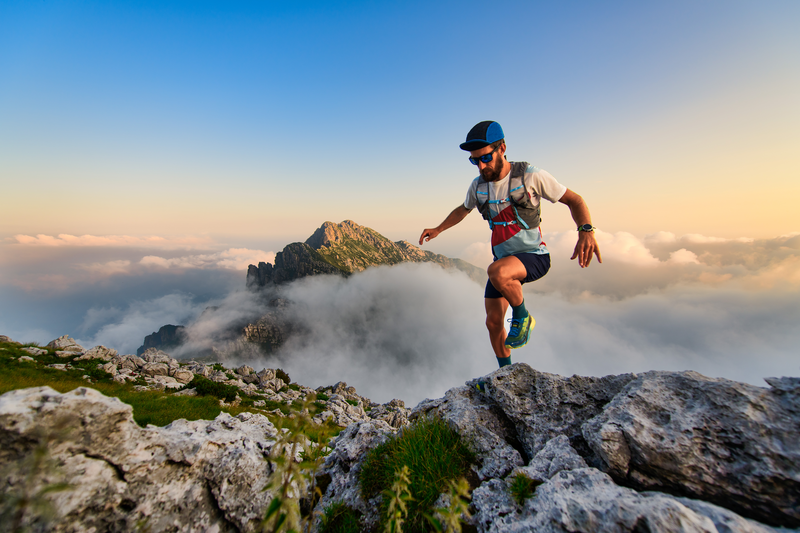
95% of researchers rate our articles as excellent or good
Learn more about the work of our research integrity team to safeguard the quality of each article we publish.
Find out more
ORIGINAL RESEARCH article
Front. Vet. Sci. , 02 July 2024
Sec. Animal Nutrition and Metabolism
Volume 11 - 2024 | https://doi.org/10.3389/fvets.2024.1393335
Background: The potential significance and importance of green iron nanoparticles (Nano-Fe) in poultry production lie in their capability to effectively tackle iron deficiency in poultry. Iron, an indispensable mineral for numerous physiological functions in birds, such as oxygen transport, energy metabolism, and immune response, underscores the critical need for adequate iron levels. Nevertheless, conventional iron supplementation methods frequently face hurdles like limited bioavailability rates in poultry. To enhance performance, and promote sustainable broiler productivity, Nano-Fe showed promise as an efficient feed supplement for broiler chickens. The objective of this study was to assess the impact of green Nano-Fe inclusions in diets on growth, ammonia excretion, carcass criteria, and meat quality in broiler chickens.
Methods: A total of 192 one-day-old male Ross 308 broiler chicks, were assigned to three treatment diets including Nano-Fe oxide at 0, 20, or 40 mg/kg, respectively, for 42 days. Each treatment comprised eight replicates, each with eight broiler chicks. Two phases comprised the 42-day study (0 to 21 days for the starter and 21 to 42 days for the finisher).
Results: In comparison to the control group, the Nano-Fe oxide groups 20 mg/kg and 40 mg/kg linearly improved (p < 0.05) body weight (R2 = 0.574) and body weight gain (R2 = 0.367) under hot climatic conditions at 42 days of age. Furthermore, Nano-Fe oxide to broiler diets, improved (linear, p < 0.05) feed conversion ratio (R2 = 0.424) throughout whole periods. The feed intake did not show any significant difference (p > 0.05) among groups during the experimental periods under hot climatic conditions. The ammonia content of excreta (R2 = 0.454) was linearly decreased (p < 0.05) with increasing Nano-Fe oxide levels in broiler diets compared to control at 21 and 42 days of age under hot climatic conditions. Nano-Fe oxide positively influences cook loss, water-holding capacity, and iron content in various tissues. Moreover, it contributes to a healthier carcass yield and reduced abdominal fat.
Conclusion: In conclusion, broiler chickens fed diets containing Nano-Fe oxide at 20 mg/kg and 40 mg/kg demonstrated enhanced growth performance, improved meat quality, increased iron content in tissues, higher dressing percentage, and reduced abdominal fat deposition. Future research should explore the impact of green Nano-Fe oxide on additional factors such as the microbiome and gene expression related to immunity and heat stress.
Iron (Fe) is essential for various physiological functions in chickens, including oxygen transport, enzyme activation, and immune system function (1). In broiler chickens, iron is primarily found in hemoglobin (60–70%), myoglobin, cytochromes, and other iron-containing enzymes (10%), alongside ferritin and hemosiderin (20–30%) (2). Maintaining systemic iron balance in poultry primarily involves controlling dietary iron uptake and release from recycling macrophages and hepatocytes, as the body does not regulate iron losses (3). However, conventional methods of iron supplementation often rely on inorganic iron sources, which can exhibit lower bioavailability and may contribute to environmental pollution through runoff and leaching (4). Moreover, Fe in broiler chicken feed can often be combined with phytate, a compound commonly found in plant-based feed ingredients like grains (5). Phytate binds to minerals such as iron, forming complexes that are not easily absorbed by the bird’s digestive system. This reduces the bioavailability of iron, meaning that even though it’s present in the feed, the chickens may not be able to absorb and utilize it effectively (6). This can lead to suboptimal iron levels in the birds, impacting their growth, health, and productivity. To address this issue, feed formulators may explore alternative forms of iron supplementation, such as Nano-Fe, which could potentially improve iron absorption and utilization in broiler chickens. Nano-Fe, or nanoscale iron particles, offers a promising solution to this problem by providing a more efficient and sustainable alternative for iron supplementation in broiler diets (7). Nano-Fe exhibits enhanced bioavailability due to its smaller particle size and increased surface area. This enhanced bioavailability allows for better absorption and utilization of iron by broiler chickens, leading to improved growth rates, feed efficiency, and overall health. Moreover, Nano-Fe supplementation has the potential to reduce the environmental burden associated with iron supplementation in broiler diets (8). By utilizing nanotechnology, Nano-Fe can be precisely engineered to deliver iron in a targeted and controlled manner, minimizing waste and reducing the need for excess supplementation (9) which may help preserve ecosystems and promotes environmental sustainability in broiler production systems. Additionally, Nano-Fe particles can be designed to be more stable in the gastrointestinal tract, ensuring that a higher proportion of the supplemented iron is absorbed by the bird rather than excreted (10). Nano-Fe offers a promising avenue for supporting sustainable broiler chicken meat production by reducing the need for dietary iron extraction (11, 12).
