- 1Department of Specialty Medicine, College of Veterinary Medicine, Midwestern University, Glendale, AZ, United States
- 2Department of Veterinary Medicine and Surgery, College of Veterinary Medicine, University of Missouri, Columbia, MO, United States
- 3Department of Pathology and Population Medicine, College of Veterinary Medicine, Midwestern University, Glendale, AZ, United States
- 4Department of Physiology, College of Graduate Studies, Midwestern University, Glendale, AZ,United States
Naturally occurring diabetes mellitus (NODM) is one of the most common endocrine disorders in dogs and its etiology closely resembles type 1 diabetes mellitus (T1DM) in people. Human patients with T1DM commonly have cellular derangements consistent with inflammation, impaired immune function, and hypovitaminosis D. There is little information available regarding inflammatory biomarkers, immune function, and vitamin D status in diabetic dogs. Therefore, our objectives were to assess inflammatory biomarkers, vitamin D metabolites, and phagocytic capacity in diabetic dogs and determine whether associations exist with these variables and the level of clinical control or vitamin D metabolites. This was a prospective case–control study that included 20 otherwise healthy diabetic dogs (clinically controlled, n = 10; uncontrolled, n = 10) and 20 non-diabetic, healthy, age (± 2 years), breed, and sex matched controls. Complete blood count, biochemical panel, urinalysis, and fructosamine were performed at a single commercial reference laboratory. Basal plasma tumor necrosis factor (TNF)-α, interleukin (IL)-6, IL-8, and IL-10 were measured using a canine-specific multiplex bead-based assay. Serum C-reactive protein (CRP) was measured using a commercially available ELISA kit. Serum 25-hydroxyvitamin (OH)D3 and 24,25-dihydroxyvitamin (OH)2D3 were measured with HPLC. Phagocytosis of opsonized-Escherichia coli (E. coli) was evaluated with flow cytometry. Diabetic dogs had higher serum CRP concentrations than controls (p = 0.02). Plasma IL-8 concentrations were higher in diabetic dogs with uncontrolled clinical disease compared to controls (p = 0.02). Diabetic dogs had a lower percentage of leukocytes that phagocytized opsonized-E. coli (p = 0.02), but an increased number of bacteria phagocytized per cell (p < 0.001) compared to controls. No between-group differences were identified in vitamin D metabolites, nor were associations found between vitamin D and any variables. Fructosamine had a positive association with serum CRP concentration (rho = 0.35, p = 0.03) and number of bacteria phagocytized per cell (rho = 0.45, p = 0.004) in our cohort (n = 40). Like people with T1DM, diabetic dogs have a proinflammatory phenotype and phagocytic dysregulation that may be correlated with glycemic control.
Introduction
Diabetes mellitus is one of the most common endocrine disorders in middle-to older-aged dogs with a prevalence in the pet population worldwide that ranges from 0.2 to 1.3% (1–9). Diabetes mellitus is characterized by persistent hyperglycemia because of reduced pancreatic β-cell secretion of insulin, insulin sensitivity in peripheral tissues, or both. The most commonly recognized form of naturally occurring diabetes mellitus (NODM) in dogs is similar to type 1 diabetes mellitus (T1DM) in people and is characterized by hypoinsulinemia and a dependence on exogenous insulin administration to maintain euglycemia, mitigate ketosis, and survive (10). Common clinical signs demonstrated by diabetic dogs include one or more of polyuria, polydipsia, polyphagia, and weight loss (11).
Diabetic complications in human patients are related to protracted hyperglycemia and resultant downstream effects which contribute to cytokine dysregulation characterized by a proinflammatory state and immune dysfunction (12–20). Furthermore, proinflammatory cytokines directly and indirectly contribute to insulin resistance that negatively affects glycemic control in people with T1DM (17, 21, 22). Therefore, it is intuitive that proinflammatory biomarkers, including C-reactive protein (CRP) and cytokines, have been shown to be useful adjunctive tools to assess glycemic control and predict risk for diabetic related complications in human patients (16, 23, 24). There is a fraction of available information regarding the inflammatory state and immune function in dogs with NODM despite similar risks for infectious and inflammatory disorders and the development of insulin resistance (25–28). Expanding our knowledge of the inflammatory milieu and immune function in diabetic dogs will improve our understanding of this endocrinopathy.
Numerous studies have demonstrated a link between T1DM in people with hypovitaminosis D and direct comparisons of absolute blood 25-hydroxyvitamin (OH)D concentrations are consistently lower than non-diabetic controls (29–31). Several studies have shown that hypovitaminosis D at different life stages is associated with the eventual development of T1DM in human patients (32–35). These results suggest that vitamin D status may be considered an environmental risk factor for T1DM in people, especially at some life stages. However, hypovitaminosis D in some diabetic patients may be multifactorial or exclusively a result of the diabetic syndrome rather than causal. Regardless of whether vitamin D is a contributory factor or consequence, understanding the vitamin D status in diabetic people has proven useful because of its association with glycemic control and development of complications (36–38). Moreover, vitamin D supplementation in people with T1DM decreases proinflammatory biomarkers and improves glycemic control, although conflicting reports exist (39–41).
This prospective case–control study had three objectives (i) to compare granulocyte/monocyte (GM) phagocytic capacity of Escherichia coli (E. coli), concentrations of serum vitamin D metabolites, CRP, and basal plasma cytokines in diabetic dogs and non-diabetic healthy controls, (ii) assess whether clinical control of diabetes mellitus affects these variables, and (iii) evaluate whether associations exist between vitamin D metabolites and inflammatory biomarkers or phagocytic capacity. We hypothesized that diabetic dogs would have higher inflammatory biomarkers, phagocytic dysregulation, and altered vitamin D metabolites compared to controls and differences would be magnified in diabetic dogs with uncontrolled clinical signs. In addition, we hypothesized that one or more vitamin D metabolites would be associated with ≥1 of serum CRP, plasma cytokine, or phagocytic capacity of E. coli.
Materials and methods
Animals and study design
Client-owned dogs with NODM that were otherwise healthy and treated with ≥0.25 units/kg of insulin administered once every 12 h were eligible for inclusion. Diagnosis of diabetes mellitus was performed according to criteria outlined in the 2018 AAHA Diabetes Management for Dogs and Cats (11). Specifically, the presence of hyperglycemia, glucosuria, and ≥ 1 related clinical sign (e.g., polyuria, polydipsia, polyphagia, and weight loss). Diabetic dogs were classified as having clinically controlled diabetes mellitus if the dog exhibited no polyuria, polydipsia, polyphagia, and there had been no dose adjustments to insulin within 4 weeks of enrollment. Therefore, dogs were considered to have uncontrolled diabetes mellitus if they exhibited ≥1 of related clinical signs without a rationale alternative cause. Exclusion criteria included obesity (i.e., body condition score (BCS) ≥ 6/9), vaccination within 1-month, concurrent illness within 2 months of enrollment, or presence of a clinically relevant comorbid disorder. A board-certified small animal internist (JAJ) determined whether a comorbid condition was clinically relevant. A second population of healthy, age (i.e., ± 2 years), breed, and sex-matched non-diabetic healthy control dogs were enrolled. Control dogs were included after review of clinical history, physical examination, complete blood count, urinalysis, and serum chemistry by a single investigator (JAJ). Control dogs were enrolled in the study if they met the following requirements: non-obese, no illnesses within 6 months of enrollment, and had not received any vaccinations within 1 month of enrollment. The study protocol was approved by the Midwestern University Animal Care and Use Committee (protocol #2944) with written owner consent. Dogs were enrolled from December 2019 to July 2020. Dogs included in this study were used in a separate study focused on the ex vivo effects of calcitriol on several immunological variables in diabetic dogs (42).
Data and sample collection
Medical records were reviewed for each dog enrolled. The age, sex, weight, BCS, and breed were recorded. Other salient details from the medical records included: maintenance diet information, date of initial diabetes mellitus diagnosis, insulin type, and dose. Hematology, serum biochemistry, serum fructosamine, and urinalysis tests from the day of enrollment were measured at a commercial laboratory (Antech Diagnostics, United States).
Blood was collected from a jugular or peripheral vein and transferred into serum separator blood tubes and lithium heparin anticoagulated blood tubes. Blood from the lithium heparin anticoagulated blood tube was processed within 1 h of collection for experiments related to phagocytosis. The remaining lithium heparin anticoagulated blood was centrifuged, and plasma transferred to freezer-resistant conical microcentrifuge tubes and stored at −80°C for batch analysis of cytokines. Similarly, blood in the serum separator blood tubes was allowed to clot and then centrifuged with serum transferred to freezer-resistant conical microcentrifuge tubes and stored at −80°C for batch analysis of 25-hydroxyvitamin (OH)D3, 24,25-dihydroxyvitamin (OH)2D3, and CRP.
Constitutive plasma cytokine concentrations
Plasma samples were thawed, after which tumor necrosis factor (TNF)-α, interleukin (IL)-6, IL-8, and IL-10 were quantified with a canine cytokine-specific multiplex bead-based assay (Milliplex MAP, EMD Millipore Corp) as previously described (43). The median fluorescence intensity and cytokine concentration in each sample was measured in duplicate with appropriate controls and associated data analysis software (Milliplex Analyst version 5.1, EMD Millipore Corp). The lower limit of detection for TNF-ɑ, IL-6, and IL-10 was 48.8 pg./mL and IL-8 was 195 pg./mL.
C-reactive protein concentrations
Serum samples were thawed, and CRP was measured with a commercially available canine-specific ELISA (Abcam, Cambridge, United Kingdom) as previously described (44, 45). Samples were measured in duplicate with concurrent standard curves using kit-provided canine standards; the lower limit of detection was 1.1 ng/mL. The optical density of the samples was determined with a Biotek Cytation 3 microplate reader (Biotek, Vermont, United States) set to a wavelength of 450 nm. Background absorbance was measured at 700 nm and subtracted from sample absorbance. Sample CRP concentration was determined by plotting the kit standards using a linear curve.
25(OH)D3 and 24,25(OH)2D3 concentrations
Serum concentrations of 25(OH)D3 and 24,25(OH)2D3 were determined in thawed samples using previously reported HPLC methods (46). The lower limit of quantification of 25(OH)D3 and 24,25(OH)2D3 was 3.0 ng/mL and 9.1 ng/mL, respectively. The vitamin D metabolite ratio (VMR) has been suggested to be a superior surrogate marker for vitamin D status compared to 25(OH)D3 alone because it is not influenced by concentrations and genetic variants of vitamin D binding protein in people (47). The VMR is calculated with the following formula: 24,25(OH)2D3/25(OH)D3.
Phagocytosis of E. coli
Phagocytic capacity of GM was determined with a commercially available assay (PhagoTest, Orpegen Pharma), validated for use in canines. Heparinized blood was incubated with FITC-labelled, opsonized-E. coli strain LE392. The control samples were incubated on ice for 10 min while test samples were incubated in a 37°C water bath for 10 min (43). Phagocytosis was then arrested with samples placed on ice and a quenching solution was added to extinguish surface bound FITC-labelled-E. coli. The cells were then washed, erythrocytes lysed, and cells washed again before DNA staining (R-phycoerythrin) solution was added to facilitate exclusion of aggregated artifacts of bacteria or cells without intact DNA.
Flow cytometry
Flow cytometry was performed at the Midwestern University College of Veterinary Medicine Immunology Laboratory using a flow cytometer (Guava easyCyte HT, Luminex Corporation) and associated data analysis software (GuavaSoft 3.2, Luminex Corporation). A minimum of 20,000 events/sample were recorded. Our gating scheme has previously been reported (43). For assessment of phagocytosis, data was recorded as the percentage of GM cells having internalized FITC-labelled E. coli, as well as their mean fluorescent intensity (MFI), a method of quantifying the phagocytosed bacteria per cell.
