- 1School of Biological Engineering, Dalian Polytechnic University, Dalian, China
- 2Dalian Sanyi Biotechnology Research Institute, Dalian Sanyi Animal Medicine Co., Ltd., Dalian Liaoning, China
- 3Research Quality Control Center, Jiangsu Sanyi Animal Nutrition Technology Co., Ltd., Xuzhou, China
- 4Harbin Academy of Agricultural Sciences, Harbin Heilongjiang, China
The experiment aimed to evaluate the effects of different ratios of Clostridium autoethanogenum protein (CAP) used in the diets on the growth performance, muscle quality, serum indexes, and mTOR pathway of white feather broilers. Four hundred and eighty 1-day-old Arbor Acres (AA) broilers, comprising equal numbers of males and females, were randomly assigned to one of four treatments, and each treatment consisted of 12 replicates of 10 birds. Four diets were formulated based on isoenergetic and isonitrogenous principles. The control group (CAP 0) did not receive any CAP, while the experimental groups received 2% (CAP 2), 3% (CAP 3), and 4% (CAP 4) of CAP for six weeks. Compared with the CAP0, (1) The feed conversion ratio (FCR) was lower (p < 0.05), and the leg muscle yield was higher (p < 0.05) in the CAP3 and CAP4; (2) The serum levels of TP, ALB, T-AOC, and SOD were improved in the CAP3 (p < 0.05); (3) The expression of Lipin-1 gene was down-regulated and AMPKɑ2, Akt, and 4E-BP1 genes were up-regulated in the experiment group (p < 0.05); (4) The inclusion of 3% CAP in the diet increased the levels of 4E-BP1, S6K1, Akt, and AMPKɑ2 phosphorylation by modulating the mTOR signaling pathway (p < 0.05). In conclusion, broiler diets containing 3% CAP can activate the mTOR signaling pathway to promote muscle synthesis and improve growth performance.
Introduction
The world’s population has increased dramatically in recent years and could reach 10 billion by 2050 (1, 2). As a result, the global demand for meat is expected to exceed 400 million tons, while the demand for dairy products will surpass 800 million tons (3). Soybean meal is widely used in the breeding industry because of its high protein content and relatively low antinutritional factors. However, China relies heavily on soybean imports and unstable prices, severely limiting the growth of the animal husbandry industry. Therefore, reducing the use of crude protein (CP) in diets and developing and using new protein sources are essential to alleviate the shortage of protein resources.
Low protein diets are based on the ideal protein model by adding certain synthetic amino acids to compensate for the reduction in protein so that the type, amount, and ratio of amino acids required for animal growth are met, which can improve feed conversion, animal nitrogen deposition, and emissions while ensuring that the normal production performance of livestock and poultry is maintained (4–6). Compared with traditional rations, low-protein diets can reduce feeding costs, improve animal health, and have great advantages in the area of environmentally friendly and healthy breeding (7, 8). Supported by national policies, low-protein diets have been widely used in the breeding industry of China.
Microorganisms such as yeasts, algae, fungi, and bacteria can produce single-cell proteins (SCPs) that contain high amounts of protein, vitamins and minerals. SCP can effectively substitute protein feedstocks like fishmeal and soybean meal (9, 10). In addition, the production of SCP is not limited by time and geography and can be factory-produced on a large scale (11). Numerous studies have confirmed that SCP can be used in broiler diets to reduce the need for soybean meal. Furthermore, the appropriate amount of SCP can increase the breast and leg muscle yields while also improving the weight gain, FCR, and economic efficiency of broilers (12–14).
CAP produced by Clostridium autoethanogenum (CA) is a SCP with a relatively balanced amino acid profile (15, 16). It has potential as a replacement for protein raw materials in livestock, poultry, and aquatic products. Research has demonstrated that CAP can serve as a viable replacement for fishmeal due to its high protein and amino acid content, lack of anti-nutritional factors, and low biogenic amine content (17, 18). Besides, CA can convert inorganic matter into organic matter and decrease the discharge of industrial waste, making it a valuable contributor to the promotion of environmentally friendly and sustainable economic development (19).
The mTOR pathway is responsible for nutrient sensing and regulates organismal growth and metabolism (20–22). Inhibiting the mTOR pathway reduces protein synthesis while activating it promotes protein synthesis (23, 24). Additionally, it promotes cell growth and proliferation by increasing protein and lipid synthesis and decreasing cellular autophagy (25). Numerous studies in aquaculture have demonstrated that CAP can regulate the mTOR signaling pathway, improve organismal growth, and enhance growth performance (26–28). However, there is a lack of research on CAP in livestock and poultry, and the mechanisms of metabolism and growth regulation remain unclear. Therefore, studying the effects and regulatory mechanisms of CAP feeding in broilers can provide theoretical support for the application of CAP in broilers, which is of great research value and theoretical significance. We hypothesized that the appropriate proportions of CAP could regulate broiler growth through the mTOR signaling pathway. Therefore, a feeding trial was conducted using four gradients of CAP (CAP0, CAP2, CAP3, and CAP4) to assess its effects on broiler performance, muscle quality, serum indexes, and the mTOR pathway.
