- 1School of Life Science and Engineering, Southwest University of Science and Technology, Mianyang, China
- 2Sichuan Academy of Animal Science, Chengdu, China
Probiotics are a group of active microorganisms that form colonies within the body and alter the composition of the flora in a specific area to provide benefits to the host. In this study, a total of 96 Duroc × Landrace × Yorkshire weaned piglets with an initial body weight (BW) of 8.56 ± 0.53 kg were employed in a randomized complete block design for a 28-day experiment. Pigs were randomly divided into two treatment groups: the control group (CON) and the complex probiotic group (CON + 0.2% probiotics), respectively. The study found that through the 28-day experiment, the average daily gain (ADG) of the complex probiotic group was significantly higher than that of the CON (p < 0.05). However, compared with the CON, the feed conversion efficiency significantly decreased on days 0–14 (p < 0.05). The addition of dietary complex probiotic significantly increased the villus height (VH) of duodenum and ileum, acetate, propionate, butyrate, and total short-chain fatty acids (SCFAs) in feces, and decreased fecal methyl mercaptans, acetic acid, and CO2 (p < 0.05). It concluded that feeding weaned piglets 0.2% complex probiotic increased the VH of duodenum and ileum, as well as changed the content of SCFAs in feces. This ultimately led to an increase in ADG.
1 Introduction
Weaning is a crucial stage in a pig’s life cycle. Stress during weaning can cause temporary or long-lasting damage to the intestinal barrier function, which will result in disease infections, anorexia, and economic losses in weaned piglets (1–3). In addition, weaning stress can reduce intestinal villus height (VH), which subsequently affects nutrient absorption (4). Therefore, it is important to alleviate weaning stress in piglets to minimize economic losses on farms.
Probiotics are a group of active microorganisms that form colonies within the body and alter the composition of the flora in a specific area to provide benefits to the host (5, 6). In recent years, probiotics have been extensively studied. The results showed that probiotics colonized the animal body and used nutrients in the intestinal tract that are difficult for the host to utilize (such as crude fiber) to carry out autotrophic metabolism and produce vitamins, amino acids, organic acids, enzymes, and other substances. This improves nutrient absorption by the animal, promotes intestinal health, and provides a certain degree of antimicrobial properties (2, 5–10). In addition, probiotics are considered an effective alternative to antibiotics, which have been partially banned due to the risk of antibiotic resistance (11). Therefore, adding probiotics to the diet is an effective way to alleviate weaning stress in piglets. Numerous previous studies have been conducted on the inclusion of probiotics in piglet diets (12–14). However, the efficacy of different probiotics to alleviate weaning stress requires further evaluation, particularly with the development of various types of probiotics. Previous studies have demonstrated that the addition of Bacillus licheniformis, Bacillus subtilis, Lactobacillus acidophilus, and Saccharomyces cerevisiae to the diet of weaned piglets has a significant positive impact on piglet growth performance (15–18). The resistance of different strains to the gastrointestinal environment varies. Furthermore, the effects of probiotics from different strains are multifactorial and strain-specific (19). Further investigation is required to ascertain whether different combinations of probiotics are beneficial or detrimental to piglets. The previous studies did not evaluate the addition of the mixture of four probiotics, namely Bacillus licheniformis, Bacillus subtilis, Lactobacillus acidophilus, and Saccharomyces cerevisiae, to piglets’ diet. Thus, this experiment aimed to evaluate the effects of the above probiotic mixture on growth performance, which could provide reference data for the use of different probiotics in piglets’ diet.
2 Materials and methods
2.1 Ethics statements and probiotics
The Institutional Review Board of the Southwest University of Science and Technology approved the animal study protocol (protocol code SM20220025). The probiotic product used in the study consisted of four types of mixed probiotics with an effective content of 5.1 × 107 CFU/g Bacillus licheniformis, 6.3 × 107 CFU/g Bacillus subtilis, 4.3 × 107 CFU/g Lactobacillus acidophilus, and 2.5 × 107 CFU/g Saccharomyces cerevisiae. The probiotics in this study were obtained from Sichuan Gao Fu Ji Biological Technology Co., Ltd. The amount of addition was 0.2% as recommended by the company. The production process adheres to production hygiene standards, and all probiotics that have undergone analysis have been found to be devoid of antibiotic resistant genes.
2.2 Experimental design, animals, and housing
A total of 96 4-week-old Duroc × Landrace × Yorkshire weaned piglets with an initial body weight (BW) of 8.56 ± 0.53 kg and weaned at 28 ± 1 day of age were employed in a randomized complete block design for a 28-day experiment. Pigs were randomly divided into two treatment groups based on BW and sex before the experiment. Each dietary treatment had 12 replicates with four pigs per replicate (two barrows and two gilts). The study involved two treatment groups: the control group (CON) and the probiotic group (CON + 0.2% probiotics). The diet used in CON (Table 1) was a compound feed in mash form, which was formulated to meet the nutrient requirements of weaned piglets (20). During the study, the pigs were housed in plastic floor pens (0.9 m × 1.6 m). They had unlimited access to feed and water via a self-feeder or nipple drinker. All pigs were disease free. The target room temperature of 20°C and humidity of 60% were maintained throughout the study.
2.3 Fecal score and sample collection
The fecal consistency was evaluated twice a day at 08:00 and 20:00 in each pen by observing the feces discharged on the floor and a five-point scale (1: hard, dry pellets; 2: firm, formed stools; 3: soft, moist stools that retain shape; 4: soft, unformed stools that assume the shape of the container; and 5: watery liquid that can be poured) was used to score the feces. The fecal score values were averaged after summarizing the values on a weekly basis in circles. BW of each pig was measured on days 0, 14, and 28 to determine the average daily gain (ADG). Feed consumption was recorded daily by measuring the amount of feed fed and remaining in the pen to calculate the average daily feed intake (ADFI) and feed-to-gain ratio (F: G). Chromium oxide, an indigestible marker, was added to the diet at a rate of 0.5% for 4 days (during days 11–14 and days 25–28) before fecal collection by rectal massage on days 12–14 and 26–28 to calculate the digestibility values of dry matter (DM), crude protein (CP), and gross energy (GE). At 8 o’ clock on day 28, two pigs (one male and one female) were selected from each pen for jugular vein blood collection. The blood collection was placed in heparinized tubes for further analysis. After collecting, one pig per pen was randomly selected for euthanasia and weighed to ensure a 1:1 ratio of males to females within the treatment group. The patient was administered intramuscular ketamine and thiazide anesthesia, followed by intravenous pentobarbital sodium. The remaining pigs were individually weighed and their data were recorded. Following the execution, a midline incision was made to the abdomen and thorax. Duodenal specimens were collected 30 cm from the stomach, while jejunal and ileal specimens were collected 2 m and 30 cm before the ileocecal junction, respectively. The tissue samples were then rinsed in cold 0.9% saline and stored in a 10% formalin buffer to fix the villi and crypts. Additionally, samples from the end of the colonic contents (replacement of feces) weighing 20, 40, and 100 g were collected to assess the presence of microbiota, short-chain fatty acids (SCFAs), and noxious gases, respectively.
