- 1Institute of Pathology, Wild Animals, Fish and Bees, Veterinary Faculty, University of Ljubljana, Ljubljana, Slovenia
- 2Institute of Microbiology and Parasitology, Veterinary Faculty, University of Ljubljana, Ljubljana, Slovenia
- 3Institute for Biostatistics and Medical Informatics, University of Ljubljana, Ljubljana, Slovenia
- 4Faculty of Mathematics, Natural Sciences and Information Technologies, University of Primorska, Koper, Slovenia
Introduction: Wildlife represents an increasingly important source of pathogens of medical and veterinary importance. Surveillance in wildlife offers an insight on current epidemiological status of selected pathogens and help to prevent spillovers to humans and livestock.
Material and methods: Our study included 312 wild ruminants belonging to five species: Roe deer (n = 134), red deer (n = 113), Alpine chamois (n = 53), European mouflon (n = 10) and Alpine ibex (n = 2). Seven pathogens that may have profound effect on human/livestock health and economic viability of the farms were tested using serological methods.
Results: Antibodies against Toxoplasma gondii, Neospora caninum, Coxiella burnetii, Brucella spp., Chlamydophila abortus, Mycobacterium avium subsp. paratuberculosis (MAP) and Mycobacterium bovis were detected in 34.62% (108/312), 0.96% (3/312), 2.24% (7/312), 0, 0.96% (3/312), 0, 0.64% (2/312) of animals tested, respectively. Because of low prevalences, risk factors were assessed only for T. gondii. Sex (female>male) and species (roe deer>red deer, roe deer>Alpine chamois) were significantly associated with the T. gondii positive outcome, while age was not.
Discussion: Adult males had the lowest T. gondii prevalence which offers future research opportunities. The lower seroprevalence of most investigated pathogens suggests game meat, if properly cooked, as being relatively safe for human consumption. This is the first study investigating the seroprevalence and associated risk factors of selected pathogens in wild ruminants in Slovenia.
1 Introduction
Wild animals act as reservoirs for numerous diseases and can play different roles in the epidemiology of various pathogens. The recent outbreaks of infectious diseases in wildlife worldwide emphasize the importance of ensuring constant surveillance not only of animal pathogens but also of potential zoonotic agents (1). Diseases deriving from wildlife were recognized as a major cause of mortality and loss of biodiversity in animals, but they also have a devastating economic impact (2). Moreover, most emerging infectious diseases in humans are zoonoses or of animal origin (3). The significant impact of infectious diseases in wildlife has also been recognized by the World Organization for Animal Health (WOAH) and the World Health Organization (WHO), which have increased their efforts to establish early detection mechanisms for important zoonotic and conservation pathogens (4). In Slovenia, as in the whole of Europe, a significant increase in the population and geographical distribution of all wild ungulate species has been observed after the Second World War (5, 6). The roe deer (Capreolus capreolus) is the most widespread wild ungulate species in Slovenia, followed by other wild ruminants such as the red deer (Cervus elaphus), the Alpine chamois (Rupicapra rupicapra), the European mouflon (Ovis aries musimon) and the Alpine ibex (Capra ibex) (4). A seropositive outcome to zoonotic pathogens is common among wild ruminants (7–14). The most common pathogens, such as the unicellular parasites Toxoplasma gondii and Neospora caninum, pose a considerable risk as they increase the abortion rate in wild and domestic ruminants. In addition, T. gondii is recognized as a major public health concern (15–19). The prevalence of T. gondii and N. caninum, in wild ruminants worldwide and in Europe is estimated at 23–63.5% and 0.88–23.88%, respectively (20–22). Older and female animals have occasionally been associated with an increased susceptibility to infection with T. gondii (23–26). A Slovenian study on T. gondii in wild boars (Sus scrofa) showed a 62% seroprevalence and a significant risk of infection with increasing age and weight (27). In addition, a seroprevalence of T. gondii and N. caninum in some wild ruminant species was also reported in a Slovenian zoo, which was 13–60% and 0–13%, respectively (28).
Brucella spp., Coxiella burnetii and Chlamydophila abortus are among the significant pathogens causing abortions in ruminants. Currently, Brucella spp. are rarely found in most EU Member States. Nevertheless, these species possess a significant zoonotic risk, particularly B. abortus/melitensis, and continue to be detected in ruminants originating from Greece, Italy, Spain, Portugal, and other Mediterranean areas beyond the borders of the European Union (29). Infections with B. abortus and B. suis have been documented in various wildlife species, while B. melitensis has only rarely been detected in wildlife. In Europe, only isolated cases of infection have been reported, particularly in chamois and Alpine ibex (30–32). In contrast to Brucella spp. most outbreaks of Q fever in humans caused by C. burnetii are associated with domestic and wild ruminants, with seroprevalence ranging from 0 to 29% in wild ruminants (33–35) and up to 75% in domestic ruminants (34, 36–38). This may suggest that wild ruminants could also contribute to the maintenance of C. burnetii in nature (37). Chlamydophila abortus, also a common cause of abortion in sheep and goats (38), has been detected in 0–40% of wild ruminants. Although it is evident that wildlife can serve as reservoir for Chlamydiaceae, the potential significance for human and animal health remains uncertain (39, 40).
Mycobacterium avium subsp. paratuberculosis (MAP) and Mycobacterium bovis are additional pathogens that have a significant impact on the economic sustainability of livestock farming by reducing production levels and fertility rates (41, 42). Slovenia is officially free of bovine tuberculosis (43). Most member states of the European Union have also achieved this status. In our neighboring country Italy, on the other hand, there are still outbreaks of bovine tuberculosis in some provinces (44). Tuberculosis in wildlife can affect humans and a wide range of domestic and wild mammals and is also a significant problem for global conservation efforts. Numerous wildlife species play a role in the persistence and spread of tuberculosis. It is therefore important to systematically monitor both wild and domestic hosts in order to effectively implement prevention and control strategies (45, 46). In a study carried out in Slovenia on 306 apparently healthy wild animals of 13 different species, mycobacteria were isolated in 36 (11.8%) animals of 5 species, namely red deer, roe deer, fallow deer, wild boar and golden jackal (47). In contrast to the official status of bovine tuberculosis, the seroprevalence of MAP in Slovenian dairy farms was reported to be 18.49% at herd level and 3.96% at animal level according to a study by Kusar et al. (48). In addition, the molecular prevalence of MAP is higher on larger farms, as Logar et al. (49) found.
Currently, the number of medium-sized family farms in Slovenia is decreasing, which has an impact on environmental sustainability. It is possible that some of the remaining farms will switch from exclusively keeping animals indoors to more animal-friendly free-range farming with more access to pasture. As wildlife often share pastures with livestock, pathogens can be transmitted in both directions. It is therefore important to continuously monitor both populations to ensure good health and reproductive fitness of the herds as well as safe venison for human consumption.
The aim of the present study was to: 1. Determine seroprevalences of T. gondii and other important pathogens in wild ruminants; 2. Assess species, age, sex, and coinfection as possible risk factors for infection; 3. Evaluate wild ruminants as a potential reservoir for diseases of medical and veterinary importance.
2 Materials and methods
2.1 Materials
Wild ruminant serum samples (n = 312) were collected during the 2017 and 2018 hunting season from apparently healthy free-ranging animals throughout Slovenia (Figure 1). Game wardens and hunters were asked to submit samples from animals shot during the regular annual cull. In Slovenia, the regular annual culling months are from May to December (roe deer), from July to January (red deer) and from August to December (Alpine ibex, chamois and mouflon). The blood was collected shortly after death from the jugular vein or the heart by trained hunters equipped with field sampling kits. Due to autolysis and post-mortem changes, the samples were checked at the Veterinary faculty and haemolysed samples were rejected at the pre-analysis stage. Samples were collected from 134 roe deer, 113 red deer, 53 Alpine chamois, 10 European mouflons and 2 Alpine ibex of various sex and age.
