- 1Department of Veterinary Internal Medicine and Surgery, School of Agricultural and Veterinarian Sciences, São Paulo State University (Unesp), Jaboticabal, SP, Brazil
- 2Faculdade Ciências Médicas de Minas Gerais, Belo Horizonte, MG, Brazil
Objective: To compare the efficacy of intranasal (IN) and intramuscular (IM) administrations of azaperone (3 mg kg−1), midazolam (0. 3 mg kg−1), and ketamine (7 mg kg−1) combination (AMK) in pigs. Study design: Randomized clinical trial. Animals: sixteen adult male pigs, immunocastrated, of mixed lineage.
Methods: In phase I, these animals were randomly assigned to intranasal (GIN, n = 8) and intramuscular (GIM, n = 8) groups for arterial blood sample collection at 10, 20, 30, 45, 60, and 90 min after AMK administrations for gas and electrolyte analysis. In phase II, performed 1 week after phase I, the 16 pigs were allocated to both groups (GIM, n = 16/GIN, n = 16) and submitted to the same chemical restraint (CR) protocol, with a 96-h interval between administrations. Behavioral parameters (degree of CR, muscle relaxation, loss of postural reflex, and sound stimulus response) and vital parameters (pulse rate, respiratory rate, oxygen saturation, and rectal temperature) were evaluated after recumbency (Trec) and at 5, 15, 30, 45, 60, and 90 min after administrations. In addition, the latency period and duration of CR were determined.
Results: Latency to recumbency and duration of CR in GIN were shorter. CR scores did not vary between groups. Muscle relaxation was more intense in GIN at Trec. An initial tachycardia was observed, followed by a reduction in heart rate from T5 to T90 in both treatments (p < 0.05). The respiratory rate was higher at T45, T60, and T90 in GIN compared to baseline. Rectal temperature reduced in GIM from T45 onwards. elevated at T90 in the GIM (p < 0.05) and there was an incidence of mild hypoxemia in 47% of the animals in the GIM.
Conclusions and clinical relevance: IN administration was as effective as IM administration in promoting safe chemical restraint, with minimal changes in homeostasis, with a shorter duration and latency period.
1 Introduction
The advancement of therapeutic medicine has been greatly facilitated by the utilization of animal models to investigate the etiopathogenesis and pathophysiological mechanisms underlying various diseases. Due to their abundant anatomical, physiological, and genomic similarities with humans, pigs have become a preferred model for investigating cardiovascular (1–3), skeletal (4, 5), urinary (6) systems, as well as metabolic, neurodegenerative, and genetic diseases (7). In comparison to primates, pigs exhibit short generation intervals, rapid growth rates, prolific litters, and standardized breeding techniques (8).
Ensuring the wellbeing of animals involved in biomedical studies is the responsibility of the researcher and is essential for obtaining reliable results (9). Pigs are poorly tolerant of physical restraint, which can induce metabolic changes such as hyperthermia and respiratory distress (10). In this context, chemical restraint, while reducing stress, can be associated with local anesthesia for surgical purposes and offers certain advantages over general anesthesia, including easier administration, lower cost, reduced equipment requirements, shorter recovery time, and lower risk of complications (11).
The combination of azaperone, midazolam, and ketamine was chosen for this study due to their complementary effects and suitability for use in swine. Azaperone provides reliable tranquilization and muscle relaxation, making it effective for procedures requiring immobilization (12–14). Midazolam enhances sedation, promotes muscle relaxation, reduces the requirement for other anesthetics, and mitigates the unwanted effects of dissociative agents (15, 16). Ketamine is considered a safe anesthetic agent due to its stimulating effects on the cardiovascular system (17, 18) and minimal respiratory depression (19). Additionally, ketamine offers dissociative anesthesia with a rapid onset of action and a short duration, which is beneficial for quickly achieving and maintaining the desired anesthetic state (16). These properties contribute to its broad dosage range. In swine, the recommended doses for these three drugs 1–4 mg/kg of azaperone (20, 21), 0.2–0.4 mg/kg of midazolam (22), and 1–20 mg/kg of ketamine (21, 23).
The intramuscular (IM) route has been widely used in swine for the administration of sedatives and tranquilizers. However, there is the potential risk of muscle damage and extended recovery time due to drug deposition in muscle tissue (10, 20, 24). On the other hand, intravenous (IV) administration is challenging in conscious animals, as venous access requires forceful physical restraint (11).
Intranasal (IN) administration is a painless and less invasive method (25), with drug absorption facilitated by the olfactory and trigeminal neural pathways, bypassing the blood-brain barrier (BBB) (26). The use of this route for administering sedatives, tranquilizers and analgesics has been described in several species, including humans and pigs (10, 13, 27–29).
Therefore, this study aimed to propose a less invasive alternative for chemical restraint (CR) in pigs, with a focus on maintaining homeostasis by minimizing the stress associated with physical restraint.
2 Materials and methods
2.1 Animals and housing
The trial was carried out at the Swine Studies Laboratory (LabSui) of the Animal Science Department at São Paulo State University (Unesp), School of Agricultural and Veterinarian Sciences (FCAV), Jaboticabal-SP, Brazil. This study was approved by the Ethics Committee on the Use of Animals (CEUA) of FCAV/Unesp/Jaboticabal (protocol 2296/2021). Sample size determination followed the formula proposed by the “Guidelines for the care and use of mammals in neuroscience and behavioral research” (30), with sixteen adult pigs utilized (assuming two groups, α = 0.05, and 1 – β = 0.8). The subjects were immunocastrated males, of mixed lineage (Landrace x Large White), with an average weight of 102 ± 12 kg.
The pigs were housed in individual pens (2.7 m2) with a fully slatted plastic floor and controlled room temperature (21°C−27°C). They had access to water through nipple-type drinkers and were provided with feed (semi-automatic trough feeder) ad libitum. The diet consisted primarily of corn and soy, with nutritional content comprising 10.96% crude protein, 0.66% digestible lysine, 0.500% calcium, 0.230% phosphorus, and 2,525 Kcal Kg−1 net energy. The health of the animals was confirmed through both clinical and laboratory examinations. Prior to the CR protocol, the pigs underwent a solid food fast (6–8 h) while maintaining free access to water. Following the completion of the longest washout period (equivalent to 10 half-lives of ketamine), the animals were sent to slaughter.