Materials and methods
Experimental design and diets
In our experiment, a total of 480 one-day-old Arbor Acres (AA) broilers (initial body weight 43.84 ± 1.38 g) purchased from Zhenghe Poultry Industry Co., Ltd. in Shangqiu City were randomly divided into four treatment groups, with 12 replicates of 10 birds per treatment (half male and half female). The broilers were fed with four isoenergetic and isonitrogenous diets containing CAP at 0 g/kg (Control, CAP0), 20 g/kg (CAP2), 30 g/kg (CAP3), and 40 g/kg (CAP4) respectively, and the experimental feed formulation complied with DB22_T3207-2020 “Technical specifications for low protein diet for broiler chickens.” The experiment consisted of two stages. The early-stage diet was fed to the subjects from 1 to 21 days, while the later-stage diet was fed from 22 to 42 days. For the nutritional composition and ingredients of the diets, refer to Tables 1, 2. The temperature was kept at 33°C for the initial three days, after which it was gradually decreased by 1°C every two days until it reached 20°C. The light period consisted of three consecutive days after hatching, followed by 23 h of light and 1 h of darkness throughout the remainder experimental period. The broilers were vaccinated according to the immunization schedule and had free access to feed and fresh water, while the relative humidity was maintained at 60%.
Production performance
At the end of 1, 21, and 42 d, chickens (after a 12 h fast) were weighed, and feed intake and quantity of dead broiler were recorded for each treatment pen for the calculation of average daily gain (ADG), average daily feed intake (ADFI), and FCR. At 42 d, blood samples were collected from 24 broilers (Randomly selected 6 chickens for each treatment, male and female halves) via bleeding from the jugular vein. After obtaining the blood sample, it was centrifuged at 3,000 g at 4°C for 10 min. In this way, the serum is obtained. Following the jugular vein bloodletting and execution, the slaughtering performance was evaluated by the NYT823-2020 standard.
Muscle composition
Breast muscle samples were baked in an oven at 65°C to make air-dried samples, and then analyzed according to the (29) standard method. Briefly, the samples were dried in an oven at 105°C until constant weight for calculation of dry matter. The CP content was determined by the Kjeldahl nitrogen method. The crude lipid content was measured by ether extraction using a Soxhlet extractor. The ash content was determined by combustion at 550°C in a muffle furnace for 6 h.
Serum biochemical and antioxidant indexes
Alanine aminotransferase (ALT), aspartate transaminase (AST), alkaline phosphatase (ALP), lactate dehydrogenase (LDH), amylase (AMS), total protein (TP), albumin (ALB), uric acid (UA), glucose (GLU), triglyceride (TG), total cholesterol (TC), high-density lipoprotein (HDL), low-density lipoprotein (LDL), total antioxidant capacity (T-AOC), catalase (CAT), malondialdehyde (MDA), glutathione peroxidase (GSH-Px), and superoxide dismutase (SOD) were determined by assay kits (Nanjing Jiancheng Co., Nanjing, China).
Determination of immunological indexes
Procalcitonin (PCT), immunoglobulin A (IgA), immunoglobulin G (IgG), interleukin-1 (IL-1), interleukin-6 (IL-6), and interleukin-8 (IL-8) were determined by assay kits (Shanghai Enzyme Link Biotechnology Co., Shanghai, China).
2.6 RNA extraction, cDNA synthesis, and real-time PCR
RNA was first extracted from breast muscle and liver samples using the RNAiso Plus kit (Takara, Japan). The concentrations and OD260/OD280 of RNA were then assessed using a Micro-Drop spectrophotometer (BIO-DL, United States), and complementary DNA (cDNA) was synthesized using a PrimeScript™ RT reagent kit (Takara, Japan). The reaction system was prepared according to the fluorescent quantitative PCR kit. The following were the thermocycling conditions for the target genes: 95°C for 30 s, 95°C for 15 s, and 60°C for 30 s to collect the fluorescence signals, and 40 cycles were performed. Quantification of relative gene expression was performed using 2-ΔΔCT. The targeted primers for several genes in this study are indicated in Table 3.
2.7 Protein extraction and Western blot
According to the mass and volume of each sample, add the corresponding volume of lysate, let it stand for 5 min, centrifuge at 12,000 r for 10 min, and the supernatant is the protein extract. The protein concentration of the samples was determined according to the instructions of the BCA Protein Concentration Assay Kit (Solarbio Beijing, China). After determining the quantity of protein to be loaded, diluted protein samples with 5 × Loading Buffer and PBS and boiled at 100°C for 5 min. Equal amounts of protein were loaded into the wells of the SDS-PAGE gel, and the proteins were then transferred from the gel to the PVDF membrane. Subsequently, the membrane was closed with 5% skimmed milk powder for an hour. After incubation with primary and secondary antibodies, the PVDF membrane was placed in a chemical exposure apparatus, evenly sprinkled with ECL chemiluminescent solution, and the exposure time was set to expose the membrane. The results were analyzed using a gel image processing system (ImageJ) to analyze the gray scale values of the target bands. The antibodies used in the present study included: phospho-mTOR (Ser2448), mTOR, 4EBP1, AKT, and phospho-AKT (Ser473) purchased from Proteintech Group; phospho-p70S6 Kinase (Thr389), AMPK alpha-2, and phospho-AMPK alpha-1,2 (Thr172, Thr183) purchased from Thermo Fisher Scientific; p70S6 Kinase purchased from Affinity; phospho-4E-BP1 (Thr37/46) (236B4) purchased from cell signaling Technology.
Statistical analysis
The experimental data was collated using Excel 2021, and the Shapiro–Wilk test was performed, followed by one-way ANOVA (one-way ANOVA) using SPSS 26.0 and Duncan’s method for multiple comparisons. The values were presented as mean ± SEM, and differences were considered statistically significant at p < 0.05.