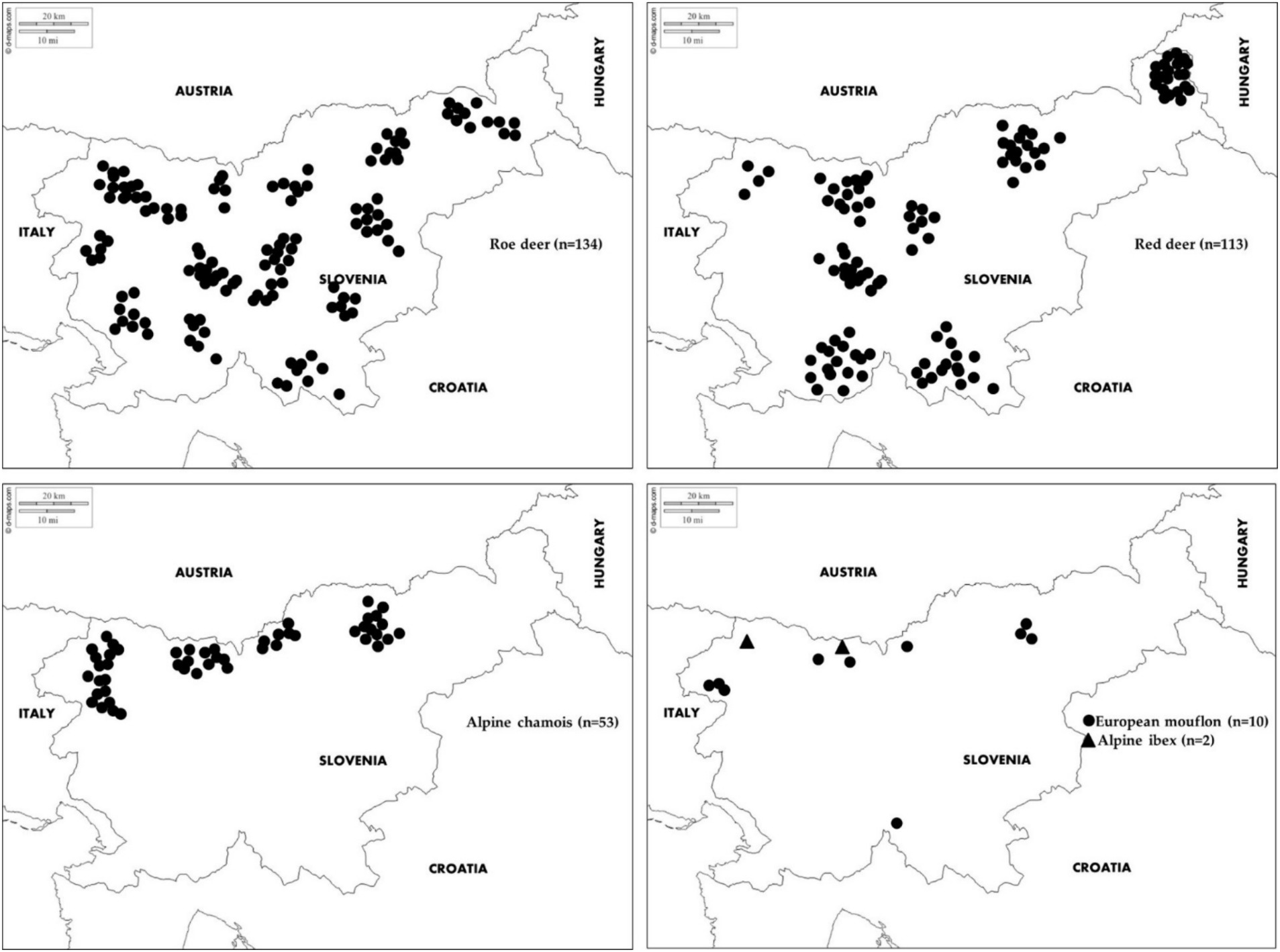
Figure 1. Geographical locations of samples collected from roe deer, red deer, Alpine chamois, mouflon and Alpine ibex in Slovenia.
The samples were transported to the laboratory, where they were centrifuged for 15 min at 4000 rpm. Sera were transferred with serum pipettes into sterile Eppendorf tubes and stored at −20°C until testing. Only animals whose species, sex, and age were recorded by the hunters were used for this study. The age of the animals was estimated after the time of culling by an authorized committee of hunters during the mandatory annual inspection of harvested ungulates. For roe deer, eruption patterns and tooth wear were used to estimate age, while for chamois and mouflon the horn growth ring method was used. Depending on the age of the animals, they were categorized into one of two age groups: young (<1 year old) and adult (>1 year old). Most samples (n = 254) were obtained from animals older than 1 year, namely from 1 to 18 years, with an average age of 2 years.
2.2 Methods
2.2.1 Serological methods
Commercial test kits were used for the detection of antibodies to the selected pathogens. Antibodies against T. gondii were tested using ID Screen® Toxoplasmosis Indirect Multi-species ELISA (ID.vet, Montpellier, France), which detects antibodies against T. gondii P30 antigen in sera, plasma, and meat juices. Antibodies to N. caninum were tested using ID Screen® Neospora caninum Competition kit (ID.vet, Montpellier, France). Sera for brucellosis were screened by rose bengal plate test (RBPT) using Pourquier® Rose Bengal Anti-gen (IDEXX, Montpellier, France). It was followed by indirect-Enzyme Linked Immuno-sorbent Assay (iELISA) using ID Screen® Brucellosis Serum Indirect Multi-species (ID.vet, Montpellier, France). Antibodies against C. abortus were screened using ID Screen® Chlamydophila abortus Indirect Multi-species (ID.vet, Montpellier, France). It is used to detect anti-C. abortus IgG antibodies against a synthetic antigen from a major outer-membrane protein (MOMP), which is specific to C. abortus in serum or plasma. Antibodies against C. burnetii were tested using Q-Fever (Coxiella burnetii) Antibody Test Kit (IDEXX, Liebefeld-Bern, Switzerland). For the detection of specific antibodies against MAP we used IDEXX Paratuberculosis screening Ab test (IDEXX Laboratories, Inc., Westbrook, ME, USA). Antibodies to Mycobacterium tuberculosis complex were screened in MPB83 antigen sandwich ELISA using INGEZIM Tuberculosis DR ELISA kit (Ingenasa, Spain). All tests are suitable for the detection of antibodies in ruminant serum and assays were performed according to the manufacturer’s instructions. Data about sensitivity and specificity for each commercial kit should be available on the manufacturer’s website. Thresholds were determined for each kit following the manufacturer’s instructions. Doubtful results were considered negative.
2.2.2 Statistical analyses
Data were summarized as frequencies (%) with corresponding (exact) binomial 95% confidence intervals.
Differences between the groups (positive vs. negative) for gender (male, female), age (young, adult), gender vs. age (adult female, adult male, young female, young male), and species (roe deer, red deer, alpine chamois, mouflon, alpine ibex) were tested using Fisher’s exact test; for larger than 2 by 2 tables we used the approach proposed by Mehta and Patel (50).
The association between the outcome and a predetermined set of covariates was estimated using multiple binomial penalized regression utilizing Firth’s penalty (51). Results are presented as odds ratios (OR) with 95% confidence intervals (CI). CIs were obtained by profiling the (penalized) likelihood. A p-value lower than 0.05 was considered statistically significant. R statistical language (version 3.6.1) was used for the analyses. R package logistf was used to fit the models (52).
