- 1Department Biological Safety, German Federal Institute for Risk Assessment (BfR), Berlin, Germany
- 2Department Safety in the Food Chain, German Federal Institute for Risk Assessment (BfR), Berlin, Germany
- 3Department of Animal Sciences, University of Göttingen, Göttingen, Germany
- 4TUM School of Life Sciences, Animal Nutrition and Metabolism,Technical University of Munich, Freising-Weihenstephan, Germany
Introduction: Data regarding the occurrence and virulence of Staphylococcus (S.) aureus in wild living animals is rare. However, S. aureus may carry a multitude of virulence factors and express resistance to several antimicrobial substances. Handling game meat may thus lead to serious infections or food poisoning. The aim of this study was to provide insights into the occurrence and characteristics of S. aureus in wild ungulates from Brandenburg, Germany.
Methods: Nasal swabs of externally healthy-looking wild boars, roe, fallow and red deer were collected in hunts during season 2021/2022 and analyzed for S. aureus by selective enrichment. Species were determined using matrix assisted laser desorption ionization mass spectrometry and tested for phenotypic antimicrobial resistance. Whole-genome sequencing was conducted for genotyping, determination of virulence associated genes and analysis of phylogenetic relationships.
Results: S. aureus were recovered from approximately 8% of nasal swabs. However, the strains were only obtained from the sampled wild ruminants. S. aureus isolates were associated with sequence types (ST) 1, ST30, ST133, ST425, ST582 and ST6238. Isolates of ST1 clustered closely together in the phylogenetic analysis. Genes encoding staphylococcal enterotoxin (SE) or SE-like (SEl) were found in 14/17 isolates. In particular, a seh gene was present in 12/17 isolates. Moreover, two isolates harbored a multiplicity of genes encoding SE or SEl. In addition, the toxic shock syndrome toxin encoding tst gene was detected in one isolate. This isolate was resistant to penicillin and cefoxitin and accordingly harbored the blaZ gene.
Discussion: Wild ungulates intended for human consumption may carry potentially virulent S. aureus. In one case, the close phylogenetic relationship of S. aureus isolates indicates a possible intraspecific spread within a common territory. However, for others, the origin or the spread pattern can only be inferred. Handling of animals or their carcasses might contribute to staphylococcal infections in humans. Moreover, food poisoning due to SE producing strains may occur, if recommended hygiene practices are not applied during processing of game meat.
1 Introduction
Staphylococcus (S.) aureus is a coccoid formed and Gram-positive bacterium that inhabits a wide variety of organs and tissues, such as the skin and mucosa of animals and humans. S. aureus may carry a large number of virulence associated genes; however, animals and humans may be colonized without showing disease symptoms. The ability to evade the human immune system as well as its capacity to express leucocidins and further toxins makes it to one of the most harmful bacteria for human and animal health. For instance, infections with S. aureus may lead to wound infections in all mammalian species, mastitis in dairy herds, skeletal disorders in poultry, or even a sepsis in humans (1–3). Moreover, due to the zoonotic potential of S. aureus, a transmission from animals to humans or vice versa may occur. In particular, the production of staphylococcal enterotoxin (SE) by S. aureus is a significant threat with regard to food poisoning. Thereby, the classical SE types A to E have the highest potential to cause food poisoning (4). Ingestion of SE contaminated food may lead to nausea, vomiting, diarrhea or abdominal pain. Besides SEs, the toxic shock syndrome toxin is of high importance for human health when being infected with S. aureus (5). Concerning antimicrobial resistance, methicillin-resistant S. aureus (MRSA) is of great concern for animal and human health, since treatment options may fail during an infection with MRSA. MRSA are resistant to virtual all beta-lactam antibiotics and often carry resistances to more antimicrobial classes. The beta-lactam antibiotic resistance in MRSA is most commonly transmitted by the mecA gene, however, in wildlife species such as wild hedgehogs, rabbits, and rodents, the mecC gene is also frequently found (6–9).
Data regarding S. aureus in wildlife is still rare in comparison to livestock or companion animals. S. aureus was found in a multiplicity of wild animal species such as rat, hedgehog, beaver, squirrel, fox, wild boar, deer or even bat, elephant and dolphin (10, 11). Also different MRSA clones were detected in a multitude of wildlife samples, expressing the potential of wild animals to act as reservoir and sentinel for MRSA carriage (12). Several studies have been conducted with regard to the genotypic characterization of S. aureus in wild animals. Thereby, a large variety of sequence types and clonal complexes was detected (10, 13). Moreover, S. aureus from wildlife and game meat was shown to exhibit the potential to produce virulence factors such as Panton-Valentine leucocidin (14, 15) or staphylococcal enterotoxins (16, 17). According to the European Union One Health 2022 Zoonoses Report, S. aureus enterotoxins were the second most frequently reported bacterial toxin-producing agents in foodborne outbreaks, and first for the number of hospitalizations and deaths in the year 2022 (18).
In Germany, game meat from wild boar, fallow, red and roe deer is frequently consumed and its handling may possess a possible risk for S. aureus transmission to humans. However, only few studies were conducted so far with respect to the S. aureus occurrence in wild animals that are hunted for human consumption. Therefore, the objective of this study was to investigate the occurrence of S. aureus in wild ungulates hunted in the German Federal State of Brandenburg. Furthermore, the presence of genes associated with virulence as well as antimicrobial resistance (AMR) was determined by whole-genome sequencing and bioinformatics.
2 Materials and methods
2.1 Sample collection and isolation
Sampling activities were conducted within a framework agreement involving the German Federal Institute for Risk Assessment (BfR) and the German Institute for Federal Real Estate (BImA). For details regarding the sampling strategy, please refer to Maaz et al. (19). In total, 23 driven hunts were visited in 16 different districts during the hunting season 2021/2022 from October 2021 to January 2022 in Brandenburg, Germany. Hunting was conducted several times per week. Two hundred and fifteen nasal swab samples of wild boars (n = 78), roe (n = 84), fallow (n = 38) and red deer (n = 15) were collected (Table 1). The samples were transported to the laboratory on day of collection and stored at 4°C until further analysis. Swab samples were analyzed for S. aureus by selective enrichment using the ISO 6888-3:2003 method (20), which is in particular applied when expecting low numbers of S. aureus. This protocol includes an initial enrichment step in modified Giolitti-Cantoni broth (Merck Millipore, USA) with addition of a 1% potassium tellurite solution (Merck Millipore, USA) under anaerobic conditions and subsequent streaking on Baird-Parker agar plates (Oxoid, UK) including egg yolk tellurite emulsion (Oxoid, UK). According to differences in colony morphologies, a loop of one to three presumptive S. aureus colonies was transferred to sheep blood agar plates (Oxoid, UK) and incubated for 20–24 h at 37°C. Species were determined using matrix assisted laser desorption ionization mass spectrometry (MALDI-TOF-MS; Bruker, USA). Colonies were spotted on the MALDI-TOF-MS target via direct transfer method and covered with 0.8 μl of α-Cyano-4-hydroxycinnamic acid (Bruker Scientific LLC). According to the manufacturer’s recommendations, the threshold score for acceptable S. aureus species identification was ≥2.000. The reference database was provided by Bruker (MBT-BDAL-8468).
