- College of Animal Science and Technology, Qingdao Agricultural University, Qingdao, China
Recent studies have demonstrated that postbiotics possess bioactivities comparable to those of probiotics. Therefore, our experiment aimed to evaluate the effects of postbiotics derived from Enterococcus faecium on the growth performance and intestinal health of growing male minks. A total of 120 growing male minks were randomly assigned to 4 groups, each with 15 replicates of 2 minks. The minks in the 4 groups were fed a basal diet supplemented with 0 (control), 0.05, 0.1, and 0.15% postbiotics derived from E. faecium (PEF), respectively. Compared to the control, PEF improved feed/gain (F/G) during the first 4 weeks and the entire 8 weeks of the study (p < 0.05); in addition, 0.1% PEF improved average daily gain (ADG) during the first 4 weeks and the entire 8 weeks of the study (p < 0.05), while 0.15% PEF improved ADG during the first 4 weeks of the study (p < 0.05). Consequently, 0.1% PEF minks displayed greater body weight (BW) at weeks 4 and 8 (p < 0.05), and 0.15% PEF minks had greater BW at week 4 (p < 0.05) than minks in the control. Furthermore, compared to the control, both 0.05 and 0.1% PEF enhanced the apparent digestibility of crude protein (CP) and ether extract (EE) (p < 0.05) in the initial 4 weeks, while both 0.1 and 0.15% PEF enhanced the apparent digestibility of CP and DM in the final 4 weeks (p < 0.05). Additionally, trypsin activity was elevated in the 0.1 and 0.15% PEF groups compared to the control (p < 0.05). In terms of intestinal morphology, PEF increased the villus height and villus/crypt (V/C) in the jejunum (p < 0.05), and both 0.1 and 0.15% PEF decreased the crypt depth and increased the villus height and V/C in the duodenum (p < 0.05) compared to the control group. Supplementation with 0.1% PEF increased the SIgA levels but decreased the IL-2, IL-8, and TNF-α levels in the jejunum (p < 0.05). Compared to the control, E. faecium postbiotics decreased the relative abundances of Serratia and Fusobacterium (p < 0.05). In conclusion, the results indicate that the growth performance, digestibility, immunity, and intestine development of minks are considerably affected by E. faecium postbiotics. In particular, dietary supplementation with 0.1% E. faecium postbiotics provides greater benefits than supplementation with 0.05 and 0.15%.
1 Introduction
Minks have been domesticated for approximately 100 years (1). On commercial mink farms, intestinal diseases such as enteritis and diarrhea are a considerable threat to the health, growth, and survival of minks during their developing period (2). Traditionally, antibiotics have been widely used to prevent these intestinal diseases (3), which promote the growth of the animals. However, the misuse of antibiotics has resulted in the emergence of antibiotic-resistant bacteria and genes, reducing their therapeutic efficacy against diseases in both humans and animals (4). Consequently, many countries have prohibited the use of antibiotics for growth promotion in animal feed (5). With the implementation of this ban, the search for viable alternatives to antibiotics has increasingly attracted attention.
Enterococcus faecium is a lactic acid bacterium recognized and approved for use as a direct-fed microbial by the Ministry of Agriculture (MOA) in China (6). Some studies have demonstrated that E. faecium is beneficial as a feed additive for improving growth performance (7), digestibility (8), and immunity (9), while reducing diarrhea occurrence (7) and alleviating salmonella infection (9, 10). However, some strains of E. faecium have been identified as opportunistic pathogens with resistance to many antibiotics (11, 12). Consequently, concerns have been raised regarding the safety of E. faecium as a probiotic. Furthermore, ensuring the stringent storage and transportation conditions necessary for lactic acid bacteria presents a significant challenge (13). As some studies have demonstrated that the viability of bacteria is not essential for all probiotic effects, the inactivated microorganisms and their derived fractions, termed postbiotics by the International Scientific Association for Probiotics and Prebiotics (ISAPP) (14), possess bioactivities comparable to those of live probiotic bacteria (15, 16). The beneficial impact of the probiotic is partly due to the various metabolites generated by viable probiotics (17). Therefore, it is reasonable to expect that E. faecium postbiotics may offer more advantages than E. faecium probiotics due to their higher safety and stability (18).
At present, the probiotic effects of E. faecium on livestock and poultry production have been extensively documented (19–21). However, there is limited research on postbiotics derived from E. faecium, especially in mink. The present experiment was conducted to evaluate the effects of the postbiotics derived from mink-origin E. faecium on male minks by analyzing growth performance, nutrient apparent digestibility, digestive enzyme activity, intestinal morphology, intestinal mucosal immunity, and gut microbiota composition.
2 Materials and methods
2.1 Ethics approval
The Animal Care and Use Committee of Animal Science and Technology at Qingdao Agricultural University reviewed and approved the experimental protocol (DKY20230524-2). This study was conducted in accordance with the ARRIVE 2.0 guidelines.
2.2 Enterococcus faecium postbiotics
The strain of E. faecium was previously isolated from the rectal contents of mink, identified by 16S rRNA gene sequence analysis, and preserved in the China General Microbiological Culture Collection Center (No. 29262). The 16S rRNA gene sequence was deposited in the National Center for Biotechnology Information (NCBI) database under the accession number PP886227. The isolated strain of E. faecium was inoculated in MRS medium and cultured at 37°C for 24 h. The viable E. faecium in the suspension was more than 107 cfu/mL by colony count. The E. faecium in suspension was inactivated by heat, and then the postbiotics derived from E. faecium were obtained. The E. faecium postbiotic sample was subjected to chromatographic separation using a SHIMADZU-LC30 ultra-high-performance liquid chromatography (UHPLC) system, equipped with an ACQUITY UPLC® HSS T3 (2.1 × 150 mm, 1.8 μm) (Waters, Milford, MA, United States) column. Following the UHPLC separation, the sample was analyzed using mass spectrometry with a QE Plus mass spectrometer (Thermo Scientific). Metabolomic analysis indicated that E. faecium postbiotics contained 28.71% organic acids and their derivatives as well as 19.01% lipids and lipid-like molecules.
2.3 Animals and experimental design
The experiment was carried out on a commercial mink farm in Haiyang, Yantai. A total of 120 male minks (Regal White) at 12 weeks of age with an initial body weight (IBW) of 1281.52 ± 5.98 g were randomly assigned to 4 numerically equal groups. Each group consisted of 15 replicates with 2 minks in each replicate. The minks in the 4 groups were fed a basal diet with E. faecium postbiotics at 0, 0.05, 0.1, and 0.15% of the diet, respectively. Our previous study (22) has demonstrated that postbiotics derived from another Lactobacillus species exhibit probiotic effects in minks at comparable levels of supplementation. So, similar levels of E. faecium postbiotics supplementation were adopted in this study. The experiment lasted 8 weeks following a 1-week adaptation period.
2.4 Diet and management
All minks were housed in a two-row shelter with two open sides. Two minks were kept in a cage with the dimensions of 30 × 75 × 45 cm3 (width × depth × height). Each cage was equipped with a wooden nest box (30 cm × 30 cm × 30 cm, L × W × H) with a metal mesh ceiling. Minks had free access to the home cage and nest box via the entrance. During the trial period, the health status of the minks was checked twice daily, and any minks with poor health or compromised welfare were promptly removed from the study. During the period of the study, minks were fed twice per day. The paste diets were formulated from sea fishes and byproducts, chicken byproducts, and egg products. The composition of the experimental diets and the nutrient levels are presented in Table 1. Each cage was equipped with one drinker, and minks had ad libitum access to drinking water by the drinker. The ambient temperature was maintained at 26.24°C (± 0.05), relative humidity was 65.27% (± 0.05), and the light schedule was natural light regime throughout the study.
2.5 Samples and data collection
2.5.1 Evaluation of growth performance
Animals were individually weighed at the beginning (week 0), week 4, and week 8 of the study to determine the initial (week 0), week 4, and final (week 8) body weight. The average daily gain (ADG) of minks was calculated. During the experimental period, the feed supplied and leftovers were accurately weighed and recorded over 3 days per week. The average daily feed intake (ADFI) and feed/gain (F/G) of minks were calculated for each mink individually.
2.5.2 Digestive experiment
A digestive experiment using the endogenous indicator method was performed to evaluate the apparent digestibility of nutrients at weeks 3 and 7 of the experiment. Fecal samples were collected via an inclined stainless steel plate hung under the cage. A total of 24 uncontaminated fecal samples in the four groups (with six replicates in each group) were sampled to approximately 200 g over 3 days, respectively. The 3-day fecal samples were mixed and then kept at −20°C until analysis. Meanwhile, the diets of each group were sampled daily during the 3 days before feeding the minks, then pooled to obtain representative samples, and stored at −20°C until analysis. The diet and fecal samples were air-dried at 65°C to obtain the initial moisture content. All air-dried samples were ground and passed through a 40-mesh sieve. Ground diet and fecal samples were analyzed for dry matter (DM) (GB/T 6435–2014), crude ash (GB/T 6438–2007), hydrochloric acid insoluble ash (GB/T 23742–2009), crude protein (CP) (GB/T 6432–2018), and ether extract (EE) (GB/T 6433–2006).