3 Results
The results for all pathogens tested in 312 wild ruminants are shown in Table 1. Toxoplasma gondii proved to be the most prevalent pathogen with 108/312 (34.62%) seropositive animals. Females showed a higher prevalence than males (73/182 vs. 35/130, p = 0.02). In relation to age and sex (p = 0.02), adult and young females had a prevalence of 40.91% (63/154) and 40% (12/30), respectively. Young males had a similar, but lower prevalence of 35.71% (10/28), while adult males had the lowest prevalence of 23% (23/100). The most affected species (p < 0.01) was roe deer with 46.27% (62/134), followed by European mouflon and red deer with 30% (3/10) and 28.32% (32/113), respectively. Alpine chamois had a T. gondii seroprevalence of 20% (11/53), while both Alpine ibexes were seronegative (0/2). Coxiella burnetii antibodies were detected in 7 samples (6 females, 1 male). It was detected in one Alpine ibex (50%, 1/2), one European mouflon (10%, 1/10), in two Alpine chamois (3.77%, 2/53), three roe deer (2.24%, 3/134) and in none of the sampled red deer (0/113). Neospora caninum and C. abortus antibodies were detected in three samples each from roe deer and red deer, while only red deer (1.77%, 2/113) were seropositive for Mycobacterium bovis. Antibodies against Brucella spp. and MAP were not detected in any of the samples.
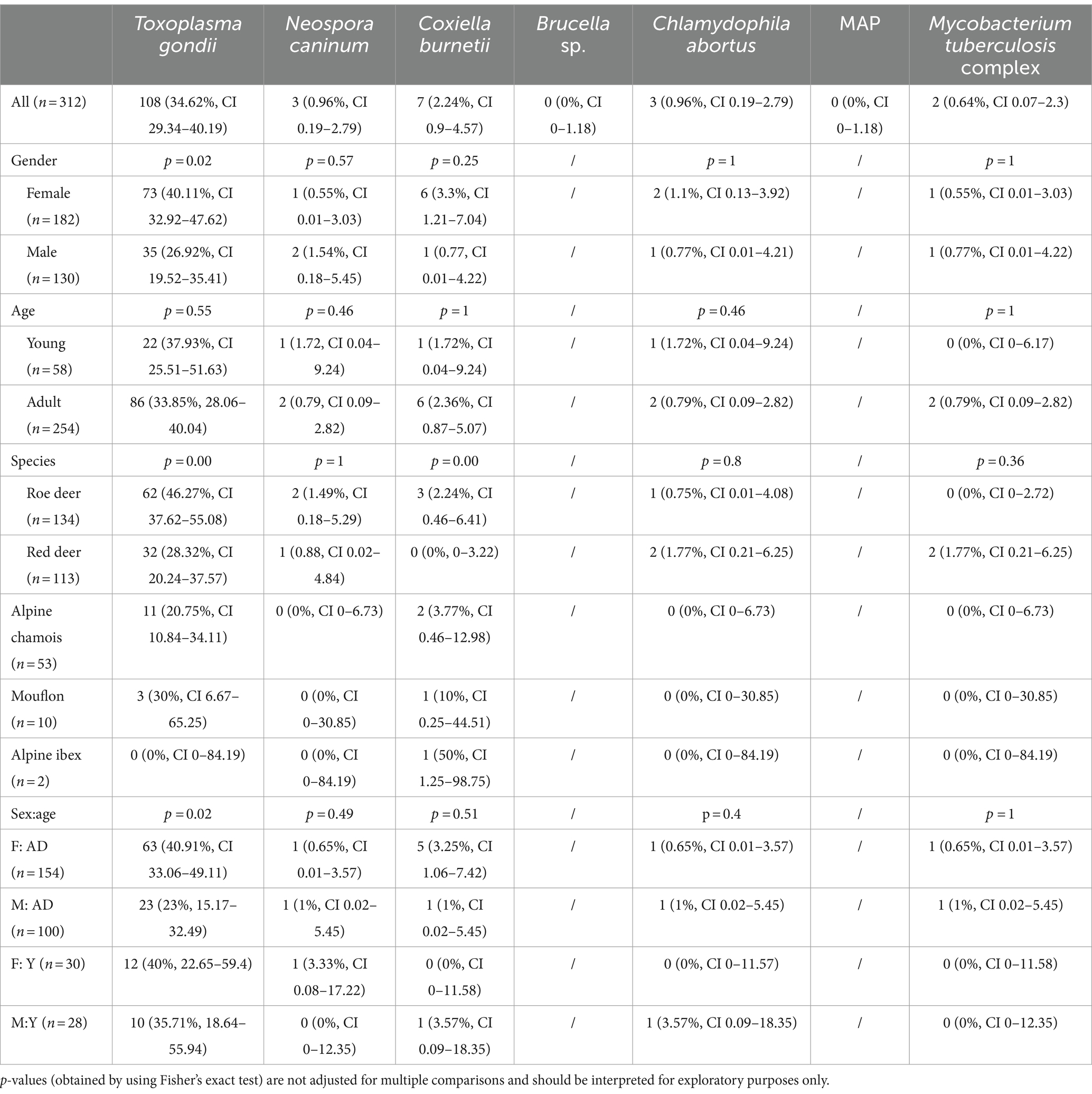
Table 1. Univariate analysis of various diseases in the Slovenian wild ruminant population detected by serology.
As most pathogens had a low prevalence, a multivariate analysis was only performed for T. gondii (Table 2). The results show that female animals have a significantly higher risk of T. gondii infection (p = 0.03), with an odds ratio of 1.77. Adult animals are also more frequently infected, but the effect was not significant (p = 0.20). Among the animal species, red deer and chamois are both less susceptible to infection than roe deer (p < 0.01). No statistical significance was observed between the infection rate of roe deer versus European mouflon (p = 0.28) and Alpine ibex (p = 0.32).
The prevalence of T. gondii in 3 most prevalent species based on animals age and sex showed females animals to be more likely to be positive for T. gondii in roe deer (p = 0.01) and alpine chamois (p = 0.15), although the later was not statistically significant (Table 3). Older animals were more likely (not statistically significant) to be seropositive for T. gondii than younger animals in red deer (p = 0.25) and alpine chamois (p = 0.2), compared to roe deer (p = 0.97) (Table 3).
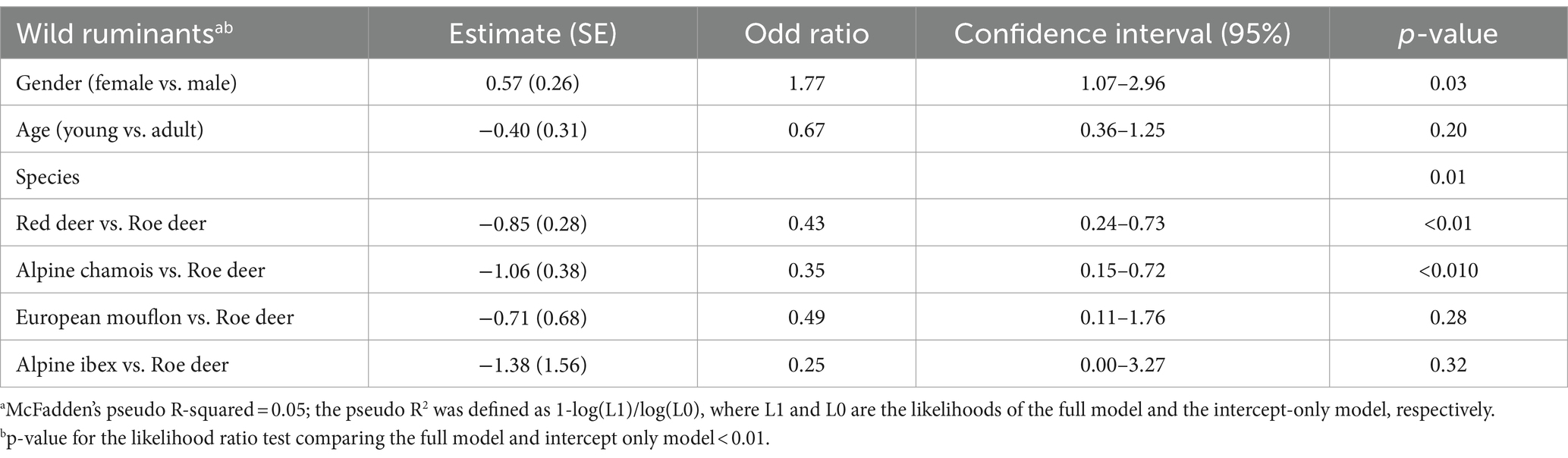
Table 2. Risk factors for Toxoplasma gondii seroprevalence by sex, age, species and comparison between species of wild ruminants.