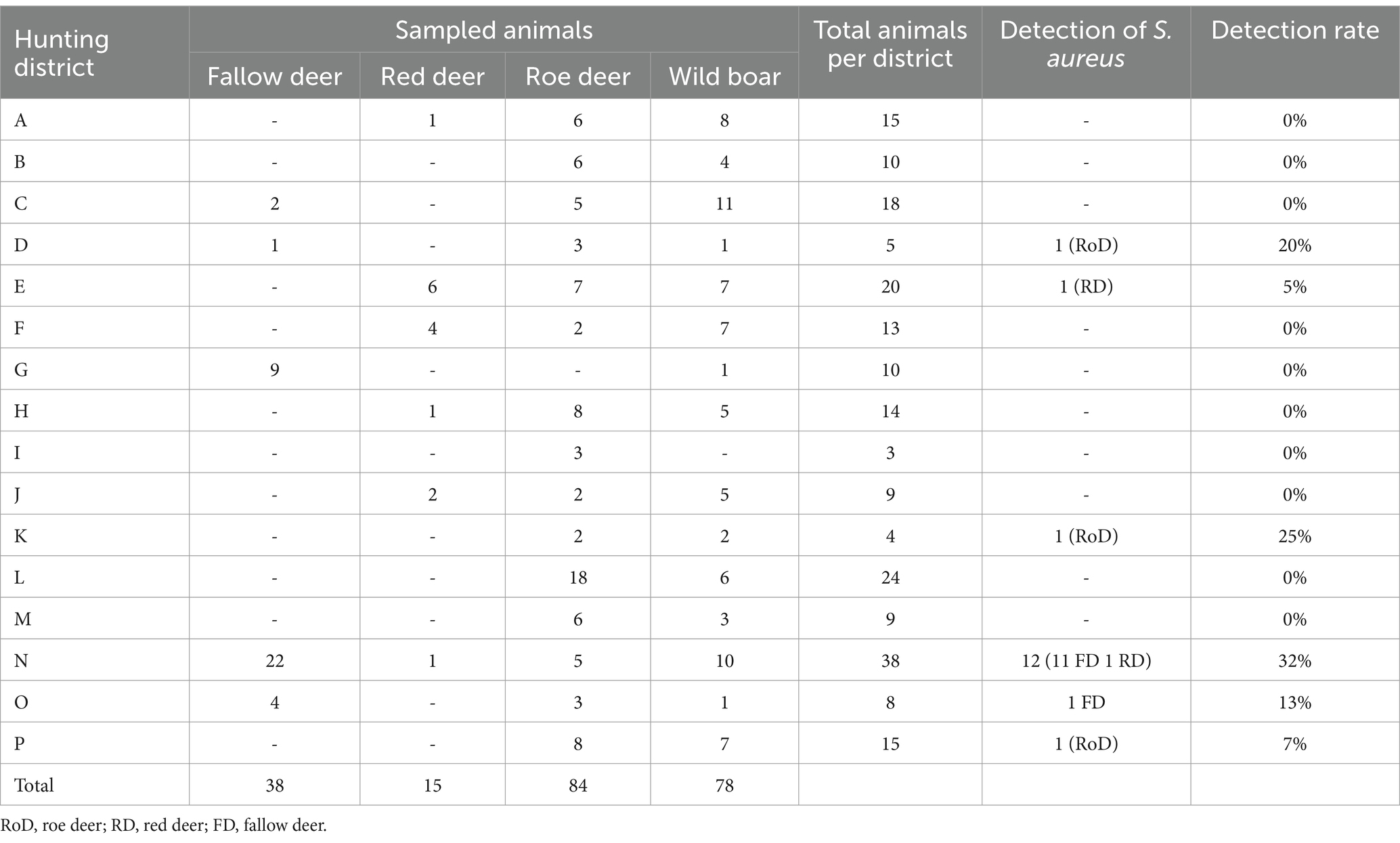
Table 1. Number of sampled animals and detection rate of S. aureus with respect to the hunting districts in Brandenburg, Germany.
2.2 Whole-genome sequencing and bioinformatic analysis
All isolates that were identified by MALDI-TOF-MS as being S. aureus were inoculated in 5 ml brain-heart-infusion broth and incubated at 37°C for 24 h. DNA of 1 ml culture was extracted using the Qiagen DNeasy Blood and Tissue Kit (Qiagen, Germany) according to the manufacturer’s protocol modified by adding 10 μl lysostaphin (Sigma Aldrich, USA) to the lysis buffer. The DNA library was prepared using an Illumina DNA Prep kit (Illumina Inc., USA) and the 150 bp paired-end sequencing run was performed on an Illumina NextSeq 500 instrument. Raw Illumina reads were trimmed and de novo assembled with the in-house developed AQUAMIS pipeline (21). Bacterial characterization such as determination of sequence types and analysis of AMR and virulence associated genes was done with the in-house developed Bakcharak pipeline.1 Phylogenetic analyses using core genome multi-locus-sequence-typing (cgMLST) as well spa type determination were conducted using Ridom SeqSphere+ version 7.0.4 according to the S. aureus cgMLST scheme comparing 1861 alleles (22). Spa types were additionally verified by PCR analysis and subsequent sanger sequencing (Eurofins, Luxembourg).
2.3 Antimicrobial susceptibility testing
Antimicrobial susceptibility testing was performed at the National Reference Laboratory for Antimicrobial Resistance at the BfR by broth microdilution according to international guidelines [ISO 20776-1:2006 (23)/CLSI M07 Ed 12 (24)]. It was carried out using a commercial standardized antibiotic panel (Sensititre EUST scheme from Thermo Fisher Scientific, UK) that is recommended by the European Food Safety Authority (EFSA) for resistance monitoring in MRSA from livestock and food (25). For interpretation of the minimum inhibitory concentration (MIC) of the individual strains, the following EUCAST ECOFFs for S. aureus (accessed 2024-06-11) were used: Penicillin >0.125 mg/L; Cefoxitin >4 mg/L; Chloramphenicol >16 mg/L; Ciprofloxacin >2 mg/L; Clindamycin >0.25 mg/L; Erythromycin >1 mg/L; Fusidic acid >0.5 mg/L; Gentamicin >2 mg/L; Kanamycin >8 mg/L; Linezolid >4 mg/L; Mupirocin >1 mg/L; Rifampin >0.016 mg/L; Streptomycin >16 mg/L; Quinupristin-Dalfopristin >1 mg/L; Tetracycline >1 mg/L; Tiamulin >2 mg/L; Trimethoprim >2 mg/L; Vancomycin >2 mg/L. For quality control of resistance testing, the S. aureus strain ATCC 29213 and Enterococcus faecalis strain ATCC 29212 were used.
3 Results
3.1 Occurrence and genotypic characteristics of S. aureus in wild ungulates
S. aureus was recovered from 17/215 nasal swabs in six of the 16 hunting districts (D, E, K, N, O, P) by selective enrichment. This corresponds to a total detection rate of approximately 8% among the sampled artiodactyl species. The positive rate per district was 32% (N), 25% (K), 20% (D), 13% (O), 7% (P) and 5% (E; Table 1). Most isolates were retrieved from fallow deer (n = 12; 32% positive rate), whereas fewer isolates were retrieved from red (n = 2; 13% positive rate) and roe deer (n = 3; 4% positive rate; Table 1). S. aureus was not found in nasal swabs from wild boars in the respective hunting season. S. aureus isolates were associated with spa types t127 (n = 10), t1872 (n = 1), t693 (n = 2), t3043 (n = 1), t3583 (n = 1), t085 (n = 1), and t472 (n = 1). Sequence types (ST) 1 (n = 12), ST30 (n = 1), ST133 (n = 1), ST425 (n = 1), ST582 (n = 1), and ST6238 (n = 1) were determined (Table 1).
