- 1Department of Aquaculture, Faculty of Fisheries, Kasetsart University, Bangkok, Thailand
- 2Department of Animal Science, Faculty of Agriculture at Kamphaeng Saen, Kasetsart University, Nakhon Pathom, Thailand
- 3Department of Pathology, Faculty of Veterinary Science, Kasetsart University, Bangkok, Thailand
- 4INVE Aquaculture, Nonthaburi, Thailand
- 5Aquaculture Pathology Laboratory, School of Animal and Comparative Biomedical Sciences, The University of Arizona, Tucson, AZ, United States
- 6Aquaculture and Aquatic Resources Management, Department of Food Agriculture and Bioresources, School of Environment, Resources and Development, Asian Institute of Technology, Pathum Thani, Thailand
Siamese fishing fish (Betta splendens) or betta are usually subjected to a special method of transportation for global trade, where they are individually conveyed in plastic bags containing just enough water to cover their bodies. This study aimed to investigate the effects of transportation on their stress response by measuring hematological values, stress hormone levels, glucose levels, and stress-related gene expression. Betta fish (average body weight 1.91 ± 0.42 g; n = 30) were exposed to simulated transport in a water volume of 40 mL for 12, 24, and 48 h. Baseline levels (pre-transport) were measured prior to the experiment. The control group was transported using water without adding clove oil. Two treatment groups were transported using water with the addition of 1 and 3 mg/L concentrations of clove oil, respectively. The results revealed that transportation can be a factor that affects water quality. The pH and dissolved oxygen levels were significantly lower than baseline, while nitrite and total ammonia concentrations significantly increased. Correlating to the stress responses, significantly increasing total red blood cell counts, plasma cortisol levels, and up-regulating the expression of stress-related genes, including HSP70, GR, MR, and HIF-1α. The addition of 1 mg/L clove oil was found to reduce stress during the transport simulation, as evidenced by a reduction in these stress parameters. Conversely, increasing the concentration of clove oil to 3 mg/L significantly increased plasma cortisol after 12 h of simulated transport, and up-regulated GR, MR, and HIF-1α expression. This study revealed that the transport process can stimulates stress in betta fish but adding a concentration of 1 mg/L clove oil to the transport water could mitigate this stress response and promote animal welfare during their transportation.
1 Introduction
Transportation is recognized as a significant source of stress resulting in measurable responses (1, 2). This stress is attributed to various risks arising from inadequate inappropriate stocking densities (3) vibration, potential manhandling, temperature changes (4), and deterioration in water quality (5). Such conditions can render fish susceptible to injuries (6) or can result in mortality during transit. Siamese fighting fish (Betta splendens), commonly known as betta, holds economic significance in the global ornamental fish trade. Male bettas are characterized by their aggressive behavior (7, 8) and are therefore typically transported individually in small plastic bags containing approximately 40 mL of water, maintaining a water-to-air ratio of 1:3 (7). This unique species-specific transport procedure has raised concerns from the People for the Ethical Treatment of Animals (PETA) regarding potential stress and welfare for betta fish (9). Prior studies have explored transportation stress responses in various species such as the dog snapper, Lutjanus jocu [(see 10)], channel catfish, Ictalurus punctatus [(see 11)], meagre, Argyrosomus regius [(see 12)], and in Nile tilapia, Oreochromis niloticus [(see 13)]. These stresses experienced by fish are linked to physiological responses, particularly endocrine alterations that induce changes in metabolic aspects, such as glucose levels, their hematological profile, and cellular changes. These changes encompass, the expression of stress-related genes in fish, varying depending on the species (14–18). In betta fish, an increase in the expression of trypsin, which is potentially associated with stress-related physiological effects, has been observed during transportation in the lowest water volume (40 mL) (19). However, there is limited literature specifically addressing the transportation of betta fish, particularly in terms of direct stress assessment.
Minimizing stress during live-fish transport poses a considerable challenge (20). In recent years, considerable additive extracts have been studied and integrated into welfare improvement (21–23). Especially, the incorporation of clove oil into the water during transportation has been explored in various fish species, such as common carp, Cyprinus carpio [(see 24)], meagre (25), betta fish (26), clown fish, Amphiprion ocellaris [(see 27)], and the European catfish, Silurus glanis [(see 28)]. Clove oil, being a natural anesthetic, is readily available, cost-effective, safe, and effective in stress alleviation (29–32). Its active ingredients are eugenol and iso-eugenol, which not only reduces fish mortality but also enables rapid recovery (31). Dosing is crucial and varies based on species, fish size (33) exposure time, and environmental conditions (31). The concentration of clove oil used in the betta fish trials adhered to the recommendations provided by Sintuprom et al. (34), suggesting that the addition of clove oil could potentially aid in controlling water quality within an appropriate range during transportation. However, concentrations of 5 and 10 mg/L resulted in higher stress levels, possibly indicating that these concentrations were too high. Consequently, this study utilized this information to determine the optimal concentration of clove oil.
This study investigated stress indicators in Siamese fighting fish during simulated transport, including cortisol levels, glucose levels, hematological parameters, and the expression of stress-related genes. Additionally, the study assessed the efficacy of clove oil at concentrations of 1 and 3 mg/L to identify the most practical solution for farmers in mitigating stress and enhancing the welfare of bettas during transportation.
2 Materials and methods
2.1 Experimental design and transport procedures
Adult mixed-sex betta fish (B. splendens) aged 5 months, with an average body weight of 1.91 ± 0.42 g and an average standard length of 4.08 ± 0.39 cm (n = 30), were sourced from a local farm in Nakhon Pathom province, Thailand. The fish were individually housed in 250 mL glass bottles containing 140 mL of water. A total of 825 apparently healthy bettas were subjected to a 24-h fasting period and re-examined before the study began to ensure that they showed no external injuries or clinical signs.
Clove oil, sourced from Sigma-Aldrich, Singapore, with an eugenol content of 85% w/w was used. The concentration of clove oil used for the trials followed the recommendation provided by Sintuprom et al. (34). The preparation involved mixing clove oil with 95% ethanol (C₂H₅OH) in a 1:9 ratio (26, 31), to produce final concentrations of 1 and 3 mg/L of clove oil. All stock solutions were prepared immediately before the experiment.
The experiment was conducted with fish divided into four different groups. Before the experiment began, after a 24-h fasting period and just before the transport procedure, 75 fish were selected for the baseline-level group (pre-transport). These fish were organized into three replicates of 25 fish each. The remaining 750 fish were randomly assigned to one of three experimental groups: a control group without adding clove oil, and two treatment groups to which either 1 mg/L or 3 mg/L of clove oil was added. Each of the three experimental groups were further sub-divided and ran as three replicates. An overview of the experimental workflow in this study are illustrated in Figure 1.
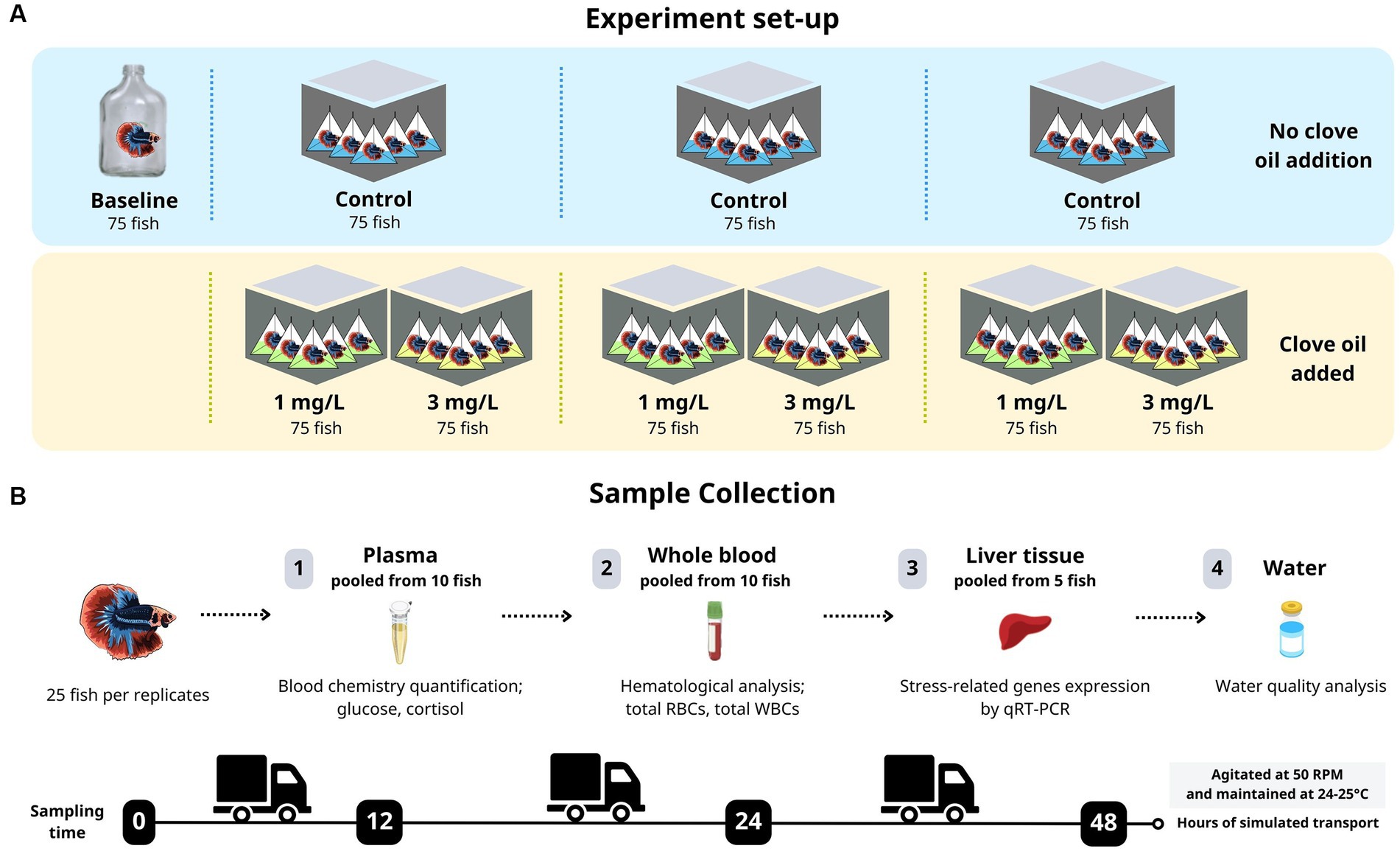
Figure 1. An overview of the experimental workflow implemented in this study. (A) Experiment set-up, (B) Sample collection.