4 Discussion
Wildlife diseases manifest in different forms and affect a wide range of animal species worldwide. Diseases surveillance is therefore an important tool that provides crucial information on the health status of animal populations and thus ensures the protection of human health. The use of postmortem examinations of wildlife carcasses found in nature or carcasses of animals harvested due to observed poor health offers valuable insight into the general health of a population and the influence of sex, age, and geographical distribution on the cause of death (1, 53). The present study investigated the seroprevalence of seven zoonotic and/or economically important pathogens of apparently healthy animals from five different species of wild ruminants that may have profound effect on human/livestock health and economic viability of the farms. This is the first study investigating the seroprevalence and related risk factors of T. gondii, N. caninum, C. burnetii, Brucella spp., C. abortus, MAP and M. bovis in wild ruminants in Slovenia.
Game meat is a precious source of protein that is increasing in popularity (54). Due to wild ruminant expansion, especially roe deer, the availability of game meat is high (5, 6, 54). However, an apparently healthy animal can harbour many diseases (54) that pose an immediate danger to livestock, hunters or those who handle the carcasses, such as toxoplasmosis, brucellosis, Q fever, chlamydiosis and tuberculosis (27, 55–57). They can also harbour pathogens that can indirectly affect the health of livestock (54), such as neosporosis and paratuberculosis (21, 58). The seroprevalence of many of these diseases is usually much higher in domestic ruminants, than in wild ruminants (59, 60). Ruminants generally exhibit a higher susceptibility to MAP infections compared to other animal species (61). In Slovenian cattle, the reported prevalence of paratuberculosis is about 20% (48), whereas it is 0% in the wild ruminants observed in this study. It appears that a low seroprevalence of MAP in wild ruminants is not uncommon in Europe. Tavernier et al. (10) reported a prevalence of 4.1% in the Belgian roe deer population, while a recent Italian study found a similarly low overall seroprevalence of 5.9% in red deer using different diagnostic tools (62). In addition, Machackova et al. (63) found no significant difference in the seroprevalence reported for MAP (5.3%) between farmed and wild red deer.
In this study we reported a 2.24% seroprevalence against C. burnetii in wild ruminants, which is considerably lower comparing to a Slovenian study in sheep with a prevalence of up to 40.4% (37). However, it should be acknowledged that sheep from the study of Knap et al. (37) most likely represent a much higher seroprevalence than what would be a true seroprevalence in Slovenia as only Q fever positive herds with a history of reproductive failure were tested. Another possible reason for the low prevalence in wild ruminants could be that some of the infected animals do not seroconvert (64). Ticks taken from red deer and wild rabbit in Spain were shown to transmit the disease during blood feeding (65) and a study from Portugal reported a seroprevalence of 1.9% in red deer (66). The reported prevalence is low, but still higher than reported in our study, where all samples off red deer were negative for Q fever antibodies. Following Q fever, chlamydiosis is often considered the second most common abortive pathogen in small domestic ruminants, with prevalence rates ranging from 18.2 to 96.5% (38, 67, 68). Only limited information is available on chlamydiosis in wild ruminants. Serological techniques to identify the specific chlamydial species are lacking, although serological studies conducted in Europe indicate that wild ruminants are a possible but less likely reservoir for chlamydial infections (69, 70). In our study, the presence of C. abortus antibodies was confirmed in only one sample from red deer and two samples from roe deer, which corresponds to 0.96% of the tested wild ruminants. The low prevalence of C.abortus in wild ruminants is therefore similar to the Italian study, in which no seropositive samples were found in red deer (9), while in Belgium Tavernier et al. (10) reported a prevalence of C. abortus of 6.7% in roe deer.
The infective potential of N. caninum extends to various hosts, yet its most pronounced effects are evident in cattle and dogs. There was no evidence for the occurrence of vertical N. caninum infections and their significance in wildlife, and the results of Zanet et al. (71) indicated the possibility of congenital transmission of N. caninum in roe deer, wild boar and red fox. Dubey et al. (72) reported the clinical case of neosporosis in deer, but N. caninum antibodies and/or DNA have been found in several European wild ruminant species, i.e., red deer (7, 8, 73), roe deer (7, 73), fallow deer (74), Alpine chamois (69, 73, 75), European mouflon (76), European bison (Bison bonasus bonasus) (77), Alpine ibex (75) and Spanish ibex (Capra pyrenaica hispanica) (78). The seroprevalence of N. caninum in wild ruminants in Slovenia is 0.96%. The presence of antibodies was confirmed in both autochthonous deer species, roe deer (1.4%) and red deer (0.8%). Currently, no published data are available on livestock in Slovenia. This finding emphasizes the need for further research to establish the role of N. caninum, as it is widely believed that intensive livestock farming creates the conditions for the occurrence and spread of this unicellular parasite (59).
The only pathogen that is hardy affected by human land management is T. gondii. It is possibly the most common parasite within the domestic and sylvatic cycle (79). Domestic and wild ruminants can serve as a source of infection for animals and humans through the consumption of meat. The primary route of infection for herbivores is the ingestion of oocysts, which are shed into the environment by their definitive hosts, which in Central Europe are the domestic cat (Felis catus), the wild cat (Felis silvestris) and the Eurasian lynx (Lynx lynx) (11, 12, 14, 80). Toxoplasma gondii is also the pathogen with the highest prevalence in wild ruminants in our study. The seroprevalence in our study was 46.2% in roe deer and 28.3% in red deer, which is above the European average for both species of 29 and 15%, respectively (11). A high seroprevalence of T. gondii was also found in the Slovenian wild boar population at 62% (27). An overall high seroprevalence in Slovenian wild animal population would need further research as it is surprising. Slovenian climate, which is Mediterranean/Continental/pre-Alpine might allow for T. gondii to thrive (27). Risk factors associated with higher T. gondii exposure are usually species, sex, age, and geographical distribution (23, 26, 81–83). Roe deer are more likely to be T. gondii positive than red deer and chamois. These results confirm the findings of researchers in Denmark and Norway, where roe deer were more likely to be T. gondii positive than fallow deer (83), moose, red deer or reindeer (23). The most consistent risk factor associated with increased T. gondii seroprevalence is usually the increasing age of the animals (23, 24, 84–88) as antibodies can persist for several years and the likelihood of seroconversion increases over an animal’s lifetime (80). However, our study did not show any significance due to the age of the animal when all species were included in the analysis. Further analysis of the most representative species (roe deer, red deer, alpine chamois) in our study, showed that younger animals were less likely (although not reaching statistical significance) to be positive than older animals for red deer and alpine chamois, while for roe deer the age seemed to be unimportant. On the other hand, the sex of the animal is not consistently associated with a higher prevalence of T. gondii. Several studies found no correlation (23, 84, 86, 88), including our study in wild boar population (27). Only a few studies found that females are more frequently infected than males (24, 26, 89), while one study found that a male population of white-tailed deer was significantly more seropositive than females (24). In our study, females were significantly more seropositive for T. gondii than males (p = 0.03). When analysing the relationship between age and gender, it was found that adult and young females had an almost identical prevalence rate (40.91% vs. 40%), young males had a similar but lower prevalence of 35.71%. The difference was significant in adult males, who had a prevalence of only 23% (p = 0.02). The lower prevalence in adult males does not fit with the theory of increased exposure over time theory. Nor does it fit with the same seroprevalence in young and adult females. It could be, that infection with T. gondii has no effect on the survival of females, but it does on the survival of males. A more detailed species specific analysis in our study revealed a similar effect of gender for roe deer and alpine chamois (more positive between females than males), but no association was observed for red deer. Toxoplasma gondii has been found to affect the sex ratio in humans and mice, with more males being born than females (90, 91). Studies in mice showed that males infected with T. gondii had decreased levels of hormones and other parameters that impaired the animal’s general reproductive capacity (92). Several studies have also confirmed that wildlife generally found at lower altitudes and below the tree line such as roe deer and red deer, have a high seroprevalence of T. gondii. A lower prevalence was found in species that favour rocky terrain at moderately high altitudes such as chamois (11%) and mouflon (3%) as well as alpine ibex (0%). This finding confirms the significant effect of altitude as a protective factor due probably to less contact with oocysts in areas of high altitude, as animals from higher altitudes were less likely to be seropositive (13, 80, 93). Scherrer et al. (80) reported a high seroprevalence of 82% in Eurasian lynx, one of the potential definitive hosts of T. gondii in Europe. This may indicate a higher prevalence in roe deer and chamois, the main pray animals of lynx. In this study, we were unable to evaluate coinfection as a risk factor for T. gondii infection, as the prevalence of other pathogens was low in the animals examined. This indicates that apart from T. gondii, all other investigated pathogens are not of major importance in the sylvatic cycle in Slovenia and thus do not represent an important source of disease for humans or animals. Regardless, precautions with raw and undercooked meat, including game meat, are still needed when handling wildlife and their carcasses.