Results
Effects of using CAP in diets on broiler growth performance
The effects of different proportions of CAP added to the diets on the growth performance of broilers are shown in Table 4. At 22–42 d, the FCR was lower in the CAP3 group than in the CAP0 group (p < 0.05). At the 1–42 d, the FCR was lower in the CAP3 and CAP4 groups than in the CAP0 group (p < 0.05).
Effects of using CAP in diets on the slaughtering performance of broiler chickens
As shown in Table 5, CAP had no significant effect on dressing percentage, percentage of half-eviscerated yield, and percentage of eviscerated yield (p > 0.05) but had an impact on breast muscle yield and leg muscle yield (p < 0.05). The breast muscle yield of the CAP3 group was higher than the other groups (p < 0.05), and the leg muscle yield of the CAP0 group was lower than the CAP3 and CAP4 groups (p < 0.05).
Effects of using CAP in diets on muscle composition of broiler chickens
Table 6 describes the effects of adding CAP on meat quality and composition of broiler. The muscle crude protein content in the CAP0 group was lower than the CAP3 group (p < 0.05).
Effects of using CAP in diets on serum biochemical indices of broiler chickens
As shown in Table 7, dietary CAP inclusion levels influenced the serum biochemical indexes of broilers. The CAP0 and CAP4 groups had lower TP levels than the CAP3 group (p < 0.05). Broilers fed with CAP0 and CAP2 diets had lower UA content compared to the CAP3 and CAP4 diets (p < 0.05). The CAP3 group had the lowest TG levels, followed by the CAP4 group. The AST activity in CAP4 was higher than CAP0 and CAP2 groups (p < 0.05).
Effects of using CAP in diets on antioxidant indexes in broiler chickens
As shown in Table 8, the T-AOC in the CAP0 group was lower than in the CAP2 and CAP3 groups (p < 0.05). As for the GSH-Px, the CAP2, CAP3, and CAP4 groups were higher than the CAP0 group (p < 0.05). SOD was higher in the CAP3 group than in the CAP0 and CAP4 groups (p < 0.05).
Effects of using CAP in diets on immune and inflammatory factors in broiler chickens
Table 9 shows the effects of adding CAP on the immune and inflammatory factors of the broiler. Broilers fed with CAP3 and CAP4 diets had significantly higher IgG levels compared to those fed with CAP0 and CAP2 diets (p < 0.05).
Gene expression associated with mammalian targets of the mTOR signaling pathway in breast muscle and liver
The mRNA levels of genes related to the mTOR signaling pathway in chest muscle are shown in Figure 1. The expression level of Lipin-1 was lower in the CAP2, CAP3, and CAP4 groups compared to the CAP0 group, with the lowest level observed in the CAP3 group (p < 0.05). In contrast, AKT expression was higher in the CAP4 groups than in the CAP0 groups (p < 0.05). Broiler-fed diets CAP4 had higher expression of PI3K than those fed diet CAP0 (p < 0.05). Broilers fed CAP3 and CAP4 diets showed a higher mRNA level of AMPKɑ2 and 4EBP1 compared with broiler-fed diets CAP0 and CAP2 (p < 0.05). Additionally, a difference was observed between the CAP3 and CAP4 groups in the AMPKɑ2 gene (p < 0.05).
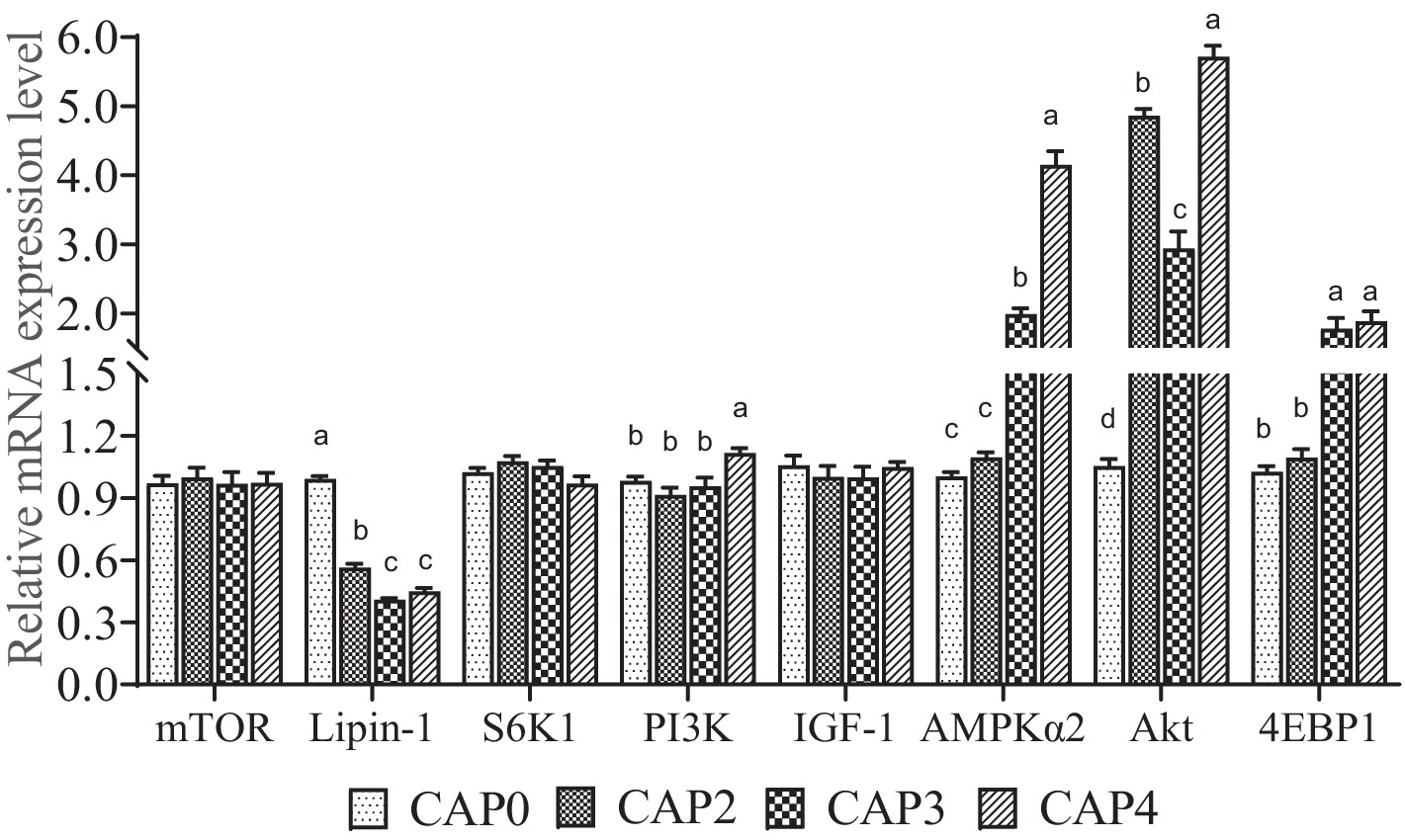
Figure 1. Effects of CAP on the expression pattern of key genes of the mTOR pathway in breast muscle. Data are presented as means and standard errors (±SE) (n = 6).