Individual fish were placed in 3 × 5.5 inch plastic bags, containing 40 mL (i.e., one-third full) dechlorinated water with or without the respective amount of clove oil, following the methodology of Thongprajukaew et al. (19). The bags were then filled with pure oxygen and then sealed with an FRD 1,000 LW/S continuous sealer (Brother, China). These procedures align with established international transportation protocols (Dokkaew, personal communication, 2022) followed by betta farmers. The simulated transport procedures adhered to the methodology of Wu et al. (35). The pre-packaged fish were then placed in 11.8 × 16.9 × 11.4 inch styrofoam boxes with lids to protect them from light. Subsequently, they were subjected to an orbital shaker at 50 RPM for 48 h, and maintained at an internal temperature of 24°C–25°C. Samples of fish were then randomly collected from each of the three post-transport groups after 12, 24, and 48 h of simulated transport. The survival rate of the fish in each group was determined at each time point.
Prior to sample collection, the fish were euthanized promptly using 0.1% clove oil for 1–2 min that minimizes the stress response, following the procedure described by Davis et al. (36). The experimental procedures and the utilization of animals received approval from the Institutional Animal Care and Use Committee of Kasetsart University (approval ID: ACKU63-FIS-008).
2.2 Water quality measurement
Water quality was examined at each sampling point throughout the experiment. pH values were determined using an Ecoscan pH5/6 device (Eutech, United States). Temperature and dissolved oxygen (DO) levels were directly measured using an EcoSense DO 200-4 M instrument (YSI, Germany). Alkalinity was determined through a titration method (37), while nitrite (NO2−) concentration was assessed using a colorimetric method (37). Additionally, the total ammonia nitrogen (TAN) concentration was determined using Koroleff’s indophenol blue method (37).
2.3 Blood parameters
Blood samples from the fish processed at 0, 12, 24, and 48 h were assessed using two different methods for hematological analysis (i.e., total red/white blood cell counts) and blood chemistry quantification (i.e., concentration of plasma cortisol and plasma glucose levels). The procedures are outlined below.
For the hematological analysis, whole blood was collected using a heparinized capillary tube following tail ablation. Each sample of whole blood consisted of a pool of whole blood collected 10 fish. Three replicated were prepared. The whole blood pool sample was then divided and mixed with Natt and Herrick’s solution (at a ratio of 1:200) to allow for the subsequent determination of representative total RBC and WBC counts. Blood counts were determined from replicate counts made using a hemocytometer under an Olympus CX22 compound microscope (magnification 40X).
For blood chemistry analysis, the plasma collection method was adapted from Babaei et al. (38) with a modification involving the use of a perforated 0.5 mL tube inserted into a 1.5 mL standard microcentrifuge tube. Plasma was collected from a pool of 10 fish per replicate; the three replicates were then centrifuged at 400 × g for 5 min at 12°C. The samples were subjected to further centrifugation at 4,000 × g for 15 min at 12°C to separate the blood cells. The plasma was then collected and stored at −20°C until the cortisol and glucose content of each sample was determined. A volume of 40 μL of plasma from each replicate was used for the quantification of glucose using a GLU VT assay kit (IDEXX Laboratories, United States) with the VetTest Chemistry Analyzer (IDEXX Laboratories, United States). An additional aliquot of 40 μL from each replicate was taken to determine plasma cortisol levels using the General Cortisol ELISA kit (MyBioSource, United States), with measurements conducted within the range of 0.5–300 ng/mL. The optical density (OD value) of the samples thereafter was then determined at 490 nm using a BK-EL10C ELISA microplate reader (Biobase, China).
2.4 Stress-related genes expression by qRT-PCR
For this component of the study, livers pooled from five fish per replicate were used for analysis. These samples were promptly flash-frozen in liquid nitrogen and stored at −80°C until total RNA extraction. Total RNA extractions were conducted following the manufacturer’s protocols, utilizing TRIzol reagent (Thermo Fisher Scientific, United States). RNA samples were then processed using the GeneJET RNA Cleanup and Concentration Micro Kit (Thermo Fisher Scientific, United States), following the Total RNA Clean-up protocol. Qualitative and quantitative assessments of RNA were performed using a NanoDrop Eight Spectrophotometer (Thermo Fisher Scientific, United States).
To synthesize complementary DNA (cDNA), 1 μg of total RNA per sample was used with the RevertAid First Strand cDNA Synthesis kit (Thermo Fisher Scientific, United States). Primer sequences used for the qRT-PCR analyses are listed in Table 1. The study investigated transcript levels of heat shock protein (HSP70), heat shock protein (HSP90), glucocorticoid receptor (GR), mineralocorticoid receptor (MR), and hypoxia-inducible factor 1-alpha (HIF-1α), by employing β-actin as a housekeeping gene.
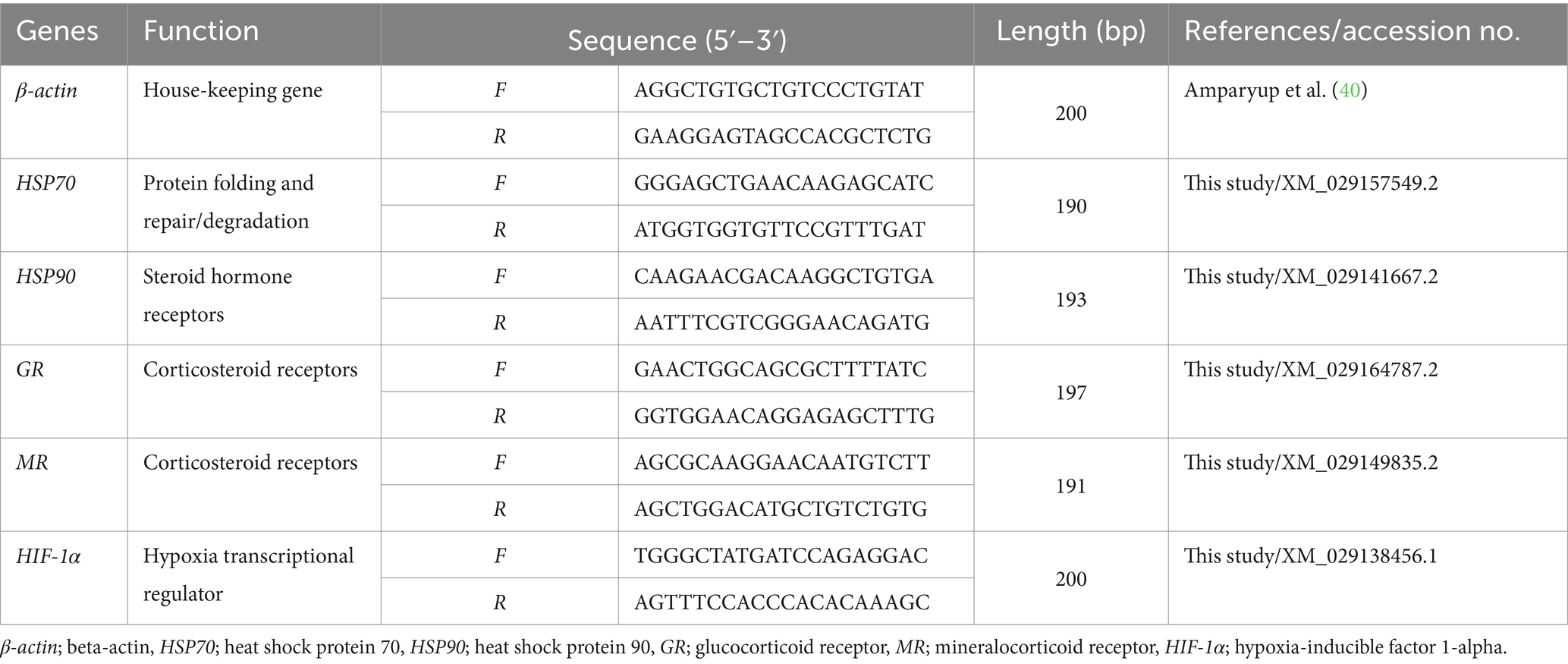
Table 1. Primer sequences used for the gene expression analysis in Siamese fighting fish (Betta splendens).
qRT-PCR reactions were prepared in 25 μL volumes, comprising 12.5 μL of 2X SYBR Green QPCR master mix and 10 μM of each primer. The amplification conditions were as follows: an initial pre-denaturation at 94°C for 2 min, followed by 40 cycles of denaturation at 98°C for 10 s, annealing at 55°C for 30 s, extension at 68°C for 10 s, and a final extension at 68°C for 10 s. These reactions were carried out utilizing the Mastercycler ep realplex Real-time PCR System (Eppendorf, Germany). Relative gene expression analysis was performed using the 2−ΔΔ CT method, as described by Livak and Schmittgen (39).
