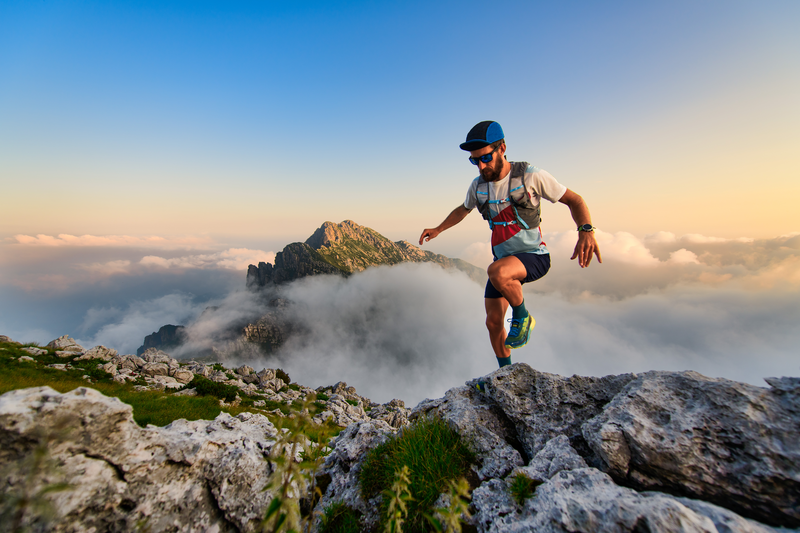
94% of researchers rate our articles as excellent or good
Learn more about the work of our research integrity team to safeguard the quality of each article we publish.
Find out more
ORIGINAL RESEARCH article
Front. Vet. Sci. , 12 December 2024
Sec. Animal Nutrition and Metabolism
Volume 11 - 2024 | https://doi.org/10.3389/fvets.2024.1431091
This article is part of the Research Topic Functional Nutritional Strategies as Alternatives to Antimicrobials View all 19 articles
Introduction: As the livestock industry grapples with the need for sustainable land, maintaining production systems, and reducing antimicrobial resistance, the application of functional nutrition emerges as a potential solution.
Aim: In line with the One Health principles, this study aims to evaluate functional properties of Ascophyllum nodosum and Phymatolithon calcareum, and assess the effects of their dietary supplementation on piglets’ health.
Materials and methods: A chemical-functional characterization was conducted before and after in vitro digestion. Total Polyphenols Content (TPC) and Total Flavonoid Content (TFC) were determined through colorimetric assays, while antioxidant activity was determined using ABTS assay, and the microdilution method was used to evaluate the antimicrobial capacity. For the in vivo trial twenty-four post-weaning pigs (28 ± 2 days, 6.89 ± 0.820 Kg) were enrolled in two homogeneous groups (n = 12/group): control group (CTRL) fed a commercial diet, and treated group (ALGAE) fed commercial diet with the addition of 1.5% of A. nodosum and 0.5% of P. calcareum for 27 days. Weekly, zootechnical performances were assessed monitoring the body weight and the individual feed intake. Fecal samples were collected to evaluate the abundance of total, lactic acid and coliform bacteria through plate counting. Serum were obtained at day 0 and day 27 to assess the antioxidant barrier.
Results and discussion: The chemical characterization discloses that the minerals’ level remains below the maximum thresholds defined for their use in piglets nutrition. TPC was 330.42 ± 21.372 mg TAE/g of the sample and 11.45 ± 0.521 mg TAE/g of the sample for A. nodosum and P. calcareum, respectively, and a similar trend was found in the TFC evaluation (213.85 ± 20.557 and 2.71 ± 0.900 mg CE/g of sample, respectively). Our results also highlighted that polyphenols and flavonoid compounds persisted after in vitro digestion as well as the functional properties. The administration of algae in piglets diet, although it slightly affected feed efficiency in the first period of the trial, did not affect the animal growth in terms of weight and average daily gain. Microbiological analysis of feces showed similar values between the two experimental groups over 27 days. A significantly higher serum antioxidant barrier was registered in ALGAE compared to CTRL group at day 27 (363.26 ± 16.241 vs. 230.69 ± 32.078 HClO/mL, p < 0.05).
Conclusion: In conclusion, the supplementation with A. nodosum and P. calcareum could be considered a promising dietary strategy to enhance the oxidative barrier in weaned piglets.
In recent years, sustainable development has become a prominent topic of discussion, fueled by the depletion of natural resources and environmental shifts caused by both population growth and the expansion of agro-industrial production (1). In this context, embracing the principles of agroecology is becoming increasingly relevant. Agroecology offers holistic and environmentally friendly approaches to agricultural practices, emphasizing the integration of ecological principles into food production systems (2). Indeed, in the livestock sector, the concept of nutritional ecology has become crucial (3). This approach emphasizes understanding the nutritional requirements of animals in their ecological context, considering factors such as diet composition, feeding behavior, and interactions with the environment. By applying principles of nutritional ecology, livestock producers can optimize feed efficiency, minimize environmental impacts, and promote animal health and welfare (4). In this scenario, another pressing concern is antimicrobial resistance (AMR), recognized by the World Health Organization (WHO) as one of the top ten public health threats worldwide, which poses a significant threat to animal health, human health, and global food security. For these reasons, given the imperative to reduce antibiotics (5) and promote their responsible use (6), exploring alternatives to antibiotics is essential especially during the critical phases of animal production. In this context, animal nutrition emerges as a pivotal factor. Beyond meeting essential nutrient requirements, functional ingredients can play a crucial role in promoting animal health and diminishing reliance on antibiotics (7). In the pig farming system, antibiotic drugs are commonly employed to manage diseases occurring during critical phases of the animal’s life, such as the weaning phase. During this period, piglets, still immature in terms of digestion and immunity, encounter various stressors including separation from the mother, environmental and social changes, hierarchical struggles for food access, transitioning from milk to starter feed, and gradual loss of passive immunity (8). These stressors activate the hypothalamic–pituitary–adrenal axis, releasing glucocorticoids that heighten susceptibility to potential pathogens (9). A frequent disorder during the weaning phase is post-weaning diarrhea (PWD), primarily attributed to certain strains of Escherichia coli. PWD leads to significant economic losses, elevated rates of morbidity and mortality, and a decline in productive performance (10–12). Hence, there is a clear need to reduce the occurrence of PWD enhancing animal health and the profitability of agricultural sector. Simultaneously, it’s crucial to minimize antibiotic usage to mitigate antibiotic resistance problems (13–15). With this premise, animal nutrition should aim not only to meet the nutritional requirements of animals but also to adhere to agroecological principles and contribute to the reduction of antibiotics in livestock production. In this regard, functional ingredients can be supplemented into animal feed to enhance overall health, improve performance, or address specific health concerns. Functional ingredients can include a wide range of substances such as antioxidants, natural by-products, and other bioactive compounds. Among all possible ingredients proposed, algae, emerges due to their composition, and the fact that they can be grown on non-arable land, making them valuable as functional ingredients. In addition to their nutritional qualities, they are a rich source of many biologically active compounds and one of the richest sources of natural antioxidants and antimicrobial compounds (16), this makes them ingredients deserving special attention for animal nutrition purposes. Algae are the most common organisms in aquatic environments and belong to a complex heterogeneous group in terms of ecological, taxonomic, morphological and biochemical aspects and are therefore divided into two main categories (microalgae and seaweeds) both actually used in animal nutrition (17, 18). Among these, Ascophyllum nodosum is a brown alga commonly studied in animal nutrition due to its content of vitamins, trace elements, lipids, carbohydrates, proteins, and iodine (19). Also, red algae are studied; among these, Phymatolithon calcareum (formerly Lithothamnium calcareum) (Rhodophyta) has been successfully used in cattle feed where, in addition to being an alternative mineral source, it can modulate the rumen pH (20, 21). A previous study conducted by our research group showed that the combination of A. nodosum and P. calcareum might exhibit a synergistic effect disclosing a high antioxidant and antimicrobial power (22).
The use of algae in animal nutrition, especially in pig farming, still needs to be explored. Existing literature reports variable and sometimes conflicting results, mainly focusing on supplementing individual algal species. Therefore, this study aimed to investigate the chemical-functional properties of A. nodosum and P. calcareum in vitro. Subsequently, these algal species were supplemented into a post-weaning pigs’ diet to evaluate their effect on zootechnical performance and health status.
To determine the main nutritional components (ash, crude fiber, crude protein, ether extract), the “Official Methods of Analysis” according to AOAC were used (23). Briefly, dry matter (DM) was obtained by drying the samples in a forced-air oven at 65°C for 24 h (AOAC method 930.15). Ash (Ash) was obtained by placing the samples in a muffle furnace at 550°C for at least 3 h (AOAC method 942.05). Crude fiber (CF) was determined by the filter bag method (AOCS method Ba 6a-05) (24). Crude protein (CP) was determined by the Kjeldahl method using 6.25 as nitrogen conversion factor (AOAC method 2001.11). Ether extract (EE) was determined by ether extraction in the Soxtec system (AOAC 2003.05).
The content of micro- and macro-elements (Ag; Al; As; B; Ba; Ca; Cd; Co; Cr; Cu; Fe; K; Mg; Mn; Mo; Na; Ni; P; Pb; Sb; Se; Sr.; Th; Ti 47; Ti 48; Tl; U; V; Zn) contained in the two algae was evaluated after mineralization with inductively coupled plasma mass spectrometry (ICP-MS). Firstly, the dried algae were digested with a high-performance Microwave Digestion system Milestone ETHOS UP. Specifically, 500 mg of A. nodosum was diluted with 9 mL of 69% HNO3 plus 1 mL of H2O2 and digested by applying a one-step temperature ramp (at 190°C in 15 min and maintained for 15 min; 1800 MW). While for P. calcareum 250 mg of dried sample was diluted with 10 mL of 69% HNO3 and digested by applying a one-step temperature ramp (at 210°C in 20 min and maintained for 20 min; 1800 MW). Then, the samples were diluted to the concentration of 14.84 mg/L and 7.44 mg/L for A. nodosum and P. calcareum, respectively. Before proceeding with the ICP-MS analysis a calibration curves for each element considered were obtained using certified reference materials purchased by Agilent (Santa Clara, CA, United State). The concentration of elements was measured by ICP-MS (Agilent 7,900). The data obtained were finally analyzed with Software MassHunter Workstation for 7,900 ICP-MS, Version C.01.01 (Agilent Technologies 2015).
According to Gouvinhas et al. (25), solvent extraction was performed with some adaptations. Briefly, 40 mg of A. nodosum and P. calcareum dried meal were dissolved in 1.5 mL of methanol/deionized water (1:1, v/v). The mixture was then vortexed and stirred at room temperature (RT) for 30 min. Samples were centrifuged for 15 min at 10,000 rpm at 4°C. The supernatants were collected, filtered through a 0.20 μm syringe filter and stored at −20°C until the analysis.
The phenolic content of algae extracts was evaluated by the Folin–Ciocalteu method, according to Attard (26). Briefly, the assay was performed by reacting 50 μL of sample with 500 μL of 1:10 Folin–Ciocalteu reagent and 400 μL sodium carbonate (1 M). After 15 min of incubation at RT in the dark absorbance was read at 630 nm. The Total Phenolic Content (TPC) was expressed as tannic acid equivalents equivalents (mg TAE/100 g). Each sample and standard were run in triplicate.
The flavonoid content of algae extracts was evaluated according to Herald (27). Briefly, 250 μL of extract, 1 mL of distilled water, and 75 μL of NaNO2 (50 g/L) were combined and mixed. Next, 150 μL of AlCl3 (100 g/L) was added to the solution. After 6 min 500 μL of NaOH (1 mol/L) and 500 μL of distilled water were added. The solution was centrifuged at 3220 g for 5 min at RT, the supernatant was collected, and absorbance was measured at 510 nm. Total flavonoid concentration was expressed as mg catechin equivalent (CE) g-1 sample. Each sample and standard were run in triplicate.