The mRNA levels of genes related to the mTOR signaling pathway in the liver are shown in Figure 2. Broiler-fed diets CAP3 and CAP4 showed a higher mRNA level of mTOR than broiler-fed diets CAP0 and CAP2 (p < 0.05). Moreover, the CAP3 group was higher than the CAP4 (p < 0.05). The mRNA level of the Lipin-1 in the CAP0 group was higher than the other groups (p < 0.05), and the CAP4 group had significantly higher expression of AMPKɑ2 than the other groups (p < 0.05), which is consistent with the trend in the chest muscle. Akt relative mRNA expression level was higher in the groups CAP2 and CAP4 compared with the CAP0 and CAP3 groups (p < 0.05). 4E-BP1 expressions increased in treatment groups compared with the control group, and the CAP3 group was higher than the other groups (p < 0.05).
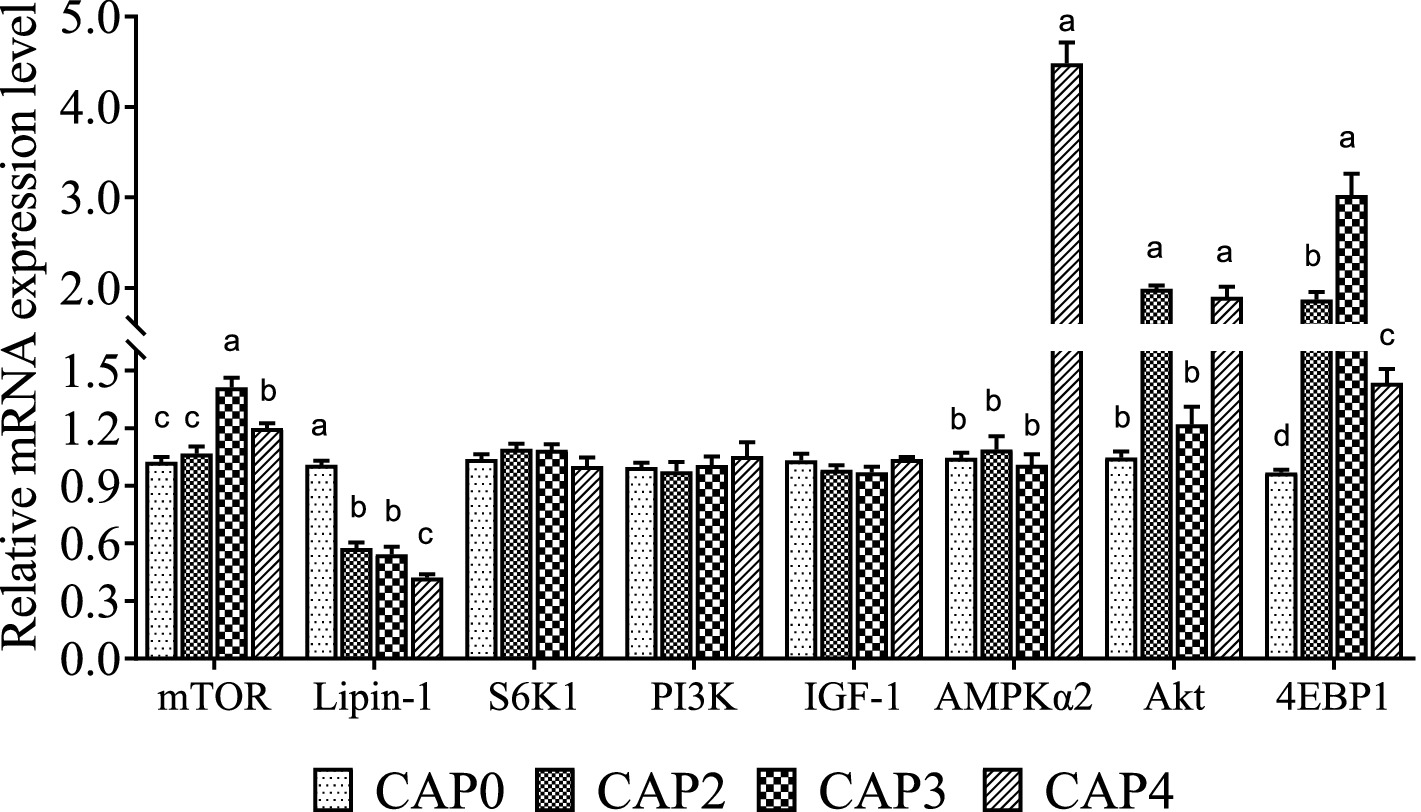
Figure 2. Effects of CAP on the expression pattern of key genes of the mTOR pathway in the liver. Data are presented as means and standard errors (±SE) (n = 6). Significant differences (p < 0.05) are indicated by different alphabetical superscripts above the bars, while the same letter indicates no difference.