Statistical analysis
Statistical analyses were performed using a commercial software (SigmaPlot, Systat Software version 14.5). Categorical data were presented as proportions. Normality was assessed using the Shapiro–Wilk test. Non-normally distributed data were presented as median, interquartile range (IQR), and range when indicated. Normally distributed data were presented as mean and standard deviation (SD). When the measured cytokine, CRP, 25(OH)D3, or 24,25(OH)2D3 concentration fell below the lower limit of detection, data were recorded at the lower limit of detection for statistical purposes. Student’s t-test was used for two group comparisons of normally distributed continuous variables, and Mann–Whitney rank sum test for non-normally distributed continuous variables. A Kruskal-Wallis One-Way Analysis of Variance (ANOVA) test, followed by a Dunn’s test for multiple comparisons was used for multi-group comparisons of non-normally distributed data while a One-Way ANOVA was used for normally distributed data. Spearman’s correlation coefficient was used to assess the strength of relationship between serum 25(OH)D3, 24,25(OH)2D3, and VMR with CRP, IL-8, and phagocytic function. As outlined below, TNF-α, IL-6, and IL-10 were below the lower limit of detection in most samples precluding their inclusion in statistical analyses. The strength level of the relationship between variables was defined with the following Spearman’s rho intervals: weak (0.1–0.39), moderate (0.4–0.69), strong (0.7–0.89), and very strong (0.9–1.0) (48). A P-value of < 0.05 was considered significant.
Results
Animals
Forty-one dogs were eligible for inclusion in this study. One dog was excluded because a matched non-diabetic control dog was not identified, leaving 40 dogs included in the study (NODM, n = 20; controls, n = 20). Demographic information for this cohort is published (42). The median duration of time from diagnosis of NODM to enrollment was 302.5 days (IQR, range; 363.25, 41–1,113 days). Diabetic dogs were treated with either neutral protamine Hagedorn (NPH) (55%, 11/20) or porcine Lente (45%, 9/20) insulin. The median dosage of insulin administered once every 12 h to diabetic dogs was 0.75 units/kg (IQR, range; 0.48, 0.3–1.2 units/kg). Dogs were fed a commercially available pet food (Supplementary material). Three control dogs were suspected to have mild osteoarthritis. These 3 dogs were administered a joint health supplement alone (n = 1) or in conjunction with gabapentin (n = 1) or gabapentin alone (n = 1). The joint health supplement administered to both dogs was Cosequin (Nutramax Laboratories, Veterinary Sciences, Inc). Gabapentin was administered to the two dogs as needed and was not given within 30 days of enrollment. None of the remaining dogs were administered supplements or medications.
Ten dogs each were clinically controlled or uncontrolled. Demographic information is summarized in Table 1. There were no differences in age, weight, BCS, insulin dosage, duration of time with NODM, serum fructosamine or glucose concentrations between diabetic dogs that were clinically controlled and those that were uncontrolled (p > 0.05; Table 1).
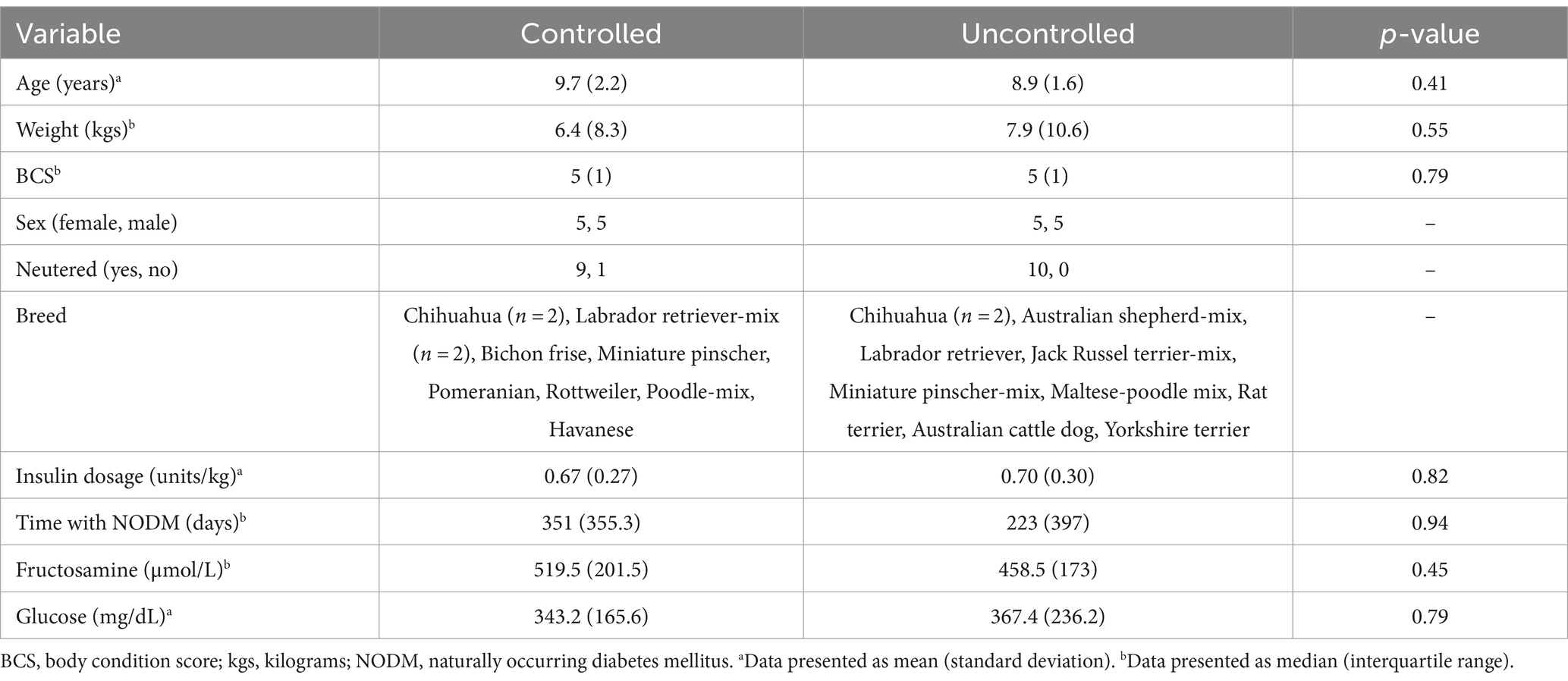
Table 1. Descriptive characteristics in dogs with naturally occurring diabetes mellitus (n = 20) that were clinically controlled (n = 10) or uncontrolled (n = 10).
Basal plasma cytokines
There was no difference in plasma IL-8 concentrations between diabetic dogs and controls (p = 0.10, Figure 1A). However, subgroup analysis revealed a difference between controls, and diabetic dogs that were clinically controlled and uncontrolled (p = 0.02). Diabetic dogs that were clinically uncontrolled had higher plasma IL-8 concentrations than controls (p = 0.02, Figure 1B).
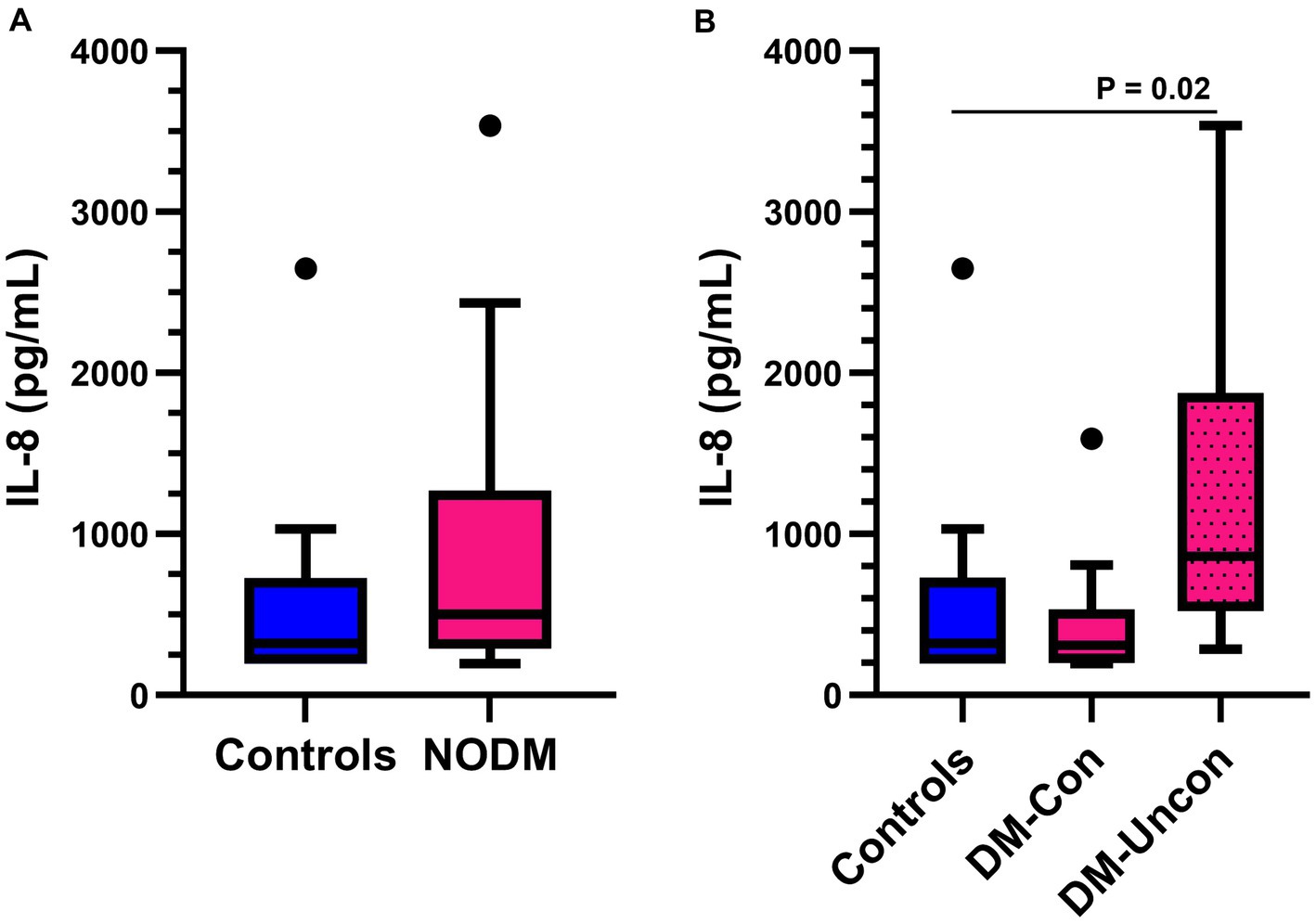
Figure 1. Box and whisker plots comparing basal plasma interleukin (IL)-8 concentrations between (A) dogs with naturally occurring diabetes mellitus (NODM) and controls, and between (B) diabetic dogs that were clinically controlled (DM-Con), uncontrolled (DM-Uncon), and controls. Line at median, bounds of box at the 25th and 75th percentile, whiskers at the upper and lower adjacent values (Tukey method), and dots at outliers beyond the adjacent values. (A) There was no difference in plasma IL-8 concentrations between diabetic dogs (median, IQR; 502.5 pg./mL, 981.4) and controls (323.2 pg./mL, 531.9, p = 0.09). (B) There was a difference between the medians for controls, DM-Con (307.3 pg./mL, 349.5, n = 10), and DM-Uncon (860.1 pg./mL, 1354.7, n = 10, p = 0.02). Plasma IL-8 concentrations were higher in DM-Uncon compared to controls (p = 0.02). No differences were found between DM-Con and controls (p = 1.00) or DM-Con and DM-Uncon (p = 0.06).
Plasma TNF-α, IL-6, and IL-10 concentrations were below the limit of detection in most dogs, regardless of group. Specifically, TNF-α, IL-6, and IL-10 concentrations were below the lower limit of detection in 85% (34/40) (NODM, n = 19; controls, n = 15), 85% (34/40) (NODM, n = 19; controls, n = 15), and 98% (39/40) (NODM, n = 20; controls, n = 19) of dogs, respectively. The same 6 dogs had both quantifiable plasma TNF-α and IL-6 concentrations. The median TNF-α and IL-6 concentrations in these dogs was 131.4 pg./mL (IQR, 222) and 94.6 pg./mL (IQR, 133.2). The one dog with a quantifiable plasma IL-10 concentration (78.6 pg./mL) had TNF-α and IL-6 below the limit of detection. The lack of quantifiable results for these cytokines precluded group or multigroup comparisons.