2.2 Experimental design and group formation
The experiment was carried out in two stages. In Phase I, conducted over 5 days (D1 to D5), the 16 pigs were allocated into 2 groups (n = 8) and received a combination of 3 drugs (AMK): 3 mg kg−1 of azaperone (Destress® Desvet, SP, Brazil), 0.3 mg kg−1 of midazolam (Dormire® Cristália, SP, Brazil), and 7 mg kg−1 of ketamine (Ketalex® Dechra, PR, Brazil). One group received the AMK combination intranasally (GIN, n = 8) and the other intramuscularly (GIM, n = 8). In this phase, arterial blood samples were collected for the analysis of gases and electrolytes. The distribution of animals into groups was done by ordering them by increasing weight and pairing them two by two, followed by eight draws until the groups were filled. This method was employed to reduce weight discrepancies between the GIN and GIM. In Phase II, conducted between D7 and D13, animals from both groups (GIN and GIM, n = 16) underwent the same CR protocol as described in Phase I, with a 96-h interval between administrations. This phase aimed to assess clinical and behavioral parameters. The two-stage assay was necessary to avoid additional stress from manipulation during Phase I, which could affect the behavioral parameters evaluated in Phase II. A 96-h interval was established, approximately twice the “washout” period of the longest-acting drugs (ketamine and midazolam), to ensure complete elimination of these substances before the next administration. Administrations were performed between 8:00 and 12:30 h to avoid the hottest periods of the day. In Phase II, two animals were treated simultaneously every 1.5 h, totaling 6 animals per day. A consistent time was maintained for administration in each pig, for example, animal 1 received AMK at 8:00 h in GIM and at the same time when integrated into GIN.
2.3 Intranasal and intramuscular administration
In both phases, restraint for the administration of the AMK combination was achieved using a snubbing rope, positioned behind the pig's tusks for a brief period (30–60 seconds). Administration via the intranasal route (GIN) was carried out over 20 seconds, exclusively in the right nostril, using a 20 mL disposable syringe. The total volume of AMK (21 ± 3.5 mL) was divided into two syringes, when necessary, and its administration was guided by a 14 G × 45 mm polytetrafluoroethylene catheter. The pigs' snouts were elevated for 30 seconds. In GIM, the AMK combination was applied intramuscularly to the middle third of the neck. This was achieved using appropriate size syringes and 40 × 1.2 mm disposable needles after skin antisepsis with a surgical pad soaked in 70% alcohol.
2.4 Arterial blood collection (phase I)
The ears were cleansed with 70% alcohol, followed by the application of topical lidocaine 10% (Xylestesin® Cristália, SP, Brazil) for skin desensitization. After administration of the AMK combination, a 22G peripheral catheter (Descarpack® SP, Brazil) was inserted into the auricular artery for sample collection at T10, T20, T30, T45, T60, and T90 (each subscript number corresponds to the moment, in minutes, counted after administration). Syringes containing lytic heparin (A-Line 3 mL SAG; BD™; MG, Brasil) were used and these samples were processed immediately using a blood gas analyzer (cobas b 123 POC system; Roche, SP, Brazil). The measured parameters included pH, , , base excess (BE), anion gap (AG), and concentrations of Na+, K+, Ca2+, Cl−, , glucose, and lactate.
2.5 Physiological and behavioral parameters (phase II)
Assessments were conducted at recumbency (Trec), T5, T10, T15, T30, T45, T60, and T90 following the administration of AMK. Physiological parameters, including pulse rate (PR) and oxyhemoglobin saturation (SpO2), were evaluated using pulse oximetry (CONTEC08C; Contec™, Hebei, China); respiratory rate (fR) was assessed by observing rib cage movements, and rectal temperature (Trectal) was measured using a digital thermometer. Behavioral parameters were evaluated during the same periods, extending until the animal remained recumbent, as detailed in Table 1. In addition, the latency period and duration of CR were determined. The latency period was defined as the time elapsed until decubitus occurred following the administration of AMK. The duration of CR was documented from the onset of recumbency until the animal returned to the quadrupedal position. Due to the small size of the team conducting the trial, a double-blind evaluation was not feasible.
2.6 Statistics
All analyzes were performed using the Graphpad Prism program (version 9.0.0. 121). Normality of variables pertaining to blood gas analysis, vital parameters, latency and duration of CR was confirmed by the Shapiro-Wilk test. For comparison between groups, the T test was applied to gasometric variables, while physiological variables, latency and duration of CR were evaluated using the paired T test. To investigate variations over time, Repeated Measures Analysis of Variance (RM-ANOVA) was used. Additionally, the Dunnett's test was used to identify any differences between the first collection/evaluation moment and subsequent ones. The behavioral variables expressed in scores were subjected to the Friedman test, followed by Dunn, to analyze variations over time, and the Wilcoxon test, aiming to detect differences between groups. Concerning missing data, they were more prevalent in the later time points, particularly in GIN, as animals in this group exhibited quicker recovery, and data were only collected while the animals were in recumbency. Consequently, comparisons between groups were feasible until T90.
3 Results
3.1 Considerations
The trial began with a total of 20 animals. One of them showed signs of prostration on the 2nd day (D2) and, after clinical and laboratory evaluation, was removed from the trial to receive the necessary care and did not receive the AMK combination. During phase II, another animal reacted with head movements during intranasal administration and was removed from the evaluations. In general, all administrations were carried out uneventfully, and all animals were recumbent after administration of AMK.
3.2 Characteristics of the chemical restraint
The latency period was shorter in GIN (GIN: 63 ± 47 seconds; GIM: 113 ± 39 seconds; p = 0.002). GIN also exhibited a shorter duration of CR (119 ± 54 min) compared to GIM (163 ± 47 min; p = 0.0015). CR scores remained consistent between groups and times, with an overall median of three. Intranasal administration induced immediate muscle relaxation (Trec: GIN = 3 vs. GIM = 1; p = 0.008). In GIM, maximum muscle relaxation occurred at T15, T30, and T45, compared to baseline (p = 0.0015). Scores for posture and response to sound did not vary between groups and times.
3.3 Physiological and gasometric parameters
The assessed vital parameters (PR, fR, SpO2, and Trectal) are presented in Table 2 (mean values ± standard deviation) and Table 3 (percentage representation of alterations). A reduction in PR was observed in both groups relative to baseline (p < 0.05). Table 3 indicates that most animals exhibited tachycardia in Trec. GIN displayed a higher percentage of tachycardia at T45, T60, and T90 (Table 3), differing from GIM (T45: p = 0.0288; T60: p = 0.0127; T90: p = 0.0203).