Protein expression associated with mammalian targets of the mTOR signaling pathway in breast muscle and liver
The present study evaluated the protein phosphorylation of key regulators in the mTOR signaling pathway in the breast muscle (Figure 3). The phosphorylation levels of 4E-BP1 and Akt showed the same trend and were higher in the CAP3 group than in the other groups (p < 0.05). Phosphorylation levels of AMPKɑ2 were higher in the CAP2 and CAP3 groups compared to the control group (p < 0.05).
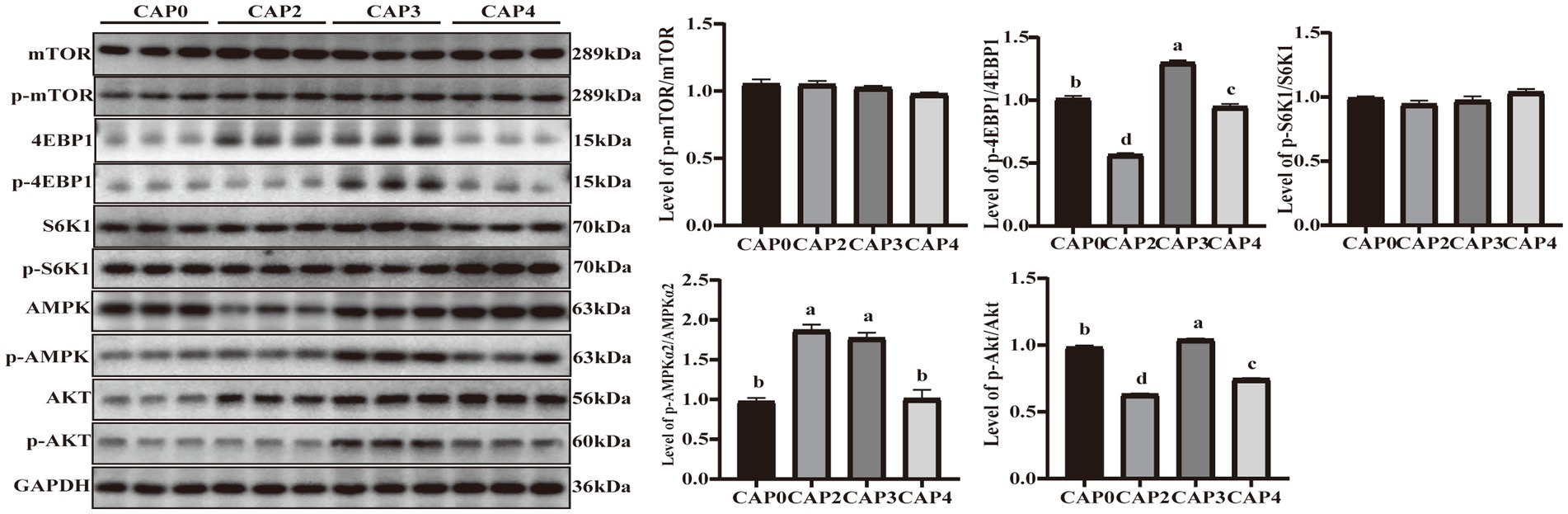
Figure 3. Protein expression levels and phosphorylation levels of some selected breast muscle genes involved in protein synthesis and energy metabolism in broilers fed CAP diets. Data are presented as means and standard errors (±SE) (n = 6). Significant differences (p < 0.05) are indicated by different alphabetical superscripts above the bars, while the same letter indicates no difference.
Figure 4 presents the protein phosphorylation level of key regulators in mTOR signaling compared between the different treatments in the liver. The mTOR, 4EBP1, and S6K1 phosphorylation levels in the CAP3 and CAP4 groups were higher than the CAP0 and CAP2 groups (p < 0.05). The phosphorylation level of AMPKɑ2 was higher in the CAP2 group than in the other groups (p < 0.05).