5 Conclusion
In conclusion, the health status of wild ruminants remains largely unknown, but studies, including ours, suggest that some pathogens are less prevalent than in domestic ruminants. Surveillance of infective agents in wildlife provides insight into the current epidemiological situation of selected pathogens. It can help prevent spillovers from wildlife to humans and livestock and detect spillbacks from humans and livestock to wildlife. Toxoplasma gondii was the most predominant pathogen investigated with a significantly higher seroprevalence in roe deer and females. The lower prevalence in adult males provides an interesting research window for future studies. The results of our study also emphasize the potential risk for hunters, veterinary pathologist, farmers and general public associated with the consumption of venison and handling of carcasses from wild ruminants. Personal protective measures should be taken and proper food preparation are suggested as a preventive measure, no less important than when handling raw meat from domestic animals.
Data availability statement
The raw data supporting the conclusions of this article will be made available by the authors, without undue reservation available upon request.
Ethics statement
Ethical approval was not required for the study involving animals in accordance with the local legislation and institutional requirements because the approval of the Ethics Committee/Welfare Authority was not required as all samples were taken post-mortem.
Author contributions
DŽV: Conceptualization, Data curation, Investigation, Visualization, Writing – original draft, Writing – review & editing. BK: Conceptualization, Data curation, Formal analysis, Investigation, Methodology, Validation, Writing – review & editing. RB: Data curation, Formal analysis, Methodology, Software, Writing – review & editing. GV: Conceptualization, Data curation, Funding acquisition, Investigation, Project administration, Resources, Supervision, Visualization, Writing – review & editing. PB: Conceptualization, Data curation, Funding acquisition, Visualization, Writing – original draft, Writing – review & editing.
Funding
The author(s) declare that financial support was received for the research, authorship, and/or publication of this article. This research was funded by the Slovenian Research Agency (research core funding No. P4-0092 and P3-0154) and by the Administration of the Republic of Slovenia for Food Safety, Veterinary Service and Plant Protection.
Acknowledgments
The authors thank the Slovenian hunters and the Slovenian Hunting Association for their valuable help in collecting the samples. We thank Alenka Magdalena Usenik for her help in processing the samples.
Conflict of interest
The authors declare that the research was conducted in the absence of any commercial or financial relationships that could be construed as a potential conflict of interest.
Publisher’s note
All claims expressed in this article are solely those of the authors and do not necessarily represent those of their affiliated organizations, or those of the publisher, the editors and the reviewers. Any product that may be evaluated in this article, or claim that may be made by its manufacturer, is not guaranteed or endorsed by the publisher.
References
1. Žele Vengušt, D, Kuhar, U, Jerina, K, and Vengušt, G. Twenty years of passive disease surveillance of roe deer (Capreolus capreolus) in Slovenia. Animals. (2021) 11:407. doi: 10.3390/ani11020407
2. Martin, C, Pastoret, PP, Brochier, B, Humblet, MF, and Saegerman, C. A survey of the transmission of infectious diseases/infections between wild and domestic ungulates in Europe. Vet Res. (2011) 42:70. doi: 10.1186/1297-9716-42-70
3. González-Barrio, D, and Ruiz-Fons, F. Coxiella burnetii in wild mammals: a systematic review. Transbound Emerg Dis. (2019) 66:662–71. doi: 10.1111/tbed.13085
4. World Organisation for Animal Health . Training manual on surveillance and international reporting of diseases in wild animals second cycle. Workshop for OIE National Focal Points for wildlife. Paris: OIE (2015).
5. Andersen, R, Putman, RJ, and Apollonio, M. European ungulates and their management in the 21st century. 1st ed. Cambridge, UK: Cambridge University Press (2009).
6. Jerina, K, Stergar, M, Jelenko, I, and Pokorny, B. Spatial distibution, fitness, and population dynamics of ungulates in Slovenia: Studies on the effects of spatially explicite habitat and species-specific factors and predicting future trends. Ljubljana, Slovenia: Biotechnical Faculty of the University of Ljubljana (2010).
7. Almería, S, Vidal, D, Ferrer, D, Pabón, M, Fernández-de-Mera, MIG, Ruiz-Fons, F, et al. Seroprevalence of Neospora caninum in non-carnivorous wildlife from Spain. Vet Parasitol. (2007) 143:21–8. doi: 10.1016/j.vetpar.2006.07.027
8. Goździk, K, Jakubek, EB, Björkman, C, Bień, J, Moskwa, B, and Cabaj, W. Seroprevalence of Neospora caninum in free living and farmed red deer (Cervus elaphus) in Poland. Pol J Vet Sci. (2010) 13:117–20.