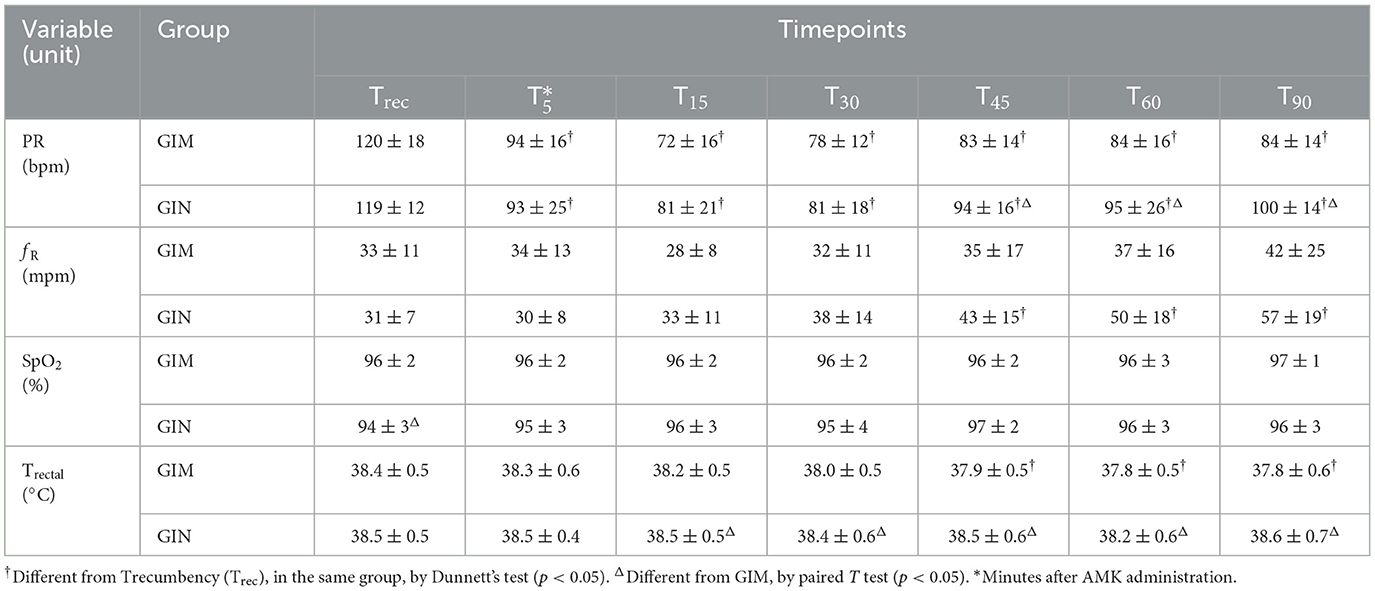
Table 2. Values (mean ± standard deviation) of pulse rate (PR), respiratory rate (fR), oxygen saturation (SpO2), and rectal temperature (Trectal) of adult pigs, which received a combination of azaperone (3 mg kg−1), midazolam (0.3 mg kg−1) and ketamine (7 mg kg−1), via intramuscular (GIM, n = 16) and intranasal (GIN, n = 16) routes.
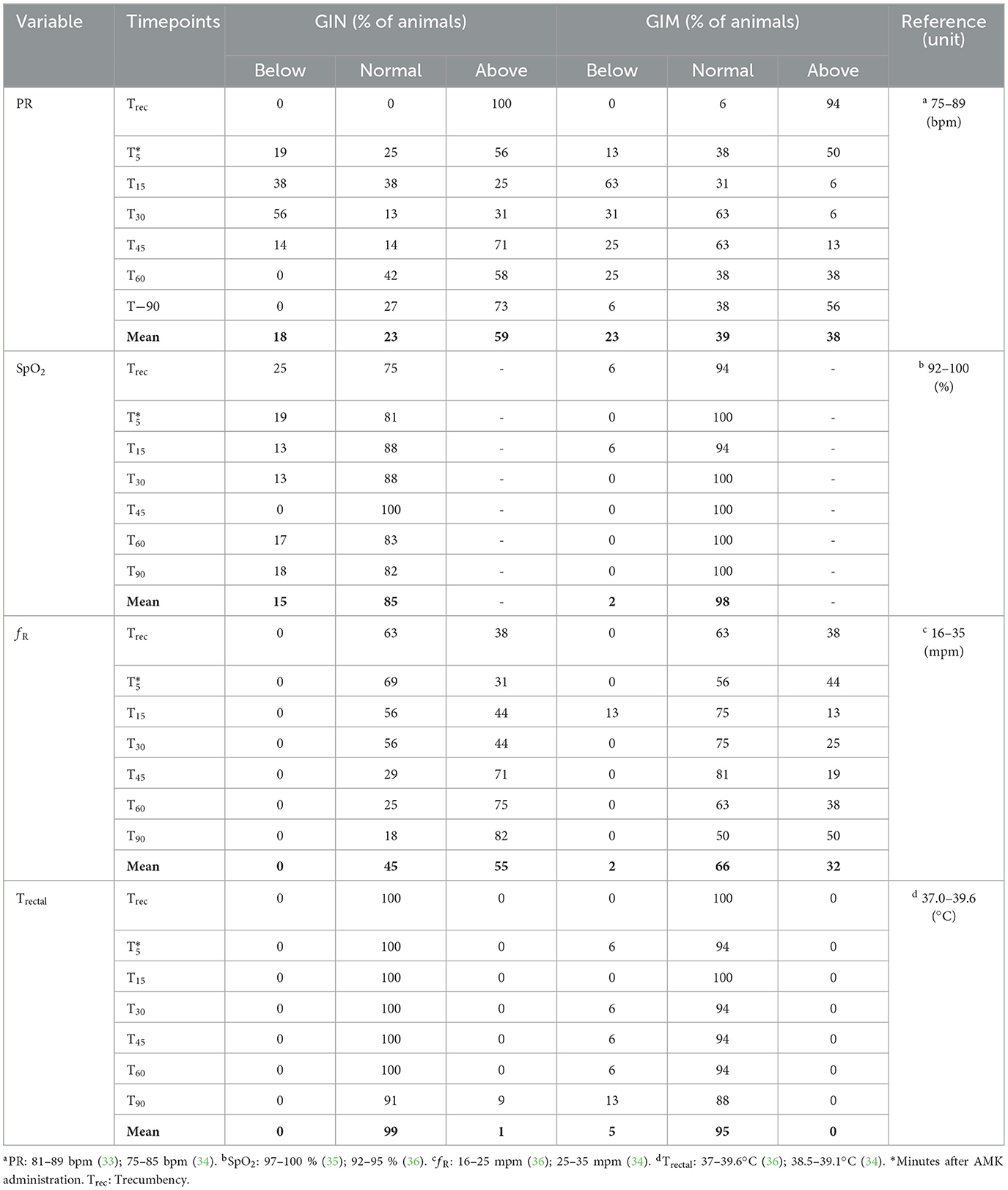
Table 3. Percentage representation of pulse rate (PR), respiratory rate (fR), oxygen saturation (SpO2), and rectal temperature (Trectal) of adult pigs, which received a combination azaperone (3 mg kg−1), midazolam (0.3 mg kg−1), and ketamine (7 mg kg−1), via intramuscular (GIM, n = 16) and intranasal (GIN, n = 16) routes.