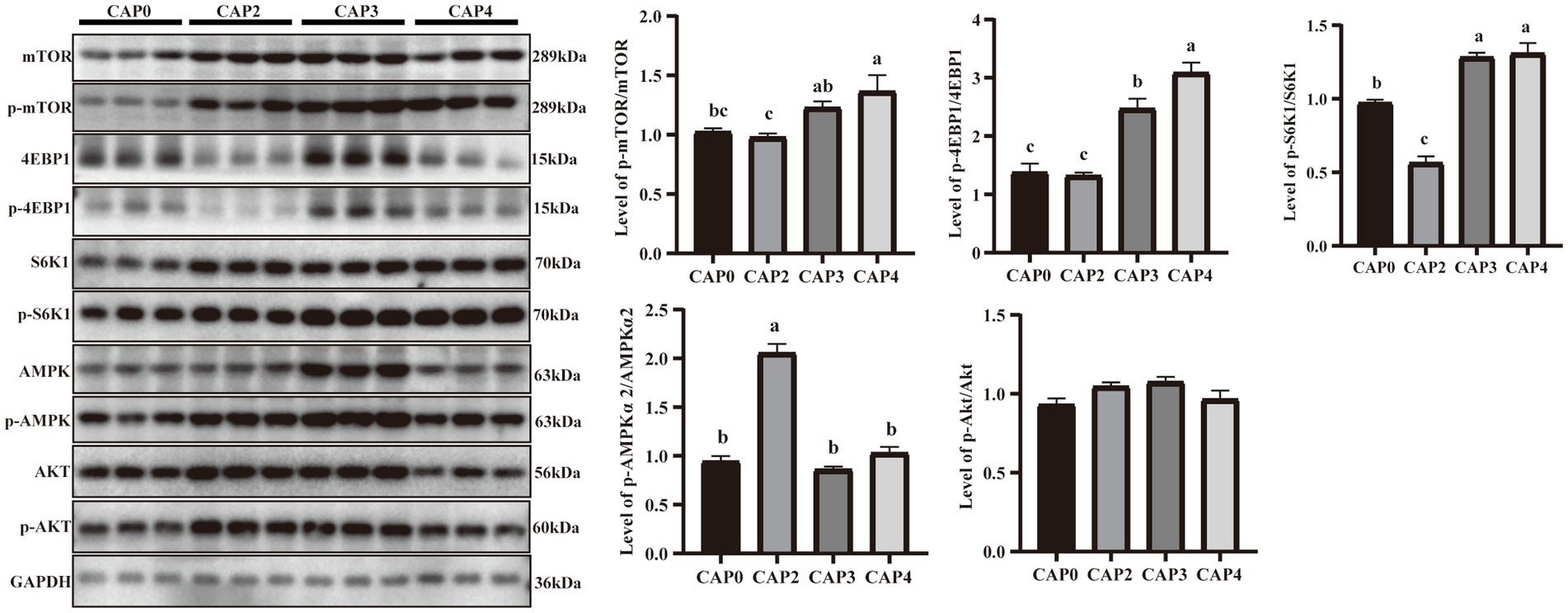
Figure 4. Protein expression levels and phosphorylation levels of some selected liver genes involved in protein synthesis and energy metabolism in broilers fed CAP diets. Data are presented as means and standard errors (±SE) (n = 6). Significant differences (p < 0.05) are indicated by different alphabetical superscripts above the bars, while the same letter indicates no difference.
Discussion
There is currently limited research on CAP in livestock and poultry, with most studies focusing on aquatic animals. Several studies have demonstrated that an appropriate proportion of CAP can enhance animal growth and development, improve production performance, and promote good health (26, 27, 30, 31). However, a high proportion of CAP substitution may lead to a decline in animal production performance (32, 33), as well as intestinal and liver damage, and increased mortality (15). Wu et al. (34) found that adding the proper amount of CAP in broiler diets improved growth rate and feed conversion efficiency, which is consistent with our findings. Therefore, the function of CAP may be related to the ease of digestion (15, 34).
Assessing the slaughtering performance is crucial in determining the quality of livestock and poultry carcasses. Therefore, it is important to consider the breast and leg muscle yields as they are crucial indicators of meat production performance. Previous research has shown that supplying an appropriate level of SCP in broiler diets can improve carcass quality and increase breast and leg muscle yields (14), which is consistent with the results of this study. Another study reported that the supplement of different proportions of SCP in broiler diets did not affect the dressing percentage, which aligns with the findings of the present study (35). According to the study, including CAP in the diet of broilers increased the CP content in muscle, suggesting an acceleration of protein synthesis and deposition. These findings are consistent with previous studies conducted by Xue et al. (28) and Wu et al. (10) in aquatic animals.
Serum biochemical indicators change according to the body’s health and metabolic status. Measuring these indicators can visually reflect the body’s health, nutrition, and metabolic status. TP reflects the state of protein absorption and synthesis, and high TP content indicates vigorous protein metabolism, which can enhance the body’s immune function and promote growth and development (36, 37). ALB plays a crucial role in stabilizing blood osmolality and transporting metabolic substances (38). UA is a protein metabolite produced mainly in the liver and excreted through the kidneys and intestines. Hyperuricemia and gout can be caused by overproduction or reduced excretion of UA (39). Previous study has shown that the inclusion of CAP in diets can increase TP and ALB levels, and UA levels also tend to increase with increasing CAP content (34), which is consistent with the results of the present study. TC and TG are the main lipids in the blood and important indicators of lipid absorption, metabolism, and transport (40, 41). In the present study, CAP had a significant effect on the lipid metabolism of broilers, as evidenced by the decrease in TG levels. This finding is consistent with the results reported by Wu et al. (34) but differs from those observed in aquatic animals (11, 27), which may be attributed to species differences.
AST and ALT are often used as indicators of liver injury (42). ALP acts as a dephosphorylator and has an important biological function, commonly used to assess liver injury and disease (43–45). It is worth noting that ALP levels increase during skeletal development when the organism is growing rapidly (46). In the present study, AST and ALP tended to increase when adding CAP to the diet. It was suggested that CAP may cause some degree of damage to liver function while promoting rapid growth of the organism. Consistent with the results of the present study, previous studies have shown an increasing trend in AST with increasing CAP (15, 33). Meanwhile, Maulu et al. (31) showed that 5 and 10% CAP increased ALP activity, which is similar to the results of the present study.