2.5 Statistical analysis
All data analyses were performed using IBM SPSS Statistics 26. One-way analysis of variance (ANOVA) tests were used to examine differences between the three groups at each time interval and differences within each group across various time intervals, followed by Duncan’s post-hoc test for pairwise comparisons. Statistical significance was set at a threshold of p < 0.05.
3 Results
3.1 Survival rate and water quality parameters
The survival rate (%) of the fish following exposure to the simulated transport conditions was determined at the end of the trial and calculated from a total of 250 fish per group. The addition of 1 mg/L clove oil resulted in the highest survival rate of 98.4%, followed by 97.6% in the control group, and 96.8% in the group receiving 3 mg/L clove oil.
The mean values of water temperature, alkalinity, pH, DO, nitrite, and TAN are presented in Figure 2. In the post-transport groups, the average temperature was 24.84 ± 0.59°C (Figure 2A). Alkalinity showed significant differences compared to the baseline level (p < 0.05) observed in the control group and in the group of fish receiving 3 mg/L clove oil after 24 h of simulated transport (Figure 2B). The pH values (Figure 2C) and in DO levels (Figure 2D) decreased significantly in post-transport groups when compared to the baseline level (p < 0.05); these parameters gradually decreased with increasing transportation time. Nitrite concentrations (Figure 2E) showed significant differences (p < 0.05) after 48 h of simulated transport. TAN concentrations (Figure 2F) showed a significant increase in post-transport groups (p < 0.05), with the highest recorded value at 48 h of simulated transport.
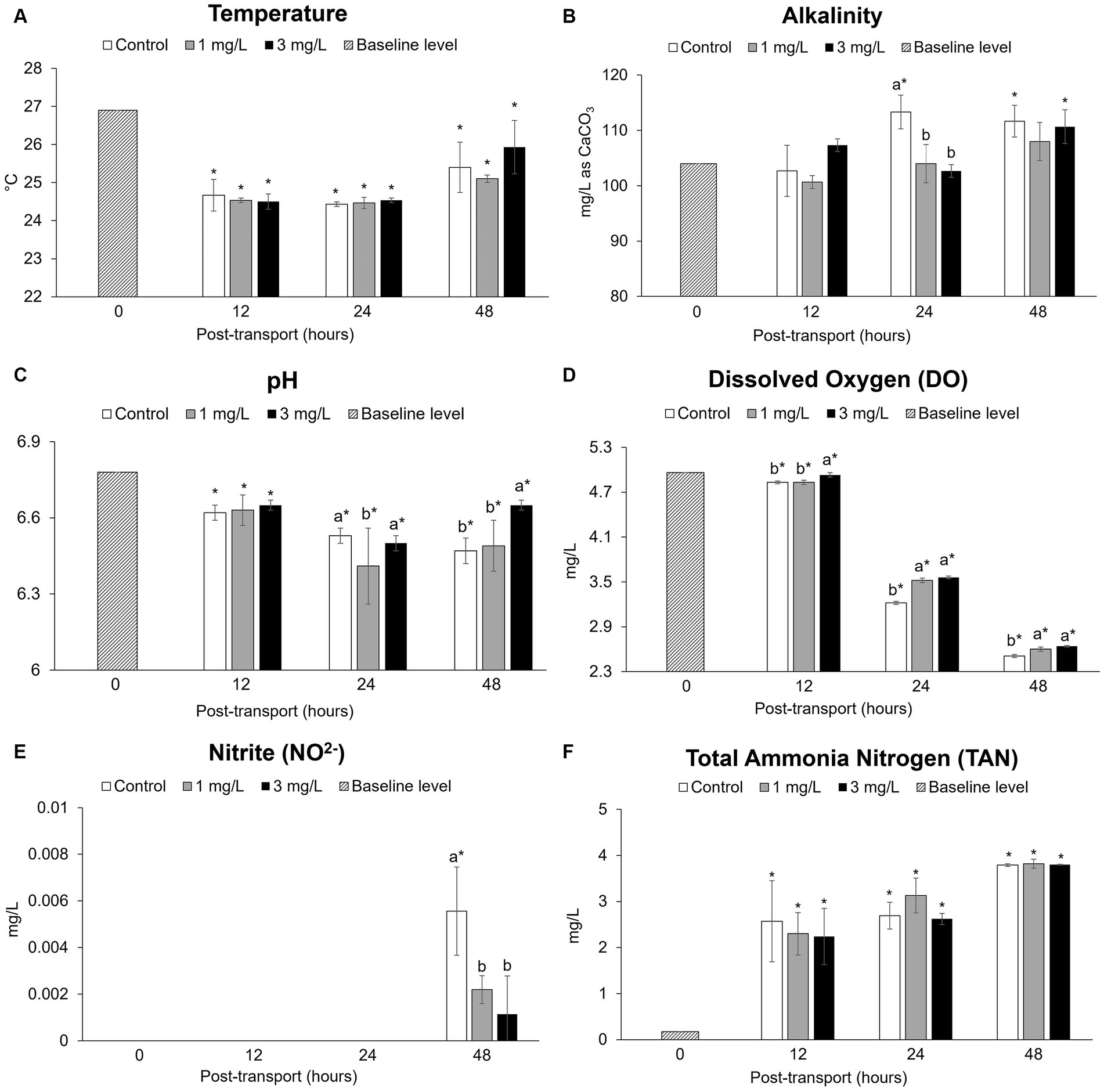
Figure 2. Water quality parameters during the Betta splendens (n = 3) transportation simulation. (A) Temperature, (B) Alkalinity, (C) pH, (D) Dissolved oxygen, (E) Nitrite, (F) Total ammonia nitrogen value. Vertical bars indicate standard deviation; mean with different lowercase letters indicate significant differences (p < 0.05) among treatments at each time interval, according to one way-ANOVA. Asterisks indicate significant differences (p < 0.05) between the groups and the baseline level, according to the ANOVA.
3.2 Hematological values
The values for the control group had increased significantly (p < 0.05), reaching the highest total RBC count at 48 h (p < 0.05) after the start of the simulated transport. In a contrast to this, the treatment groups receiving 1 mg/L clove oil had significantly lower total RBC counts (p < 0.05), with no significant differences when compared to the baseline level (p > 0.05) throughout the experiment (Figure 3A).
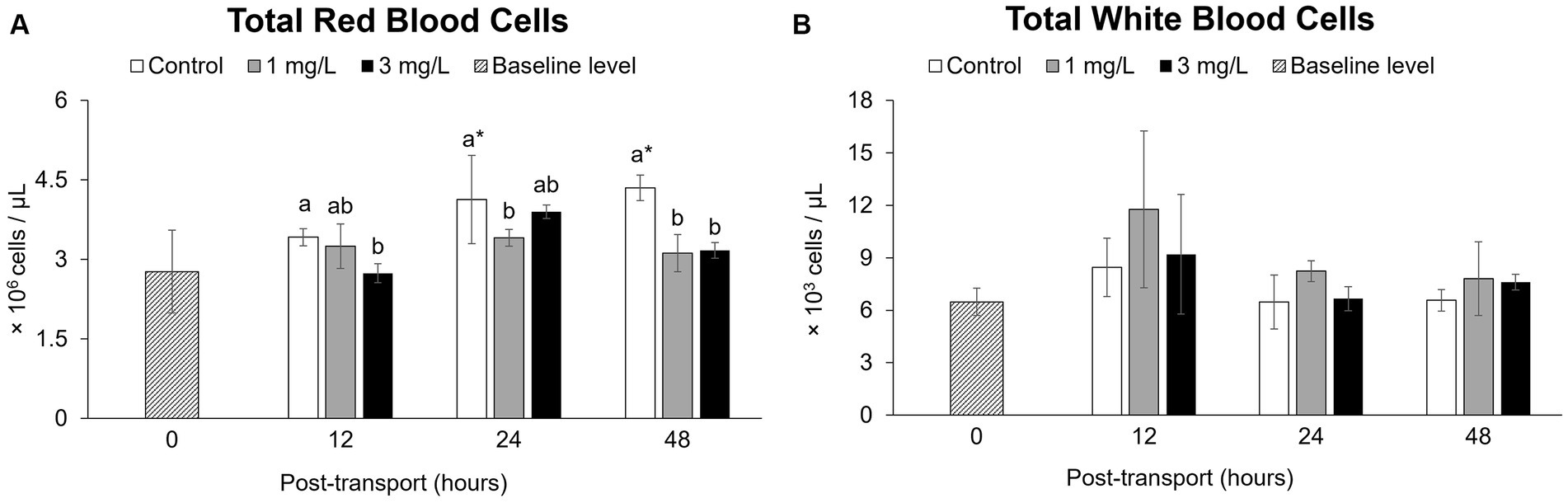
Figure 3. Hematological parameters during the Betta splendens (n = 3) transportation simulation. (A) Total red blood cells, (B) Total white blood cells. Vertical bars indicate standard deviation; mean with different lowercase letters indicate significant differences (p < 0.05) among treatments at each time interval, according to one-way ANOVA. Asterisks indicate significant differences (p < 0.05) between the groups and the baseline level, according to the ANOVA.
No significant differences were noted when comparing these total WBC counts with those obtained from the control and two treatment groups at each time interval (p > 0.05) by the end of the trial (p > 0.05; Figure 3B).