Green nanotechnology refers to the use of environmentally friendly processes and materials in the synthesis of nanoparticles. This approach often involves using natural sources, such as plant extracts or microorganisms, to reduce and stabilize nanoparticles. The use of green nanotechnology is driven by the desire to minimize the environmental impact and potential toxicity associated with traditional nanoparticle synthesis methods (13). Basil (Ocimum basilicum) in the diets of broiler chickens increased immune systems, nutrient digestibility, antioxidant activities, and productivity (14), which is mainly due to various phytochemicals, including polyphenols, flavonoids, and terpenoids (15), and may also act as stabilizing agents in the synthesis of nanoparticles.
Overall, green Nano-Fe represents may a promising solution to the problem of iron supplementation in broiler diets. Its enhanced bioavailability, targeted delivery, and reduced environmental impact make it an attractive option for poultry producers seeking to optimize broiler performance while promoting sustainability. In our prior investigation, it was demonstrated that incorporating green Nano-Fe into broiler diets, either with Halimeda opuntia as a carrier or independently, has the potential to enhance broiler performance and health, particularly in hot environmental conditions (16). There is still much to learn about the long-term effects, optimal dosage, and specific mechanisms of action of Nano-Fe in poultry. Continued research is necessary to fill these gaps and provide a more comprehensive understanding of their potential benefits and risks. Likewise, optimizing growth performance, improving meat quality, increasing iron content in tissues, achieving higher dressing percentage, and reducing abdominal fat deposition are essential facets of broiler chicken production. Therefore, this study aimed to investigate the effects of Nano-Fe oxide on growth, excreta ammonia emission, carcass criteria, and meat quality of male broiler chicken.
The animal study protocol received approval from the Institutional Animal Care and Use Committee at the University of Alexandria, Egypt (AU08220810298). A total of 192 one-day-old male Ross 308 broiler chicks (with an average body weight of 42.98 ± 0.2 grams) were allocated to three treatment diets, each containing Nano-Fe oxide at 0, 20, or 40 mg/kg, respectively, over a 42-day period. Each treatment group consisted of eight replicates, with eight broiler chicks in each replicate. The birds were fed three different levels of Nano-Fe within a basal control diet: 0, 20, and 40 mg/kg Nano-Fe for the three treatments. The study comprised two phases: the starter phase (0 to 21 days) and the finisher phase (22 to 42 days). Notably, the 20 mg and 40 mg Nano-Fe oxide/kg experimental diets used in this study fell below the minimally recommended dose of 85 mg Fe oxide/kg according to NRC guidelines (17). The chosen Nano-Fe levels aligned with existing research that suggested dietary Fe concentrations ranging from 10 to 60 mg/kg in diets without supplements, up to approximately 160 mg in diets supplemented with 140 mg Fe-Gly or 100 mg Nano-Fe (18, 19). The diets were meticulously crafted to adhere to the precise nutritional requirements outlined for Ross 308 broilers (Table 1), across all growth phases. Throughout the experimental period, the chicks had unrestricted access to feed and water. The study was conducted at the Poultry Center within the Faculty of Agriculture at South Valley University in Qena, Egypt. The chicken cages measured 120 × 70 × 50 cm (length, breadth, and height), with each cage considered as a replicate. Within each cage, there were four nipple drinkers and hanging linear feeders. The nipple line height was adjusted as the birds grew. The chicks were kept on a 23/1 light/dark photoperiod during the whole experimental period. The light intensity was maintained at 35 lux until day 7 and was then reduced to 5–10 lux on day 8 onwards. During the dark period, the light intensity remained below 4 lux according to management requirements outlined for Ross 308 broilers. Indoor conditions included an average ambient temperature of 34.5°C (45% relative humidity) from 1 to 21 days of age, followed by 28.5°C, 40% relative humidity, and a temperature-humidity index (THI) of 29.9 from 22 to 42 days of age. The THI calculation is as follows: THI = db°C − ((0.31–0.31RH) × (db°C − 14.4)), where db is the dry bulb temperature in degrees Celsius and RH is the relative humidity percentage/100. THI values calculated were then classified as follows: 27.8 = no heat stress, 27.8–28.9 = moderate heat stress, 28.9–30.0 = severe heat stress, and >30.0 = extremely severe heat stress (20). The main dietary ingredients for broiler chickens included corn, sorghum, and soybean meal. Sorghum stands out as a valuable feed option for poultry due to its cost-effectiveness and favorable nutrient profile. The diets’ chemical compositions in Table 1 were determined according to the methods of the Association of Official Agricultural Chemists (AOAC) (21). While phytase and carbohydrase enzymes may offer benefits in certain scenarios, their inclusion can also increase feed costs. Therefore, to isolate the effects of Nano-Fe supplementation and minimize potential confounding factors, we did not include these enzymes in the experimental diets.
Fresh basil leaves (Ocimum basilicum) were sourced from the farm of the Faculty of Agriculture at South Valley University in Qena, Egypt, where basil was cultivated. The leaves were carefully harvested, cleaned under running water, and rinsed with deionized water. After detaching the leaves from the stems, they were left to air dry at room temperature and subsequently ground into a fine powder. In an Erlenmeyer flask, 100 grams of powdered basil sample were mixed with 1,000 milliliters of double-distilled water while continuously swirling for 15 min to create the extract. Once the extract reached room temperature, it was filtered using Whatman filter paper.
Following the maceration technique described by Khalil et al. (22), we synthesized green iron oxide nanoparticles using leaf extract from Ocimum basilicum. In summary, we heated 30 grams of plant powder and 200 milliliters of distilled water to 80°C for 1 h using a hot-plate magnetic stirrer. Solid residues were removed by triple filtration with Whatman No. 1 filter paper. The resulting filtered solution, with a pH of 5.7, was further heated for 2 h at 85°C. Next, we introduced 100 milliliters of Fe (III) chloride (6 grams) as a precursor salt. The solution transitioned from brownish to violet in color, and its pH was recorded. After allowing the mixture to cool to room temperature, we employed decantation to extract the iron oxide nanoparticles. Following three cycles of distilled water washing, the Fe oxide particles were air-dried at room temperature. These particles were subsequently characterized using a transmission electron microscope (TEM) (Figure 1).