Where A1 is the content of hydrochloric acid-insoluble ash in the diet, A2 is the content of hydrochloric acid-insoluble ash in the fecal samples, B1 is the content of a certain nutrient in the diet, and B2 is the content of a certain nutrient in the fecal sample.
2.5.3 Collection and detection of intestinal samples
At the end of the study (week 8), minks (n = 8) from each group were randomly selected and euthanized. Approximately 2–5 g of contents of the duodenum, 5 cm sections of the duodenum and jejunum, 2–5 g of the jejunum mucosal tissue, and a rectal mucosal swab were sampled per mink.
The contents of the duodenum were centrifuged at 3500 ×g at 4°C for 10 min. The supernatant was used to measure the activity of α-amylase, trypsin, and lipase using assay kits (Jiancheng Bioengineering Research Institute, Nanjing, China).
The duodenum and jejunum samples were rinsed with saline and then placed into 4% paraformaldehyde fixative. After rinsing with flowing water for 24 h, the samples were dehydrated through a graded series of ethanol solutions, cleared with xylene, and embedded in paraffin wax. The samples were sectioned into 5-μm-thick sections, stained with hematoxylin and eosin (H&E), mounted with coverslips, and sealed with neutral resin for subsequent histological evaluation. The villus height and crypt depth were visualized under a light microscope (Carl Zeiss, Germany), and the images captured were analyzed using the software ZEN 2011 (Blue version). The villus height was determined from the tip of the villus to the villus–crypt junction, while the crypt depth was measured from the base of the crypt to the same junction. For each sample, the average of villus heights and crypt depths was calculated from 9 measurements taken at 3 discontinuous fields (50×), with 3 measuring points per field (23). Subsequently, the villus height to crypt depth ratio (V/C) was calculated (24).
The jejunum mucosal tissue of the mink was taken, diluted with 0.9% saline (1:9 w/v), and homogenized. The homogenate was centrifuged at 3500 ×g at 4°C for 10 min to obtain the supernatant, which was then analyzed for immune components, including SIgA and cytokines (IL-1β, IL-8, IL-10, IL-2, IL-6, IL-12, TNF-α, and IFN-γ). These indicators were detected using the Enzyme-Linked Immunosorbent Assay (ELISA) Kit (Jiancheng Bioengineering Research Institute, Nanjing, China), and the OD values were measured at a wavelength of 450 nm using a full-spectrum microplate reader (Tecan Austria GmbH, Grodig, Austria).
The total DNA of the rectal mucosa samples of mink was extracted using the Fast DNA Spin Kit for Soil (MP, Santa Ana, CA, United States) (22). The extracted genomic DNA was detected by 1% agarose gel electrophoresis. The primers 338F (5’-ACTCCTACGGGAGGCAGCAG-3′) and 806R (5’-GGACTACHVGGGTWTCTAAT-3′) were used to amplify the V3-V4 region of the 16S rRNA gene. PCR amplification was performed on an ABI Gene Amp PCR system 9,700 thermal cycler with a program consisting of an initial denaturation at 95°C for 3 min, followed by 27 cycles of denaturation at 95°C for 30 s, annealing at 55°C for 30 s, and extension at 72°C for 30 s, concluding with a final extension at 72°C for 10 min (25). The amplicons were excised from the 2% agarose gel, purified using the AxyPrep DNA Gel Extraction Kit (Axygen, Union City, CA, United States), and tested by 2% agarose gel electrophoresis. Quantification was performed using the QuantiFluor™-ST Blue fluorescence quantification system (Promega, Madison, WI, United States). A PE 300 library was constructed based on the Illumina MiSeq platform and sequenced using the Illumina MiSeq PE 300 platform (26).
2.6 Statistical analysis
The data on growth performance, nutrient digestibility, digestive enzyme activity, intestinal morphology, and jejunum mucosal immune components were expressed as means ± standard error (SE) and were analyzed using one-way ANOVA with SPSS 25.0 (SPSS Institute Inc., Chicago, USA). A p < 0.05 means a significant difference. Duncan’s tests were used to analyze differences between groups.
The intestinal flora data were analyzed on the I-Sanger cloud platform. FLASH 1.2.11 software was used for pair-end double-ended sequence splicing. The Spearman correlation coefficient was adopted to analyze the correlation between the intestinal flora and the immunity of minks.
3 Results
3.1 Effect of PEF on growth performance
The postbiotics of E. faecium had significant effects on BW, ADG, and F/G of minks during the study (p < 0.05; Table 2). Compared to the control minks, the minks in the 0.15% PEF group were heavier (p < 0.05) at week 4 of the study and had greater ADG (p < 0.05) during the initial 4 weeks, while the minks in the 0.1% PEF group were heavier (p < 0.05) at weeks 4 and 8 of the study and had greater ADG (p < 0.05) during the initial 4 weeks and the entire 8 weeks of the study. The minks in the PEF groups had less F/G (p < 0.05) than the minks in the control group during the initial 4 weeks and the entire 8-week period of the study.
3.2 Effect of PEF on nutrient apparent digestibility
Enterococcus faecium postbiotics had significant effects on the apparent digestibility of CP, EE, and DM (p < 0.05, Table 3). Compared to the control, 0.05% PEF significantly improved digestibility of CP (p < 0.05) and EE (p < 0.05) during the initial 4 weeks, 0.1% PEF increased digestibility of CP (p < 0.05) and EE (p < 0.05) during the first 4 weeks as well as increased digestibility of CP (p < 0.05) during the final 4 weeks, and 0.15% PEF increased digestibility of CP (p < 0.05) and DM (p < 0.05) during the final 4 weeks. In addition, the 0.15% PEF group had greater digestibility of DM (p < 0.05) than the 0.05% PEF group during the final 4 weeks.
3.3 Effect of PEF on digestive enzyme activities
Enterococcus faecium postbiotics had significant effects on trypsin activity (p < 0.05, Table 4). Compared to the control, both 0.1 and 0.15% PEF significantly increased trypsin activity (p < 0.05).
3.4 Effect of PEF on intestinal morphology
Enterococcus faecium postbiotics had significant effects on intestinal morphology indicators (p < 0.05; Table 5; Figure 1). Compared to the control, 0.1% PEF increased the villus height and V/C in both the duodenum and jejunum (p < 0.05) and decreased the crypt depth (p < 0.05) in the duodenum, 0.15% PEF increased the villus height of jejunum and the V/C in both the duodenum and jejunum (p < 0.05) and similarly decreased the crypt depth in the duodenum (p < 0.05), and 0.05% PEF increased the villus height and the V/C in the jejunum (p < 0.05). In addition, the 0.1 and 0.15% PEF minks had less crypt depth of duodenum than the minks in the 0.05% PEF group (p < 0.05).
