- 1Department of Clinical Laboratory Sciences, College of Applied Medical Sciences, Shaqra University, Riyadh, Saudi Arabia
- 2Division of General Surgery, Department of Surgery, Ditmanson Medical Foundation Chia-Yi Christian Hospital, Chiayi, Taiwan
- 3Department of Zoology, Abdul Wali Khan University Mardan, Mardan, Pakistan
- 4Faculty of Veterinary and Animal Sciences, University of Poonch Rawalakot Azad Kashmir, Rawalakot, Pakistan
- 5Department of Pathology, Ditmanson Medical Foundation Chia-Yi Christian Hospital, Chiayi, Taiwan
- 6Department of Cosmetic Science, Chia Nan University of Pharmacy and Science, Tainan, Taiwan
- 7Ph.D. Program in Translational Medicine and Rong Hsing Translational Medicine Research Center, National Chung Hsing University, Taichung, Taiwan
- 8Department of Biotechnology and Bioindustry Sciences, College of Bioscience and Biotechnology, National Cheng Kung University, Tainan, Taiwan
African swine fever is a hemorrhagic disease of pigs with high mortality rates. Since its first characterization in 1921, there has been sufficient information about African swine fever virus (ASFV) and related diseases. The virus has been found and maintained in the sylvatic cycle involving ticks and domestic and wild boars in affected regions. The ASFV is spread through direct and indirect contact with infected pigs, their products and carrier vectors especially Ornithodoros ticks. Severe economic losses and a decline in pig production have been observed in ASFV affected countries, particularly in sub-Saharan Africa and Europe. At the end of 2018, the ASFV adversely affected China, the world’s leading pork-producer. Control strategies for the disease remained challenging due to the unavailability of effective vaccines and the lack of successful therapeutic measures. However, considerable efforts have been made in recent years to understand the biology of the virus, surveillance and effective control measures. This review emphasizes and summarizes the current state of information regarding the knowledge of etiology, epidemiology, transmission, and vaccine-based control measures against ASFV.
Introduction
African swine fever virus (ASFV) belongs to the family Asfarviridae is a double-stranded DNA virus that causes African swine fever in Suidae (1, 2). In its ancestral African habitat, the ASFV evolved approximately 300 years ago in its arthropod vector in a sylvatic cycle, specifically involving common warthogs (Phacochoerus africanus) and the soft tick (Ornithodoros moubata) (3). The ASFV is epidemic in nature, causing large-scale mortality in the infected pig population (4). Serious economic consequences accompany outbreaks of the disease and therefore require proactive surveillance and management (5). Horizontal transmission of ASFV occurs through the feeding of swill containing infectious pig meat, contaminated pig-related products, and competent vector species, especially Ornithodoros ticks (6). Transmission of the ASFV occurs within warthog burrows, primarily between ticks and warthogs (7).
In the late 1800s, the swine industry became larger in Kenya under British colonization, which significantly contributed to the prevalence of ASF and its subsequent distribution in Africa (8). The virus spread out of Africa in two different instance. The first incursion occurred in 1957 in Portugal, where ASFV-infected and contaminated waste materials were thrown from airline to feed the pigs, leading to the virus spreading through Russia, Western Europe, the Caribbean and Brazil over three decades. However, except for Sardinia, the disease was eradicated from all these affected areas by the mid-1990s (9). In 2007, a second incursion occurred in Georgia, expanded to the Russian Federation and Eastern Europe, and then spread globally (3). Globally, China is the largest pork producing country that is adversely affected by ASFV. In Europe, successful precautionary measures were limited to the Czech Republic, Belgium, Sweden, and Germany. There are high risks of the ASFV introduction to the United States, which is the third largest pork producing country in the world after China and the European Union (10). In July 2021, the USDA confirmed the entry of ASF into the Dominican Republic which then reached Haiti by September, marking a considerable geographical invasion and emphasizing the risks of ASFV being introduced into mainland North America. Large-scale movements of humans and animals are the major threat to those countries, that are free from ASFV (11). The increase in global trade of various goods and animals within or outside the country, provides a favorable means in the transmission and expansion of ASFV (12). Reducing the risks of ASFV, it requires a global attention to limit further expansion of this havoc-transmitting agent. Comprehensive knowledge of ASFV is necessary to overcome and eradicate the disease; for this purpose, this review is designed to highlight the etiology, epidemiology, transmission sources, and current development in prevention and control measures against ASFV.
Microbiology of ASFV
Structure of the virus
The shape of ASFV is icosahedral, and has an average diameter of 200 nm. There are four concentric layers in the structure of ASFV, an outer hexagonal membrane acquired by originating through the cell plasma membrane. The capsid is the outermost layer of the virion. The internal core of the ASFV particle is formed by the central genome and contains nucleoids, which are coated by a thick capsid in a layer called the core-shell (Figure 1) (13). For immunological interactions with the host, viral genomes encode genes essential for the replication of virus assembly (1). The virus replication process occurs mainly in the cytoplasm of infected macrophages and monocytes; however, it has also been observed in the nucleus at the early stages of infection (14). The ASFV is enveloped by a two-membraned collapsed cisterna, which is originates from the endoplasmic reticulum (15). Four classes of viral genes have been identified comprising immediate-early, early, intermediate, and late transcripts. Viral genes are usually express before and after the onset of DNA replication (16, 17). Enzymes are required for the ASFV replication and are expressed after the entry of the virus into the cytoplasm (18).
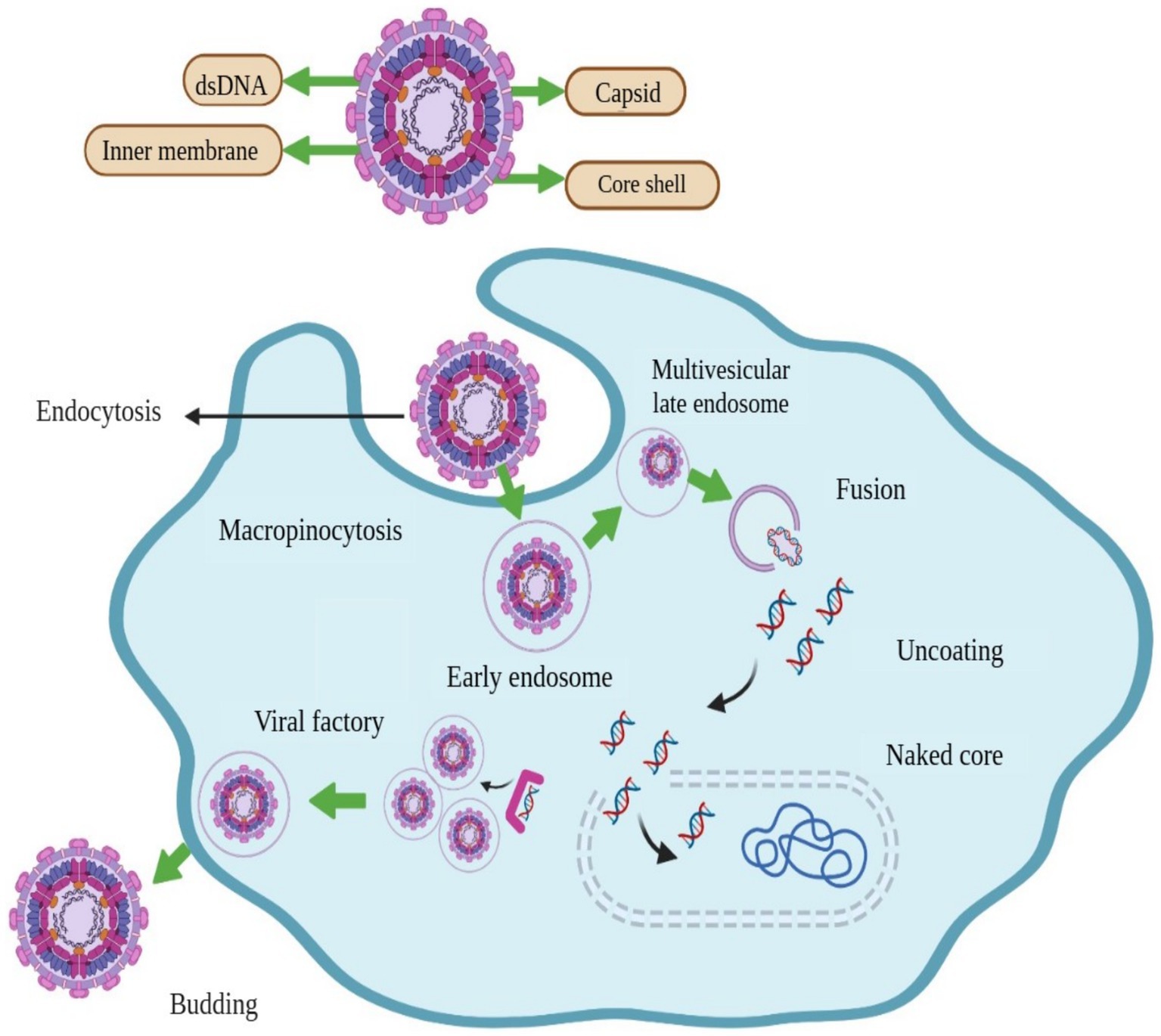
Figure 1. Structure and schematic presentation of African swine fever virus and mechanism of its entry into host cell, replication, and release.