2.4 Nutrient digestibility and hematological profiles analyses
After drying in a forced-air oven and grinding through a 1 mm sieve, the dietary and fecal samples were analyzed. The method of Liu et al. (16) was used to determine the levels of calcium (Ca) and phosphorus (P) in the diet. The DM content of the feces was determined by drying them in a forced-air oven at 105°C for 2 h (21). The concentration of CP in the experimental diets and feces was determined by using the combustion method (21). Dietary and fecal GE were measured by using a fully automated calorimeter (BYLRY-3000 W). After nitrification, a spectrophotometer was used to measure the concentration of Cr2O3 in the diets and feces by reading the absorbance at 450 nm. The apparent total tract digestibility was determined by the levels of Cr, DM, CP, and GE in the feed and feces. Calculate the apparent total tract digestibility of the nutrient using the following equation ATTD = [1 − (Crd/Crf) × (Nf/Nd)] × 100% (Crd = Cr concentration in diets; Crf = Cr concentration in feaces; Nf = nutrient concentration in feaces; Nd = nutrient concentration in diets). Blood indicators were measured by using an automatic biochemical analyzer (BK-600, Biobased, Jinan City, Shandong Province, China) (21). Concentrations of interleukin-1β (IL-1β), interleukin-6 (IL-6), and interleukin-10 (IL-10) in weaned piglets were measured by using the ELISA kit from the Jiancheng Institute of Biological Technology in Nanjing, China. The cytokine analysis was conducted in duplicate following the manufacturer’s instructions. ELISA kits validated for weaned piglets (Nanjing Jiancheng Biology Co., Ltd.) were used to analyze the concentrations of IgA, IgG, and IgM, following the described methods.
2.5 Microbiota analysis
Fecal microbial assays were conducted by following the protocol described by Liu et al. (22). Total DNA from fecal samples was extracted by using Omega BioTec’s stool DNA isolation kit (Norcross, GA, United States). The experiment aimed to determine the concentrations of six bacterial species: Prevotella, Bacteroides, Bifidobacterium, Escherichia coli, Lactobacillus, and Bacillus. Table 2 displays the primer sequences and corresponding annealing temperatures. In the fluorescence-quantitative PCR reaction system, 11 μL were used, comprising of 2 μL of DNA, 2.7 μL of RNase-free water, 0.4 μL (10 M) of upstream and downstream primers, and 5.5 μL of 2 KAPA SYBR FAST qPCR Kit Master Mix. The reaction conditions were as follows: the temperature was set to 50°C for 2 min, followed by 95°C for 10 min. Then, 40 cycles of denaturation/annealing were carried out at 95°C for 15 s and 60°C for 1 min. Finally, a melting curve process was performed from 70 to 90°C, increasing by 0.5°C every 5 s. The fluorescence-quantitative reaction was performed on an ABI 7600 instrument.
2.6 Determination of SCFAs
The homogenized powders obtained after lyophilization and pestling were extracted with 1 mL of methanol (gradient grade for liquid chromatography Li Chrosolv R Reag. Ph Eur EA). After 10 min of sonication, the samples were centrifuged at 6,000 rpm for 10 min, and the resulting supernatants were used for GC–MS analysis. The GC-MS analysis was performed by using a GC-MS-QP2010 Ultra with an autosampler (SHIMADZU) and the Rtx-wax capillary column (30 m, 0.25 mm i.d., 0.25 mm film thickness; SHIMADZU). The oven temperature was programmed to increase from 60 to 100°C at a rate of 5°C/min, with a 1 min hold; then to 150°C at a rate of 5°C/min, with a 5 min hold; and finally, to 225°C at a rate of 30°C/min, with a 20 min hold. A 2 mL of sample was injected at 230°C. Helium was used as the carrier gas with a flow rate of 1.2 mL/min. Electronic impact was recorded at 70 eV.
2.7 Gas emissions analyses
The fecal samples (100 g each) from each pen were placed in separate 2.6-L plastic containers with a small hole in the middle of one side. The containers were sealed with tape. The samples were allowed to ferment at room temperature (25°C) for 24 h. Then, approximately 2.0 cm above the fecal sample, 100 mL of headspace air was sampled. The small hole for gas detection was closed again after the gas was collected. Prior to measurement, the fecal samples were shaken manually for approximately 30 s. This was to break up any crusts on the surface and to homogenize the samples. A portable 6-in-1 gas detector (SGA-606, ingoan, Shenzhen, Guangdong Province, China) was used to measure the concentrations of NH3, H2S, mercaptan, acetic acid, and CO2.
2.8 Intestinal morphometry
The small intestine segments were cut into 5 mm sections and stained by using the HE method according to previous study (29). The VH and crypt depth (CD) were measured by randomly selecting 10 VH and 10 CD per section from images captured under a Carl Zeiss light microscope from each sample.
2.9 Statistical analyses
Data were analyzed by using the SAS (SAS Inst. Inc., Cary, NC, United States). After testing, all data are normally distributed. The t-test statistically analyzed the experimental data. Growth performance, nutrient digestibility, and fecal score were analyzed by using the pen as the experimental unit. Intestinal morphology, hematological profiles, fecal microbiota, fecal SCFAs, and fecal gas emissions were analyzed by using the individual pig as the experimental unit. Results with p < 0.05 were considered statistically significant.
3 Results
3.1 Growth performance, nutrient digestibility, and fecal score
There were significant changes in body weight (Day 14 and Day 28) and ADG (Day 0–28), and it was extremely significant (p < 0.01) on final body weight (Day 28) and ADG (Day 0–28) with the addition of dietary complex probiotics (Table 3; p < 0.05). F: G decreased when added complex probiotics to the diet on days 0–14 (p < 0.05). But compared with CON pigs, dietary complex probiotics supplementation did not affect ADFI (p > 0.05). Meanwhile, there was no effect on nutrient digestibility in weaned pigs after adding the complex probiotics to the diet, whether on day 14 or 28 (Table 4; p > 0.05). The addition of dietary complex probiotic had no significant effect on fecal score (Table 5; p > 0.05).
