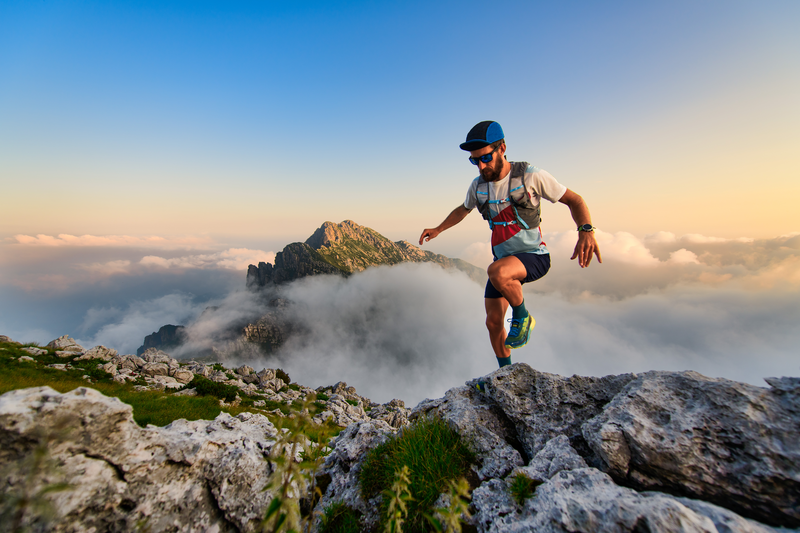
94% of researchers rate our articles as excellent or good
Learn more about the work of our research integrity team to safeguard the quality of each article we publish.
Find out more
ORIGINAL RESEARCH article
Front. Vet. Sci. , 26 September 2024
Sec. Animal Reproduction - Theriogenology
Volume 11 - 2024 | https://doi.org/10.3389/fvets.2024.1445058
Introduction: Heat stress negatively affects both animal reproductive performance and their overall wellbeing and welfare. When temperatures soar, the body responds to maintain balance, resulting in heat stress. This triggers various responses, including the buildup of reactive oxygen species (ROS), which can harm sperm vitality through lipid peroxidation. Oxidative damage can result in sperm dysfunction. This study aimed to evaluate the effects of environmental heat stress on canine quantitative and qualitative ejaculate parameters.
Methods: Thirty-six male crossbred dogs were involved in the trial. This study was performed in 2022, precisely from May to August. Dogs were subdivided in two groups, one heat stressed (HS) and one in thermoneutrality (TN). Thermo Hygrometric Index (THI) was hourly measured and ranged from 60 to 71 in TN dogs and from 60 to 83 for HS dogs. Semen and blood samples were collected at 30-day intervals, starting from May (0 days), and then at 30 days, 60 days, and 90 days and analysis for evaluating biochemical profile, semen oxidative status, and semen quality were performed.
Results: In HS dogs, serum total protein, albumin, and urea concentrations showed a significant decrease after 60 days (P < 0.01), with values lower than those observed in TN dogs (P < 0.01). Both catalase and glutathione peroxidase concentrations were reduced after 60 days in HS dogs, showing lower levels than the TN group (P < 0.01 and P < 0.05, respectively). Antioxidant potential increased over time in HS dogs, reaching higher values at 60 days (P < 0.05) and 90 days (P < 0.01). On the other hand, ROS in the sperm of HS animals rose by day 90, surpassing the values recorded at previous time points and in TN dogs (P < 0.01). Semen concentration (P > 0.01) and total sperm count (P < 0.05) declined after 30 days in the HS group and remained lower than the TN group throughout the trial.
Discussion: The study demonstrates that heat stress negatively affects the oxidative status and sperm quality of male dogs, reducing reproductive performance. However, further research is needed due to the lack of complete breed homogeneity in the study groups.
It is now widely recognized within the scientific community that our planet is experiencing global climate change (1–3). Since the industrial revolution, human activities have contributed to a rise in global temperatures (2). This increase has exceeded 1°C since 1950, with the last 7 years being the hottest on record (4, 5). In response to the growing challenge posed by climate change, there has been increased focus on the relationships among heat stress, behavior, coat and skin characteristics, thermoregulatory ability, oxidative stress, and fertility (6, 71). For dogs, a Thermo Hygrometric Index (THI) value exceeding 72–74 is generally considered a critical threshold beyond which heat stress begins. This value serves as a general guideline and can vary based on factors such as breed, age, health, and the physical condition of the animal. Brachycephalic breeds (such as Bulldogs and Pugs) may be more sensitive to heat compared to other breeds (46, 69). In regions affected by climate change, heat stress is the primary factor responsible for declines in fertility and productivity (1, 70). The current harshening of heat stress scenarios negatively impacts reproduction (7). Heat stress promotes the formation of reactive oxygen species (ROS) (8), which primarily target the polyunsaturated fatty acids found in the membrane phospholipids of spermatozoa. The modification of these fatty acids leads to the disruption of cell structure and function. Specifically, heat stress-induced ROS generation can result in decreased sperm concentration, average pathway velocity, straight-line velocity, curvilinear velocity, amplitude of lateral head displacement, straightness, and linearity. This oxidative stress not only causes cellular damage but also initiates the process of apoptosis (9). Testes are particularly susceptible to hyperthermia, as the pathway from spermatogonia to spermatozoa involves numerous cellular divisions, including the loss of cellular machinery and high DNA condensation, making this process highly vulnerable to external threats such as elevated temperatures (10–12). Ideally, testicular temperature should be maintained 2 to 8°C below body temperature in various species [boar: (13); bull: (14); ram: (15); stallion: (16); dog: (17)]. To protect against environmental heat stress, the testes utilize a sophisticated thermal regulation mechanism. The scrotal skin, which is thin, with minimal adipose tissue and hair, and a well-developed blood and lymphatic system, plays a crucial role in maintaining lower testicular temperatures. These characteristics facilitate heat dissipation through radiation and evaporation (18). Additionally, the pampiniform plexus acts as a heat exchange mechanism that allow cooling of the arterial blood entering the testis. The cremaster muscle and dartos tunic work together to retract and relax the testes relative to the abdomen, further aiding in temperature regulation (19, 20). However, when temperatures rise beyond the regulatory capacity of these mechanisms, it can lead to a range of reproductive issues. In dogs, elevated temperatures are associated with reduced libido, impaired spermatogenesis, lower sperm concentration, poor sperm quality, decreased testicular weight, and a temporary period of partial or total infertility (14, 16, 21–23). Sperm parameters, including motility and vigor, may be negatively impacted, while morphological defects such as acrosome degradation, proximal cytoplasmic droplets, bent tails at the head, small heads, and isolated heads become more prevalent, as observed in bulls (24). Despite these significant findings, there is limited research (17) on the effects of heat stress on the health and semen quality of dogs. This study, therefore, aims to assess the impact of high environmental temperatures on clinical and biochemical parameters, oxidative status, and sperm quality in dogs. However, it is important to note that the study faces limitations due to challenges in standardizing the groups, particularly in terms of the dogs' breeds and environmental conditions. Since the dogs involved are privately owned, this introduces a small but unpredictable variable, as their living conditions, while similar, cannot be perfectly controlled.