Genomic aspects
The ASFV genome is a single linear molecule of double stranded DNA having covalently closed double stranded DNA (19, 20). The P72 is the primary capsid protein, encoded by the B646L gene. The genotyping and sequencing of ASFV depend on a variable region of the B646L gene within the respective C-terminal of the gene. The ASFV genome ranges from 170 to 190 kilobases (kb) encoding 150–200 viral proteins, and has predicted open reading frames ranging from 151 to 186 kb (21). The ASFV has low mutation rate due to the accurate DNA proofreading.
The sequencing of the ASFV genome has resulted in the generation of several ASFV genomes of viral and low pathogenic isolates. Genomic analysis of ASFV has provided important information on structure, variation, and precise phylogenetic reconstruction (22). The BA71V, first strain of ASFV sequenced in 1995 (23), has been used as a comparative model for ASFV, which accumulates large scale information related to its biochemical, morphogenetic and genetic behavior (24). The genotyping and sequencing of ASFV depend on a variable region of the B646L gene within the respective C-terminal of the gene (25). Different virulent and pathogenic sequences have been identified and characterized from different origins and deposited in GenBank for information and experimentation (22). Until to 2018, 19 full-length sequences of ASFV were available which were generated using the Sanger sequencing; the number of sequences increased to 114 in October 2021 (27, 26). The multigene family (MGF) are responsible for the variation of ASFV genomes (1). The deletion and insertion of copied regions occur within these five MGF genes, suggesting the role of MGF in generating antigenic variability, thus helping the virus to evade host immune response (28). Based on the B646L gene, ASFV is categorized and divided into 24 different genotypes, the p72 capsid protein is coded by the B646L gene of ASFV (14).
Different isolates of ASFV can induce variable severity of infection. Although mild and moderate complications are caused by mutated strains (1). Pathogenic and virulent strains of ASFV are responsible for mild or severe infection accompanied by symptoms like hemorrhages in the skin and internal organs with a high fever and at the final stage causes death. Sudden deaths of animals can lead to 90% of fatalities (29). It has been suggested that the range of host and virulence of ASFV depend on the members of MGF 360 and 505. Some reports have demonstrated that the removal of eight genes from family 360 and two genes from family 505 affects its virulence to infect macrophages (30). The virulence of ASFV is due to four genes: the thymidine kinase coding genes, 9GL (B119L in BA71V), United Kingdom gene (DP96R in BA71V) and NL-S gene (DP71L in BA71V) (31). Therefore, further studies are necessary to understand the viral genome of ASFV as well as the genes associated with its pathogenicity: such genes will be supportive of the development for efficient diagnosis and treatment.
Genome sequences highlight variations between viruses in terms of insertions, deletions, or mutations. Comparative genomic analysis facilitates evolutionary studies. In this context, the first detected variation was the insertion of tandem repeat sequences (TRS), of the 10-nucleotide “TATATAGGAA” present between 173R and 1,329. This insertion has been recognized for the first time in ASFV infecting wild boar in Poland and Lithuania in 2014, and now it is considered a new sub-genotype marker (14).
In China, comparison of identified sequences of two strains, DB/LN/2018 and Pig/HLJ/2018 indicated mutations due to the insertion and deletion of nucleotides at multiple positions in the genome (32). The length of the genome sequence of both viruses was found to be 189,404 bp, very similar to the genomes of PoL/2017, GA/2007/ and ASFV-SY18. A comparison of DB/LN/2018 and Pig/HLJ/2018 with GA/2007 revealed 16 inserted nucleotides and 9 deleted nucleotides at 15 and 5 positions, respectively. In the PoL/2017 viruses three deletions appeared, whereas no insertion or deletion is present in ASFY-SY18. Furthermore, five mutations observed in the ORFs of Pig/HLJ/2018 and DB/LN/2018, ASFV-SY18 and PoL/2017 strain. However, these mutations were not observed in the GA/2007 genome. Due to mutations in ASFV, as a result of insertion and deletion of nucleotides, it is confirmed that changes and alterations occur in ORFs. Although the comparisons with the database provided clues about genes that may modulate the virus-host relationship. It remains to be examined how these alterations occur and affect the pathogenic properties of the ORFs of ASFV.
Epidemiological profiles of ASFV
Warthogs and domestic pigs are the main reservoirs for the ASFV. It is known from the last 10 years’ data on ASF in the global context that the country will be potentially at risk where pigs are commercially as well as having wild reservoirs. Ticks are responsible for the transmission of ASFV and act as “reservoirs.” Infected animals blood has the highest concentration of ASFV; therefore, virus transmission occurs through direct and close interaction with infected animals. Use of infected pork products and fomites or contact with them and the mechanical vectors, e.g., biting flies, may also aid in the transmission of the ASFV to the hosts which are uninfected from ASFV (33).
The ASF is not zoonotic; there are only reports of animal infections and transmission; the epidemiology of ASF reflects the circulation of the virus within animal and arthropod reservoirs. The prevalence of the disease varies from region to region due to epidemiological differences. Virus epidemics or outbreak situations have been observed based on the geographical conditions of the associated area. Large-scale outbreaks of ASFV usually occur between 2007 (China, Thailand) and 2009 (Vietnam) (34).
Reservoirs of the ASFV
Although pigs are the most frequent reservoirs of ASFV, they exist in the sylvatic cycle between arthropod vectors specifically soft ticks, O. moubata and wild Suidae especially warthogs (35). Warthog burrows are the habitat of these ticks, where virus transmission occurs between warthogs and ticks (2). Recently, ASFV has been shown to grow within leeches (36), suggesting the participation of these hosts in the environmental perseverance of the virus (37).
Role of Suidae
Members of the pig family known to be susceptible to ASFV include domestic pigs and wild boars (Sus scrofa), bush pigs (Potamochoerus larvatus and Potamochoerus porcus), warthogs (Phacochoerus spp.) and giant forest hogs (Hylochoerus spp.) (7, 38. Since wild boars and domestic pigs are the main reservoirs responsible for disease outbreaks, the prevention and control of infected pig herds are the main issues (39). Wild boars and domestic pigs show various clinical signs, from acute to chronic (40). Although death is the first indication of the disease in a per-acute form, loss of appetite, depression, cutaneous hyperaemia and elevated body temperature (> 41°C) are other clinical manifestations associated with the per-acute form. The acute form of the disease is typically characterized by pulmonary edema, respiratory distress, abortion in pregnant females, nasal and conjunctival discharge, skin hemorrhages, splenomegaly, extensive necrosis and high mortalities (41). For the first time, the chronic form of the disease was reported in the Iberian Peninsula. The chronic form of ASFV has also been evidenced in experimental inoculation of animals with European isolates (14). Wild boar shows the same signs as domesticated pigs; however, no signs are observed before death in virulent strains. In warthogs and bush pigs, ASF is most frequently asymptomatic (42). It has been observed that survivors of ASFV may not only play a key role in ASFV perseverance in endemic regions but also contribute to periodic incidences, outbreaks, and invasions of ASFV to uninfected animals in the disease free zone (43).
Role of ticks
Following the classical epidemiological patterns of infectious diseases, ASFV circulates between animals and blood sucking arthropods. Hematophagy has been considered as a critical factor for the transmission and acquisition of ASFV by arthropods. Like other vector-borne diseases, the presence of an arthropod and blood sucking vector is important for the invasion and transmission of ASFV from the reservoir to healthy animals (44). An important aspect of the epizootic cycle of ASFV is the specificity of the vector. Among ticks, soft ticks are known competent vectors for ASFV. This pathogen has been isolated from 20 species of soft ticks and many other hematophagous arthropods, including competent louse and flies (Table 1). Even leeches have been considered susceptible to ASFV. Moreover, leeches were also able to transfer ASFV to experimental animals (36). Viral DNA has also been detected in hard ticks (Dermacentor reticulatus and Ixodes ricinus) collected from the bodies of dead ASFV positive wild boars and also in flies (54).