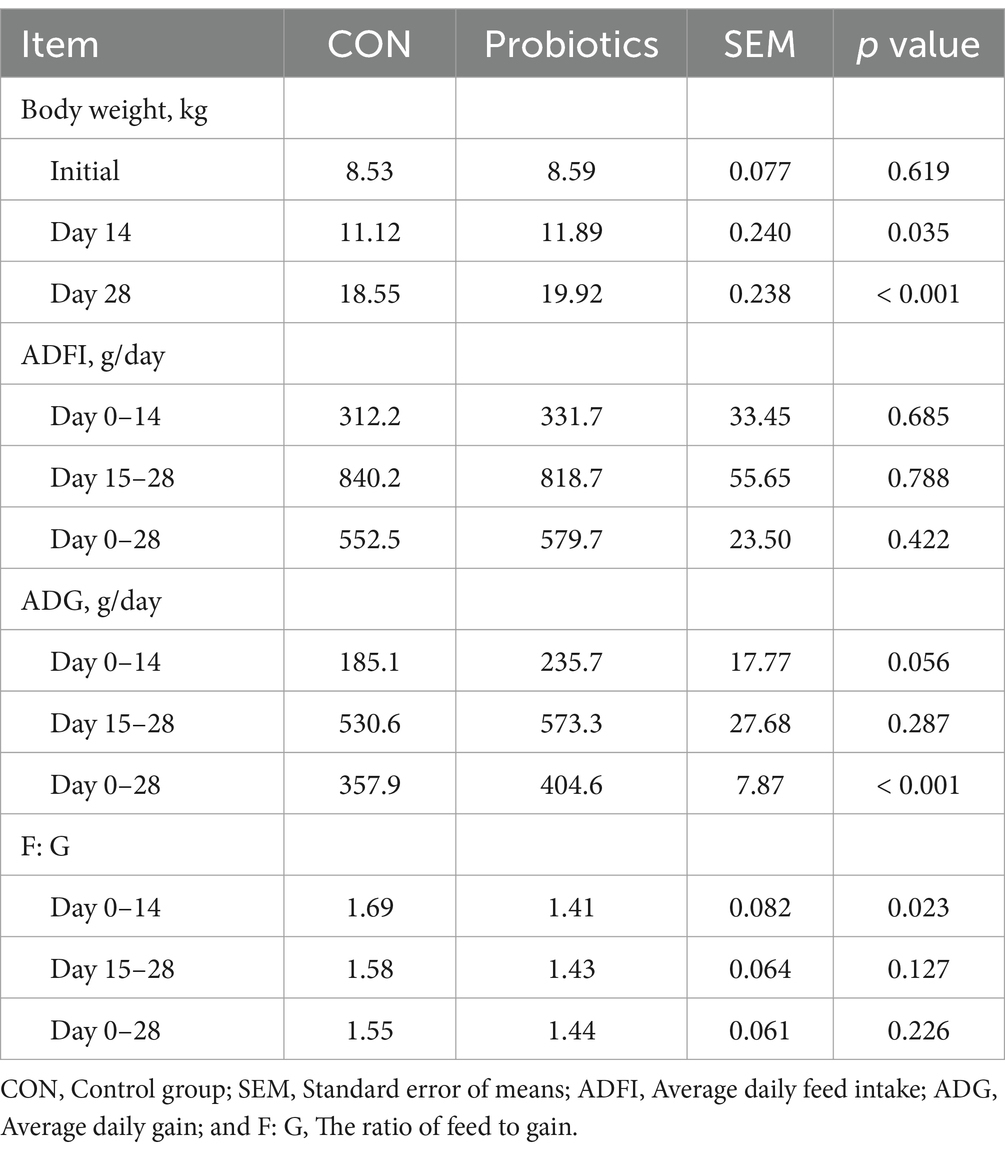
Table 3. Effects of dietary complex probiotics supplementation on growth performance in weaned piglets.
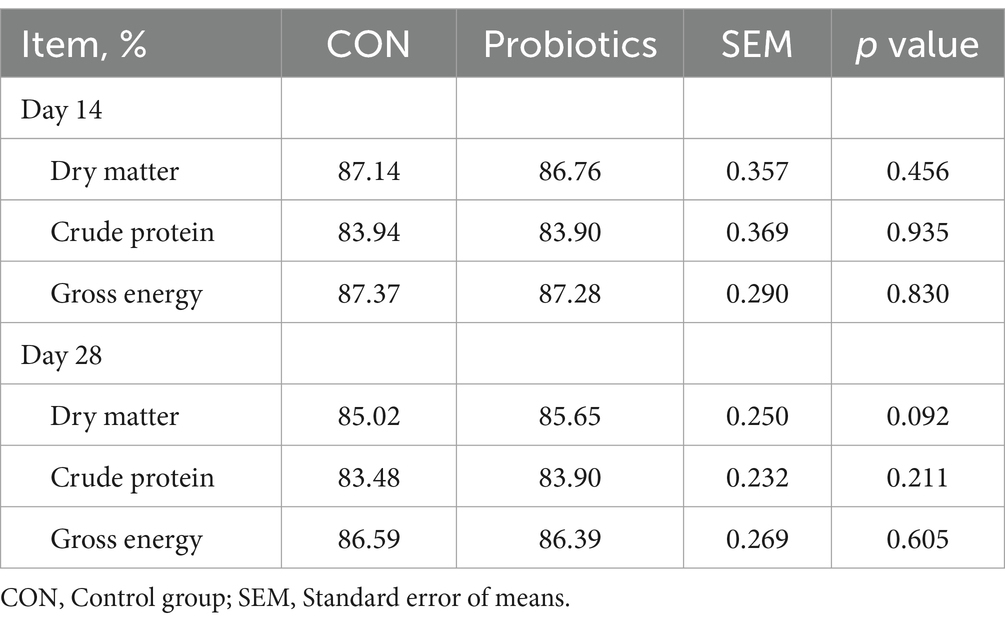
Table 4. Effects of dietary complex probiotics supplementation on apparent total tract digestibility of nutrient in weaned piglets.
3.2 Hematological profiles
The addition of complex probiotic to the diet resulted in a significant reduction in blood glucose concentration compared to CON (Table 6; p < 0.05), while the addition of complex probiotic to the diet did not affect the blood concentrations of IgA, IgG, IgM, IL-1β, IL-6, IL-10, albumin, total cholesterol, and total protein (p > 0.05).
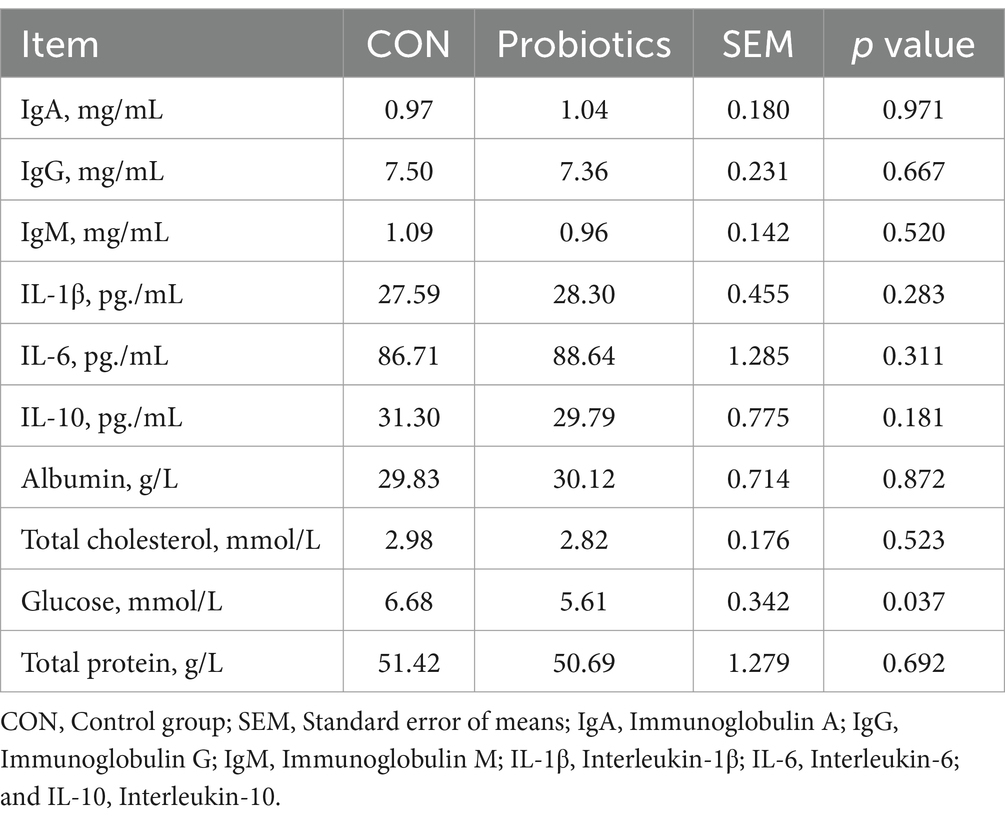
Table 6. Effects of dietary complex probiotics supplementation on hematological profiles in weaned piglets.