The scavenging activity of algae extracts was evaluated through ABTS assay, as previously performed in our previous study (28). Briefly, 10 μL of the sample was added to 1 mL of ABTS•+ working solution. The absorbance was recorded at 734 nm after 6 min of incubation, performing all the determinations in triplicate. ABTS radical scavenging activity was expressed as the percentage of the inhibition of radical scavenging activity (PI%).
Evaluation of the antimicrobial activity of A. nodosum and P. calcareum was carried out by a growth inhibition assay based on liquid culture with F4+ Escherichia coli. In detail, a culture of F4+ E. coli prepared in Luria-Bertani (LB) medium and allowed to grow overnight was used as the inoculum for the experiments. The growth inhibition assay was performed as follows: algal extracts were diluted in LB liquid medium to obtain a dilution of 1:8 (v/v). Then 100 μL of the diluted extract was placed in a 96-well microtiter plate into which 30 μL of the E. coli inoculum was added. Positive controls were prepared by adding 30 μL of the E. coli inoculum to the methanol/distilled water (1:1, v/v) solution to assess bacterial growth without external influences. Negative controls, on the other hand, were prepared by adding 30 μL of LB without the E. coli inoculum. Then all samples were incubated at 37°C in a shaking incubator for six hours. The growth of E. coli was assessed every hour for six hours by measuring the absorbance with a microplate reading spectrophotometer at an optical density (OD) of 620 nm. The measured optical density was converted to log10 of the number of cells/mL, considering 1 OD = 1 × 109 cells/mL. All assays were performed in technical quadruplicates and with three biological triplicates that were intended to verify the replicability of the experiment using the same procedures, which included repeating the experiment from sample extraction and repeating the assay on different days (29).
In vitro digestion was performed according to the COST INFOGEST method, simulating the three main stages of digestion (oral, gastric, and intestinal) using salivary, gastric, and intestinal fluids made according to the protocol (Supplementary Table S1) (30). Briefly, 2 g of algae powder was mixed with 2 mL simulated saliva fluid stock solution, and 200 μL of α-amylase (Sigma–Aldrich A3176–500KU) solution of 1,500 U/mL was added. Then, pH was adjusted to 7 with 1 mol/L NaOH and incubated in a water bath at 37°C for 2 min with constant shaking. In the gastric phase, the oral bolus was mixed with 3.2 mL of simulated gastric fluid stock solution followed by 200 μL pepsin (Sigma–Aldrich P7000) solution of 25,000 U/mL. Then, pH was adjusted to 3 with 1 mol/L HCl, followed by an incubation during 2 h under the same conditions as in the oral phase. In the final phase, gastric chyme was mixed with 3.4 mL of simulated intestinal fluid stock solution, 2 mL of pancreatin (Sigma–Aldrich P1625) solution of 800 U/mL, and 1 mL of bile (Sigma–Aldrich B8631) and NaOH (to adjust the pH 7). NaOH (1 mol/L) was used to set the pH back to 7. A final incubation was carried out at 37°C for 2 h. Following in vitro digestion, the resulting undigested fraction (UF) was filtered through pre-weighed Whatman no. 54 filter paper in a porcelain funnel and dried overnight at 65°C. This dried UF was used to calculate the in vitro digestibility (IVD) according to the following formula (31):
At the end of each digestive phase, 2 mL aliquots of the digested fraction were taken and stored at −80°C to stop the enzymatic activity. Subsequently, they were used to evaluate the TPC and TFC during in vitro digestion following the procedure described in Sections 2.2.2 and 2.2.3 above.
The assessment of antioxidant activity following in vitro digestion phases was performed by evaluating the ABTS radical scavenging activity according to Hsu et al. (32), as described in Section 2.2.4. On the other hand, the determination of the growth inhibitory activity against F4+ E. coli was determined as described in Section 2.2.5.
The experimental trial was approved by the Animal Welfare Organization of University of Milan and the Italian Ministry of Health (n° 884/2021-PR) and performed following European regulations (33). The trial was held at the swine sector of the Experimental Zootechnical Education Center of the University of Milan, Faculty of Veterinary Medicine, based in Lodi. Twenty-four piglets (Landrace X Large White) weaned at 28 ± 2 days were identified by individual ear tags and randomly divided into two experimental groups (CTRL and ALGAE) homogeneous by sex (50% male and 50% female) and weight (6.89 ± 0.820 kg). Homogeneous macro- and microclimatic conditions were maintained throughout the experimental phase with passive forced ventilation, and the temperature was kept around 26°C with a relative humidity of 65%. Animals were housed, for 27 days, and after six days of an adaptation period during which the animals were fed the same basal diet, piglets were assigned to two experimental groups and were fed ad libitum: the control group (CTRL: 12 piglets, 12 pens, 7.81 ± 0.786 kg) was fed with the basal diet; the ALGAE group (ALGAE: 12 piglets, 12 pens, 7.75 ± 1.157 kg) fed with the basal diet supplemented with the addition of 1.5% of A. nodosum plus 0.5% of P. calcareum. All the diets were isonitrogenous and isoenergetic (Table 1) balanced using Plurimix System®software (Fabermatica, Cremona, Italy) in line with nutritional requirements for post-weaned piglets (NCR, 2012) and were provided by Ferraroni S.p.A. (Cremona, Italy). Considering the small inclusion percentage, to ensure a homogeneous dispersion of seaweeds meal, A. nodosum and P. calcareum were premixed with 5 kg of basal feed and then mixed for 20 min with the basal diet. Experimental diets were analyzed in duplicate for principal nutrient content: dry matter (DM), crude protein (CP), ether extract (EE), crude fiber (CF), and ash concentrations, according to “Official Methods of Analysis” (23) as described above. Moreover, the starch content was determined through the Total Starch Assay Kit purchased by Megazyme (Ireland) following the manufacture instruction.
Body weight (BW) was recorded individually at day 0 (T0), day 7 (T1), day 13 (T2), day 21 (T3), and day 24 (T4). Feed intake was recorded weekly by measuring the feed refused for each pen, considering the pen as the experimental unit. Other performance parameters: average daily gain (ADG), average daily feed intake (ADFI), and feed conversion ratio (FCR), were calculated according to the following formulas:
Fecal samples for microbiological analysis were collected from each piglet on day 0 (T0), day 7 (T1), day 13 (T2), day 21 (T3), and day 24 (T4) (Figure 1). Diarrhea occurrence was recorded daily by evaluating the fecal consistency, which was given a fecal score: a four-level scale (0 = dried consistency, 1 = soft consistency, 2 = mild diarrhea, 3 = severe diarrhea) according to Dell’Anno et al. (34). The fecal color was evaluated through a three-level color scale: 1 = yellowish, 2 = greenish, and 3 = brown; considering ≥2 as a normal score (35). Blood samples were obtained from the jugular vein at T0, T2, and T4 through vacuum tubes without anticoagulants for oxidative barrier analysis.
Figure 1. Experimental design from arrival date (28 days of animal age) to T4 (63 days of animal age) divided according to the diet administered.
Fecal samples were analyzed for the total countable bacteria, lactic acid bacteria, and coliform bacteria through three different types of culture media: Plate Count Agar (PCA), De Man, Rogosa, and Sharpe Agar (MRS), and Violet Red Bile Agar (VRBA), respectively. Briefly, one gram of fecal sample was diluted 1:10 with sterile 0.9% NaCl solution and homogenized by vortexing. Samples were then serially diluted tenfold, and microorganisms were enumerated by plate counting after (i) 36 h at 30°C for total bacteria and Lactic acid bacteria, (ii) 18–24 h 35°C under microaerophilic conditions for coliform bacteria (36). The lactic acid/coliform bacteria ratio was calculated using MRS and VRBA agar plate counting data. The results were expressed as log10 colony-forming units per gram of fresh feces (log10 CFU/g).
The following formula was used to calculate protein intake according to Augenstein et al. (37):
Feces collected at T0 and T4 were dried in a forced-air oven and analyzed for nitrogen content (AOAC method 930.15; AOAC method 2001.11) (23). Protein content was calculated using 6.25 as a conversion factor (38). Apparent protein digestibility was assessed through an indirect marker analyzing the content of acid-insoluble ash (AIA) in both feed and feces (39). The relative digestibility of protein was calculated using the following formula:
To determine the serum antioxidant barrier, blood samples collected at T0, T2, and T4 were centrifuged at 3000 rpm for 15 min at 4°C. The obtained serum was analyzed through an Oxy-adsorbent test (Diacron, Grosseto, Italy) according to the manufacturer’s instructions. Absorbances were measured after 10 min of incubation at 37°C using a UV–Vis spectrophotometer (V630 UV–Vis, Jasco GmBH, Pfungstadt, Germany) at 546 nm.
The results were then expressed as μmol di HClO/mL according to the following formula:
The data concerning the chemical and functional characterization of A. nodosum, P. calcareum and their combination were analysed using GraphPad Prism (version 9.0.0, Boston, MA, USA). The normality of the distribution of the data and residuals was evaluated by Shapiro–Wilk and D’Agostino–Pearson tests. For the total polyphenol and flavonoid content, and the antioxidant activity of the extract the data were analysed using one-way analysis of variance (ANOVA). For the growth inhibition assay, the data were analyzed using a two-way analysis of variance, which included the effects of treatment, time, and their interaction. As well, all the analyses concerning the functional characterization after the in vitro digestion were performed by applying the two-way analysis of variance. Post hoc pairwise comparisons were performed using Sidak’s test. Results derived from biological replicates were considered for statistical analysis of the in vitro assays. For the in vivo trial the number of animals (twelve for each group) was defined a priori using the GPower software, version 3.1.9.7., considering: (i) a power test of 80%, (ii) a protection level of 95%, (iii) an effect size of 0.5 (40–42). The effect size was defined according to previous studies that adopted similar experimental design for limiting the number of experimental animals according to the 3R principle (35, 43). Analyses were conducted using a repeated-measures two-way ANOVA. The results were evaluated using a full factorial model (Treatment: Trt, Time: Time, Interaction: Trt X Time). Diarrhea frequency was obtained by converting the fecal score data into a dichotomous variable (presence or absence) to evaluate observed frequencies through Pearson’s Chi-square test. For the in vivo trial, the individual animal constituted the experimental unit, so the number of replications was 12 for each group. The data are reported as the mean ± standard deviation (SD) or as mean ± standard error (SEM), and differences were statistically significant at p ≤ 0.05.
Table 2 shows that both species tested had a high ash content, equal to 25.33 and 92.75%, respectively, for A. nodosum and P. calcareum. Specifically, analysis by ICP/MS allowed for the identification of the minerals’ profile present in the analyzed seaweeds (Table 2). In particular, the ICP/MS analysis disclosed that both A. nodosum and P. calcareum were rich in Strontium (Sr) (618.14 ± 8.166 mg/Kg and 2110.60 ± 33.640 mg/kg respectively), Iron (Fe) (407.94 ± 0.467 mg/Kg and 1004.90 ± 1.755 mg/Kg respectively) and Aluminum (Al) (211.28 ± 7.027 mg/Kg and 213.72 ± 6.386 mg/Kg respectively). Moreover, A. nodosum was mainly rich in Boron (B) and Manganese (Mn) (162.21 ± 1.613 mg/Kg and 100.80 ± 1.242 mg/kg respectively), while Titanium 48 (Ti 48) was particularly abundant in L. calcareum (456.87 ± 11.233 mg/Kg).
Table 2. Analyzed nutrient composition and mineral composition of Ascophyllum nodosum and Lithothamnium calcareum.
The evaluation of the Total Polyphenol Content (TPC) disclosed that A. nodosum had the highest concentration of TPC, equal to 330.42 ± 21.372 mg TAE/g of the sample, while P. calcareum showed a value of TPC equal to 11.45 ± 0.521 mg TAE/g of the sample (p < 0.001). A similar trend was also shown in the Total Flavonoid Content (TFC) evaluation. Obtained values were 213.85 ± 20.557 and 2.71 ± 0.900 mg CE/g of sample, respectively, for A. nodosum and P. calcareum (p < 0.001). Both assays showed values for the combination (1:1) of the two algae, which were half of those found for A. nodosum. Specifically, the TPC was found to be 140.42 ± 10.230 mg TAE/g of sample, while the TFC was found to be 107.07 ± 27.079 mg CE/g of sample.