This study was performed in accordance with the ethical guidelines of the Animal Welfare Committee. Institutional Review Board approval of the study was obtained with approval number (656/18). Informed owner consent was obtained for all dogs.
The study was performed from May to August 2022, in the south of Italy. A total of 36 male dogs, body condition score of 3 (±0.5), were involved in the trial. All dogs were mixed breeds, owned; 18 were consistently kept indoors with functioning air conditioning systems (thermoneutrality group, TN), while 18 were consistently kept outdoors (heat stress group, HS) (Table 1 reported all animals characteristics). All animals were individually housed. Groups were balanced for coat length and color. All dogs were clinically examined to ensure their health status 30 days before the beginning of the trial. Each dog has submitted for a clinical examination and blood analyses. An ultrasound exam of the re-productive tract was also performed, and it was verified that all dogs reacted positively to sperm collection by digital manipulation. Exclusion criteria included obesity, white or long hair, and use of medications within the previous 30 days. All owners were provided with a temperature and humidity monitoring system (Tinytag from Data Loggers, Gemini Data Loggers Ltd, West Sussex, United Kingdom), placed within the indoor space for the TN group dogs and in the outdoor area for the HS group dogs. The data loggers were set to record temperature and humidity on an hourly basis. During each control time for semen and blood sampling, the data logger was brought to the Obstetric, Gynecological, and Andrological Clinic of the Veterinary Medicine Department of the “Aldo Moro” University of Bari (Italy), and the data were downloaded to calculate the Temperature Humidity Index (THI). This dataset was employed to compute the hourly THI utilizing the formula outlined by Maggiolino et al. (25):
where AT is the environmental temperature expressed in degrees Celsius, so that the term (1.8 × AT + 32) represents the conversion of temperature data in degrees Fahrenheit, and RH is the relative humidity as a fraction of unit. Average maximum THI values registered for each experimental group were reported in Figure 1. All dogs were fed a standardized feed starting 30 days before the beginning of the trial [10 g/kg of body weight (BW) daily, composition reported in Table 2] two times a day.
Figure 1. Average maximum THI registered for the two experimental groups. HS, heat stress; TN, thermoneutrality.
The trial lasted 120 days, after 30 days of adaptation to new diet, there was our starting time point (T0) and all sampling procedures and analysis were performed every 30 days (T30, T60, T90) until the end of the trial. The experimental design is reported in Figure 2.
Blood was collected aseptically via puncture of the cephalic vein using 22-gauge needles, employing a negative pressure 4 ml tube system for serum (without anticoagulant) and plasma (with 15 USP U/ml of heparin) (Becton, Dickinson Canada Inc, Vacutainer 1, Oakville, Canada). Tubes intended for plasma were promptly centrifuged (1,500 × g for 10 min), while tubes for serum were allowed to clot at a refrigerated temperature for 10 min before being centrifuged (1,500 × g for 10 min). All plasma and serum aliquots were stored at −80°C until analysis (26). All blood samples were taken in the morning by 10 am with the animals fasting for at least 8 h.
Clinical biochemistry parameters were obtained from the serum samples using an automated biochemistry analyzer (CS-300B; Dirui, Changchun, China) as described by De Palo et al. (27). The following parameters were analyzed: alanine aminotransferase (ALT), aspartate aminotransferase (AST), creatine phosphokinase (CPK), lactate dehydrogenase (LDH), alkaline phosphatase (ALP), glucose (Glu), blood urea nitrogen (BUN), creatinine (Crea), total serum protein (TP), albumin (Alb), cholesterol (Chol), triglycerides (Trig), non-esterified fatty acids (NEFA), calcium (Ca), phosphate (P), magnesium (Mg), chloride (Cl), (Gesan Production Kit, Campobello di Mazara, Trapani, Italy). Globulins (Glob) and albumin/globulin ratio (Alb/Glob) were calculated starting from total protein and albumin parameters. Cortisol was determined using a commercial radioimmunoassay kit (Coat-a-count Cortisol, Siemens Medical Solution Diagnostics, Los Angeles, CA), according to the manufacturer's protocol (28).
Plasma samples were used for oxidation parameters and antioxidant enzyme activities assays. Thiobarbituric acid reactive substances (TBARs) were determined spectrophotometrically, by adding 100 ml of plasma to a 3.7 μl/ml thiobarbituric acid solution. Plasma reactive carbonyl derivative (RCD) levels were determined according to Faure and Lafond (29) using the carbonyl reagent DNPH as described by Forte et al. (30). The absorbance was measured at 380 nm. Hydroperoxides (Hy) were determined spectrophotometrically by an iodometric method as described by Maggiolino et al. (31). Protein carbonyls (PC) levels were determined spectrophotometrically as reported by Salzano et al. (72). The superoxide dismutase (SOD) (EC 1.15.1.1) activity was examined according to Misra (32), and the enzymatic activity was based on the 50% inhibition rate of epinephrine autooxidation at 480 nm. The catalase (CAT) (EC 1.11.1.6) activity was assayed by the method of Clairborne (33). The glutathione peroxidase (GPx) (EC1.11.1.9.) activity was measured according to Dinardo et al. (34).