In GIN, fR increased at T45 (p = 0.0312 and 71% of tachypnea), T60 (p = 0.0144 and 75% of tachypnea), and T90 (p = 0.0051 and 82% of tachypnea), compared to baseline (Trec). SpO2 remained constant across all times, but 25% of GIN animals exhibited reduced SpO2 values at Trec, differing from GIM (p = 0.0364). In GIM, Trectal reduced relative to baseline at T45, T60, and T90 (p < 0.05) and compared to GIN from T15 onwards. In GIN, 99% of animals remained within the normothermia range (Table 3). On the other hand, 5% of the GIM animals showed a drop in body temperature during the CR period.
Gasometric parameters are presented in Table 4 (average values ± standard deviation) and Table 5 (percentage representation of changes). decreased at T90 in GIM compared to T10 (p = 0.0139) but remained constant across groups, averaging 45 ± 6 mmHg throughout. In GIN, an incidence of hypocapnia ( < 35 mmHg) was identified in 14% and 20% of animals at T60 and T90 (Table 5), respectively. values did not demonstrate significant variations between groups and moments (p > 0.05). However, an average of 11% of the animals in the GIN and 47% of the animals in the GIM had values below 82 mmHg (Table 5).
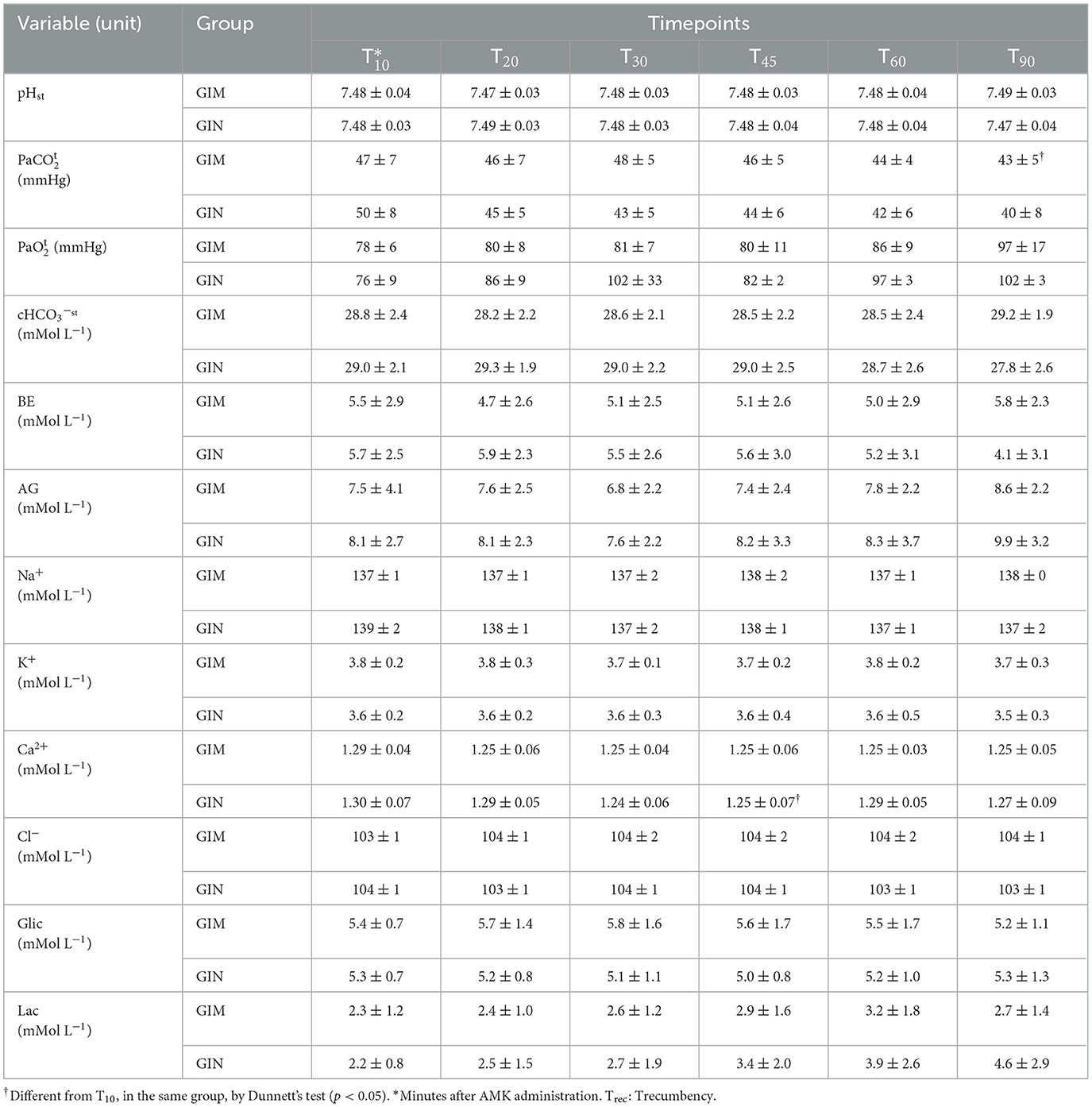
Table 4. Values (mean ± standard deviation) of gasometric parameters of adult pigs, which received a combination of azaperone (3 mg kg−1), midazolam (0.3 mg kg−1), and ketamine (7 mg kg−1), via intramuscular (GIM, n = 8) and intranasal (GIN, n = 8) routes.