The evaluation of the antioxidant activity of algae compounds showed that A. nodosum possessed higher antioxidant activity than P. calcareum (p < 0.001). In fact, the percentage of the inhibition of radical scavenging activity (PI%) of A. nodosum was 57.73 ± 1.444%, corresponding to 1313.22 ± 24.781 μMol Trolox eq/ g. On the other hand, the PI% of P. calcareum was of 2.96 ± 0.130%, which is equal to 20.07 ± 3.087 μMol Trolox eq/g. In addition, the extracts of the two algae placed in combination were analyzed to assess a possible synergistic effect. The results obtained showed that the PI% of the combination of the considered algae species is 48.85 ± 1.356%, resulting significantly higher (p = 0.0001) than that obtained by summing the inhibition rates of the algal extracts taken individually (45.10 ± 1.731%).
Growth inhibition was evaluated against F4+ Escherichia coli, belonging to bacterial strain collection of the Department of Veterinary Medicine and Animal Science at University of Milan (44). The results highlight that A. nodosum showed a higher growth inhibition capacity compared to both the combination and P. calcareum. In fact, it was observed that at a dilution of 1:8, starting from the first hour of incubation until the sixth hour of incubation, co-culture of A. nodosum with E. coli was able to lower significantly the growth of the microbial strain (p < 0.001) (Figure 2).
Figure 2. Ascophyllum nodosum, Lithothamnium calcareum, and their combination extract growth inhibition capacity against F4+ E. coli. The data are shown as the means and standard errors. Asterisks (n = 3) with different superscripts indicate significantly different means; **p = 0.002, ***p < 0.001. Time: p < 0.001; Treatment: p < 0.001; Time x Treatment: p < 0.001.
After the in vitro simulation of digestion process A. nodosum disclosed a percentage of digestibility equal to 21.06 ± 0.142%, while P. calcareum highlights a higher digestibility, which has been around 44.16 ± 0.488%.
The TPC and TFC were evaluated following the in vitro digestion process to assess the behavior of bioactive molecules following the digestive process. Our results, shown in Figure 3A, revealed that the polyphenol content increases as the digestive phases proceed. Specifically, after the oral phase, bioactive molecules of A. nodosum undergo a partial degradation that highlights a TPC content equal to 678.81 ± 53.684 mg TEA/g of sample. Following the subsequent digestive phases, the polyphenol content significantly increases to a value of 989.65 ± 16.968 mg TEA/g of sample after the intestinal phase (p < 0.001). As well as also for the combination of the algae species a significantly increasing trend was shown (p < 0.001), since starting from the end of the oral phase until the end of the intestinal phase the TPC is, respectively, 304.81 ± 46.089; 584.56 ± 9.391; 873.55 ± 52.294 mg TEA/g of sample. The evaluation of TPC of P. calcareum underlines that also in this case the TPC may slightly increase as the digestive process proceeds, although the difference is not statistically significant (p > 0.05). The same trend can be observed in flavonoids (Figure 3B). As highlighted from the polyphenol analysis during the first phase of digestion, the release of flavonoids was lower (113.78 ± 5.322 mg CE/g of sample for A. nodosum; 18.56 ± 2.634 mg CE/g of sample for P. calcareum; 89.63 ± 4.310 mg CE/g of sample for the combination), and then increase in the gastric and intestinal digestion phase (respectively: 396.61 ± 32.541 and 549.93 ± 16.856 mg CE/g of sample for A. nodosum, p < 0.05; 17.52 ± 3.040 and 21.26 ± 7.859 mg CE/g of sample for P. calcareum; 222.48 ± 8.490 and 445.31 ± 17.562 mg CE/g of sample for the combination, p < 0.05).
Figure 3. Phenolic and flavonoid compounds in Ascophyllum nodosum, Lithothamnium calcareum and their combination after the in vitro digestion. (A) Total phenolic content (TPC) after in vitro digestion. Time: p < 0.001; Treatment: p < 0.001; Time x Treatment: p < 0.001; (B) Total flavonoid content (TFC) after in vitro digestion. Time: p < 0.001; Treatment: p < 0.001; Time x Treatment: p < 0.001. TAE, tannic acid equivalent; CE, catechin equivalent. The data are shown as the means ± standard deviations (SDs) (n = 3). Asterisks (n = 3) with different superscripts are significantly different; ***p < 0.001.
Regarding the functional activity of the selected algal species the antioxidant activity and the growth inhibitory activity against F4+ E. coli were evaluated. Concerning the antioxidant activity, as shown in Figure 4, the percentage of inhibition increases proceeding with the digestive process for all the samples tested. In particular this increase was statistically significant for A. nodosum (p < 0.001) and for the combination (p < 0.04). As well as the algal extracts show an ability to inhibit the growth of F4+ E. coli this property remains even after the digestive process. In fact, both algal species considered and their combination when co-cultured with F4+ E. coli after undergoing the in vitro digestion process are able to significantly slow down its proliferation. As shown in Figure 5, it can be observed that from the second hour of incubation, the reduction in microbial growth for A. nodosum, P. calcareum and their combination.
Figure 4. Percentage inhibition of radical scavenging activity (PI%) after in vitro digestion of Ascophyllum nodosum, Lithothamnium calcareum, and their combination. The data are shown as the means ± standard deviations (SDs) (n = 3). Asterisks (n = 3) with different superscripts are significantly different; ***p < 0.001; *p = 0.04. Time: p < 0.001; Treatment: p < 0.001; Time x Treatment: p = 0.001.
Figure 5. Growth inhibition of Ascophyllum nodosum, Lithothamnium calcareum and their combination against E. coli F4+ after the digestive process. The data are shown as the means ± standard deviations (SDs) (n = 3). Asterisks (n = 3) with different superscripts are significantly different; ***p < 0.001, **p < 0.004, *p = 0.02. Time: p < 0.001; Treatment: p < 0.001; Time x Treatment: p < 0.001; Subject: p = 0.007.
Experimental diet evaluation of principal nutrient content revealed that nutrient concentrations are in line with NRC guidelines (45), thus fulfilling the nutritional requirements of weaned piglets. The inclusion of algae species did not influence the nutrient profile of treatment groups (Table 3).
The individual Body Weigh (BW) recorded weekly showed no significant differences throughout the experimental period (Figure 6A). Similarly, the Average Daily Gain (ADG) also showed no significant differences between the two experimental groups. In particular in the first part of the trial the ADG of the CTRL group was 135.70 ± 26.249 g/day and those of the ALGAE group was 187.50 ± 18.736 g/day, while in the second part of the trial the ADG was of 710.10 ± 26.862 g/day and 668.50 ± 29.377 g/day, respectively for the CRTL and ALGAE group. During the second part of the trial, the Average Daily Feed Intake (ADFI) of the CTRL group was significantly lower than that of the ALGAE group (587.50 ± 34.883 g/day and 726.20 ± 32.152 g/day, respectively; p = 0.0030). Finally, the Feed Conversion Ratio in the first half of the trial the control group has a statistically higher conversion index than the ALGAE group (3.44 ± 0.364 and 2.60 ± 0.254, respectively; p = 0.016), while in the second half of the trial both conversion indices decreased without showing a significant difference between the two groups (Figures 6B–D).
Figure 6. Zootechnical performance from T0 to T4. (A) Body weight at T0, T1, T2, T3, T4. Time: p = 0.0903; Treatment: p < 0.001; Time x Treatment: p = 0.5326; (B) Average Daily Gain (ADG) in the time interval T0 - T2 and T2 - T4. Time: p = 0.8631; Treatment: p < 0.001; Time x Treatment: p = 0.0426; Subject: p = 0.0914; (C) Average Daily Feed Intake (ADFI) in the time interval T0 - T2 and T2 - T4. Time: p = 0.2105; Treatment: p < 0.001; Time x Treatment: p < 0.002; Subject: p = 0.011; (D) Feed Conversion Rate (FCR) in the time interval T0 - T2 and T2 - T4. Time: p = 0.1860; Treatment: p < 0.001; Time x Treatment: p = 0.0152. The data are shown as the means ± standard error (SEM). Asterisks with different superscripts are significantly different; **p = 0.003, *p = 0.0166.
Considering the entire duration of the trial, the cases of diarrhea that occurred were the same for both the CTRL and ALGAE groups. Notably, cases of diarrhea were only observed in the first period of the trial where for both experimental groups the frequency of observed diarrhea was 3.85% out of the total number of observations. On the other hand, during the second period of the trial no cases of diarrhea were observed in either group.
Bacterial counts were performed on fecal samples for the determination of colony-forming units (CFU) of total, lactic, and coliform bacteria. The results obtained from the microbiological analysis of the feces (Figure 7A) showed that in both groups at the initial time (T0) the total bacteria present amounted to 2.14×107 ± 5.031×106 CFU/g, of these the majority were lactic acid bacteria (1.82×107 ± 2.038×107 CFU/g), while coliforms were present in a reason of 0.5% of the total. At T2 it is possible to observe that in both groups there was a 22% reduction in coliform bacteria count (Figure 7C). With regard to the evaluation of lactic acid bacteria at the same time point, it is possible to find, from the data obtained (Figure 7B), the administration of the feed fortified with algae allowed an increase in this bacterial genus, which was found to be present in an amount of CFU/g equal to 2.28×107 ± 4.070×107 in the ALGAE group versus 1.75×107 ± 3.102×107 CFU/g in the CTRL group. At the final time (T4), it is possible to observe that the coliform bacteria count of the ALGAE group is lower, although not significantly (p > 0.05), compared with the count at T2 (3.28×103 ± 9.936×103 CFU/g vs. 1.19×104 ± 2.274×104 CFU/g, respectively).
Figure 7. Microbiological analysis of feces. (A) Microbiological evaluation of total bacteria on PCA medium. Time: p = 0.9419; Treatment: p = 0.5631; Time x Treatment: p = 0.0697; (B) Microbiological evaluation of lactic acid bacteria on MRS medium. Time: p = 0.0838; Treatment: p = 0.8488; Time x Treatment: p = 0.8823; (C) Microbiological evaluation of coliform bacteria on VRBL medium. Time: p = 0.0432; Treatment: p = 0.0769; Time x Treatment: p = 0.5892. The data are shown as the means ± standard error (SEM).
The calculation of protein intake revealed that during the first period of the trial, the protein intake did not differ significantly between the two experimental groups (CTRL: 85.93 ± 14.123 g/day; ALGAE: 77.50 ± 14.470 g/day; p > 0.05). On the other hand during the second period of the trial the protein intake of the ALGAE group (127.08 ± 19.491 g/day) was significantly higher than the CTRL group (102.81 ± 21.147 g/day) (p = 0.008). The nitrogen content of fecal sample revealed no significant differences between the experimental group compared to the CTRL group (5.20 ± 1.201% for CTRL and 3.35 ± 0.212% for ALGAE group, on dry matter basis) at T4. These results were also reflected in the evaluation of the apparent protein digestibility that, as well as, revealed no statistical difference between the CTRL and the ALGAE groups at T4 (87.89 ± 3.110% and 88.01 ± 1.745% respectively).
The oxidative status of the serum barrier was evaluated as the ability of the latter to oppose the oxidizing action of a hypochlorous acid (HClO) solution using the OXY adsorbent test. The results obtained showed that the addition of A. nodosum and P. calcareum within the diet fed to post-weaning pigs is able to bring about an improvement in serum barrier antioxidant activity. In fact, comparing the CTRL group with the ALGAE group, it can be observed that at both T2 and T4 the serum barrier antioxidant activity values of the ALGAE group are 396.83 ± 10.125 and 347.35 ± 2.892 μmol of HClO/mL, respectively, resulting significantly higher (p < 0.010) than those obtained at the same time points in the CTRL group (253.10 ± 31.364 and 270.61 ± 27.439 μmol of HClO/mL, respectively) (Figure 8).
Figure 8. Serum values of Oxy Adsorbent test measured at T0, T2, and T4 of trial in CTRL and ALGAE groups. The data are shown as the means ± standard error (SEM). Asterisks with different superscripts are significantly different; ****p < 0.0001, **p < 0.001. Time: p < 0.001; Treatment: p = 0.003; Time x Treatment: p < 0.001.