3.3 Stress hormone levels
The concentrations of plasma cortisol (Figure 4) in the control and in the treatment group receiving 3 mg/L clove oil after 12 h of simulated transport were significantly higher (p < 0.05) than the baseline level. In contrast, the treatment group receiving 1 mg/L clove oil exhibited a significantly lower (p < 0.05) level of plasma cortisol compared to the other two groups, with no statistically significant differences compared to the baseline level (p > 0.05). After 24 h, the cortisol levels in the control group exhibited a significantly elevated plasma cortisol concentration when compared to the baseline group (p < 0.05). There were no significant differences (p > 0.05) between the control and the two treatment groups at any other time point, and they remained comparable to the baseline (p > 0.05) at 48 h.
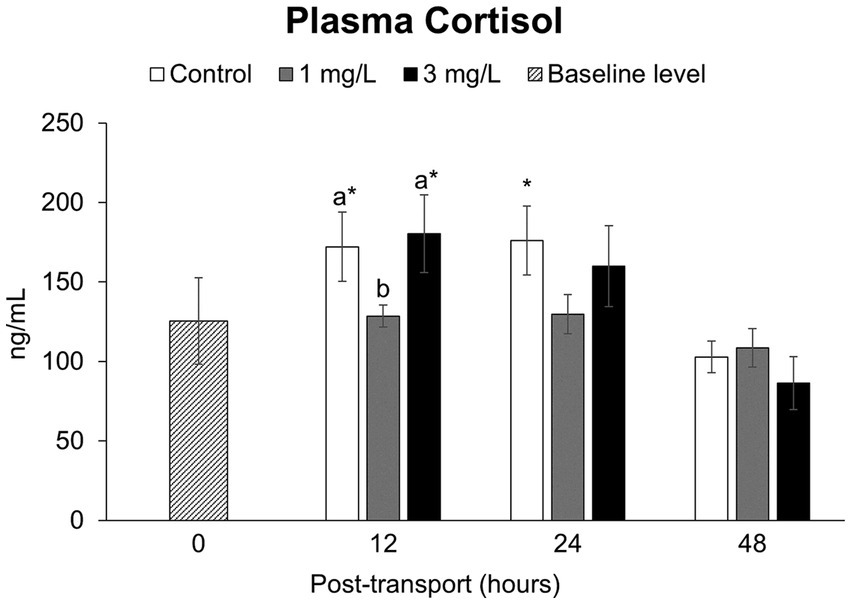
Figure 4. Stress hormone levels (plasma cortisol levels) during the Betta splendens (n = 3) transportation simulation. Vertical bars indicate standard deviation; mean with different lowercase letters indicate significant differences (p < 0.05) among treatments at each time interval, according to one-way ANOVA. Asterisks indicate significant differences (p < 0.05) between the groups and the baseline level, according to the ANOVA.
3.4 Plasma glucose levels
There were no statistically significant differences in the concentration of plasma glucose (Figure 5) between the control and two treatment groups, as well as between three groups and the baseline level, over the 48 h under simulated transport (p > 0.05).
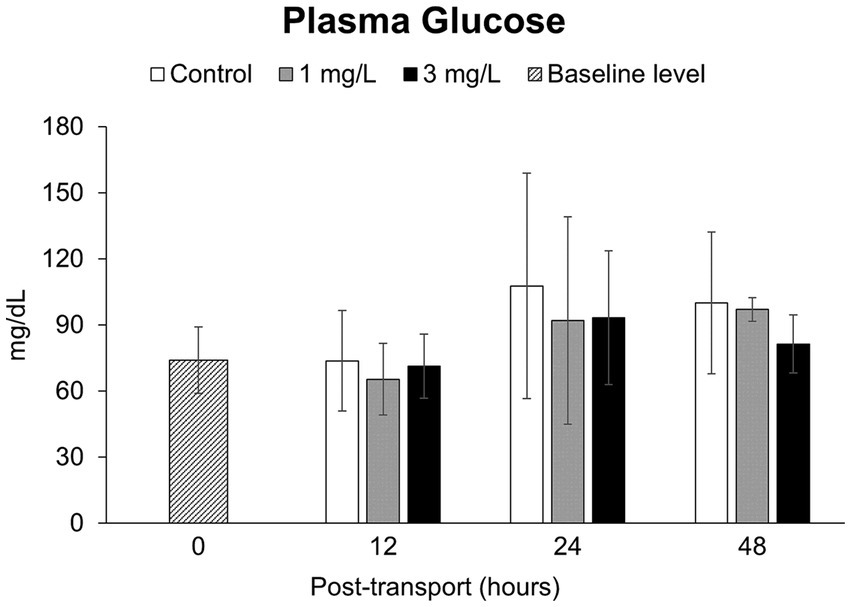
Figure 5. Plasma glucose levels during the Betta splendens (n = 3) transportation simulation. Vertical bars indicate standard deviation; mean with different lowercase letters indicate significant differences (p < 0.05) among treatments at each time interval, according to one-way ANOVA. Asterisks indicate significant differences (p < 0.05) between the groups and the baseline level, according to the ANOVA.
3.5 Stress-related gene expression
There was a significant difference (p < 0.05) in the relative expression of HSP70 (Figure 6A) between the control and the two treatment groups following the transport simulation. After 12 h, levels in the control group had significantly increased (p < 0.05) when compared to the baseline level, this remained significantly higher than the other tests groups. Conversely, the two treatment groups were significantly lower (p < 0.05) than the control throughout the experiment. The addition of 1 mg/L clove oil resulted in a significant down-regulation (p < 0.05) in HSP70 expression and significant differences when compared to the baseline level at 24 and 48 h.
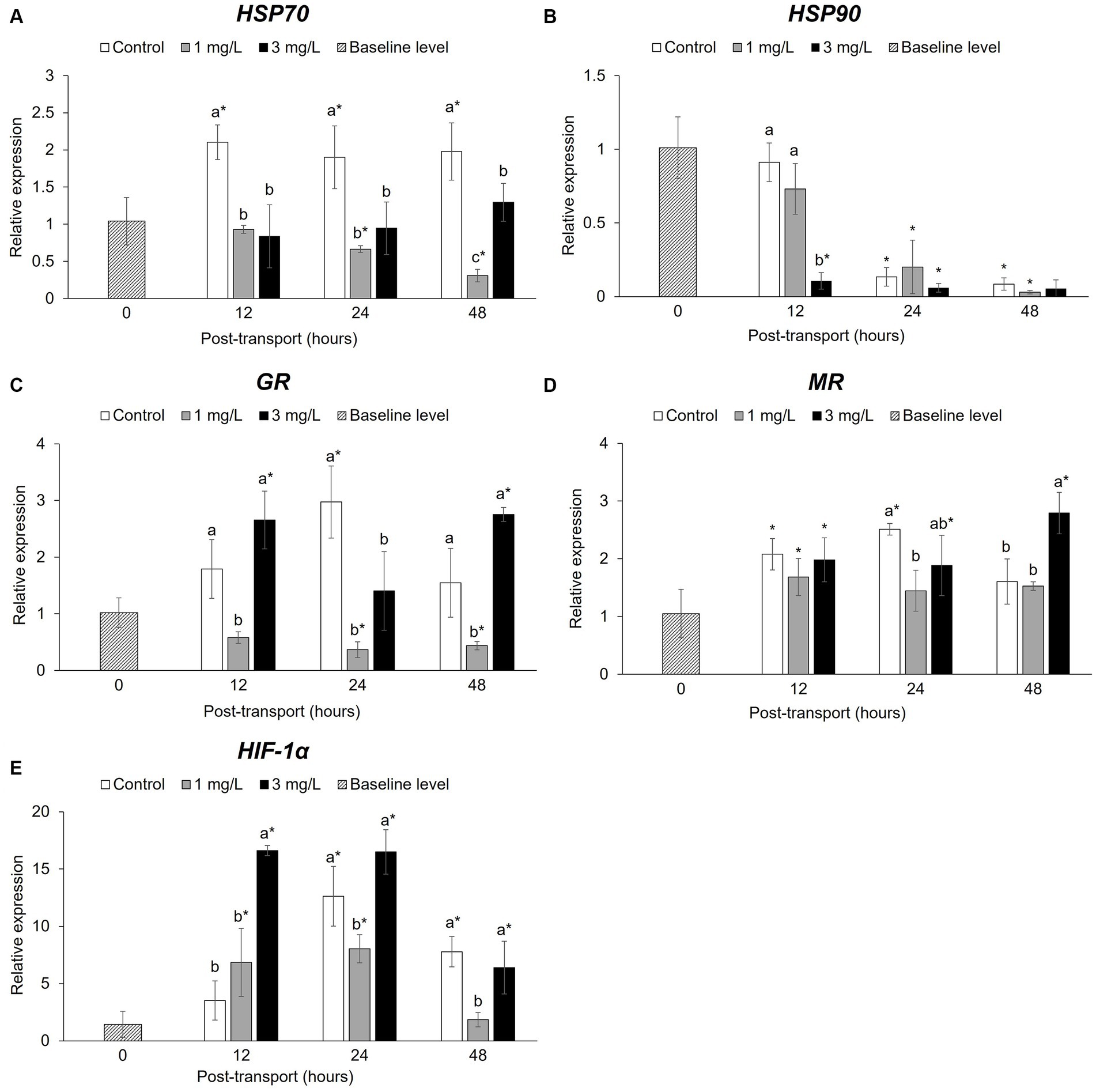
Figure 6. Stress-related gene expression during the Betta splendens (n = 3) transportation simulation. (A) HSP70, (B) HSP90, (C) GR, (D) MR, (E) HIF-1α. Vertical bars indicate standard deviation; mean with different lowercase letters indicate significant differences (p < 0.05) among treatments at each time interval, according to one-way ANOVA. Asterisks indicate significant differences (p < 0.05) between the groups and the baseline level, according to the ANOVA.