C-reactive protein
Diabetic dogs had higher serum CRP concentrations than controls (p = 0.02, Figure 2A). However, multigroup comparisons revealed no difference between diabetic dogs that were clinically controlled or uncontrolled, and controls (p = 0.06, Figure 2B).
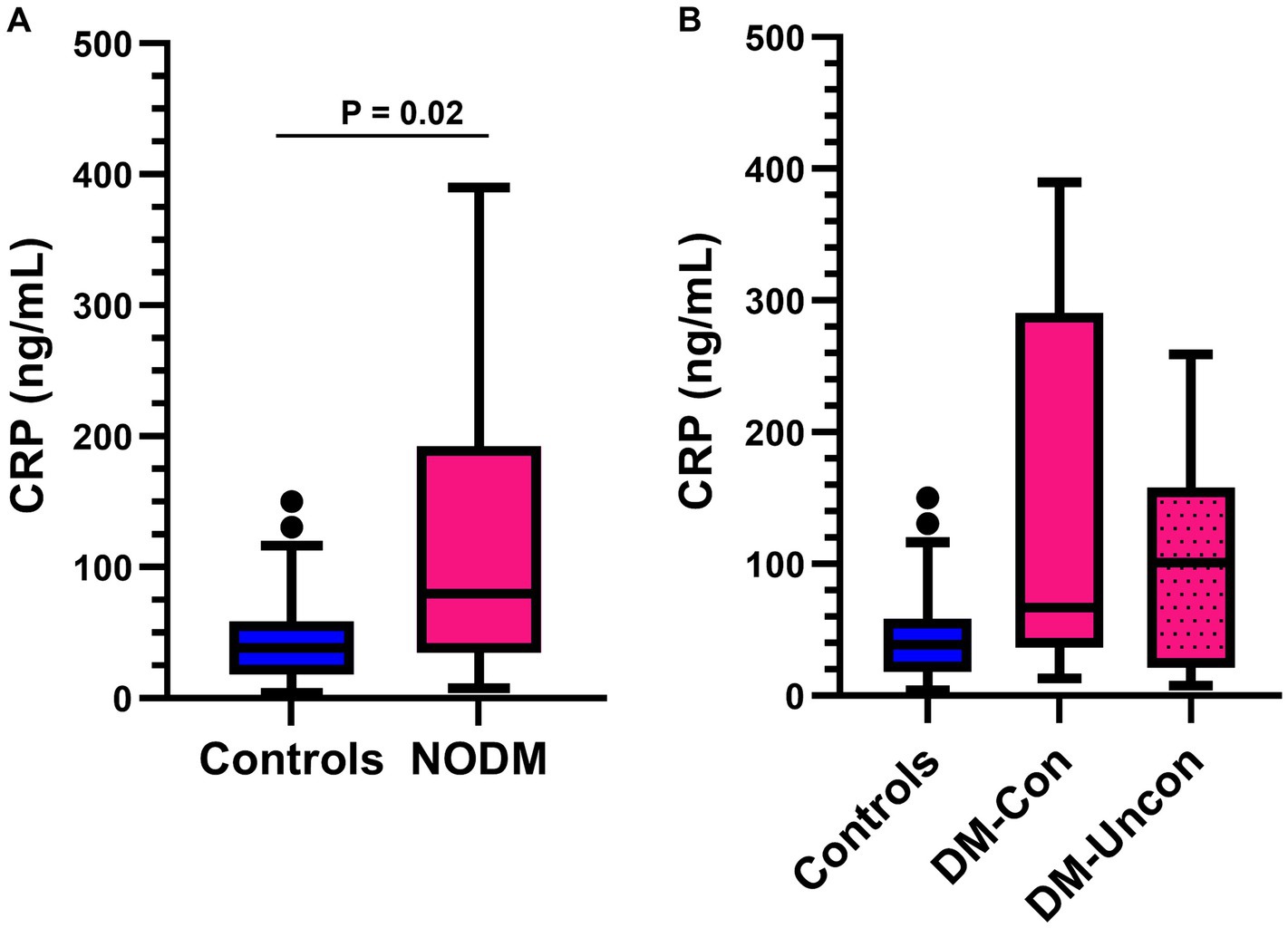
Figure 2. Box and whisker plots comparing serum C-reactive protein (CRP) between (A) dogs with naturally occurring diabetes mellitus (NODM) and controls, and between (B) diabetic dogs that were clinically controlled (DM-Con), uncontrolled (DM-Uncon), and controls. Line at median, bounds of box at the 25th and 75th percentile, whiskers at the upper and lower adjacent values (Tukey method), and dots at outliers beyond the adjacent values. (A) Diabetic dogs had higher serum CRP concentrations (median, IQR; 79.6 ng/mL, 157.4, n = 20) than controls (38.4 ng/mL, 40.4, n = 20, p = 0.02). (B) There was no difference between the medians for controls, DM-Con (66.8 ng/mL, 254.1, n = 10), and DM-Uncon (101.0 ng/mL, 137.1 n = 10, p = 0.06).
Phagocytic function of E. coli
Diabetic dogs had a lower percentage of GM that phagocytized opsonized-E. coli than controls (p = 0.02, Figure 3A) and differences were identified in multigroup comparisons (p = 0.04). Diabetic dogs that were clinically uncontrolled had a lower phagocytic percentage than controls (p = 0.05; Figure 3B).
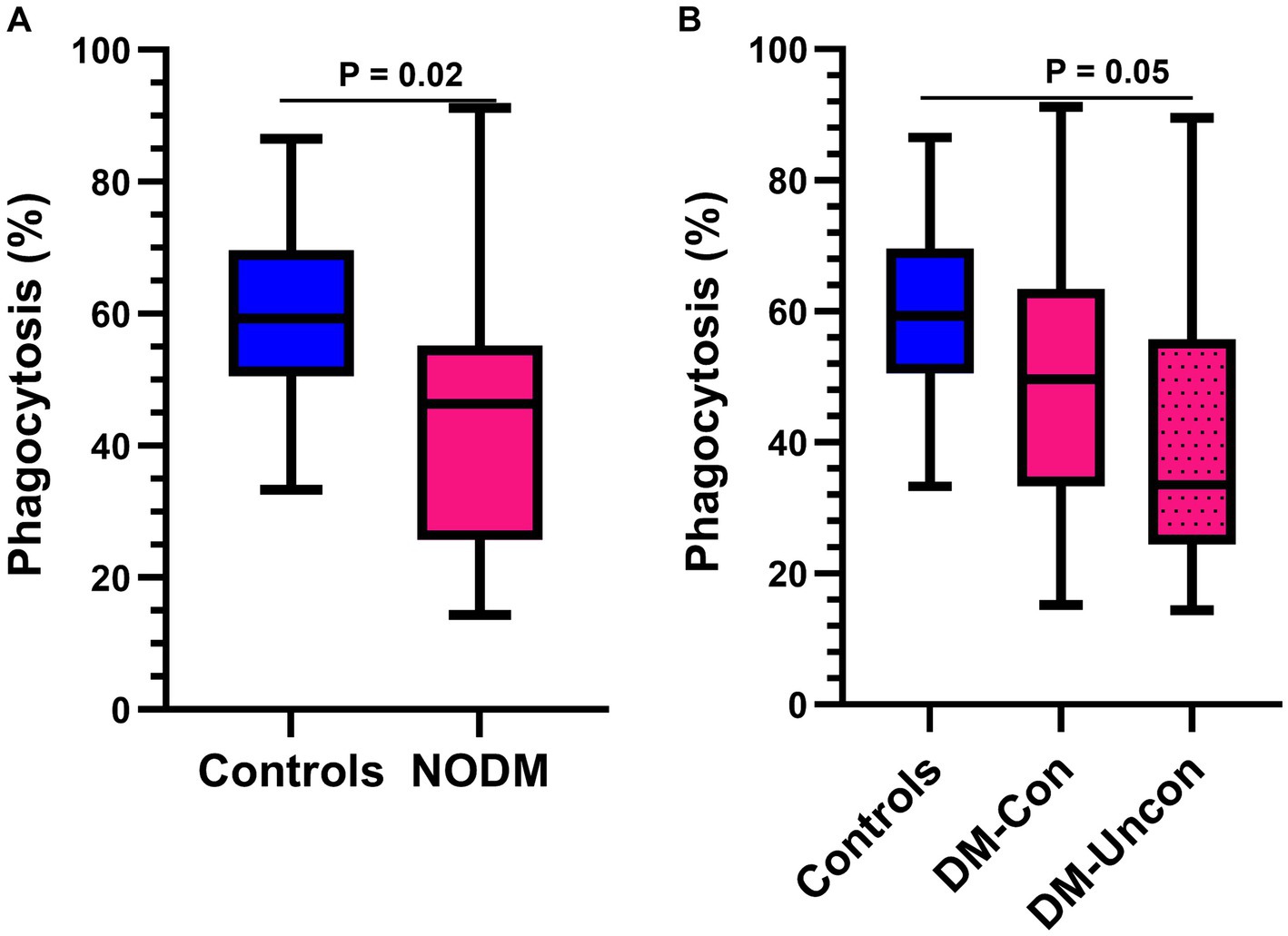
Figure 3. Box and whisker plots comparing percentage of granulocytes and monocytes performing phagocytosis of Escherichia coli (E. coli) between (A) dogs with naturally occurring diabetes mellitus (NODM) and controls, and between (B) diabetic dogs that were clinically controlled (DM-Con), uncontrolled (DM-Uncon), and controls. Line at median, bounds of box at the 25th and 75th percentile, whiskers at the upper and lower adjacent values (Tukey method), and dots at outliers beyond the adjacent values. (A) Diabetic dogs had a lower percentage of cells performing phagocytosis of E. coli (median, IQR; 46.3%, 29.5, n = 20) than controls (59.3%, 19.1, n = 20; p = 0.02). (B) There was a difference between the medians for phagocytic percentage for controls, DM-Con (49.7%, 30.2, n = 10), and DM-Uncon (33.5%, 31.3, n = 10, p = 0.04). Phagocytic percentage was lower in DM-Uncon compared to controls (p = 0.05). No differences were identified between DM-Con and controls (p = 0.39) or between DM-Con and DM-Uncon (p = 1.00).
The number of phagocytized opsonized-E. coli per cell was higher in diabetic dogs compared with controls (p < 0.001, Figure 4A) and differences were also identified in multigroup comparisons (p < 0.001). Diabetic dogs that were clinically controlled (p = 0.02) and those that were uncontrolled (p = 0.002) had a higher number of phagocytized opsonized-E. coli per cell than controls (Figure 4B).