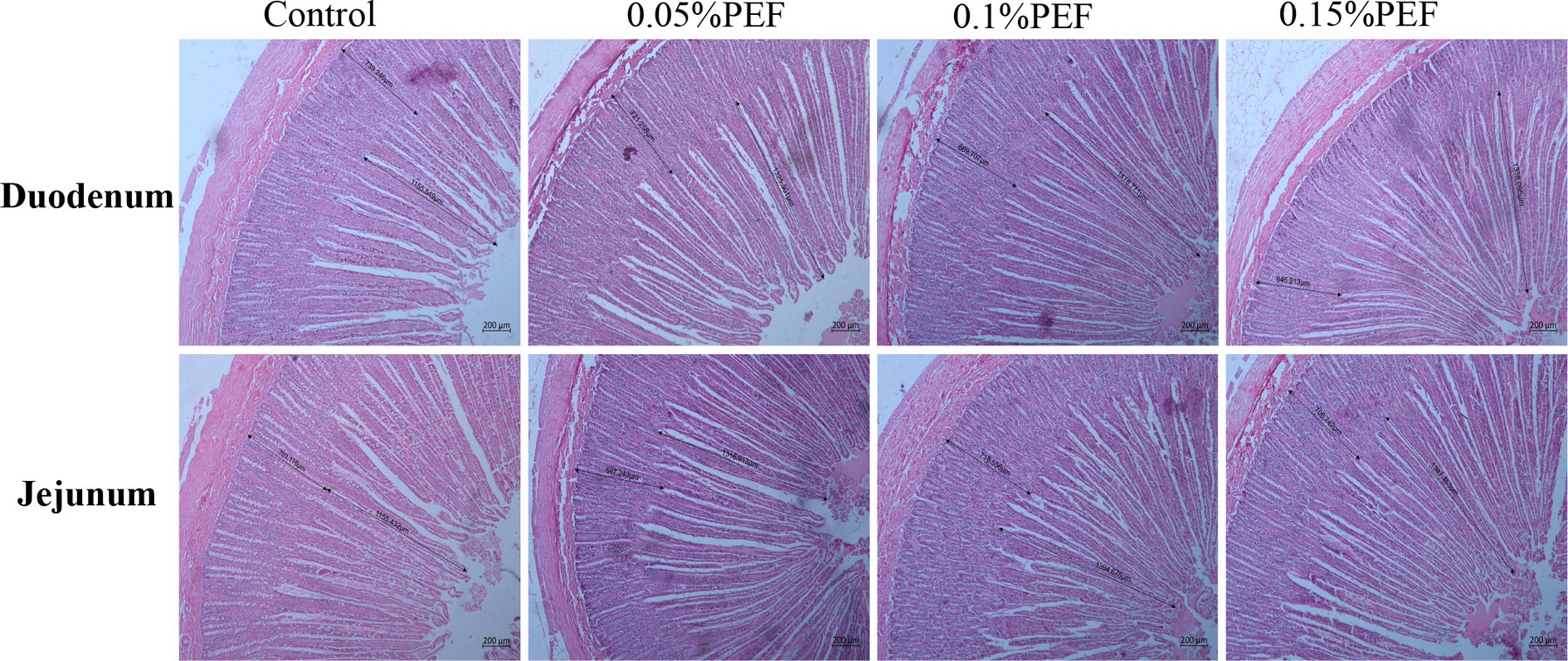
Figure 1. Effect of Enterococcus faecium postbiotics on duodenum and jejunum tissue sections of growing male minks (n = 8). Pictures were observed at 50 × magnification. The villus height was measured from the tip of the villus to the villus–crypt junction. The crypt depth was measured from the base of the crypt to villus–crypt junction.
3.5 Effect of PEF on jejunum mucosal immunity indexes
Enterococcus faecium postbiotics had significant effects on the levels of SIgA, IL-2, IL-8, IL-10, and TNF-α in the jejunum mucosa (p < 0.05, Table 6). Compared to the control, 0.05% PEF decreased IL-2 (p < 0.05) and TNF-α (p < 0.05) levels, 0.1% PEF increased SIgA levels (p < 0.05) and decreased IL-2 (p < 0.05), IL-8 (p < 0.05), and TNF-α levels (p < 0.05), and 0.15% PEF decreased IL-2 levels (p < 0.05). Compared to the 0.1% PEF minks, the 0.05% PEF minks had less IL-10 levels (p < 0.05), and the 0.15% PEF minks had greater IL-8 levels (p < 0.05).
3.6 Effect of PEF on intestinal flora
The 16S rRNA sequence was assigned as an OTU with at least 97% sequence similarity. As shown in Figure 2, the end of the curve tends to be flat, indicating that the amount of sequencing data is reasonable, and all samples have sufficient sequencing depth. There were no differences among the four groups in the ACE, Chao, Shannon, Simpson, and Sobs indexes (p > 0.05, Figure 3).
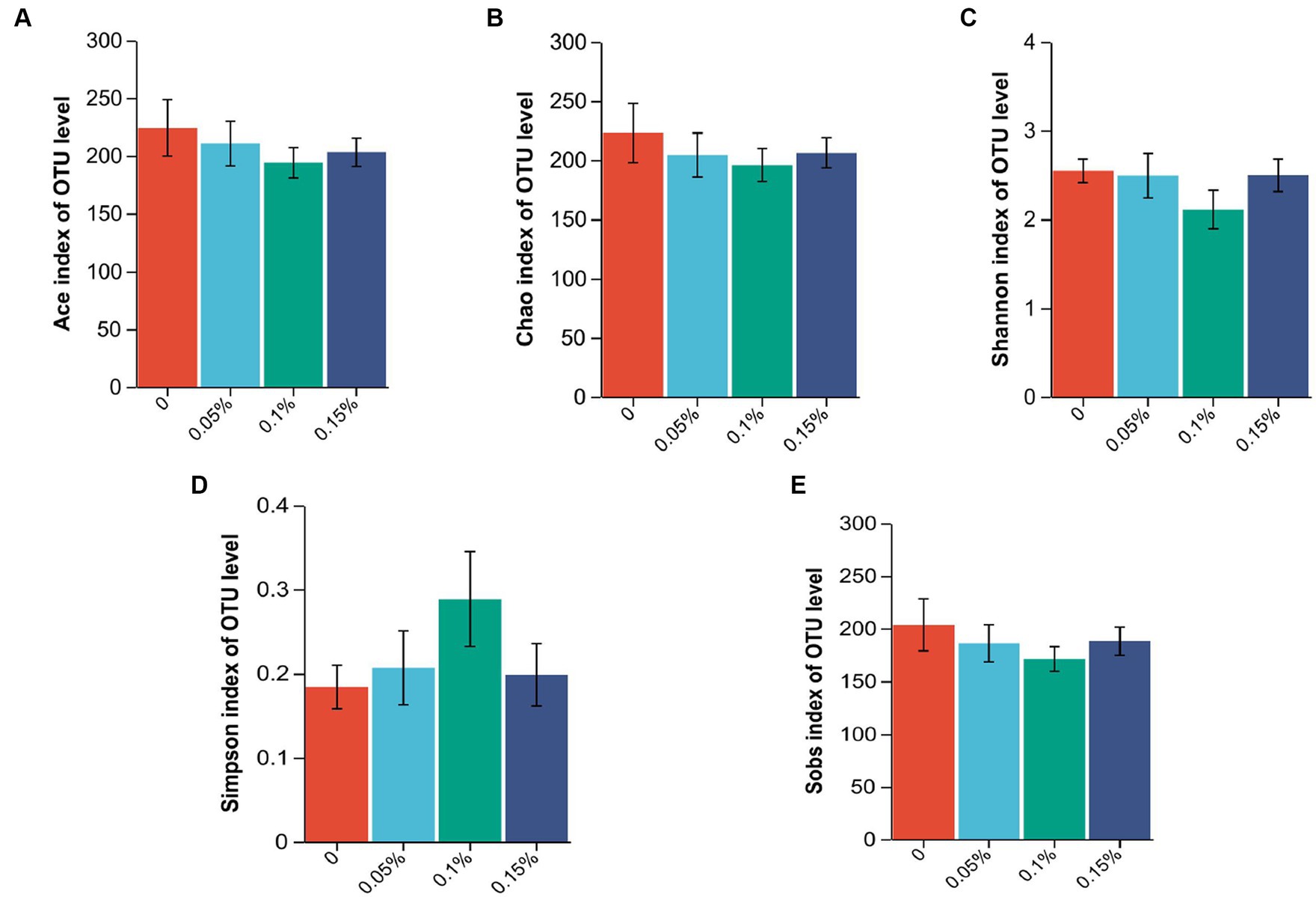
Figure 3. Effect of Enterococcus faecium postbiotics on alpha diversity indices of the growing male minks in different groups (n = 8). (A) Ace index, (B) Chao index, (C) Shannon index, (D) Simpson index, and (E) Sobs index.
At the phylum level, Firmicutes, Proteobacteria, and unclassified_k__norank_d__Bacteria were consistently the most abundant phyla in the four groups, which together constituted more than 96.00% of the gut microbiota (Figure 4A). At the genus level, the data obtained confirmed that Mycoplasma, unclassified_k__norank_d__Bacteria, Lactococcus, Sphingobium, and Acinetobacter were the top five dominant genera in the control group and the 0.15% PEF group (Figure 4B). Paeniclostridium, unclassified_k__norank_d__Bacteria, Candidatus_Arthromitus, Sphingobium, and Acinetobacter were the top five dominant genera in the 0.05% PEF group. Mycoplasma, unclassified unclassified_k__norank_d__Bacteria, Candidatus_Arthromitus, Acinetobacter, and Sphingobium were the top five dominant genera in the 0.1% PEF group. Further analysis of bacterial taxa among the groups indicated that the control group had a significantly higher abundance of Serratia than the other groups (p < 0.05, Figure 4C). In addition, the 0.15% PEF group displayed the highest richness of Fusobacterium than other groups (p < 0.05).