In recent years, there has been a growing interest in finding functional and sustainable ingredients that can be used in animal nutrition to promote health, reduce the use of antibiotics, and ensure the profitability of farms (46). This aspect is emphasized in pig farming, where numerous multifactorial pathologies occur during weaning, and nutrition plays a central role (47). On this aspect, the combination of multiple functional ingredients that can increase their individual spectrum of action has emerged as an interesting approach. The broad spectrum can indeed be related not only to synergistic effects but also to the different mechanisms of action targeting various pathways. By combining functional ingredients with diverse modes of action, such as immune modulation, gut health promotion, antioxidant activity, and anti-inflammatory effects, the overall impact on animal health and performance can be amplified. This comprehensive approach addresses multiple aspects of animal physiology and metabolism, resulting in a more holistic and effective nutritional strategy (48, 49).
With these assumptions, our study is built upon the promising findings reported by Frazzini et al. (22), where the combination of A. nodosum and P. calcareum demonstrated synergistic activity in inhibiting pathogenic bacterial strain of E. coli and antioxidant properties in vitro. Particularly, after testing the conservation of functional properties following the simulated in vitro digestive process, we aimed to test the combination of algae in vivo to gain a comprehensive understanding of the biological effects of functional ingredients in animals and to inform evidence-based practices in animal nutrition and health management.
The chemical composition of algae should be taken into account to maintain the nutrient balance of the experimental diet. Despite their low percentage of inclusion, algae may not significantly influence the total percentage of macronutrients in the diet. However, their influence on micronutrients can be crucial.
The chemical evaluation of A. nodosum and P. calcareum showed results in line with the scientific literature once again highlighting the high mineral content present in these algal species (50). The high calcium (Ca) content of P. calcareum (51) requires careful evaluation at the time of inclusion in the diet. In fact, when formulating diets, in addition to the most important nutritional components, specific ratios must also be taken into account, including the calcium-phosphorus ratio. If this ratio is not maintained correctly, a series of disturbances are created at the metabolic pathway level that have important repercussions on animal health (52, 53). In fact, as reported by Sun M. et al. (54), imbalances in the metabolism of calcium and phosphorus can lead to skeletal and cardiovascular diseases. A. nodosum also displayed a high mineral content. Still, the attention paid to this alga at a nutritional level is due to its protein composition, which is mostly of high nutritional value (55). Although the total mineral content of both algae is high the analyses conducted in ICP-MS ensured that those elements considered undesirable in animal nutrition such as arsenic (As), cadmium (Cd), lead (Pb), cobalt (Co), and molybdenum (Mo) did not exceed the threshold levels for feed established by regulation suggestion the lack of toxic risk for feed formulation considering low percentages of seaweeds inclusion. Likewise, Iron (Fe), manganese (Mn), and selenium (Se) did not exceed the threshold limits in swine nutrition (56, 57). Additionally, great importance has been placed on dietary zinc levels. Zinc (Zn) is indeed an essential micronutrient required for proper growth and development of the animal as it is involved in several structural and biological functions, such as enzymatic reactions, DNA and RNA metabolism, protein synthesis, gene expression, cell proliferation and differentiation and cell-mediated immunity (58, 59). From June 2022, the maximal allowed dietary Zn level for weaned piglets in the EU is 150 mg/kg diet (60, 61). Analyses have shown that the algae used have low levels of zinc, which would therefore not unduly influence the legal limit for a complete feed, particularly when they are included in the feed as functional ingredients and therefore with an inclusion rate of no more than 2%.
The undeniable nutritional characteristics of algae are also associated with good functional properties due to the presence of various bioactive compounds (22, 62–64). A. nodosum and P. calcareum are both marine algae rich in bioactive compounds, including flavonoids and polyphenols (65). This molecule class is a group of plant pigments that have been shown to have a variety of health benefits, including antioxidant and anti-inflammatory properties, protecting against cell damage, and improving blood vessels’ health (66, 67).
A. nodosum stands out in the algae kingdom due to its impressive content of total polyphenols and flavonoids (68). While total phenols comprise a broader range of antioxidant compounds, flavonoids represent a specific subclass known for their health benefits. The presence of different bioactive compounds and the molecular interaction among them is particularly interesting since the high total phenolic content, attributable to the content of phenols, including phlorotannins, and the presence of laminarin and fucoidan (69, 70) suggests a strong antioxidant capacity, potentially reducing free radical damage and inflammation (71, 72). Moreover, the functional components of brown algae, such as phlorotannins, which are also known to have bacteriostatic and bactericidal activity, can contribute to the antimicrobial properties highlighted in the study (73, 74).
On the other hand, being P. calcareum mainly recognized for its mineral component, particularly calcium, magnesium, and carbonate, research has currently focused on these minerals and their potential benefits and they are lacking in investigating the bioactive properties of this algae (75, 76). Analyses conducted in this study on the matrix of P. calcareum showed a, albeit minimal, polyphenols and flavonoids content. The presence of different bioactive molecule could explain the synergistic effect revealed by the analysis of the functional properties of the combination of the two algal extracts as demonstrated in a previous work (22).
In fact, has been demonstrated that the combination of different antioxidant sources could enhance their effect on radical scavenging activity (71, 72, 77). Moreover, P. calcareum extract was found to be able to reduce the growth of F4+ E. coli, thus probably due to the ability of red algae to produce antimicrobial metabolites, such as diterpenoids (78), monoterpenes (79), phenolic compounds (80), sterols (81), polysaccharides (82), and fatty acids (83).
In vitro digestion models mimic the conditions of the gastrointestinal tract, including the stomach and intestine, by allowing the theoretical determination of the digestibility of a given ingredient. Thus, helping to evaluate the inclusion of specific ingredients within the diet. Algae, as reported in the literature, are not always extremely digestible; this depends on the species to which they belong. Our results were in line with this statement. In fact, our findings revealed that A. nodosum had a low digestibility, probably due to the high phlorotannin content (84, 85), which, as reported by Ford et al. (86), significantly affects the digestibility of this seaweed. Therefore, the inclusion of poorly digestible foods, as in the case of A. nodosum must be carefully considered when formulating the diet where it is advisable to include such ingredients in not too high percentages that consequently do not affect digestibility. In the case of high inclusions of ingredients rich in phlorotannin, such as A. nodosum it is also possible to introduce within the feed additives such as polyethylene glycol and polyvinylpyrrolidone that make the phlorotannin themselves more digestible (87). On the other hand, P. calcareum revealed a higher digestibility, probably due to its mineral composition, which showed a higher solubility of this alga than other limestone sources, suggesting better digestibility and also higher bioavailability of calcium when used in animal diets (88). However, its digestibility, such as for A. nodosum is influenced by other factors such as the presence of phenolic compounds, which can inversely affect protein digestibility, and the structural characteristics of seaweeds, play a significant role in their overall nutritional quality (89). The in vitro digestion models allowing also to simulate how functional ingredients maintain their activities after digestion. This is essential for understanding how functional ingredients behave within the gastrointestinal tract and predicting their physiological effects, thereby informing the development of effective and bioavailable products for promoting health and welfare.
The interest in bioactive molecules from algae has surged in recent years due to their potential health benefits and applications in various industries. Algae, both macroalgae and microalgae, are rich in diverse bioactive compounds that exhibit a wide range of biological activities, including antioxidant, anticancer, anti-inflammatory, and immune-modulating effects (90, 91). Therefore, it is also important to focus attention on the knowledge of bioactive compounds bioavailability, and their modification after the digestion process. The results obtained disclose that the total phenolic content and total flavonoid content of A. nodosum and their combination showed a gradual and significant increase during in vitro digestion. This is due to the fact that, initially, during the oral phase, only simple phenolics, such as phlorotannin, gallic acid, protocatechuic acid, and 4-hydroxybenzoic acid, are released due to the short digestion time (92). Once in the gastric phase, the decrease of pH and the presence of pepsin enzyme allowed the degradation, oxidation, and polymerization of a higher amount of phenolic compounds (93). Finally, when, in the intestinal phase, the samples come into contact with an alkaline environment and with the presence of pancreatic enzymes, a complete release of the polyphenolic component occurs. This is due to the fact that a neutral pH allows the release of those polyphenolic molecules that are instable in an acidic environment, and it is also reported that the presence of pancreatin affects the binding of the polyphenolic molecules with the other molecules in the food matrix, resulting in the release of this molecular class (94). Moreover, in addition to the different environments of the three digestive phases, the polyphenol content released, as reported by Wang and colleagues (95), is also related to chemical compositions, dietary fiber, hydrophobic interactions, hydrogen bonding, and covalent bonds of the digestive matrix. At the same time as an increase in bioactive components was observed during the different digestion steps, the relative antioxidant activity also showed a significant increase (p < 0.05) as digestion progressed. This behavior could be due, as well as for the bioactive molecules, to pH variation during in vitro digestion. In fact, the transition from the acid medium to the alkaline favors the release of phenols and flavonoids which are correlated to the antioxidant activity (96, 97). Additionally, the antioxidant capacity is further linked to the interplay of phenolic compounds with other substances, such as minerals or dietary fiber, released during the digestion process. These substances in which algae are rich can influence solubility and the availability of phenols. In any case, in the interpretation of these results, we have to take into account that during the digestion process other non-antioxidant food components, such as amino acids, sugars, and uronic acids, can be released and these might interfere with the Trolox equivalent antioxidant capacity assays increasing the values of antioxidant activity observed (98). Finally, as reported by Chen et al. (99), polyphenols, thanks to their molecular structure, are able to develop antimicrobial activity. Gram-negative bacteria, such as E. coli, are more resistant to the antibacterial activity of phenolic compounds thanks to the high levels of phospholipids on the lipophilic outer membrane (100). Therefore, the antibacterial mechanism operates through the buildup of hydroxylic groups within lipid bilayers, disrupting the interaction of lipoproteins and enhancing the permeability of the cell membrane (101). In any case, these findings need cautious interpretation because is important to note that polyphenols and other antioxidant and antimicrobial compounds may undergo metabolism by gut microbiota (102). Therefore, the results obtainable through the in vitro digestion process may not fully reflect what happens in a living organism’s intestinal system.
In pig farming, the weight that the animal reaches during the weaning phase is fundamental, as it has a knock-on effect on the entire production cycle of pigs. In fact, higher weights at weaning will lead to an earlier attainment of slaughter weight, reflecting positively on the income of the farmer (103). A higher weight also translates into better intestinal health, consequently improving the performance of the animal (104). The study carried out showed that the inclusion of A. nodosum and P. calcareum in the diet of post-weaning pigs did not have a depressive effect on the growth of the animals, maintaining the growth curve unchanged, and consequently also the average daily weight gain, between the CTRL group and the ALGAE group. Although there are currently no studies in the literature evaluating the inclusion of the chosen algal combination in the diet of post-weaning piglets, the data obtained are in line with other studies considering the inclusion of individual algal species. Michiels et al. (105) following the administration of three different amounts (2.5, 5.0 or 10.0 g of dried seaweed per kg) of A. nodosum in 21-day-old piglets observed no significant differences between the growth curves of the control and treatment groups. On the other hand, the administration of 3 g/kg; 6 g/kg; 9 g/kg of A. nodosum in post weaning piglets for 28 days disclosed that daily gain, and carcass weight were reduced in a linear manner with the inclusion of increasing levels of the brown seaweed (106). Even at higher doses of inclusions of A. nodosum (20 g seaweed kg-1 of feed) no significant increases in weight of the animals in the treatment group were found (107). Furthermore, the data obtained suggest that in the first part of the trial, feed consumption did not vary between the two groups, emphasizing that the introduction of the algae did not impact the palatability of the feed. This is of great importance, because food intake is crucial both for ensuring body growth but also for ensuring the integrity of the intestinal mucosa. In fact, is reported that maintenance of nutrition after weaning would prevent the normal decline in villous height and increase in crypt depth and hence preserve the structure and function of the small intestine, consequently preserving intestinal health (108, 109). Nevertheless, as the animals increased in age, and particularly from the 49th day of age, an increase in food consumption was observed, the introduction of the algae may possibly have had a positive effect and potentially a difference in weight could be observed over longer experimental periods (110). Finally, a significant difference in the FCR value could be observed during the test period. This may suggest that the inclusion of a small amount of algae may have had a negative impact on feed efficiency as the animals had to adapt to a new diet with a different fibrous composition. In fact, many studies report that the ‘recalcitrant’ cell wall of algae makes the digestion of some of their compounds more difficult, often suggesting treatments before feeding them to monogastric (111–113). Nevertheless, the animals reached a similar weight to the CTRL group while maintaining a growth rate that did not differ significantly.