3.2 Phylogenetic relationships
The cgMLST analysis showed that t127-ST1 isolates of fallow deer (No. 3, 4, 5, 6, 7, 9, 10, 12, 14) obtained from hunting district N generally had less than 150 allelic differences (AD). The isolates from other districts were, despite of one case, considerably different based on genomic analysis. As shown in Figure 1, a cluster was built of five closely related t127-ST1 S. aureus isolates (No. 3, 5, 6, 10, 12) from district N that only differed in a maximum of seven alleles. In addition, two other closely related clusters consisting of two t127-ST1 (No. 9, 14) and two t693-ST1 isolates (No. 8, 11), respectively, appeared. The additional two other t127-ST1 isolates from fallow deer (No. 4, 7) and district N were only distantly related to the t127-ST1 cluster isolates with AD >100. Interestingly, the only isolate of red deer (No. 13) obtained in hunting district N was associated to ST6238 as well as spa type t3043 and considerably differed from the other t127-ST1 isolates of fallow deer. In contrast and despite the geographical distance between hunting districts E and N (linear distance ca. 75 km), the t127-ST1 isolate (No. 1) of red deer from hunting district E had comparably lower AD to one isolate of fallow deer from hunting district N (No. 4; Figure 1).
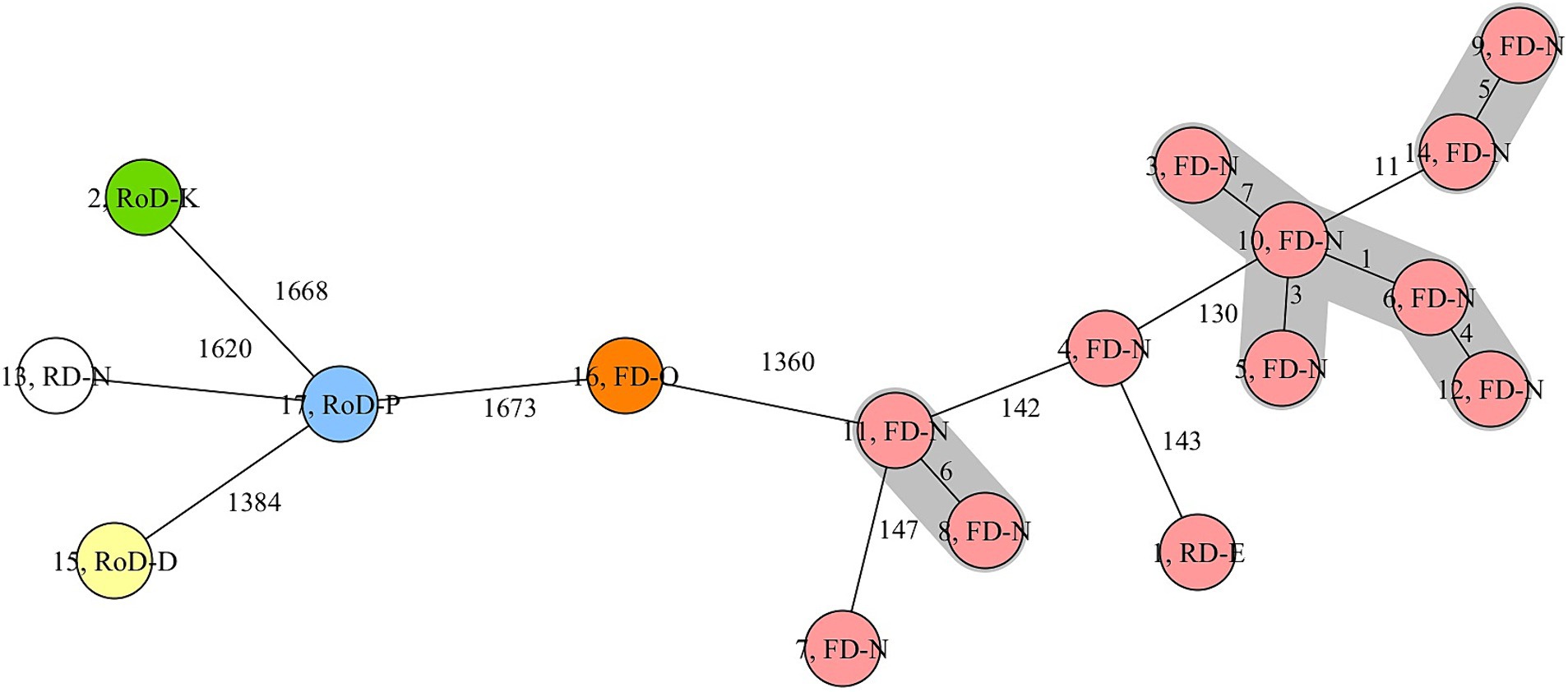
Figure 1. Phylogenetic analysis (core genome multilocus sequence typing) of S. aureus isolates with various sequence types (colours) from different wild ungulates (1–17) and districts (letters). FD, fallow deer; RD, red deer; RoD, roe deer. Red, ST1; Orange, ST582; Blue, ST425; Green, ST30; Yellow, ST133; White, ST6238.
3.3 Antimicrobial resistance and virulence associated genes
The number of AMR genes was rather low (Table 2). Four isolates carried the fosfomycin resistance transmitting fosB gene. One of these isolates additionally harbored the blaZ gene and expressed phenotypic resistance to penicillin (MIC >2 mg/L) and cefoxitin (8 mg/L) in the antimicrobial susceptibility testing. The isolates were susceptible to all other antimicrobial substances according to the EUCAST ECOFFs mentioned in section 2.3. This t1872-ST30 isolate was obtained from a roe deer fawn (<1 year old) sampled in a hunting district located at the periphery of the metropolitan area of Berlin-Potsdam. A high number of virulence associated genes was detected in each isolate. Genes encoding SE or SE-like (SEl) were found in 14/17 isolates (Table 2). In particular, an seh gene was present in all t127-ST1 isolates disregarding the hunting district of origin. The t1872-ST30 isolate harbored genes encoding SEA, SEG, SEI, SEM, SEN, SEO and SElU and the t3043-ST6238 isolate of the hunting district C carried genes for SEI, SEM, SEN, SEO and SElU. Besides SE and SE-like genes, the toxic shock syndrome toxin (TSST) encoding tst gene was detected in the t1872-ST30 isolate (Table 2). Moreover, the t1872-ST30 isolate carried the immune evasion cluster genes scn, chp and sak.