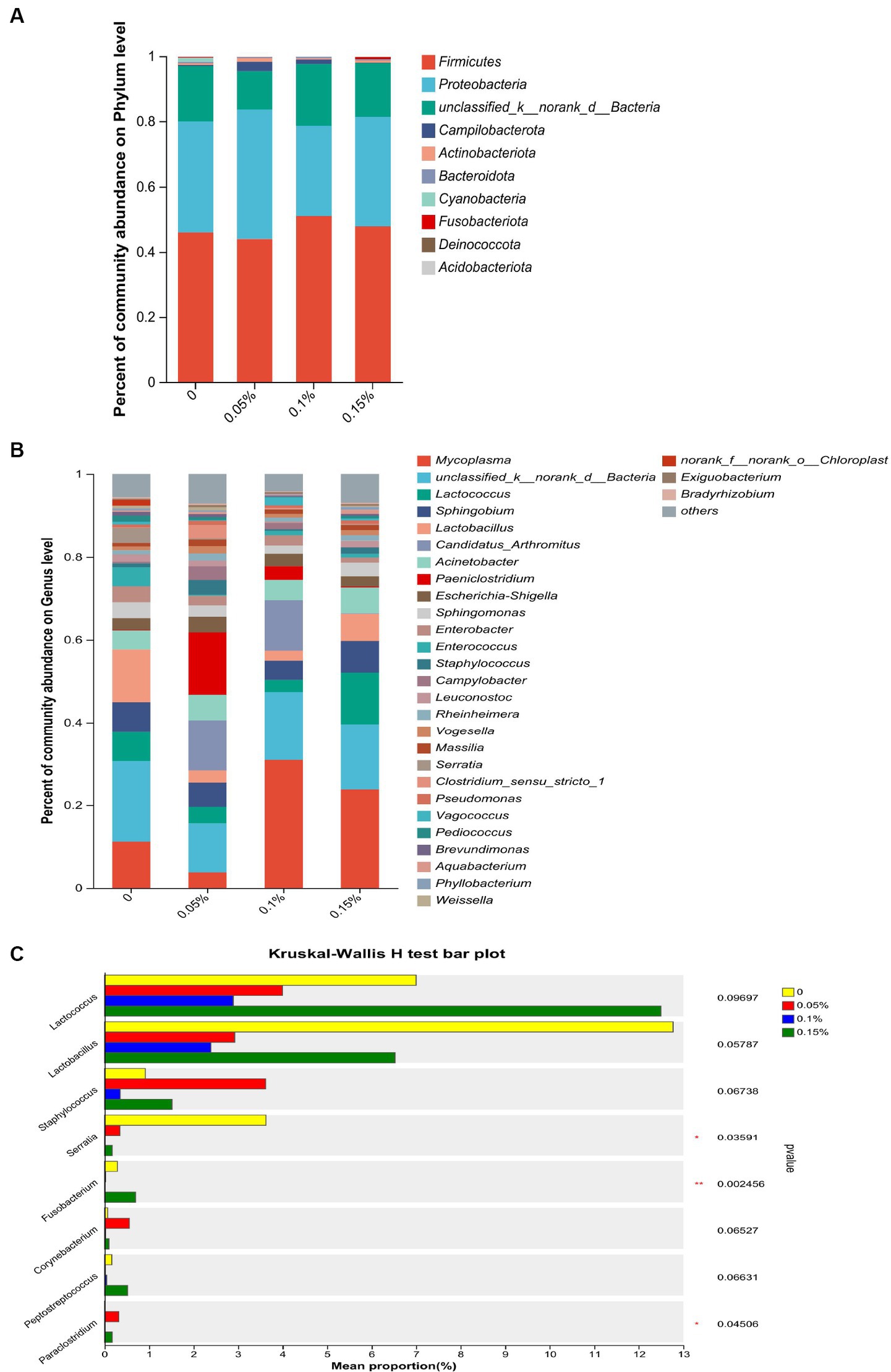
Figure 4. Effect of Enterococcus faecium postbiotics on gut microbiota composition of growing male minks. (A) Distribution of bacterial community structure at the phylum level (n = 8). (B) Distribution of bacterial community structure at the genus level (n = 8). (C) The significance of differences among the four groups of the same species (*represents p < 0.05, and ** represents p < 0.01). The result was statistically analyzed through non-parametric Kruskal–Wallis tests (n = 8).
3.7 Correlation analysis of gut microbiota and immunity
The Spearman correlation heatmap results showed that Acinetobacter had negative correlations with IL-1β, IL-10, TNF-α, and IFN-γ (p < 0.05, Figure 5). Sphingobium had a negative correlation with IL-1β (p < 0.05). Sphingomonas had negative correlations with IL-1β and IL-10 (p < 0.05). Clostridium_sensu_stricto_1 had a negative correlation with SIgA (p < 0.05). Staphylococcus correlated positively with IL-2 (p < 0.05). Lactococcus correlated positively with TNF-α and negatively with SIgA (p < 0.05).
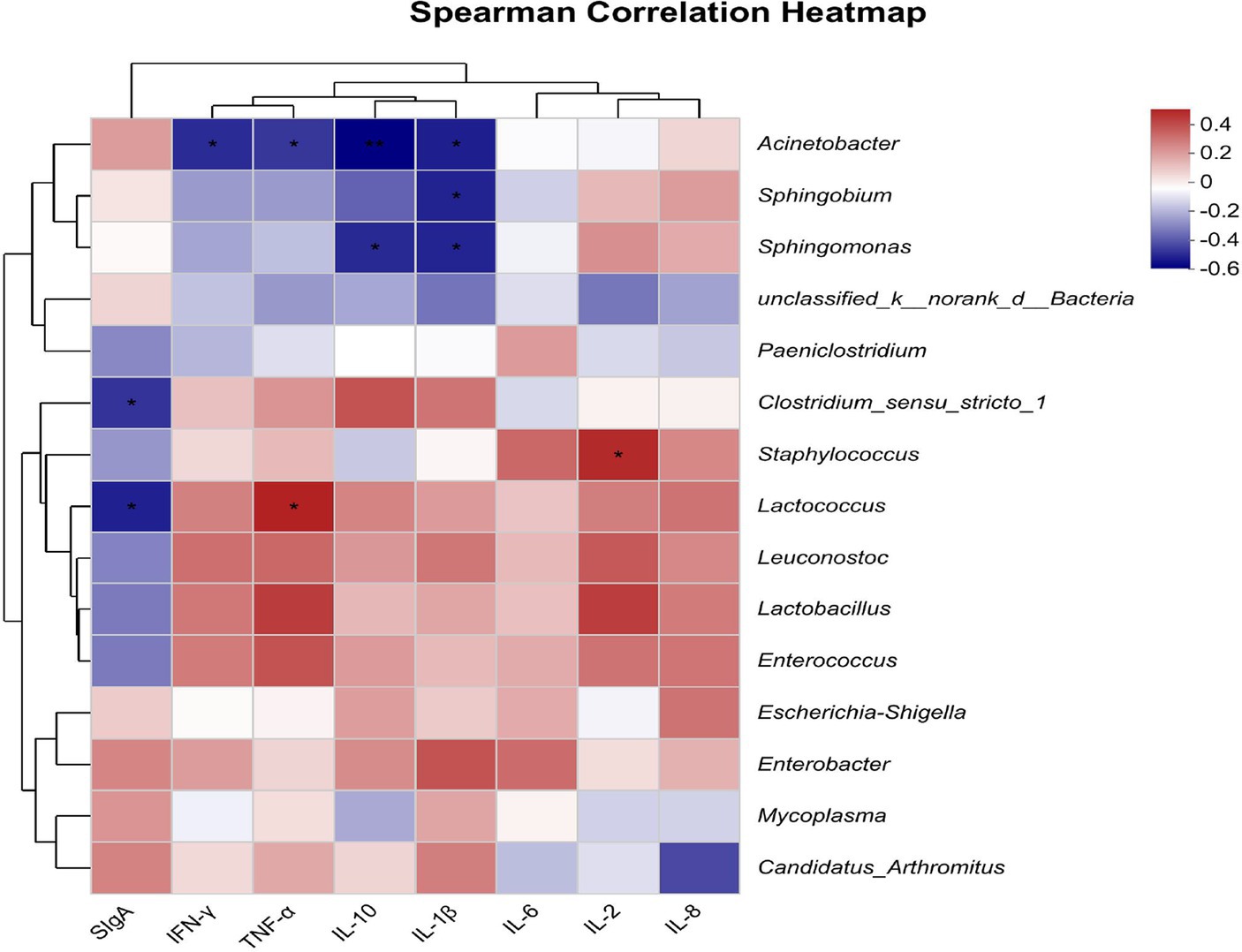
Figure 5. Heatmap shows the correlation between intestinal flora (genus level) and intestinal immune indicators (n = 8). The X-axis and Y-axis are intestinal immune indicators and species, respectively, and the correlation R-values and p-values are obtained through calculation. R-values are displayed in different colors in the figure. If the p < 0.05, they are marked with *. The legend on the right is the color range of different R-values; the left and upper sides present the species and immune indicator cluster trees; *represents p < 0.05, and **represents p < 0.01.