If, on the one hand, the inclusion of algae in the diet did not influence the zootechnical performance, as well as the fecal score and consequently the diarrhea frequency that was unvaried between the two experimental groups. On the other hand, it revealed potential beneficial effects on intestinal bacterial populations. At T2, a reduction in coliform bacteria was observed in both groups. This shows that, in the time between T0 and T2, the experimental animals, regardless of the diet they received, achieved physiological well-being, which allowed them to be less susceptible to coliform infections, which are known to be the most common in stressful phases of pigs (8). As for lactic acid bacteria, at the same time point, it is possible to find that the administration of feed supplemented with algae has allowed an increase in this type of bacteria, which is known to be beneficial for the intestinal health of pigs (114). In fact, several studies report that these microorganisms help with the digestion of nutrients, provide mucosal protection and fight other pathogenic bacteria, including coliforms (115). Moreover, the greater the amount of beneficial bacteria in piglets microbiota, the lower the chance that they will develop diarrhea, given that the concentration of lactobacilli is directly proportional to good intestinal health (116). Huang et al. (117) reported that lactobacilli improved microbial balance in the digestive tract of piglets during the first 2 weeks after weaning and resulted in fewer cases of diarrhea and an increase in growth. Supporting the beneficial effect of algae feeding in the post-weaning piglet diet different studies report that the compounds present in the algae, particularly in brown seaweeds stimulated the growth of Lactobacilli (118, 119), and reduced the enterobacteria population or Escherichia coli (120, 121). Dierick et al. (107) revealed that the administration of A. nodosum (10 g kg − 1) in weaned piglets had a reducing effect on the E. coli load in the stomach and small intestine, while the lactobacilli/E. coli ratio was enhanced in the small intestine, indicating a beneficial shift in the microbial population. Other studies report also that the administration of extracts of bioactive components of algae, such as laminarin and fucoidan, lead to an increase in Lactobacillus spp. and a reduction of E. coli in swine feces (122–124). In conclusion, our data showed the positive potential of algae, but an evaluation of the entire microbiota is probably necessary in order to assess the overall effect of this innovative combination of algae in weaned piglets.
Our results regarding protein intake are in line with what was shown in the evaluation of feed intake, which was found to be higher in the ALGAE group during the second period of the trial. Despite this, the analysis of the fecal composition disclosed that the supplementation of algae within the diet did not affect the digestibility of the protein component as well as the nitrogen utilization. This is probably due to the inclusion of seaweed, which rich in polyphenols reduces protein digestibility (125, 126). However, the present study proved that using low levels of seaweed inclusion in the diet did not negatively affect the protein digestibility of the diet in post-weaning pigs. Although feces-based apparent digestibility analysis is an important parameter for estimating nutrient utilization efficiency using a minimally invasive method, few studies to date report the impact of algae on diet digestibility. However, our results are in line with those of Czech and colleagues who did not register significant differences in crude protein digestibility, supplementing 0.6 and 1% of A. nodosum from 18 to 64 days of age in weaned piglets (127). As well as another study focused on the supplementation of 0.05, 0.1, and 0.15% of Ecklonia cava in postweaning pigs showed no significant differences in the crude protein digestibility at 14 and 28 days after weaning (120). Although it is known that the inclusion of seaweed in the diet can slow down the digestion and absorption of nutrients (127), our results can be explained by the presence in seaweed of several antioxidant compounds that could protect the digestive tract from oxidative damage, improving intestinal health and digestive processes (128).
Many factors, such as physiological, environmental, and dietary, can induce the host to produce a large number of free radicals, which can cause oxidative damage in pigs that is often accompanied by other pathological factors, which have a direct negative impact on pig performance and healthy growth.
Nowadays, the higher pressure due to maximizing production in pig farming is one of the causes of the phenomenon of oxidative stress, a condition harmful to pig health and leading to higher feed costs. Therefore, developing effective methods to reduce oxidative stress is becoming essential. Since, under oxidative stress, body nutrient metabolism direction changes, current research efforts are primarily focused on alleviating oxidative stress in pigs by supplementing diets with additional supplementation of different nutrients or non-nutritional additives (129–131). Our study highlighted that the inclusion of A. nodosum and P. calcareum in the diet administered to post-weaning pigs is also able to improve the antioxidant activity of the serum barrier thereby protecting cells from damage caused by free radicals, probably thanks to the bioactive compounds with antioxidant capacity present in the algae considered. This result is supported by several studies in the literature where polyphenols were found to be attractive feed additives for nutritional management in pig production with the aim of mitigating oxidative stress in animals (132–134). Additionally, the study carried out by Czech and colleagues (127) highlights that the supplementation of A. nodosum can bring improvements in the oxidative state, since evidence were found in the reduction in lipid peroxidation markers and increased activity of redox enzymes in various tissues.
The present work first investigated the chemical and functional properties of two algal species A. nodosum and P. calcareum. Our study disclosed that the algae considered possess strong antioxidant and antimicrobial capabilities and that these improve once the algae are put in combination suggesting a possible synergistic effect given by the interaction of the biomolecules present. Moreover, it is also highlighted that the functional properties of A. nodosum and P. calcareum were maintained during the digestion process, further reinforcing the beneficial effects of introducing this ingredient within the diet. Therefore, was decided to evaluate the inclusion of the combination of the two algal species in the diet of post-weaning piglets. This part of the study showed that A. nodosum and P. calcareum within the feed did not alter zootechnical performance and increades the feed intake during the last week of trial. However, their inclusion highlighted the bioactive properties of these algal species. The group that consumed algae in their feed showed a stronger serum antioxidant barrier. Moreover, including algae led to a slight reduction in the presence of coliform bacteria. The results obtained in this study, although preliminary, also confirmed the bioactive potential of the algal species considered in vivo. Therefore, this study may represent a good starting point to investigate the bioactive properties of algae further in order to include them in animal nutrition to reduce the use of antibiotics by preventing the colonization of pathogenic bacterial strains during the most critical stages of pig farming.
The original contributions presented in the study are included in the article/Supplementary material, further inquiries can be directed to the corresponding author.
The animal study was approved by Animal Welfare Organization of University of Milan and the Italian Ministry of Health (n° 884/2021-PR). The study was conducted in accordance with the local legislation and institutional requirements.
SF: Conceptualization, Data curation, Formal analysis, Investigation, Methodology, Software, Visualization, Writing – original draft, Writing – review & editing. SR: Investigation, Methodology, Writing – review & editing. MD: Conceptualization, Data curation, Formal analysis, Investigation, Methodology, Software, Writing – review & editing. AF: Investigation, Methodology, Writing – review & editing. ES: Investigation, Writing – review & editing. IF: Investigation, Writing – review & editing. LR: Conceptualization, Funding acquisition, Project administration, Resources, Supervision, Validation, Visualization, Writing – review & editing.
The author(s) declare that financial support was received for the research, authorship, and/or publication of this article. This research was funded by the University of Milan under the grant Seal of Excellence (SoE) -SEED 2020: “Algae-based alternatives to in-feed antibiotics in pig production -ASAP Algae-baSed Pig” project. Founding number 35409.
The authors would like to thank Ferraroni S.p.A. for providing the feed, and in particular Giancarlo Selmini for his contribution in the diet formulation. The authors would like to thank Dr. Pilu for his contribution in the work done concerning the analysis of micro- and macro- elements in algae.
AF was employed by Biotecnologie B.T. Srl. No commercial manufacturing products of Biotecnologie B.T. Srl. were used in this study.
The remaining authors declare that the research was conducted in the absence of any commercial or financial relationships that could be construed as a potential conflict of interest.
All claims expressed in this article are solely those of the authors and do not necessarily represent those of their affiliated organizations, or those of the publisher, the editors and the reviewers. Any product that may be evaluated in this article, or claim that may be made by its manufacturer, is not guaranteed or endorsed by the publisher.
The Supplementary material for this article can be found online at: https://www.frontiersin.org/articles/10.3389/fvets.2024.1431091/full#supplementary-material
1. FAO. World Livestock: Transforming the livestock sector through the sustainable development goals. Rome: FAO (2019).
2. Gargano, G, Licciardo, F, Verrascina, M, and Zanetti, B. The Agroecological approach as a model for multifunctional agriculture and farming towards the European green Deal 2030—some evidence from the Italian experience. Sustain For. (2021) 13:2215. doi: 10.3390/su13042215
3. Hejna, M, Gottardo, D, Baldi, A, Dell’Orto, V, Cheli, F, Zaninelli, M, et al. Review: nutritional ecology of heavy metals. Animal. (2018) 12:2156–70. doi: 10.1017/S175173111700355X
4. Hejna, M, Moscatelli, A, Onelli, E, Baldi, A, Pilu, S, and Rossi, L. Evaluation of concentration of heavy metals in animal rearing system. Ital J Anim Sci. (2019) 18:1372–84. doi: 10.1080/1828051X.2019.1642806
5. Murphy, D, Ricci, A, Auce, Z, Beechinor, JG, Bergendahl, H, Breathnach, R, et al. EMA and EFSA joint scientific opinion on measures to reduce the need to use antimicrobial agents in animal husbandry in the European Union, and the resulting impacts on food safety (RONAFA). EFSA J. (2017) 15:e04666. doi: 10.2903/j.efsa.2017.4666
6. European Commission. Regulation (EU) 2019/6 of the European Parliament and of the council of 11 December 2018 on veterinary medicinal products and repealing directive 2001/82/EC. Brussels: European Commission (2019).
7. Pandey, AK, Kumar, P, and Saxena, MJ. Feed Additives in Animal health In: Nutraceuticals in veterinary medicine. Cham: Springer International Publishing (2019). 345–62.
8. Rossi, L, Dell’Orto, V, Vagni, S, Sala, V, Reggi, S, and Baldi, A. Protective effect of oral administration of transgenic tobacco seeds against verocytotoxic Escherichia coli strain in piglets. Vet Res Commun. (2014) 38:39–49. doi: 10.1007/s11259-013-9583-9
9. Rymut, HE, Rund, LA, Bolt, CR, Villamil, MB, Southey, BR, Johnson, RW, et al. The combined effect of weaning stress and immune activation during pig gestation on serum cytokine and Analyte concentrations. Animals. (2021) 11:2274. doi: 10.3390/ani11082274
10. Sotira, S, Dell’Anno, M, Caprarulo, V, Hejna, M, Pirrone, F, Callegari, ML, et al. Effects of Tributyrin supplementation on growth performance, insulin, blood metabolites and gut microbiota in weaned piglets. Animals. (2020) 10:726. doi: 10.3390/ani10040726
11. Amezcua, R, Friendship, RM, Dewey, CE, Gyles, C, and Fairbrother, JM. Presentation of postweaning Escherichia coli diarrhea in southern Ontario, prevalence of hemolytic E. coli serogroups involved, and their antimicrobial resistance patterns. Can J Vet Res. (2002) 66:73–8.
12. Verdonck, F, Cox, E, van Gog, K, van der Stede, Y, Duchateau, L, Deprez, P, et al. Different kinetic of antibody responses following infection of newly weaned pigs with an F4 enterotoxigenic Escherichia coli strain or an F18 verotoxigenic Escherichia coli strain. Vaccine. (2002) 20:2995–3004. doi: 10.1016/S0264-410X(02)00220-7
13. Windisch, W, Schedle, K, Plitzner, C, and Kroismayr, A. Use of phytogenic products as feed additives for swine and poultry1. J Anim Sci. (2008) 86:E140–8. doi: 10.2527/jas.2007-0459
14. Hejna, M, Onelli, E, Moscatelli, A, Bellotto, M, Cristiani, C, Stroppa, N, et al. Heavy-metal phytoremediation from livestock wastewater and exploitation of exhausted biomass. Int J Environ Res Public Health. (2021) 18:2239. doi: 10.3390/ijerph18052239
15. EU Commission. Regulation EC 1831/2003. Of the European Parliament and of the council, of 22 September 2003 on Additives for use in Animal nutrition (text with EEA relevance). Brussels: EU Commission (2003).