The ROS and BAP (biological antioxidant potential) serum concentrations were obtained by the means of a photometric analytical system (FREE Carpe Diem®, Diacron International srl, Grosseto, Italy) (35). The d-ROMs (reactive oxygen metabolites) test determines the concentration of reactive oxygen metabolites (ROMs) in a biological sample, particularly hydroperoxides, deriving from the oxidative attack of many bio-chemical substrates (glycids, lipids, amino acids, proteins, nucleotides, etc.) (36). They were measured using a free radical elective evaluator (FREE Carpe Diem; Diacron International) that included a spectrophotometric device reader, and measurement kits (d-ROMs test, Wismerll Co. Ltd., Tokyo, Japan) were optimized to the FREE Carpe Diem® System according to the manufacturer's protocol. Briefly, a 20 μl plasma sample and 1 mL buffered solution (R2 kit reagent, pH 4.8) were gently mixed in a cuvette, and 20 μl of chromogenic substrate (R1 kit reagent) was then added to the cuvette (35, 36). After mixing well, the cuvette was immediately incubated in the thermostatic block of the analyzer for 5 min at 37°C, and absorbance at 505 nm was recorded. The results were expressed in arbitrary units, the Carratelli Units (U/CARR), one unit corresponds to 0.8 mg/L of hydrogen peroxide. The BAP was measured using a free radical elective evaluator (FREE Carpe Diem®) that included a spectrophotometric device reader, and measurement kits (BAP test) (Wismerll Co. Ltd.) were optimized to the FREE Carpe Diem® System according to the manufacturer's protocol. Briefly, 50 μl of chromogenic substrate (R2 kit reagent) and 1 ml of reactive solution (R1 kit reagent) were gently mixed in a cuvette, and absorbance at 505 nm was recorded. A 10 μl plasma sample was then immediately added to the cuvette. After mixing, the cuvette was immediately incubated in the thermostatic block of the analyzer for 5 min at 37°C, and absorbance at 505 nm was recorded. The results were expressed as mmol/L of reduced ferric ions (35, 36).
Dog's semen was collected into a sterile container by manual stimulation, while the dogs sniffed swabs of bitches in estrous. The semen collection was divided in three Falcon tubes, one for each part of the ejaculate: urethral, spermatic, and prostatic (37). The ejaculation analysis was performed as described by Alonge et al. (38). Only the spermatic part was analyzed by the Computer Assisted Sperm Analyzer (39) (CASA, IVOS-Sperm CASA system, Version 12.3, Hamilton Thorne, MA, USA). The CASA software (IVOS 12.3 version) was set up for canine semen specific parameters (Table 3). According to the manufacturer's instructions, for each analysis, a 3 μl drop from each sperm sample was diluted 5 times in Tris-Fructose extender and put on a Leja slide (four chambers) of 20 μm (Leja Products B.V. Nieuw Vennep, The Netherlands). The Leja slide was positioned in the dedicated chamber of the microscope, allowing it to settle for a few seconds before analysis. The CASA scanned five random non-consecutive microscopic fields. The parameters evaluated were total number of counted cells (TSC); semen concentration; total motility, and percentage of motile spermatozoa (progressive motility). Velocity average pathway (VAP) was elaborated by the software as average velocity of smoothed cell path, expressed in m/s. Then, the overall sperm population was divided into four groups, based on the velocity, according to low VAP cut-off (LVV) and medium VAP cut-off (MVV). Thus, sperms were classified as follows: rapid spermatozoa, with VAP > MVV; medium spermatozoa, with LVV < VAP < MVV; slow spermatozoa, with VAP < LVV; and static spermatozoa, represented by the fraction of those cells not moving during the analysis.
Each animal represented an experimental unit. All data sets were tested for normal distribution (Shapiro-Wilk) and variance homogeneity (Bartlett test). All parameters were subjected to analysis of variance (ANOVA) according to the General Linear Model (GLM) procedure as reported the following model:
where yijk represents all blood variables; μ is the overall mean; αi is the constant of the individual dog random effect (i = 1,…, 36); G represents the effect of the jth group (j = 1, 2), T was the effect of the kth time (k = 1, …, 4), G × T represents the binary interaction between the jth group and the kth time (1,…, 8). Significance was set at P < 0.05, and the results were expressed as means and mean standard error. All the analysis were per-formed using SAS software (40).
The results concerning the clinical biochemical profile of the dogs are reported in Tables 4, 5. Total protein, albumin, urea, glycemia, ALT, AST and ALP were affected by heat stress, time exposure and their binary interaction (P < 0.01). Serum total protein, albumin and urea concentrations decreased after 60 days in HS dogs (P < 0.01), showing lower values than TN (P < 0.01). Glycemia decreased as early as 30 days in HS dogs (P < 0.01), maintaining lower values than TN dogs from 30 to 90 days of trial (P < 0.01). The ALT, AST, and ALP concentration, instead, increased in HS dogs after 30 days of trial (P < 0.01), showing higher values than TN from 30 to 90 days of trial (P < 0.01). Cortisol values and oxidative profile results are reported in Table 6. Cortisol, TBARs, SOD, CAT and GSPx plasma concentration were affected by heat stress, time exposure and their binary interaction (P < 0.01). Cortisol plasma concentration increased constantly until 60 days of trial in HS dogs (P < 0.01) and then remained constant, showing higher values than TN from 30 to 90 days (P < 0.01). TBARs concentration increased at 30 days in HS dogs (P < 0.01), showing higher values than TN group from 30 to 90 days (P < 0.01). The SOD concentration decreased after 30 days of trial in HS dogs, with lower values than TN until the end of the trial (P < 0.01). Differently, CAT and GSPx concentration decreased after 60 days in HS dogs, with lower values than TN group (respectively P < 0.01 and P < 0.05). Bap values increased during time in HS animals, recording at 60 (P < 0.05) and 90 (P < 0.01) days higher values than previous experimental times. Moreover, at 60 (P < 0.05) and 90 (P < 0.01) days, these values were lower in HS dogs compared to TN ones. Differently, ROS values increased in spermatozoa of HS animals at 90 days compared to previous days and to TN animals (P < 0.01). Tables 7, 8 showed semen parameters. Semen concentration (P > 0.01) and TSN (P < 0.05) decreased after 30 days in HS dogs, and values remained lower than TN group until the end of the trial. Differently, VAP values did not show to be affected by time (P > 0.05), but HS dogs registered lower values than TN ones at 30 (P < 0.05), 60 and 90 days (P < 01). VCL values decreased at 90 days compared to 30 days in TN group (P < 0.01) and at 30 days in the HS group (P < 0.05) and then remains constant, with lower values in HS than TN dogs only at 30 days (P < 0.05). STR values of HS animals at 90 days were lower than what observed at 0, 30 (P < 0.01) and 60 days (P < 0.05) in same dogs and then TN dogs at same time (P < 0.01). The LIN values of HS dogs were lower at 90 days than 0 days of same dogs and then TN dogs at same time (P < 0.05). Total motility de-creased in HS dogs after 90 days compared to 0 and 30 days (P < 0.01). These animals showed lower values at 60 and 90 days compared to TN dogs (P < 0.01). Also, progressive motility decreased in HS dogs during time, with lower values at 60 days (P < 0.05) and 90 days (P < 0.01) compared to 0 and 30 days. Moreover, from 60 to 90 days HS animals showed lower progressive motility than TN ones (P < 0.01). Rapid movements observed in HS animals decreased at 30, 60 (P < 0.05) and 90 days (P < 0.01) compared to 0 days, with lower values from 30 to 90 days than what registered in TN animals (P < 0.01). Contrarily, slow movements increased in HS animals just after 30 days (P < 0.01) and remained constant with higher values than TN dogs from 30 to 90 days (P < 0.01). Static movements, instead, were constantly higher in HS animals from 30 to 90 days of the trial (P < 0.05).