The study also found significant differences in the levels of HSP90 (Figure 6B) between the control and the two treatment groups. At 12 h, the addition of 3 mg/L clove oil demonstrated a significant reduction (p < 0.05) in HSP90 expression compared to the baseline level; this was significantly lower (p < 0.05) than the other groups. After 24 and 48 h of simulated transport, the levels of HSP90 in all post-transport groups had significantly decreased (p < 0.05), with no significant differences (p > 0.05) between the control and the two treatment groups.
After 12 h simulated transport, GR levels (Figure 6C) had significantly increased (p < 0.05) in the control and 3 mg/L clove oil groups. The addition of 1 mg/L clove oil led to a significant decrease (p < 0.05) in GR expression, which was lower than the other groups, including the baseline level, at 24 and 48 h. While the control group was not significantly different (p > 0.05) from the baseline level, the addition of 3 mg/L clove oil resulted in a significant increase (p < 0.05) in the GR expression of the fish evaluated at 48 h.
The expression of MR (Figure 6D) was found to have significantly increased (p < 0.05) in all post-transport groups at 12 h compared to the baseline level. By 24 h, however, expression levels in the fish receiving the 1 mg/L clove oil treatment significantly decreased (p < 0.05). The control and other treatment groups also displayed variations in expression, notably in the group receiving 3 mg/L clove oil, which were significantly higher when compared to the baseline throughout the experiment. In contrast, slight down regulation of GR was observed in fish that received 1 mg/L clove oil at 24 and 48 h.
There were also significant increases (p < 0.05) in the expression levels of HIF-1α (Figure 6E) in the two treatment groups at 12 h. After 24 h, all post-transport groups showed a significant difference (p < 0.05) in HIF-1α when compared with the baseline. The 1 mg/L clove oil group had a significantly lower (p < 0.05) level of expression than those in the control and the 3 mg/L clove oil groups. At 48 h, HIF-1α expression in the fish receiving the 1 mg/L clove oil treatment a decreased and this was not significantly different from the baseline level.
4 Discussion
The burgeoning ornamental fish industry necessitates the transportation of live fish. Ornamental fish, with their unique characteristics, often endure long-distance travel in sealed plastic bags (41). Water quality deterioration is a predictable adverse effect of transportation (5). This is one of the most important triggers that can lead to stress (42). The observed decrease in dissolved oxygen in this study suggests an increase in carbon dioxide, a consequence of fish respiration, which can result in a sequential reduction in pH as was seen in the control group. The concentration of nitrite in each group was below detectable levels for much of the trial, however, levels were found to have increased after 48 h with an increase in fish metabolite accumulation (6). The increase in total ammonia nitrogen concentrations indicates metabolic waste accumulation, although the addition of 1 and 3 mg/L clove oil during transport showed no significant influence on reducing the amount of ammonia produced. Following the recommendations for betta transport, maintaining a constant water temperature during transport has advantages as it affects the solubility of oxygen and decreases ammonia toxicity (20). Therefore, even the calculated highest record of unionized ammonia (NH3-N) was 0.0081 mg/L, which are within the threshold for general aquaculture production (43). According to Hong et al. (44), a study involving golden pompano (Trachinotus ovatus) found that pH values dropped and the concentration of high unionized ammonia increased after 8 h of simulated transport; the trial concluded that most of the physiological and biochemical indicators that were measured were not significantly affected. This indicates adverse changes in pH, ammonia, or nitrite are considered a common issue that occurs with the transportation process (11, 19, 44) and within an acceptable threshold, according to Laongsiriwong (43).
Clove oil has been shown to slow respiration rates and oxygen consumption (30, 45), as evidenced by higher dissolved oxygen levels in the groups receiving the 1 and 3 mg/L clove oil treatments than in the control group. Furthermore, nitrite concentrations were significantly lower than in the control group of this study due to the slowdown of metabolic excretion.
Transport can provoke an increase in the number of total RBCs. This has been reported several studies investigating levels in a range of fish species, including common carp (46) and in dog snapper Lutjanus jocu [(see 10)]. Transport generated stress on betta fish in the current study, resulted in a marked increase in total RBC counts after 12 h of simulated transport. Coping with stress in the first period requires high energy demand, which includes mobilizing the number of RBCs. This response is essential, allowing the fish maximize the oxygen transport capabilities of its hemoglobin to serve increased energy demand (47, 48). Adding clove oil at a concentration of 1 and 3 mg/L, however, has the potential to reduce total RBC counts throughout the 48-h transport simulation when compared to the control without clove oil. This result follows the properties of anesthetics, which reduce metabolic activity and oxygen consumption (20, 31). Short-term stress sometimes results in an increase in total WBCs (49). According to Jia et al. (50), clove oil has antioxidant properties that can improve the immune system and has been shown to increase WBC number or increase phagocytic activity during bacterial challenge (51). This was not observed in the current study, in which clove oil did not significantly alter the total WBC counts during betta fish transport. The findings differ from previous research on short-term stress, such as handling stress or infected conditions mentioned above.
Stress is an energy-consuming process in which the fish’s perception of a stressor triggers three main cascade responses (52). The primary reaction is a neuroendocrine response that involve an increased release of corticosteroid hormone. Cortisol levels often rise during transportation (42), which boosts metabolism as an immediate energy source for fight or flight responses (14, 53). These observations have been documented in studies with several fish species, including golden pompano, Trachinotus ovatus [(see 44)], rohu, Labeo rohita [(see 54)], blood parrot cichlid, Amphilophus citrinellus × Cichlasoma synspilum, and koi, C. carpio [(see 35)]. The betta fish transportation approach used in the current study induced plasma cortisol for a brief period of 12 h, which then reverted to baseline levels throughout the remainder of the trial (i.e., as evaluated at the 24 and 48 post-start time points). This aligns with prior research that found suitable transportation conditions ensure adequate density and water quality are within acceptable criteria; thereafter, stress-related parameters can spontaneously return to normal within 24 h (11, 55, 56).
Furthermore, the addition of 1 mg/L clove oil can relieve stress, as indicated by the reduction of cortisol levels throughout the experiment when compared to the control group without clove oil. Clove oil is classified as a local anesthetic, which affects systemically by inhibiting the respiratory center in the medulla oblongata linked to central nervous system depression. In the context of this study, light clove oil doses (i.e., 1 mg/L) reduce transport stress by allowing fish to maintain equilibrium and reduce swimming activity (20, 31), resulting in serenity and reduced utilization of energy in stressful conditions. According to Akar (29), using 1 mg/L clove oil in blue tilapia, Oreochromis aureus, for 72 h during their transportation can reduce cortisol levels. In contrast to this, raising the concentration to 3 mg/L of clove oil can induce cortisol levels at the 12-h evaluation point like the findings of this study. Earlier studies have shown that using a high concentration of clove oil can induce the production of cortisol (29, 32, 57). Elevated levels may lead to hypoxia and acidosis due to a decrease in oxygen and an increase in carbon dioxide in the blood, resulting in stress or death (58, 59).
Elevated glucose levels are result of an increased catabolism through the gluconeogenesis and glycogenolysis pathways (14, 47). This increase is associated with a range of secondary responses that are modulated by hormones and circulate in the bloodstream to ensure immediate availability of energy. In the current study, no significant variations in plasma glucose were found, which is considered favorable signaling (60). This finding supports the opinion of Martínez-Porchas et al. (61) who stated that glucose should be used as a complement to stress assessments rather than being used as the main indication of stress.
Heat shock proteins (HSPs) mainly resolve or degrade damaged proteins under stressful circumstances (62). HSP70 is a major stress-induced protein in the HSP family (63) and quantifying it can be useful for determining the impact of a particular environment. HSP90, by comparison, influences cytoskeleton components and steroid hormone receptor regulation (64). Several studies have indicated that HSP70 and HSP90 expression can be utilized as cellular stress biomarkers for animals in transport (11). In the current study, the betta transport resulted in elevated HSP70 expression, which was significantly increased in the control group throughout the trial. This finding aligns with previous studies on spotted knifejaw (Oplegnathus punctatus) [(see 65)], common carp (C. carpio), rainbow trout (Oncorhynchus mykiss) [(see 66)], and on European seabass (Dicentrarchus labrax) [(see 67)]. This study suggests that the addition of clove oil at doses of 1 and 3 mg/L were able to inhibit HSP70 expression throughout the transport process whereas in normal conditions without clove oil, the expression of HSP70 would be triggered. The findings of the current study are consistent with those of Teles et al. (68), who conducted in a study using 42.7 ± 6.8 g gilthead seabream (Sparus aurata) found that a dose of 2.5 mg/L clove oil was able to reduce HSP70 levels for 24 h during their transportation. In contrast, the addition of clove oil appeared to have no significant effect on the expression of HSP90 under simulated transportation condition.
Cortisol mediates its effects by activating the glucocorticoid receptor (GR) and the mineralocorticoid receptor (MR), which stimulates gene expression for physiological changes (69, 70). The transport process employed for betta did not exhibit an increase in GR expression, while the expression of MR was found to have increased in all post-transport groups at the 12-h evaluation point. A study conducted by Bortoletti et al. (12) using meagre fish (Argyrosomus regius), found that transport did not result in an increase in GR levels in the liver. The results of the current study indicate that adding 1 mg/L clove oil can reduce GR and MR expression when compared to a control group that did not receive a dose of clove oil or to those fish which were transported in 3 mg/L clove oil. This finding suggests that low doses of clove oil when used for this species might have stress-relieving capabilities.