4 Discussion
The objective of this study was to investigate whether postbiotics derived from E. faecium could have potential probiotic effects on growing minks. This study demonstrated that E. faecium postbiotics improved the growth performance of male minks. The results were consistent with previous studies of E. faecium on piglets (24, 27) and broilers (28). In the current study, the increased ADG was associated with improvement of F/G, indicating that the minks in the E. faecium postbiotics groups were efficient in utilizing dietary nutrients for growth. This could be due to the E. faecium postbiotics containing many functional compounds such as short-chain fatty acids (SCFA), microbial fractions, functional proteins, secreted polysaccharides, extracellular polysaccharides (EPSs), cell lysates, and teichoic acid (29), which could improve immune function (30), inhibit pathogenic bacteria (31), promote the development of intestinal villi (32), enhance the activities of intestinal digestive enzymes (33), and improve the efficiency of nutrient utilization (24). Consequently, the growth performance of the animals was improved. The study findings confirmed that postbiotics derived from E. faecium at 0.1 and 0.15% were effective in enhancing both the ADG and feed efficiency of minks. In contrast, 0.05% E. faecium postbiotics improved feed efficiency without effect on ADG, thereby demonstrating a dose-dependent effect of the postbiotics. These results suggest that a higher dosage of postbiotic supplementation is necessary to achieve improvements in ADG. However, these improvements were only evident in the initial 4 weeks. The findings indicate that E. faecium postbiotics may have short-term effects on growth performance.
In the current study, E. faecium postbiotics increased the apparent digestibility of CP, EE, and DM. The results were consistent with previous studies on pigs, which showed that E. faecium improved nutrient digestibility (34, 35). Chen et al. (36) also found that E. faecium could improve the digestibility of DM in pigs. However, it is interesting to note that 0.05 and 0.15% E. faecium postbiotics only improved the apparent digestibility of CP in minks during the initial and last 4 weeks of the study, respectively. In contrast, 0.1% E. faecium postbiotics enhanced the apparent digestibility of CP throughout the study period. This suggests that 0.1% E. faecium postbiotics are the optimal dosage for improving the apparent digestibility of CP in growing minks. Omar et al. (37) suggested that the digestive enzyme activities contributed to feed utilization associated with the growth performance of animals. This may be due to the enhancement of digestive enzyme activity. Digestive enzymes break down nutrients into smaller molecules, facilitating their absorption by the animal (38). Lipases hydrolyze triglycerides into glycerol and long-chain fatty acids (39), amylase breaks down starches into monosaccharides such as glucose, and protease degrades proteins into peptides and amino acids (40). As a carnivore, the mink has a high capacity for fat digestion but a limited capacity for carbohydrate digestion due to the low activity of α-amylase (41). Furthermore, the mink has a higher protein requirement than other domestic animals (42). The increased activity of trypsin likely facilitated protein digestibility in this study. The results were consistent with the findings of previous research on fish (43) and broilers (44, 45), which showed that dietary supplementation with probiotics could increase digestive enzyme activity. It is probable that short-chain fatty acids (SCFAs) present in E. faecium postbiotics help maintain a healthy intestinal environment conducive to the optimal functioning of digestive enzymes. Consequently, this enhancement in nutrient digestibility improves the growth performance of the animal (46).
Furthermore, the study revealed that 0.1% E. faecium postbiotics increased the villus height and the V/C in both the duodenum and jejunum of minks and decreased the crypt depth in the duodenum. Several previous studies have reported that E. faecium probiotic or heat-killed E. faecium significantly increased the villus height (19, 47) and V/C (24) and decreased the crypt depth (48). The measurements of the villus height and crypt depth are indicative of gut health and function (49). To a certain extent, increases in the villus height and reductions in the crypt depth enhance digestibility and absorptivity (50). The V/C indicates the integrity of the intestinal mucosa and is associated with digestion and absorption capacity (51). These findings further elucidate that E. faecium postbiotics contribute to the promotion of intestinal development, thereby improving the digestibility of nutrients in minks.
In this study, 0.1% E. faecium postbiotics was observed to reduce IL-8, IL-2, and TNF-α levels and increase sigma levels in jejunum mucosa. However, 0.05 and 0.15% E. faecium postbiotics had no significant effect on the levels of IL-8 and sigma. The findings suggest that 0.1% E. faecium postbiotics are more effective in modulating intestinal immunity compared to the 0.05 and 0.15% supplementation. The intestinal mucosal immune system comprises lymph nodes, lamina propria, and epithelial cells, which constitute a protective barrier for maintaining intestinal integrity (52). M cells secrete SIgA through the polymeric immunoglobulin receptor in the crypts, effectively defending against the invasion of pathogens and commensal microorganisms (53, 54). As the predominant immunoglobulin in the intestine, Shiga provides immune protection to prevent the penetration of microorganisms and mucosal antigens into the mucosal barrier through immune exclusion (55). The experimental results indicated that the SIgA levels initially increased and subsequently declined with the increasing supplementation of E. faecium postbiotics. This suggests that E. faecium postbiotics may stimulate polymeric immunoglobulin receptor expression by activating pattern recognition receptors on intestinal epithelial and immune cells and increasing the concentration of SIgA in the intestinal lumen (53). While postbiotics can stimulate the immune system of the host and enhance SIgA production within an optimal dosage range, excessively high supplementation might trigger an immune suppressive or resistance, potentially resulting in a reduction of SIgA levels (56).
The intestinal epithelium can generate cytokines including IL-2, IL-8, and TNF-α, which are closely involved in triggering the inflammatory response (57). Maintaining the balance between pro-inflammatory and anti-inflammatory cytokines is essential for regulating intestinal inflammation (58). A previous study on piglets confirmed that supplementation with E. faecium reduced the relative expression of the IL-8 gene and the level of TNF-α in the jejunum mucosa and increased the relative expression of the IL-10 and TGF-β genes in the ileum mucosa (59). Similar results in macrophage have been reported, indicating that both live and heat-killed E. faecium promote IL-10 secretion and inhibit TNF-α release, respectively (60). In this study, the results indicated that E. faecium postbiotics could regulate immunity and inflammatory responses. A previous study confirmed that postbiotics derived from E. faecium SF68 could reversibly inhibit the activation of the NF-κB and JNK signaling pathway in intestinal epithelial cells and counteract the effects of bacterial and other toll-like receptors (TLRs) (61). Therefore, it is suggested that the E. faecium postbiotics likely regulate the immune functions of male minks by inhibiting the activation of the NF-κB and JNK signaling pathways.
The gut microbiota is associated with the metabolism, immunity, digestibility, and health of the host (62). Establishing and maintaining beneficial interactions between the host and microbiota is important to maintain host health (63). However, the results of alpha diversity in the current study did not show any effect of E. faecium postbiotics on the enrichment and diversity of the gut microbiota. In contrast, a previous study on piglets demonstrated that probiotic E. faecium increased the Sobs, Chao, ACE, and Shannon indexes and decreased the Simpson index from days 1 to 14 (20). This may be due to colonization of viable E. faecium on the intestinal mucosa, which contributed to the enhancement of community richness (64). In agreement with previous studies on mink, Firmicutes and Proteobacteria were identified as the most dominant phyla on the rectal mucosa in male minks, consistent with observations in the colon (65) and feces (66) of mink. In fact, Firmicutes and Proteobacteria are widely found in the gastrointestinal tract of carnivores such as otters and raccoon dogs (67). At the genus level, we observed changes in the abundance of flora. The relative abundance of Serratia was reduced in all PEF groups, and Fusobacterium showed a decrease in 0.05 and 0.1% PEF groups, respectively. Serratia marcescens, a member of the Serratia (68), is an opportunistic pathogen related to respiratory, urinary, and digestive tract infections (69). Fusobacterium is a gram-negative anaerobic bacterium that is typically found as part of the normal flora in the oral cavity and gastrointestinal tract (70). Several members of the Fusobacterium genus are opportunistic pathogens that can cause bacteremia and acute infections (71). These results illustrate that E. faecium postbiotics may prevent harmful bacteria from adhering to the intestinal mucosa and reduce the occurrence of inflammation.
The interaction between the intestinal microbial flora and immunity has been extensively described in many published reports (22, 72, 73). Our study specifically investigated the interaction between specific intestinal microbiota genera and gut immune indicators. We discovered a positive association between the genus Lactococcus and the inflammatory marker TNF-α, and an inverse relationship with SIgA. These findings suggest that Lactococcus may not contribute positively to mink health. The intestinal microbiome can protect the integrity of the mucosal barrier by acting on the host immune system (72), thus inhibiting the occurrence of intestinal inflammation. In addition, several previous studies suggested that alterations in microbiota may lead to immune-mediated diseases because microbial communities affect barrier surfaces as well as remote organs, including the lungs and skin (74, 75).