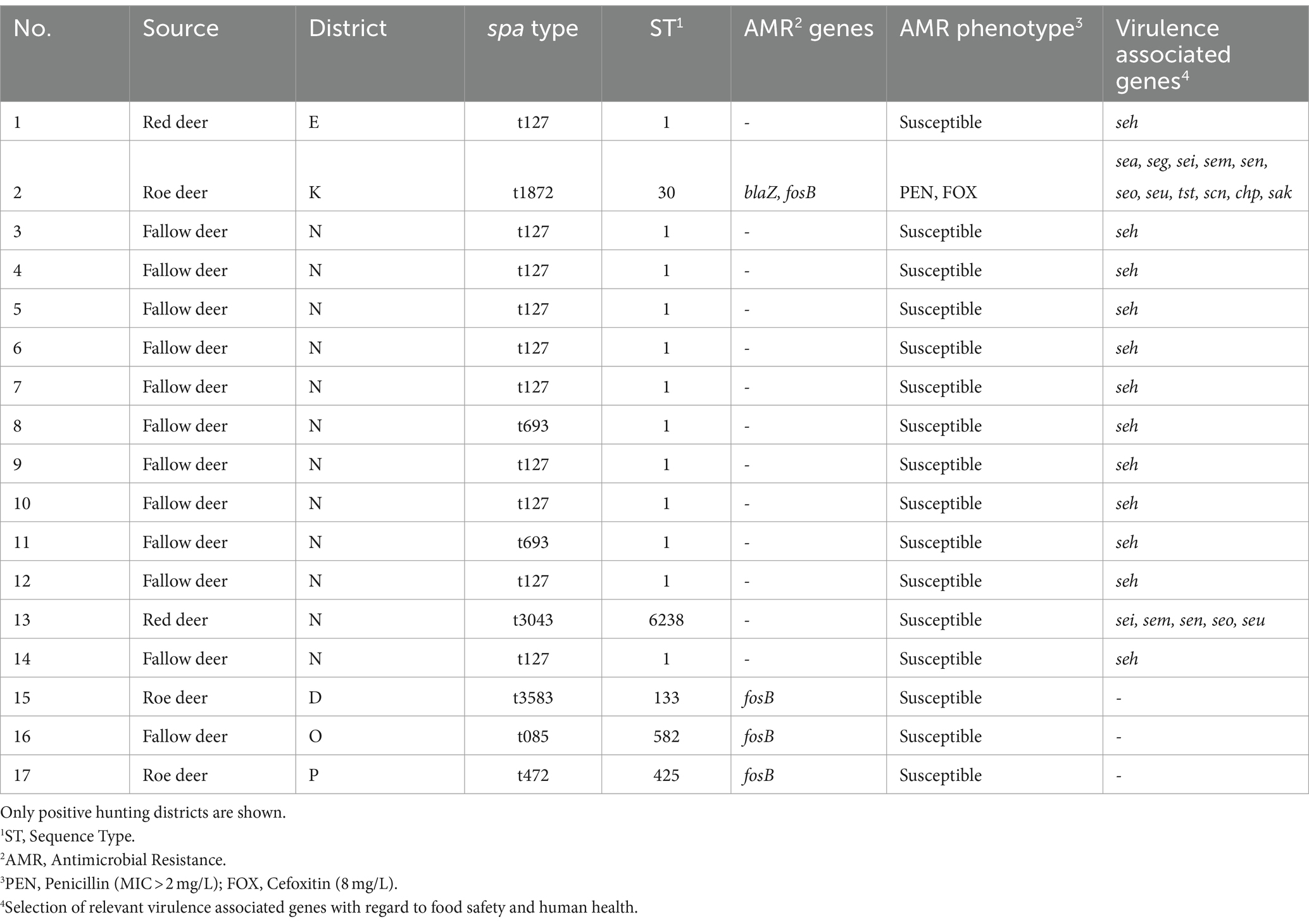
Table 2. Genotypic characteristics, antimicrobial resistance (AMR), and virulence associated genes in S. aureus isolates from wild ungulates from different districts and animals in Brandenburg, Germany.
4 Discussion
Wild ungulates may be carriers of potentially pathogenic microorganisms such as S. aureus. Since game meat is frequently consumed, food safety aspects are elementary in this area. In this study, the occurrence and genotypic characteristics of S. aureus were determined in hunting districts of the federal forest division of the BImA in the German Federal State of Brandenburg in the hunting season 2021/22.
4.1 Occurrence and sequence types
In total, approximately 8% of all sampled wild ungulates carried S. aureus in their nostrils; however, the positive rates in fallow deer (32%) and red deer (13%) were higher than in roe deer (4%). On the contrary to other studies, which showed a prevalence of up to 18% in Spain (26), 33% in Portugal (27) or 37% in Germany (28), in this study, S. aureus was not found in the nostrils of wild boars in the respective hunting season.
A recent Portuguese study elucidates the epidemiological links of S. aureus at the animal-human interface (29). Several sequence types were determined in the detected S. aureus isolates in this study. Although some S. aureus can be classified into livestock-associated, healthcare-associated or community-associated according to their ST, other STs have been reported from various sources e. g. humans, livestock, companion animals and wildlife (30). The latter seems to be the case in this study for those being associated to ST1, ST30, ST133, ST425, ST582, and ST6238. S. aureus with ST1, ST30, ST133, and ST425 were already reported in different species of wild animals before (10) and their presence was also shown in humans (31). S. aureus ST582 seems to be more prevalent in human clinical samples (32–34) than in animal samples; however, in a previous study, ST582 was detected in a purulent subcutaneous lesion of a farm rabbit (35). S. aureus ST6238 is a relatively new sequence type and was originally detected in a horse in the year 2022 (36).
4.2 Transmission of S. aureus in wild ungulates
Interestingly, several t127-ST1 S. aureus isolates obtained from fallow deer of the same district and during the same hunt clustered closely together in the phylogenetic analysis comparing their core genomes. The close genetic relationship of the respective isolates indicates a common source of colonization, possibly accompanied by intraspecific spread, within the local fallow deer population. There may be several reasons for the clonality of the isolates. One possible hypothesis is that these wild artiodactyls might have been colonized in the same area in this specific district, where S. aureus inhabits a particular niche and was transmitted to the carriers. S. aureus may for instance be present in surface water (37) or being secreted via animal feces (38, 39), so that a transmission may take place in close contact to the contaminated area; e.g. during sniffing or rolling on the ground. However, neither of the ten wild boars nor the five roe deer sampled within the same hunting district carried S. aureus, which would contradict this assumption. A further possibility is that positive individuals with clonal S. aureus isolates might be related to each other. Fallow deer are gregarious animals, often forming herds of does accompanied by fawns and few males during various seasons (40). ST1 isolates were obtained primarily from fawns and young fallow deer (<2 years) and therefore could have been transmitted from one animal to another due to close contact within the herd. The need of close contact may be further supported by the notable difference to the S. aureus isolate of the red deer sampled at the same hunting district. It is also noteworthy that European herds of fallow deer usually stay within a relatively small territory (in average 3 to 5 km2) compared to European red deer [in average 9 to 36 km2; (41)]. Some red deer populations additionally have long migration routes (42, 43). Thus, the infection of this red deer individual may have occurred under other habitat conditions. Moreover, the distant relation of S. aureus isolates from different districts illustrates that, at least in the sampled areas, there was no obvious transmission of S. aureus between the districts, probably due to the long distances between hunting districts or to local habitat fragmentation (44).
