resolving superfamily of mediators. J Clin Invest. (2018) 128:2657–69. doi: 10.1172/JCI97943
67. Fontes, JD, Rahman, F, Lacey, S, Larson, MG, Vasan, RS, Benjamin, EJ, et al. Red blood cell fatty acids and biomarkers of inflammation: a cross-sectional study in a community-based cohort. Atherosclerosis. (2015) 240:431–6. doi: 10.1016/j.atherosclerosis.2015.03.043
68. Wander, RC, Hall, JA, Gradin, JL, Du, SH, and Jewell, DE. The ratio of dietary (n-6) to (n-3) fatty acids influences immune system function, eicosanoid metabolism, lipid peroxidation and vitamin E status in aged dogs. J Nutr. (1997) 127:1198–205. doi: 10.1093/jn/127.6.1198
69. Waldron, MK, Hannah, SS, and Bauer, JE. Plasma phospholipid fatty acid and ex vivo neutrophil responses are differentially altered in dogs fed fish- and linseed-oil containing diets at the same n-6:n-3 fatty acid ratio. Lipids. (2012) 47:425–34. doi: 10.1007/s11745-012-3652-7
70. de Madron, É. 2 - Normal echocardiographic values: TM, 2D, and doppler spectral modes In: V Chetboul and C Bussadori, editors. Clinical echocardiography of the dog and cat. St. Louis, MI: Elsevier Masson (2015). 21–37.
71. Oyama, MA. Using cardiac biomarkers in veterinary practice. Vet Clin North Am Small Anim Pract. (2013) 43:1261–72, vi. doi: 10.1016/j.cvsm.2013.07.010
72. Rishniw, M, and Erb, HN. Evaluation of four 2-dimensional echocardiographic methods of assessing left atrial size in dogs. J Vet Intern Med. (2000) 14:429–35.
73. Li, Q, Heaney, A, Langenfeld-McCoy, N, Boler, BV, and Laflamme, DP. Dietary intervention reduces left atrial enlargement in dogs with early preclinical myxomatous mitral valve disease: a blinded randomized controlled study in 36 dogs. BMC Vet Res. (2019) 15:425. doi: 10.1186/s12917-019-2169-1
74. Kuleš, J, Bilić, P, Horvatić, A, Kovačević, A, Guillemin, N, Ljubić, BB, et al. Serum proteome profiling in canine chronic valve disease using a TMT-based quantitative proteomics approach. J Proteome. (2020) 223:103825. doi: 10.1016/j.jprot.2020.103825
75. Kim, HS, Kang, JH, Jeung, EB, and Yang, MP. Serum concentrations of leptin and adiponectin in dogs with myxomatous mitral valve disease. J Vet Intern Med. (2016) 30:1589–600. doi: 10.1111/jvim.14570
76. Peng, J, Chen, Q, and Wu, C. The role of adiponectin in cardiovascular disease. Cardiovas Pathol Off J Soc Cardiovas Pathol. (2023) 64:107514. doi: 10.1016/j.carpath.2022.107514
77. Zhao, S, Kusminski, CM, and Scherer, PE. Adiponectin, leptin and cardiovascular disorders. Circ Res. (2021) 128:136–49. doi: 10.1161/CIRCRESAHA.120.314458
78. Sotillo, S, Ward, JL, Guillot, E, Domenig, O, Yuan, L, Smith, JS, et al. Dose-response of benazepril on biomarkers of the classical and alternative pathways of the renin-angiotensin-aldosterone system in dogs. Sci Rep. (2023) 13:2684. doi: 10.1038/s41598-023-29771-x
79. Cerutti, A, Chen, K, and Chorny, A. Immunoglobulin responses at the mucosal interface. Annu Rev Immunol. (2011) 29:273–93. doi: 10.1146/annurev-immunol-031210-101317
80. Nakajima, A, Vogelzang, A, Maruya, M, Miyajima, M, Murata, M, Son, A, et al. IgA regulates the composition and metabolic function of gut microbiota by promoting symbiosis between bacteria. J Exp Med. (2018) 215:2019–34. doi: 10.1084/jem.20180427
81. Yang, C, Mogno, I, Contijoch, EJ, Borgerding, JN, Aggarwala, V, Li, Z, et al. Fecal IgA levels are determined by strain-level differences in Bacteroides ovatus and are modifiable by gut microbiota manipulation. Cell Host Microbe. (2020) 27:467–75. doi: 10.1016/j.chom.2020.01.016
82. Bunker, JJ, Drees, C, Watson, AR, Plunkett, CH, Nagler, CR, Schneewind, O, et al. B cell superantigens in the human intestinal microbiota. Sci Transl Med. (2019) 11:9356. doi: 10.1126/scitranslmed.aau9356