Ornithodoros ticks play a major role in the transmission of ASFV. All stages of their development can be easily infected with the virus, in the blood meal when the virus is taken from an infected pig. However, under experimental conditions, not all ticks that feed on infected pigs or artificial membranes become infected. For instance, in the case of the Ornithodoros erraticus infection model, an infection rate of ticks of 83.1% (pig-feeding ticks) and 53.4% (membrane-feeding ticks) infection rate of ticks has been observed (48).
Localization within tick
Rock (55) performed the first experiment on the localization of ASFV in, Ornithodoros porcinus. Initial replication of ASFV was observed in hemocytes (types I and II), epithelium of the midget, phagocytic cell, connective tissue, salivary gland, coxal gland and reproductive tissue, which were the secondary sites of virus replication. Similarly, the highest viral titers were detected in salivary glands and reproductive tissue after 91 days of infection (28).
Survival of ASFV in ticks
It is known that ASFV once infects the tick, is capable of remaining viable within the tick’s body for a long period between 23 and 239 days, depending the tick species (56). Much longer survival has also been reported: 3 years in O. moubata, 5 years in O. erraticus and 502 days in Ornithodoros coriaceus (29, 50, 57). The data suggest that without contact with the swine population, the ASFV can survive within the tick population, therefore, making an alarming source of reinfection.
Transstadial and transovarial transmission
Ticks can transmit infectious agents, retained within their body, to their next stage (transstadial) or the next generation (transovarial transmission). Similarly, soft ticks are believed to transmit ASFV transstadially, as well as transovarially through sexual contact, and directly to susceptible animals (29, 58, 59). However, in the case of transstadial transmission, the transmission rate of ASFV has been found to decrease with each molt (60).
Pig-to-pig transmission
Direct contact with infectious pigs has been established to be an effective mechanism of disease transmission. The domestic pig can transmit the virus through nasal fluid, secretion, and in excretion through urine. In a recent study, healthy pigs housed together with infected ones became infected after one to 9 days after exposure. Delayed infectivity of healthy pigs was also observed in those separated from infected ones (14). During the fights, environmental contamination occurs due to the shedding of the blood from the wounds of infected pigs or from fecal contamination by infected Suidae (38). The possibility of a “carrier” state persists in pigs as well as in other Suidae. In the Netherlands, carrier pigs recovered from an acute ASFV infection with the lower pathogenicity strain were found to transfer the disease by direct contact with the uninfected pig population. It is believed that the overcoming and disappearance of clinical signs and symptoms occur, and after a month the infected pigs shed the virus. However, the effect on transmission will depend on the survival and duration of the carrier status of the pig (61).
Transmission through the ingestion of contaminated feed
Direct contact with the environment or infected pigs can introduce the virus into their bodies through mucous membranes. However, some animals are infected via ingestion of infected food. The presence of ASFV has been confirmed in pork products such as pig fat, skin, meat, and other pig products used for different purposes (62). Similarly, seed contamination and fresh grass containing wild boars secretions are the key risks and threat for the transmission (63). Therefore, these sources should also be considered to prevent transmission to naive pigs.
Wild boar-to-pig transmission
For ASFV transmissions, recent research has confirmed that wild boars act as a susceptible host for the transmission to domestic pigs. In domestic pigs, ASFV can develop nonspecific signs and symptoms (63). Several fields studies have confirmed the spread of ASFV from wild boars to domestic pigs. In this context, a study conducted in Russia detected several cases of ASFV primarily in wild boars before being observed in domestic pigs, and the death of wild boars caused by ASF was observed in the vicinity of ASF-affected farms (64). Similarly, the housing of susceptible pigs with ASFV-infected wild boars became infectious after 6 to 12 days post-exposure (65). The European Commission tasked the European Food Safety Authority (EFSA) to study and review the evolutionary ability and tendency of matrices, including arable crops, vegetables, wood chips, sawdust, hay, straw and other related agents that are the threats of transmitting ASFV (66).
Although ASFV is likely to transmit from wild boar to domestic pigs, long-range invasions of ASFV are mostly caused by anthropogenic activities such as improper disposal of carcasses of infected wild boars or infected pigs, disposed of by hunters and farmers. The sedentary nature of wild boars is considered the basic reason behind this issue. Generally, wild boars spend much time seated, up to 100 kilometers in 6 months; therefore, mostly they cannot cross long distances (67). Transmission of ASFV occurs during the migration of an adult male or female for reproduction purposes, the life span of the disease is short in this case ranging from five to 7 days (63). We assume that long-range ASFV incursions are not associated with wild boars; however, one cannot ignore their role in the transmission of ASFV to domestic pigs in close contact.
Movement of virus through fomites
Recent experiments have suggested that the ASFV can remain in the blood, feces and urine of infected pigs. As ASFV can easily contaminate the environment, therefore, anything that is contaminated may act as a virus source (68). An example of this type of incidence occurred in Europe, where the disease was introduced by ships containing ASFV-contaminated kitchen and catering waste used to feed pigs near the surrounding areas. Subsequently, the disease spread to the Caucasian region, the countries of the European Union (69). However, fomites are usually considered equipment, clothing, bedding, footwear, or transport which is contaminated and whereby the virus can be moved to a new area (70). From the observation of these experimental procedures, the transfer and movements of ASFV with fomites should be considered as a possible way for ASFV to spread to virus-free areas.
Anthropogenic factors
Human activities are important risk factors for ASFV transmission (26). Anthropogenic activities responsible for virus transmission include legal and illegal transport of pigs and pig’s products, insufficient biosecurity measures for pig holdings and noncompliance with hunting restrictions and control strategies during and after the ASFV outbreaks (71). The primary cause of ASFV transmission is anthropogenic activities that cause long-distance transmission events and the introduction of pig farms (72). In China an initial outbreak of ASFV was linked to the feeding of pigs with contaminated table scraps (73), and in Vietnam contaminated pork products were likely responsible for the first outbreak (74). In Asia, anthropogenic activities have played a key role in maintenance and transmission (74). Targeted interventions and advanced biosecurity measures are necessary to eradicate the transmission of ASFV due to human activities.
African swine fever in Asia
In Asia, ASFV is more prevalent, following a pathway from northeast to southeast. In these countries, the transmission of ASFV is favored by compromised safety measures related to human activities including the transport of infected and contaminated fomites and pig products (75).
The introduction of ASFV in Asia and especially the invasion of China had a drastic impact on the pig industry (76). Despite these efforts, the virus has persistently crossed international borders rapidly. Subsequent introductions have occurred in Cambodia, Bhutan, Malaysia, India, China, Indonesia, Mongolia, Thailand, North Korea, South Korea, Myanmar, Vietnam, East Russia, Timor Leste, the Philippines, and Hong Kong (Figure 2) (3). In 2019, ASFV outbreak reported in Mongolia was confirmed by the Organization for Animal Health or Office International des Epizooties (OIE) (77). Many of these countries are characterized by rural pig farms and small-holder operations, making the resulting outbreaks particularly challenging to monitor and manage effectively. The dynamic nature of the virus’s spread necessitates ongoing scientific efforts to understand and address the complexities of ASFV transmission within diverse agricultural and economic contexts (26).
African swine fever in China
The rapid development of the Chinese economy has brought about significant changes in food consumption patterns. Similarly, demands for meat and meat products, especially pork, have shown a constant increase over the last few years (78). Taking this advantage, the swine sector of the country has integrated production systems to become an industry. Three types of pig farming have been practiced based on the number of pigs produced; small, medium and large farms. Farms close to large cities have been shifted from backyards to modern intensive farms (34).
In China, the first outbreak of ASFV was reported on August 3, 2018, when 400 pigs on a farm near Shenyang City in the north-eastern Liaoning province, developed acute clinical disease after consuming table scraps. The mortality rate was 100%, leading to abandonment of the farm. Subsequently, similar cases were observed on nearby farms (73). At the end of 2019, a total of 33 outbreaks were reported in eight provinces of the country, ASF has led to the deaths of more than 100,000 pigs with an estimated loss of USD 111.2 billion (79, 80). Without the availability of effective control measures, including vaccination, the resumption of production will be problematic. As a result, in China, pig production was reduced by 40% in 2019 compared to 2018. Similarly, the price of pork was doubled in 2019 (81). Therefore, the spread of this disease has posed threats to the large population of domestic pigs and wild boars in China, as well as in neighboring countries.