Table 4. Total protein, albumin, urea, uric acid, creatinine, bilirubin, triglyceride, glycemia, alanine amino transferase (ALT), aspartate amino transferase (AST), alkaline phosphatase (ALP), and cholesterol serum concentration in dogs in thermoneutrality (TN) and heat stress (HS) condition for 90 days.
Table 5. Chlorine, sodium, potassium, magnesium, phosphorus, and calcium serum concentration in dogs in thermoneutrality (TN) and heat stress (HS) condition for 90 days.
Table 6. Thiobarbituric acid reactive substances (TBARs), hydroperoxides, protein carbonyls, ferric reducing antioxidant power (FRAP), superoxide dismutase (SOD), catalase (CAT), and glutathione peroxidase (GSPx) serum concentration in dogs in thermoneutrality (TN) and heat stress (HS) condition for 90 days.
Table 7. Volume, concentration, total sperm number (TSN), velocity average pathway (VAP), straight line velocity (VLS), curvilinear velocity (VCL), amplitude of lateral head displacement (ALH), beat-cross frequency (BCF), straightness (STR), and linearity (LIN) of second fraction of ejaculate in dog in thermoneutrality (TN) and heat stress (HS) condition at 0, 30, 60 and 90 days after the starting of the experimentation.
Table 8. Total motility, progressive motility, rapid, medium, slow, and static movements of spermatozoa in dog in thermoneutrality (TN) and heat stress (HS) condition for at 0, 30, 60 and 90 days after the starting of the experimentation.
The aim of this study was to evaluate the effects of high environmental temperatures on the clinical and biochemical parameters, oxidative status, and sperm parameters of dogs. It is well-known that thermal stress affects the biological functioning of various organs, resulting in a decrease in their overall performance (41). In this study, the data obtained from the serum biochemical and electrolyte profiles showed that some parameters were affected by heat stress, although they never showed values over the physiological range of the species (42, 43). As regarding serum biochemical profile, total protein, albumin, glycemia, ALT, AST and ALP were affected by heat stress. In particular, total protein, albumin, urea and glycemia reduced in HS dogs, while ALT, AST, and ALP increased after heat exposition. These variations are due to the direct impact of high temperatures on the body's thermal regulation process. The decrease of total protein and albumin could be due to thermal injury that cause cellular necrosis through protein denaturation (44). Following peripheric vasodilation, there is a constriction of capillaries in internal organs like the liver. This constriction can lead to liver cell damage, reflected in increased concentrations of AST and ALT in animals exposed to thermal stress (45). Moreover, heat stress induced hypoglycemia, as just reported by other authors, due to higher metabolism. It increased dilatation of blood vessels and consequently increased absorption of insulin (46, 47). In addition to all this, chloride levels and urea levels have also changed, showing a decrease under heat stress conditions. Dogs in such circum-stances tend to drink more, leading to overhydration and hence these results (48). An increase in cortisol levels was noticed as a reaction to heat stress (49). Circulating cortisol levels have been recognized as a highly sensitive indicator of heat stress due to decreased tolerance to extreme climates. Dogs experiencing heat stress have been observed to exhibit cortisol levels exceeding the normal range (50). The increasing values of cortisol observed in this study indicate that dogs were experiencing the heat stress effects (46). It is known that heat also induces oxidative stress (8, 51). Also in this study, it was demonstrated that the parameters of oxidative stress (TBARs and ROS) were increased in HS groups, while antioxidants (SOD, CAT, GSPx, and BAP) were reduced. This is indicative of oxidative stress, that is the unbalance between the production of oxidation by-products and reduction of antioxidant enzymatic activity (52). Currently, there are no studies that can substantiate such evidence because, based on our current knowledge, no researcher has yet studied the oxidative stress during heat stress in dogs. Considering the damage caused by testicular heat stress, it is important to investigate the alterations caused by heat stress in sperm development to improve animal welfare in dogs living outdoors in the summer months. In other species (bovines, rabbits, mice), instead, it was demonstrated how the heat stress induces oxidative stress and interferes with reproduction (53–55). In livestock industry, global warming has negatively influenced animal production performance, in addition to their wellbeing and welfare. In the present study, heat induced-oxidative stress affected the sperm parameters. Prior to heat stress (T0), every parameter examined in both group of dogs (volume, motility, vigor, sperm concentration, total sperm count, and morphology) fell within the range deemed as normal (21, 24). In the summer months, with an increase in THI, the sperm parameters worsen. It was demonstrated that ROS, produced after scrotal exposure to ambient high temperatures, can stop spermatogenesis (22, 56). The decrease in sperm concentration, velocity average pathway, straight line velocity, curvilinear velocity, amplitude of lateral head displacement, straightness, and linearity is a result of heat stress-induced generation of reactive oxygen species, which causes cellular damage and the start of apoptosis (17, 57). Apoptosis may result in sperm cells death when heat stress acts on the testes (58). This study has highlighted how heat stress can alter the oxidative status of male dogs, promoting increased production of oxidative catabolites and reducing antioxidant enzyme activity. This is also observed at the spermatic level with an increased production of ROS. In fact, the current study demonstrates the detrimental effect of high environmental temperatures on sperm quality in dog. This occurs due to the direct effect of heat on spermatozoa and the production of ROS which damage sperm cells.