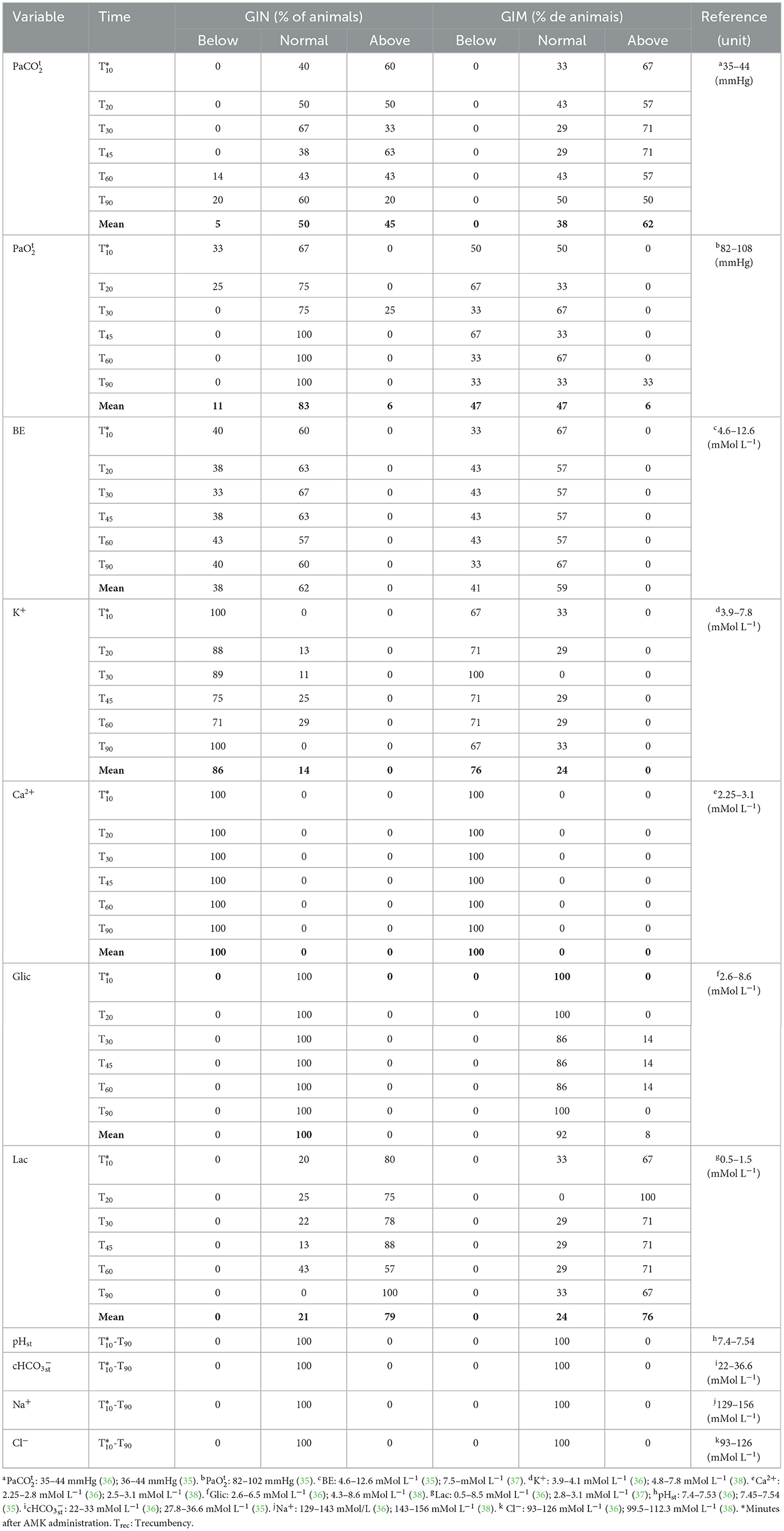
Table 5. Percentage representation of gasometric parameters of adult pigs, which received a combination of azaperone (3 mg kg−1), midazolam (0.3 mg kg−1), and ketamine (7 mg kg−1), via intramuscular (GIM, n = 8) and intranasal (GIN, n = 8) routes.
BE values did not vary between groups or timepoints but remained below physiological limits in 38% and 41% of GIN and GIM animals, respectively. Lactate values remained consistent between groups and times (Table 4) but exceeded physiological limits in both groups, with an average occurrence of hyperlactatemia of 79% in GIN and 76% in GIM.
Ca2+ values did not differ between groups (Table 4) but decreased at T45 in GIN compared to T10 (p = 0.0265). As displayed in Table 5, both groups exhibited a high percentage of hypocalcemia (Ca2+ < 2.25 mMol/L) and hypokalemia (K+ < 3.9 mMol/L).
4 Discussion
4.1 Characteristics of the chemical restraint
Several studies have explored the intranasal administration of azaperone (13, 14, 21, 27), midazolam (22), and ketamine (13, 14, 27) for CR in pigs, either alone or in combination. These studies reveal variations in latency, duration, and restraint characteristics. Discrepancies can be attributed to variables including the combination of medications, doses, the presence of nociceptive stimuli, and the age/weight of the animals.
A study closely resembling AMK was conducted by (13), using ketamine (15 mg kg−1), azaperone (1 mg kg−1), and climazolam (1.5 mg kg−1) to restrain piglets aged 4 to 7 days. Latency periods ranged from 4 to 5 min, longer than those observed in our study. This difference may be attributed to the animals' weight. Considering a weight-based dosing regimen, it's essential to acknowledge that finishing pigs have a higher fat percentage, resulting in a relatively higher dose for a 110 kg animal than a 2.3 kg animal. In both studies, intranasal administration led to shorter CR durations, emphasizing the effectiveness of this route for procedures that require CR of short and medium duration.
Intranasal administration is characterized by the high permeability of the nasal mucosa, proximity to the central nervous system (31, 32), and facilitated drug absorption through trigeminal and olfactory pathways, avoiding the blood-brain barrier (26). This explains the substantially shorter latency period observed in GIN compared to GIM. The rapid onset of relaxation is a notable advantage, especially considering the swine's susceptibility to stress.
The AMK combination offers advantages over other protocols. Azaperone alone, administered intranasally and intramuscularly to piglets, induced decubitus after 30 and 90 min, respectively (21). Alfaxalone, given intranasally, did not achieve sufficient sedation in adult pigs for handling or clinical procedures (10). Another study showed that S (+) ketamine, in combination with azaperone, did not provide better anesthetic quality than racemic ketamine when administered intranasally (27). These findings underscore the synergistic effect of the combination used in our study, ensuring effective CR regardless of the administration route. The immediate muscle relaxation in GIN animals may be linked to the shorter latency period of drug action in this group.
It's crucial to highlight that the AMK combination provided effective CR and muscle relaxation in the absence of nociceptive stimuli. In Axiak et al.'s study, the azaperone, climazolam, and ketamine combination did not eliminate pain scores during piglet castration, particularly when administered intranasally (13). The substantial final solution volume is one of the limitations of the intranasal route, leading to drug swallowing and compromised absorption due to the first-pass effect (13). Our test involved adult pigs, and the AMK solution had considerable final volumes (21 ± 3.5 mL). Although we did not observe large variations in sedation quality among GIN individuals, formulating more concentrated preparations for intranasal administration, coupled with aerosol-generating spray devices, could be highly advantageous.