3.3 Intestinal morphology and fecal microbiota
Table 7 and Figure 1 showed that the dietary complex probiotic addition significantly increased the VH and VH: CD of duodenum and ileum in weaned piglets, compared to CON (p < 0.05). However, the addition of complex probiotic to the diet had no significant effect on the morphology of the jejunum in piglets (p > 0.05). In addition, we did not find differences in fecal microbiota (Table 8, p > 0.05).
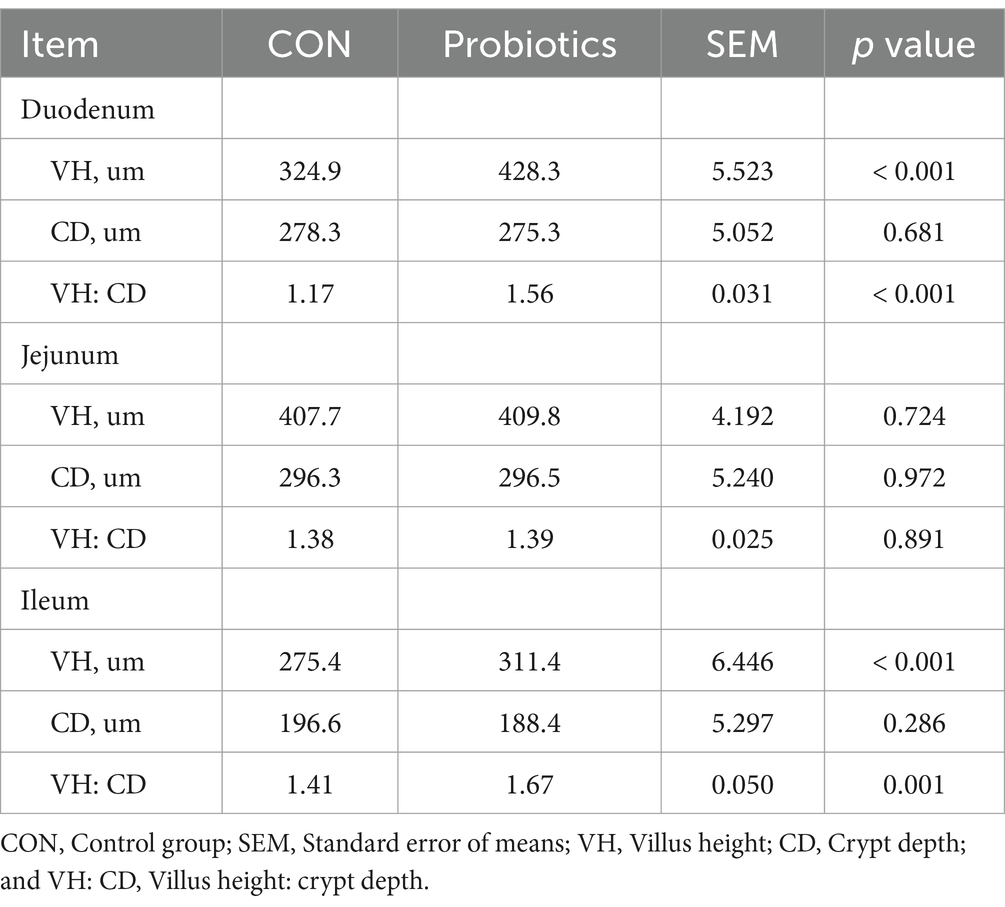
Table 7. Effects of dietary complex probiotics supplementation on intestinal morphology in weaned piglets.
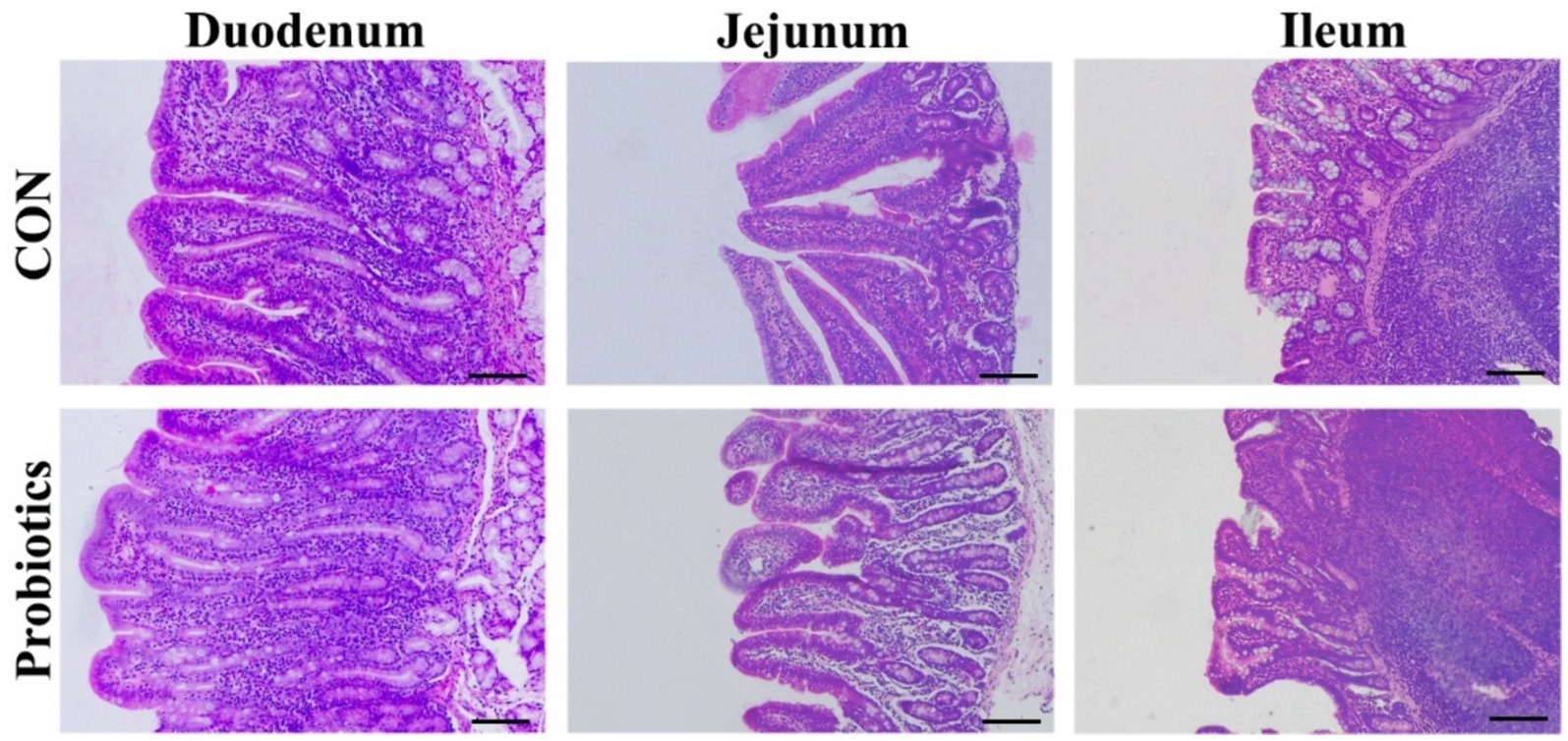
Figure 1. The morphology of duodenum, jejunum, and ileum in weaned piglets fed with basal diets supplemented with complex probiotics (Scale bar: 100 μm).