16. Gupta, S, and Abu-Ghannam, N. Recent developments in the application of seaweeds or seaweed extracts as a means for enhancing the safety and quality attributes of foods. Innovative Food Sci Emerg Technol. (2011) 12:600–9. doi: 10.1016/j.ifset.2011.07.004
17. De Clerck, O, Bogaert, KA, and Leliaert, F. Diversity and evolution of algae. Adv Botan Res. (2012) 64:55–86. doi: 10.1016/B978-0-12-391499-6.00002-5
18. Guiry, MD. How many species of algae are there? J Phycol. (2012) 48:1057–63. doi: 10.1111/j.1529-8817.2012.01222.x
19. Pulz, O, and Gross, W. Valuable products from biotechnology of microalgae. Appl Microbiol Biotechnol. (2004) 65:635–48. doi: 10.1007/s00253-004-1647-x
20. Spolaore, P, Joannis-Cassan, C, Duran, E, and Isambert, A. Commercial applications of microalgae. J Biosci Bioeng. (2006) 101:87–96. doi: 10.1263/jbb.101.87
21. Pereira, L, Morrison, L, Shukla, PS, and Critchley, AT. A concise review of the brown macroalga Ascophyllum nodosum (Linnaeus) Le Jolis. J Appl Phycol. (2020) 32:3561–84. doi: 10.1007/s10811-020-02246-6
22. Frazzini, S, Scaglia, E, Dell’Anno, M, Reggi, S, Panseri, S, Giromini, C, et al. Antioxidant and antimicrobial activity of algal and cyanobacterial extracts: an in vitro study. Antioxidants. (2022) 11:992. doi: 10.3390/antiox11050992
23. AOAC. Official methods of analysis. 16th ed. Washington, DC: Association of Official Analytical Chemists (2019). 64 p.
24. AOCS. Approved procedure Ba 6a-05: Crude fiber analysis in feeds by filter bag technique. In official methods and recommended practices. 4th ed. Champaign, IL: American Oil Chemists’ Society (2009).
25. Gouvinhas, I, Santos, RA, Queiroz, M, Leal, C, Saavedra, MJ, Domínguez-Perles, R, et al. Monitoring the antioxidant and antimicrobial power of grape (Vitis vinifera L.) stems phenolics over long-term storage. Ind Crop Prod. (2018) 126:83–91. doi: 10.1016/j.indcrop.2018.10.006
26. Attard, E. A rapid microtitre plate Folin-Ciocalteu method for the assessment of polyphenols. Open Life Sci. (2013) 8:48–53. doi: 10.2478/s11535-012-0107-3
27. Herald, TJ, Gadgil, P, and Tilley, M. High-throughput micro plate assays for screening flavonoid content and DPPH-scavenging activity in sorghum bran and flour. J Sci Food Agric. (2012) 92:2326–31. doi: 10.1002/jsfa.5633
28. Frazzini, S, Zuorro, A, Panseri, S, Pavlovic, R, Sgoifo Rossi, CA, and Rossi, L. Repurposing hazelnut waste Products for a sustainable economy: a Metabolomic analysis of cuticles and shells to highlight their antioxidant potential and inhibitory activity against Verocytotoxic Escherichia coli. Sustain For. (2023) 15:3268. doi: 10.3390/su15043268
29. Myers, JA, Curtis, BS, and Curtis, WR. Improving accuracy of cell and chromophore concentration measurements using optical density. BMC Biophys. (2013) 6:4. doi: 10.1186/2046-1682-6-4
30. Brodkorb, A, Egger, L, Alminger, M, Alvito, P, Assunção, R, Ballance, S, et al. INFOGEST static in vitro simulation of gastrointestinal food digestion. Nat Protoc. (2019) 14:991–1014. doi: 10.1038/s41596-018-0119-1
31. Frazzini, S, Torresani, MC, Roda, G, Dell’Anno, M, Ruffo, G, and Rossi, L. Chemical and functional characterization of the main bioactive molecules contained in hulled Cannabis sativa L. seeds for use as functional ingredients. J Agric Food Res. (2024) 16:101084:101084. doi: 10.1016/j.jafr.2024.101084
32. Hsu, CF, Peng, H, Basle, C, Travas-Sejdic, J, and Kilmartin, PA. ABTS•+scavenging activity of polypyrrole, polyaniline and poly(3,4‐ethylenedioxythiophene). Polym Int. (2011) 60:69–77. doi: 10.1002/pi.2912
33. European Commission. Amending annex I to directive 2002/32/EC of the European Parliament and of the council as regards mercury, free gossypol, nitrites and Mowrah, Bassia, Madhuca; commission directive EU 6/2010. Brussels: European Commission (2010).
34. Dell’Anno, M, Frazzini, S, Ferri, I, Tuberti, S, Bonaldo, E, Botti, B, et al. Effect of dietary supplementation of chestnut and Quebracho tannin supplementation on neonatal Diarrhoea in Preweaning calves. Antioxidants. (2024) 13:237. doi: 10.3390/antiox13020237
35. Rossi, L, Dell’Anno, M, Turin, L, Reggi, S, Lombardi, A, Alborali, GL, et al. Tobacco seed-based Oral vaccination against Verocytotoxic O138 Escherichia coli as alternative approach to antibiotics in weaned piglets. Antibiotics. (2023) 12:715. doi: 10.3390/antibiotics12040715
36. Scaglia, E, Reggi, S, Canala, B, Frazzini, S, Dell’Anno, M, Hejna, M, et al. The effects of Milk replacer supplemented with Ascophyllum nodosum as a novel ingredient to prevent neonatal diarrhea in dairy calves and improve their health status. Vet Sci. (2023) 10:618. doi: 10.3390/vetsci10100618
37. Augenstein, M, Johnston, L, Shurson, G, Hawton, J, and Pettigrew, J. (2024) Formulating farm-specific swine diets. Available online at: https://extension.umn.edu/swine-nutrition/formulating-farm-specific-swine-diets#sources-1671910 (Accessed November 10, 2024)
38. Krul, ES. Calculation of nitrogen-to-protein conversion factors: a review with a focus on soy protein. J Am Oil Chem Soc. (2019) 96:339–64. doi: 10.1002/aocs.12196
39. Czech, A, Grela, ER, and Kiesz, M. Dietary fermented rapeseed or/and soybean meal additives on performance and intestinal health of piglets. Sci Rep. (2021) 11:16952. doi: 10.1038/s41598-021-96117-w
40. Erdoğan, S. Determination of sample size using resource equation methods in analysis of variance models in Animal studies. Dicle Üniversitesi Veteriner Fakültesi Dergisi. (2024) 17:1–7. doi: 10.47027/duvetfd.1308466
41. Reilly, P, O’Doherty, JV, Pierce, KM, Callan, JJ, O’Sullivan, JT, and Sweeney, T. The effects of seaweed extract inclusion on gut morphology, selected intestinal microbiota, nutrient digestibility, volatile fatty acid concentrations and the immune status of the weaned pig. Animal. (2008) 2:1465–73. doi: 10.1017/S1751731108002711
42. Furbeyre, H, van Milgen, J, Mener, T, Gloaguen, M, and Labussière, E. Effects of dietary supplementation with freshwater microalgae on growth performance, nutrient digestibility and gut health in weaned piglets. Animal. (2017) 11:183–92. doi: 10.1017/S1751731116001543
43. Rossi, L, Turin, L, Alborali, GL, Demartini, E, Filipe, JFS, Riva, F, et al. Translational approach to induce and evaluate Verocytotoxic E. coli O138 based disease in piglets. Animals. (2021) 11:2415. doi: 10.3390/ani11082415
44. Reggi, S, Giromini, C, Dell’Anno, M, Baldi, A, Rebucci, R, and Rossi, L. In vitro digestion of chestnut and Quebracho tannin extracts: antimicrobial effect, antioxidant capacity and Cytomodulatory activity in swine intestinal IPEC-J2 cells. Animals. (2020) 10:195. doi: 10.3390/ani10020195
45. National Research Council. Nutrient requirements of swine. Ottawa, ON: National Research Council (2012).
46. Oyebade Ikusika, O, Haruzivi, C, and Conference Mpendulo, T. Alternatives to the use of antibiotics in Animal production. Anim Sci. (2023) 13:105922. doi: 10.5772/intechopen.105922
47. René, R, Sebastian, V, Marlies, D, Lukas, S, Annemarie, K, and Andrea, L. Risk factors associated with post-weaning diarrhoea in Austrian piglet-producing farms. Porcine Health Manag. (2023) 9:20. doi: 10.1186/s40813-023-00315-z
48. Gong, Y, Wang, H, and Sun, J. AMP-mimetic antimicrobial polymer-involved synergic therapy with various Coagents for improved efficiency. Biomacromolecules. (2024) 25:4619–38. doi: 10.1021/acs.biomac.3c01458
49. Jacquier, EF, van de Wouw, M, Nekrasov, E, Contractor, N, Kassis, A, and Marcu, D. Local and systemic effects of bioactive food ingredients: is there a role for functional foods to prime the gut for resilience? Food Secur. (2024) 13:739. doi: 10.3390/foods13050739
50. Makkar, HPS, Tran, G, Heuzé, V, Giger-Reverdin, S, Lessire, M, Lebas, F, et al. Seaweeds for livestock diets: a review. Anim Feed Sci Technol. (2016) 212:1–17. doi: 10.1016/j.anifeedsci.2015.09.018
51. Aslam, MN, Kreider, JM, Paruchuri, T, Bhagavathula, N, DaSilva, M, Zernicke, RF, et al. A mineral-rich extract from the red marine algae Lithothamnion calcareum preserves bone structure and function in female mice on a Western-style diet. Calcif Tissue Int. (2010) 86:313–24. doi: 10.1007/s00223-010-9340-9
52. Merriman, LA, Walk, CL, Murphy, MR, Parsons, CM, and Stein, HH. Inclusion of excess dietary calcium in diets for 100- to 130-kg growing pigs reduces feed intake and daily gain if dietary phosphorus is at or below the requirement1. J Anim Sci. (2017) 95:5439–46. doi: 10.2527/jas2017.1995
53. Lautrou, M, Narcy, A, Dourmad, J-Y, Pomar, C, Schmidely, P, and Létourneau Montminy, M-P. Dietary phosphorus and calcium utilization in growing pigs: requirements and improvements. Front Vet Sci. (2021) 8:734365. doi: 10.3389/fvets.2021.734365
54. Sun, M, Wu, X, Yu, Y, Wang, L, Xie, D, Zhang, Z, et al. Disorders of calcium and phosphorus metabolism and the proteomics/metabolomics-based research. Front Cell Dev Biol. (2020) 8:576110. doi: 10.3389/fcell.2020.576110
55. Thiviya, P, Gamage, A, Gama-Arachchige, NS, Merah, O, and Madhujith, T. Seaweeds as a source of functional proteins. Phycology. (2022) 2:216–43. doi: 10.3390/phycology2020012
56. Hrólfsdóttir, AÞ, Arason, S, Sveinsdóttir, HI, and Gudjónsdóttir, M. Added value of Ascophyllum nodosum Side stream utilization during seaweed meal processing. Mar Drugs. (2022) 20:340. doi: 10.3390/md20060340
57. Sampath, V, Sureshkumar, S, Seok, WJ, and Kim, IH. Role and functions of micro and macro-minerals in swine nutrition: a short review. J Anim Sci Technol. (2023) 65:479–89. doi: 10.5187/jast.2023.e9
58. Kambe, T, Weaver, BP, and Andrews, GK. The genetics of essential metal homeostasis during development. Genesis. (2008) 46:214–28. doi: 10.1002/dvg.20382
59. Salgueiro, MJ, Zubillaga, M, Lysionek, A, Sarabia, MI, Caro, R, De Paoli, T, et al. Zinc as an essential micronutrient: a review. Nutr Res. (2000) 20:737–55. doi: 10.1016/S0271-5317(00)00163-9
60. European Commission. Commission implementing regulation (EU) 2016/1095 of July 2016 concerning the authorisation of zinc acetate Dihydrate, Zinc Chloride Anhydrous, Zinc Oxide, Zinc Sulphate Heptahydrate, Zinc Sulphate Monohydrate, Zinc Chelate of Amino Acid Hydrate, Zinc Che. Brussels: European Union (2016).