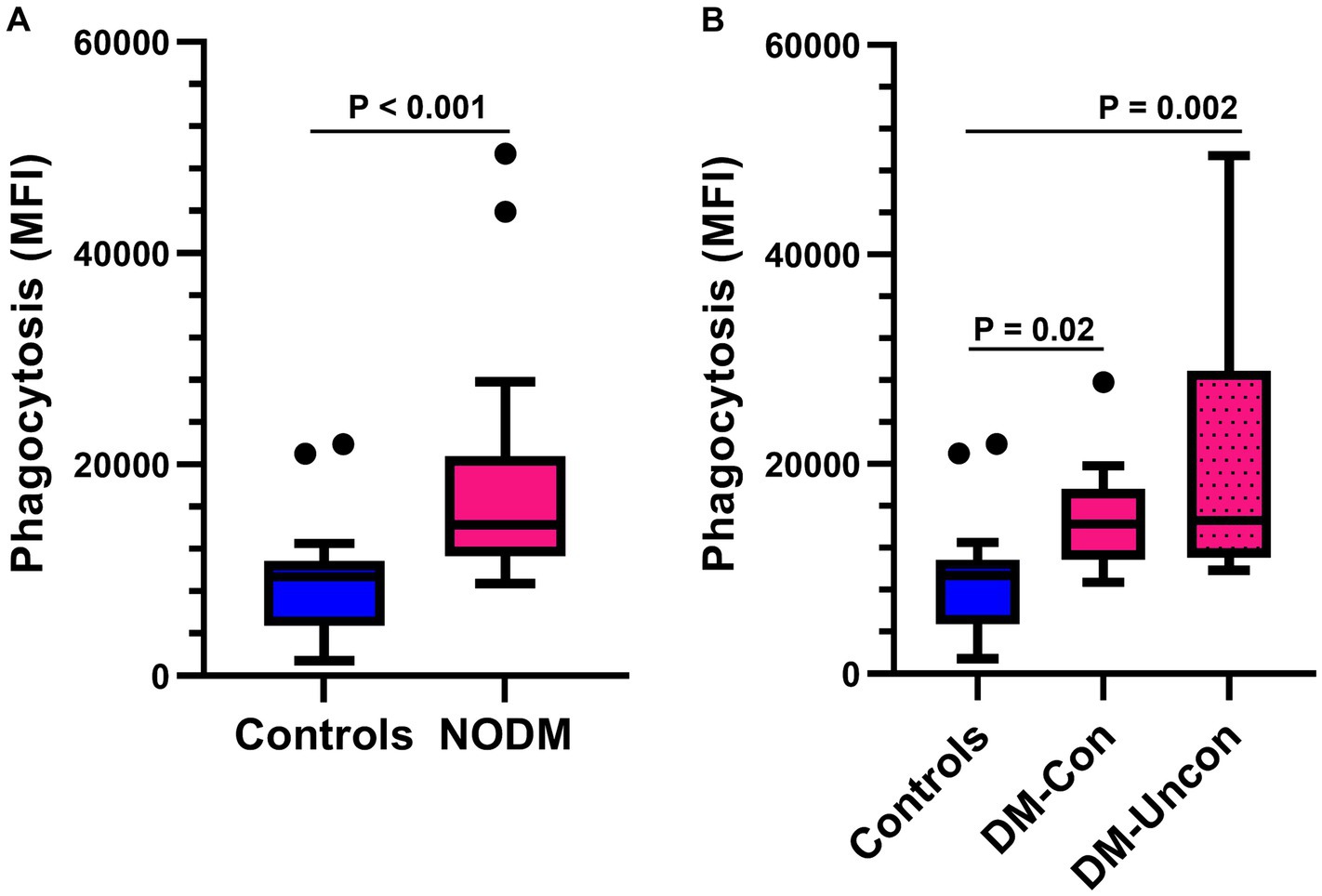
Figure 4. Box and whisker plots comparing the number of Escherichia coli (E. coli) phagocytized per cell between (A) dogs with naturally occurring diabetes mellitus (NODM) and controls, and between (B) diabetic dogs that were clinically controlled (DM-Con), uncontrolled (DM-Uncon), and controls. Line at median, bounds of box at the 25th and 75th percentile, whiskers at the upper and lower adjacent values (Tukey method), and dots at outliers beyond the adjacent values. (A) Diabetic dogs had a higher number of bacteria phagocytized per cell (median, IQR; 14,250 per cell, 9,475, n = 20) than controls (9392.6 per cell, 6129.9, n = 20, p < 0.001). (B) There was a difference between the medians for number of bacteria phagocytized per cell for controls, DM-Con (14,250 per cell, 6725.6, n = 10), and DM-Uncon (14,600 per cell, 17,825, n = 10, p < 0.001). The number of phagocytized bacteria per cell was higher in DM-Con (p = 0.02) and DM-Uncon (p = 0.002) compared to controls. No differences were identified between DM-Con and DM-Uncon (p = 0.50).
Vitamin D metabolites
There were no differences in two group or multigroup comparisons of serum 25(OH)D3, 24,25(OH)2D3, and VMR (p > 0.05; Figures 5, 6). The median serum 25(OH)D3 concentrations for the three control dogs with suspected mild osteoarthritis was 27.6 ng/mL (range, 24.2–38.2 ng/mL).
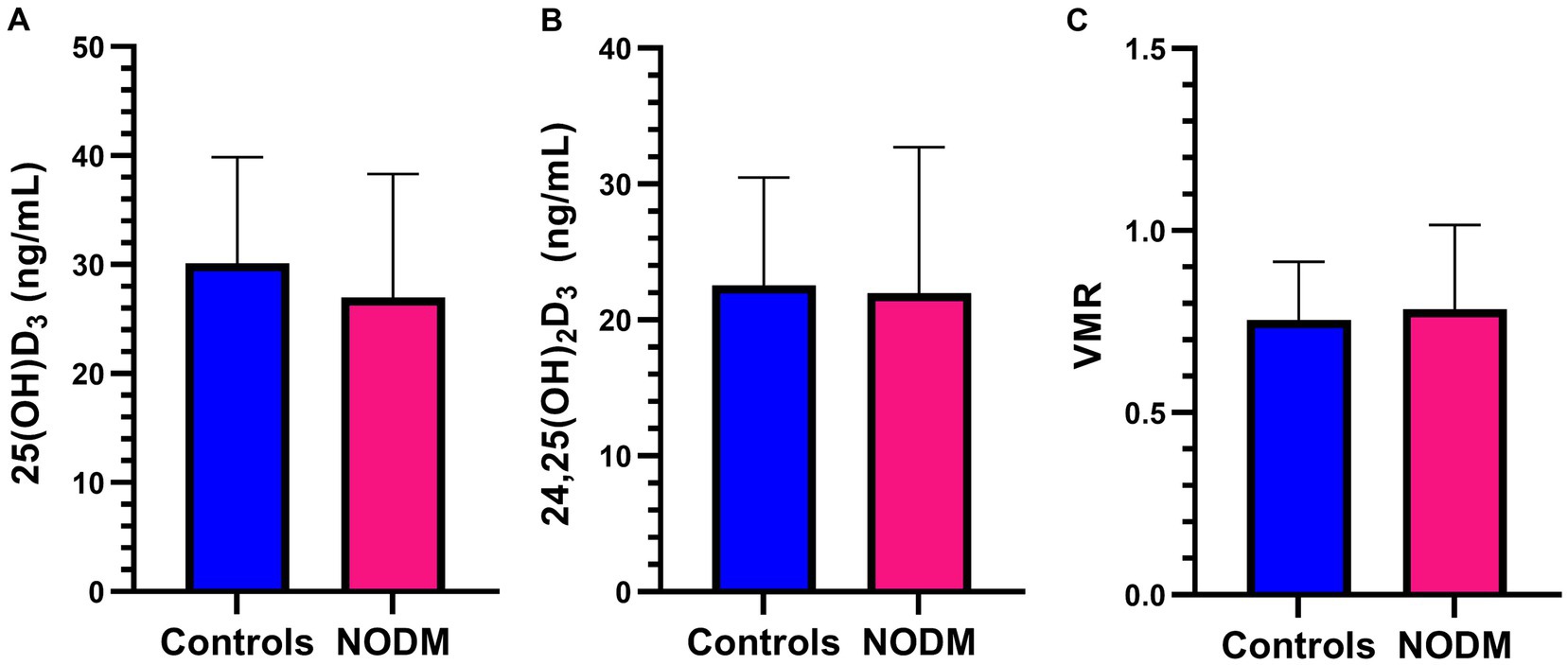
Figure 5. Bar graph comparing means of (A) 25-hydroxyvitamin (OH)D3, (B) 24,25-dihydroxyvitamin (OH)2D3, and (C) Vitamin D metabolite ratio (VMR) between dogs with naturally occurring diabetes mellitus (NODM) and controls. Bar represents standard deviation. There were no differences in the means for 25(OH)D3 (NODM: 27 ng/mL, 11.3, n = 20; controls: 30.1 ng/mL, 9.7, n = 20, p = 0.35), 24,25(OH)2D3 (NODM: 22.4 ng/mL, 10.1, n = 19; controls: 22.6 ng/mL, 7.8, n = 20, p = 0.94), or VMR (NODM: 0.82, 0.20, n = 19; controls: 0.76, 0.16, n = 20, p = 0.31).
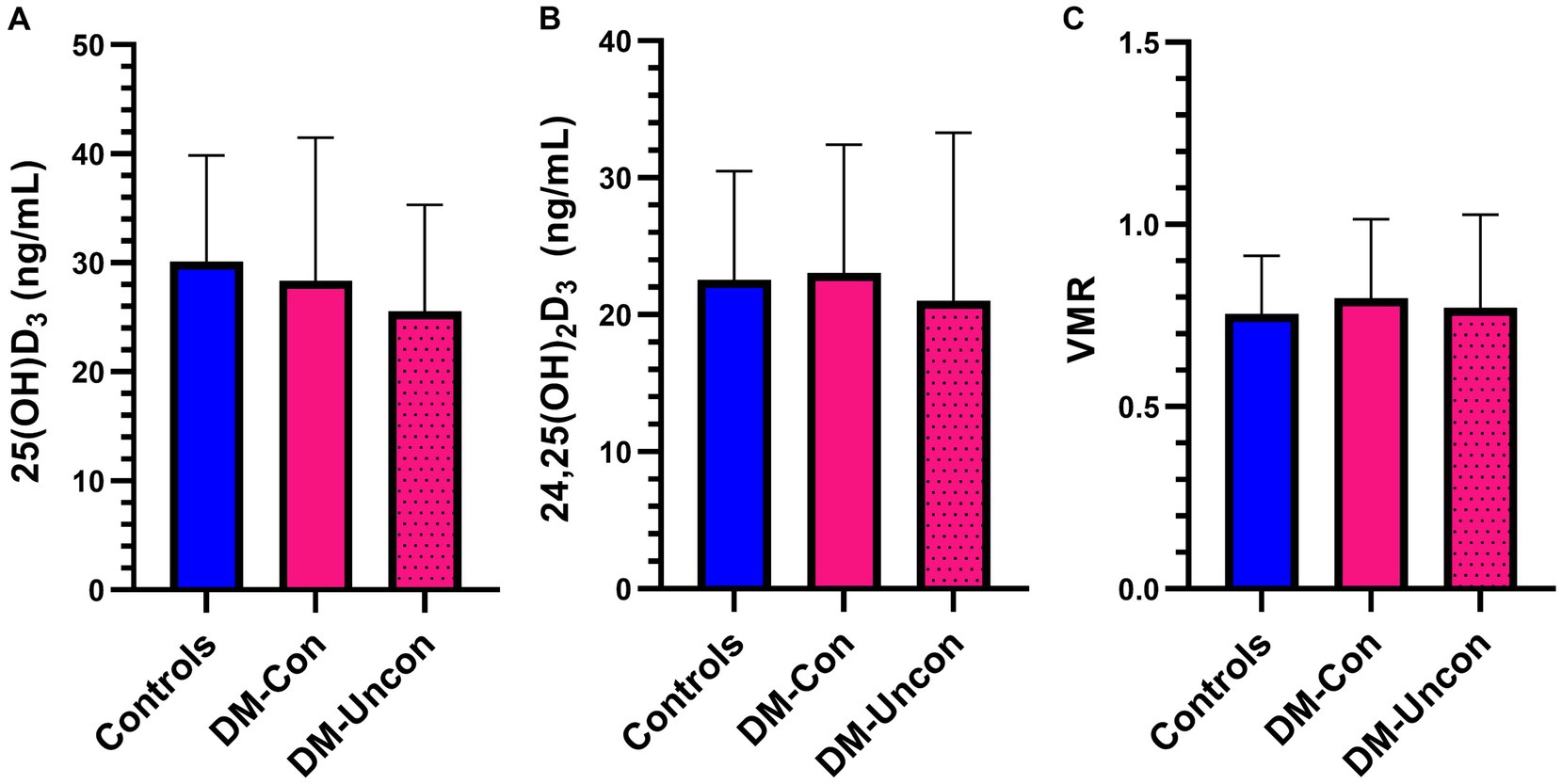
Figure 6. Bar graph comparing means of (A) 25-hydroxyvitamin (OH)D3, (B) 24,25-dihydroxyvitamin (OH)2D3, and (C) Vitamin D metabolite ratio (VMR) between diabetic dogs that were clinically controlled (DM-Con), uncontrolled (DM-Uncon), and controls. Bar represents standard deviation. There was no difference between the means for 25(OH)D3 (DM-Con: 28.4 ng/mL, 13.1, n = 10; DM-Uncon: 25.6 ng/mL, 9.7, n = 10; controls: 30.1 ng/mL, 9.7, n = 20, p = 0.55), 24,25(OH)2D3 (DM-Con: 23.1 ng/mL, 9.4, n = 9; DM-Uncon: 21.8 ng/mL, 11.3, n = 10; controls: 22.6 ng/mL, 7.9, n = 20, p = 0.95) or VMR (DM-Con: 0.80, 0.22, n = 9; DM-Uncon: 0.83, 0.20, n = 10; controls: 0.76, 0.16, n = 20, p = 0.55).