A strategy to control the outbreak has been developed by Chinese authorities (Ministry of Agriculture and Rural Affairs; MARA) and implemented soon after the emergence of the first outbreak. Culling all pigs within 3 km of the infected area and capturing all infected pigs, their disposals, and contaminants became mandatory. For the prevention and control of the ASFV outbreak, the Ministry of Agriculture and Rural Affairs has taken several preventive measures to control the outbreaks. Several preventive biosecurity measures were implemented including restricted pig movement, complete biosecurity protocols both inside and outside of pig farms, and systematic monitoring and recycling of pig products and waste materials. Quarantine measures have been enforced on farms, and high temperatures were applied for the treatment of feed and other waste materials of pigs (82). Despite these measurements, the epidemiological status in China become worse after the occurrence of ASFV new cases (83). The alarming spread and expansion of ASFV necessitates a dynamic scientific approach to control ASFV in various regions (84).
African swine fever in Africa
In Africa, ASFV evolved through a sylvatic cycle between the soft tick O. moubata and warthogs (85). From 1989 to 2017, 5,134 ASFV outbreaks were recorded with 88.5% occurring in the domestic pig population and wild Suidae (33). The expansion and transmission of ASFV to new areas occurs due to the movement of infected pigs into ASFV free-areas, while warthogs and wild suids also play a major role in its transmission and propagation in Africa (9, 64). In Africa, the spread of ASFV to new areas beyond its endemic region causes severe losses (33). Outbreaks arise from transmission between domestic pigs and the sylvatic cycle, with warthogs being translocated into the southern part of the continent over the past four decades (7). In 2017, ASF outbreaks had been reported in Cabo Verde, Côte d’Ivoire, Burundi, the Central African Republic, Chad, Kenya, Madagascar, Mali, Mozambique, Namibia, Niger, Sierra Leone, South Africa, Zambia, Zimbabwe, Tanzania, Sahara, Zanzibar, Malawi, Angola, Botswana, Ethiopia, Djibouti, Liberia, Senegal, Mauritania, Morocco, Mali, and Tunisia [(Figure 3; (86)]. The rapid expansion of the African population has demanded an increase in meat production. This has a direct impact on the pig population, which constitutes approximately 5% of the global pig population (33, 87). Some African countries, namely South Africa, Madagascar, Mozambique, Namibia, and Nigeria, reported one to multiple outbreaks. Likewise, the unquantified presence of the disease has also been reported in countries like Cameroon and Cape Verde (33, 85). At the end of 2017, 69 cases of ASFV were confirmed in which warthogs act as a source of the outbreak that occurred in Kenya, Namibia, Botswana, Zambia, Zimbabwe, South Africa and Tanzania (33). Restriction of wild pigs to conservation areas has reduced their likely role in the epidemiology of ASFV (75).
African swine fever in Europe
In Europe, the past 6 years have seen the introduction of ASFV to Belgium, Bulgaria, the Czech Republic, France, Germany, Greece, Hungary, Italy, Lithuania, Malta, Moldova, the Netherlands, North Macedonia, Poland, Romania, Serbia, Slovakia, Spain, Sweden, Georgia, Portugal, Latvia, Estonia and Romania (Figure 4) (88). Recently, the Czech Republic and Belgium, in which domestic pigs were not infected, now appear to have eradicated ASFV via biosecurity measures. Elsewhere (including Bulgaria, Hungary, Poland, Romania, and Slovakia in particular), the virus generally appears to be beginning ground, with numerous outbreaks, especially on smallholder farms (86). Similarly, the introduction of ASFV in northeast Lithuania resulted in the death of more than 20,000 pigs (14). Epidemiological investigations have revealed some details of the ASFV transmission patterns unique to these countries (e.g., Poland, where wild boar infections are dominant, versus Romania, where domestic outbreaks are more common) and have also identified the apparent evolution of lower-virulence ASFV strains in Estonia and Latvia (89). The ASFV genotype I was restricted to the African continent from its first recognition in 1920 until 1957, when the outbreak was reported in Portugal. Spain was the first European country to report ASFV cases, followed by Italy, France, Malta, Belgium, and the Netherlands (28, 90). Pork meat imported directly from Spain was thought to be the source of the first ASFV case in Belgium in 1985. The first outbreak of ASF resulted in the slaughter of 34,000 pigs housed on 60 holdings (29). The pig population in the Netherlands was severely affected from 1960 to 1995 due to ASF (29, 91). Although ASF was successfully eradicated from most European countries by 1995, a notable exception was the Mediterranean island of Sardinia (Italy). The main factors that were responsible for the persistence of the disease in that area were the keeping of more than 70% of the pig population in extensive systems and backyard farms, combined with the proximity of wild boars (92).
The lack of consistency in ASF contingency plans and preventive measures resulted in the second spread of ASFV in European countries (29). In this context, the first case was recognized in Georgia in 2007,followed by numerous outbreaks of domestic pigs and wild boars (28, 29, 64, 90). The specific origin of the virus responsible for the outbreak is still unknown; however, the virus genotype has been linked with that in Madagascar and Mozambique (90). The disease spread from Georgia to native European countries and was recently reported in Latvia, Estonia, Hungary, and the Netherlands (90). Not only in western Europe, the pig population was also severely affected in eastern Europe. Poland, a country where 66% of the pig population is kept on small farms, reported the first case of ASF in 2014 (90, 93). Since the first outbreak in Poland, authorities have reported several outbreaks, with a total of 5,333 cases of the disease being confirmed in wild boars (29).
Since the first appearance of ASF in Europe in (1960), it took 30 years to successfully eradicate the virus from affected countries (94, 95). The second spread of the disease (2007), contributed to virus migration in nearby regions, as well as a high probability of outbreaks in neighboring countries (90). Efforts to control disease in Europe have not been successful. Impediments to the development of successful eradication programs include low biosecurity (human factor), free-ranging wild boar populations, and a high prevalence of the virus in surrounding bordering countries (90). Low or non-existent biosecurity measures at small-scale pig holdings increase the risk of introducing viruses on the farm. Ticks, wild boar populations, and the illegal trade of infected meat products are the other factors responsible for maintaining and circulating the virus within pig populations (57). Despite the challenges, the European authorities have implemented multifaceted preventive and hygiene procedures. In addition, government institutions are strongly convinced of achieving the eradication of disease in a short period of time.
African swine fever in the United States and its future assessment
The United States (United States) is believed to manage pig production under high biosecurity conditions. Economic losses due to ASFV introduction into the U. S are estimated in between $15 and $50 billion, depending on the disease spread in the feral swine population (96). Commercial swine production is a closed system from farrowing to slaughter as a means of reducing the risk of pathogen introduction (97). To limit cross contamination, transport vehicles, animal feed, personnel and other fomites are closely managed. Despite the high-profile biosecurity measures, transport equipment contributed to the spread of the porcine epidemic diarrhea virus (PEDV) in 2013 (59). This indicates that despite stringent biosecurity protocols, it can be difficult to control ASF. Keeping this in mind, the US has substantially contributed to the implementation of a series of preventive measures designed for the importation of live animals and their products.
Prevention and control strategies
The escalating intensification of animal movements and product exchanges have increased the risk of ASF in new regions, particularly those developing trade ties with new eastern EU member states (98). Despite of this threat, despite efforts, there is no effective vaccine available for global eradication. The developing of an effective vaccine becomes crucial to controlling one of the major pig diseases in Africa. Such a vaccine would offer an alternative to animal slaughter, mitigating the spread of ASF in both Africa and Europe (99). Challenges to eradication efforts include free-range production systems, interactions with Ornithodoros ticks and/or wild suids, and endemicity involving asymptomatic carriers (Wamwatila et al., 2015) (100). Success relies highly on effective communication between all involved parties in an outbreak, including diagnostic laboratories, farmers, field and official veterinarians, disease crisis centers, and media participation. Implementing improvements in pig housing to minimize contact with ticks and wild animals have proven highly efficient in reducing infection to eradication levels and should not be overlooked (1). A cost–benefit analysis should determine whether contingency efforts would be directed toward control or eradication (101).
In the absence of a vaccine and considering the role of the sylvatic cycle in the epidemiology of southern and eastern Africa’s disease, logical measures for ASF control include the physical separation of domestic pigs from wild hosts and treating pig premises with acaricides in areas where tick-infected by ASFV occurs (28). Control based on the physical separation between wildlife and pigs has been proven successful in controlling ASFV, even in animals from those regions where the virus circulates among infected warthog populations (7).