4.3 Antimicrobial resistance and virulence factors
S. aureus is one of the high priority pathogens with regard to annual deaths and antimicrobial resistance (45). Especially, the most prevalent ST1 in this study has also been frequently reported as MRSA in either a clinical, human context, in which the strains often harbor the staphylococcal chromosome cassette (SCC) mec type IV as well as virulence genes for human adaptation like the immune evasion cluster genes sak and scn, or in a livestock context harboring the SCCmec V in combination with resistance genes erm(A) and vga(A) (46, 47). In contrast, the ST1 isolates in this study lack all of these typical genes for human or livestock adaptation and seem to be unaffected from antimicrobial pressure. Only the t1872-ST30 S. aureus isolate in this study showed phenotypic resistance to the beta-lactam antibiotics penicillin and cefoxitin, whereas the other isolates were susceptible to all tested antimicrobials. Accordingly, the t1872-ST30 isolate harbored the beta-lactamase coding blaZ gene, which confers resistance to penicillin and, in the case of hyperproduction, may also confer resistance to oxacillin or cefoxitin (48, 49). Interestingly, this strain was obtained from an individual hunted in a district near Berlin and Potsdam, which is among the areas with the higher settlement coverage of all hunting districts regularly sampled by the BfR (41). Moreover, the isolate was obtained from a roe deer fawn. As reviewed before (41), this individual likely did not roam long distances before sampling, as European females of this deer species typically have a territory size <1 km2. Thus, it is highly probable that transmission occurred directly from the immediate environment or the mother doe. Except the t1872-ST30 isolate, all other S. aureus isolates of this study were susceptible to the tested antimicrobial substances and did not harbor any AMR genes despite the fosfomycin resistance transmitting fosB gene discovered in four isolates. A recent study shows an increasing prevalence of the fosB gene in S. aureus (50). The high susceptibility to most antimicrobials seems to be reasonable, since antimicrobial pressure in the hunting areas predominantly covered by forest may be low, there is no need for bacteria to develop or keep antimicrobial resistance to survive. However, wild ungulates living in regions more intensively used for livestock farming were observed to carry Staphylococcaceae isolates with reduced susceptibility to cefoxitin more often than wild ungulates in less anthropized areas (41, 51). Despite these observations and coincidences, we deliberately refrain from delving further into the possible transmission routes and sources of the sampled animals due to the general lack of epidemiological information in this field. Future studies should focus on providing more detailed insights into possible transmission routes in anthropized habitats.
S. aureus may carry a multiplicity of virulence associated genes. With respect to food safety, in particular staphylococcal enterotoxin or staphylococcal enterotoxin-like genes are of great importance (4). The uptake of SEs via food such as milk or meat may lead to nausea, vomiting, diarrhea or abdominal pain. Especially young and old people or persons with immunodeficiencies are prone to suffer severely from staphylococcal food poisoning. In this study, 14/17 isolates harbored SE or SEl genes. In particular, an seh gene was detected in all of the t127-ST1 S. aureus isolates and is highly conserved in strains of this ST. (47) Although SEH is regarded as less potent than other SEs, food poisoning by SEH due to consumption of contaminated food was occasionally reported (52, 53). Two isolates carried a multiplicity of SE or SEl genes in their genomes in this study. The t3043-ST6238 S. aureus isolate was characterized by genes for SEI, SEM, SEN, SEO and SElU. Those genes are located in the so-called enterotoxin gene cluster (egc) (54). Food poisoning caused by S. aureus that carried the sei gene was already described in previous studies (55, 56). However, SEI seems to have a rather low emetic activity (57) and sei carrying strains only produce a relative low amount of SEI (58). However, isolates carrying the egc were shown to be the causative agent of food poisoning and were already related to food-borne outbreaks (59). The highest toxicogenic potential was detected in the t1872-ST30 S. aureus isolate in this study. ST30 S. aureus are characterized as human-adapted strains (60) and was isolated from a comparably strong anthropized area. Besides its antimicrobial resistance, as mentioned above, this isolate carried genes encoding a high number of staphylococcal enterotoxins as well as genes for the toxic shock syndrome toxin and the immune evasion cluster. Elements of the immune evasion cluster function as evading mechanism with respect to the human immune system and the toxic shock syndrome toxin may act as superantigen in the human body (5). Regarding food safety, the t1872-ST30 S. aureus isolate in this study also carried the egc [SEG, SEI, SEM, SEN, SEO, SElU; (54)]. Moreover, the t1872-ST30 isolate harbored the sea gene. The appearance of sea and tst genes in t1872-ST30 S. aureus is common (61). The sea gene is often located within the immune evasion cluster complex (62). SEA is the most common toxin in relation to food poisoning (63) and several food-borne outbreaks caused by SEA were reported (64, 65).
The finding of potentially virulent S. aureus in wild ungulates elucidates that during handling of hunted animals as well as processing of game meat strict hygienic measures are of significant importance to prevent direct transmission of S. aureus from animals to humans or meat surfaces, and thus the possibility of serious infections and food poisoning. This study is limited to wild ungulates from the German Federal State of Brandenburg. However, this data expands the knowledge about zoonotic pathogens in specific regions in Germany.
5 Conclusion
Wild animals may carry potentially virulent S. aureus as indicated by the findings of this study. The close phylogenetic relationship of S. aureus isolates indicates a possible spread between animals from the same territory or a common source of colonization. Handling of animals or their carcasses might contribute to staphylococcal infections in humans. Moreover, meat contamination and subsequent food poisoning due to SE producing strains may occur, if recommended hygiene measures are not applied during processing game meat. Monitoring of pathogens in wild game animals is of great importance to evaluate pathogen ecology, zoonotic potential, significant risks and develop recommendations.
Data availability statement
The datasets presented in this study can be found in online repositories. The names of the repository/repositories and accession number(s) can be found at: https://www.ncbi.nlm.nih.gov/bioproject, PRJNA641762.
Ethics statement
Ethical approval was not required for the study involving animals in accordance with the local legislation and institutional requirements because the basis of the sampling approach was a framework agreement involving the German Federal Institute for Risk Assessment (BfR) and the German Institute for Federal Real Estate (BImA). The federal forest division of the BImA is responsible for forest and game management over 476,000 ha of hunting area in Germany.
Author contributions
TL: Conceptualization, Data curation, Funding acquisition, Investigation, Methodology, Writing – original draft, Writing – review & editing. RM: Conceptualization, Data curation, Writing – original draft, Writing – review & editing. JS-W: Conceptualization, Writing – original draft, Writing – review & editing. MR: Conceptualization, Writing – original draft, Writing – review & editing. SM: Conceptualization, Funding acquisition, Writing – original draft, Writing – review & editing.
Funding
The author(s) declare that financial support was received for the research, authorship, and/or publication of this article. This study was carried out in the framework of the BfR internal project No. 1322-769.
Acknowledgments
Sampling was part of a framework agreement between BfR and BImA and was performed through coordination of Center for Land Use Related Evaluation Methods, One Health Approaches of the BfR. We greatly thank all employees of the federal forest division of the BImA and the state forest of Brandenburg and all hunters for their support during sampling. We gratefully acknowledge the active participation of Denny Maaz in sample and data management. Special thanks to Katja Drache, Daniel Leeser-Boek, Pascal Witt, and Ylanna Kelner-Burgos for laboratory support and NGS preparation. We also thank Carlus Deneke and Simon Tausch for bioinformatical analysis and Mirjam Grobbel for antimicrobial susceptibility testing.
Conflict of interest
The authors declare that the research was conducted in the absence of any commercial or financial relationships that could be construed as a potential conflict of interest.
Publisher’s note
All claims expressed in this article are solely those of the authors and do not necessarily represent those of their affiliated organizations, or those of the publisher, the editors and the reviewers. Any product that may be evaluated in this article, or claim that may be made by its manufacturer, is not guaranteed or endorsed by the publisher.