Throughout the entire experiment, we diligently recorded the body weight of each cage once a week. Additionally, on the days when the birds were weighed, we carefully measured feed residue to determine the amount of feed consumed between weigh-ins. The feed conversion ratio was then calculated by dividing the weight of feed consumed by the body weight gain of each cage.
To assess ammonia excretion, feces were collected at 21 and 42 days of age per cage, following the method proposed by Miles et al. (23). Freshly collected excrement (200 grams) was placed in a 1,000 mL jar. The upper portion of the jar was sealed with a rubber stopper, featuring both an exhaust pipe and an intake pipe connected to a U-shaped bubble absorption tube. Shielded from light, the U-shaped tube contained approximately 10 mL of a 2% boric acid solution. The other end of the U-shaped tube was linked to an inflating pump via a second buffering device, while the intake pipe was connected to a separate buffer device. In an acidic environment, the 2% boric acid solution effectively captured the ammonia gas produced from chicken feces, converting it into stable . Using the Kjeldahl nitrogen determination method, we quantified the nitrogen content in the absorption solution, which was then translated into NH3 content based on the unit mass of feces (fresh weight basis).
A total of 40 birds were selected that represented the pen. Each treatment group consisted of eight replicates, with five broiler chicks in each replicate for measuring the carcass criteria and internal organs at 42 days of age. These birds were weighed, humanely slaughtered using the Halal method, and then plucked. The remaining portion of the body was weighed to determine the dressed weight after removing the head, neck, shanks, viscera, abdominal fat, digestive tract, spleen, liver, gizzard, and heart. The dressing percentage was calculated as the ratio of dressed weight to live weight, multiplied by 100. Additionally, the individual weights of each bird’s liver, heart, empty gizzard, and abdominal fat were recorded, and their proportions relative to the live body weight were determined.
To evaluate water holding capacity (WHC) and cooking loss, we examined the left side of the breast muscle and the left leg in 40 birds per treatment consisted of eight replicates, with five broiler chicks in each replicate. The low-speed centrifugation technique was employed to quantify WHC in breast muscles with minimal adjustments (24). Specifically, 10 grams of intact breast muscle were placed in a falcon tube containing glass beads and centrifuged for 20 min at 10,000 g and 5°C. The resulting precipitated meat was promptly removed, dried using filter paper, and reweighed. WHC was calculated based on the weight loss in muscle samples after centrifugation. Cooking loss was also determined, as previously described (25). In summary, muscle fillets were individually placed in thin-walled, thermotolerant polyethylene bags and cooked in a water bath until their core temperatures reached 70°C. Subsequently, the samples were refrigerated in crushed ice until they reached 5°C, and cooking loss was calculated by reweighing them. Additionally, samples of liver, breast, leg, and blood were collected and stored at −20°C for subsequent iron (Fe) analysis.
Blood was collected from the wing veins of 40 birds per treatment (5 birds per replication) and placed in vacutainer tubes to obtain serum. Samples of breast, leg, liver, and serum were obtained from slaughtered chickens (n = 40) and immediately placed in −20°C freezers for Fe analysis. The Fe concentrations were measured using an atomic absorption spectrophotometer (Perkin Elmer Analyst 800 model, Shelton, CT, United States).
In this study, a complete randomized design was employed. The data underwent analysis using SAS 9.2 software, utilizing the General Linear Models (GLM) technique for statistical assessment SAS Institute (26). The supplementation dosage remained constant throughout the model. Growth performance was evaluated at the cage level, while ammonia, carcass criteria, meat quality, and tissue iron content were assessed at the individual bird level. Graphs and a normal distribution test (specifically, the Anderson-Darling test for normality) were generated using GraphPad Prism software, version 9 (GraphPad Software, La Jolla, CA, United States). The effects of increasing Nano-Fe supplementations were determined through orthogonal polynomial contrasts. Duncan multiple range tests were employed for mean comparisons. A significance level of p < 0.05 was utilized, with p-values less than 0.001 expressed as “<0.001.”
The findings demonstrated that Nano-Fe oxide supplementation significantly increased (p < 0.05) body weight at starter and finisher periods as well as daily body weight gain compared to control at overall periods (Table 2). In comparison to the control group, the Nano-Fe oxide groups 20 mg/kg and 40 mg/kg improved (p < 0.05) body weight and body weight gain under hot climatic conditions. Furthermore, there were no significant differences between 20 mg/ kg and 40 mg/kg in body weight and body weight gain. Adding Nano-Fe oxide to broiler diets, improved (p < 0.05) feed conversion ratio throughout the experimental finisher and whole periods (Table 3). Under hot climatic conditions, the supplementation of 40 mg/kg Nano-Fe oxide had a significantly better feed conversion ratio than 20 mg/ kg Nano-Fe oxide and control. The feed intake did not show any significant difference among groups during the experimental periods under hot climatic conditions (Table 3).
Effects of Nano-Fe oxide supplementations on feces ammonia content during 21 days of age and 42 days of age are presented in Figure 2. The ammonia content of excreta was significantly decreased (p < 0.05) with increasing Nano-Fe oxide levels in broiler diets compared to control during 21 and 42 days of age under hot climatic conditions. In addition, there were no significant differences in the ammonia concentration of excreta between 20 and 40 mg/kg.
Figure 2. Effects of Nano-Fe oxide supplementation on broiler chicken feces ammonia contents at 21 (A) and 42 (B) days of age. Bars with different letters (a, b) are significantly different (p < 0.05). SEM: Standard error of the means (n = 8).
According to the data of carcass criteria, broilers fed diets containing Nano-Fe oxide at 20 mg/kg and 40 mg/kg showed increases (p < 0.05) in dressing percentage and decreases (p < 0.05) in abdominal fat compared to control (Table 4). However, there were no differences in the dressing percentage and abdominal fat between 20 mg/kg and 40 mg/kg under hot climatic conditions. Supplementation of Nano-Fe oxide had no effect (p > 0.05) on the percentages of liver, heart, gizzard, and spleen of broilers.