Control strategy based on genetically modified vaccines
Despite the implementation of strong biosecurity measures, it is a difficult task to control the spread of disease in the pig population. Control measures rely on prompt reporting, repeat testing, and culling infected and at-risk animals. Moreover, in underdeveloped countries these methods and their establishment are difficult to apply. As an alternative, researchers are now struggling to develop an effective vaccine against ASFV. For this purpose, several attempts have been made in recent decades. Different vaccine strategies such as DNA vaccines, adenovirus vector vaccines, subunit vaccines, and inactivated vaccines have been tested and proven to be unsuccessful (2). Similarly, extracts of infected cells, purified and inactivated virions, infected leukocyte blood supernatants, infected glutaraldehyde fixed macrophages, or infected alveolar macrophages have been used to produce immunity against ASFV (62). However, all of these attempts failed to produce desirable results. Meanwhile, it was observed that pigs infected with attenuated or virulent variants of ASFV may establish resistance to homologous virus challenge (102). These observations led scientists to develop an effective live attenuated virus by deleting genes not associated with ASFV replication. Virulent isolates of ASFV have been modified with deletions of genes to attenuate the virus. Keeping this in mind, the BeninΔDP148R virus was genetically modified by deleting the DP148R gene to isolate the virulent strain, Benin97/1. Deletion of the gene reduced the pathogenicity of the BeninΔDP148R virus in pigs. All the pigs immunized with the virus showed only mild transient clinical signs and survived infection. Moreover, high level of protection was observed against the parental virulent strain (103). The same level of safety and protection was observed after immunization of the pig with ASFV-G-ΔI177L. After immunization, the pig showed a strong and specific antibody response and low viremia titers (104) (Table 2).
Based on available knowledge, the use of genetically modified viruses is the most reasonable approach to establishing an effective ASFV vaccine. Genetic modification and deletion of one or more genes change the virus from virulent to less virulent (104, 108). In domestic pigs ASAFV-G-Δ8DR is responsible for disease state. Pigs infected with the ancestral virus ASAFV-G-Δ8DR show the same viremia values. More attention is needed for the selection of targeted genes. Attenuated viruses of different genotypes should be tested to obtain strains that protect isolates circulating in different regions. Moreover, optimized targeted genes are used for safety standards. Similarly, the issue of the availability of a licensed cell line to grow live attenuated viruses for vaccine production needs to be resolved. For efficient control measures, immune responses induced by virus antigenic proteins are necessary to enhance the protection of infected animals (108). Therefore, the rational development of novel ASFV vaccines requires caution and more work to optimize commercial production.
Conclusion
The current situation of ASF signifies a constant risk to the livestock sector. Recent exploration and flourishing of ASFV have demonstrated the ability of the virus to spread over long distances. As a result, there is a tremendous decrease in both the production and farming of pigs. Furthermore, the implications of the trade related to ASFV in swine have severely affected the pork industry. Veterinary services need to perform rigorous surveillance in countries that consume pigs, as the inaccessibility to effective medication persists, leading to high mortality rates are the main reasons. Biosecurity measures are crucial to prevent the transmission of viruses. Inadequate biosecurity practices can create opportunities for the spread of viruses, which pose risks to human and animal health. Vaccines have given some favorable results; however, further investigation is required to prove them as the only choice to treat and control the disease.
Author contributions
BA: Formal analysis, Investigation, Methodology, Visualization, Writing – original draft, Writing – review & editing. C-HW: Writing – original draft, Writing – review & editing. MK: Writing – original draft, Writing – review & editing. MN: Writing – original draft, Writing – review & editing. C-CC: Writing – original draft, Writing – review & editing. AA: Conceptualization, Data curation, Formal analysis, Funding acquisition, Investigation, Methodology, Project administration, Resources, Software, Supervision, Validation, Visualization, Writing – original draft, Writing – review & editing.
Funding
The author(s) declare that no financial support was received for the research, authorship, and/or publication of this article.
Acknowledgments
We are gratefull to the Higher Education Commission (HEC) and the Pakistan Science Foundation (PSF).
Conflict of interest
The authors declare that the research was conducted in the absence of any commercial or financial relationships that could be construed as a potential conflict of interest.
Publisher’s note
All claims expressed in this article are solely those of the authors and do not necessarily represent those of their affiliated organizations, or those of the publisher, the editors and the reviewers. Any product that may be evaluated in this article, or claim that may be made by its manufacturer, is not guaranteed or endorsed by the publisher.
References
1. Dixon, LK, Stahl, K, Jori, F, Vial, L, and Pfeiffer, DU. African swine fever epidemiology and control. Annu Rev Anim Biosci. (2020) 8:221–46. doi: 10.1146/annurev-animal-021419-083741
2. Murgia, MV, Mogler, M, Certoma, A, Green, D, Monaghan, P, Williams, DT, et al. Evaluation of an African swine fever (ASF) vaccine strategy incorporating priming with an alphavirus-expressed antigen followed by boosting with attenuated ASF virus. Arch Virol. (2019) 164:359–70. doi: 10.1007/s00705-018-4071-8
4. Penrith, ML, Van Heerden, J, Heath, L, Abworo, EO, and Bastos, AD. Review of the pig-adapted African swine fever viruses in and outside Africa. Pathogens. (2022) 11:1190. doi: 10.3390/pathogens11101190
5. Fasina, FO, Lazarus, DD, Spencer, BT, Makinde, AA, and Bastos, AD. Cost implications of African swine fever in smallholder farrow-to-finish units: economic benefits of disease prevention through biosecurity. Transbound Emerg Dis. (2012) 59:244–55. doi: 10.1111/j.1865-1682.2011.01261.x
6. Misinzo, G, Magambo, J, Masambu, J, Yongolo, MG, Van Doorsselaere, J, and Nauwynck, HJ. Genetic characterization of African swine fever viruses from a 2008 outbreak in Tanzania. Transbound Emerg Dis. (2011) 58:86–92. doi: 10.1111/j.1865-1682.2010.01177.x
7. Craig, AF . Interrelationships of warthogs (Phacochoerus africanus), Ornithodoros ticks and African swine fever virus in South Africa (Doctoral dissertation, University of Pretoria SouthAfrica). (2022). Available at: https://www.proquest.com/openview/54c64ebf01718211ee0c6288dcfa91af/1?pq-origsite=gscholar&cbl=2026366&diss=y.
8. Alkhamis, MA, Gallardo, C, Jurado, C, Soler, A, Arias, M, and Sanchez-Vizcaino, JM. Phylodynamics and evolutionary epidemiology of African swine fever p72-CVR genes in Eurasia and Africa. PLoS One. (2018) 13:e0192565. doi: 10.1371/journal.pone.0192565
9. Brown, AA, Penrith, M-L, Fasina, FO, and Beltran-Alcrudo, D. The African swine fever epidemic in West Africa, 1996–2002., 2018. Transbound Emerg Dis. (2018) 65:64–76. doi: 10.1111/tbed.12673
10. Szymańska, EJ, and Dziwulaki, M. Development of African swine fever in Poland. Agriculture. (2022) 12:119. doi: 10.3390/agriculture12010119
11. Bora, M, Bora, DP, Manu, M, Barman, NN, Dutta, LJ, Kumar, PP, et al. Assessment of risk factors of African swine fever in India: perspectives on future outbreaks and control strategies. Pathogens. (2020) 9:1044. doi: 10.3390/pathogens9121044
12. Vineis, P. Health without borders: Epidemics in the era of globalization. Springer. (2017). Available at: https://books.google.com.pk/books?hl=en&lr=&id.
13. Salas, ML, and Andrés, G. African swine fever virus morphogenesis. Virus Res. (2013) 173:29–41. doi: 10.1016/j.virusres.2012.09.016
14. Gallardo, C, Nurmoja, I, Soler, A, Delicado, V, Simón, A, Martín, E, et al. Evolution in Europe of African swine fever genotype II viruses from highly to moderately virulent. Vet Microbiol. (2018) 219:70–9. doi: 10.1016/j.vetmic.2018.04.001
15. Chapman, DA, Tcherepanov, V, Upton, C, and Dixon, LK. Comparison of the genome sequences of non-pathogenic and pathogenic African swine fever virus isolates. J Gen Virol. (2008) 89:397–408. doi: 10.1099/vir.0.83343-0
16. Almazán, F, Rodríguez, JM, Andrés, G, Pérez, R, Viñuela, E, and Rodríguez, JF. Transcriptional analysis of multigene family 110 of African swine fever virus. J Virol. (1992) 66:6655–67. doi: 10.1128/jvi.66.11.6655-6667.1992
17. Rodríguez, JM, Salas, ML, and Viñuela, E. Intermediate class of mRNAs in African swine fever virus. J Virol. (1996) 70:8584–9. doi: 10.1128/jvi.70.12.8584-8589.1996
18. Almazan, F, Rodriguez, JM, Angulo, A, Vinuela, E, and Rodriguez, JF. Transcriptional mapping of a late gene coding for the p12 attachment protein of African swine fever virus. J Virol. (1993) 67:553–6. doi: 10.1128/jvi.67.1.553-556.1993
19. Galindo, I, and Alonso, C. African swine fever virus: a review. Viruses, (2021) 9:103 (2017). doi: 10.3390/v9050103,
20. Wang, G, Xie, M, Wu, W, and Chen, Z. Structures and functional diversities of ASFV proteins. Viruses. (2021) 13:2124. doi: 10.3390/v13112124
21. Njau, E . Detection and genetic characterization of sylvatic and outbreak African swine fever virus isolates in selected zones of Tanzania (Doctoral dissertation, NM-AIST). (2022). Available at: https://dspace.mm-aist.ac.tz.