Correlation analyses
First, we were interested in evaluating the relationships between vitamin D metabolites and various immunologic variables and fructosamine. No associations were identified between serum 25(OH)D3, 24,25(OH)2D3, or VMR with fructosamine, CRP, IL-8, or phagocytosis of E. coli (Table 2). There was a strong positive association between 25(OH)D3 and 24,25(OH)2D3 (rho = 0.78; n = 38, p < 0.001; Supplementary Figure S1).
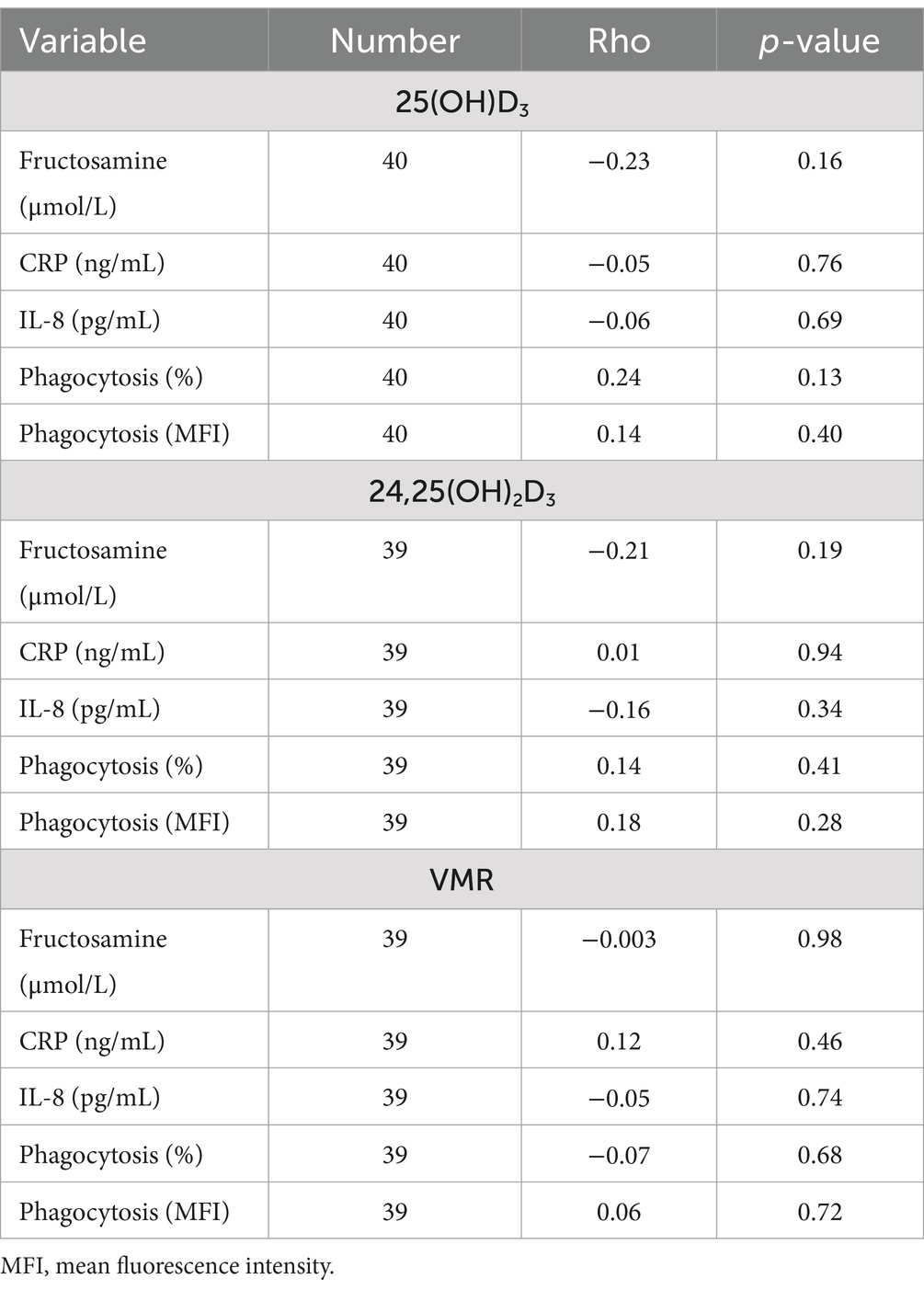
Table 2. Association between 25-hydroxyvitamin (OH)D3, 24,25-dihydroxyvitamin (OH)2D3, and vitamin D metabolite ratio (VMR) with C-reactive protein (CRP), interleukin (IL)-8, fructosamine, and phagocytic function of Escherichia coli in 40 dogs that were either non-diabetic healthy controls (n = 20) or dogs with naturally occurring diabetes mellitus (n = 20).
Next, we aimed to determine whether there were any associations between serum fructosamine and various immunologic variables. There was a weak positive association between serum fructosamine and CRP concentrations (rho = 0.35, p = 0.03, n = 40; Table 3). A moderate positive association was identified between serum fructosamine and the number of phagocytized opsonized-E. coli per cell (rho = 0.45, p = 0.004, n = 40; Table 3). No associations were identified when just the diabetic dogs were included in these correlation tests (p > 0.05, n = 20; Supplementary Table S1).
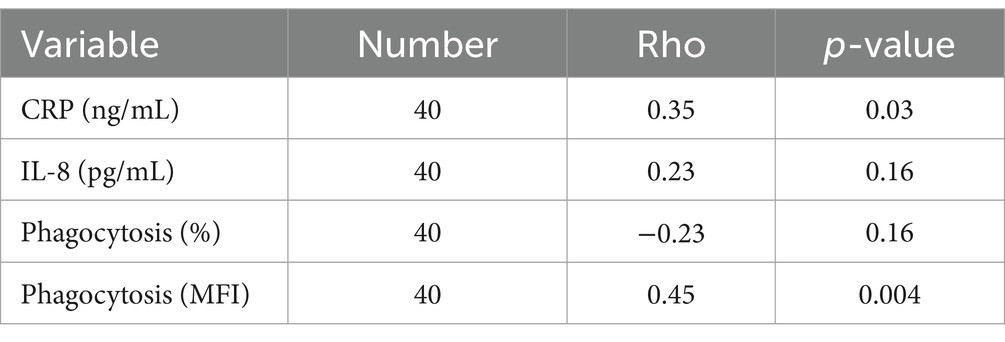
Table 3. Association between serum fructosamine and C-reactive protein (CRP), interleukin (IL)-8, and phagocytic function of Escherichia coli in 40 dogs that were either non-diabetic healthy controls (n = 20) or dogs with naturally occurring diabetes mellitus (n = 20).
Discussion
The inflammatory milieu, immunologic response, and role of vitamin D in people with T1DM has been well characterized but a relative void exists in dogs with NODM. Therefore, the goal of this prospective case–control study was to gather information to better understand cytokine homeostasis, phagocytic capacity of E. coli, vitamin D metabolites and the effect that clinical control has on these variables. We found that diabetic dogs had a higher proclivity for an inflammatory phenotype as seen with higher serum CRP concentrations compared to controls. Plasma cytokine concentrations were below the limit of detection in most dogs, regardless of group, except for IL-8. There was no difference in plasma IL-8 concentrations between all diabetic dogs compared to controls; however, diabetic dogs with clinically uncontrolled disease had higher levels compared with controls. Diabetic dogs exhibited abnormal phagocytosis and clinical status influenced these derangements. There were no two-group or multigroup differences in vitamin D metabolites nor were any associations with fructosamine, CRP, IL-8, or phagocytosis of E. coli identified. Lastly, positive associations were identified between fructosamine and CRP and the number of phagocytized bacteria per cell.
Serum CRP concentrations were higher in diabetic dogs compared to controls. The multigroup comparison revealed no differences in CRP concentrations with a p-value just outside of statistical significance (p = 0.06). Observationally, the medians for diabetic dogs that were uncontrolled (101 mg/L) and controlled (66.8 mg/L) were higher than non-diabetic controls (38.4 mg/L). Moreover, a mild positive significant association was detected between serum CRP and fructosamine concentrations. Collectively, these results suggest that otherwise healthy diabetic dogs have a pro-inflammatory phenotype with changes in severity occurring in tandem with fructosamine, a surrogate marker for glycemic control in dogs (49). Ours is the first study to evaluate the role of CRP in diabetic dogs and its association with diabetic management; however, this positive acute phase protein has been extensively studied in people with T1DM because of its association with glycemic control and diabetic complications (12, 16, 23, 24, 50–52). Importantly, the direction of relationship between serum CRP and fructosamine could not be ascertained in our study because of the observational design. It is equally plausible that hyperglycemia induced CRP secretion contributing to inflammation as it is that increased CRP secondary to an unrelated source of inflammation-induced insulin resistance that affected glucose metabolism (53–56). Prospective studies with longitudinal assessment of serum CRP and glycemic control are needed to better define causality in this association.
Plasma IL-8 concentrations were higher in all diabetic dogs compared to controls, but this difference did not reach statistical significance (p = 0.09). These results contrast with findings from the only other study to have investigated blood IL-8 concentrations in diabetic dogs (27). The aforementioned study using the same canine specific multiplex bead-based assay to quantify cytokine concentrations found that diabetic dogs had higher basal serum IL-8 concentrations (median, range; 2,590 pg./mL, 1,410–3,580 pg./mL, n = 9) compared with healthy controls (1,070 pg./mL, 828–1910 pg./mL, n = 9) (27). One possible explanation for the conflicting results is that the reported IL-8 concentrations for diabetic dogs were 5 times higher in the O’Neill et al. study compared to our diabetic cohort. The included diabetic dogs in that study were enrolled at a routine follow-up visit and the presence of concurrent disease was not exclusionary. Therefore, it is reasonable to suspect that some of the dogs had comorbid disorders that influenced serum IL-8 concentrations. We only included otherwise healthy diabetic dogs in our study to avoid confounding effects of other disorders on immunologic findings. Alternatively, or in addition, our study may have been underpowered to detect a difference if one existed (i.e., Type 2 error). The latter theory seems reasonable as elevated basal blood IL-8 concentrations in people with T1DM is one of the most consistently reported cytokine derangements (57–61).
Diabetic dogs with uncontrolled clinical disease had higher basal plasma IL-8 concentrations than non-diabetic controls. However, there were no differences identified between diabetic dogs with controlled clinical signs and either uncontrolled diabetics or non-diabetic controls. Collectively, these results suggests that dogs with poorly regulated diabetes are more likely to have higher basal circulating levels of IL-8. This theory is supported by results from one study that showed basal serum IL-8 concentrations were higher in children with T1DM that had poor glycemic control compared to those with favorable control and there was a positive correlation between IL-8 concentrations and HbA1c (60). We cannot determine based on the design of our study whether elevated basal plasma IL-8 concentrations were a cause, consequence, or a combination thereof, for poorly regulated diabetic clinical control. Persistent uncontrolled hyperglycemia can directly increase production of IL-8. This has been highlighted in previous work in which hyperglycemia induced transcription of the gene for IL-8 in endothelial cells and monocytes (62, 63). However, increases in IL-8 can cause insulin resistance and thus worsen hyperglycemia via inhibition of insulin-induced Akt phosphorylation (64). Overall, IL-8 is one of the most important molecules involved with initiation and amplification of the acute inflammatory response and has been linked with development of diabetes related complications in people (65, 66). The combination of our findings in dogs and increased risk for diabetes-related complications in people with elevated IL-8 support the need for further investigation into the role of this chemokine in diabetic dogs.