Footnotes
References
1. Holmes, MA, and Zadoks, RN. Methicillin resistant S. aureus in human and bovine mastitis. J Mammary Gland Biol Neoplasia. (2011) 16:373–82. doi: 10.1007/s10911-011-9237-x
2. Kwiecinski, JM, and Horswill, AR. Staphylococcus aureus bloodstream infections: pathogenesis and regulatory mechanisms. Curr Opin Microbiol. (2020) 53:51–60. doi: 10.1016/j.mib.2020.02.005
3. Szafraniec, GM, Szeleszczuk, P, and Dolka, B. Review on skeletal disorders caused by Staphylococcus spp. in poultry. Vet Q. (2022) 42:21–40. doi: 10.1080/01652176.2022.2033880
4. Hennekinne, JA, De Buyser, ML, and Dragacci, S. Staphylococcus aureus and its food poisoning toxins: characterization and outbreak investigation. FEMS Microbiol Rev. (2012) 36:815–36. doi: 10.1111/j.1574-6976.2011.00311.x
5. Dinges, MM, Orwin, PM, and Schlievert, PM. Exotoxins of Staphylococcus aureus. Clin Microbiol Rev. (2000) 13:16–34. doi: 10.1128/CMR.13.1.16
6. Rasmussen, SL, Larsen, J, Van Wijk, RE, Jones, OR, Berg, TB, Angen, Ø, et al. European hedgehogs (Erinaceus europaeus) as a natural reservoir of methicillin-resistant Staphylococcus aureus carrying mecC in Denmark. PLoS One. (2019) 14:e0222031. doi: 10.1371/journal.pone.0222031
7. Ruiz-Ripa, L, Alcalá, L, Simón, C, Gómez, P, Mama, OM, Rezusta, A, et al. Diversity of Staphylococcus aureus clones in wild mammals in Aragon, Spain, with detection of MRSA ST130-mecC in wild rabbits. J Appl Microbiol. (2019) 127:284–91. doi: 10.1111/jam.14301
8. Sahin-Tóth, J, Albert, E, Juhász, A, Ghidán, Á, Juhász, J, Horváth, A, et al. Prevalence of Staphylococcus aureus in wild hedgehogs (Erinaceus europaeus) and first report of mecC-MRSA in Hungary. Sci Total Environ. (2022) 815:152858. doi: 10.1016/j.scitotenv.2021.152858
9. Silva, V, Gabriel, SI, Borrego, SB, Tejedor-Junco, MT, Manageiro, V, Ferreira, E, et al. Antimicrobial resistance and genetic lineages of Staphylococcus aureus from wild rodents: first report of mecC-positive methicillin-Resistant S. aureus (MRSA) in Portugal. Animals (Basel). (2021) 11:1537. doi: 10.3390/ani11061537
10. Heaton, CJ, Gerbig, GR, Sensius, LD, Patel, V, and Smith, TC. Staphylococcus aureus epidemiology in wildlife: A systematic review. Antibiotics. (2020) 9:89. doi: 10.3390/antibiotics9020089
11. Martínez-Seijas, C, Mascarós, P, Lizana, V, Martí-Marco, A, Arnau-Bonachera, A, Chillida-Martínez, E, et al. Genomic characterization of Staphylococcus aureus in wildlife. Animals (Basel). (2023) 13:1064. doi: 10.3390/ani13061064
12. Abdullahi, IN, Fernández-Fernández, R, Juárez-Fernández, G, Martínez-Álvarez, S, Eguizábal, P, Zarazaga, M, et al. Wild animals are reservoirs and sentinels of Staphylococcus aureus and MRSA clones: A problem with "one health" concern. Antibiotics (Basel). (2021) 10:1556. doi: 10.3390/antibiotics10121556
13. Silva, V, Capelo, JL, Igrejas, G, and Poeta, P. Molecular epidemiology of Staphylococcus aureus lineages in wild animals in Europe: A review. Antibiotics (Basel). (2020) 9:122. doi: 10.3390/antibiotics9030122
14. Hahaj-Siembida, A, Nowakiewicz, A, Korzeniowska-Kowal, A, Szecówka, K, Trościańczyk, A, Zięba, P, et al. Red foxes (Vulpes vulpes) as a specific and underappreciated reservoir of resistant and virulent coagulase-positive Staphylococcus spp. strains. Res Vet Sci. (2024) 166:105111. doi: 10.1016/j.rvsc.2023.105111
15. Kraushaar, B, and Fetsch, A. First description of PVL-positive methicillin-resistant Staphylococcus aureus (MRSA) in wild boar meat. Int J Food Microbiol. (2014) 186:68–73. doi: 10.1016/j.ijfoodmicro.2014.06.018
16. Banaszkiewicz, S, Tabiś, A, Wałecki, B, Łyżwińska, K, Bystroń, J, and Bania, J. Spa types and staphylococcal enterotoxin production of Staphylococcus aureus isolated from wild boar. Microb Ecol. (2023) 86:2184–91. doi: 10.1007/s00248-023-02236-4
17. Burgold-Voigt, S, Monecke, S, Busch, A, Bocklisch, H, Braun, SD, Diezel, C, et al. Characterisation of a Staphylococcus aureus isolate carrying phage-borne enterotoxin E from a European badger (Meles meles). Pathogens. (2023) 12:704. doi: 10.3390/pathogens12050704
18. EFSA and ECDC . The European Union one health 2022 Zoonoses report. EFSA J. (2023) 21:e8442. doi: 10.2903/j.efsa.2023.8442
19. Maaz, D, Gremse, C, Stollberg, KC, Jäckel, C, Sutrave, S, Kästner, C, et al. Standardised sampling approach for investigating pathogens or environmental Chemicals in Wild Game at community hunts. Animals (Basel). (2022) 12:12. doi: 10.3390/ani12070888
20. ISO (2003). "ISO 6888-3:2003 Microbiology of food and animal feeding stuffs — Horizontal method for the enumeration of coagulase-positive staphylococci (Staphylococcus aureus and other species) — Part 3: Detection and MPN technique for low numbers".).
21. Deneke, C, Brendebach, H, Uelze, L, Borowiak, M, Malorny, B, and Tausch, SH. Species-specific quality control, assembly and contamination detection in microbial isolate sequences with AQUAMIS. Genes. (2021) 12:644. doi: 10.3390/genes12050644
22. Jünemann, S, Sedlazeck, FJ, Prior, K, Albersmeier, A, John, U, Kalinowski, J, et al. Updating benchtop sequencing performance comparison. Nat Biotechnol. (2013) 31:294–6. doi: 10.1038/nbt.2522
23. ISO (2006). "Clinical laboratory testing and in vitro diagnostic test systems - Susceptibility testing of infectious agents and evaluation of performance of antimicrobial susceptibility test devices - Part 1: Reference method for testing the in vitro activity of antimicrobial agents against rapidly growing aerobic bacteria involved in infectious diseases".).