22. Rodríguez, JM, Moreno, LT, Alejo, A, Lacasta, A, Rodríguez, F, and Salas, ML. Genome sequence of African swine fever virus BA71, the virulent parental strain of the nonpathogenic and tissue-culture adapted BA71V. PLoS One. (2015) 10:e0142889. doi: 10.1371/journal.pone.0142889
23. Yáñez, RJ, Rodriguez, JM, Nogal, ML, Yuste, L, Enriquez, C, Rodriguez, JF, et al. Analysis of the complete nucleotide sequence of African swine fever virus. Virology. (1995) 208:249–78. doi: 10.1006/viro.1995.1149
24. Bishop, RP, Fleischauer, C, de Villiers, EP, Okoth, EA, Arias, M, Gallardo, C, et al. Comparative analysis of the complete genome sequences of Kenyan African swine fever virus isolates within p72 genotypes IX and X. Virus Genes. (2015) 50:303–9. doi: 10.1007/s11262-014-1156-7
25. Wade, A, Achenbach, JE, Gallardo, C, Settypalli, TBK, Souley, A, Djonwe, G, et al. Genetic characterization of African swine fever virus in Cameroon, 2010–2018. J Microbiol. (2019) 57:316–24. doi: 10.1007/s12275-019-8457-4
26. Lim, JS, Andraud, M, Kim, E, and Vergne, T. Three years of African swine fever in South Korea (2019–2021): a scoping review of epidemiological understanding. Transbound Emerg Dis. (2023) 2023:1–15. doi: 10.1155/2023/4686980
27. Gladue, DP, Ramirez-Medina, E, Vuono, E, Silva, E, Rai, A, Pruitt, S, et al. Deletion of the A137R gene from the pandemic strain of African swine fever virus attenuates the strain and offers protection against the virulent pandemic virus. Journal of virology. (2021) 2021:10–1128. doi: 10.1128/jvi.01139-21
28. Sánchez-Vizcaíno, JM, Martínez-López, B, Martínez-Avilés, M, Martins, C, Boinas, F, Vialc, L, et al. Scientific review on African swine fever. EFSA Support Publ. (2009) 6:5E. doi: 10.2903/sp.efsa.2009.EN-5
29. EFSA Panel on Animal Health and Welfare . Scientific opinion on the role of tick vectors in the epidemiology of Crimean-Congo hemorrhagic fever and African swine fever in Eurasia. EFSA J. (2010) 8:1703. doi: 10.2903/j.efsa.2010.1703
30. Afonso, CL, Piccone, ME, Zaffuto, KM, Neilan, J, Kutish, GF, Lu, Z, et al. African swine fever virus multigene family 360 and 530 genes affect host interferon response. J Virol. (2004) 78:1858–64. doi: 10.1128/JVI.78.4.1858-1864.2004
31. Gladue, DP, and Borca, MV. Recombinant ASF live attenuated virus strains as experimental vaccine candidates. Viruses (2022) 14:878. HYPERLINK “https://doi.org/10.3390/v14050878” doi: 10.3390/v14050878
32. Wen, X, He, X, Zhang, X, Zhang, X, Liu, L, Guan, Y, et al. Genome sequences derived from pig and dried blood pig feed samples provide important insights into the transmission of African swine fever virus in China in 2018. Emerg Microb Infect. (2019) 8:303–6. doi: 10.1080/22221751.2019.1565915
33. Penrith, ML, Bastos, AD, Etter, EM, and Beltrán-Alcrudo, D. Epidemiology of African swine fever in Africa today: sylvatic cycle versus socio-economic imperatives. Transbound Emerg Dis. (2019) 66:672–86. doi: 10.1111/tbed.13117
34. Kedkovid, R, Sirisereewan, C, and Thanawongnuwech, R. Major swine viral diseases: an Asian perspective after the African swine fever introduction. Porcine. Health Manage Forum. (2020) 6:20. doi: 10.1186/s40813-020-00159
35. Jori, F, Bastos, A, Boinas, F, Van Heerden, J, Heath, L, Jourdan-Pineau, H, et al. An updated review of Ornithodoros ticks as reservoirs of African swine fever in sub-Saharan Africa and Madagascar. Pathogens. (2023) 12:469. doi: 10.3390/pathogens12030469
36. Karalyan, Z, Avetisyan, A, Avagyan, H, Ghazaryan, H, Vardanyan, T, Manukyan, A, et al. Presence and survival of African swine fever virus in leeches. Vet Microbiol. (2019) 237:108421. doi: 10.1016/j.vetmic.2019.108421
37. Avagyan, HR, Hakobyan, SA, Avetisyan, AS, Bayramyan, NV, Hakobyan, LH, Poghosyan, AA, et al. The pattern of stability of African swine fever virus in leeches. Vet Microbiol. (2023) 284:109835. doi: 10.1016/j.vetmic.2023.109835
38. Jori, F, and Bastos, AD. Role of wild suids in the epidemiology of African swine fever. EcoHealth. (2009) 2009:296–310. doi: 10.1007/s10393-009-0248-7
39. Alarcón, LV, Allepuz, A, and Mateu, E. Biosecurity in pig farms: a review. Porcine Health Manage. (2021) 7:5. doi: 10.1186/s40813-020-00181-z
40. Pikalo, J, Zani, L, Hühr, J, Beer, M, and Blome, S. Pathogenesis of African swine fever in domestic pigs and European wild boar–lessons learned from recent animal trials. Virus Res. (2019) 271:197614. doi: 10.1016/j.virusres.2019.04.001
41. Ebwanga, EJ . Molecular and epidemiological characterization of the African swine fever virus in Cameroon. ODI (2022). Available at: https://kuleuven.limo.libis.be/discovery/fulldisplay?docid=lirias3819905&context.
42. Nišavić, J, Radalj, A, Milić, N, Živulj, A, Benković, D, Stanojković, A, et al. A review of some important viral diseases of wild boars. Biotechnol Anim Husbandry. (2021) 37:235–54. doi: 10.2298/BAH2104235N
43. Netherton, CL, Connell, S, Benfield, CT, and Dixon, LK. The genetics of life and death: virus-host interactions underpinning resistance to African swine fever, a viral hemorrhagic disease. Front Genet. (2019) 10:413787. doi: 10.3389/fgene.2019.00402
44. Socha, W, Kwasnik, M, Larska, M, Rola, J, and Rozek, W. Vector-borne viral diseases as a current threat for human and animal health–one health perspective. J Clin Med. (2022) 11:3026. doi: 10.3390/jcm11113026
45. Lv, T, Xie, X, Song, N, Zhang, S, Ding, Y, Liu, K, et al. Expounding the role of tick in Africa swine fever virus transmission and seeking effective prevention measures: a review. Front Immunol. (2022) 13:1093599. doi: 10.3389/fimmu.2022.1093599
46. Ravaomanana, J, Michaud, V, Jori, F, Andriatsimahavandy, A, Roger, F, Albina, E, et al. First detection of African swine fever virus in Ornithodoros porcinus in Madagascar and new insights into tick distribution and taxonomy. Parasit Vectors. (2010) 3:1–9. doi: 10.1186/1756-3305-3-115
47. Basto, AP, Nix, RJ, Boinas, F, Mendes, S, Silva, MJ, Cartaxeiro, C, et al. Kinetics of African swine fever virus infection in Ornithodoros erraticus ticks. J Gen Virol. (2006) 87:1863–71. doi: 10.1099/vir.0.81765-0
48. Ribeiro, R, Otte, J, Madeira, S, Hutchings, GH, and Boinas, F. Experimental infection of Ornithodoros erraticus sensu stricto with two Portuguese African swine fever virus strains. Study of factors involved in the dynamics of infection in ticks. PLoS One. (2015) 10:e0137718. doi: 10.1371/journal.pone.0137718
49. Ravaomanana, J, Jori, F, Vial, L, Pérez-Sánchez, R, Blanco, E, Michaud, V, et al. Assessment of interactions between African swine fever virus, bushpigs (Potamochoerus larvatus), Ornithodoros ticks and domestic pigs in North-Western Madagascar. Transbound Emerg Dis. (2011) 58:247–54. doi: 10.1111/j.1865-1682.2011.01207.x
50. Kleiboeker, SB, and Scoles, GA. Pathogenesis of African swine fever virus in Ornithodoros ticks. Anim Health Res Rev. (2001) 2:121–8. doi: 10.1079/AHRR200133
51. Herm, R, Tummeleht, L, Jürison, M, Vilem, A, and Viltrop, A. Trace amounts of African swine fever virus DNA detected in insects collected from an infected pig farm in Estonia. Vet Med Sci. (2020) 6:100–4. doi: 10.1002/vms3.200
52. Mellor, PS, Kitching, RP, and Wilkinson, PJ. Mechanical transmission of capripox virus and African swine fever virus by Stomoxys calcitrans. Res Vet Sci. (1987) 43:109–12. doi: 10.1016/S0034-5288(18)30753-7
53. Olesen, AS, Hansen, MF, Rasmussen, TB, Belsham, GJ, Bødker, R, and Bøtner, A. Survival and localization of African swine fever virus in stable flies (Stomoxys calcitrans) after feeding on viremic blood using a membrane feeder. Vet Microbiol. (2018) 222:25–9. doi: 10.1016/j.vetmic.2018.06.010
54. Costa JFDGDM . Understanding the dynamics of African swine fever spread at the interface between wild boar and domestic swine in Sweden (Doctoral dissertation, Universidade de Lisboa, Faculdade de Medicina Veterinária). (2017) Available at: https://www.proquest.com/openview/1ff37cfa2469cbe12b52dd743f8a1b62.