9. Di Francesco, A, Donati, M, Nicoloso, S, Orlandi, L, Baldelli, R, Salvatore, D, et al. Chlamydiosis: seroepidemiologic survey in a red deer (Cervus elaphus) population in Italy. J Wildl Dis. (2012) 48:488–91. doi: 10.7589/0090-3558-48.2.488
10. Tavernier, P, Sys, SU, De Clercq, K, De Leeuw, I, Caij, AB, De Baere, M, et al. Serologic screening for 13 infectious agents in roe deer (Capreolus capreolus) in Flanders. Infect Ecol Epidemiol. (2015) 5:29862. doi: 10.3402/iee.v5.29862
11. Fanelli, A, Battisti, E, Zanet, S, Trisciuoglio, A, and Ferroglio, E. A systematic review and meta-analysis of Toxoplasma gondii in roe deer (Capreolus capreolus) and red deer (Cervus elaphus) in Europe. Zoonoses Public Health. (2021) 68:182–93. doi: 10.1111/zph.12780
12. Dubey, JP, Murata, FHA, Cerqueira-Cézar, CK, and Kwok, OCK. Epidemiologic and public health significance of Toxoplasma gondii infections in venison: 2009–2020. J Parasitol. (2021) 107:309–19. doi: 10.1645/20-162
13. Basso, W, Holenweger, F, Schares, G, Müller, N, Campero, LM, Ardüser, F, et al. Toxoplasma gondii and Neospora caninum infections in sheep and goats in Switzerland: seroprevalence and occurrence in aborted foetuses. Food Waterborne Parasitol. (2022) 28:e00176. doi: 10.1016/j.fawpar.2022.e00176
14. Conrady, CD, Besirli, CG, Baumal, CR, Kovach, JL, Etzel, JD, Tsui, JC, et al. Ocular toxoplasmosis after exposure to wild game. Ocul Immunol Inflamm. (2022) 30:527–32. doi: 10.1080/09273948.2020.1854316
15. Lindsay, DS, and Dubey, JP. Neosporosis, toxoplasmosis, and sarcocystosis in ruminants: an update. Vet Clin North Am Food Anim Pract. (2020) 36:205–22. doi: 10.1016/j.cvfa.2019.11.004
16. Barber, JS, Payne-Johnson, CE, and Trees, AJ. Distribution of Neospora caninum within the central nervous system and other tissues of six dogs with clinical neosporosis. J Small Anim Pract. (1996) 37:568–74. doi: 10.1111/j.1748-5827.1996.tb02332.x
17. Nayeri, T, Moosazadeh, M, Sarvi, S, and Daryan, IA. Neospora caninum infection in aborting bovines and lost fetuses: a systematic review and meta-analysis. PLoS One. (2022) 17:e0268903. doi: 10.1371/journal.pone.0268903
18. Nayeri, T, Sarvi, S, Moosazadeh, M, and Daryani, A. The global prevalence of Neospora caninum infection in sheep and goats that had an abortion and aborted fetuses: a systematic review and meta-analysis. Front Vet Sci. (2022) 9:870904. doi: 10.3389/fvets.2022.870904
19. Soler, JP, Moré, G, Urtizbiría, F, Hecker, YP, Cirone, KM, Scioli, MV, et al. Epidemic abortions due to Neospora caninum infection in farmed red deer (Cervus elaphus). Parasitol Res. (2022) 121:1475–85. doi: 10.1007/s00436-022-07488-6
20. San Miguel, JM, Gutiérrez-Expósito, D, Aguado-Martínez, A, González-Zotes, E, Pereira-Bueno, J, Gómez-Bautista, M, et al. Effect of different ecosystems and management practices on Toxoplasma gondii and Neospora caninum infections in wild ruminants in Spain. J Wildl Dis. (2016) 52:293–300. doi: 10.7589/2015-07-176
21. Rocchigiani, G, Nardoni, S, D'Ascenzi, C, Nicoloso, S, Picciolli, F, Papini, RA, et al. Seroprevalence of Toxoplasma gondii and Neospora caninum in red deer from Central Italy. Ann Agric Environ Med. (2016) 23:699–701. doi: 10.5604/12321966.1226870
22. Zeng, A, Gong, QL, Wang, Q, Wang, CR, and Zhang, XX. The global seroprevalence of Toxoplasma gondii in deer from 1978 to 2019: a systematic review and meta-analysis. Acta Trop. (2020) 208:105529. doi: 10.1016/j.actatropica.2020.105529
23. Vikøren, T, Tharaldsen, J, Fredriksen, B, and Handeland, K. Prevalence of Toxoplasma gondii antibodies in wild red deer, roe deer, moose, and reindeer from Norway. Vet Parasitol. (2004) 120:159–69. doi: 10.1016/j.vetpar.2003.12.015
24. Jokelainen, P, Näreaho, A, Knaapi, S, Oksanen, A, Rikula, U, and Sukura, A. Toxoplasma gondii in wild cervids and sheep in Finland: north-south gradient in seroprevalence. Vet Parasitol. (2010) 171:331–6. doi: 10.1016/j.vetpar.2010.04.008
25. Candela, MG, Serrano, E, Sevila, J, León, L, Caro, MR, and Verheyden, H. Pathogens of zoonotic and biological importance in roe deer (Capreolus capreolus): Seroprevalence in an agro-system population in France. Res Vet Sci. (2014) 96:254–9. doi: 10.1016/j.rvsc.2014.02.003
26. Remes, N, Kärssin, A, Must, K, Tagel, M, Lassen, B, and Jokelainen, P. Toxoplasma gondii seroprevalence in free-ranging moose (Alces alces) hunted for human consumption in Estonia: indicator host species for environmental Toxoplasma gondii oocyst contamination. Vet Parasitol Reg Stud Reports. (2018) 11:6–11. doi: 10.1016/j.vprsr.2017.11.001
27. Bandelj, P, Vengušt, DŽ, Blagus, R, Vergles Rataj, A, and Krt, B. High Seroprevalence of Toxoplasma gondii in Slovenian wild boars (Sus scrofa). Animals. (2021) 11:3139. doi: 10.3390/ani11113139
28. Kvapil, P, Račnik, J, Kastelic, M, Marková, J, Murat, JB, Kobédová, K, et al. Biosurveillance of selected pathogens with zoonotic potential in a zoo. Pathogens. (2021) 10:428. doi: 10.3390/pathogens10040428
29. European Centre for Disease Prevention and Control . Brucellosis EDC In: Annual epidemiological report for 2021. Stockholm (2023). Available at: https://www.ecdc.europa.eu/sites/default/files/documents/AER-Brucellosis-2021.pdf
30. Ferroglio, E, Tolari, F, Bollo, E, and Bassano, B. Isolation of Brucella melitensis from alpine ibex. J Wildl Dis. (1998) 34:400–2. doi: 10.7589/0090-3558-34.2.400
31. Godfroid, J, Garin-Bastuji, B, Saegerman, C, and Blasco, JM. Brucellosis in terrestrial wildlife. Rev Sci Tech. (2013) 32:27–42. doi: 10.20506/rst.32.1.2180
32. Garin-Bastuji, B, Hars, J, Drapeau, A, Cherfa, MA, Game, Y, Le Horgne, JM, et al. Reemergence of Brucella melitensis in wildlife. France Emerg Infect Dis. (2014) 20:1570–1. doi: 10.3201/eid2009.131517
33. Hubálek, Z, Juricová, Z, Svobodová, S, and Halouzka, J. A serologic survey for some bacterial and viral zoonoses in game animals in the Czech Republic. J Wildl Dis. (1993) 29:604–7. doi: 10.7589/0090-3558-29.4.604
34. Ruiz-Fons, F, Rodríguez, O, Torina, A, Naranjo, V, Gortázar, C, and de la Fuente, J. Prevalence of Coxiella burnetti infection in wild and farmed ungulates. Vet Microbiol. (2008) 126:282–6. doi: 10.1016/j.vetmic.2007.06.020
35. Fernández-Aguilar, X, Cabezón, Ó, Colom-Cadena, A, Lavín, S, and López-Olvera, JR. Serological survey of Coxiella burnetii at the wildlife-livestock interface in the eastern Pyrenees, Spain. Acta Vet Scand. (2016) 58:26. doi: 10.1186/s13028-016-0209-4
36. Guatteo, R, Seegers, H, Taurel, AF, Joly, A, and Beaudeau, F. Prevalence of Coxiella burnetii infection in domestic ruminants: a critical review. Vet Microbiol. (2011) 149:1–16. doi: 10.1016/j.vetmic.2010.10.007
37. Knap, N, Žele, D, Glinšek Biškup, U, Avšič-Županc, T, and Vengušt, G. The prevalence of Coxiella burnetii in ticks and animals in Slovenia. BMC Vet Res. (2019) 15:368. doi: 10.1186/s12917-019-2130-3
38. Ramo, MLA, Benito, AA, Quílez, J, Monteagudo, LV, Baselga, C, and Tejedor, MT. Coxiella burnetii and co-infections with other major pathogens causing abortion in small ruminant flocks in the Iberian Peninsula. Animals. (2022) 12:3454. doi: 10.3390/ani12243454
39. Salinas, J, Caro, MR, Vicente, J, Cuello, F, Reyes-Garcia, AR, Buendía, AJ, et al. High prevalence of antibodies against Chlamydiaceae and Chlamydophila abortus in wild ungulates using two "in house" blocking-ELISA tests. Vet Microbiol. (2009) 135:46–53. doi: 10.1016/j.vetmic.2008.10.001
40. Kreizinger, Z, Szeredi, L, Bacsadi, Á, Nemes, C, Sugár, L, Varga, T, et al. Occurrence of Coxiella burnetii and Chlamydiales species in abortions of domestic ruminants and in wild ruminants in Hungary. Central Europe J Vet Diagn Invest. (2015) 27:206–10. doi: 10.1177/1040638714563566
41. Ozsvari, L, Harnos, A, Lang, Z, Monostori, A, Strain, S, and Fodor, I. The impact of paratuberculosis on milk production, fertility, and culling in large commercial hungarian dairy herds. Front Vet Sci. (2020) 7:565324. doi: 10.3389/fvets.2020.565324
42. Borham, M, Oreiby, A, El-Gedawy, A, Hegazy, Y, Khalifa, HO, Al-Gaabary, M, et al. Review on bovine tuberculosis: an emerging disease associated with multidrug-resistant mycobacterium species. Pathogens. (2022) 11:715. doi: 10.3390/pathogens11070715
43. European commission. Bovine and swine diseases . Situation (2019). Available at: https://food.ec.europa.eu/system/files/2020-12/la_annual-situation_2019.pdf (Accessed March 14, 2024).