83. Nearing, JT, Douglas, GM, Hayes, MG, MacDonald, J, Desai, DK, Allward, N, et al. Microbiome differential abundance methods produce different results across 38 datasets. Nat Commun. (2022) 13:342. doi: 10.1038/s41467-022-28034-z
84. Martínez-López, LM, Pepper, A, Pilla, R, Woodward, AP, Suchodolski, JS, and Mansfield, C. Effect of sequentially fed high protein, hydrolyzed protein, and high fiber diets on the fecal microbiota of healthy dogs: a cross-over study. Anim Microb. (2021) 3:42. doi: 10.1186/s42523-021-00101-8
85. Bermingham, EN, Maclean, P, Thomas, DG, Cave, NJ, and Young, W. Key bacterial families (Clostridiaceae, Erysipelotrichaceae and Bacteroidaceae) are related to the digestion of protein and energy in dogs. PeerJ. (2017) 5:e3019. doi: 10.7717/peerj.3019
86. Phimister, FD, Anderson, RC, Thomas, DG, Farquhar, MJ, Maclean, P, Jauregui, R, et al. Using meta-analysis to understand the impacts of dietary protein and fat content on the composition of fecal microbiota of domestic dogs (Canis lupus familiaris): a pilot study. Microbiology. (2024) 13:e1404. doi: 10.1002/mbo3.1404
87. Gevers, D, Kugathasan, S, Denson, LA, Vázquez-Baeza, Y, Van Treuren, W, Ren, B, et al. The treatment-naive microbiome in new-onset Crohn’s disease. Cell Host Microbe. (2014) 15:382–92. doi: 10.1016/j.chom.2014.02.005
88. Castellarin, M, Warren, R, Freeman, J, Dreolini, L, Krzywinski, M, Strauss, J, et al. Fusobacterium nucleatum infection is prevalent in human colorectal carcinoma. Genome Res. (2012) 22:299–306. doi: 10.1101/gr.126516.111
89. Vázquez-Baeza, Y, Hyde, E, Suchodolski, J, and Knight, R. Dog and human inflammatory bowel disease rely on overlapping yet distinct dysbiosis networks. Nat Microbiol. (2016) 1:16177. doi: 10.1038/nmicrobiol.2016.177
90. Liu, Y, Luo, L, Luo, Y, Zhang, J, Wang, X, Sun, K, et al. Prebiotic properties of green and dark tea contribute to protective effects in chemical-induced colitis in mice: a fecal microbiota transplantation study. J Agric Food Chem. (2020) 68:6368–80. doi: 10.1021/acs.jafc.0c02336
91. Truong, V-L, and Jeong, W-S. Antioxidant and anti-inflammatory roles of tea polyphenols in inflammatory bowel diseases. Food Sci Human Wellness. (2022) 11:502–11. doi: 10.1016/j.fshw.2021.12.008
Keywords: cardiac evaluation, fecal microbiota, fish waste, functionality, immune response, pet food
Citation: Cabrita ARJ, Barroso C, Fontes-Sousa AP, Correia A, Teixeira L, Maia MRG, Vilanova M, Yergaliyev T, Camarinha-Silva A and Fonseca AJM (2024) Assessing functional properties of diet protein hydrolysate and oil from fish waste on canine immune parameters, cardiac biomarkers, and fecal microbiota. Front. Vet. Sci. 11:1449141. doi: 10.3389/fvets.2024.1449141
Edited by:
Patricia Massae Oba, University of Illinois at Urbana-Champaign, United StatesReviewed by:
Ananda Felix, Federal University of Paraná, BrazilVivian Pedrinelli, NutricareVet, Brazil
Copyright © 2024 Cabrita, Barroso, Fontes-Sousa, Correia, Teixeira, Maia, Vilanova, Yergaliyev, Camarinha-Silva and Fonseca. This is an open-access article distributed under the terms of the Creative Commons Attribution License (CC BY). The use, distribution or reproduction in other forums is permitted, provided the original author(s) and the copyright owner(s) are credited and that the original publication in this journal is cited, in accordance with accepted academic practice. No use, distribution or reproduction is permitted which does not comply with these terms.
*Correspondence: Ana R. J. Cabrita, YXJjYWJyaXRhQGljYmFzLnVwLnB0
†Present address: Margarida R. G. Maia, Associated Laboratory TERRA, Instituto Superior de Agronomia, LEAF - Linking Landscape, Environment, Agriculture and Food Research Center, Universidade de Lisboa, Lisboa, Portugal