Table 4. Effects of Nano-Fe oxide on dressing, abdominal fat, and internal organs (as the percentages of live body weight) of broiler chickens (42 days of age).
Regarding the physicochemical qualities of meat, the WHC % of the breast muscles and leg muscles improved linearly (p < 0.05) with respect to the levels of Nano-Fe oxide at 42 days of age in hot climates (Figure 3). Under hot climate conditions, the cook loss percentage of the breast (p < 0.05) and leg (p < 0.05) muscles at 42 days of age was lowered at 20 mg/kg and 40 mg/kg Nano-Fe oxide levels, respectively (Figure 4). The results revealed that WHC% in breast or leg meat was not different between 20 mg/kg and 40 mg/kg Nano-Fe oxide. However, Nano-Fe oxide supplementation at 20 mg/kg decreased cook loss compared to 40 mg/kg in breast muscles, but no differences were found in leg meat under hot climatic conditions.
Figure 3. Effects of Nano-Fe oxide supplementation on water holding capacity (WHC) of the breast (A) and leg (B) muscles in broilers at 42 days of age. Bars with distinct letters (a, b) differ significantly (p < 0.05). SEM: Standard error of the means (n = 40).
Figure 4. Effects of Nano-Fe oxide supplementation on cooking loss of the breast (A) and leg (B) muscles in broilers at 42 days of age. Bars with distinct letters (a, b, c) differ significantly (p < 0.05). SEM: Standard error of the means (n = 40).
Supplementation of Nano-Fe oxide to broiler diets improved (p < 0.05) the Fe contents in the breast and leg meat compared to control at 42 days of age (Figure 5). Overall, the Fe content in breast and leg meat was higher in birds fed on Nano-Fe oxide at 40 mg/kg, compared to birds fed on Nano-Fe at 20 mg/kg and control diets, respectively. The Fe content in liver tissues and serum increased (p < 0.05) with the increasing levels of Nano-Fe oxide levels compared to control under hot climatic conditions (Figure 6).
Figure 5. Effects of Nano-Fe oxide supplementation on Fe content in the breast (A) and leg (B) muscles of broilers at 42 days of age. Bars with different letters (a, b, c) are significantly different (p < 0.05). SEM: Standard error of the means (n = 40).
Figure 6. Effects of Nano-Fe oxide supplementation on Fe content of the liver muscles (A) and serum (B) in broilers at 42 days of age. Bars with different letters (a, b, c) are significantly different (p < 0.05). SEM: Standard error of the means (n = 40).
In the current study, the iron (Fe) concentrations in the starter and grower diets for broilers were 0.024 and 0.026%, respectively. Additionally, green Nano-Fe was supplemented to the broiler diets at levels of 20 mg/kg and 40 mg/kg. The Fe concentrations in these diets varied, with levels typically ranging from 20 to 80 mg/kg as in other previous studies (17–19). The addition of different iron (Fe) concentrations to broiler diets from previous studies reveals inconsistent outcomes, suggesting a complex interplay of factors influencing Fe utilization and its effects on broiler performance. One significant factor contributing to these inconsistencies is the form and bioavailability of Fe sources used in the diets. Research has shown that the bioavailability of Fe can vary significantly depending on its chemical form and particle size. For example, studies comparing the efficacy of various Fe sources, such as inorganic Fe salts, organic Fe chelates, and Fe nanoparticles, have demonstrated differences in their absorption and utilization by broilers (27, 28). Additionally, variations in dietary composition and nutrient interactions can impact Fe absorption and utilization in broilers. Certain dietary components, such as phytate, can form complexes with Fe, reducing its bioavailability in the gastrointestinal tract. Conversely, other dietary factors, such as vitamin C and organic acids, may enhance Fe absorption (29). Furthermore, genetic factors play a role in determining broiler Fe requirements and response to Fe supplementation. Variations in broiler genetics can influence Fe metabolism and utilization efficiency, affecting growth performance and other physiological parameters (30).
In the current study, supplementation of Nano-Fe oxide at 20 mg/kg and 40 mg/kg to broiler diets improved growth performance compared to control. Green nanotechnology feeding is one of the most potential nutritional techniques, and it deserves more research to improve productive performance of broiler chickens. Fe is a necessary nutrient for chickens, and its bioavailability can be increased by using nanoparticles. Although nanoparticles frequently have more surface area, nanoparticles may be better absorbed in the digestive system. The improved bioavailability of Nano-Fe oxide during hot climatic conditions can benefit broiler growth and overall health. Because Fe is a component of hemoglobin (31), it has a significant role in cellular and whole-body energy metabolism and is essential for protein metabolism (32), chickens are particularly vulnerable to Fe deficiency. Utilizing Nano-Fe in poultry feed displays potential for enhancing feed efficiency. Research on incorporating green Nano-Fe oxide as a feed supplement for poultry in hot climates is limited. Our recent study demonstrates that supplementing Nano-Fe in broiler diets enhances feed conversion ratio between days 21–42 and 1–42 in hot climates, which holds significant importance for the economic viability of poultry production. Few investigations have explored the use of green Nano-Fe as a dietary additive for chickens. Rehman et al. (33) reported that the addition of Nano-Fe and xylanase to broiler feed increased body weight by 45% and improved the feed conversion ratio values at 35 days of age than the control group. The addition of 40–160 mg Fe/kg from Fe-Gly resulted in increasing responses, with the highest body weight gain and feed conversion ratio recorded in broiler chicks fed 100 mg Fe/kg (19). Nikonov et al. (34) observed enhanced production rates in broiler chickens by incorporating Fe oxide nanoparticles into their diet, without any adverse effects. Similarly, Srinivasan et al. (19) discovered that adding 10–20 mg/kg of FeO nanoparticles to Macrobrachium rosenbergii feed led to improved growth and satisfactory feed conversion ratios. However, supplementing prawn feed with 30–50 mg/kg FeO nanoparticles resulted in negative effects, increasing feed conversion ratios. Sarlak et al. (35) demonstrated that dietary Fe supplementation in chicken diets improved performance parameters such as feed intake and feed conversion ratio compared to control treatments. Iron is crucial for hemoglobin and myoglobin, facilitating oxygen delivery and cellular utilization. Supplementing Nano-Fe in broiler diets notably increased body weight gain by 8% compared to diets lacking Nano-Fe (36). Furthermore, in the current study, the ammonia content of feces was decreased with increasing green Nano-Fe levels compared to control under hot climatic conditions. Nano-Fe might help to upregulate the functional pathway of nitrogen