55. Rock, DL . Thoughts on African swine fever vaccines. Viruses. (2021) 13:943. doi: 10.3390/v13050943
56. Frant, M, Woźniakowski, G, and Pejsak, Z. African swine fever (ASF) and ticks. No risk of tick-mediated ASF spread in Poland and Baltic states. J Vet Res. (2017) 61:375–80. doi: 10.1515/jvetres-2017-0055
57. Costard, S, Mur, L, Lubroth, J, Sanchez-Vizcaino, JM, and Pfeiffer, DU. Epidemiology of African swine fever virus. Virus Res. (2013) 173:191–7. doi: 10.1016/j.virusres.2012.10.030
58. Butler, JF, and Gibbs, EPJ. Distribution of potential soft tick vectors of African swine fever in the Caribbean region (Acari: Argasidae). Prev Vet Med. (1984) 2:63–70. doi: 10.1016/0167-5877(84)90049-7
59. Bowman, AS, Krogwold, RA, Price, T, Davis, M, and Moeller, SJ. Investigating the introduction of porcine epidemic diarrhea virus into an Ohio swine operation. BMC Vet Res. (2015) 11:1–7. doi: 10.1186/s12917-015-0348-2
60. Bonnet, SI, Bouhsira, E, De Regge, N, Fite, J, Etoré, F, Garigliany, MM, et al. Putative role of arthropod vectors in African swine fever virus transmission in relation to their bio-ecological properties. Viruses. (2020) 12:778. doi: 10.3390/v12070778
61. Eblé, PL, Hagenaars, TJ, Weesendorp, E, Quak, S, Moonen-Leusen, HW, and Loeffen, WLA. Transmission of African swine fever virus via carrier (survivor) pigs does occur. Vet Microbiol. (2019) 237:108345. doi: 10.1016/j.vetmic.2019.06.018
62. Mebus, C, Arias, M, Pineda, JM, Tapiador, J, House, C, and Sánchez-Vizcaíno, JM. Survival of several porcine viruses in different Spanish dry-cured meat products. Food Chem. (1997) 59:555–9. doi: 10.1016/S0308-8146(97)00006-X
63. Guinat, C, Gogin, A, Blome, S, Keil, G, Pollin, R, Pfeiffer, DU, et al. Transmission routes of African swine fever virus to domestic pigs: current knowledge and future research directions. Vet Rec. (2016) 178:262–7. doi: 10.1136/vr.103593
64. Gogin, A, Gerasimov, V, Malogolovkin, A, and Kolbasov, D. African swine fever in the North Caucasus region and the Russian Federation in years 2007–2012. Virus Res. (2013) 173:198–203. doi: 10.1016/j.virusres.2012.12.007
65. Pietschmann, J, Guinat, C, Beer, M, Pronin, V, Tauscher, K, Petrov, A, et al. Course and transmission characteristics of oral low-dose infection of domestic pigs and European wild boar with a Caucasian African swine fever virus isolate. Arch Virol. (2015) 160:1657–67. doi: 10.1007/s00705-015-2430-2
66. European Food Safety Authority (EFSA)Gervelmeyer, A . Public consultation on the draft data section on the ability of ASFV to survive and remain viable in different matrices of the scientific opinion on risk assessment of African swine fever and the ability of products or materials to present a risk to transmit ASF virus, No. 19. 7183E. (2022).
67. Jerina, K, Pokorny, B, and Stergar, M. First evidence of long-distance dispersal of adult female wild boar (Sus scrofa) with piglets. Eur J Wildl Res. (2014) 60:367–70. doi: 10.1007/s10344-014-0796-1
68. Turner, C, and Williams, SM. Laboratory-scale inactivation of African swine fever virus and swine vesicular disease virus in pig slurry. J Appl Microbiol. (1999) 87:148–57. doi: 10.1046/j.1365-2672.1999.00802.x
69. Chenais, E, Depner, K, Guberti, V, Dietze, K, Viltrop, A, and Ståhl, K. Epidemiological considerations on African swine fever in Europe 2014–2018. Porcine Health Manage. (2019) 5:6. doi: 10.1186/s40813-018-0109-2
70. Bloomfield, SF, Exner, M, Signorelli, C, Nath, KJ, and Scott, EA. The chain of infection transmission in the home and everyday life settings, and the role of hygiene in reducing the risk of infection. In: International scientific forum on home hygiene. (2012). Available at: http://www.ifhhomehygiene.org/IntegratedCRD.nsf/111e68ea0824afe1802575070003f039/9df1597d905889868025729700617093.
71. Bergmann, H, Schulz, K, Conraths, FJ, and Sauter-Louis, C. A review of environmental risk factors for African swine fever in European wild boar. Animals. (2021) 11:2692. doi: 10.3390/ani11092692
72. Bellini, S, Casadei, G, De Lorenzi, G, and Tamba, M. A review of risk factors of African swine fever incursion in pig farming within the European Union scenario. Pathogens. (2021) 10:84. doi: 10.3390/pathogens10010084
73. Zhou, X, Li, N, Luo, Y, Liu, YE, Miao, F, Chen, T, et al. Emergence of African swine fever in China, 2018. Transbound Emerg Dis. (2018) 65:1482–4. doi: 10.1111/tbed.12989
74. Mur, L, Boadella, M, Martínez-López, B, Gallardo, C, Gortazar, C, and Sánchez-Vizcaíno, JM. Monitoring of African swine fever in the wild boar population of the most recent endemic area of Spain. Transbound Emerg Dis. (2012) 59:526–31. doi: 10.1111/j.1865-1682.2012.01308.x
75. Oberin, M, Hillman, A, Ward, MP, Holley, C, Firestone, S, and Cowled, B. The potential role of wild suids in African swine fever spread in Asia and the Pacific region. Viruses. (2022) 15:61. doi: 10.3390/v15010061
76. Álvarez, J, Bicout, D, Boklund, A, Bøtner, A, Depner, K, More, SJ, et al. Research gap analysis on African swine fever. EFSA J. (2019) 17:e05811. doi: 10.2903/j.efsa.2019.5811
77. Ankhanbaatar, U, Sainnokhoi, T, Khanui, B, Ulziibat, G, Jargalsaikhan, T, Purevtseren, D, et al. African swine fever virus genotype II in Mongolia, 2019. Transbound Emerg Dis. (2021) 68:2787–94. doi: 10.1111/tbed.14095
78. Huang, J, and Bouis, H. Structural changes in the demand for food in Asia: empirical evidence from Taiwan. Agric Econ. (2001) 26:57–69. doi: 10.1111/j.1574-0862.2001.tb00054.x
79. Ding, Y, and Wang, Y. Big government: the fight against the African swine fever in China. J Biosaf Biosecur. (2020) 2:44–9. doi: 10.1016/j.jobb.2020.04.001
80. Frost, L, Tully, M, Dixon, L, Hicks, HM, Bennett, J, Stokes, I, et al. Evaluation of the efficacy of commercial disinfectants against African swine fever virus. Pathogens. (2023) 12:855. doi: 10.3390/pathogens12070855
81. Chen, W, Zhao, D, He, X, Liu, R, Wang, Z, Zhang, X, et al. A seven-gene-deleted African swine fever virus is safe and effective as a live attenuated vaccine in pigs. Sci China Life Sci. (2020) 63:623–34. doi: 10.1007/s11427-020-1657-9
82. Li, Y, Liu, Y, Xiu, F, Wang, J, Cong, H, He, S, et al. Characterization of exosomes derived from toxoplasma gondii and their functions in modulating immune responses. Int J Nanomedicine. (2018) 13:467–77. doi: 10.2147/IJN.S151110
83. Food and Agriculture Organization . A risk assessment for the introduction of African swine fever (2022). Available at: https://openknowledge.fao.org
84. Cagnasso, F., Maurella, C., Benvenuti, E., Borrelli, A., Bottero, E., Bruno, B., et al. Neutrophil-to-lymphocyte ratio, monocyte-to-lymphocyte ratio, platelet-to-lymphocyte ratio, albumin/globulin ratio, C-reactive protein/albumin ratio in dogs with inflammatory protein losing enteropathy. In: Atti 75 Convegno, p. 209. (2022). Available at: https://iris.unito.it/bitstream/2318/1878892/1/ATTI_DEF-SISVET.