4.2 Physiological and gasometric parameters
Interpreting physiological variables poses a consistent challenge, particularly given the unique aspects of the swine species. When reviewing literature, considering study-specific factors, including age, genetic lineage, and environmental conditions, is crucial. Caution is warranted in making comparisons and establishing normal or abnormal values. To address this, we adopted a descriptive approach (Tables 3, 5) to complement inferential analyses. Reference values for each parameter were standardized using selected studies, prioritizing similar methodologies (33–38).
The elevated PR at Trec was probably a result of physical restraint. Table 3 reveals a higher incidence of tachycardia than bradycardia in both groups, which could reflect systemic effects induced by the sedative drugs. In individuals with normal serum catecholamine levels, ketamine's sympathomimetic effect increases heart rate, cardiac output, contractile strength, and arterial pressures (18). Additionally, azaperone, through α1-adrenergic blockade, lowers systemic vascular resistance, compensated by an increased heart rate (39).
Only GIN animals exhibited an increased fR. Typically, the drugs used have minimal impact on the respiratory system (19). These effects, if present, tend to be more associated with respiratory depression (39, 40), except for azaperone, which elevated fR in rats, horses, and pigs (41). The intranasal route may account for the observed differences in fR between groups and the decreased SpO2 in GIN at Trec. Slow administration aimed to minimize swallowing and drug entry into the lower respiratory tract, but no method ensured liquid confinement to nasal and tracheal mucosa. Consequently, it is believed that AMK contact with lung epithelium may transiently reduce SpO2, leading to tachypnea in GIN animals at T45, T60, and T90.
It is crucial to emphasize that the shed temperature was regulated, and the sedation durations for the animals were standardized, thereby minimizing the impact of external factors on Trectal. Consequently, we can infer that the thermoregulation system of GIN animals experienced less disruption compared to GIM animals, supporting the findings of Axiak et al. (13).
Regarding acid-base balance, the observed base deficit in both groups may be linked to elevated lactate levels, possibly induced by physical restraint. A study by Hamilton et al. noted lactate levels of 4.9 mMol/L in pigs under light handling and 19.1 mMol/L in those subjected to heavy handling (37). Nevertheless, it is crucial to emphasize that the rise in lactate did not induce alterations in pH and serum bicarbonate levels. This implies that primary buffering systems effectively contributed to maintaining homeostasis in both groups.
The hypocapnia noted in certain GIN animals at T60 and T90 aligns with the concurrent increase in respiratory rate during these intervals in this group. Hyperventilation may perhaps justify the absence of hypoxia in GIN between T45 and T90, unlike GIM, in which 47% and 33% of animals had values lower than 82 mmHg, at T45 and T90, respectively (Table 5).
Depletion of potassium and calcium can be ascribed to fasting. A prior study involving crossbred pigs weighing around 30 kg showed no significant potassium level variations after a period of food deprivation (42). However, this study noted a decrease in calcium levels only after prolonged fasting (51, 95, and 167 h), contrasting with our observations of hypokalemia and hypocalcemia in animals with significantly higher weights subjected to an 8-h fast. Our findings align with Becerril-Herrera et al. (43), who assessed biochemical and gasometric parameters in Pietran, Large White, and Yorkshire pigs designated for slaughter. They observed a reduction in the levels of these two electrolytes after 8 and 16 h of food deprivation (43), suggesting that weight and bloodline may influence the metabolic response during fasting.
5 Conclusions
Intranasal administration of the AMK combination produced immediate chemical restraint, with quality similar to that obtained after intramuscular administration, with minimal clinical changes. The latency and duration of the effect make the intranasal route a suitable option for carrying out usual ambulatorial or surgical procedures, in association with local anesthesia. Furthermore, given the association of many adverse effects of anesthesia with prolonged recovery periods, the use of this route suggests a reduction in the incidence of these effects compared to intramuscular administration.
Data availability statement
The raw data supporting the conclusions of this article will be made available by the authors, without undue reservation.
Ethics statement
The animal study was approved by the Comissão de Ética no Uso de Animais - Jaboticabal - FCAV. The study was conducted in accordance with the local legislation and institutional requirements.
Author contributions
IR: Conceptualization, Data curation, Formal analysis, Investigation, Methodology, Project administration, Validation, Visualization, Writing – original draft, Writing – review & editing. CG: Conceptualization, Data curation, Investigation, Methodology, Project administration, Visualization, Writing – review & editing. IV: Data curation, Methodology, Writing – review & editing. VP: Data curation, Methodology, Writing – review & editing. AR: Data curation, Methodology, Writing – review & editing. SR: Conceptualization, Investigation, Methodology, Visualization, Writing – review & editing. CV: Conceptualization, Data curation, Funding acquisition, Investigation, Methodology, Project administration, Resources, Supervision, Validation, Visualization, Writing – review & editing.
Funding
The author(s) declare financial support was received for the research, authorship, and/or publication of this article. This work was financially supported by the “Fundação de Amparo à Pesquisa do Estado de São Paulo” (FAPESP - Process 2020/09633-0) and scholarship provided by the “Coordenação de Aperfeiçoamento de Pessoal de Nível Superior” (CAPES)–Finance Code 001.
Acknowledgments
We would like to express our gratitude the “Unesp Research Support Laboratory” of “Internal Medicine and Veterinary Surgery Department” and the Swine Studies Laboratory (LabSui) of the Animal Science Department at São Paulo State University (Unesp), School of Agricultural and Veterinarian Sciences (FCAV), Jaboticabal, SP, Brazil. We would also like to thank the company Desvet, for providing Destress R to carry out this experiment. During the preparation of this work the authors used ChatGPT (GPT-4 Open AI) to review the text for possible grammatical, spelling, and semantic errors, that may have occurred when translating into English. After using this tool, the authors reviewed and edited the content as needed and take full responsibility for the content of the publication.
Conflict of interest
The authors declare that the research was conducted in the absence of any commercial or financial relationships that could be construed as a potential conflict of interest.
Publisher's note
All claims expressed in this article are solely those of the authors and do not necessarily represent those of their affiliated organizations, or those of the publisher, the editors and the reviewers. Any product that may be evaluated in this article, or claim that may be made by its manufacturer, is not guaranteed or endorsed by the publisher.
Supplementary material
The Supplementary Material for this article can be found online at: https://www.frontiersin.org/articles/10.3389/fvets.2024.1408103/full#supplementary-material
Supplementary Video 1 | Intranasal administration of azaperone, midazolam, and ketamine (AMK) combination, over 20 seconds, in an adult male pig.