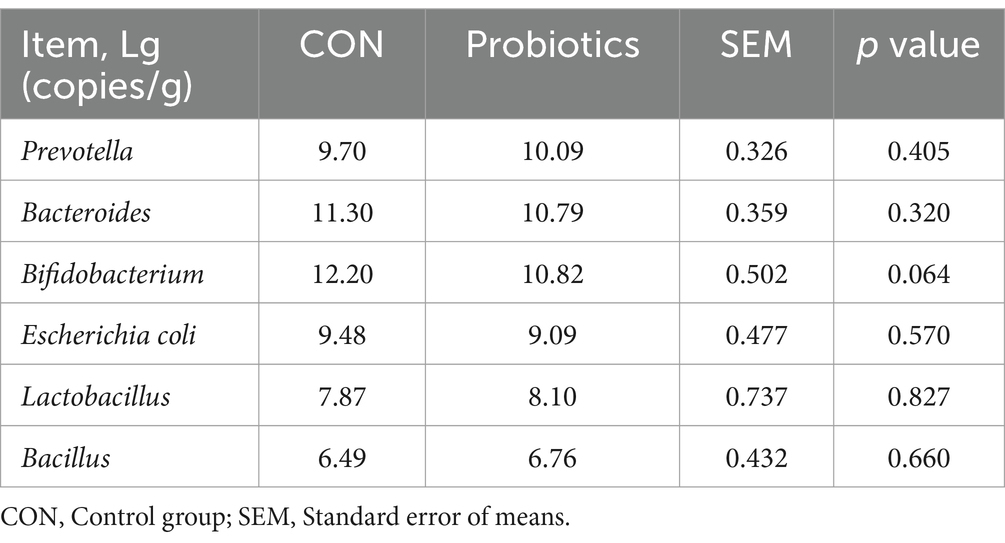
Table 8. Effects of dietary complex probiotics supplementation on fecal microbiota in weaned piglets.
3.4 Fecal SCFAs and fecal gas emissions
Concentrations of acetate, propionate, butyrate, and total SCFAs in feces were significantly higher in the complex probiotic group than in CON (Table 9; p < 0.05). Furthermore, the dietary inclusion of complex probiotic significantly decreased the concentrations of methyl mercaptans, acetic acid, and CO2 in the feces of piglets on day 28 (Table 10; p < 0.05). However, the addition of complex probiotic did not have a significant effect on the concentrations of fecal microbiota (Table 8), isobutyrate, isovalerate, valerate, NH3, and H2S (p > 0.05).
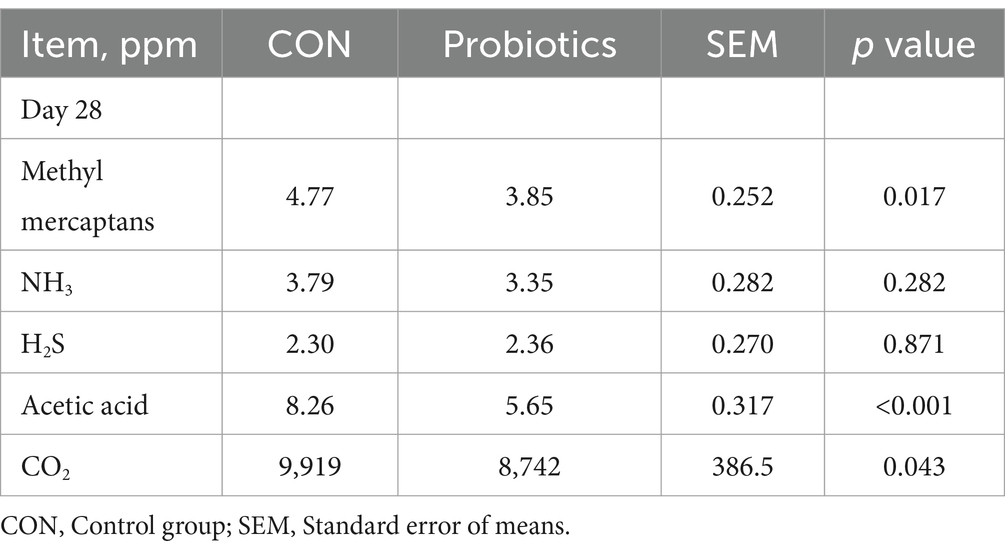
Table 10. Effects of dietary complex probiotics supplementation on fecal gas emissions in weaned piglets.
4 Discussion
Weaning stress in piglets can have negative economic effects (1, 2). It is a straightforward approach to evaluate economic effects through growth performance. A variety of factors can influence growth performance, feed nutrients, management practices, and external stimuli (30–34). The study results indicated that adding complex probiotic to piglets’ diets effectively increased ADG and decreased F: G during days 0–14. However, there was no effect on ADG and F: G during days 15–28. Throughout the trial, there was no significant difference in ADFI between the two treatment groups. The present study compared the effect of adding four different probiotics mixture to the diet. Previous study has shown that the addition of probiotics either alone or in combination had no impact on feed intake and F: G. Furthermore, the enhancement of the growth performance of piglets by the dietary addition of probiotics was primarily achieved by an increase in the daily weight (17). However, the results of this study showed that the addition of complex probiotic alleviated the negative effects of weaning stress by reducing F: G (1.69–1.41) and enhancing ADG (185.1–235.7 g) in the early stage of weaning (days 0–14). Although ADG (530.6–573.3 g) and F: G (1.58–1.43) were changed to a certain extent in the second half of the piglet’s life (days 15–28), but the effect was not significant. However, in a study where Bacillus licheniformis was added alone, the results indicated that there was no significant effect on the ADG of piglets during the first 14 days, but there was a significant increase in ADG on days 14–28 (35). Another study showed that the addition of Saccharomyces cerevisiae to diets also observed significant differences in body weight over time (15). The above results are not consistent with the results of the present study. In conclusion, the aforementioned results indicated that the phase of interest when studying the dietary complex probiotics addition may be 2 weeks after weaning. Previous research has indicated that weaning stress can cause diarrhea and decrease the height of the intestinal villi (3, 4). This study found that there was no significant difference in fecal scores between the two treatment groups. The mean value of the scores ranged from 3 to 4 (moist stool that retains shape). This study did not find any evidence of severe diarrhea. The same result was observed in the study of Bacillus licheniformis as a sole component of the diet.