Basal plasma concentrations of TNF-α, IL-6, and IL-10 were below the limits of detection in most dogs, regardless of group. These results are in contrast with common findings in diabetic people but are similar to results found in the diabetic dog study by O’Neill et al. (15, 16, 27, 58). One potential explanation for the lack of quantifiable cytokine detection is that diabetes mellitus is a dynamic disorder with expected changes in the inflammatory milieu from the time of diagnosis to various time-points after institution of insulin therapy. One study found that only children with newly diagnosed (i.e., < 1 year) T1DM had increased basal serum TNF-α and IL-6 concentrations (58). Another study found that mitogen-stimulated peripheral blood mononuclear cell production of TNF-α in supernatant decreased over time in patients as glycemic control improved (19). We evaluated plasma cytokine concentrations on a single occasion a median of 302 days after dogs were diagnosed with NODM. Therefore, it is possible we missed elevations in these cytokines because of when samples were procured. Another point to consider is that the multiplex assay used in this study may have been relatively insensitive to identify small changes in cytokine concentrations in dogs. While this multiplex assay is widely used in dogs, some cytokine concentrations are commonly below the limits of detection independent of health status (27, 67–71).
Leukocytes from diabetic dogs exhibited abnormal phagocytic activity of E. coli characterized by a decreased percentage of cells performing phagocytosis with a concomitant increase in number of bacteria phagocytized per cell compared to non-diabetic controls. The cumulative effect of these findings on the overall phagocytic capacity of E. coli in diabetic dogs is unknown. One possible explanation for these results is that there is a global reduction in leukocytes performing phagocytosis and the remaining available cells must increase activity to compensate. There have been several studies over the last 40 years that have highlighted impaired leukocyte phagocytic activity of various substrates in people with T1DM and animal models (20, 72–78). Studies in rabbits and mice demonstrate a clear causal role for hyperglycemia decreasing leukocyte phagocytosis that is restored with insulin (79–81). Diabetes-associated impaired phagocytosis is multifactorial with several purported possible mechanisms that include intracellular accumulation of sorbitol, decreased phosphofructokinase activity, and dysregulated metabolism of arachidonic acid (20). The positive association between fructosamine and the number of bacteria phagocytized per cell was an unexpected finding in our study. Explanations for this finding are beyond the scope of this study but warrant further investigation.
There were no differences in vitamin D metabolites between diabetic dogs and controls, nor were differences identified based on the level of diabetic clinical control. These findings are in contrast with our hypothesis and commonly reported results from people with T1DM (29–31). The modest size of the sample population in our study may have contributed to type 2 error as well as limited genetic variability. Studies in human patients with T1DM have identified genetic polymorphisms in vitamin D handling proteins that can affect vitamin D blood concentrations, cellular availability, and metabolism (82–84). Therefore, it is possible a much larger sample population would have accounted for this potential genetic variability. It must also be considered that the lack of differences in vitamin D metabolites in our study is real and may represent a species difference. We did not find associations between vitamin D metabolites and any immune function variables in our study. A potential explanation for this is the low proportion of dogs with severely low serum 25(OH)D concentrations (< 25–30 ng/mL) (85). The risk for various disorders and associative immune dysregulation is higher in people with vitamin D deficiency (i.e., serum/plasma 25(OH)D of <25–30 ng/mL) (86, 87).
Our study had several limitations that must be considered. The diabetic dogs in this study were determined to be otherwise healthy based on a collective interpretation of history, physical examination, complete blood count, biochemical panel, and urinalysis by a board-certified small animal internist. It is possible some diabetic dogs included in this study had an occult disorder that affected assessment of inflammatory biomarkers and immune function testing. Commercially available dog foods have varying vitamin D content that may influence serum 25(OH)D concentrations (85). However, there is a curvilinear phenomenon in the association between vitamin D intake and blood 25(OH)D concentrations in dogs. As vitamin D intake increases, the response in rising 25(OH)D3 diminishes. At the low end of vitamin D intake, circulating 25(OH)D3 rises sharply with minor increases in vitamin D intake (88). Commercial dog food is typically adequately supplied with vitamin D. Therefore, otherwise healthy dogs offered these dog foods are unlikely to be vitamin D deficient. We chose to allow dogs to continue their typical maintenance diets rather than switch the entire cohort to a single diet for two primary reasons. First, changing the offered diet would have likely affected diabetic regulation in some dogs and possibly caused adverse food reactions in others. Secondly, we wanted our cohort to be representative of a typical population of diabetic and control dogs. Regardless, the lack of difference in vitamin D metabolites indicates that diet had a minimal influence in our study. Diabetic dogs were grouped based on the level of clinical control, which does not always parallel glycemic control. Several recent studies have highlighted limitations of relying on biomarkers such as fructosamine to determine glycemic control in dogs (89, 90). Studies that investigate the role of glycemic control on inflammatory biomarkers and immune function may be better served grouping dogs based on a combination of clinical status and data from continuous glucose monitors. Assessment of phagocytosis was limited to opsonized-E. coli only and thus our results cannot be extrapolated as an overall indictment of phagocytic capacity in diabetic dogs. Future studies including various substrates to assess phagocytosis would be useful to attain a more comprehensive assessment. We were intentional in our investigation of CRP, TNF-α, IL-6, IL-8, and IL-10 because these biomarkers are most commonly implicated in diabetic people (12, 15–17, 19, 24, 36, 91, 92). However, a more comprehensive profile including more cytokines and acute phase proteins may provide a more global picture of the inflammatory microenvironment in diabetic dogs. Three control dogs were suspected to have mild osteoarthritis. Hypovitaminosis D is a relatively common finding in people with osteoarthritis but has not been thoroughly investigated in dogs (93). Therefore, it is possible that osteoarthritis in these dogs may have had a mild effect on serum 25(OH)D3 concentrations but was not believed to have substantially impacted statistical analyses because the median serum 25(OH)D3 concentration in these three control dogs were comparable to the mean results for both controls and diabetics. Lastly, we collected samples for our experiments at a single time-point after initiation of insulin therapy. A longitudinal study design starting at the time of diagnosis (before insulin therapy) with serial sampling through the time that adequate glycemic control is achieved would provide integral information regarding immunologic changes over time while also more confidently concluding causality.
Conclusion
In conclusion, our study demonstrates that diabetic dogs have an inflammatory phenotype and phagocytic dysregulation of opsonized-E. coli. Uncontrolled clinical disease in diabetic dogs may worsen the magnitude of inflammation and phagocytic derangements. Lastly, higher serum fructosamine concentrations were correlated with increasing CRP concentrations and number of bacteria phagocytized per cell but causality was not determined.
Data availability statement
The raw data supporting the conclusions of this article will be made available by the authors, without undue reservation.
Ethics statement
This study was conducted in accordance with guidelines for clinical studies and approved by the Midwestern University Animal Care and Use Committee (protocol # 2944) with written owner consent. The studies were conducted in accordance with the local legislation and institutional requirements. Written informed consent was obtained from the owners for the participation of their animals in this study.
Author contributions
JJ: Conceptualization, Data curation, Formal analysis, Funding acquisition, Investigation, Methodology, Resources, Writing – original draft, Writing – review & editing. RB: Conceptualization, Data curation, Formal analysis, Investigation, Resources, Writing – original draft, Writing – review & editing. RK: Formal analysis, Investigation, Writing – original draft, Writing – review & editing. TG: Conceptualization, Formal analysis, Funding acquisition, Investigation, Writing – original draft, Writing – review & editing, Project administration. LA-N: Conceptualization, Formal analysis, Funding acquisition, Investigation, Writing – original draft, Writing – review & editing. LA: Data curation, Formal analysis, Funding acquisition, Investigation, Writing – original draft, Writing – review & editing.
Funding
The author(s) declare that financial support was received for the research, authorship, and/or publication of this article. This study was funded by the Midwestern University One Health Faculty Stimulus grant.
Acknowledgments
The authors would like to thank Sasha Willis, Paige Hunsinger, Ross Monasky, and Zenan Tao, for their technical assistance.
Conflict of interest
The authors declare that the research was conducted in the absence of any commercial or financial relationships that could be construed as a potential conflict of interest.
Publisher’s note
All claims expressed in this article are solely those of the authors and do not necessarily represent those of their affiliated organizations, or those of the publisher, the editors and the reviewers. Any product that may be evaluated in this article, or claim that may be made by its manufacturer, is not guaranteed or endorsed by the publisher.
Supplementary material
The Supplementary material for this article can be found online at: https://www.frontiersin.org/articles/10.3389/fvets.2024.1441993/full#supplementary-material
References
1. Brito-Casillas, Y, Melian, C, Holder, A, Wiebe, JC, Navarro, A, Quesada-Canales, O, et al. Studying the heterogeneous pathogenesis of canine diabetes: observational characterization of an island population. Vet Med Sci. (2021) 7:1071–81. doi: 10.1002/vms3.452
2. Catchpole, B, Adams, JP, Holder, AL, Short, AD, Ollier, WE, and Kennedy, LJ. Genetics of canine diabetes mellitus: are the diabetes susceptibility genes identified in humans involved in breed susceptibility to diabetes mellitus in dogs? Vet J. (2013) 195:139–47. doi: 10.1016/j.tvjl.2012.11.013
3. Davison, LJ, Herrtage, ME, and Catchpole, B. Study of 253 dogs in the United Kingdom with diabetes mellitus. Vet Rec. (2005) 156:467–71. doi: 10.1136/vr.156.15.467
4. Fall, T, Hamlin, HH, Hedhammar, A, Kampe, O, and Egenvall, A. Diabetes mellitus in a population of 180,000 insured dogs: incidence, survival, and breed distribution. J Vet Intern Med. (2007) 21:1209–16. doi: 10.1111/j.1939-1676.2007.tb01940.x
5. Fracassi, F, Pietra, M, Boari, A, Aste, G, Giunti, M, and Famigli-Bergamini, P. Breed distribution of canine diabetes mellitus in Italy. Vet Res Commun. (2004) 28:339–42. doi: 10.1023/b:verc.0000045441.77213.3b
6. Guptill, L, Glickman, L, and Glickman, N. Time trends and risk factors for diabetes mellitus in dogs: analysis of veterinary medical data base records (1970-1999). Vet J. (2003) 165:240–7. doi: 10.1016/s1090-0233(02)00242-3
7. Hess, RS, Kass, PH, and Ward, CR. Breed distribution of dogs with diabetes mellitus admitted to a tertiary care facility. J Am Vet Med Assoc. (2000) 216:1414–7. doi: 10.2460/javma.2000.216.1414
8. Mattin, M, O'Neill, D, Church, D, McGreevy, PD, Thomson, PC, and Brodbelt, D. An epidemiological study of diabetes mellitus in dogs attending first opinion practice in the UK. Vet Rec. (2014) 174:349–9. doi: 10.1136/vr.101950
9. Yoon, S, Fleeman, LM, Wilson, BJ, Mansfield, CS, and McGreevy, P. Epidemiological study of dogs with diabetes mellitus attending primary care veterinary clinics in Australia. Vet Rec. (2020) 187:e22. doi: 10.1136/vr.105467
10. Gilor, C, Niessen, SJ, Furrow, E, and DiBartola, SP. What's in a name? Classification of diabetes mellitus in veterinary medicine and why it matters. J Vet Intern Med. (2016) 30:927–40. doi: 10.1111/jvim.14357
11. Behrend, E, Holford, A, Lathan, P, Rucinsky, R, and Schulman, R. 2018 AAHA diabetes management guidelines for dogs and cats. J Am Anim Hosp Assoc. (2018) 54:1–21. doi: 10.5326/JAAHA-MS-6822
12. Basu, S, Larsson, A, Vessby, J, Vessby, B, and Berne, C. Type 1 diabetes is associated with increased cyclooxygenase-and cytokine-mediated inflammation. Diabetes Care. (2005) 28:1371–5. doi: 10.2337/diacare.28.6.1371
13. Chanchamroen, S, Kewcharoenwong, C, Susaengrat, W, Ato, M, and Lertmemongkolchai, G. Human polymorphonuclear neutrophil responses to Burkholderia pseudomallei in healthy and diabetic subjects. Infect Immun. (2009) 77:456–63. doi: 10.1128/IAI.00503-08
14. Delamaire, M, Maugendre, D, Moreno, M, Le Goff, MC, Allannic, H, and Genetet, B. Impaired leucocyte functions in diabetic patients. Diabet Med. (1997) 14:29–34. doi: 10.1002/(SICI)1096-9136(199701)14:1<29::AID-DIA300>3.0.CO;2-V
15. Devaraj, S, Dasu, MR, Rockwood, J, Winter, W, Griffen, SC, and Jialal, I. Increased toll-like receptor (TLR) 2 and TLR4 expression in monocytes from patients with type 1 diabetes: further evidence of a proinflammatory state. J Clin Endocrinol Metabol. (2008) 93:578–83. doi: 10.1210/jc.2007-2185
16. Fawaz, L, Elwan, AE, Kamel, YH, Farid, TM, Kamel, A, and Mohamed, WA. Value of C-reactive protein and IL-6 measurements in type 1 diabetes mellitus. Arch Med Sci. (2009) 5:383–90.