61. European Commission. (2017). Implementing decision of 26.6.2017 concerning, in the framework of article 35 of directive 2001/82/EC of the European Parliament and of the council, the marketing Authorisations for veterinary medicinal Products containing “zinc oxide” to be administered orally to food producing species. Official journal of the European Union. Available online at: https://ec.europa.eu/health/documents/community-register/2017/20170626136754/dec_136754_en.pdf (Accessed May 10, 2024)
62. Costa, M, Cardoso, C, Afonso, C, Bandarra, NM, and Prates, JAM. Current knowledge and future perspectives of the use of seaweeds for livestock production and meat quality: a systematic review. J Anim Physiol Anim Nutr. (2021) 105:1075–102. doi: 10.1111/jpn.13509
63. Agregán, R, Munekata, P, Franco, D, Carballo, J, Barba, F, and Lorenzo, J. Antioxidant potential of extracts obtained from macro- (Ascophyllum nodosum, Fucus vesiculosus and Bifurcaria bifurcata) and Micro-algae (Chlorella vulgaris and Spirulina platensis) assisted by ultrasound. Medicines. (2018) 5:33. doi: 10.3390/medicines5020033
64. Dong, J-W, Cai, L, Xing, Y, Yu, J, and Ding, Z-T. Re-evaluation of ABTS•+ assay for Total antioxidant capacity of natural Products. Nat Prod Commun. (2015) 10:1934578X1501001. doi: 10.1177/1934578X1501001239
65. Ismail, MM, El Zokm, GM, and Miranda Lopez, JM. Nutritional, bioactive compounds content, and antioxidant activity of brown seaweeds from the Red Sea. Front Nutr. (2023) 10:1210934. doi: 10.3389/fnut.2023.1210934
66. Liga, S, Paul, C, and Péter, F. Flavonoids: overview of biosynthesis, biological activity, and current extraction techniques. Plan Theory. (2023) 12:2732. doi: 10.3390/plants12142732
67. Cory, H, Passarelli, S, Szeto, J, Tamez, M, and Mattei, J. The role of polyphenols in human health and food systems: a mini-review. Front Nutr. (2018) 5:87. doi: 10.3389/fnut.2018.00087
68. Obluchinskaya, ED, Pozharitskaya, ON, Gorshenina, EV, Daurtseva, AV, Flisyuk, EV, Generalova, YE, et al. Ascophyllum nodosum (Linnaeus) Le Jolis from Arctic: its biochemical composition, antiradical potential, and human health risk. Mar Drugs. (2024) 22:48. doi: 10.3390/md22010048
69. Sathya, R, Kanaga, N, Sankar, P, and Jeeva, S. Antioxidant properties of phlorotannins from brown seaweed Cystoseira trinodis (Forsskål) C. Agardh. Arab J Chem. (2017) 10:S2608–14. doi: 10.1016/j.arabjc.2013.09.039
70. Kadam, SU, Tiwari, BK, and O'Donnell, CP. Extraction, structure and biofunctional activities of laminarin from brown algae. Int J Food Sci Technol. (2015) 50:24–31. doi: 10.1111/ijfs.12692
71. Assadi, I, Elfalleh, W, Benabderrahim, MA, Hannachi, H, Chaalen, W, and Ferchichi, A. Nutritional quality and antioxidant capacity of a combination of pomegranate and date juices. Int J Fruit Sci. (2019) 19:300–14. doi: 10.1080/15538362.2018.1512438
72. Makanjuola, SA, Enujiugha, VN, Omoba, OS, and Sanni, DM. Combination of antioxidants from different sources could offer synergistic benefits: a case study of tea and ginger blend. Nat Prod Commun. (2015) 10:1829–32. doi: 10.1177/1934578X1501001110
73. Wang, J, Zhang, Q, Zhang, Z, and Li, Z. Antioxidant activity of sulfated polysaccharide fractions extracted from Laminaria japonica. Int J Biol Macromol. (2008) 42:127–32. doi: 10.1016/j.ijbiomac.2007.10.003
74. Daglia, M. Polyphenols as antimicrobial agents. Curr Opin Biotechnol. (2012) 23:174–81. doi: 10.1016/j.copbio.2011.08.007
75. da Silva, RP, Kawai, GSD, Andrade, FRD, Bezzon, VDN, and Ferraz, HG. Characterisation and traceability of calcium carbonate from the seaweed Lithothamnium calcareum. Solids. (2021) 2:192–211. doi: 10.3390/solids2020013
76. Zhu, Y, Connolly, A, Guyon, A, and FitzGerald, RJ. Solubilisation of calcium and magnesium from the marine red algaeLithothamnion calcareum. Int J Food Sci Technol. (2014) 49:1600–6. doi: 10.1111/ijfs.12459
77. Liu, D, Shi, J, Colina Ibarra, A, Kakuda, Y, and Jun Xue, S. The scavenging capacity and synergistic effects of lycopene, vitamin E, vitamin C, and β-carotene mixtures on the DPPH free radical. LWT Food Sci Technol. (2008) 41:1344–9. doi: 10.1016/j.lwt.2007.08.001
78. Etahiri, S, Bultel-Poncé, V, Caux, C, and Guyot, M. New Bromoditerpenes from the red alga Sphaerococcus coronopifolius. J Nat Prod. (2001) 64:1024–7. doi: 10.1021/np0002684
79. Darias, J, Rovirosa, J, San Martin, A, Díaz, A-R, Dorta, E, and Cueto, M. Furoplocamioids a−C, novel Polyhalogenated Furanoid monoterpenes fromPlocamiumcartilagineum. J Nat Prod. (2001) 64:1383–7. doi: 10.1021/np010297u
80. Barreto, M, and Meyer, JJM. Isolation and antimicrobial activity of a lanosol derivative from Osmundaria serrata (Rhodophyta) and a visual exploration of its biofilm covering. S Afr J Bot. (2006) 72:521–8. doi: 10.1016/j.sajb.2006.01.006
81. Kavita, K, Singh, VK, and Jha, B. 24-branched Δ5 sterols from Laurencia papillosa red seaweed with antibacterial activity against human pathogenic bacteria. Microbiol Res. (2014) 169:301–6. doi: 10.1016/j.micres.2013.07.002
82. Amorim, R, Rodrigues, JAG, Holanda, ML, Quinderé, ALG, Paula, RCM, Melo, VMM, et al. Antimicrobial effect of a crude sulfated polysaccharide from the red seaweed Gracilaria ornata. Braz Arch Biol Technol. (2012) 55:171–81. doi: 10.1590/S1516-89132012000200001
83. Stabili, L, Acquaviva, MI, Biandolino, F, Cavallo, RA, De Pascali, SA, Fanizzi, FP, et al. The lipidic extract of the seaweed Gracilariopsis longissima (Rhodophyta, Gracilariales): a potential resource for biotechnological purposes? New Biotechnol. (2012) 29:443–50. doi: 10.1016/j.nbt.2011.11.003
84. Catarino, M, Silva, A, and Cardoso, S. Fucaceae: a source of bioactive Phlorotannins. Int J Mol Sci. (2017) 18:1327. doi: 10.3390/ijms18061327
85. Peng, Y, Hu, J, Yang, B, Lin, X-P, Zhou, X-F, Yang, X-W, et al. Chemical composition of seaweeds. Seaweed Sustain. (2015):79–124. doi: 10.1016/B978-0-12-418697-2.00005-2
86. Ford, L, Curry, C, Campbell, M, Theodoridou, K, Sheldrake, G, Dick, J, et al. Effect of Phlorotannins from Brown seaweeds on the in vitro digestibility of pig Feed. Animals. (2020) 10:2193. doi: 10.3390/ani10112193
87. Makkar, HPS. Effects and fate of tannins in ruminant animals, adaptation to tannins, and strategies to overcome detrimental effects of feeding tannin-rich feeds. Small Rumin Res. (2003) 49:241–56. doi: 10.1016/S0921-4488(03)00142-1
88. Bury de Azevedo dos Santos, L, Genova, JL, de Oliveira Carvalho, PL, Evaristo Rupolo, P, Teixeira Carvalho, S, and Teixeira, CS. Calcitic seaweed. Anim Prod Sci. (2021) 61:662–72. doi: 10.1071/AN20008
89. Tibbetts, SM, Milley, JE, and Lall, SP. Nutritional quality of some wild and cultivated seaweeds: nutrient composition, total phenolic content and in vitro digestibility. J Appl Phycol. (2016) 28:3575–85. doi: 10.1007/s10811-016-0863-y
90. Brown, EM, Allsopp, PJ, Magee, PJ, Gill, CI, Nitecki, S, Strain, CR, et al. Seaweed and human health. Nutr Rev. (2014) 72:205–16. doi: 10.1111/nure.12091
91. Chater, PI, Wilcox, MD, Houghton, D, and Pearson, JP. The role of seaweed bioactives in the control of digestion: implications for obesity treatments. Food Funct. (2015) 6:3420–7. doi: 10.1039/C5FO00293A
92. Subbiah, V, Ebrahimi, F, Agar, OT, Dunshea, FR, Barrow, CJ, and Suleria, HAR. In vitro digestion and colonic fermentation of phenolic compounds and their antioxidant potential in Australian beach-cast seaweeds. Sci Rep. (2024) 14:4335. doi: 10.1038/s41598-024-54312-5
93. Wojtunik-Kulesza, K, Oniszczuk, A, Oniszczuk, T, Combrzyński, M, Nowakowska, D, and Matwijczuk, A. Influence of in vitro digestion on composition, bioaccessibility and antioxidant activity of food polyphenols—a non-systematic review. Nutrients. (2020) 12:1401. doi: 10.3390/nu12051401
94. Attri, S, Sharma, K, Raigond, P, and Goel, G. Colonic fermentation of polyphenolics from sea buckthorn (Hippophae rhamnoides) berries: assessment of effects on microbial diversity by principal component analysis. Food Res Int. (2018) 105:324–32. doi: 10.1016/j.foodres.2017.11.032
95. Wang, C, Wu, H, Liu, Z, Barrow, C, Dunshea, F, and Suleria, HAR. Bioaccessibility and movement of phenolic compounds from tomato (Solanum lycopersicum) during in vitro gastrointestinal digestion and colonic fermentation. Food Funct. (2022) 13:4954–66. doi: 10.1039/D2FO00223J
96. Dutra, RLT, Dantas, AM, Marques, DA, Batista, JDF, Meireles, BRLA, de Magalhães Cordeiro, ÂMT, et al. Bioaccessibility and antioxidant activity of phenolic compounds in frozen pulps of Brazilian exotic fruits exposed to simulated gastrointestinal conditions. Food Res Int. (2017) 100:650–7. doi: 10.1016/j.foodres.2017.07.047
97. Dantas, AM, Mafaldo, IM, Oliveira, PML, Lima, MS, Magnani, M, and Borges, GSC. Bioaccessibility of phenolic compounds in native and exotic frozen pulps explored in Brazil using a digestion model coupled with a simulated intestinal barrier. Food Chem. (2019) 274:202–14. doi: 10.1016/j.foodchem.2018.08.099
98. Cilla, A, Bosch, L, Barberá, R, and Alegría, A. Effect of processing on the bioaccessibility of bioactive compounds – a review focusing on carotenoids, minerals, ascorbic acid, tocopherols and polyphenols. J Food Compos Anal. (2018) 68:3–15. doi: 10.1016/j.jfca.2017.01.009
99. Chen, X, Lan, W, and Xie, J. Natural phenolic compounds: antimicrobial properties, antimicrobial mechanisms, and potential utilization in the preservation of aquatic products. Food Chem. (2024) 440:138198. doi: 10.1016/j.foodchem.2023.138198
100. Efenberger-Szmechtyk, M, Nowak, A, and Czyzowska, A. Plant extracts rich in polyphenols: antibacterial agents and natural preservatives for meat and meat products. Crit Rev Food Sci Nutr. (2021) 61:149–78. doi: 10.1080/10408398.2020.1722060
101. Lobiuc, A, Pavăl, N-E, Mangalagiu, II, Gheorghiță, R, Teliban, G-C, Amăriucăi-Mantu, D, et al. Future antimicrobials: natural and functionalized Phenolics. Molecules. (2023) 28:1114. doi: 10.3390/molecules28031114