5 Conclusion
The study findings confirm that postbiotics derived from E. faecium exhibit probiotic effects on growing male minks. In particular, dietary supplementation with 0.1% E. faecium postbiotics improves growth performance (ADG and F/G during the initial 4 weeks and the entire 8 weeks of the study), the apparent digestibility of nutrients (CP, EE, and DM), and impacts immune status and intestinal morphology in the minks. Therefore, it can be concluded that supplementation with 0.1% provides greater benefits than supplementation with 0.05 and 0.15%.
Data availability statement
The data presented in the study are deposited in the National Center for Biotechnology Information (NCBI) repository, accession number PP886227.
Ethics statement
The animal study was approved by the Animal Care and Use Committee of Animal Science and Technology at Qingdao Agricultural University. The study was conducted in accordance with the local legislation and institutional requirements.
Author contributions
LC: Data curation, Writing – original draft, Writing – review & editing. FS: Investigation, Writing – original draft. QR: Formal analysis, Investigation, Writing – review & editing. ZJ: Formal analysis, Writing – review & editing. JC: Conceptualization, Methodology, Writing – review & editing. YL: Conceptualization, Methodology, Writing – review & editing. LW: Funding acquisition, Supervision, Writing – review & editing.
Funding
The author(s) declare financial support was received for the research, authorship, and/or publication of this article. This research was funded by the Shandong Province Agricultural Innovation Team (SDAIT-21).
Acknowledgments
The authors thank the Shandong Province Agricultural Innovation Team (SDAIT-21) for supporting this study.
Conflict of interest
The authors declare that the research was conducted in the absence of any commercial or financial relationships that could be construed as a potential conflict of interest.
Publisher’s note
All claims expressed in this article are solely those of the authors and do not necessarily represent those of their affiliated organizations, or those of the publisher, the editors and the reviewers. Any product that may be evaluated in this article, or claim that may be made by its manufacturer, is not guaranteed or endorsed by the publisher.
References
1. Hänninen, S, Mononen, J, Harjunpää, S, Pyykönen, T, Sepponen, J, and Ahola, L. Effects of family housing on some behavioural and physiological parameters of juvenile farmed mink (Mustela vison). Appl Anim Behav Sci. (2008) 109:384–95. doi: 10.1016/j.applanim.2007.03.002
2. Birch, JM, Ullman, K, Struve, T, Agger, JF, Hammer, AS, Leijon, M, et al. Investigation of the viral and bacterial microbiota in intestinal samples from mink (Neovison vison) with pre-weaning diarrhea syndrome using next generation sequencing. PLoS One. (2018) 13:e0205890. doi: 10.1371/journal.pone.0205890
3. Bahl, MI, Honoré, AL, Skønager, ST, Honoré, OL, Clausen, T, Andresen, L, et al. The microbiota of farmed mink (Neovison vison) follows a successional development and is affected by early life antibiotic exposure. Sci Rep. (2020) 10:20434. doi: 10.1038/s41598-020-77417-z
4. Qiao, M, Ying, G-G, Singer, AC, and Zhu, Y-G. Review of antibiotic resistance in China and its environment. Environ Int. (2018) 110:160–72. doi: 10.1016/j.envint.2017.10.016
5. Buonavoglia, A, Leone, P, Solimando, AG, Fasano, R, Malerba, E, Prete, M, et al. Antibiotics or no antibiotics, that is the question: An update on efficient and effective use of antibiotics in dental practice. Antibiotics. (2021) 10:550. doi: 10.3390/ANTIBIOTICS10050550
6. Zl, L, Yj, C, Ql, M, Zhang, X, and Xl, W. Progress in the application of Enterococcus faecium in animal husbandry. Front Cell Infect Microbiol. (2023) 13:1168189. doi: 10.3389/FCIMB.2023.1168189
7. Biricik, H, Brav, FC, Çetin, E, Aydin, L, Fantinati, P, and Cappellozza, BI. Effects of supplementing a direct-fed microbial containing Enterococcus faecium 669 on performance, health, and metabolic responses of pre-weaning Holstein dairy calves. J Dairy Sci. (2023) 106:8684–93. doi: 10.3168/JDS.2023-23581
8. Lan, RX, Kim, JK, Liu, YH, Yun, HM, and Kim, IH. Effects of dietary supplementation with a probiotic (Enterococcus faecium) on growth performance, nutrient digestibility, fecal microbiota, and fecal score in weanling pigs. J Anim Sci. (2017) 95:80. doi: 10.2527/asasmw.2017.12.169
9. Khalifa, A, and Ibrahim, HIM. Enterococcus faecium from chicken feces improves chicken immune response and alleviates Salmonella infections: a pilot study. J Anim Sci. (2023) 101:skad016. doi: 10.1093/JAS/SKAD016
10. Olsen, MSR, Thøfner, I, Sandvang, D, and Poulsen, LL. Research note: the effect of a probiotic E. faecium 669 mitigating Salmonella Enteritidis colonization of broiler chickens by improved gut integrity. Poultry Sci. (2022) 101:102029. doi: 10.1016/J.PSJ.2022.102029
11. Moon, BY, Ali, MS, Choi, JH, Heo, YE, Lee, YH, Kang, HS, et al. Antimicrobial resistance profiles of Enterococcus faecium and Enterococcus faecalis isolated from healthy dogs and cats in South Korea. Microorganisms. (2023) 11:2991. doi: 10.3390/MICROORGANISMS11122991
12. Zelaya, C, Arriagada, G, Galarce, N, Sanchez, F, Escobar, B, Miranda, M, et al. A preliminary report on critical antimicrobial resistance in Escherichia coli, enterococcus faecalis, and Enterococcus faecium strains isolated from healthy dogs in Chile during 2021-2022. Prev Vet Med. (2024) 224:106139. doi: 10.1016/J.PREVETMED.2024.106139
13. Derunets, AS, Selimzyanova, AI, Rykov, SV, Kuznetsov, AE, and Berezina, OV. Strategies to enhance stress tolerance in lactic acid bacteria across diverse stress conditions. World J Microb Biot. (2024) 40:126. doi: 10.1007/S11274-024-03905-3
14. Salminen, S, Collado, MC, Endo, A, Hill, C, Lebeer, S, Quigley, EMM, et al. The international scientific Association of Probiotics and Prebiotics (ISAPP) consensus statement on the definition and scope of postbiotics. Nat Rev Gastro Hepat. (2021) 18:649–67. doi: 10.1038/S41575-021-00440-6
15. Aguilar-Toalá, JE, Garcia-Varela, R, Garcia, HS, Mata-Haro, V, González-Córdova, AF, Vallejo-Cordoba, B, et al. Postbiotics: An evolving term within the functional foods field. Trends Food Sci Tech. (2018) 75:105–14. doi: 10.1016/j.tifs.2018.03.009
16. Gao, J, Li, Y, Wan, Y, Hu, T, Liu, L, Yang, S, et al. A novel Postbiotic from Lactobacillus rhamnosus GG with a beneficial effect on intestinal barrier function. Front Microbiol. (2019) 10:477. doi: 10.3389/fmicb.2019.00477
17. Żółkiewicz, J, Marzec, A, Ruszczyński, M, and Feleszko, W. Postbiotics—a step beyond pre-and probiotics. Nutrients. (2020) 12:2189. doi: 10.3390/nu12082189
18. Heniedy, AM, Mahdy, DM, Elenien, WIA, Mourad, S, and Kadi, RAE. Postbiotics as a health-promoting technique: a review article on scientific and commercial interest. Process Biochem. (2024) 144:6–19. doi: 10.1016/J.PROCBIO.2024.05.010
19. Shin, S, Yasuhiro, I, Kaoruko, Y, Shengbin, R, Kentaro, O, Fumihiro, A, et al. Effects of oral administration of heat-killed Enterococcus faecium strain NHRD IHARA in post-weaning piglets. Anim Sci J. (2014) 85:454–60. doi: 10.1111/asj.12163
20. Hu, C, Xing, W, Liu, X, Zhang, X, Li, K, Liu, J, et al. Effects of dietary supplementation of probiotic Enterococcus faecium on growth performance and gut microbiota in weaned piglets. AMB Express. (2019) 9:33–12. doi: 10.1186/s13568-019-0755-z