24. CLSI ed. Methods for dilution antimicrobial susceptibility tests for Bacteria that grow aerobically In: CLSI standard M07. 12th eds. B. L. Zimmer, D. E. Carpenter, G. Esparza, K. Alby, A. Bhatnagar, and A. L. Ferrell, et al.Clinical and Laboratory Standards Institute. (2024)
25. EFSA . Technical specifications on the harmonised monitoring and reporting of antimicrobial resistance in methicillin-resistant Staphylococcus aureus in food-producing animals and food. EFSA J. (2012) 10. doi: 10.2903/j.efsa.2012.2897
26. Porrero, MC, Mentaberre, G, Sánchez, S, Fernández-Llario, P, Casas-Díaz, E, Mateos, A, et al. Carriage of Staphylococcus aureus by free-living wild animals in Spain. Appl Environ Microbiol. (2014) 80:4865–70. doi: 10.1128/AEM.00647-14
27. Sousa, M, Silva, N, Manageiro, V, Ramos, S, Coelho, A, Gonçalves, D, et al. First report on MRSA CC398 recovered from wild boars in the north of Portugal. Are we facing a problem? Sci Total Environ. (2017) 596-597:26–31. doi: 10.1016/j.scitotenv.2017.04.054
28. Seinige, D, Von Altrock, A, and Kehrenberg, C. Genetic diversity and antibiotic susceptibility of Staphylococcus aureus isolates from wild boars. Comp Immunol Microbiol Infect Dis. (2017) 54:7–12. doi: 10.1016/j.cimid.2017.07.003
29. Ramos, B, and Cunha, MV. The mobilome of Staphylococcus aureus from wild ungulates reveals epidemiological links at the animal-human interface. Environ Pollut. (2024) 356:124241. doi: 10.1016/j.envpol.2024.124241
30. Cuny, C, Layer-Nicolaou, F, Werner, G, and Witte, W. A look at staphylococci from the one health perspective. Int J Med Microbiol. (2024) 314:151604. doi: 10.1016/j.ijmm.2024.151604
31. Cuny, C, Wieler, LH, and Witte, W. Livestock-associated MRSA: the impact on humans. Antibiotics (Basel). (2015) 4:521–43. doi: 10.3390/antibiotics4040521
32. Azarian, T, Cella, E, Baines, SL, Shumaker, MJ, Samel, C, Jubair, M, et al. Genomic epidemiology and global population structure of Exfoliative toxin A-producing Staphylococcus aureus strains associated with staphylococcal scalded skin syndrome. Front Microbiol. (2021) 12:663831. doi: 10.3389/fmicb.2021.663831
33. Cella, E, David, MZ, Jubair, M, Baines, SL, Pegues, DA, and Azarian, T. Complete genome sequence of Exfoliative toxin-producing Staphylococcus aureus strain MSSA_SSSS_01, obtained from a case of staphylococcal scalded-skin syndrome. Microbiol Resour Announc. (2021) 10:10. doi: 10.1128/MRA.01335-20
34. Weber, RE, Layer, F, Fuchs, S, Bender, JK, Fiedler, S, Werner, G, et al. Complete genome sequences of two methicillin-sensitive Staphylococcus aureus isolates representing a population subset highly prevalent in human colonization. Genome Announc. (2016) 4:e00716–16. doi: 10.1128/genomeA.00716-16
35. Silva, V, Sousa, T, Gomez, P, Sabenca, C, Vieira-Pinto, M, Capita, R, et al. Livestock-associated methicillin-resistant Staphylococcus aureus (MRSA) in purulent subcutaneous lesions of farm rabbits. Food Secur. (2020) 9:439. doi: 10.3390/foods9040439
36. Kaiser-Thom, S, Gerber, V, Collaud, A, Hurni, J, and Perreten, V. Prevalence and WGS-based characteristics of Staphylococcus aureus in the nasal mucosa and pastern of horses with equine pastern dermatitis. BMC Vet Res. (2022) 18:79. doi: 10.1186/s12917-021-03053-y
37. Silva, V, Ferreira, E, Manageiro, V, Reis, L, Tejedor-Junco, MT, Sampaio, A, et al. Distribution and clonal diversity of Staphylococcus aureus and other staphylococci in surface waters: detection of ST425-t742 and ST130-t843 mecC-positive MRSA strains. Antibiotics (Basel). (2021) 10:1416. doi: 10.3390/antibiotics10111416
38. Suzuki, Y, Ishitsuka, T, Takagi, M, Sasaki, Y, Kakuda, T, Kobayashi, K, et al. Isolation and genetic characterization of Staphylococcus aureus from wild animal feces and game meats. Folia Microbiol (Praha). (2023) 69:347–60. doi: 10.1007/s12223-023-01071-x
39. Tareen, AR, and Zahra, R. Community acquired methicillin resistant staphylococci (CA-MRS) in fecal matter of wild birds - A 'one health' point of concern. J Infect Public Health. (2023) 16:877–83. doi: 10.1016/j.jiph.2023.04.002
40. Mattiello, S, Mattiangeli, V, Bianchi, L, and Carenzi, C. Feeding and social behavior of fallow deer (Dama dama L.) under intensive pasture confinement. J Anim Sci. (1997) 75:339–47. doi: 10.2527/1997.752339x
41. Mateus-Vargas, RH, Lienen, T, Maaz, D, Richter, M, Maurischat, S, and Steinhoff-Wagner, J. Evaluation of the occurrence of Staphylococcaceae with reduced susceptibility to Cefoxitin in wild ungulates in Brandenburg, Germany, based on land use-related factors. Microbiol Spect. (2022) 10:e02560–22. doi: 10.1128/spectrum.02560-22
42. Kropil, R, Smolko, P, and Garaj, P. Home range and migration patterns of male red deer Cervus elaphus in Western Carpathians. Eur J Wildl Res. (2015) 61:63–72. doi: 10.1007/s10344-014-0874-4
43. Luccarini, S, Mauri, L, Ciuti, S, Lamberti, P, and Apollonio, M. Red deer (Cervus elaphus) spatial use in the Italian Alps: home range patterns, seasonal migrations, and effects of snow and winter feeding. Ethol Ecol Evol. (2006) 18:127–45. doi: 10.1080/08927014.2006.9522718
44. Freudenberger, L, Hobson, PR, Rupic, S, Pe’er, G, Schluck, M, Sauermann, J, et al. Spatial road disturbance index (SPROADI) for conservation planning: a novel landscape index, demonstrated for the state of Brandenburg, Germany. Landsc Ecol. (2013) 28:1353–69. doi: 10.1007/s10980-013-9887-8
45. Ikuta, KS, Swetschinski, LR, Robles Aguilar, G, Sharara, F, Mestrovic, T, Gray, AP, et al. Global mortality associated with 33 bacterial pathogens in 2019: a systematic analysis for the global burden of disease study 2019. Lancet. (2022) 400:2221–48. doi: 10.1016/S0140-6736(22)02185-7
46. Alba, P, Feltrin, F, Cordaro, G, Porrero, MC, Kraushaar, B, Argudín, MA, et al. Livestock-associated methicillin resistant and methicillin susceptible Staphylococcus aureus sequence type (CC)1 in European farmed animals: high genetic relatedness of isolates from Italian cattle herds and humans. PLoS One. (2015) 10:e0137143. doi: 10.1371/journal.pone.0137143