17. Hatanaka, E, Monteagudo, PT, Marrocos, MS, and Campa, A. Neutrophils and monocytes as potentially important sources of proinflammatory cytokines in diabetes. Clin Exp Immunol. (2006) 146:443–7. doi: 10.1111/j.1365-2249.2006.03229.x
18. Katz, S, Klein, B, Elian, I, Fishman, P, and Djaldetti, M. Phagocytotic activity of monocytes from diabetic patients. Diabetes Care. (1983) 6:479–82. doi: 10.2337/diacare.6.5.479
19. Kulseng, B, Skjak-Braek, G, Folling, I, and Espevik, T. TNF production from peripheral blood mononuclear cells in diabetic patients after stimulation with alginate and lipopolysaccharide. Scand J Immunol. (1996) 43:335–40. doi: 10.1046/j.1365-3083.1996.d01-43.x
20. Marhoffer, W, Stein, M, Schleinkofer, L, and Federlin, K. Evidence of ex vivo and in vitro impaired neutrophil oxidative burst and phagocytic capacity in type 1 diabetes mellitus. Diabetes Res Clin Pract. (1993) 19:183–8. doi: 10.1016/0168-8227(93)90112-I
21. Hotamisligil, GS, and Spiegelman, BM. Tumor necrosis factor alpha: a key component of the obesity-diabetes link. Diabetes. (1994) 43:1271–8. doi: 10.2337/diab.43.11.1271
22. Wieser, V, Moschen, AR, and Tilg, H. Inflammation, cytokines and insulin resistance: a clinical perspective. Arch Immunol Ther Exp. (2013) 61:119–25. doi: 10.1007/s00005-012-0210-1
23. Cutaș, A, Drugan, C, Roman, G, Rusu, A, Istrate, D, Achimaș-Cadariu, A, et al. Inflammatory response and timeline of chronic complications in patients with type 1 and 2 diabetes mellitus. Int J Diabetes Dev Ctries. (2020) 40:561–9. doi: 10.1007/s13410-020-00824-5
24. King, DE, Mainous, AG III, Buchanan, TA, and Pearson, WS. C-reactive protein and glycemic control in adults with diabetes. Diabetes Care. (2003) 26:1535–9. doi: 10.2337/diacare.26.5.1535
25. DeClue, AE, Nickell, J, Chang, CH, and Honaker, A. Upregulation of proinflammatory cytokine production in response to bacterial pathogen-associated molecular patterns in dogs with diabetes mellitus undergoing insulin therapy. J Diabetes Sci Technol. (2012) 6:496–502. doi: 10.1177/193229681200600303
26. Latimer, KS, and Mahaffey, EA. Neutrophil adherence and movement in poorly and well-controlled diabetic dogs. Am J Vet Res. (1984) 45:1498–500.
27. O'Neill, S, Drobatz, K, Satyaraj, E, and Hess, R. Evaluation of cytokines and hormones in dogs before and after treatment of diabetic ketoacidosis and in uncomplicated diabetes mellitus. Vet Immunol Immunopathol. (2012) 148:276–83. doi: 10.1016/j.vetimm.2012.06.027
28. Vaitaitis, G, Webb, T, Webb, C, and Wagner, DH. Canine diabetes mellitus demonstrates multiple markers of chronic inflammation including Th40 cell increases and elevated systemic-immune inflammation index, consistent with autoimmune dysregulation. Front Immunol. (2024) 14:1319947. doi: 10.3389/fimmu.2023.1319947
29. Daga, RA, Laway, BA, Shah, ZA, Mir, SA, Kotwal, SK, and Zargar, AH. High prevalence of vitamin D deficiency among newly diagnosed youth-onset diabetes mellitus in North India. Arq Bras Endocrinol Metabol. (2012) 56:423–8. doi: 10.1590/s0004-27302012000700003
30. Greer, RM, Portelli, SL, Hung, BS, Cleghorn, GJ, McMahon, SK, Batch, JA, et al. Serum vitamin D levels are lower in Australian children and adolescents with type 1 diabetes than in children without diabetes. Pediatr Diabetes. (2013) 14:31–41. doi: 10.1111/j.1399-5448.2012.00890.x
31. Littorin, B, Blom, P, Scholin, A, Arnqvist, HJ, Blohme, G, Bolinder, J, et al. Lower levels of plasma 25-hydroxyvitamin D among young adults at diagnosis of autoimmune type 1 diabetes compared with control subjects: results from the nationwide diabetes incidence study in Sweden (DISS). Diabetologia. (2006) 49:2847–52. doi: 10.1007/s00125-006-0426-x
32. Gorham, ED, Garland, CF, Burgi, AA, Mohr, SB, Zeng, K, Hofflich, H, et al. Lower prediagnostic serum 25-hydroxyvitamin D concentration is associated with higher risk of insulin-requiring diabetes: a nested case-control study. Diabetologia. (2012) 55:3224–7. doi: 10.1007/s00125-012-2709-8
33. Hou, Y, Song, A, Jin, Y, Xia, Q, Song, G, and Xing, X. A dose-response meta-analysis between serum concentration of 25-hydroxy vitamin D and risk of type 1 diabetes mellitus. Eur J Clin Nutr. (2021) 75:1010–23. doi: 10.1038/s41430-020-00813-1
34. Hypponen, E, Laara, E, Reunanen, A, Jarvelin, MR, and Virtanen, SM. Intake of vitamin D and risk of type 1 diabetes: a birth-cohort study. Lancet. (2001) 358:1500–3. doi: 10.1016/S0140-6736(01)06580-1
35. Sorensen, IM, Joner, G, Jenum, PA, Eskild, A, Brunborg, C, Torjesen, PA, et al. Vitamin D-binding protein and 25-hydroxyvitamin D during pregnancy in mothers whose children later developed type 1 diabetes. Diabetes Metab Res Rev. (2016) 32:883–90. doi: 10.1002/dmrr.2812
36. Devaraj, S, Yun, JM, Duncan-Staley, CR, and Jialal, I. Low vitamin D levels correlate with the proinflammatory state in type 1 diabetic subjects with and without microvascular complications. Am J Clin Pathol. (2011) 135:429–33. doi: 10.1309/AJCPJGZQX42BIAXL
37. Greenhagen, RM, Frykberg, RG, and Wukich, DK. Serum vitamin D and diabetic foot complications. Diabet Foot Ankle. (2019) 10:1579631. doi: 10.1080/2000625X.2019.1579631
38. Savastio, S, Cadario, F, Genoni, G, Bellomo, G, Bagnati, M, Secco, G, et al. Vitamin D deficiency and glycemic status in children and adolescents with type 1 diabetes mellitus. PLoS One. (2016) 11:e0162554. doi: 10.1371/journal.pone.0162554
39. Giri, D, Pintus, D, Burnside, G, Ghatak, A, Mehta, F, Paul, P, et al. Treating vitamin D deficiency in children with type I diabetes could improve their glycaemic control. BMC Res Notes. (2017) 10:1–5. doi: 10.1186/s13104-017-2794-3
40. Hafez, M, Hassan, M, Musa, N, Abdel Atty, S, and Azim, SA. Vitamin D status in Egyptian children with type 1 diabetes and the role of vitamin D replacement in glycemic control. J Pediatr Endocrinol Metab. (2017) 30:389–94. doi: 10.1515/jpem-2016-0292
41. Sharma, S, Biswal, N, Bethou, A, Rajappa, M, Kumar, S, and Vinayagam, V. Does vitamin D supplementation improve Glycaemic control in children with type 1 diabetes mellitus?- a randomized controlled trial. J Clin Diagn Res. (2017) 11:SC15-SC17. doi: 10.7860/JCDR/2017/27321.10645
42. Jaffey, JA, Kreisler, R, Graves, TK, Al-Nakkash, L, Backus, RC, and Allison, L. Ex vivo immune function and modulatory effects of calcitriol in dogs with naturally occurring diabetes mellitus. Vet Sci. (2024) 11:193. doi: 10.3390/vetsci11050193
43. Jaffey, JA, Amorim, J, and DeClue, AE. Effects of calcitriol on phagocytic function, toll-like receptor 4 expression, and cytokine production of canine leukocytes. Am J Vet Res. (2018) 79:1064–70. doi: 10.2460/ajvr.79.10.1064
44. Jaffey, JA, Kreisler, R, Shubitz, LF, Backus, RC, Hostnik, ET, Wycislo, KL, et al. Serum 25-hydroxyvitamin D concentrations in dogs with coccidioidomycosis and variables associated with extent of clinically evident disease. J Vet Intern Med. (2023) 37:150–60. doi: 10.1111/jvim.16629
45. Ryu, MO, Kim, BG, Choi, US, Baek, KH, Song, YK, Li, Q, et al. Extracellular cyclic adenosine monophosphate-dependent protein kinase a autoantibody and C-reactive protein as serum biomarkers for diagnosis of cancer in dogs. Vet Comp Oncol. (2019) 17:99–106. doi: 10.1111/vco.12450
46. Backus, RC, and Ueda, DC. Age-dependent changes in plasma concentrations of 25-hydroxyvitamin D may complicate vitamin D status assessment of immature cats. Front Vet Sci. (2024) 11:1365204. doi: 10.3389/fvets.2024.1365204
47. Ginsberg, C, Hoofnagle, AN, Katz, R, Becker, JO, Kritchevsky, SB, Shlipak, MG, et al. The vitamin D metabolite ratio is independent of vitamin D binding protein concentration. Clin Chem. (2021) 67:385–93. doi: 10.1093/clinchem/hvaa238
48. Schober, P, Boer, C, and Schwarte, LA. Correlation coefficients: appropriate use and interpretation. Anesth Analg. (2018) 126:1763–8. doi: 10.1213/ANE.0000000000002864
49. Reusch, C, Liehs, M, and Hoyer, M. Fructosamine. A new parameter for diagnosis and metabolic control in diabetic dogs and cats. J Vet Intern Med. (1993) 7:177–82. doi: 10.1111/j.1939-1676.1993.tb03183.x
50. Kaza, M, Tsentidis, C, Vlachopapadopoulou, E, Sakou, I-I, Karanasios, S, Mastorakos, G, et al. The effect of metabolic profile on leptin, adiponectin, and hs-crp in children and adolescents with type 1 diabetes. Children. (2022) 9:1162. doi: 10.3390/children9081162
51. Llauradó, G, Gallart, L, Tirado, R, Megia, A, Simón, I, Caixàs, A, et al. Insulin resistance, low-grade inflammation and type 1 diabetes mellitus. Acta Diabetol. (2012) 49:33–9. doi: 10.1007/s00592-011-0257-1
52. Shelbaya, S, Amer, H, Seddik, S, Allah, A, Sabry, I, Mohamed, T, et al. Study of the role of interleukin-6 and highly sensitive C-reactive protein in diabetic nephropathy in type 1 diabetic patients. Eur Rev Med Pharmacol Sci. (2012) 16:176–82.