102. Rowland, I, Gibson, G, Heinken, A, Scott, K, Swann, J, Thiele, I, et al. Gut microbiota functions: metabolism of nutrients and other food components. Eur J Nutr. (2018) 57:1–24. doi: 10.1007/s00394-017-1445-8
103. KO, KB, Kim, G, Kang, D, Kim, Y, Yang, I, and Ryu, Y. The influences of weaning age and weight on carcass traits and meat quality of pigs. Anim Sci J. (2015) 86:428–34. doi: 10.1111/asj.12314
104. Celi, P, Cowieson, AJ, Fru-Nji, F, Steinert, RE, Kluenter, A-M, and Verlhac, V. Gastrointestinal functionality in animal nutrition and health: new opportunities for sustainable animal production. Anim Feed Sci Technol. (2017) 234:88–100. doi: 10.1016/j.anifeedsci.2017.09.012
105. Michiels, JA, Kevers, C, Pincemail, J, Defraigne, JO, and Dommes, J. Extraction conditions can greatly influence antioxidant capacity assays in plant food matrices. Food Chem. (2012) 130:986–93. doi: 10.1016/j.foodchem.2011.07.117
106. Gardiner, GE, Campbell, AJ, O’Doherty, JV, Pierce, E, Lynch, PB, Leonard, FC, et al. Effect of Ascophyllum nodosum extract on growth performance, digestibility, carcass characteristics and selected intestinal microflora populations of grower–finisher pigs. Anim Feed Sci Technol. (2008) 141:259–73. doi: 10.1016/j.anifeedsci.2007.06.011
107. Dierick, N, Ovyn, A, and De Smet, S. Effect of feeding intact brown Seaweedascophyllum nodosumon some digestive parameters and on iodine content in edible tissues in pigs. J Sci Food Agric. (2009) 89:584–94. doi: 10.1002/jsfa.3480
108. Tang, X, Xiong, K, Fang, R, and Li, M. Weaning stress and intestinal health of piglets: a review. Front Immunol. (2022) 13:1042778. doi: 10.3389/fimmu.2022.1042778
109. Zheng, L, Duarte, ME, Sevarolli Loftus, A, and Kim, SW. Intestinal health of pigs upon weaning: challenges and nutritional intervention. Front Vet Sci. (2021) 8:628258. doi: 10.3389/fvets.2021.628258
110. EFSA Panel on Additives and Products or Substances used in Animal Feed (FEEDAP)Rychen, G, Aquilina, G, Azimonti, G, Bampidis, V, Bastos, M d L, et al. Guidance on the assessment of the safety of feed additives for the target species. EFSA J. (2017) 15:e05021. doi: 10.2903/j.efsa.2017.5021
111. Skrede, A, Mydland, L, Ahlstrøm, Ø, Reitan, K, Gislerød, H, and Øverland, M. Evaluation of microalgae as sources of digestible nutrients for monogastric animals. J Anim Feed Sci. (2011) 20:131–42. doi: 10.22358/jafs/66164/2011
112. Spínola, MP, Costa, MM, and Prates, JAM. Enhancing digestibility of Chlorella vulgaris biomass in Monogastric diets: strategies and insights. Animals. (2023) 13:1017. doi: 10.3390/ani13061017
113. Øverland, M, Mydland, LT, and Skrede, A. Marine macroalgae as sources of protein and bioactive compounds in feed for monogastric animals. J Sci Food Agric. (2019) 99:13–24. doi: 10.1002/jsfa.9143
114. Dempsey, E, and Corr, SC. Lactobacillus spp. for gastrointestinal health: current and future perspectives. Front Immunol. (2022) 13:840245. doi: 10.3389/fimmu.2022.840245
115. Yang, F, Hou, C, Zeng, X, and Qiao, S. The use of lactic acid Bacteria as a probiotic in swine diets. Pathogens. (2015) 4:34–45. doi: 10.3390/pathogens4010034
116. Roselli, M, Pieper, R, Rogel-Gaillard, C, de Vries, H, Bailey, M, Smidt, H, et al. Immunomodulating effects of probiotics for microbiota modulation, gut health and disease resistance in pigs. Anim Feed Sci Technol. (2017) 233:104–19. doi: 10.1016/j.anifeedsci.2017.07.011
117. Huang, C, Qiao, S, Li, D, Piao, X, and Ren, J. Effects of lactobacilli on the performance, diarrhea incidence, VFA concentration and gastrointestinal microbial Flora of weaning pigs. Asian Australas J Anim Sci. (2004) 17:401–9. doi: 10.5713/ajas.2004.401
118. O'Shea, CJ, McAlpine, P, Sweeney, T, Varley, PF, and O'Doherty, JV. Effect of the interaction of seaweed extracts containing laminarin and fucoidan with zinc oxide on the growth performance, digestibility and faecal characteristics of growing piglets. Br J Nutr. (2014) 111:798–807. doi: 10.1017/S0007114513003280
119. Wan, J, Jiang, F, Xu, Q, Chen, D, and He, J. Alginic acid oligosaccharide accelerates weaned pig growth through regulating antioxidant capacity, immunity and intestinal development. RSC Adv. (2016) 6:87026–35. doi: 10.1039/C6RA18135J
120. Choi, Y, Hosseindoust, A, Goel, A, Lee, S, Jha, PK, Kwon, IK, et al. Effects of Ecklonia cava as fucoidan-rich algae on growth performance, nutrient digestibility, intestinal morphology and caecal microflora in weanling pigs. Asian Australas J Anim Sci. (2017) 30:64–70. doi: 10.5713/ajas.16.0102
121. Bouwhuis, MA, Sweeney, T, Mukhopadhya, A, McDonnell, MJ, and O’Doherty, JV. Maternal laminarin supplementation decreases Salmonella Typhimurium shedding and improves intestinal health in piglets following an experimental challenge with S. typhimurium post-weaning. Anim Feed Sci Technol. (2017) 223:156–68. doi: 10.1016/j.anifeedsci.2016.11.007
122. Rattigan, R, Sweeney, T, Maher, S, Thornton, K, Rajauria, G, and O’Doherty, JV. Laminarin-rich extract improves growth performance, small intestinal morphology, gene expression of nutrient transporters and the large intestinal microbial composition of piglets during the critical post-weaning period. Br J Nutr. (2020) 123:255–63. doi: 10.1017/S0007114519002678
123. Walsh, AM, Sweeney, T, O'Shea, CJ, Doyle, DN, and Doherty, JVO. Effect of supplementing varying inclusion levels of laminarin and fucoidan on growth performance, digestibility of diet components, selected faecal microbial populations and volatile fatty acid concentrations in weaned pigs. Anim Feed Sci Technol. (2013) 183:151–9. doi: 10.1016/j.anifeedsci.2013.04.013
124. McDonnell, P, Figat, S, and O’Doherty, JV. The effect of dietary laminarin and fucoidan in the diet of the weanling piglet on performance, selected faecal microbial populations and volatile fatty acid concentrations. Animal. (2010) 4:579–85. doi: 10.1017/S1751731109991376
125. Ozdal, T, Capanoglu, E, and Altay, F. A review on protein–phenolic interactions and associated changes. Food Res Int. (2013) 51:954–70. doi: 10.1016/j.foodres.2013.02.009
126. Cirkovic Velickovic, TD, and Stanic-Vucinic, DJ. The role of dietary phenolic compounds in protein digestion and processing technologies to improve their Antinutritive properties. Compr Rev Food Sci Food Saf. (2018) 17:82–103. doi: 10.1111/1541-4337.12320
127. Czech, A, Woś, K, Muszyński, S, and Tomaszewska, E. Nutritional and Antioxidative benefits of dietary macroalgae supplementation in weaned piglets. Animals. (2024) 14:549. doi: 10.3390/ani14040549
128. Vignaud, J, Loiseau, C, Hérault, J, Mayer, C, Côme, M, Martin, I, et al. Microalgae produce antioxidant molecules with potential preventive effects on mitochondrial functions and skeletal muscular oxidative stress. Antioxidants. (2023) 12:1050. doi: 10.3390/antiox12051050
129. Chen, J, Huang, Z, Cao, X, Chen, X, Zou, T, and You, J. Plant-derived polyphenols as Nrf2 activators to counteract oxidative stress and intestinal toxicity induced by Deoxynivalenol in swine: an emerging research direction. Antioxidants. (2022) 11:2379. doi: 10.3390/antiox11122379
130. Garcia-Contreras, C, Vazquez-Gomez, M, Barbero, A, Pesantez, J, Zinellu, A, Berlinguer, F, et al. Polyphenols and IUGR pregnancies: effects of maternal Hydroxytyrosol supplementation on placental gene expression and fetal antioxidant status, DNA-methylation and phenotype. Int J Mol Sci. (2019) 20:1187. doi: 10.3390/ijms20051187
131. Yin, J, Han, H, Liu, Z, He, X, Li, T, and Yin, Y. Regulation of functional amino acids on oxidative stress in pigs. Scientia Sinica Vitae. (2019) 49:193–201. doi: 10.1360/N052018-00159
132. Scott, MB, Styring, AK, and McCullagh, JSO. Polyphenols: bioavailability, microbiome interactions and cellular effects on health in humans and animals. Pathogens. (2022) 11:770. doi: 10.3390/pathogens11070770
133. Lipiński, K, Antoszkiewicz, Z, Mazur-Kuśnirek, M, Korniewicz, D, and Kotlarczyk, S. The effect of polyphenols on the performance and antioxidant status of sows and piglets. Ital J Anim Sci. (2019) 18:174–81. doi: 10.1080/1828051X.2018.1503043
Keywords: Ascophyllum nodosum , Lithothamnium calcareum , seaweeds, bioactive compounds, antioxidant, antimicrobial, functional ingredients, post-weaning pigs
Citation: Frazzini S, Reggi S, Dell’Anno M, Fifi AP, Scaglia E, Ferri I and Rossi L (2024) Chemical-functional characterization of Ascophyllum nodosum and Phymatolithon calcareum and dietary supplementation in post-weaning pigs. Front. Vet. Sci. 11:1431091. doi: 10.3389/fvets.2024.1431091
Received: 11 May 2024; Accepted: 14 November 2024;
Published: 12 December 2024.
Edited by:
Bangyuan Wu, China West Normal University, ChinaReviewed by:
Leonel Pereira, University of Coimbra, PortugalCopyright © 2024 Frazzini, Reggi, Dell’Anno, Fifi, Scaglia, Ferri and Rossi. This is an open-access article distributed under the terms of the Creative Commons Attribution License (CC BY). The use, distribution or reproduction in other forums is permitted, provided the original author(s) and the copyright owner(s) are credited and that the original publication in this journal is cited, in accordance with accepted academic practice. No use, distribution or reproduction is permitted which does not comply with these terms.
*Correspondence: Matteo Dell’Anno, bWF0dGVvLmRlbGxhbm5vQHVuaW1pLml0
Disclaimer: All claims expressed in this article are solely those of the authors and do not necessarily represent those of their affiliated organizations, or those of the publisher, the editors and the reviewers. Any product that may be evaluated in this article or claim that may be made by its manufacturer is not guaranteed or endorsed by the publisher.
Research integrity at Frontiers
Learn more about the work of our research integrity team to safeguard the quality of each article we publish.