References
1. Gabisonia K, Prosdocimo G, Aquaro GD, Carlucci L, Zentilin L, Secco I, et al. MicroRNA therapy stimulates uncontrolled cardiac repair after myocardial infarction in pigs. Nature. (2019) 569:418–22. doi: 10.1038/s41586-019-1191-6
2. Hobby ARH, Sharp TE, Berretta RM, Borghetti G, Feldsott E, Mohsin S, et al. Cortical bone-derived stem cell therapy reduces apoptosis after myocardial infarction. Am J Physiol Heart Circ Physiol. (2019) 317:H820–H829. doi: 10.1152/ajpheart.00144.2019
3. Yuan X, Pan J, Wen L, Gong B, Li J, Gao H, et al. MiR-590-3p regulates proliferation, migration and collagen synthesis of cardiac fibroblast by targeting ZEB1. J Cell Mol Med. (2020) 24:227. doi: 10.1111/jcmm.14704
4. Peck Y, He P, Chilla GSVN, Poh CL, Wang DA. A preclinical evaluation of an autologous living hyaline-like cartilaginous graft for articular cartilage repair: a pilot study. Sci Rep. (2015) 5:16225. doi: 10.1038/srep1622510.1038/srep16225
5. Hai T, Guo W, Yao J, Cao C, Luo A, Qi M, et al. Creation of miniature pig model of human Waardenburg syndrome type 2A by ENU mutagenesis. Hum Genet. (2017) 136:1463–75. doi: 10.1007/s00439-017-1851-2
6. Rodríguez RR, González-Bulnes A, Garcia-Contreras C, Elena Rodriguez-Rodriguez A, Astiz S, Vazquez-Gomez M, et al. The Iberian pig fed with high-fat diet: a model of renal disease in obesity and metabolic syndrome. Int J Obes. (2020) 44:457–65. doi: 10.1038/s41366-019-0434-9
7. Hou N, Du X, Wu S. Advances in pig models of human diseases. Animal Model Exp Med. (2022) 5:141–52. doi: 10.1002/ame2.12223
8. Lunney JK, Van Goor A, Walker KE, Hailstock T, Franklin J, Dai C. Importance of the pig as a human biomedical model. Sci Transl Med. (2021) 13:621. doi: 10.1126/scitranslmed.abd5758
9. Kells NJ. The Five Domains model and promoting positive welfare in pigs. Animal. (2022) 16:100378. doi: 10.1016/j.animal.2021.100378
10. Hampton CE, Takawira C, Ross JM, Liu CC. Sedation characteristics of intranasal alfaxalone in adult yucatan swine. J Am Assoc Lab Anim Sci. (2021) 60:184–7. doi: 10.30802/AALAS-JAALAS-20-000099
11. Lin H. Preanesthetic considerations. In:Lin H, Passler T, Clark-Price S, , editors. Farm Animal Anesthesia: Cattle, Small Ruminants, Camelids, and Pigs. 2nd ed. Hoboken: John Wiley & Sons (2022). p. 1–15. doi: 10.1002/9781119672661.ch1
12. Posner LP. Sedatives and tranquilizers. In:Riviere JE, Papich MG, , editors. Veterinary pharmacology and therapeutics. 10th ed. Hoboken: John Wiley & Sons (2018). p. 324–50.
13. Axiak SM, Jäggin N, Wenger S, Doherr MG, Schatzmann U. Anaesthesia for castration of piglets: comparison between intranasal and intramuscular application of ketamine, climazolam and azaperone. Schweiz Arch Tierheilkd. (2007) 149:395–402. doi: 10.1024/0036-7281.149.9.395
14. Heinonen ML, Raekallio MR, Oliviero C, Ahokas S, Peltoniemi OAT. Comparison of azaperone-detomidine-butorphanol-ketamine and azaperone-tiletamine-zolazepam for anaesthesia in piglets. Vet Anaesth Analg. (2009) 36:151–7. doi: 10.1111/j.1467-2995.2008.00443.x
15. David C. Rankin. Sedatives and tranquilizers. In:Grimm KA, Lamont LA, Tranquilli WJ, Greene SA, Robertson SA, , editors. Veterinary anesthesia and analgesia: the fifth edition of Lumb and Jones. 5th ed. Ames: John Wiley & Sons (2015). p. 196–206. doi: 10.1002/9781119421375.ch10
16. Clarke KW, Trim CM, Hall LW. Chapter 4 - Principles of sedation, anticholinergic agents, and principles of premedication. In:Clarke KW, Trim CM, Hall LW, , editors. Veterinary Anaesthesia (Eleventh Edition). Eleventh Edition. Oxford: W.B. Saunders (2014). p. 79–100.
17. Annetta M, Iemma D, Garisto C, Tafani C, Proietti R. Ketamine: new indications for an old drug. Curr Drug Targets. (2005) 6:789–94. doi: 10.2174/138945005774574533
19. Soliman MG, Brindle GF, Kuster G. Response to hypercapnia under ketamine anaesthesia. Can Anaesth Soc J. (1975) 22:486–94. doi: 10.1007/BF03004864
20. Mestorino N, Marchetti ML, Daniele M, Martínez MA, Martínez-Larrañaga MR, Anadón A. Tissue depletion of azaperone and its metabolite azaperol after oral administration of azaperone in food-producing pigs. Rev Toxicol. (2013) 30:125–30.
21. Svoboda M, Blahova J, Jarkovsky J, Zacharda A, Hajkova S, Vanhara J, et al. Efficacy of the intranasal application of azaperone for sedation in weaned piglets. Vet Med (Praha). (2023) 68:145. doi: 10.17221/21/2023-VETMED
22. Lacoste L, Bouquet S, Ingrand P, Caritez JC, Carretier M, Debaene B. Intranasal midazolam in piglets: pharmacodynamics (02 vs 04 mg/kg) and pharmacokinetics (04 mg/kg) with bioavailability determination. Lab Anim. (2000) 34:29–35. doi: 10.1258/002367700780578073
23. Clarke KW, Trim CM, Hall LW. General pharmacology of the injectable agents used in anaesthesia. Veter Anaesth. (2014) 36:135–53. doi: 10.1016/B978-0-7020-2793-2.00006-2
24. Kmiec M. Die Kastration von Saugferkeln ohne und mit Allgemeinanaesthesie (Azaperone-Ketamine): Praktikabilitaet, Wohlbefinden und Wirtschaftlichkeit. Berlin: Freie Universität Berlin (2005).