The negative impact of a heat stress on quantitative and qualitative sperm traits has been demonstrated in a variety of species (59, 60). In bulls, a notable decrease in sperm motility (MOT), velocity (VEL), viability (VIA), acrosome integrity (ACR), and progressive motility index (PMI) has been documented during summer, accompanied by an increase in sperm abnormalities, particularly in the head, midpiece, and tail regions (61). Similarly, a morphology analysis conducted on Bos taurus and Bos indicus exposed to an environmental temperature of 40°C revealed an increase in misshapen sperm heads (especially pyriform heads), decapitated spermatozoa, and bent tails (62). Likewise, cats exhibited the poorest seminal quality on high-temperature days (63). The negative impact of heat stress on sperm quality has also been reported in rams (64), boars (65), and men (66). Consistent with these findings, we observed a detrimental effect of heat stress on sperm motility, velocity, sperm morphology, and viability in dogs. To the best of our knowledge, this is the first report demonstrating the effective-ness of heat stress in assessing the degree of stress induced by climatic conditions in dogs. Although extensively studied in farm animals to mitigate economic losses, research on this topic has been limited in wild animals and pets. In wild animals, studies have focused on ensuring the wellbeing and welfare of captive animals, addressing behaviors, habitat enrichment, or stressors. However, little is known about heat stress and temperature thresholds for many wild species (67). There exists a temperature threshold, influenced by the duration of exposure, beyond which germ cell degeneration occurs, as demonstrated in mice and dogs (17). There are several factors that influence seminal testosterone and semen production in dogs, for example the characteristics of the subject, the length of the day, the environmental humidity (68). All these factors were standardized in the two groups, to show the effect of temperature alone in the selected dogs. This study has limitations: the imperfect standardization of the groups, with physical and sperm production differences in the between them. Furthermore, it was not possible to follow the dogs for some months after the end of the trial to have more data after the summer season.
This study has highlighted how heat stress can alter the oxidative status of male dogs, leading to increased production of oxidative catabolites and a reduction in antioxidant enzyme activity. This phenomenon is also observed at the spermatic level, with a marked increase in the production of ROS. In fact, the current research demonstrates the detrimental effect of high environmental temperatures on sperm quality in dogs. This occurs due to the direct impact of heat on spermatozoa, coupled with the increased production of ROS, which damages sperm cells. The effect was observed in animals exposed to high temperatures during the summer months in which the study was conducted, leading to a decrease in reproductive performance in male dogs kept outdoors and subjected to extreme heat. It is important to note, however, that one limitation of the study is the lack of complete homogeneity among the groups in terms of breed. While the study provides valuable insights into the impact of heat stress on oxidative status and sperm quality in dogs, further research is required to gain a deeper understanding of these effects.
The raw data supporting the conclusions of this article will be made available by the authors, without undue reservation.
The animal studies were approved by Institutional Review Board approval number 656/18. The studies were conducted in accordance with the local legislation and institutional requirements. Written informed consent was obtained from the owners for the participation of their animals in this study.
MB: Formal analysis, Methodology, Software, Writing – original draft. LF: Data curation, Formal analysis, Methodology, Software, Writing – original draft. AP: Data curation, Formal analysis, Methodology, Software, Writing – original draft. AM: Conceptualization, Funding acquisition, Investigation, Project administration, Resources, Validation, Writing – review & editing. PD: Supervision, Validation, Visualization, Writing – review & editing. GA: Methodology, Supervision, Visualization, Writing – review & editing. AR: Conceptualization, Funding acquisition, Validation, Writing – review & editing. AC: Data curation, Formal analysis, Methodology, Software, Writing – original draft. GL: Supervision, Validation, Visualization, Writing – review & editing. VC: Investigation, Project administration, Resources, Writing – review & editing.
The author(s) declare that no financial support was received for the research, authorship, and/or publication of this article.
The authors are grateful to Dr. Giovanna Calzaretti for her technical support.
The authors declare that the research was conducted in the absence of any commercial or financial relationships that could be construed as a potential conflict of interest.
All claims expressed in this article are solely those of the authors and do not necessarily represent those of their affiliated organizations, or those of the publisher, the editors and the reviewers. Any product that may be evaluated in this article, or claim that may be made by its manufacturer, is not guaranteed or endorsed by the publisher.