21. Castañeda, CD, Dittoe, DK, Wamsley, KK, McDaniel, CD, Blanch, A, Sandvang, D, et al. Discovering the optimal concentration of an Enterococcus faecium–based product to enhance broiler hatchability, live performance, and intestinal morphology. Poultry Sci. (2020) 99:6163–72. doi: 10.1016/j.psj.2020.08.002
22. Li, Y, Zhen, S, Cao, L, Sun, F, and Wang, L. Effects of Lactobacillus plantarum Postbiotics on growth performance, immune status, and intestinal microflora of growing minks. Animals. (2023) 13:2958. doi: 10.3390/ANI13182958
23. Shuai, C, Chen, D, Yu, B, Luo, Y, Zheng, P, Huang, Z, et al. Effect of fermented rapeseed meal on growth performance, nutrient digestibility, and intestinal health in growing pigs. Anim Nutr. (2023) 15:420–9. doi: 10.1016/J.ANINU.2023.06.011
24. Xie, YH, Zhang, CY, Wang, LX, Shang, QH, Zhang, GG, and Yang, WR. Effects of dietary supplementation of Enterococcus faecium on growth performance, intestinal morphology, and selected microbial populations of piglets. Livest Sci. (2018) 210:111–7. doi: 10.1016/j.livsci.2018.02.010
25. Nan, W, Si, H, Yang, Q, Shi, H, Zhang, T, Shi, Q, et al. Effect of vitamin a supplementation on growth performance, serum biochemical parameters, intestinal immunity response and gut microbiota in American mink (Neovison vison). Animals. (2021) 11:1577. doi: 10.3390/ani11061577
26. Xu, Y, Yu, Y, Shen, Y, Li, Q, Lan, J, Wu, Y, et al. Effects of Bacillus subtilis and Bacillus licheniformis on growth performance, immunity, short chain fatty acid production, antioxidant capacity, and cecal microflora in broilers. Poultry Sci. (2021) 100:101358. doi: 10.1016/J.PSJ.2021.101358
27. Giang, HH, Viet, TQ, Ogle, B, and Lindberg, JE. Effects of different probiotic complexes of lactic acid bacteria on growth performance and gut environment of weaned piglets. Livest Sci. (2010) 133:182–4. doi: 10.1016/j.livsci.2010.06.059
28. Suvorov, A, Zhao, S, Leontieva, G, Alekhina, G, Yang, J, Tsapieva, A, et al. Evaluation of the efficacy of Enterococcus faecium L3 as a feed probiotic additive in chicken. Probiotics Antimicro. (2022) 15:1169–79. doi: 10.1007/S12602-022-09970-0
29. Izuddin, WI, Humam, AM, Loh, TC, Foo, HL, and Samsudin, AA. Dietary Postbiotic Lactobacillus plantarum improves serum and ruminal antioxidant activity and upregulates hepatic antioxidant enzymes and ruminal barrier function in post-weaning lambs. Antioxidants. (2020) 9:250. doi: 10.3390/antiox9030250
30. Pimentel, TC, Cruz, AG, Pereira, E, Costa, WKA, Rocha, RS, Pedrosa, GTS, et al. Postbiotics: An overview of concepts, inactivation technologies, health effects, and driver trends. Trends Food Sci Tech. (2023) 138:199–214. doi: 10.1016/J.TIFS.2023.06.009
31. Teame, T, Wang, A, Xie, M, Zhang, Z, Yang, Y, Ding, Q, et al. Paraprobiotics and Postbiotics of probiotic lactobacilli, their positive effects on the host and action mechanisms: a review. Front Nutr. (2020) 7:570344. doi: 10.3389/fnut.2020.570344
32. Zhang, Y, Wu, T, Chen, Z, Meng, Y, Zhu, Z, Wang, Q, et al. Dietary supplementation with Enterococcus faecium R1 attenuates intestinal and liver injury in piglets challenged by lipopolysaccharide. Animals. (2021) 11:1424. doi: 10.3390/ANI11051424
33. Liu, J, Cao, SC, Liu, J, Xie, YN, and Zhang, HF. Effect of probiotics and xylo-oligosaccharide supplementation on nutrient digestibility, intestinal health and noxious gas emission in weanling pigs. Asian Austral J Anim. (2018) 31:1660–9. doi: 10.5713/ajas.17.0908
34. Zhang, ZF, Lee, JM, and Kim, IH. Effects of Enterococcus faecium DSM 7134 on weanling pigs were influenced by dietary energy and crude protein density. Livest Sci. (2014) 169:106–11. doi: 10.1016/j.livsci.2014.09.022
35. Xin, JL, Sang, IL, Kwang, YL, Dinh, HN, and In, HK. Effects of a blend of organic acids and medium-chain fatty acids with and without Enterococcus faecium on growth performance, nutrient digestibility, blood parameters, and meat quality in finishing pigs. Can J Anim Sci. (2018) 98:852–9. doi: 10.1139/cjas-2017-0126
36. Chen, YJ, Min, BJ, Cho, JH, Kwon, OS, Son, KS, Kim, IH, et al. Effects of dietary Enterococcus faecium SF68 on growth performance, nutrient digestibility, blood characteristics and Faecal noxious gas content in finishing pigs. Asian Austral J Anim. (2006) 19:406–11. doi: 10.5713/ajas.2006.406
37. Omar, AE, Al-Khalaifah, HS, Ismail, TA, Abd El-Aziz, RM, El-Mandrawy, SAM, Shalaby, SI, et al. Performance, serum biochemical and immunological parameters, and digestive enzyme and intestinal barrier-related gene expression of broiler chickens fed fermented fava bean by-products as a substitute for conventional feed. Front Vet Sci. (2021) 8:696841. doi: 10.3389/fvets.2021.696841
38. Liu, X, Ju, Y, Huang, L, Liu, M, Bo, J, Zhou, T, et al. Effects of a new fermented soya bean meal on growth performance, serum biochemistry profile, intestinal immune status and digestive enzyme activities in piglets. J Anim Physiol An N. (2021) 106:1046–59. doi: 10.1111/JPN.13649
39. Kozan, DW, Derrick, JT, Ludington, WB, and Farber, SA. From worms to humans: understanding intestinal lipid metabolism via model organisms. BBA-Mol Cell Biol L. (2023) 1868:159290. doi: 10.1016/J.BBALIP.2023.159290
40. Ehrmann, M, and Clausen, T. PROTEOLYSIS AS A REGULATORY MECHANISM. Annu Rev Genet. (2004) 38:709–24. doi: 10.1146/annurev.genet.38.072902.093416
41. Jensen, K, Simpson, SJ, Nielsen, VH, Hunt, J, Raubenheimer, D, and Mayntz, D. Nutrient-specific compensatory feeding in a mammalian carnivore, the mink, Neovison vison. Br J Nutr. (2014) 112:1226–33. doi: 10.1017/S0007114514001664
42. Jiang, Q, Li, G, Zhang, T, Zhang, H, Gao, X, Xing, X, et al. Effects of dietary protein level on nutrients digestibility and reproductive performance of female mink (Neovison vison) during gestation. Anim Nutr. (2015) 1:65–9. doi: 10.1016/j.aninu.2015.05.002
43. Sun, YZ, Yang, HL, Ma, RL, Song, K, and Li, JS. Effect of Lactococcus lactis and Enterococcus faecium on growth performance, digestive enzymes and immune response of grouper Epinephelus coioides. Aquac Nutr. (2012) 18:281–9. doi: 10.1111/j.1365-2095.2011.00894.x
44. Gong, L, Wang, B, Mei, X, Xu, H, Qin, Y, Li, W, et al. Effects of three probiotic Bacillus on growth performance, digestive enzyme activities, antioxidative capacity, serum immunity, and biochemical parameters in broilers. Anim Sci J. (2018) 89:1561–71. doi: 10.1111/asj.13089
45. Sun, Y, Zhang, Y, Liu, M, Li, J, Lai, W, Geng, S, et al. Effects of dietary Bacillus amyloliquefaciens CECT 5940 supplementation on growth performance, antioxidant status, immunity, and digestive enzyme activity of broilers fed corn-wheat-soybean meal diets. Poultry Sci. (2021) 101:101585. doi: 10.1016/J.PSJ.2021.101585
46. Blaak, EE, Canfora, EE, Theis, S, Frost, G, Groen, AK, Mithieux, G, et al. Short chain fatty acids in human gut and metabolic health. Benef Microbes. (2020) 11:411–55. doi: 10.3920/bm2020.0057
47. Samli, HE, Senkoylu, N, Koc, F, Kanter, M, and Agma, A. Effects of Enterococcus faecium and dried whey on broiler performance, gut histomorphology and intestinal microbiota. Arch Anim Nutr. (2007) 61:42–9. doi: 10.1080/17450390601106655
48. Cao, GT, Zeng, XF, Chen, AG, Zhou, L, Zhang, L, Xiao, YP, et al. Effects of a probiotic, Enterococcus faecium, on growth performance, intestinal morphology, immune response, and cecal microflora in broiler chickens challenged with Escherichia coli K88. Poultry Sci. (2013) 92:2949–55. doi: 10.3382/ps.2013-03366