44. Bovine TBC Italy , (2022). Available at: https://hadea.ec.europa.eu/system/files/2022-03/Bovine%20tuberculosis_Italy.pdf (Accessed October 23, 2023).
45. Caminiti, E. (2019). The socio-economic costs of bovine tuberculosis. Available at: https://oiebulletin.com/?panorama=3-05-tb-costs-en. (Accessed January 22, 2024).
46. Thomas, J, Balseiro, A, Gortázar, C, and Risalde, MA. Diagnosis of tuberculosis in wildlife: a systematic review. Vet Res. (2021) 52:31. doi: 10.1186/s13567-020-00881-y
47. Pate, M, Zajc, U, Kušar, D, Žele, D, Vengušt, G, Pirš, T, et al. Mycobacterium spp. in wild game in Slovenia. Vet J. (2016) 208:93–5. doi: 10.1016/j.tvjl.2015.10.004
48. Kušar, D, Ocepek, M, Logar, K, Pate, M, and Krt, B. Seroprevalence of cattle paratuberculosis in Slovenia in 2008 and a comparison of data from current and previous studies. Slo Vet Res. (2011) 48:39–44.
49. Logar, K, Kopinč, R, Bandelj, P, Starič, J, Lapanje, A, and Ocepek, M. Evaluation of combined high-efficiency DNA extraction and real-time PCR for detection of Mycobacterium avium subsp. paratuberculosis in subclinically infected dairy cattle: comparison with faecal culture, milk real-time PCR and milk ELISA. BMC Vet Res. (2012) 8:49. doi: 10.1186/1746-6148-8-49
50. Mehta, CR, and Patel, NR. A network algorithm for performing Fisher's exact test in r x c contingency tables. JASA. (1983) 78:427–34. doi: 10.1080/01621459.1983.10477989
51. Firth, D . Bias reduction of maximum likelihood estimates. Biometrika. (1993) 80:27–38. doi: 10.1093/biomet/80.1.27
52. Heinze, G., Ploner, M., Dunkler, D., Southworth, H., Jiricka, L., and Steiner, G. Logistf: Firth's bias-reduced logistic regression. R package version 1260. (2023). Available at: https://cran.r-project.org/web/packages/logistf/index.html (Accessed March 26, 2024).
53. Pewsner, M, Origgi, FC, Frey, J, and Ryser-Degiorgis, MP. Assessing fifty years of general health surveillance of roe deer in Switzerland: a retrospective analysis of necropsy reports. PLoS One. (2017) 12:e0170338. doi: 10.1371/journal.pone.0170338
54. Needham, T, Bureš, D, Černý, J, and Hoffman, LC. Overview of game meat utilization challenges and opportunities: a European perspective. Meat Sci. (2023) 204:109284. doi: 10.1016/j.meatsci.2023.109284
55. Hedman, HD, Varga, C, Duquette, J, Novakofski, J, and Mateus-Pinilla, NE. Food safety considerations related to the consumption and handling of game meat in North America. Vet Sci. (2020) 7:188. doi: 10.3390/vetsci7040188
56. Clausi, MT, Ciambrone, L, Zanoni, M, Costanzo, N, Pacciarini, M, and Casalinuovo, F. Evaluation of the presence and viability of Mycobacterium bovis in wild boar meat and meat-based preparations. Food Secur. (2021) 10:2410. doi: 10.3390/foods10102410
57. Jori, F, Hernandez-Jover, M, Magouras, I, Dürr, S, and Brookes, VJ. Wildlife-livestock interactions in animal production systems: what are the biosecurity and health implications? Anim Front. (2021) 11:8–19. doi: 10.1093/af/vfab045
58. Trimmel, NE, and Walzer, C. Infectious wildlife diseases in Austria-a literature review from 1980 until 2017. Front Vet Sci. (2020) 7:3. doi: 10.3389/fvets.2020.00003
59. Coker, R, Rushton, J, Mounier-Jack, S, Karimuribo, E, Lutumba, P, Kambarage, D, et al. Towards a conceptual framework to support one-health research for policy on emerging zoonoses. Lancet Infect Dis. (2011) 11:326–31. doi: 10.1016/S1473-3099(10)70312-1
60. Espinosa, R, Tago, D, and Treich, N. Infectious diseases and meat production. Environ Resour Econ. (2020) 76:1019–44. doi: 10.1007/s10640-020-00484-3
61. Carta, T, Álvarez, J, Pérez de la Lastra, JM, and Gortázar, C. Wildlife and paratuberculosis: a review. Res Vet Sci. (2013) 94:191–7. doi: 10.1016/j.rvsc.2012.11.002
62. Filippi, A, Garbarino, C, Nava, M, Russo, S, Soares, F, Joel, F, et al. Active surveillance of paratuberculosis in alpine-dwelling red deer (Cervus elaphus). Front Vet Sci. (2024) 11:1303096. doi: 10.3389/fvets.2024.1303096
63. Machackova, M, Svastova, P, Lamka, J, Parmova, I, Liska, V, Smolik, J, et al. Paratuberculosis in farmed and free-living wild ruminants in the Czech Republic (1999–2001). Vet Microbiol. (2004) 101:225–34. doi: 10.1016/j.vetmic.2004.04.001
64. González-Barrio, D, Fernández-de-Mera, IG, Ortiz, JA, Queirós, J, and Ruiz-Fons, F. Long-term dynamics of Coxiella burnetii in farmed red deer (Cervus elaphus). Front Vet Sci. (2015) 2:74. doi: 10.3389/fvets.2015.00074
65. González, J, González, MG, Valcárcel, F, Sánchez, M, Martín-Hernández, R, Tercero, JM, et al. Transstadial transmission from nymph to adult of Coxiella burnetii by naturally infected Hyalomma lusitanicum. Pathogens. (2020) 9:884. doi: 10.3390/pathogens9110884
66. Pires, H, Cardoso, L, Lopes, AP, Fontes, MDC, Matos, M, Pintado, C, et al. Seropositivity for Coxiella burnetii in wild boar (Sus scrofa) and red deer (Cervus elaphus) in Portugal. Pathogens. (2023) 12:421. doi: 10.3390/pathogens12030421
67. Mainar-Jaime, R, Cruz, C, and Vázquez-Boland, J. Epidemiologic study of chlamydial infection in sheep farms in Madrid, Spain. Small Rumin Res. (1998) 28:131–8. doi: 10.1016/S0921-4488(97)00084-9
68. Díaz, JM, Fernández, G, Prieto, A, Valverde, S, Lago, N, Díaz, P, et al. Epidemiology of reproductive pathogens in semi-intensive lamb-producing flocks in north-West Spain: a comparative serological study. Vet J. (2014) 200:335–8. doi: 10.1016/j.tvjl.2014.02.022
69. Gaffuri, A, Giacometti, M, Tranquillo, VM, Magnino, S, Cordioli, P, and Lanfranchi, P. Serosurvey of roe deer, chamois and domestic sheep in the central Italian Alps. J Wildl Dis. (2006) 42:685–90. doi: 10.7589/0090-3558-42.3.685
70. Di Francesco, A, Donati, M, Laroucau, K, Balboni, A, Galuppi, R, Merialdi, G, et al. Chlamydiae in corvids. Vet Rec. (2015) 177:466. doi: 10.1136/vr.103218
71. Zanet, S, Poncina, M, and Ferroglio, E. Congenital transmission of Neospora caninum in wild ungulates and foxes. Front Vet Sci. (2023) 10:1109986. doi: 10.3389/fvets.2023.1109986
72. Dubey, JP, Rigoulet, J, Lagourette, P, George, C, Longeart, L, and LeNet, JL. Fatal transplacental neosporosis in a deer (Cervus eldi siamensis). J Parasitol. (1996) 82:338–9. doi: 10.2307/3284175
73. Ferroglio, E, and Rossi, L. Prevalence of Neospora caninum antibodies in wild ruminants from the Italian Alps. Vet Rec. (2001) 148:754–5. doi: 10.1136/vr.148.24.754
74. Soldati, S, Kiupel, M, Wise, A, Maes, R, Botteron, C, and Robert, N. Meningoencephalomyelitis caused by Neospora caninum in a juvenile fallow deer (Dama dama). J Vet Med A Physiol Pathol Clin Med. (2004) 51:280–3. doi: 10.1111/j.1439-0442.2004.00646.x
75. Bregoli, M, Gioia, C, Stefano, N, Mariapia, C, and Claudio, P. Serological survey of Neospora caninum in free-ranging wild ruminants. Vet Arhiv. (2006) 76:S111–5.