47. Earls, MR, Steinig, EJ, Monecke, S, Samaniego Castruita, JA, Simbeck, A, Schneider-Brachert, W, et al. Exploring the evolution and epidemiology of European CC1-MRSA-IV: tracking a multidrug-resistant community-associated meticillin-resistant Staphylococcus aureus clone. Microb Genom. (2021) 7:1416. doi: 10.1099/mgen.0.000601
48. Argudín, MA, Roisin, S, Nienhaus, L, Dodémont, M, De Mendonça, R, Nonhoff, C, et al. Genetic diversity among Staphylococcus aureus isolates showing Oxacillin and/or Cefoxitin resistance not linked to the presence of mec genes. Antimicrob Agents Chemother. (2018) 62:e00091–18. doi: 10.1128/aac.00091-18
49. Scholtzek, AD, Hanke, D, Walther, B, Eichhorn, I, Stockle, SD, Klein, KS, et al. Molecular characterization of equine Staphylococcus aureus isolates exhibiting reduced Oxacillin susceptibility. Toxins (Basel). (2019) 11:11. doi: 10.3390/toxins11090535
50. Monte, DFM, and De Oliveira, CJB. Global trends in the increasing prevalence of the fosfomycin resistance gene in Staphylococcus aureus. Lancet Microbe. (2024) 5:e104. doi: 10.1016/S2666-5247(23)00339-7
51. Ramos, B, Rosalino, LM, Palmeira, JD, Torres, RT, and Cunha, MV. Antimicrobial resistance in commensal Staphylococcus aureus from wild ungulates is driven by agricultural land cover and livestock farming. Environ Pollut. (2022) 303:119116. doi: 10.1016/j.envpol.2022.119116
52. Ikeda, T, Tamate, N, Yamaguchi, K, and Makino, S. Mass outbreak of food poisoning disease caused by small amounts of staphylococcal enterotoxins A and H. Appl Environ Microbiol. (2005) 71:2793–5. doi: 10.1128/AEM.71.5.2793-2795.2005
53. Jørgensen, HJ, Mathisen, T, Løvseth, A, Omoe, K, Qvale, KS, and Loncarevic, S. An outbreak of staphylococcal food poisoning caused by enterotoxin H in mashed potato made with raw milk. FEMS Microbiol Lett. (2005) 252:267–72. doi: 10.1016/j.femsle.2005.09.005
54. Jarraud, S, Peyrat, MA, Lim, A, Tristan, A, Bes, M, Mougel, C, et al. Egc, a highly prevalent operon of enterotoxin gene, forms a putative nursery of superantigens in Staphylococcus aureus. J Immunol. (2001) 166:669–77. doi: 10.4049/jimmunol.166.1.669
55. Baumgartner, A, Niederhauser, I, and Johler, S. Virulence and resistance gene profiles of staphylococcus aureus strains isolated from ready-to-eat foods. J Food Prot. (2014) 77:1232–6. doi: 10.4315/0362-028X.JFP-14-027
56. Yan, X, Wang, B, Tao, X, Hu, Q, Cui, Z, Zhang, J, et al. Characterization of Staphylococcus aureus strains associated with food poisoning in Shenzhen, China. Appl Environ Microbiol. (2012) 78:6637–42. doi: 10.1128/AEM.01165-12
57. Munson, SH, Tremaine, MT, Betley, MJ, and Welch, RA. Identification and characterization of staphylococcal enterotoxin types G and I from Staphylococcus aureus. Infect Immun. (1998) 66:3337–48. doi: 10.1128/IAI.66.7.3337-3348.1998
58. Omoe, K, Ishikawa, M, Shimoda, Y, Hu, DL, Ueda, S, and Shinagawa, K. Detection of seg, seh, and sei genes in Staphylococcus aureus isolates and determination of the enterotoxin productivities of S. aureus isolates harboring seg, seh, or sei genes. J Clin Microbiol. (2002) 40:857–62. doi: 10.1128/JCM.40.3.857-862.2002
59. Johler, S, Giannini, P, Jermini, M, Hummerjohann, J, Baumgartner, A, and Stephan, R. Further evidence for staphylococcal food poisoning outbreaks caused by egc-encoded enterotoxins. Toxins (Basel). (2015a) 7:997–1004. doi: 10.3390/toxins7030997
60. Melles, DC, Gorkink, RF, Boelens, HA, Snijders, SV, Peeters, JK, Moorhouse, MJ, et al. Natural population dynamics and expansion of pathogenic clones of Staphylococcus aureus. J Clin Invest. (2004) 114:1732–40. doi: 10.1172/JCI200423083
61. Peacock, SJ, Moore, CE, Justice, A, Kantzanou, M, Story, L, Mackie, K, et al. Virulent combinations of adhesin and toxin genes in natural populations of Staphylococcus aureus. Infect Immun. (2002) 70:4987–96. doi: 10.1128/IAI.70.9.4987-4996.2002
62. Van Wamel, WJ, Rooijakkers, SH, Ruyken, M, Van Kessel, KP, and Van Strijp, JA. The innate immune modulators staphylococcal complement inhibitor and chemotaxis inhibitory protein of Staphylococcus aureus are located on beta-hemolysin-converting bacteriophages. J Bacteriol. (2006) 188:1310–5. doi: 10.1128/JB.188.4.1310-1315.2006
63. Pinchuk, IV, Beswick, EJ, and Reyes, VE. Staphylococcal enterotoxins. Toxins (Basel). (2010) 2:2177–97. doi: 10.3390/toxins2082177
64. Johler, S, Tichaczek-Dischinger, PS, Rau, J, Sihto, HM, Lehner, A, Adam, M, et al. Outbreak of staphylococcal food poisoning due to SEA-producing Staphylococcus aureus. Foodborne Pathog Dis. (2013) 10:777–81. doi: 10.1089/fpd.2013.1503
Keywords: Staphylococcus aureus , wild ungulates, virulence, antimicrobial resistance, phylogeny
Citation: Lienen T, Mateus-Vargas RH, Steinhoff-Wagner J, Richter MH and Maurischat S (2024) High toxinogenic potential of Staphylococcus aureus from wild ungulates in Brandenburg, Germany with a low level of antibiotic resistance. Front. Vet. Sci. 11:1445413. doi: 10.3389/fvets.2024.1445413
Edited by:
Magdalena Baymakova, Military Medical Academy (Bulgaria), BulgariaReviewed by:
Rumyana Markovska, Medical University Sofia, BulgariaFrancesca Pedonese, University of Pisa, Italy
Yordan Hodzhev, National Center of Infectious and Parasitic Diseases (NCIPD), Bulgaria
Tsvetelina Paunova-Krasteva, Bulgarian Academy of Sciences, Bulgaria
Natalia Wiktorczyk-Kapischke, Nicolaus Copernicus University in Toruń, Poland
Copyright © 2024 Lienen, Mateus-Vargas, Steinhoff-Wagner, Richter and Maurischat. This is an open-access article distributed under the terms of the Creative Commons Attribution License (CC BY). The use, distribution or reproduction in other forums is permitted, provided the original author(s) and the copyright owner(s) are credited and that the original publication in this journal is cited, in accordance with accepted academic practice. No use, distribution or reproduction is permitted which does not comply with these terms.
*Correspondence: Tobias Lienen, dG9iaWFzLmxpZW5lbkBiZnIuYnVuZC5kZQ==
†ORCID: Tobias Lienen, https://orcid.org/0000-0003-2564-1472
Rafael Hernán Mateus-Vargas, https://orcid.org/0000-0001-5489-2452
Julia Steinhoff-Wagner, https://orcid.org/0000-0003-0180-9859
Martin H. Richter, https://orcid.org/0000-0002-8649-5248
Sven Maurischat, https://orcid.org/0000-0003-4730-6392