Betta fish are air-breathing anabantids that possess a suprabranchial organ that serves as a site of aerial respiration; this is also known as the labyrinth organ (71). The natural habitats of betta fish are frequently hypoxic and anoxic at the bottom, due to high water temperatures and high organic content (72). In their development, betta fish can at least partially escape hypoxia (72–75), and they may have a greater tolerance to aquatic hypoxia (7, 76). Hypoxia-inducible factors (HIFs), however, are critical regulators of the transcriptional response to hypoxic stress (77, 78). Previous research on HIF-1α in fish such as rainbow trout (50, 78, 79) and spotted knifejaw (77) has demonstrated its role in regulating oxygen adaptation and hypoxia tolerance (50, 77–79). In the current study, the transport process employed for betta exhibited an increase in HIF-1α expression, which returned to the baseline after 48 h of simulated transport in the treatment group that received a dose of 1 mg/L clove oil. The other experimental groups tended to show a decrease in expression. According to Da Silva et al. (80), most aquatic organisms appear to be well adapted to cope with periodic fluctuations in oxygen levels, influenced by various factors such as loading density, water temperature, and pure oxygen infusion. Similarly, Honryo et al. (81) discovered an increase in HIF-1α expression levels in the gills, as well as in whole-body cortisol levels, in juvenile Pacific bluefin tuna, Thunnus orientalis, exposed to transport stress immediately after their release into sea cage; the fish, however, were reported to recover within 24 h.
5 Conclusion
The investigation of stress indicators during the transportation of Siamese fighting fish has provided important insights. Transport variables were identified as potential sources of stress, as evidenced by elevated levels of total RBC and plasma cortisol, as well as upregulation of specific stress-related genes such as HSP70, GR, MR and HIF-1α. This highlights the physiological and cellular responses of fish to transportation compared to pre-transport fish, which have fewer stress indicators. However, such responses are common during fish transportation. It was found that the addition of 1 mg/L clove oil to the water reduced these stress indicators compared to the untreated control group. Conversely, increasing the clove oil concentration to 3 mg/L significantly increased some stress parameters, including plasma cortisol, GR, MR and HIF-1α. Consequently, these results suggest that the addition of clove oil at a concentration of 1 mg/L to the water is an appropriate strategy to reduce stress levels during short-term (12 h) or long-term (24–48 h) transportation of betta fish.
Data availability statement
The original contributions presented in the study are included in the article/supplementary material, further inquiries can be directed to the corresponding author.
Ethics statement
The animal study was approved by the Institutional Animal Care and Use Committee of Kasetsart University (approval ID: ACKU63-FIS-008). The study was conducted in accordance with the local legislation and institutional requirements.
Author contributions
CS: Conceptualization, Formal analysis, Investigation, Methodology, Writing – original draft, Writing – review & editing, Validation. WN: Validation, Writing – original draft, Writing – review & editing. SD: Writing – original draft, Writing – review & editing, Resources. CA: Writing – original draft, Writing – review & editing, Validation. RP: Methodology, Resources, Validation, Writing – original draft, Writing – review & editing. AS: Validation, Writing – original draft, Writing – review & editing. RW: Validation, Writing – original draft, Writing – review & editing. ND-H: Validation, Writing – original draft, Writing – review & editing. HD: Validation, Writing – original draft, Writing – review & editing. SC: Conceptualization, Funding acquisition, Project administration, Resources, Supervision, Validation, Writing – original draft, Writing – review & editing.
Funding
The author(s) declare that financial support was received for the research, authorship, and/or publication of this article. This research was funded by Kasetsart University through the Graduate School Fellowship Program and further supported by funding from Kasetsart University, Kamphaeng Saen Campus (KPS-RDI 2021-015).
Conflict of interest
AS and RW were employed by INVE Aquaculture.
The remaining authors declare that the research was conducted in the absence of any commercial or financial relationships that could be construed as a potential conflict of interest.
Publisher’s note
All claims expressed in this article are solely those of the authors and do not necessarily represent those of their affiliated organizations, or those of the publisher, the editors and the reviewers. Any product that may be evaluated in this article, or claim that may be made by its manufacturer, is not guaranteed or endorsed by the publisher.
References
1. Ashley, PJ. Fish welfare: current issues in aquaculture. Appl Anim Behav Sci. (2007) 104:199–235. doi: 10.1016/j.applanim.2006.09.001
2. Omeji, SA, Apochi, J, and Egwumah, KA. Stress concept in transportation of live fishes-A review. J Res Forest Wildlife Environ. (2017) 63:57–8. doi: 10.1515/forj-2017-0032
3. Bai, C, Qi, X, Wang, Z, Wang, J, Qiu, L, Li, H, et al. Effect of density stress on the physiological, biochemical, and immunological parameters of juvenile Pelteobagrus fulvidraco during simulated transportation. Aquacult Rep. (2024) 34:101911. doi: 10.1016/j.aqrep.2023.101911
4. Fang, D, Mei, J, Xie, J, and Qiu, W. The effects of transport stress (temperature and vibration) on blood biochemical parameters, oxidative stress, and gill histomorphology of pearl gentian groupers. Aust Fish. (2023) 8:218. doi: 10.3390/fishes8040218
5. Sampaio, FDF, and Freire, CA. An overview of stress physiology of fish transport: changes in water quality as a function of transport duration. Fish Fish. (2016) 17:1055–72. doi: 10.1111/faf.12158
6. Wang, Q, Ye, W, Tao, Y, Li, Y, Lu, S, Xu, P, et al. Transport stress induces oxidative stress and immune response in juvenile largemouth bass (Micropterus salmoides): analysis of oxidative and immunological parameters and the gut microbiome. Antioxidants. (2023) 12:157. doi: 10.3390/antiox12010157
7. Monvises, A, Nuangsaeng, B, Sriwattanarothai, N, and Panijpan, B. The Siamese fighting fish: well-known generally but little-known scientifically. ScienceAsia. (2009) 35:8–16. doi: 10.2306/scienceasia1513-1874.2009.35.008
8. Pleeging, C, and Moons, CPH. Potential welfare issues of the Siamese fighting fish (Betta splendens) at the retailer and in the hobbyist aquarium. Vlaams Diergeneeskundig Tijdschrift. (2017) 86:213–23. doi: 10.21825/vdt.v86i4.16182
9. People for the Ethical Treatment of Animals (PETA). PETA asia reveals horrors in the global betta fish trade (2020). Available at: https://investigations.peta.org/petco-betta-fish-supplier-thailand/ (Accessed 1 July 2022).
10. Oliveira, B, Souza, R, Filho, J, Gomes, L, and Fernandes, LF. Hematological and biochemical characteristics during the transport of dog snapper Lutjanus jocu (Perciformes: Lutjanidae). Neotrop Ichthyol. (2018) 16:e170140. doi: 10.1590/1982-0224-20170140
11. Refaey, MM, and Li, D. Transport stress changes blood biochemistry, antioxidant defense system, and hepatic hsps mrna expressions of channel catfish Ictalurus punctatus. Front Physiol. (2018) 9:1628. doi: 10.3389/fphys.2018.01628
12. Bortoletti, M, Maccatrozzo, L, Radaelli, G, Caberlotto, S, and Bertotto, D. Muscle cortisol levels, expression of glucocorticoid receptor and oxidative stress markers in the teleost fish Argyrosomus regius exposed to transport stress. Animals. (2021) 11:1160. doi: 10.3390/ani11041160
13. Yousefi, M, Hoseini, SM, Weber, RA, da Silva, E, Rajabiesterabadi, H, Arghideh, M, et al. Alleviation of transportation-induced stress in Nile tilapia, Oreochromis niloticus, using brackish water. Aquacult Rep. (2022) 27:101378. doi: 10.1016/j.aqrep.2022.101378
14. Barton, BA. Stress in fishes: a diversity of responses with particular reference to changes in circulating corticosteroids. Integr Comp Biol. (2002) 42:517–25. doi: 10.1093/icb/42.3.517
15. Fazio, F, Costa, A, Capparucci, F, Costa, G, Parrino, V, and Arfuso, F. Automated hematological approach and protein electrophoretic pattern in tilapia (Oreochromis niloticus): an innovative and experimental model for aquaculture. Animals. (2024) 14:392. doi: 10.3390/ani14030392
16. Pankhurst, NW. The endocrinology of stress in fish: an environmental perspective. Gen Comp Endocrinol. (2011) 170:265–75. doi: 10.1016/j.ygcen.2010.07.017
17. Pottinger, T. The stress response in fish-mechanisms, effects and measurement In: JB Edward, editor. Fish welfare. New Jersey: Blackwell Publishing (2008). 32–48.