76. Bartova, E, Sedlak, K, and Literak, I. Prevalence of Toxoplasma gondii and Neospora caninum antibodies in wild boars in the Czech Republic. Vet Parasitol. (2006) 142:150–3. doi: 10.1016/j.vetpar.2006.06.022
77. Bien, J, Moskwa, B, and Cabaj, W. In vitro isolation and identification of the first Neospora caninum isolate from European bison (Bison bonasus bonasus L.). Vet Parasitol. (2010) 173:200–5. doi: 10.1016/j.vetpar.2010.06.038
78. García-Bocanegra, I, Cabezón, O, Pabón, M, Gómez-Guillamón, F, Arenas, A, Alcaide, E, et al. Prevalence of Toxoplasma gondii and Neospora caninum antibodies in Spanish ibex (Capra pyrenaica hispanica). Vet J. (2012) 191:257–60. doi: 10.1016/j.tvjl.2010.11.011
79. Tong, WH, Pavey, C, O'Handley, R, and Vyas, A. Behavioral biology of Toxoplasma gondii infection. Parasit Vectors. (2021) 14:77. doi: 10.1186/s13071-020-04528-x
80. Scherrer, P, Ryser-Degiorgis, MP, Marti, IA, Borel, S, Frey, CF, Mueller, N, et al. Exploring the epidemiological role of the Eurasian lynx (Lynx lynx) in the life cycle of Toxoplasma gondii. Int J Parasitol Parasites Wildl. (2023) 21:1–10. doi: 10.1016/j.ijppaw.2023.03.005
81. Gauss, CB, Dubey, JP, Vidal, D, Cabezón, O, Ruiz-Fons, F, Vicente, J, et al. Prevalence of Toxoplasma gondii antibodies in red deer (Cervus elaphus) and other wild ruminants from Spain. Vet Parasitol. (2006) 136:193–200. doi: 10.1016/j.vetpar.2005.11.013
82. Hoshina, T, Fukumoto, S, Aonuma, H, Saiki, E, Hori, S, and Kanuka, H. Seroprevalence of Toxoplasma gondii in wild sika deer in Japan. Parasitol Int. (2019) 71:76–9. doi: 10.1016/j.parint.2019.03.016
83. Stensgaard, AS, Sengupta, ME, Chriel, M, Nielsen, ST, and Petersen, HH. Seroprevalence and risk factors of Toxoplasma gondii infection in wild cervids in Denmark. Int J Parasitol Parasites Wildl. (2022) 17:288–94. doi: 10.1016/j.ijppaw.2022.03.010
84. Bier, NS, Stollberg, K, Mayer-Scholl, A, Johne, A, Nöckler, K, and Richter, M. Seroprevalence of Toxoplasma gondii in wild boar and deer in Brandenburg. Germany Zoonoses Public Health. (2020) 67:601–6. doi: 10.1111/zph.12702
85. Gamarra, JA, Cabezón, O, Pabón, M, Arnal, MC, Luco, DF, Dubey, JP, et al. Prevalence of anti-bodies against Toxoplasma gondii in roe deer from Spain. Vet Parasitol. (2008) 153:152–6. doi: 10.1016/j.vetpar.2008.01.028
86. Malmsten, J, Jakubek, EB, and Björkman, C. Prevalence of antibodies against Toxoplasma gondii and Neospora caninum in moose (Alces alces) and roe deer (Capreolus capreolus) in Sweden. Vet Parasitol. (2011) 177:275–80. doi: 10.1016/j.vetpar.2010.11.051
87. Almería, S, Cabezón, O, Paniagua, J, Cano-Terriza, D, Jiménez-Ruiz, S, Arenas-Montes, A, et al. Toxoplasma gondii in sympatric domestic and wild ungulates in the Mediterranean ecosystem. Parasitol Res. (2018) 117:665–71. doi: 10.1007/s00436-017-5705-6
88. Heddergott, M, Steinbach, P, Pohl, D, and Frantz, AC. First report on the sero-epidemiology of Toxoplasma gondii infection in German roe deer (Capreolus capreolus). Parasite. (2018) 25:52. doi: 10.1051/parasite/2018052
89. Halová, D, Mulcahy, G, Rafter, P, Turčeková, L, Grant, T, and de Waal, T. Toxoplasma gondii in Ireland: seroprevalence and novel molecular detection method in sheep, pigs, deer and chickens. Zoonoses Public Health. (2013) 60:168–73. doi: 10.1111/j.1863-2378.2012.01514.x
90. Kanková, S, Sulc, J, Nouzová, K, Fajfrlík, K, Frynta, D, and Flegr, J. Women infected with parasite toxoplasma have more sons. Naturwissenschaften. (2007) 94:122–7. doi: 10.1007/s00114-006-0166-2
91. Kanková, S, Kodym, P, Frynta, D, Vavrinová, R, Kubena, A, and Flegr, J. Influence of latent toxoplasmosis on the secondary sex ratio in mice. Parasitology. (2007) 134:1709–17. doi: 10.1017/S0031182007003253
92. Dvorakova-Hortova, K, Sidlova, A, Ded, L, Hladovcova, D, Vieweg, M, Weidner, W, et al. Toxoplasma gondii decreases the reproductive fitness in mice. PLoS One. (2014) 9:e96770. doi: 10.1371/journal.pone.0096770
Keywords: wild ruminants, roe deer, red deer, serology, infectious diseases, Toxoplasma gondii, risk factors, One Health
Citation: Žele Vengušt D, Krt B, Blagus R, Vengušt G and Bandelj P (2024) Seroprevalence of infectious pathogens of zoonotic and veterinary importance in wild ruminants from Slovenia. Front. Vet. Sci. 11:1415304. doi: 10.3389/fvets.2024.1415304
Edited by:
Gianmarco Ferrara, University of Naples Federico II, ItalyReviewed by:
Sonia Almeria, United States Food and Drug Administration, United StatesElshafie I. Elshafie, Sultan Qaboos University, Oman
Han Sang Yoo, Seoul National University, Republic of Korea
Lawrence Mugisha, Makerere University, Uganda
Copyright © 2024 Žele Vengušt, Krt, Blagus, Vengušt and Bandelj. This is an open-access article distributed under the terms of the Creative Commons Attribution License (CC BY). The use, distribution or reproduction in other forums is permitted, provided the original author(s) and the copyright owner(s) are credited and that the original publication in this journal is cited, in accordance with accepted academic practice. No use, distribution or reproduction is permitted which does not comply with these terms.
*Correspondence: Petra Bandelj, cGV0cmEuYmFuZGVsakB2Zi51bmktbGouc2k=