SOD reduces oxidative damage by scavenging free radicals and generating oxygen molecules and hydrogen peroxide. T-AOC reflects the body’s total antioxidant capacity, and GSH-Px converts harmful peroxides into harmless hydroxyl compounds, protecting the normal structure and function of cell membranes (47). In the present study, including CAP in the diet increased GSH-Px and SOD activities, as well as T-AOC, indicating that the appropriate proportion of CAP can enhance the organism’s antioxidant capacity. This finding is consistent with previous studies that have shown CAP’s ability to increase antioxidant enzyme activities and improve the organism’s antioxidant capacity (30, 31, 34). Conversely, a high proportion of CAP may impair the organism’s antioxidant defense system, leading to oxidative damage (11, 17). Therefore, we speculate that this situation could be due to substitution ratio, species differences, and the absence of specific nutrients required by certain animals in CAP. For instance, it was reported that taurine deficiency results in elevated levels of oxidative damage in fish, which has a detrimental impact on health and economic performance. (48).
IgG is the main Ig in the organism, accounting for about 75% of the total amount of serum Ig (49), and IgA is the second most abundant, accounting for 10–20% of the total serum Ig (50). Previous studies have shown that CAP can upregulate immune-related indicators and enhance immune function, which is consistent with the results of the present study (18, 26, 31). IL-1, IL-6, and IL-8 are members of the interleukin family and play a crucial role in regulating inflammation and immune responses in the body (51, 52). Maulu et al. (31) reported that CAP did not have any adverse effects on the regulation of inflammation and immune responses, as IL-8 levels were not significantly different from the control group. Similarly, the present study found no significant differences in IL-8 levels among the groups, indicating that CAP did not cause an inflammatory response in the body.
The mTOR pathway integrates various external signals from energy, amino acids, inflammatory factors, and hormones, with a vital function in nutrient sensing and regulation (53). Additionally, it plays a significant role in protein deposition, growth and development, and metabolic regulation in the body (54). Animal weight gain is primarily due to the deposition of muscle and fat, wherein muscle deposition primarily depends on protein synthesis, and accelerating protein synthesis can promote rapid organism growth (10, 55). Numerous studies have demonstrated that the genes mTOR, 4E-BP1, and S6K were associated with protein synthesis. Activation of mTOR was typically accompanied by changes in the expression of 4E-BP1 and S6K genes, but 4E-BP1 plays a more significant role in protein synthesis than S6K (10, 27, 56, 57).
Previous studies have shown that an appropriate proportion of CAP can enhance the expression of genes associated with the mTOR signaling pathway, stimulate muscle protein synthesis, and enhance the organism’s growth performance (10, 27, 28). Furthermore, Li et al. (26) also found that the dietary inclusion of CAP improved the expression levels of genes related to growth, antioxidants, and the TOR pathway. The present study showed that the Lipin-1 gene was down-regulated, and the AMPKɑ2, Akt, and 4E-BP1 genes were up-regulated compared to the control group, suggesting that the appropriate proportion of CAP activates the mTOR signaling pathway and promotes the organism’s growth and development, which is consistent with previous studies.
The phosphorylation levels of S6K1 and 4E-BP1 are typically indicators of the degree of activation of the mTOR pathway, and activation of the mTOR pathway can promote cell proliferation and growth (56, 58, 59). In the present study, the CAP3 group significantly increased the levels of phosphorylation of 4E-BP1, S6K1, Akt, and AMPKɑ2, which provides further evidence at the protein expression level that a suitable proportion of CAP can activate the mTOR signaling pathway and regulate organism growth. Consistent with the results of this study, Maulu et al. (27) reported that the phosphorylation levels of 4E-BP1, S6K1, and AMPK significantly increased by 5 and 10% CAP. However, in contrast to the results of our study, some researchers found that adding CAP to the diet inhibits the mTOR pathway and negatively affects the organism’s growth and development (60, 61). The discrepancies in these studies may be due to species specificity and variations in the amount of substitution, and further investigation is needed to determine the exact reasons.
The activation of the mTOR pathway closely links to amino acid (AA) levels. It has been proven that AAs, particularly branched-chain amino acids (BCAAs), regulate protein synthesis through the mTOR pathway by controlling S6K1 and 4E-BP1 activities (62, 63). Leucine, the primary BCAA that regulates protein synthesis, accelerates protein synthesis in the organism by activating the mTOR pathway, thereby promoting growth (20, 64, 65). Therefore, we speculate that the growth-promoting effect of CAP may be related to its high AA content and digestibility (15, 66). However, it is worth noting that CAP has a low content of arginine and a high content of nucleic acids. Arginine is an important regulator of the mTOR pathway, and a lack of arginine leads to a decrease in protein synthesis, which may explain why a high proportion of CAP negatively affects organismal growth and development.
Conclusion
In this study, adding 3% CAP to broiler diets reduced the FCR (22–42 d) and increased the breast and leg muscle yields as well as the CP content of chicken meat. Adding 3% CAP to broiler diets also improved the antioxidant properties (T-AOC, GSH-Px, and SOD) and enhanced the IgG of the organism. In addition, the inclusion of 3% CAP to the diet activated the mTOR pathway and increased the levels of 4E-BP1, S6K1, Akt, and AMPKɑ2 phosphorylation. Notably, the addition of 4% CAP to the diet increased serum UA, AST, and ALP. Based on the above results, our recommended use level in broiler diets is 3%.