metabolism in intestinal microbes, increasing the use of nitrogenous substances in the host intestine and lowering ammonia removal through manure (37, 38). Importantly, fulvic acid supplementation to broiler diets decreased the fecal ammonia output in a dose-dependent manner as well as the urease activity (39). The microbial production of uric acid and urea in feces produces a significant amount of ammonia, which causes respiratory illnesses and chronic stress in livestock and poultry (38, 40). Ammonia is created through the deamination of amino acids and the hydrolysis of urea. Changes in ammonia content, as well as ammonia uptake through epithelial cells, have an impact on the microbiota (40). Furthermore, as ammonia levels in the gut dropped, the compensatory effect of ammonia on intestinal cells was reduced, resulting in improvements in the intestinal barrier and histomorphology of the host intestine (41). In the current study, Fe in the breast, leg, liver, and blood was significantly enhanced by adding Nano-Fe oxide to broiler diets. Fe is stored in large quantities in the body, primarily in the liver, and bone marrow reticuloendothelial cells (31). Dietary demands for Fe can be modulated to increase or decrease its rate of absorption via various known pathways depending on the body’s Fe status (3). These processes are linked to receptors on the surface of enterocytes, such as the heme carrier protein 1, which is responsible for heme-Fe absorption in the intestine (42), and the divalent metal transporter 1, which can take inorganic Fe2+ and release it directly into the cytoplasm (43). Given that organic Fe absorbs more readily than inorganic Fe, animal byproducts containing muscle tissue and blood have higher Fe availability for poultry (3). Ma et al. (44) investigated the dietary Fe needs of broilers aged 1 to 21 days and discovered that 97 to 136 mg Fe/kg was necessary to sustain their complete expression in various tissues. In addition, serum ferritin levels were considerably higher in diets supplemented with 75, 150, or 300 mg/kg Fe, but not in diets supplemented with 600 mg/kg Fe (45). The Fe content of chick serum increased progressively as the Fe level in the diet increased (35). A large dose of ferrous methionine dramatically raised the hepatic Fe concentration in Ross broilers, according to research by Seo et al. (46). According to Ma et al. (47), the Fe content in broiler liver steadily decreased when dietary Fe levels increased beyond 120 mg/kg. This might be the result of the liver’s ability to keep the right ratio of Fe in the body, preventing excessive Fe deposition that could be harmful. The broiler strains and variety, as well as their feed, may be responsible for the heterogeneity shown in earlier research.
In the current investigation, supplementation of green Nano-Fe oxide to broiler diets significantly improved percentages of carcass dressing and reduced abdominal fat without any side effects on internal organs at 42 days of age under hot climatic conditions. The possible explanation is that Nano-Fe supplementation enhances nutrient absorption and utilization efficiency, leading to more efficient energy metabolism and reduced fat deposition (48). Additionally, Nano-Fe has been reported to exert antioxidant and anti-inflammatory effects, which may contribute to improvements in metabolic health and energy metabolism in broilers (49). Furthermore, Nano-Fe nanoparticles have been shown to interact with cellular signaling pathways involved in lipid metabolism and adipogenesis, potentially leading to alterations in fat deposition patterns (50). The results are consistent with Rehman et al. (33) who found that the Nano-Fe oxide has a lot of potential for usage in chicken feed for large-scale meat production without any negative toxicological effects. Our results are consistent with Lin et al. (51) who reported that weight indices of the liver, kidneys, spleen, thymus, and bursa of Fabricius were not influenced by the different levels of Fe at 50, 70, 90, 110, 130, and 150 mg/kg compared to control. However, it’s important to note that further research is needed to elucidate the specific mechanisms by which dietary Nano-Fe influences energy metabolism and fat deposition in broiler chickens. Future studies should investigate the effects of Nano-Fe supplementation on metabolic parameters, such as hormone levels, enzyme activities, and gene expression related to energy metabolism and lipid metabolism pathways. The current study found that Nano-Fe improved meat quality including WHC and cooking loss under heat stress conditions, which could be attributed to the Fe improving antioxidant activation. Fe has a crucial role in meat quality and Fe metabolism in broilers because it is a necessary component of Fe-containing key enzymes (52). A recent study (53) found that Fe supplementation improved enzymatic antioxidant protection in chicken serum. In addition, Kurtoglu et al. (54) showed that Fe-deficiency anemia lowered plasma antioxidant activities. As far as we are aware, there are no published articles on the effect of green Nano-Fe on broiler chicken carcass criteria and meat quality. Supplementation of Fe to broiler diet with 90 mg/kg Fe decreased drip loss of breast muscle compared to the control diet (47). Fe is a necessary component of hemoglobin in erythrocytes and is used by both hemoglobin and myoglobin (55, 56) for the delivery, storage, and utilization of oxygen in muscles (57). The greatest noticeable indicator of meat quality, color, is mostly determined by hemoglobin and myoglobin (58). Nanotechnology has significant implications for poultry nutrition and it offers targeted nutrient delivery, promotes growth, enhances meat quality, and improves mineral bioavailability. Overall, nanotechnology holds promise for revolutionizing poultry production and addressing industry challenges. The application of Nano-Fe oxide in poultry production holds promise for improving nutritional efficiency and meat quality as well as Fe content in tissue under heat stress. However, thorough research, including safety assessments and regulatory considerations, is necessary to ensure the effective implementation of this technology in the poultry industry. Specific thresholds for Fe levels in broiler chickens for human consumption can differ between countries and regions. For example, in the European Union, the maximum residue limits for Fe in poultry meat is set at 150 mg/kg (Commission Regulation (EU) No 37/2010). In the United States, the Food and Drug Administration (FDA) establishes tolerances for residues of Fe in food products, including poultry meat, based on safety assessments conducted by the agency. However, to provide a more comprehensive assessment of Fe levels in broiler meat and their implications for human consumption, further studies may be warranted. Future research should explore the relationship between dietary Fe supplementation, Fe levels in broiler meat, and potential health outcomes for consumers.