53. Ahmed, N . Advanced glycation endproducts—role in pathology of diabetic complications. Diabetes Res Clin Pract. (2005) 67:3–21. doi: 10.1016/j.diabres.2004.09.004
54. Kaplan, M, Tendler, Y, Mahamid, R, Shiner, M, Aviram, M, and Hayek, T. High glucose upregulates C-reactive protein synthesis in macrophages. Clin Chem. (2010) 56:1036–8. doi: 10.1373/clinchem.2009.136838
55. Liu, F, Chen, H-Y, Huang, X, Chung, ACK, Zhou, L, Fu, P, et al. C-reactive protein promotes diabetic kidney disease in a mouse model of type 1 diabetes. Diabetologia. (2011) 54:2713–23. doi: 10.1007/s00125-011-2237-y
56. Vlassara, H, and Palace, M. Diabetes and advanced glycation endproducts. J Intern Med. (2002) 251:87–101. doi: 10.1046/j.1365-2796.2002.00932.x
57. Borilova Linhartova, P, Kavrikova, D, Tomandlova, M, Poskerova, H, Rehka, V, Dusek, L, et al. Differences in Interleukin-8 plasma levels between diabetic patients and healthy individuals independently on their periodontal status. Int J Mol Sci. (2018) 19:214. doi: 10.3390/ijms19103214
58. Erbagci, AB, Tarakcioglu, M, Coskun, Y, Sivasli, E, and Sibel Namiduru, E. Mediators of inflammation in children with type I diabetes mellitus: cytokines in type I diabetic children. Clin Biochem. (2001) 34:645–50. doi: 10.1016/s0009-9120(01)00275-2
59. Lo, HC, Lin, SC, and Wang, YM. The relationship among serum cytokines, chemokine, nitric oxide, and leptin in children with type 1 diabetes mellitus. Clin Biochem. (2004) 37:666–72. doi: 10.1016/j.clinbiochem.2004.02.002
60. Van Sickle, BJ, Simmons, J, Hall, R, Raines, M, Ness, K, and Spagnoli, A. Increased circulating IL-8 is associated with reduced IGF-1 and related to poor metabolic control in adolescents with type 1 diabetes mellitus. Cytokine. (2009) 48:290–4. doi: 10.1016/j.cyto.2009.08.011
61. Zozulinska, D, Majchrzak, A, Sobieska, M, Wiktorowicz, K, and Wierusz-Wysocka, B. Serum interleukin-8 level is increased in diabetic patients. Diabetologia. (1999) 42:117–8. doi: 10.1007/s001250051124
62. Chettab, K, Zibara, K, Belaiba, SR, and McGregor, JL. Acute hyperglycaemia induces changes in the transcription levels of 4 major genes in human endothelial cells: macroarrays-based expression analysis. Thromb Haemost. (2002) 87:141–8. doi: 10.1055/s-0037-1612957
63. Jain, SK, Rains, JL, and Croad, JL. High glucose and ketosis (acetoacetate) increases, and chromium niacinate decreases, IL-6, IL-8, and MCP-1 secretion and oxidative stress in U937 monocytes. Antioxid Redox Signal. (2007) 9:1581–90. doi: 10.1089/ars.2007.1577
64. Kobashi, C, Asamizu, S, Ishiki, M, Iwata, M, Usui, I, Yamazaki, K, et al. Inhibitory effect of IL-8 on insulin action in human adipocytes via MAP kinase pathway. J Inflamm. (2009) 6:25. doi: 10.1186/1476-9255-6-25
65. Feng, S, Yu, H, Yu, Y, Geng, Y, Li, D, Yang, C, et al. Levels of inflammatory cytokines IL-1β, IL-6, IL-8, IL-17A, and TNF-α in aqueous humour of patients with diabetic retinopathy. J Diabetes Res. (2018) 2018:1–6. doi: 10.1155/2018/8546423
66. Liu, SY, Chen, J, and Li, YF. Clinical significance of serum interleukin-8 and soluble tumor necrosis factor-like weak inducer of apoptosis levels in patients with diabetic nephropathy. J Diabetes Invest. (2018) 9:1182–8. doi: 10.1111/jdi.12828
67. Calvalido, J, Wood, GA, Mutsaers, AJ, Wood, D, Sears, W, and Woods, JP. Comparison of serum cytokine levels between dogs with multicentric lymphoma and healthy dogs. Vet Immunol Immunopathol. (2016) 182:106–14. doi: 10.1016/j.vetimm.2016.10.009
68. Goggs, R, and Letendre, JA. Evaluation of the host cytokine response in dogs with sepsis and noninfectious systemic inflammatory response syndrome. J Vet Emerg Crit Care (San Antonio). (2019) 29:593–603. doi: 10.1111/vec.12903
69. Johnson, V, Burgess, B, Morley, P, Bragg, R, Avery, A, and Dow, S. Comparison of cytokine responses between dogs with sepsis and dogs with immune-mediated hemolytic anemia. Vet Immunol Immunopathol. (2016) 180:15–20. doi: 10.1016/j.vetimm.2016.08.010
70. Karlsson, I, Hagman, R, Johannisson, A, Wang, L, Sodersten, F, and Wernersson, S. Multiplex cytokine analyses in dogs with pyometra suggest involvement of KC-like chemokine in canine bacterial sepsis. Vet Immunol Immunopathol. (2016) 170:41–6. doi: 10.1016/j.vetimm.2016.01.005
71. Kjelgaard-Hansen, M, Goggs, R, Wiinberg, B, and Chan, DL. Use of serum concentrations of interleukin-18 and monocyte chemoattractant protein-1 as prognostic indicators in primary immune-mediated hemolytic anemia in dogs. J Vet Intern Med. (2011) 25:76–82. doi: 10.1111/j.1939-1676.2010.0642.x
72. Alba-Loureiro, TC, Hirabara, S, Mendonca, J, Curi, R, and Pithon-Curi, TC. Diabetes causes marked changes in function and metabolism of rat neutrophils. J Endocrinol. (2006) 188:295–303. doi: 10.1677/joe.1.06438
73. Bilgic, S, Aktas, E, Salman, F, Ersahin, G, Erten, G, Yilmaz, M, et al. Intracytoplasmic cytokine levels and neutrophil functions in early clinical stage of type 1 diabetes. Diabetes Res Clin Pract. (2008) 79:31–6. doi: 10.1016/j.diabres.2007.06.011
74. Gin, H, Brottier, E, and Aubertin, J. Influence of glycaemic normalisation by an artificial pancreas on phagocytic and bactericidal functions of granulocytes in insulin dependent diabetic patients. J Clin Pathol. (1984) 37:1029–31. doi: 10.1136/jcp.37.9.1029
75. Marhoffer, W, Stein, M, Mäser, E, and Federlin, K. Reduced phagocytic capacity of circulating granulocytes in diabetes mellitus. Immunitat Infektion. (1992) 20:10–2.
76. Pettersson, US, Christoffersson, G, Massena, S, Ahl, D, Jansson, L, Henriksnäs, J, et al. Increased recruitment but impaired function of leukocytes during inflammation in mouse models of type 1 and type 2 diabetes. PLoS One. (2011) 6:e22480. doi: 10.1371/journal.pone.0022480
77. Rodriguez-Fernandez, S, Murillo, M, Villalba, A, Perna-Barrull, D, Cano-Sarabia, M, Gomez-Munoz, L, et al. Impaired phagocytosis in dendritic cells from pediatric patients with type 1 diabetes does not hamper their Tolerogenic potential. Front Immunol. (2019) 10:2811. doi: 10.3389/fimmu.2019.02811
78. Shetty, N, Thomas, B, and Ramesh, A. Comparison of neutrophil functions in diabetic and healthy subjects with chronic generalized periodontitis. J Indian Soc Periodontol. (2008) 12:41–4. doi: 10.4103/0972-124X.44089
79. Ellger, B, Debaveye, Y, Vanhorebeek, I, Langouche, L, Giulietti, A, Van Etten, E, et al. Survival benefits of intensive insulin therapy in critical illness: impact of maintaining normoglycemia versus glycemia-independent actions of insulin. Diabetes. (2006) 55:1096–105. doi: 10.2337/diabetes.55.04.06.db05-1434
80. Weekers, F, Giulietti, A-P, Michalaki, M, Coopmans, W, Van Herck, E, Mathieu, C, et al. Metabolic, endocrine, and immune effects of stress hyperglycemia in a rabbit model of prolonged critical illness. Endocrinology. (2003) 144:5329–38. doi: 10.1210/en.2003-0697
81. Yano, H, Kinoshita, M, Fujino, K, Nakashima, M, Yamamoto, Y, Miyazaki, H, et al. Insulin treatment directly restores neutrophil phagocytosis and bactericidal activity in diabetic mice and thereby improves surgical site Staphylococcus aureus infection. Infect Immun. (2012) 80:4409–16. doi: 10.1128/IAI.00787-12
82. Ali, R, Fawzy, I, Mohsen, I, and Settin, A. Evaluation of vitamin D receptor gene polymorphisms (Fok-I and Bsm-I) in T1DM Saudi children. J Clin Lab Anal. (2018) 32:e22397. doi: 10.1002/jcla.22397
83. Hussein, AG, Mohamed, RH, and Alghobashy, AA. Synergism of CYP2R1 and CYP27B1 polymorphisms and susceptibility to type 1 diabetes in Egyptian children. Cell Immunol. (2012) 279:42–5. doi: 10.1016/j.cellimm.2012.08.006
84. Ongagna, J, Pinget, M, and Belcourt, A. Vitamin D-binding protein gene polymorphism association with IA-2 autoantibodies in type 1 diabetes. Clin Biochem. (2005) 38:415–9. doi: 10.1016/j.clinbiochem.2004.12.013
85. Sharp, CR, Selting, KA, and Ringold, R. The effect of diet on serum 25-hydroxyvitamin D concentrations in dogs. BMC Res Notes. (2015) 8:1–6. doi: 10.1186/s13104-015-1360-0
86. Afzal, S, Brondum-Jacobsen, P, Bojesen, SE, and Nordestgaard, BG. Genetically low vitamin D concentrations and increased mortality: Mendelian randomisation analysis in three large cohorts. BMJ. (2014) 349:g6330. doi: 10.1136/bmj.g6330
87. Charoenngam, N, and Holick, MF. Immunologic effects of vitamin D on human health and disease. Nutrients. (2020) 12:2097. doi: 10.3390/nu12072097
88. Backus, RC, and Foster, LR. Investigation of the effects of dietary supplementation with 25-hydroxyvitamin D3 and vitamin D3 on indicators of vitamin D status in healthy dogs. Am J Vet Res. (2021) 82:722–36. doi: 10.2460/ajvr.82.9.722
89. Del Baldo, F, Magna, L, Dondi, F, Maramieri, P, Catrina, OM, Corradini, S, et al. Comparison of serum fructosamine and glycated hemoglobin values for assessment of glycemic control in dogs with diabetes mellitus. Am J Vet Res. (2020) 81:233–42. doi: 10.2460/ajvr.81.3.233
90. Zeugswetter, FK, Beer, R, and Schwendenwein, I. Evaluation of fructosamine concentration as an index marker for glycaemic control in diabetic dogs. Vet Rec. (2022) 190:no-no. doi: 10.1002/vetr.244
91. Foss-Freitas, MC, Foss, NT, Donadi, EA, and Foss, MC. In vitro TNF-alpha and IL-6 production by adherent peripheral blood mononuclear cells obtained from type 1 and type 2 diabetic patients evaluated according to the metabolic control. Ann N Y Acad Sci. (2006) 1079:177–80. doi: 10.1196/annals.1375.027
92. Foss-Freitas, MC, Foss, NT, Rassi, DM, Donadi, EA, and Foss, MC. Evaluation of cytokine production from peripheral blood mononuclear cells of type 1 diabetic patients: importance of the methodologic approach. Ann N Y Acad Sci. (2008) 1150:290–6. doi: 10.1196/annals.1447.053
Keywords: 25(OH)D, 25-hydroxyvitamin D, C-reactive protein, cytokines, glycemic control
Citation: Jaffey JA, Backus RC, Kreisler R, Graves TK, Al-Nakkash L and Allison L (2024) Evaluation of serum vitamin D metabolites, phagocytosis, and biomarkers of inflammation in dogs with naturally occurring diabetes mellitus. Front. Vet. Sci. 11:1441993. doi: 10.3389/fvets.2024.1441993
Edited by:
Carlos Eduardo Fonseca-Alves, Paulista University, BrazilReviewed by:
Victor Alejandro Castillo, University of Buenos Aires, ArgentinaTaesik Yun, Chungbuk National University, Republic of Korea
Copyright © 2024 Jaffey, Backus, Kreisler, Graves, Al-Nakkash and Allison. This is an open-access article distributed under the terms of the Creative Commons Attribution License (CC BY). The use, distribution or reproduction in other forums is permitted, provided the original author(s) and the copyright owner(s) are credited and that the original publication in this journal is cited, in accordance with accepted academic practice. No use, distribution or reproduction is permitted which does not comply with these terms.
*Correspondence: Jared A. Jaffey, amphZmZlQG1pZHdlc3Rlcm4uZWR1