Nutrients, including glucose, amino acids, glycerol, and fatty acids, are primarily absorbed in the small intestine. The height of the small intestinal villi affects the uptake and absorption of nutrients to some extent. Although this study found that the addition of complex probiotic increased the VH of the duodenum and ileum, there was no significant effect on the apparent total tract digestibility of the nutrients. The result suggested that the growth performance of the complex probiotic group may be improved due to the different fermentation capacities of the hindgut microorganisms.
The mammalian gut harbors a considerable and heterogeneous microbial community. The host and gut microbes are mutually dependent, coordinating and constraining each other in order to achieve homeostasis within the organism (36, 37). SCFAs are the end-products of intestinal bacterial fermentation with various metabolic characteristics. They can provide energy for the intestinal flora and the host’s epithelial cells, maintain intestinal acid–base balance, inhibit the growth of harmful pathogens, and regulate the host’s intestinal immune system so as to alleviate the inflammatory response (38). In the study, we analyzed the fecal microbiota content and found no significant difference between the two treatment groups. Then, by measuring fecal SCFAs, we found that concentrations of acetate, propionate, butyrate, and total SCFAs in feces were significantly higher in the complex probiotic group than in CON. The results indicated that the fermentation capacity of gut microorganisms was higher in the complex probiotic group. Nevertheless, the observed differences were not statistically significant in terms of the measured gut microbial content. Consequently, the use of alternative methods may be required to determine a more comprehensive range of gut microbial species and content. Butyric acid and acetate can be absorbed by the hindgut and provide a significant amount of energy for the body (39). This could be the primary reason why adding complex probiotic to the diet enhanced growth performance. Additionally, we analyzed the blood profile of the piglets. IL-6 and IL-10 are associated with inflammatory responses (40–42). IgA, IgG, and IgM are associated with immune function (43–45). However, this study did not find any differences in the aforementioned factors between the two treatment groups. However, a significant reduction in blood glucose levels was found in the probiotic complex group. Previous studies have shown that higher levels of SCFAs can significantly reduce plasma glucose levels (46, 47). Its primary function is to regulate blood glucose levels through the hepatic AMP-activated protein kinase pathway and to affect blood glucose levels by increasing intestinal satiety hormones (47). The results indicated that the probiotic complex group changed the composition of fecal SCFAs, reduced blood glucose levels, and improved growth performance.
With the intensification of the pig industry, the impact on emissions of environmental pollution and animal health becomes increasingly significant. Studies have shown that emissions from large farms can spread up to 5 km in the vicinity (48–50). Harmful gases such as methyl mercaptans, acetic acid, H2S, and NH3 can cause respiratory damage in pigs and reduce growth performance (51). The results of this study showed that the dietary complex probiotic supplementation significantly decreased the concentrations of methyl mercaptans, acetic acid, and CO2 in the feces of piglets. In conclusion, the addition of dietary complex probiotic can effectively reduce the level of harmful gases in the pig house.
5 Conclusion
Dietary supplementation with 0.2% probiotics improved gut health by increasing duodenal and ileal VH, and increased final BW by increasing ADG.
Data availability statement
The raw data supporting the conclusions of this article will be made available by the authors, without undue reservation.
Ethics statement
The animal studies were approved by Committee of Southwest University of Science and Technology. The studies were conducted in accordance with the local legislation and institutional requirements. Written informed consent was obtained from the owners for the participation of their animals in this study.
Author contributions
JZ: Conceptualization, Data curation, Writing – original draft, Writing – review & editing. ZX: Data curation, Writing – original draft. MZ: Data curation, Writing – review & editing. WT: Software, Writing – review & editing. HD: Writing – review & editing. HY: Conceptualization, Data curation, Methodology, Writing – original draft, Writing – review & editing.
Funding
The author(s) declare that financial support was received for the research, authorship, and/or publication of this article. This research was funded by Sichuan Science and Technology Program (2023YFQ0031; 2023ZHYZ0007) and Natural Science Foundation of Southwest University of Science and Technology (22zx7152).
Conflict of interest
The authors declare that the research was conducted in the absence of any commercial or financial relationships that could be construed as a potential conflict of interest.
Publisher’s note
All claims expressed in this article are solely those of the authors and do not necessarily represent those of their affiliated organizations, or those of the publisher, the editors and the reviewers. Any product that may be evaluated in this article, or claim that may be made by its manufacturer, is not guaranteed or endorsed by the publisher.