18. Pottinger, TG. A multivariate comparison of the stress response in three salmonid and three cyprinid species: evidence for inter-family differences. J Fish Biol. (2010) 76:601–21. doi: 10.1111/j.1095-8649.2009.02516.x
19. Thongprajukaew, K, Takaeh, S, Esor, N, Saekhow, S, Malawa, S, Nuntapong, N, et al. Optimal water volume for transportation of male Siamese fighting fish (Betta splendens). Aquacult Rep. (2023) 28:101430. doi: 10.1016/j.aqrep.2022.101430
20. Harmon, TS. Methods for reducing stressors and maintaining water quality associated with live fish transport in tanks: a review of the basics. Rev Aquac. (2009) 1:58–66. doi: 10.1111/j.1753-5131.2008.01003.x
21. Acar, Ü, Kesbiç, OS, Yılmaz, S, İnanan, BE, Zemheri-Navruz, F, Terzi, F, et al. Effects of essential oil derived from the bitter orange (Citrus aurantium) on growth performance, histology and gene expression levels in common carp juveniles (Cyprinus carpio). Animals. (2021) 11:1431. doi: 10.3390/ani11051431
22. Acar, Ü, Parrino, V, Kesbiç, OS, Lo Paro, G, Saoca, C, Abbate, F, et al. Effects of different levels of pomegranate seed oil on some blood parameters and disease resistance against Yersinia ruckeri in rainbow trout. Front Physiol. (2018) 9:596. doi: 10.3389/fphys.2018.00596
23. Calabrese, S, Jonassen, TM, Steigum, E, Åsnes, HØ, Imsland, AKD, Saude, CS, et al. Does sedation with AQUI-S® mitigate transport stress and post transport mortality in ballan wrasse (Labrus bergyltae)? Front Vet Sci. (2024) 11:1347062. doi: 10.3389/fvets.2024.1347062
24. Sharma, S, and Husen, A. Anaesthetics efficacy of MS-222, Benzoak® vet, AQUI-S® and clove oil on common carp (Cyprinus carpio) fry. Int J Res Fisher Aquacult. (2015) 14:104–14. doi: 10.1371/journal.pone.0211080
25. Cárdenas, C, Toni, C, Martos-Sitcha, JA, Cárdenas, S, de Las Heras, V, Baldisserotto, B, et al. Effects of clove oil, essential oil of Lippia alba and 2-phe anaesthesia on juvenile meagre, Argyrosomus regius (Asso, 1801). J Appl Ichthyol. (2016) 32:693–700. doi: 10.1111/jai.13048
26. Pattanasiri, T, Taparhudee, W, and Suppakul, P. Acute toxicity and anaesthetic effect of clove oil and eugenol on Siamese fighting fish, Betta splendens. Aquac Int. (2016) 25:163–75. doi: 10.1007/s10499-016-0020-2
27. Hekimoglu, MA, Suzer, C, Saka, Ş, and Fırat, MK. Sedative effect of clove oil and 2-phenoxyethanol on marine clownfish (Amphiprion ocellaris) and freshwater swordfish (Xiphophorus helleri). Pak J Zool. (2017) 49:2209–16. doi: 10.17582/journal.pjz/2017.49.6.2209.2216
28. Gökçek, C, Öğretmen, F, and Kanyılmaz, M. Efficacy of clove oil, 2-phenoxyethanol and benzocaine on European catfish, Silurus glanis linnaeus 1758. Turk J Fish Aquat Sci. (2017) 17:129–33. doi: 10.4194/1303-2712-v17_1_15
29. Akar, A. Effects of clove oil on the response of blue tilapia (Oreochromis aureus) by transportation stress. J Arab Aquacult Soc. (2011) 6:77–86.
30. Aydın, B, and Barbas, LAL. Sedative and anesthetic properties of essential oils and their active compounds in fish: A review. Aquaculture. (2020) 520:734999. doi: 10.1016/j.aquaculture.2020.734999
31. Javahery, S, Nekoubin, H, and Moradlu, AH. Effect of anaesthesia with clove oil in fish (review). Fish Physiol Biochem. (2012) 38:1545–52. doi: 10.1007/s10695-012-9682-5
32. Jerez-Cepa, I, Fernández-Castro, M, Del Santo O’Neill, TJ, Martos-Sitcha, JA, Martínez-Rodríguez, G, Mancera, JM, et al. Transport and recovery of gilthead seabream (Sparus aurata L.) sedated with clove oil and MS-222: effects on stress axis regulation and intermediary metabolism. Front Physiol. (2019) 10:612. doi: 10.3389/fphys.2019.00612
33. Aydın, İ, Akbulut, B, Küçük, E, and Kumlu, M. Effects of temperature, fish size and dosage of clove oil on anaesthesia in turbot (Psetta maxima Linnaeus, 1758). Turk J Fish Aquat Sci. (2015) 15:899–904. doi: 10.4194/1303-2712-v15_4_13
34. Sintuprom, C, Ploypan, R, Nuchchanart, W, and Chatchaiphan, S. Effect of clove oil on water quality and stress-related gene expression in Siamese fighting fish (Betta splendens Regan, 1910) during short-term transportation. J Sci Technol Kasetsart University. (2024) 13
35. Wu, SM, Chen, J-R, Chang, C-y, Tseng, Y-J, and Pan, BS. Potential benefit of I-tiao-gung (Glycine tomentella) extract to enhance ornamental fish welfare during live transport. Aquaculture. (2021) 534:736304. doi: 10.1016/j.aquaculture.2020.736304
36. Davis, DJ, Klug, J, Hankins, M, Doerr, HM, Monticelli, SR, Song, A, et al. Effects of clove oil as a euthanasia agent on blood collection efficiency and serum cortisol levels in Danio rerio. J Am Assoc Lab Anim Sci. (2015) 5:564–7.
37. APHA, AWWA, WEF. Standard methods for the examination of water and wastewater. 19th ed. New York: American Public Health Association Inc. (1995).
38. Babaei, F, Ramalingam, R, Tavendale, A, Liang, Y, Yan, LS, Ajuh, P, et al. Novel blood collection method allows plasma proteome analysis from single zebrafish. J Proteome Res. (2013) 12:1580–90. doi: 10.1021/pr3009226
39. Livak, KJ, and Schmittgen, TD. Analysis of relative gene expression data using real-time quantitative PCR and the 2(-Delta Delta C(T)) method. Methods. (2001) 25:402–8. doi: 10.1006/meth.2001.1262
40. Amparyup, P, Charoensapsri, W, Samaluka, N, Chumtong, P, Yocawibun, P, Imjongjirak, C, et al. Transcriptome analysis identifies immune-related genes and antimicrobial peptides in Siamese fighting fish Shellfish Immunol. Fish Shellfish Immunol. (2020) 99:403–13. doi: 10.1016/j.fsi.2020.02.030
41. Lim, LC, Dhert, P, and Sorgeloos, P. Recent developments and improvements in ornamental fish packaging systems for air transport. Aquac Res. (2003) 34:923–35. doi: 10.1046/j.1365-2109.2003.00946.x
42. Portz, D, Woodley, C, and Cech, J. Stress-associated impacts of short-term holding on fishes. Rev Fish Biol Fish. (2006) 16:125–70. doi: 10.1007/s11160-006-9012-z
44. Hong, J, Chen, X, Liu, S, Fu, Z, Han, M, Wang, Y, et al. Impact of fish density on water quality and physiological response of golden pompano (Trachinotus ovatus) flingerlings during transportation. Aquaculture. (2019) 507:260–5. doi: 10.1016/j.aquaculture.2019.04.040
45. Santos, ELR, Rezende, FP, and Moron, SE. Stress-related physiological and histological responses of tambaqui (Colossoma macropomum) to transportation in water with tea tree and clove essential oil anesthetics. Aquaculture. (2020) 523:735164. doi: 10.1016/j.aquaculture.2020.735164
46. Dobšíková, R, Svobodová, Z, Blahová, J, Modrá, H, and Velisek, J. The effect of transport on biochemical and haematological indices of common carp (Cyprinus carpio L.). Czeh J Anim Sci. (2009) 54:510–8. doi: 10.17221/52/2009-CJAS
47. Sopinka, NM, Donaldson, MR, O’Connor, CM, Suski, CD, and Cooke, SJ. 11—stress indicators in fish In: CB Schreck, L Tort, AP Farrell, and CJ Brauner, editors. Fish physiology, vol. 35: Academic Press (2016). 35:405–62.
48. Witeska, M, Kondera, E, Ługowska, K, and Bojarski, B. Hematological methods in fish – not only for beginners. Aquaculture. (2022) 547:737498. doi: 10.1016/j.aquaculture.2021.737498
49. Tort, L. Stress and immune modulation in fish. Dev Comp Immunol. (2011) 35:1366–75. doi: 10.1016/j.dci.2011.07.002
50. Jia, Y, Xie, T, Gao, Y, Qin, H, and Guan, C. Anesthetics efficacy and physiological response of MS222 and clove oil in spotted knifejaw Oplegnathus punctatus. Aquacult Rep. (2022) 25:101201. doi: 10.1016/j.aqrep.2022.101201
51. Abdelkhalek, NK, Risha, E, El-Adl, MA, Salama, MF, and Dawood, MAO. Antibacterial and antioxidant activity of clove oil against Streptococcus iniae infection in Nile tilapia (Oreochromis niloticus) and its effect on hepatic hepcidin expression. Fish Shellfish Immunol. (2020) 104:478–88. doi: 10.1016/j.fsi.2020.05.064
52. Schreck, CB, and Tort, L. 1—the concept of stress in fish In: CB Schreck, L Tort, AP Farrell, and CJ Brauner, editors. Fish physiology. Massachusetts: Academic Press (2016). 35:1–34.