85. Coetzer, JAW, Thomson, GR, and Tustin, RC. Infectious diseases of livestock with special reference to southern Africa. J S Afr Vet Assoc. (1995) 66:106.
86. Jori, F, and Bastos, AD. Role of wild suids in the epidemiology of African swine fever. EcoHealth. (2009) 2009:296–310. doi: 10.1007/s10393-009-0248-7
87. Faostat FAO . (2017). Available at: https://scholar.google.com.pk/scholar?hl=en&as_sd. (Accessed January 2018).
88. Tsakalidou, M . African swine fever (ASF): updates in current situation in Europe, clinical features, diagnosis, epidemiological considerations, control measures for the prevention and the eradication of the disease, challenges and future perspectives. (2021). Available at: https://repository.ihu.edu.gr/xmlui/handle/11544/29858.
89. Roth, J, and Cheryl Eia, JD. Factors to consider in a potential eradication plan for African swine fever in the United States: a white paper. (2023). Available at: www.cfsph.iastate.edu/Assets/ASF-eradication-plan-white-paper.pdf.
90. Cwynar, PJS, and Wlazlak, K. African swine fever status in Europe. Viruses. (2019) 11:310. doi: 10.3390/v11040310
91. Terpstra, C, and Wensvoort, G. African swine fever in the Netherlands. Tijdschr Diergeneeskd. (1986) 111:389–92.
92. Zakaryan, H, and Revilla, Y. African swine fever virus: current state and future perspectives in vaccine and antiviral research. Vet Microbiol. (2016) 185:15–9. doi: 10.1016/j.vetmic.2016.01.016
93. Authority, EFS, Boklund, A, Cay, B, Depner, K, Foldi, Z, Guberti, V, et al. Epidemiological analyses of African swine fever in the European Union (November 2017 until November 2018). EFSA J. (2018) 16:e05494. doi: 10.2903/j.efsa.2018.5494
94. Bosch-Camós, L, López, E, and Rodriguez, F. African swine fever vaccines: a promising work still in progress. Porcine Health Manage. (2020) 6:17. doi: 10.1186/s40813-020-00154-2
95. Sánchez-Vizcaíno, JM, Laddomada, A, and Arias, ML. African swine fever virus. Dis Swine. (2019) 2019:443–52. doi: 10.1002/9781119350927.ch25
96. Schambow, RA, Sampedro, F, Urriola, PE, Van de Ligt, JL, Perez, A, and Shurson, GC. Rethinking the uncertainty of African swine fever virus contamination in feed ingredients and risk of introduction into the United States. Transbound Emerg Dis. (2022) 69:157–75. doi: 10.1111/tbed.14358
97. Brown, VR, and Bevins, SN. A review of African swine fever and the potential for introduction into the United States and the possibility of subsequent establishment in feral swine and native ticks. Front Vet Sci. (2018) 5:11. doi: 10.3389/fvets.2018.00011
98. Godwin, EJO, Chandrasekaran, V, Smah, AC, and Faith, EO. Emergin.G infectious food system related zoonotic foodborne disease–a threat to global food safety and nutrition security In: EJO Godwin , editor. Foodborne pathogens-recent advances in control and detection. London: IntechOpen (2022)
99. Assavacheep, P, and Thanawongnuwech, R. Porcine respiratory disease complex: dynamics of polymicrobial infections and management strategies after the introduction of the African swine fever. Front Vet Sci. (2022) 9:1048861. doi: 10.3389/fvets.2022.1048861
100. Wamwatila, SN . Potential role of ornithodoros moubata in african swine fever epidemiology along kenya uganda border (Doctoral dissertation, University of Nairobi). (2015).
101. Bremang, A, Ho, HPJ, Conan, A, Tang, H, Oh, Y, and Pfeiffer, DU. Guidelines for African swine fever (ASF) prevention and control in smallholder pig farming in Asia: Farm biosecurity, slaughtering and restocking. Food & Agriculture Org. (2022)
102. Hamdy, FM, and Dardiri, AH. Clinical and immunologic responses of pigs to African swine fever virus isolated from the Western hemisphere. Am J Vet Res. (1984) 45:711–4.
103. Reis, AL, Goatley, LC, Jabbar, T, Sanchez-Cordon, PJ, Netherton, CL, Chapman, DA, et al. Deletion of the African swine fever virus gene DP148R does not reduce virus replication in culture but reduces virus virulence in pigs and induces high levels of protection against challenge. J Virol. (2017) 91:10–1128. doi: 10.1128/jvi.01428-17
104. Borca, MV, Ramirez-Medina, E, Silva, E, Vuono, E, Rai, A, Pruitt, S, et al. Development of a highly effective African swine fever virus vaccine by deletion of the I177L gene results in sterile immunity against the current epidemic Eurasia strain. J Virol. (2020) 94:10–1128. doi: 10.1128/JVI.02017-19
105. Sanford, B, Holinka, LG, O’donnell, V, Krug, PW, Carlson, J, Alfano, M, et al. Deletion of the thymidine kinase gene induces complete attenuation of the 16 Georgia isolate of African swine fever virus. Virus research. (2016) 2016:165–71.
106. O’Donnell, V, Holinka, LG, Gladue, DP, Sanford, B, Krug, PW, Lu, X, et al. African swine fever virus Georgia isolate harboring deletions of MGF360 and MGF505 genes is attenuated in swine and confers protection against challenge with virulent parental virus. Journal of virology. (2015) 89:6048–56. doi: 10.1128/jvi.00554-15
107. O’Donnell, V, Risatti, GR, Holinka, LG, Krug, PW, Carlson, J, Velazquez-Salinas, L, et al. Simultaneous deletion of the 9GL and UK genes from the African swine fever virus Georgia 2007 isolate offers increased safety and protection against homologous challenge. Journal of virology. (2017) 91:10–1128. doi: 10.1128/jvi.01760-16
108. Ramirez-Medina, E, Vuono, E, Rai, A, Pruitt, S, Espinoza, N, Velazquez-Salinas, L, et al. Deletion of E184L, a putative DIVA target from the pandemic strain of African swine fever virus, produces a reduction in virulence and protection against virulent challenge. J Virol. (2022) 96:e0141921–1. doi: 10.1128/JVI.01419-21
Keywords: African swine fever virus, pigs, ticks, recombinant vaccines, Ornithodoros
Citation: Alotaibi BS, Wu C-H, Khan M, Nawaz M, Chen C-C and Ali A (2024) African swine fever; insights into genomic aspects, reservoirs and transmission patterns of virus. Front. Vet. Sci. 11:1413237. doi: 10.3389/fvets.2024.1413237
Edited by:
Francesca De Falco, AREA Science Park, ItalyReviewed by:
Helen Roberts, Food and Rural Affairs, United KingdomFaisal Raza, Shanghai Jiao Tong University, China
Copyright © 2024 Alotaibi, Wu, Khan, Nawaz, Chen and Ali. This is an open-access article distributed under the terms of the Creative Commons Attribution License (CC BY). The use, distribution or reproduction in other forums is permitted, provided the original author(s) and the copyright owner(s) are credited and that the original publication in this journal is cited, in accordance with accepted academic practice. No use, distribution or reproduction is permitted which does not comply with these terms.
*Correspondence: Abid Ali, uop_ali@yahoo.com; Chien-Chin Chen, hlmarkc@gmail.com
†These authors have contributed equally to this work