References
1. Lallès, J-P, Bosi, P, Smidt, H, and Stokes, CR. Weaning — a challenge to gut physiologists. Livest Sci. (2007) 108:82–93. doi: 10.1016/j.livsci.2007.01.091
2. Liao, SF, and Nyachoti, M. Using probiotics to improve swine gut health and nutrient utilization. Anim Nutr. (2017) 3:331–43. doi: 10.1016/j.aninu.2017.06.007
3. O’Loughlin, A, McGee, M, Waters, SM, Doyle, S, and Earley, B. Examination of the bovine leukocyte environment using immunogenetic biomarkers to assess immunocompetence following exposure to weaning stress. BMC Vet Res. (2011) 7:45. doi: 10.1186/1746-6148-7-45
4. Boudry, G, Péron, V, Le Huërou-Luron, I, Lallès, JP, and Sève, B. Weaning induces both transient and long-lasting modifications of absorptive, secretory, and barrier properties of piglet intestine. J Nutr. (2004) 134:2256–62. doi: 10.1093/jn/134.9.2256
5. Arif, M, Iram, A, Bhutta, MAK, Naiel, MAE, Abd El-Hack, ME, Othman, SI, et al. The biodegradation role of Saccharomyces cerevisiae against harmful effects of mycotoxin contaminated diets on broiler performance, immunity status, and carcass characteristics. Animals. (2020) 10:238. doi: 10.3390/ani10020238
6. Alagawany, M, Abd El-Hack, ME, Farag, MR, Sachan, S, Karthik, K, and Dhama, K. The use of probiotics as eco-friendly alternatives for antibiotics in poultry nutrition. Environ Sci Pollut Res. (2018) 25:10611–8. doi: 10.1007/s11356-018-1687-x
7. Hori, T, Matsuda, K, and Oishi, K. Probiotics: a dietary factor to modulate the gut microbiome, host immune system, and gut-brain interaction. Microorganisms. (2020) 8:1401. doi: 10.3390/microorganisms8091401
8. Meng, QW, Yan, L, Ao, X, Zhou, TX, Wang, JP, Lee, JH, et al. Influence of probiotics in different energy and nutrient density diets on growth performance, nutrient digestibility, meat quality, and blood characteristics in growing-finishing pigs. J Anim Sci. (2010) 88:3320–6. doi: 10.2527/jas.2009-2308
9. Xuan, ZN, Kim, JD, Heo, KN, Jung, HJ, Lee, JH, Han, YK, et al. Study on the development of a probiotics complex for weaned pigs. Asian Australas J Anim Sci. (2001) 14:1425–8. doi: 10.5713/ajas.2001.1425
10. Giang, HH, Viet, TQ, Ogle, B, and Lindberg, JE. Effects of supplementation of probiotics on the performance, nutrient digestibility and Faecal microflora in growing-finishing pigs. Asian Australas J Anim Sci. (2011) 24:655–61. doi: 10.5713/ajas.2011.10238
11. Roselli, M, Pieper, R, Rogel-Gaillard, C, de Vries, H, Bailey, M, Smidt, H, et al. Immunomodulating effects of probiotics for microbiota modulation, gut health and disease resistance in pigs. Anim Feed Sci Technol. (2017) 233:104–19. doi: 10.1016/j.anifeedsci.2017.07.011
12. Dong, X, Zhang, N, Zhou, M, Tu, Y, Deng, K, and Diao, Q. Effects of dietary probiotics on growth performance, faecal microbiota and serum profiles in weaned piglets. Anim Prod Sci. (2013) 54:616–21. doi: 10.1071/AN12372
13. Wang, J, Li, S, Tang, W, Diao, H, Zhang, H, Yan, H, et al. Dietary complex probiotic supplementation changed the composition of intestinal short-chain fatty acids and improved the average daily gain of growing pigs. Vet Sci. (2023) 10:79. doi: 10.3390/vetsci10020079
14. Vieira, AM, Sessin, AP, Soratto, TAT, Pires, PGDS, Cardinal, KM, Wagner, G, et al. Effect of functional oils or probiotics on performance and microbiota profile of newly weaned piglets. Sci Rep. (2021) 11:19457. doi: 10.1038/s41598-021-98549-w
15. Kiros, TG, Luise, D, Derakhshani, H, Petri, R, Trevisi, P, D’Inca, R, et al. Effect of live yeast Saccharomyces cerevisiae supplementation on the performance and cecum microbial profile of suckling piglets. PLoS One. (2019) 14:e0219557. doi: 10.1371/journal.pone.0219557
16. Deng, B, Wu, J, Li, X, Zhang, C, Men, X, and Xu, Z. Effects of Bacillus subtilis on growth performance, serum parameters, digestive enzyme, intestinal morphology, and colonic microbiota in piglets. AMB Express. (2020) 10:212. doi: 10.1186/s13568-020-01150-z
17. Qiao, J, Li, H, Wang, Z, and Wang, W. Effects of Lactobacillus acidophilus dietary supplementation on the performance, intestinal barrier function, rectal microflora and serum immune function in weaned piglets challenged with Escherichia coli lipopolysaccharide. Antonie Van Leeuwenhoek. (2015) 107:883–91. doi: 10.1007/s10482-015-0380-z
18. Yu, X, Cui, Z, Qin, S, Zhang, R, Wu, Y, Liu, J, et al. Effects of Bacillus licheniformis on growth performance, diarrhea incidence, antioxidant capacity, immune function, and fecal microflora in weaned piglets. Animals. (2022) 12:1609. doi: 10.3390/ani12131609
19. Weichselbaum, E. Probiotics and health: a review of the evidence. Nutr Bull. (2009) 34:340–73. doi: 10.1111/j.1467-3010.2009.01782.x
20. National Research Council . Nutrient Requirements of Swin. 11th ed. Washington, DC: The National Academies Press (2012). 420 p.
21. AOAC (2007). Official methods of analysis. 18th ed. Arlington (VA): Association of Official Analytical Chemists. Available at: https://www.docin.com/p-933447075.html (Accessed July 31, 2022).
22. Liu, J, Pu, Y-Y, Xie, Q, Wang, J-K, and Liu, J-X. Pectin induces an in vitro rumen microbial population shift attributed to the Pectinolytic Treponema group. Curr Microbiol. (2015) 70:67–74. doi: 10.1007/s00284-014-0672-y
23. Layton, A, McKay, L, Williams, D, Garrett, V, Gentry, R, and Sayler, G. Development of Bacteroides 16S rRNA gene Taq man-based real-time PCR assays for estimation of Total, human, and bovine fecal pollution in water. Appl Environ Microbiol. (2006) 72:4214–24. doi: 10.1128/AEM.01036-05
24. Bergström, A, Licht, TR, Wilcks, A, Andersen, JB, Schmidt, LR, Grønlund, HA, et al. Introducing GUt low-density Array (GULDA) – a validated approach for qPCR-based intestinal microbial community analysis. FEMS Microbiol Lett. (2012) 337:38–47. doi: 10.1111/1574-6968.12004
25. Walker, AW, Ince, J, Duncan, SH, Webster, LM, Holtrop, G, Ze, X, et al. Dominant and diet-responsive groups of bacteria within the human colonic microbiota. ISME J. (2011) 5:220–30. doi: 10.1038/ismej.2010.118
26. Huijsdens, XW, Linskens, RK, Mak, M, Meuwissen, SGM, Vandenbroucke-Grauls, CMJE, and Savelkoul, PHM. Quantification of Bacteria adherent to gastrointestinal mucosa by real-time PCR. J Clin Microbiol. (2002) 40:4423–7. doi: 10.1128/JCM.40.12.4423-4427.2002
27. Khafipour, E, Li, S, Plaizier, JC, and Krause, DO. Rumen microbiome composition determined using two nutritional models of subacute ruminal acidosis. Appl Environ Microbiol. (2009) 75:7115–24. doi: 10.1128/AEM.00739-09