In conclusion, broiler chickens fed diets containing Nano-Fe oxide at 20 mg/kg and 40 mg/kg exhibited enhanced growth performance, improved meat quality, higher Fe content in tissues, favorable dressing percentage, and reduced abdominal fat. However, no significant effects were observed on internal organs. Future research should explore the impact of green Nano-Fe oxide on additional factors, including the microbiome and gene expression related to immunity and heat stress.
The raw data supporting the conclusions of this article will be made available by the authors, without undue reservation.
The animal study was approved by Institutional Animal Care and Use Committee of the University of Alexandria, Egypt (AU08220810298). The study was conducted in accordance with the local legislation and institutional requirements.
YA: Formal analysis, Methodology, Writing – original draft, Writing – review & editing. AE: Conceptualization, Formal analysis, Methodology, Writing – original draft, Writing – review & editing. EE: Conceptualization, Formal analysis, Methodology, Writing – original draft, Writing – review & editing. JL: Writing – original draft, Writing – review & editing. AA-W: Conceptualization, Formal analysis, Methodology, Writing – original draft, Writing – review & editing.
The author(s) declare that financial support was received for the research, authorship, and/or publication of this article.
The Poultry Production Department of the Faculty of Agriculture at South Valley University in Qena, Egypt, and the Poultry Production Department, Faculty of Agriculture, Alexandria University, Egypt are gratefully acknowledged for providing trail facilities and labs. Financial support provided by the Science & Technology Development Fund of Egypt (STDF-US-J102) to AA-W at Prairie View A&M University, Prairie View, Texas, United States as a Visiting Scientist is gratefully acknowledged.
The authors declare that the research was conducted in the absence of any commercial or financial relationships that could be construed as a potential conflict of interest.
The author(s) declared that they were an editorial board member of Frontiers, at the time of submission. This had no impact on the peer review process and the final decision.
All claims expressed in this article are solely those of the authors and do not necessarily represent those of their affiliated organizations, or those of the publisher, the editors and the reviewers. Any product that may be evaluated in this article, or claim that may be made by its manufacturer, is not guaranteed or endorsed by the publisher.
1. Jahanian, R, and Rasouli, E. Dietary iron source and level affect performance and iron metabolism in broiler chickens. J Anim Physiol Anim Nutr. (2015) 99:1131–40.
2. Theil, EC . Iron, ferritin, and nutrition. Annu Rev Nutr. (2004) 24:327–43. doi: 10.1146/annurev.nutr.24.012003.132212
3. Grotto, HZW . Iron metabolism: an overview on the main mechanisms involved in its homeostasis. Rev Bras Hematol Hemoter. (2008) 30:390–7. doi: 10.1590/S1516-84842008000200003
4. Hänsch, R, and Mendel, RR. Physiological functions of mineral micronutrients (Cu, Zn, Mn, Fe, Ni, Mo, B, Cl). Curr Opin Plant Biol. (2009) 12:259–66. doi: 10.1016/j.pbi.2009.05.006
5. Selle, PH, and Ravindran, V. Microbial phytase in poultry nutrition. Anim Feed Sci Technol. (2008) 145:101–13.
6. Olanrewaju, HA, Thaxton, JP, and Dozier, WA. Iron amino acid chelate in broiler chicken production: a review. Int J Poult Sci. (2017) 16:143–51.
7. Gibson, RS, Bailey, KB, Gibbs, M, and Ferguson, EL. A review of phytate, iron, zinc, and calcium concentrations in plant-based complementary foods used in low-income countries and implications for bioavailability. Food Nutr Bull. (2010) 31:S134–46. doi: 10.1177/15648265100312S206
8. Khorrami, B, Dastar, B, and Jahanian, R. Effects of dietary iron oxide nanoparticles supplementation on growth performance, blood metabolites, meat quality and fatty acid composition of breast and thigh muscles in broiler chickens. Anim Feed Sci Technol. (2018) 235:129–40.
9. Fard, SG, Samadi, F, Mottaghitalab, M, and Pourreza, J. Effects of different levels of iron nanoparticles on performance, biochemical parameters, immune response, and serum biochemical parameters in broiler chickens. Vet Med Int. (2017) 12:1–6.
10. Ahmadi, F, Sari, M, and Rahimi, S. Effect of different levels of nano iron supplementation on performance, carcass characteristics, and iron content of tissues in broiler chickens. Vet Res Forum. (2019) 10:231–6.
11. Suryanarayana, VVS, and Debnath, C. Dietary supplementation of nano-iron pyrophosphate improves growth performance, nutrient digestibility, hematological and antioxidative status in broiler chickens. Biol Trace Elem Res. (2019) 189:487–94.
12. Akbari, M, Kakhki, R, and Khajali, F. The effect of iron nanoparticles and vitamin E on performance, immune responses, serum metabolites, and antioxidative status in broiler chickens. Anim Feed Sci Technol. (2017) 224:33–40.
13. Khan, F, Shariq, M, Asif, M, Siddiqui, MA, Malan, P, and Ahmad, F. Green nanotechnology: plant-mediated nanoparticle synthesis and application. Nanomaterials. (2022) 12:673. doi: 10.3390/nano12040673
14. Saracila, M, Olteanu, M, and Panaite, TD. Implications of using some phytoadditives in broiler nutrition—a review. Sci Pap Ser D. (2020) 63:165–72.
15. Vlaicu, PA, Untea, AE, Turcu, RP, Saracila, M, Panaite, TD, and Cornescu, GM. Nutritional composition and bioactive compounds of basil, thyme and sage plant additives and their functionality on broiler thigh meat quality. Foods. (2022) 11:1105. doi: 10.3390/foods11081105
16. Almeldin, YAR, Eldlebshany, AE, Elkhalek, EA, AAA, A-W, and Lohakare, J. The effect of combining green iron nanoparticles and algae on the sustainability of broiler production under heat stress conditions. Front Vet Sci. (2024) 11:1359213. doi: 10.3389/fvets.2024.1359213
18. Sun, J, Liu, D, and Rubin, S. Supplemental dietary iron glycine modifies growth, immune function, and antioxidant enzyme activities in broiler chickens. Livest Sci. (2015) 176:129–34. doi: 10.1016/j.livsci.2015.03.004
19. Srinivasan, V, Bhavan, PS, Rajkumar, G, Satgurunathan, T, and Muralisankar, T. Effects of dietary iron oxide nanoparticles on the growth performance, biochemical constituents and physiological stress responses of the giant freshwater prawn Macrobrachium rosenbergii post-larvae. In J Fish Aquat Stud. (2016) 4:170–82.