1. Palatnik-de-Sousa CB, Day MJ. One Health: the global challenge of epidemic and endemic leishmaniasis. Parasit Vect. (2011) 4:197. doi: 10.1186/1756-3305-4-197
2. Rossati A. Global warming and its health impact. Int J Occup Environ Med. (2017) 8:7–20. doi: 10.15171/ijoem.2017.963
3. Paital B, Panda SK, Hati AK, Mohanty B, Mohapatra MK, Kanungo S, et al. Longevity of animals under reactive oxygen species stress and disease susceptibility due to global warming. World J Biol Chem. (2016) 7:110–27. doi: 10.4331/wjbc.v7.i1.110
4. Ma J, Zhou L, Foltz GR, Qu X, Ying J, Tokinaga H, et al. Hydrological cycle changes under global warming and their effects on multiscale climate variability. Ann N Y Acad Sci. (2020) 1472:21–48. doi: 10.1111/nyas.14335
5. Rockström J, Steffen W, Noone K, Persson Å, Chapin FS, Lambin EF, et al. A safe operating space for humanity. Nature. (2009) 461:472–5. doi: 10.1038/461472a
6. Hansen PJ. Physiological and cellular adaptations of zebu cattle to thermal stress. Anim Reprod Sci. (2004) 82–3:349–60. doi: 10.1016/j.anireprosci.2004.04.011
7. Ravagnolo O, Misztal I, Hoogenboom G. Genetic component of heat stress in dairy cattle, development of heat index function. J Dairy Sci. (2000) 83:2120–5. doi: 10.3168/jds.S0022-0302(00)75094-6
8. Belhadj Slimen I, Najar T, Ghram A, Dabbebi H, Ben Mrad M, Abdrabbah M. Reactive oxygen species, heat stress and oxidative-induced mitochondrial damage. A review. Int J Hypertherm. (2014) 30:513–23. doi: 10.3109/02656736.2014.971446
9. Wang Y, Yang C, Elsheikh NAH Li C, Yang F, Wang G, et al. HO-1 reduces heat stress-induced apoptosis in bovine granulosa cells by suppressing oxidative stress. Aging. (2019) 11:5535–47. doi: 10.18632/aging.102136
10. Rizzoto G, Boe-Hansen G, Klein C, Thundathil JC, Kastelic JP. Acute mild heat stress alters gene expression in testes and reduces sperm quality in mice. Theriogenology. (2020) 158:375–81. doi: 10.1016/j.theriogenology.2020.10.002
11. Xavier MJ, Nixon B, Roman SD, Scott RJ, Drevet JR, Aitken RJ. Paternal impacts on development: identification of genomic regions vulnerable to oxidative DNA damage in human spermatozoa. Hum Reprod. (2019) 34:1876–90. doi: 10.1093/humrep/dez153
12. Kaushik K, Kaushal N, Mittal PK, Kalla NR. Heat induced differential pattern of DNA fragmentation in male germ cells of rats. J Therm Biol. (2019) 84:351–6. doi: 10.1016/j.jtherbio.2019.07.021
13. Stravogianni V, Samaras T, Boscos CM, Markakis J, Krystallidou E, Basioura A, et al. The use of animal's body, scrotal temperature and motion monitoring in evaluating boar semen production capacity. Animals. (2022) 12:829. doi: 10.3390/ani12070829
14. Morrell JM. Heat stress and bull fertility. Theriogenology. (2020) 153:62–7. doi: 10.1016/j.theriogenology.2020.05.014
15. Barragán AL, Avendaño-Reyes L, Mellado-Bosque M, Meza-Herrera CA, Vicente-Pérez R, Castañeda VJ, et al. Seasonal heat stress compromises testicular thermoregulation and semen quality of Dorper rams raised in a desert climate. J Therm Biol. (2023) 118:103737. doi: 10.1016/j.jtherbio.2023.103737
16. Shakeel M, Yoon M. Heat stress and stallion fertility. J Anim Sci Technol. (2023) 65:683–97. doi: 10.5187/jast.2023.e29
17. Henning H, Masal C, Herr A, Wolf K, Urhausen C, Beineke A, et al. Effect of short-term scrotal hyperthermia on spermatological parameters, testicular blood flow and gonadal tissue in dogs. Reprod Domest Anim. (2014) 49:145–57. doi: 10.1111/rda.12244
18. Pham S, Schultz JS. Testicular thermoregulation with respect to spermatogenesis and contraception. J Therm Biol. (2021) 99:102954. doi: 10.1016/j.jtherbio.2021.102954
19. Senger PL. Pathways to Pregnancy and Parturition. Pullman, WA: Current Conceptions, Inc. (1997).
20. Hansen PJ. Effects of heat stress on mammalian reproduction. Philos Trans R Soc Lond B Biol Sci. (2009) 364:3341–50. doi: 10.1098/rstb.2009.0131
21. Lyrio LL, Lazaro MA, Sonegheti R, Moulin L, Coslop L, Sarto CG, et al. Effects of heat stress on sperm quality of French Bulldogs. Theriogenology. (2023) 199:131–7. doi: 10.1016/j.theriogenology.2023.01.023
22. Jannes P, Spiessens C, Van Der Auwera I, D'Hooghe T, Verhoeven G, Vanderschueren D. Male subfertility induced by acute scrotal heating affects embryo quality in normal female mice. Hum Reprod. (1998) 13:372–5. doi: 10.1093/humrep/13.2.372
23. Shadmehr S, Fatemi Tabatabaei SR, Hosseinifar S, Tabandeh MR, Amiri A. Attenuation of heat stress-induced spermatogenesis complications by betaine in mice. Theriogenology. (2018) 106:117–26. doi: 10.1016/j.theriogenology.2017.10.008
24. Kastelic JP, Rizzoto G, Thundathil J. Review: testicular vascular cone development and its association with scrotal thermoregulation, semen quality and sperm production in bulls. Animal. (2018) 12:s133–41. doi: 10.1017/S1751731118001167
25. Maggiolino A, Landi V, Bartolomeo N, Bernabucci U, Santus E, Bragaglio A, et al. Effect of heat waves on some Italian Brown Swiss Dairy Cows' production patterns. Front Anim Sci. (2022) 2:800680. doi: 10.3389/fanim.2021.800680
26. Aiudi GG, Cicirelli V, Maggiolino A, Burgio M, Bragaglio A, Tateo A, et al. Effect of Pinus taeda hydrolyzed lignin on biochemical profile, oxidative status, and semen quality of healthy dogs. Front Vet Sci. (2022) 9:866112. doi: 10.3389/fvets.2022.866112
27. De Palo P, Maggiolino A, Albenzio M, Casalino E, Neglia G, Centoducati G, et al. Survey of biochemical and oxidative profile in donkey foals suckled with one natural and one semi-artificial technique. PLoS ONE. (2018) 13:e0198774. doi: 10.1371/journal.pone.0198774
28. Gold AJ, Langlois DK, Refsal KR. Evaluation of basal serum or plasma cortisol concentrations for the diagnosis of hypoadrenocorticism in dogs. J Vet Intern Med. (2016) 30:1798–805. doi: 10.1111/jvim.14589
29. Faure P, Lafond JL. Measurement of plasma sulfhydryl and carbonyl groups as a possible indicator of protein oxidation. In:Favier AE, Cadet J, Kalyanaraman B, Fontecave M, Pierre JL, , editors. Analysis of Free Radicals in Biological Systems. Basel: Birkhäuser Basel (1995). p. 237–48.