28. Fernández-No, IC, Guarddon, M, Böhme, K, Cepeda, A, Calo-Mata, P, and Barros-Velázquez, J. Detection and quantification of spoilage and pathogenic Bacillus cereus, Bacillus subtilis and Bacillus licheniformis by real-time PCR. Food Microbiol. (2011) 28:605–10. doi: 10.1016/j.fm.2010.10.014
29. Wang, M, Wang, L, Tan, X, Wang, L, Xiong, X, Wang, Y, et al. The developmental changes in intestinal epithelial cell proliferation, differentiation, and shedding in weaning piglets. Anim Nutr. (2022) 9:214–22. doi: 10.1016/j.aninu.2021.11.006
30. Johnson, JS, Aardsma, MA, Duttlinger, AW, and Kpodo, KR. Early life thermal stress: impact on future thermotolerance, stress response, behavior, and intestinal morphology in piglets exposed to a heat stress challenge during simulated transport 1. J Anim Sci. (2018) 96:1640–53. doi: 10.1093/jas/sky107
31. Cao, SC, Gong, J, Wang, J, Yan, HL, Zhang, HF, and Liu, JB. The impact of the interaction between dietary total phosphorus level and efficacy of phytase on the performance of growing-finishing pigs. Anim Feed Sci Technol. (2023) 298:115605. doi: 10.1016/j.anifeedsci.2023.115605
32. Zhai, H, Adeola, O, and Liu, J. Phosphorus nutrition of growing pigs. Anim Nutr. (2022) 9:127–37. doi: 10.1016/j.aninu.2022.01.010
33. Cao, S, Wang, J, Zhao, J, Li, S, Tang, W, Diao, H, et al. Dietary soybean oligosaccharides addition increases growth performance and reduces lipid deposition by altering fecal short-chain fatty acids composition in growing pigs. Animals. (2023) 13:3648. doi: 10.3390/ani13233648
34. Cao, S, Yan, H, Tang, W, Zhang, H, and Liu, J. Effects of dietary coenzyme Q10 supplementation during gestation on the embryonic survival and reproductive performance of high-parity sows. J Anim Sci Biotechnol. (2023) 14:75. doi: 10.1186/s40104-023-00879-4
35. Cao, G, Yang, S, Wang, H, Zhang, R, Wu, Y, Liu, J, et al. Effects of Bacillus licheniformis on the growth performance, antioxidant capacity, Ileal morphology, intestinal short chain fatty acids, and colonic microflora in piglets challenged with lipopolysaccharide. Animals. (2023) 13:2172. doi: 10.3390/ani13132172
36. Felizardo, RJF, Watanabe, IKM, Dardi, P, Rossoni, LV, and Câmara, NOS. The interplay among gut microbiota, hypertension and kidney diseases: the role of short-chain fatty acids. Pharmacol Res. (2019) 141:366–77. doi: 10.1016/j.phrs.2019.01.019
37. Qiao, Y, Sun, J, Xia, S, Tang, X, Shi, Y, and Le, G. Effects of resveratrol on gut microbiota and fat storage in a mouse model with high-fat-induced obesity. Food Funct. (2014) 5:1241–9. doi: 10.1039/C3FO60630A
38. Sadler, R, Cramer, JV, Heindl, S, Kostidis, S, Betz, D, Zuurbier, KR, et al. Short-chain fatty acids improve Poststroke recovery via immunological mechanisms. J Neurosci. (2020) 40:1162–73. doi: 10.1523/JNEUROSCI.1359-19.2019
39. Binder, HJ. Role of colonic short-chain fatty acid transport in diarrhea. Annu Rev Physiol. (2010) 72:297–313. doi: 10.1146/annurev-physiol-021909-135817
40. Degré, M. Interferons and other cytokines in bacterial infections. J Interf Cytokine Res. (1996) 16:417–26. doi: 10.1089/jir.1996.16.417
41. Conti, P, Kempuraj, D, Kandere, K, Gioacchino, MD, Barbacane, RC, Castellani, ML, et al. IL-10, an inflammatory/inhibitory cytokine, but not always. Immunol Lett. (2003) 86:123–9. doi: 10.1016/S0165-2478(03)00002-6
42. Opal, SM, and DePalo, VA. Anti-inflammatory cytokines. Chest. (2000) 117:1162–72. doi: 10.1378/chest.117.4.1162
43. Chodirker, WB, and Tomasi, TB. Gamma-globulins: quantitative relationships in human serum and nonvascular fluids. Science. (1963) 142:1080–1. doi: 10.1126/science.142.3595.1080
44. Vidarsson, G, Dekkers, G, and Rispens, T. IgG subclasses and Allotypes: from structure to effector functions. Front Immunol. (2014) 5:520. doi: 10.3389/fimmu.2014.00520
45. Keyt, BA, Baliga, R, Sinclair, AM, Carroll, SF, and Peterson, MS. Structure, function, and therapeutic use of IgM antibodies. Antibodies. (2020) 9:53. doi: 10.3390/antib9040053
46. Fushimi, T, Suruga, K, Oshima, Y, Fukiharu, M, Tsukamoto, Y, and Goda, T. Dietary acetic acid reduces serum cholesterol and triacylglycerols in rats fed a cholesterol-rich diet. Br J Nutr. (2006) 95:916–24. doi: 10.1079/BJN20061740
47. Sakakibara, S, Yamauchi, T, Oshima, Y, Tsukamoto, Y, and Kadowaki, T. Acetic acid activates hepatic AMPK and reduces hyperglycemia in diabetic KK-A (y) mice. Biochem Biophys Res Commun. (2006) 344:597–604. doi: 10.1016/j.bbrc.2006.03.176
48. Giraldi-Díaz, MR, Castillo-González, E, De Medina-Salas, L, Velásquez-De la Cruz, R, and Huerta-Silva, HD. Environmental impacts associated with intensive production in pig farms in Mexico through life cycle assessment. Sustain For. (2021) 13:11248. doi: 10.3390/su132011248
49. Calvet, S, Hunt, J, and Misselbrook, TH. Low frequency aeration of pig slurry affects slurry characteristics and emissions of greenhouse gases and ammonia. Biosyst Eng. (2017) 159:121–32. doi: 10.1016/j.biosystemseng.2017.04.011
50. Pirlo, G, Carè, S, Casa, GD, Marchetti, R, Ponzoni, G, Faeti, V, et al. Environmental impact of heavy pig production in a sample of Italian farms. A cradle to farm-gate analysis. Sci Total Environ. (2016) 565:576–85. doi: 10.1016/j.scitotenv.2016.04.174
Keywords: weaning weaned piglets, probiotics, intestinal morphology, short-chain fatty acids, growth performance
Citation: Zhao J, Xie Z, Zheng M, Tang W, Diao H and Yin H (2024) Dietary complex probiotic supplementation changed the composition of intestinal short-chain fatty acids and improved the average daily gain of weaned piglets. Front. Vet. Sci. 11:1424855. doi: 10.3389/fvets.2024.1424855
Edited by:
Matteo Dell’Anno, University of Milan, ItalyReviewed by:
Aimin Wu, Sichuan Agricultural University, ChinaPriscila De Oliveira Moraes, Federal University of Santa Catarina, Brazil
Haihan Zhang, Hunan Agricultural University, China
Eric Auclair, Phileo Lesaffre Animal Care, France
Copyright © 2024 Zhao, Xie, Zheng, Tang, Diao and Yin. This is an open-access article distributed under the terms of the Creative Commons Attribution License (CC BY). The use, distribution or reproduction in other forums is permitted, provided the original author(s) and the copyright owner(s) are credited and that the original publication in this journal is cited, in accordance with accepted academic practice. No use, distribution or reproduction is permitted which does not comply with these terms.
*Correspondence: Heng Yin, yinheng@swust.edu.cn