49. Wang, JX, and Peng, KM. Developmental morphology of the small intestine of African ostrich chicks. Poultry Sci. (2008) 87:2629–35. doi: 10.3382/ps.2008-00163
50. Sansonetti, PJ. War and peace at mucosal surfaces. Nat Rev Immunol. (2004) 4:953–64. doi: 10.1038/nri1499
51. Wang, J, Liu, S, Ma, J, Dong, X, Long, S, and Piao, X. Growth performance, serum parameters, inflammatory responses, intestinal morphology and microbiota of weaned piglets fed 18% crude protein diets with different ratios of standardized ileal digestible isoleucine to lysine. Anim Nutr. (2024) 16:313–25. doi: 10.1016/J.ANINU.2023.11.008
52. Shi, N, Li, N, Duan, X, and Niu, H. Interaction between the gut microbiome and mucosal immune system. Military Med Res. (2017) 4:14. doi: 10.1186/s40779-017-0122-9
53. Kaetzel, CS. Cooperativity among secretory IgA, the polymeric immunoglobulin receptor, and the gut microbiota promotes host–microbial mutualism. Immunol Lett. (2014) 162:10–21. doi: 10.1016/j.imlet.2014.05.008
54. Lin, Z, Yang, G, Zhang, M, Yang, R, Wang, Y, Guo, P, et al. Dietary supplementation of mixed organic acids improves growth performance, immunity, and antioxidant capacity and maintains the intestinal barrier of Ira rabbits. Animals. (2023) 13:3140. doi: 10.3390/ANI13193140
55. Corthésy, B. Multi-faceted functions of secretory IgA at mucosal surfaces. Front Immunol. (2013) 4:185. doi: 10.3389/fimmu.2013.00185
56. Hezaveh, K, Shinde, RS, Klötgen, A, Halaby, MJ, Lamorte, S, Ciudad, MT, et al. Tryptophan-derived microbial metabolites activate the aryl hydrocarbon receptor in tumor-associated macrophages to suppress anti-tumor immunity. Immunity. (2022) 55:324–340.e8. doi: 10.1016/J.IMMUNI.2022.01.006
57. Wu, QJ, Zhu, DD, Wang, DD, Zhang, BB, Ren, A, and Zhang, ZB. Effects of dietary supplementation with glutamine on the lymphocyte proliferation and intestinal immune gene expression in broiler chickens infected with Salmonella Enteritidis. Res Vet Sci. (2021) 139:18–24. doi: 10.1016/J.RVSC.2021.06.018
58. Chen, P, Lv, H, Liu, W, Wang, Y, Zhang, K, Che, C, et al. Effects of Lactobacillus plantarum HW1 on growth performance, intestinal immune response, barrier function, and Cecal microflora of broilers with necrotic enteritis. Animals. (2023) 13:3810. doi: 10.3390/ANI13243810
59. Cui, B, Wang, L, Guo, Q, Huang, Y, and Hu, S. Effects of Enterococcus faecium on expression of intestinal tight junction proteins, cytokine responses and toll-like receptors genes in piglets. Chin. J. Animal Nutr. (2022) 34:7616–27. (in chinese). doi: 10.3969/j.issn.1006-267x.2022.12.015
60. Ren, Y, Guo, Q, Li, Y, Xiang, M, and Huang, Y. Effect of live Enterococcus faecium and heat-inactivated bacteria on TNF-c/IL-10 balance and mitogen-activated protein kinase signaling in RAW264.7 cells. Chin. J. Animal Nutr. (2020) 32:4345–57. doi: 10.3969/j.issn.1006-267x.2020.09.045
61. Ghazisaeedi, F, Meens, J, Hansche, B, Maurischat, S, Schwerk, P, Goethe, R, et al. A virulence factor as a therapeutic: the probiotic Enterococcus faecium SF68 arginine deiminase inhibits innate immune signaling pathways. Gut Microbes. (2022) 14:2106105. doi: 10.1080/19490976.2022.2106105
62. Gratiela, GP, Janie, L, Ozan, G, Nicolae, C, Iuliana, I, Luciana, O, et al. Effects of the lipid profile, type 2 diabetes and medication on the metabolic syndrome—associated gut microbiome. Int J Mol Sci. (2022) 23:7509. doi: 10.3390/IJMS23147509
63. Sommer, F, and Bäckhed, F. The gut microbiota--masters of host development and physiology. Nat Rev Microbiol. (2013) 11:227–38. doi: 10.1038/nrmicro2974
64. Li, Q, Chen, S, Zhu, K, Huang, X, Huang, Y, Shen, Z, et al. Collateral sensitivity to pleuromutilins in vancomycin-resistant Enterococcus faecium. Nat Commun. (2022) 13:1888. doi: 10.1038/S41467-022-29493-0
65. Bahl, MI, Hammer, AS, Clausen, T, Jakobsen, A, Skov, S, and Andresen, L. The gastrointestinal tract of farmed mink (Neovison vison) maintains a diverse mucosa-associated microbiota following a 3-day fasting period. Microbiology Open. (2017) 6:e00434. doi: 10.1002/mbo3.434
66. Compo, NR, Gomez, DE, Tapscott, B, Weese, SJ, and Turner, PV. Fecal bacterial microbiota of Canadian commercial mink (Neovison vison): yearly, life stage, and seasonal comparisons. PLoS One. (2018) 13:e0207111. doi: 10.1371/journal.pone.0207111
67. An, C, Okamoto, Y, Xu, S, Eo, KY, Kimura, J, and Yamamoto, N. Comparison of fecal microbiota of three captive carnivore species inhabiting Korea. J Vet Med Sci. (2017) 79:542–6. doi: 10.1292/jvms.16-0472
68. Ludueña, LM, Anzuay, MS, Angelini, JG, McIntosh, M, Becker, A, Rupp, O, et al. Strain Serratia sp. S119: a potential biofertilizer for peanut and maize and a model bacterium to study phosphate solubilization mechanisms. Appl Soil Ecol. (2018) 126:107–12. doi: 10.1016/j.apsoil.2017.12.024
69. Deorukhkar, AA, Chander, R, Ghosh, SB, and Sainis, KB. Identification of a red-pigmented bacterium producing a potent anti-tumor N-alkylated prodigiosin as Serratia marcescens. Res Microbiol. (2007) 158:399–404. doi: 10.1016/j.resmic.2007.02.010
70. Lee, SJ, Baek, YJ, Kim, JN, Lee, KH, Lee, EH, Yeom, JS, et al. Increasing Fusobacterium infections with Fusobacterium varium, an emerging pathogen. PLoS One. (2022) 17:e0266610. doi: 10.1371/JOURNAL.PONE.0266610
71. Stokowa-Sołtys, K, Wojtkowiak, K, and Jagiełło, K. Fusobacterium nucleatum – friend or foe? J Inorg Biochem. (2021) 224:111586. doi: 10.1016/J.JINORGBIO.2021.111586
72. Hirano, T, and Nakase, H. The multifaceted effects of gut microbiota on the immune system of the intestinal mucosa. Immuno. (2021) 1:583–94. doi: 10.3390/IMMUNO1040041
73. Xu, X, Duarte, ME, and Kim, SW. Postbiotics effects of Lactobacillus fermentate on intestinal health, mucosa-associated microbiota, and growth efficiency of nursery pigs challenged with F18 + Escherichia coli. J Anim Sci. (2022) 100:skac210. doi: 10.1093/JAS/SKAC210
74. Dehner, C, Fine, R, and Kriegel, MA. The microbiome in systemic autoimmune disease: mechanistic insights from recent studies. Curr Opin Rheumatol. (2019) 31:201–7. doi: 10.1097/BOR.0000000000000574
Keywords: mink, postbiotics, Enterococcus faecium, nutrients digestibility, intestinal morphology, immune status, intestinal microbiota
Citation: Cao L, Sun F, Ren Q, Jiang Z, Chen J, Li Y and Wang L (2024) Effects of dietary supplementation of Enterococcus faecium postbiotics on growth performance and intestinal health of growing male mink. Front. Vet. Sci. 11:1409127. doi: 10.3389/fvets.2024.1409127
Edited by:
Izhar Hyder Qazi, Shaheed Benazir Bhutto University of Veterinary and Animal Sciences, PakistanReviewed by:
Yanzhu Zhu, Jilin Agricultural Science and Technology College, ChinaChao Xu, Jilin Agricultural University, China
Zheng Ruan, Nanchang University, China
Copyright © 2024 Cao, Sun, Ren, Jiang, Chen, Li and Wang. This is an open-access article distributed under the terms of the Creative Commons Attribution License (CC BY). The use, distribution or reproduction in other forums is permitted, provided the original author(s) and the copyright owner(s) are credited and that the original publication in this journal is cited, in accordance with accepted academic practice. No use, distribution or reproduction is permitted which does not comply with these terms.
*Correspondence: Lihua Wang, bGh3YW5nQHFhdS5lZHUuY24=