53. Mommsen, TP, Vijayan, MM, and Moon, TW. Cortisol in teleosts: dynamics, mechanisms of action, and metabolic regulation. Rev Fish Biol Fish. (1999) 9:211–68. doi: 10.1023/A:1008924418720
54. Pakhira, C, Nagesh, TS, Abraham, TJ, Dash, G, and Behera, S. Stress responses in rohu, Labeo rohita transported at different densities. Aquacult Rep. (2015) 2:39–45. doi: 10.1016/j.aqrep.2015.06.002
55. Gomes, L, Roubach, R, Araujo-Lima, C, Chippari-Gomes, A, Lopes, N, and Urbinati, E. Effect of fish density during transportation on stress and mortality of juvenile tambaqui Colossoma macropomum. J World Aquacult Soc. (2007) 34:76–84. doi: 10.1111/j.1749-7345.2003.tb00041.x
56. Urbinati, EC, de Abreu, JS, da Silva Camargo, AC, and Landinez Parra, MA. Loading and transport stress of juvenile matrinxã (Brycon cephalus, Characidae) at various densities. Aquaculture. (2004) 229:389–400. doi: 10.1016/S0044-8486(03)00350-8
57. Navarro, RD, de França, RP, Paludo, GR, Bizarro, YWS, da Silva, RF, and Navarro, FKSP. Physiological and hematological responses of Nile tilapia (Oreochromis Niloticus) to different anesthetics during simulated transport conditions. Act Sci Technol. (2016) 38:301–6. doi: 10.4025/actascitechnol.v38i3.28377
58. Cooke, SJ, Suski, CD, Ostrand, KG, Tufts, BL, and Wahl, DH. Behavioral and physiological assessment of low concentrations of clove oil anaesthetic for handling and transporting largemouth bass (Micropterus salmoides). Aquaculture. (2004) 239:509–29. doi: 10.1016/j.aquaculture.2004.06.028
59. Souza, CF, Baldissera, MD, Baldisserotto, B, Heinzmann, BM, Martos-Sitcha, JA, and Mancera, JM. Essential oils as stress-reducing agents for fish aquaculture: a review. Front Physiol. (2019) 10:785. doi: 10.3389/fphys.2019.00785
60. Stien, LH, Bracke, M, Noble, C, and Kristiansen, TS. Assessing fish welfare in aquaculture. In: TS Kristiansen, A Fernö, MA Pavlidis, and H Visvan de, editors. The welfare of fish. Cham: Springer International Publishing (2020). p. 303–321.
61. Martínez-Porchas, M, Martínez-córdova, LR, and Ramos-Enriquez, R. Cortisol and glucose: reliable indicators of fish stress? Pan Am J Aquat Sci. (2009) 4:158–78.
62. Mohanty, BP, Mahanty, A, Mitra, T, Parija, SC, and Mohanty, S. Heat shock proteins in stress in Teleosts In: AAA Asea and P Kaur, editors. Regulation of heat shock protein responses. Cham: Springer International Publishing (2018). 71–94.
63. Iwama, GK, Thomas, PT, Forsyth, RB, and Vijayan, MM. Heat shock protein expression in fish. Rev Fish Biol Fish. (1998) 8:35–56. doi: 10.1023/A:1008812500650
64. Rangaswamy, B, Kim, WS, and Kwak, IS. Heat shock protein 70 reflected the state of inhabited fish response to water quality within lake ecosystem. Int J Environ Sci Technol. (2023) 21:643–54. doi: 10.1007/s13762-023-04971-0
65. Xie, T, Gao, Y, Qin, H, Zhang, J, Li, M, Gao, Y, et al. Physiological response of spotted knifejaw (Oplegnathus punctatus) during transportation in offshore aquaculture net pen. Aquaculture. (2023) 563:739029. doi: 10.1016/j.aquaculture.2022.739029
66. Poltronieri, C, Negrato, E, Bertotto, D, Majolini, D, Simontacchi, C, and Radaelli, G. Immunohistochemical localization of constitutive and inducible heat shock protein 70 in carp (Cyprinus carpio) and trout (Oncorhynchus mykiss) exposed to transport stress. Eur J Histochem. (2008) 52:191–8. doi: 10.4081/1211
67. Bertotto, D, Poltronieri, C, Negrato, E, Richard, J, Pascoli, F, Simontacchi, C, et al. Whole body cortisol and expression of HSP70, IGF-I and MSTN in early development of sea bass subjected to heat shock. Gen Comp Endocrinol. (2011) 174:44–50. doi: 10.1016/j.ygcen.2011.08.003
68. Teles, M, Oliveira, M, Jerez-Cepa, I, Franco-Martínez, L, Tvarijonaviciute, A, Tort, L, et al. Transport and recovery of gilthead sea bream (Sparus aurata L.) sedated with clove oil and MS222: effects on oxidative stress status. Front Physiol. (2019) 10:523. doi: 10.3389/fphys.2019.00523
69. Aedo, JE, Zuloaga, R, Aravena-Canales, D, Molina, A, and Valdés, JA. Role of glucocorticoid and mineralocorticoid receptors in rainbow trout (Oncorhynchus mykiss) skeletal muscle: A transcriptomic perspective of cortisol action. Front Physiol. (2022) 13:1048008. doi: 10.3389/fphys.2022.1048008
70. Greenwood, AK, Butler, PC, White, RB, DeMarco, U, Pearce, D, and Fernald, RD. Multiple corticosteroid receptors in a teleost fish: distinct sequences, expression patterns, and transcriptional activities. Endocrinology. (2003) 144:4226–36. doi: 10.1210/en.2003-0566
71. Alton, LA, Portugal, SJ, and White, CR. Balancing the competing requirements of air-breathing and display behaviour during male–male interactions in Siamese fighting fish Betta splendens. Comp Biochem Physiol A Mol Integr Physiol. (2013) 164:363–7. doi: 10.1016/j.cbpa.2012.11.012
72. Mendez-Sanchez, JF, and Burggren, WW. Cardiorespiratory physiological phenotypic plasticity in developing air-breathing anabantid fishes (Betta splendens and Trichopodus trichopterus). Physiol Rep. (2017) 5:e13359. doi: 10.14814/phy2.13359
73. Mendez-Sanchez, JF, and Burggren, WW. Environmental modulation of the onset of air breathing and survival of Betta splendens and Trichopodus trichopterus. J Fish Biol. (2014) 84:794–807. doi: 10.1111/jfb.12322
74. Mendez-Sanchez, JF, and Burggren, WW. Hypoxia-induced developmental plasticity of larval growth, gill and labyrinth organ morphometrics in two anabantoid fish: the facultative air-breather Siamese fighting fish (Betta splendens) and the obligate air-breather the blue gourami (Trichopodus trichopterus). J Morphol. (2019) 280:193–204. doi: 10.1002/jmor.20931
75. Mendez-Sanchez, JF, and Burggren, WW. Very high blood oxygen affinity and large Bohr shift differentiates the air-breathing siamese fighting fish (Betta splendens) from the closely related anabantoid the blue gourami (Trichopodus trichopterus). Comp Biochem Physiol Part A Mol Integr Physiol. (2019) 229:45–51. doi: 10.1016/j.cbpa.2018.11.014
76. Goldstein, RJ. The betta handbook. Barron’s pet handbooks. New york: B.E.S. Publishing (2004). 167 p.
77. Ren, Y, Men, X, Yu, Y, Li, B, Zhou, Y, and Zhao, C. Effects of transportation stress on antioxidation, immunity capacity and hypoxia tolerance of rainbow trout (Oncorhynchus mykiss). Aquacult Rep. (2022) 22:100940. doi: 10.1016/j.aqrep.2021.100940
78. Wu, S, Huang, J, Li, Y, and Pan, Y. Dynamic and systemic regulatory mechanisms in rainbow trout (Oncorhynchus mykiss) in response to acute hypoxia and reoxygenation stress. Aquaculture. (2023) 572:739540. doi: 10.1016/j.aquaculture.2023.739540
79. Wu, Y, Zhao, M, Xiong, G, Sun, W, Wu, W, Ding, A, et al. Effects of hypoxia on meat qualities and muscle metabolism in rainbow trout (Oncorhynchus mykiss) during short-time transportation and its relief by reoxygenation. Aquaculture. (2023) 570:739404. doi: 10.1016/j.aquaculture.2023.739404
80. Da Silva, TVN, Barbas, LAL, Torres, MF, Sampaio, LA, and Monserrat, JM. Lipid peroxidation and antioxidant capacity in Peckoltia oligospila (Günther, 1864) submitted to transport under different concentration of dissolved oxygen. Aquaculture. (2017) 481:72–8. doi: 10.1016/j.aquaculture.2017.08.024
Keywords: Betta splendens, transportation, stress, clove oil, animal welfare
Citation: Sintuprom C, Nuchchanart W, Dokkaew S, Aranyakanont C, Ploypan R, Shinn AP, Wongwaradechkul R, Dinh-Hung N, Dong HT and Chatchaiphan S (2024) Effects of clove oil concentrations on blood chemistry and stress-related gene expression in Siamese fighting fish (Betta splendens) during transportation. Front. Vet. Sci. 11:1392413. doi: 10.3389/fvets.2024.1392413
Edited by:
Rodrigo Muiño, Veterinary Centre Meira, SpainReviewed by:
Channarong Rodkhum, Chulalongkorn University, ThailandVincenzo Parrino, University of Messina, Italy
Copyright © 2024 Sintuprom, Nuchchanart, Dokkaew, Aranyakanont, Ploypan, Shinn, Wongwaradechkul, Dinh-Hung, Dong and Chatchaiphan. This is an open-access article distributed under the terms of the Creative Commons Attribution License (CC BY). The use, distribution or reproduction in other forums is permitted, provided the original author(s) and the copyright owner(s) are credited and that the original publication in this journal is cited, in accordance with accepted academic practice. No use, distribution or reproduction is permitted which does not comply with these terms.
*Correspondence: Satid Chatchaiphan, c2F0aWQuY2hAa3UudGg=