20. Habeeb, AA, Gad, AE, and Atta, MA. Temperature-humidity indices as indicators to heat stress of climatic conditions with relation to production and reproduction of farm animals. Int J Biotechnol Recent Adv. (2018) 1:35–50. doi: 10.18689/ijbr-1000107
21. Association Official Analytical Chemistry (AOAC) . Official methods of analysis. 19th ed. Washington, DC: Association Official Analytical Chemistry (2007).
22. Khalil, AT, Ovais, M, Ullah, I, Ali, M, Shinwari, ZK, Khamlich, S, et al. Sageretia thea (Osbeck.) mediated synthesis of zinc oxide nanoparticles and its biological applications. Nanomedicine. (2017) 12:1767–89. doi: 10.2217/nnm-2017-0124
23. Miles, DM, Owens, PR, Moore, JPA, and Rowe, DE. Instrumentation for evaluating differences in ammonia volatilization from broiler litter and cake. J Appl Poult Res. (2008) 17:340–7. doi: 10.3382/japr.2007-00112
24. Honikel, K, and Hamm, R. Measurement of water-holding capacity and juiciness In: Quality attributes and their measurement in meat, poultry and fish products. Berlin: Springer (1994). 125–61.
25. Honikel, KO . Reference methods for the assessment of physical characteristics of meat. Meat Sci. (1998) 49:447–57. doi: 10.1016/S0309-1740(98)00034-5
27. Yasar, S, Forbes, JM, and Erdem, E. Comparison of dietary iron sources on growth performance, hemoglobin, iron concentration and ferritin of broilers. Ital J Anim Sci. (2019) 18:612–9.
28. Wang, WC, Yu, DY, and Wang, F. Effects of different iron sources and levels on growth performance, blood parameters, and antioxidant enzyme activity in broilers. Biol Trace Elem Res. (2020) 197:474–81.
29. Hafez, HM, Attia, YA, and El-Tahawy, WS. The influence of different dietary levels of iron and zinc on the productive performance and biochemical parameters of broiler chicks. Ital J Anim Sci. (2015) 14:3906.
30. Pesti, GM, Bakalli, RI, and Tillman, PB. Feeding nonphytate phosphorus and phytase to broilers. Poult Sci. (2015) 94:645–53.
31. Taschetto, D, Vieira, SL, Angel, CR, Stefanello, C, Kindlein, L, Ebbing, MA, et al. Iron requirements of broiler breeder hens. Poult Sci. (2017) 96:3920–7. doi: 10.3382/ps/pex208
32. Bao, YM, and Choct, M. Trace mineral nutrition for broiler chickens and prospects of application of organically complexed trace minerals: a review. Anim Prod Sci. (2009) 49:269–82. doi: 10.1071/EA08204
33. Rehman, H, Akram, M, Kiyani, MM, Yaseen, T, Ghani, A, Saggu, JI, et al. Effect of endoxylanase and iron oxide nanoparticles on performance and histopathological features in broilers. Biol Trace Elem Res. (2020) 193:524–35. doi: 10.1007/s12011-019-01737-z
34. Nikonov, IN, Folmanis, YG, Folmanis, GE, Kovalenko, LV, Laptev, GY, Egorov, IA, et al. Iron nanoparticles as a food additive for poultry. Dokl Biol Sci. (2011) 440:328–31. doi: 10.1134/S0012496611050188
35. Sarlak, S, Tabeidian, SA, Toghyani, M, Shahraki, ADF, Goli, M, and Habibian, M. Effects of replacing inorganic with organic iron on performance, egg quality, serum and egg yolk lipids, antioxidant status, and iron accumulation in eggs of laying hens. Biol Trace Elem Res. (2021) 199:1986–99. doi: 10.1007/s12011-020-02284-8
36. Donnik, IM . Research of opportunities for using iron nanoparticles and amino acids in poultry nutrition. Int J GEOMATE. (2017) 13:124–31. doi: 10.21660/2017.40.99216
37. Tang, C, Kong, W, Wang, H, Liu, H, Shi, L, Uyanga, AV, et al. Effects of fulvic acids on gut barrier, microbial composition, fecal ammonia emission, and growth performance in broiler chickens. J Appl Poult Res. (2023) 32:100322. doi: 10.1016/j.japr.2022.100322
38. Bauer, SE, Tsigaridis, K, and Miller, R. Significant atmospheric aerosol pollution caused by world food cultivation. Geophys Res Lett. (2016) 43:5394–400. doi: 10.1002/2016GL068354
39. Zhou, Y, Zhang, M, Liu, Q, and Feng, J. The alterations of tracheal microbiota and inflammation caused by different levels of ammonia exposure in broiler chickens. Poult Sci. (2021) 100:685–96. doi: 10.1016/j.psj.2020.11.026
40. Eklou-Lawson, M, Bernard, F, Neveux, N, Chaumontet, C, Bos, C, Davila-Gay, M, et al. Colonic luminal ammonia and portal blood l-glutamine and l-arginine concentrations: a possible link between colon mucosa and liver ureagenesis. Amino Acids. (2009) 37:751–60. doi: 10.1007/s00726-008-0218-3
41. Ichikawa, H, and Sakata, T. Stimulation of epithelial cell proliferation of isolated distal colon of rats by continuous colonic infusion of ammonia or short-chain fatty acids is nonadditive. J Nutr. (1998) 128:843–7. doi: 10.1093/jn/128.5.843
42. Shayeghi, M, Latunde-Dada, GO, Oakhill, JS, Laftah, AH, Takeuchi, K, Halliday, N, et al. Identification of an intestinal heme transporter. Cell. (2005) 122:789–801. doi: 10.1016/j.cell.2005.06.025
43. Mackenzie, B, and Garrick, MD. Iron imports. II. Iron uptake at the apical membrane in the intestine. Am J Physiol Gastrointest Liver Physiol. (2005) 289:G981–6. doi: 10.1152/ajpgi.00363.2005
44. Ma, X, Liao, X, Lu, L, Li, S, Zhang, L, and Luo, X. Determination of dietary iron requirements by full expression of iron-containing enzymes in various tissues of broilers. J Nutr. (2016) 146:2267–73. doi: 10.3945/jn.116.237750
45. Wang, Z, Zhao, D, Qin, S, Shi, Z, Li, X, Wang, Y, et al. Effects of dietary supplementation with Iron in breeding pigeons on the blood iron status, tissue iron content and full expression of iron-containing enzymes of squabs. Biol Trace Elem Res. (2023) 201:4538–46. doi: 10.1007/s12011-022-03530-x
46. Seo, SH, Lee, HK, Lee, WS, Shin, KS, and Paik, IK. The effect of level and period of Fe-methionine chelate supplementation on the iron content of boiler meat. Asian Austral J Anim. (2008) 21:1501–5. doi: 10.5713/ajas.2008.80085
47. Ma, WQ, Sun, H, Zhou, Y, Wu, J, and Feng, J. Effects of iron glycine chelate on growth, tissue mineral concentrations, fecal mineral excretion, and liver antioxidant enzyme activities in broilers. Biol Trace Elem Res. (2012) 149:204–11. doi: 10.1007/s12011-012-9418-5
48. Zhu, X, Li, M, Pan, R, Tian, C, Zhu, H, and Geng, Z. Effects of different nanoscale iron oxide particles on the growth performance, oxidative stress, and immune response of broilers. Biol Trace Elem Res. (2018) 186:433–41.