30. Forte L, De Palo P, Natrella G, Aloia A, Maggiolino A. Effects of dry and wet aging on culled goat meat quality. Ital J Anim Sci. (2024). doi: 10.1080/1828051X.2024.2329708
31. Maggiolino A, Lorenzo J, Centoducati G, Domínguez R, Dinardo FR, Marino R, et al. How volatile compounds, oxidative profile and sensory evaluation can change with vacuum aging in Donkey Meat. Animals. (2020) 10:2126. doi: 10.3390/ani10112126
32. Misra HP. Adrenochrome assay. In:Greenwald RA, , editor. Handbook of Methods for Oxygen Radical Research. Boca Raton, FL: CRC Press (1985). p. 237–41.
33. Clairborne A. Catalase activity. In:Press C, , editor. Handbook of Methods for Oxygen Radical Research. Boca Raton, FL (1985). p. 283–4.
34. Dinardo FR, Maggiolino A, Martinello T, Liuzzi GM, Elia G, Zizzo N, et al. Oral administration of nucleotides in calves: Effects on oxidative status, immune response, and intestinal mucosa development. J Dairy Sci. (2022). doi: 10.3168/jds.2021-20804
35. Sciorsci RL, Galgano M, Mutinati M, Rizzo A. Oxidative state in the estrus cycle of the buffaloes: a preliminary study. Trop Anim Health Prod. (2020) 52:1331–4. doi: 10.1007/s11250-019-02133-4
36. Tufarelli V, Rizzo A, Lacalandra G, Guaricci A, Laudadio V, Valentini L. Effects of the supplementation with an high-polyphenols extra-virgin olive oil on kinetic sperm features and seminal plasma oxidative status in healthy dogs. Reprod Domest Anim. (2018) 53:582–7. doi: 10.1111/rda.13145
37. Cicirelli V, Macrì F, Di Pietro S, Leoci R, Lacalandra GM, Aiudi GG. Use of contrast-enhanced ultrasound of the testes after non-surgical sterilization of male dogs with CaCl2 in alcohol. Animals. (2022) 12:577. doi: 10.3390/ani12050577
38. Alonge S, Melandri M, Leoci R, Lacalandra GM, Caira M, Aiudi GG. The effect of dietary supplementation of vitamin E, selenium, zinc, folic acid, and N-3 polyunsaturated fatty acids on sperm motility and membrane properties in dogs. Animals. (2019) 9:34. doi: 10.3390/ani9020034
39. Cicirelli V, Accogli G, Caira M, Lacalandra GM, Aiudi G. Use of ‘Aminogam Gel' to fast the wound healing in dogs after the surgical curettage of injured penis. Vet Med Sci. (2022) 8:1001–7. doi: 10.1002/vms3.769
41. Jankair M, Al-Joubori M. Study of the effect of high temperature in a number of biochemical variables in the serum of exposed workers. J Educ Sci. (2007) 19:95–110. doi: 10.33899/edusj.2007.51021
42. Kaneko JJ. Serum Proteins and the Dysproteinemias. Clinical Biochemistry of Domestic Animals. Elsevier (1997). p. 117–38.
43. Martineau A-S, Leray V, Lepoudere A, Blanchard G, Bensalem J, Gaudout D, et al. A mixed grape and blueberry extract is safe for dogs to consume. BMC Vet Res. (2016) 12:162. doi: 10.1186/s12917-016-0786-5
44. Hagiu BG, Codreanu I. Study concerning the thermal stress impact on hematological and biochemical parameters in dogs. Sci Works Ser C Vet Med. (2022) 68:22–6.
45. Al-Hashimi A. Impact of thermal stress on health signs, hormone levels, haematological and biochemical parameters of police dogs in Iraq. Adv Anim Vet Sci. (2019) 7:340–5. doi: 10.17582/journal.aavs/2019/7.5.340.345
46. Azeez OM, Olaifa FH, Adah AS, Basiru A, Akorede GJ, Ambali HM, et al. Effect of heat stress on vital and hematobiochemical parameters of healthy dogs. Vet World. (2022) 15:722–7. doi: 10.14202/vetworld.2022.722-727
47. Kanter GS. Cause of hypoglycemia in dogs exposed to heat. Am J Physiol. (1959) 196:619–24. doi: 10.1152/ajplegacy.1959.196.3.619
48. Goggs R, De Rosa S, Fletcher DJ. Electrolyte disturbances are associated with non-survival in dogs-a multivariable analysis. Front Vet Sci. (2017) 4:135. doi: 10.3389/fvets.2017.00135
49. Sundman AS, Van Poucke E, Svensson Holm AC, Faresjö Å, Theodorsson E, Jensen P, et al. Long-term stress levels are synchronized in dogs and their owners. Sci Rep. (2019) 9:7391. doi: 10.1038/s41598-019-43851-x
50. Assia E, Epstein Y, Magazanik A, Shapiro Y, Sohar E. Plasma-cortisol levels in experimental heatstroke in dogs. Int J Biometeorol. (1989) 33:85–8. doi: 10.1007/BF01686283
51. Ngoula F, Lontio FA, Tchoffo H, Manfo Tsague FP, Djeunang R-M, Vemo BN, et al. Heat induces oxidative stress: reproductive organ weights and serum metabolite profile, testes structure, and function impairment in male Cavy (Cavia porcellus). Front Vet Sci. (2020) 7:37. doi: 10.3389/fvets.2020.00037
52. Rizzo A, Roscino M, Binetti F, Sciorsci R. Roles of reactive oxygen species in female reproduction. Reprod Domest Anim. (2012) 47:344–52. doi: 10.1111/j.1439-0531.2011.01891.x
53. Bernabucci U, Ronchi B, Lacetera N, Nardone A. Markers of oxidative status in plasma and erythrocytes of transition dairy cows during hot season1. J Dairy Sci. (2002) 85:2173–9. doi: 10.3168/jds.S0022-0302(02)74296-3
54. Oghbaei H, Hosseini L, Farajdokht F, Rahigh Aghsan S, Majdi A, Sadigh-Eteghad S, et al. Heat stress aggravates oxidative stress, apoptosis, and endoplasmic reticulum stress in the cerebellum of male C57 mice. Mol Biol Rep. (2021) 48:5881–7. doi: 10.1007/s11033-021-06582-9
55. Mutwedu VB, Nyongesa AW, Oduma JA, Kitaa JM, Mbaria JM. Thermal stress causes oxidative stress and physiological changes in female rabbits. J Therm Biol. (2021) 95:102780. doi: 10.1016/j.jtherbio.2020.102780