25. Costantino HR, Illum L, Brandt G, Johnson PH, Quay SC. Intranasal delivery: physicochemical and therapeutic aspects. Int J Pharm. (2007) 337:1–24. doi: 10.1016/j.ijpharm.2007.03.025
26. Pardeshi CV, Belgamwar VS. Direct nose to brain drug delivery via integrated nerve pathways bypassing the blood-brain barrier: an excellent platform for brain targeting. Expert Opin Drug Deliv. (2013) 10:957–72. doi: 10.1517/17425247.2013.790887
27. Becker S, Maier A, Peters S, Büttner K, Reiner G. S-ketamine and intranasal application: alternatives for the castration of male suckling piglets? BMC Vet Res. (2021) 17:1–13. doi: 10.1186/s12917-021-02826-9
28. Seak YS, Nor J, Kamauzaman THT, Arithra A, Islam MA. Efficacy and safety of intranasal ketamine for acute pain management in the emergency setting: a systematic review and meta-analysis. J Clin Med. (2021) 10:3978. doi: 10.3390/jcm10173978
29. Gómez-Manzano FJ, Laredo-Aguilera JA, Cobo-Cuenca AI, Rabanales-Sotos J, Rodríguez-Cañamero S, Martín-Espinosa N, et al. Evaluation of intranasal midazolam for pediatric sedation during the suturing of traumatic lacerations: a systematic review. Children (Basel). (2022) 9:644. doi: 10.3390/children9050644
30. Van Sluyters RC, Obernier JA. Guidelines for the care and use of mammals in neuroscience and behavioral research. Contemp Top Lab Anim Sci. (2003) 43:48–52.
31. Miller JL, Ashford JW, Archer SM, Rudy AC, Wermeling DP. Comparison of intranasal administration of haloperidol with intravenous and intramuscular administration: a pilot pharmacokinetic study. Pharmacotherapy. (2008) 28:875–82. doi: 10.1592/phco.28.7.875
32. Kerr D, Kelly AM, Dietze P, Jolley D, Barger B. Randomized controlled trial comparing the effectiveness and safety of intranasal and intramuscular naloxone for the treatment of suspected heroin overdose. Addiction. (2009) 104:2067–74. doi: 10.1111/j.1360-0443.2009.02724.x
33. Van Essen GJ, Hekkert MTL, Sorop O, Heinonen I, Van Der Velden J, Merkus D, et al. Cardiovascular function of modern pigs does not comply with allometric scaling laws. Sci Rep. (2018) 8:792. doi: 10.1038/s41598-017-18775-z
34. Catherine E D, Barbara E S. Herd examination. In:Barbara ES, Jeffery JZ, Sylvie D, David JT, , editors. Diseases of Swine. 9th ed. Hoboken: John Wiley & Sons (2006). p. 3–14.
35. Gianotti GC, Beheregaray WK, Bianchi SP, Mombach VS, Carregaro AB, Contesini EA. Suíno como modelo experimental na pesquisa biomédica: valores fisiológicos normais. Acta Sci Vet. (2010) 38:133–7. doi: 10.22456/1679-9216.16610
36. Hannon JP, Bossone CA, Wade CE. Normal physiological values for conscious pigs used in biomedical research. Lab Anim Sci. (1990) 40:293–8.
37. Hamilton DN, Ellis M, Bertol TM, Miller KD. Effects of handling intensity and live weight on blood acid-base status in finishing pigs. J Anim Sci. (2004) 82:2405–9. doi: 10.2527/2004.8282405x
38. Klem TB, Bleken E, Morberg H, Thoresen SI, Framstad T. Hematologic and biochemical reference intervals for Norwegian crossbreed grower pigs. Vet Clin Pathol. (2010) 39:221–6. doi: 10.1111/j.1939-165X.2009.00199.x
39. Posner LP. Injectable anesthetic agents. In:Riviere JE, Papich MG, , editors. Veterinary pharmacology and therapeutics. 10th ed. Hoboken: John Wiley & Sons (2018). p. 247–79.
40. McDonell WN, Kerr CL. Physiology, pathophysiology, and anesthetic management of patients with respiratory disease. In:Grimm KA, Lamont LA, Tranquilli WJ, Greene SA, Robertson SA, , editors. Veterinary Anesthesia and Analgesia. Hoboken: John Wiley & Sons (2015). p. 511–55. doi: 10.1002/9781119421375.ch27
41. Dyson MC, Jirkof P, Lofgren J, Nunamaker EA, Pang D. Anesthesia and Analgesia in Laboratory Animals. London: Academic Press. (2023). doi: 10.1016/B978-0-12-822215-7.00007-X
42. Kornegay ET, Miller ER, Brent BE, Long CH, Ullrey DE, Hoefer JA. Effect of fasting and refeeding on body weight, rectal temperature, blood volume and various blood constituents in growing swine. J Nutr. (1964) 84:295–304. doi: 10.1093/jn/84.3.295
43. Becerril-Herrera M, Alonso-Spilsbury M, Ortega MET, Guerrero-Legarreta I, Ramírez-Necoechea R, Roldan-Santiago P, et al. Changes in blood constituents of swine transported for 8 or 16 h to an Abattoir. Meat Sci. (2010) 86:945–8. doi: 10.1016/j.meatsci.2010.07.021
44. Cornick JL, Hartsfield SM. Cardiopulmonary and behavioral effects of combinations of acepromazine/butorphanol and acepromazine/oxymorphone in dogs. J Am Vet Med Assoc. (1992) 200:1952–6. doi: 10.2460/javma.1992.200.12.1952
Keywords: tranquilizers, benzodiazepines, chemical restraint, animal model, anesthesia
Citation: Rabelo IP, Gujanwski CA, Viana IS, de Paula VB, Rein A, Rabelo SP and Valadäo CAA (2024) Intranasal vs. intramuscular administration of azaperone, midazolam and ketamine in pigs. Front. Vet. Sci. 11:1408103. doi: 10.3389/fvets.2024.1408103
Received: 27 March 2024; Accepted: 09 September 2024;
Published: 25 September 2024.
Edited by:
Karine Portier, Université de Lyon, FranceReviewed by:
Aslıhan Şengelen, Istanbul University, TürkiyeDean Konjević, University of Zagreb, Croatia
Copyright © 2024 Rabelo, Gujanwski, Viana, de Paula, Rein, Rabelo and Valadäo. This is an open-access article distributed under the terms of the Creative Commons Attribution License (CC BY). The use, distribution or reproduction in other forums is permitted, provided the original author(s) and the copyright owner(s) are credited and that the original publication in this journal is cited, in accordance with accepted academic practice. No use, distribution or reproduction is permitted which does not comply with these terms.
*Correspondence: Isabela Peixoto Rabelo, SXNhYmVsYS5wZWl4b3RvJiN4MDAwNDA7dW5lc3AuYnI=