49. Rezaei, M, Jazi, V, Akbari, M, and Kheiri, F. The effects of nano-sized zinc oxide on the performance, immune response, and antioxidant status of broiler chickens. Poult Sci. (2017) 96:779–85.
50. Kim, JH, Kim, SH, Lee, DS, and Lee, DS. Iron oxide nanoparticles suppress the production of IL-1β via the secretory lysosomal pathway in murine microglial cells. Int J Nanomedicine. (2016) 11:2543–53.
51. Lin, X, Gou, Z, Wang, Y, Li, L, Fan, Q, Ding, F, et al. Effects of dietary iron level on growth performance, immune organ indices and meat quality in Chinese yellow broilers. Animals. (2020) 10:670. doi: 10.3390/ani10040670
52. Abdel-Rahman, HG, Alian, HA, and Mahmoud, MMA. Impacts of dietary supplementation with nano-iron and methionine on growth, blood chemistry, liver biomarkers, and tissue histology of heat-stressed broiler chickens. Trop Anim Health Prod. (2022) 54:126. doi: 10.1007/s11250-022-03130-w
53. Saleh, AA, Eltantawy, MS, Gawish, EM, Younis, HH, Amber, KA, Abd El-Moneim, EAE, et al. Impact of dietary organic mineral supplementation on reproductive performance, egg quality characteristics, lipid oxidation, ovarian follicular development, and immune response in laying hens under high ambient temperature. Biol Trace Elem Res. (2020) 195:506–14. doi: 10.1007/s12011-019-01861-w
54. Kurtoglu, E, Ugur, A, Baltaci, AK, and Undar, L. Effect of iron supplementation on oxidative stress and antioxidant status in iron-deficiency anemia. Biol Trace Elem Res. (2003) 96:117–24. doi: 10.1385/BTER:96:1-3:117
55. Strube, YNJ, Beard, JL, and Ross, AC. Iron deficiency and marginal vitamin a deficiency affect growth, hematological indices and the regulation of iron metabolism genes in rats. J Nutr. (2002) 132:3607–15. doi: 10.1093/jn/132.12.3607
56. Rincker, MJ, Hill, GM, Link, JE, and Rowntree, JE. Effects of dietary iron supplementation on growth performance, hematological status, and whole-body mineral concentrations of nursery pigs. J Anim Sci. (2004) 82:3189–97. doi: 10.2527/2004.82113189x
57. Anderson, GJ, and Vulpe, CD. Mammalian iron transport. Cell Mol Life Sci. (2009) 66:3241–61. doi: 10.1007/s00018-009-0051-1
Keywords: ammonia, broilers, health, iron, nanotechnology, meat quality
Citation: Almeldin YAR, Eldlebshany AE, Elkhalek EA, Lohakare J and Abdel-Wareth AAA (2024) Assessment of dietary supplementation of green iron oxide nanoparticles: impact on growth performance, ammonia emissions, carcass criteria, tissue iron content, and meat quality in broiler chickens under hot climate conditions. Front. Vet. Sci. 11:1393335. doi: 10.3389/fvets.2024.1393335
Received: 28 February 2024; Accepted: 19 June 2024;
Published: 02 July 2024.
Edited by:
Samiru Sudharaka Wickramasuriya, Agricultural Research Service (USDA), United StatesReviewed by:
Shemil Macelline, The University of Sydney, AustraliaCopyright © 2024 Almeldin, Eldlebshany, Elkhalek, Lohakare and Abdel-Wareth. This is an open-access article distributed under the terms of the Creative Commons Attribution License (CC BY). The use, distribution or reproduction in other forums is permitted, provided the original author(s) and the copyright owner(s) are credited and that the original publication in this journal is cited, in accordance with accepted academic practice. No use, distribution or reproduction is permitted which does not comply with these terms.
*Correspondence: Jayant Lohakare, amF5YW50LmxvaGFrYXJlQGdtYWlsLmNvbQ==; Ahmed A. A. Abdel-Wareth, YWFoYWJkZWx3YXJldGhAcHZhbXUuZWR1
Disclaimer: All claims expressed in this article are solely those of the authors and do not necessarily represent those of their affiliated organizations, or those of the publisher, the editors and the reviewers. Any product that may be evaluated in this article or claim that may be made by its manufacturer is not guaranteed or endorsed by the publisher.
Research integrity at Frontiers
Learn more about the work of our research integrity team to safeguard the quality of each article we publish.