56. Banks S, King SA, Irvine DS, Saunders PT. Impact of a mild scrotal heat stress on DNA integrity in murine spermatozoa. Reproduction. (2005) 129:505–14. doi: 10.1530/rep.1.00531
57. Ozawa M, Hirabayashi M, Kanai Y. Developmental competence and oxidative state of mouse zygotes heat-stressed maternally or in vitro. Reproduction. (2002) 124:683–9. doi: 10.1530/rep.0.1240683
58. Pérez-Crespo M, Pintado B, Gutiérrez-Adán A. Scrotal heat stress effects on sperm viability, sperm DNA integrity, and the offspring sex ratio in mice. Mol Reprod Dev. (2008) 75:40–7. doi: 10.1002/mrd.20759
59. Shahat AM, Rizzoto G, Kastelic JP. Amelioration of heat stress-induced damage to testes and sperm quality. Theriogenology. (2020) 158:84–96. doi: 10.1016/j.theriogenology.2020.08.034
60. Boni R. Heat stress, a serious threat to reproductive function in animals and humans. Mol Reprod Dev. (2019) 86:1307–23. doi: 10.1002/mrd.23123
61. Garcia-Oliveros LN, de Arruda RP, Batissaco L, Gonzaga VHG, Nogueira VJM, Florez-Rodriguez SA, et al. Heat stress effects on bovine sperm cells: a chronological approach to early findings. Int J Biometeorol. (2020) 64:1367–78. doi: 10.1007/s00484-020-01917-w
62. Skinner JD, Louw GN. Heat stress and spermatogenesis in Bos indicus and Bos taurus cattle. J Appl Physiol. (1966) 21:1784–90. doi: 10.1152/jappl.1966.21.6.1784
63. Nuñez Favre R, García MF, Rearte R, Stornelli MC, Corrada YA, de la Sota RL, et al. Heat stress and sperm production in the domestic cat. Theriogenology. (2022) 187:182–7. doi: 10.1016/j.theriogenology.2022.05.004
64. Alves MBR, Andrade AFCd, Arruda RPd, Batissaco L, Florez-Rodriguez SA, Oliveira BMMd, et al. Recovery of normal testicular temperature after scrotal heat stress in rams assessed by infrared thermography and its effects on seminal characteristics and testosterone blood serum concentration. Theriogenology. (2016) 86:795–805.e2. doi: 10.1016/j.theriogenology.2016.02.034
65. Kunavongkrit A, Suriyasomboon A, Lundeheim N, Heard TW, Einarsson S. Management and sperm production of boars under differing environmental conditions. Theriogenology. (2005) 63:657–67. doi: 10.1016/j.theriogenology.2004.09.039
66. Zhang M-H, Zhai L-P, Fang Z-Y, Li A-N, Xiao W, Qiu Y. Effect of scrotal heating on sperm quality, seminal biochemical substances, and reproductive hormones in human fertile men. J Cell Biochem. (2018) 119:10228–38. doi: 10.1002/jcb.27365
67. Morgan KN, Tromborg CT. Sources of stress in captivity. Appl Anim Behav Sci. (2007) 102:262–302. doi: 10.1016/j.applanim.2006.05.032
68. Albrizio M, Siniscalchi M, Sasso R, Quaranta A. Effects of the environment on dog semen parameters and testosterone concentration. Theriogenology. (2013) 80:800–4. doi: 10.1016/j.theriogenology.2013.07.005
69. Xiong Y, Qing-Shi M, Jie GAO, Tang X, Zhang H. Effects of relative humidity on animal health and welfare. J Integr Agric. (2017) 16:1653–8. doi: 10.1016/S2095-3119(16)61532-0
70. Maggiolino A, Dahl GE, Bartolomeo N, Bernabucci U, Vitali A, Serio G, et al. De Palo, P. Estimation of maximum thermo-hygrometric index thresholds affecting milk production in Italian Brown Swiss cattle. J Dairy Sci. (2020) 103:8541–53. doi: 10.3168/jds.2020-18622
71. Aloia A, Maggiolino A, Forte L, De Palo P. Heat stress measuring methods in dairy cows. Acta IMEKO. (2024) 13:1–5. doi: 10.21014/actaimeko.v13i1.1619
Keywords: heat stress, sperm quality dog, ROS, biochemical profile, antioxidant potential
Citation: Burgio M, Forte L, Prete A, Maggiolino A, De Palo P, Aiudi GG, Rizzo A, Carbonari A, Lacalandra GM and Cicirelli V (2024) Effects of heat stress on oxidative balance and sperm quality in dogs. Front. Vet. Sci. 11:1445058. doi: 10.3389/fvets.2024.1445058
Received: 06 June 2024; Accepted: 09 September 2024;
Published: 26 September 2024.
Edited by:
Khalid El Allali, Agronomic and Veterinary Institute Hassan II, MoroccoReviewed by:
Ejaz Ahmad, Bahauddin Zakariya University, PakistanCopyright © 2024 Burgio, Forte, Prete, Maggiolino, De Palo, Aiudi, Rizzo, Carbonari, Lacalandra and Cicirelli. This is an open-access article distributed under the terms of the Creative Commons Attribution License (CC BY). The use, distribution or reproduction in other forums is permitted, provided the original author(s) and the copyright owner(s) are credited and that the original publication in this journal is cited, in accordance with accepted academic practice. No use, distribution or reproduction is permitted which does not comply with these terms.
*Correspondence: Lucrezia Forte, bHVjcmV6aWEuZm9ydGVAdW5pYmEuaXQ=; Alice Carbonari, YWxpY2UuY2FyYm9uYXJpQHVuaWJhLml0
Disclaimer: All claims expressed in this article are solely those of the authors and do not necessarily represent those of their affiliated organizations, or those of the publisher, the editors and the reviewers. Any product that may be evaluated in this article or claim that may be made by its manufacturer is not guaranteed or endorsed by the publisher.
Research integrity at Frontiers
Learn more about the work of our research integrity team to safeguard the quality of each article we publish.