Data availability statement
The datasets presented in this study can be found in online repositories. The names of the repository/repositories and accession number(s) can be found in the article/Supplementary material.
Ethics statement
The animal studies were approved by Laboratory Animal Welfare Guidelines of China. The studies were conducted in accordance with the local legislation and institutional requirements. Written informed consent was obtained from the owners for the participation of their animals in this study.
Author contributions
CS: Writing – original draft, Visualization, Validation, Methodology, Investigation, Formal analysis, Data curation, Conceptualization. YL: Writing – original draft, Validation, Software, Methodology, Formal analysis, Conceptualization. CM: Writing – original draft, Validation, Resources, Methodology. CL: Writing – original draft, Software, Resources, Methodology. QL: Writing – original draft, Validation, Resources, Methodology, Investigation. SL: Writing – original draft, Software, Methodology, Formal analysis, Data curation. GJ: Writing – review & editing, Visualization, Validation, Supervision, Project administration, Funding acquisition, Conceptualization. JT: Writing – review & editing, Visualization, Validation, Supervision, Project administration, Funding acquisition, Conceptualization.
Funding
The author(s) declare financial support was received for the research, authorship, and/or publication of this article. This research work was supported by Liaoning Province “Xingliao Talents Plan” project (Project No. XLYC1803014).
Conflict of interest
YL, QL, and GJ were employed by Dalian Sanyi Animal Medicine Co., Ltd. CM and CL were employed by Jiangsu Sanyi Animal Nutrition Technology Co., Ltd.
The remaining authors declare that the research was conducted in the absence of any commercial or financial relationships that could be construed as a potential conflict of interest.
Publisher’s note
All claims expressed in this article are solely those of the authors and do not necessarily represent those of their affiliated organizations, or those of the publisher, the editors and the reviewers. Any product that may be evaluated in this article, or claim that may be made by its manufacturer, is not guaranteed or endorsed by the publisher.
References
1. Gaffney, J, Challender, M, Califf, K, and Harden, K. Building bridges between agribusiness innovation and smallholder farmers: a review. Glob Food Sec. (2019) 20:60–5. doi: 10.1016/j.gfs.2018.12.008
2. Bastawrous, A, and Suni, A-V. Thirty year projected magnitude (to 2050) of near and distance vision impairment and the economic impact if existing solutions are implemented globally. Ophthalmic Epidemiol. (2020) 27:115–20. doi: 10.1080/09286586.2019.1700532
3. Boland, MJ, Rae, AN, Vereijken, JM, Meuwissen, MP, Fischer, AR, van Boekel, MA, et al. The future supply of animal-derived protein for human consumption. Trends Food Sci Technol. (2013) 29:62–73. doi: 10.1016/j.tifs.2012.07.002
4. Woyengo, T, Knudsen, KB, and Børsting, C. Low-protein diets for broilers: current knowledge and potential strategies to improve performance and health, and to reduce environmental impact. Anim Feed Sci Technol. (2023) 115574. doi: 10.1016/j.anifeedsci.2023.115574
5. Honeyman, M. Sustainability issues of US swine production. J Anim Sci. (1996) 74:1410–7. doi: 10.2527/1996.7461410x
6. Van Harn, J, Dijkslag, M, and Van Krimpen, M. Effect of low protein diets supplemented with free amino acids on growth performance, slaughter yield, litter quality, and footpad lesions of male broilers. Poult Sci. (2019) 98:4868–77. doi: 10.3382/ps/pez229
7. Attia, YA, Bovera, F, Wang, J, Al-Harthi, MA, and Kim, WK. Multiple amino acid supplementations to low-protein diets: effect on performance, carcass yield, meat quality and nitrogen excretion of finishing broilers under hot climate conditions. Animals. (2020) 10:973. doi: 10.3390/ani10060973
8. Wang, Y, Zhou, J, Wang, G, Cai, S, Zeng, X, and Qiao, S. Advances in low-protein diets for swine. J Anim Sci Biotechnol. (2018) 9:1–14. doi: 10.1186/s40104-018-0276-7
9. Bratosin, BC, Darjan, S, and Vodnar, DC. Single cell protein: a potential substitute in human and animal nutrition. Sustain For. (2021) 13:9284. doi: 10.3390/su13169284
10. Wu, Z, Yu, X, Guo, J, Fu, Y, Guo, Y, Pan, M, et al. Replacement of dietary fish meal with Clostridium autoethanogenum protein on growth performance, digestion, mTOR pathways and muscle quality of abalone Haliotis discus hannai. Aquaculture. (2022) 553:738070. doi: 10.1016/j.aquaculture.2022.738070
11. Chen, Y, Sagada, G, Xu, B, Chao, W, Zou, F, Ng, WK, et al. Partial replacement of fishmeal with Clostridium autoethanogenum single-cell protein in the diet for juvenile black sea bream (Acanthopagrus schlegelii). Aquac Res. (2020) 51:1000–11. doi: 10.1111/are.14446
12. Saied, J, Al-Jabary, Q, and Thalij, K. Effect of dietary supplement yeast culture on production performance and hematological parameters in broiler chicks. Int J Poult Sci. (2011) 10:376–80. doi: 10.3923/ijps.2011.376.380
13. El-Naga, M. Effect of dietary yeast supplementation on broiler performance. Egypt Poult Sci J. (2012) 32:95–106.
14. Hombegowda, GP, Suresh, BN, Shivakumar, MC, Ravikumar, P, Girish, BC, Rudrappa, SM, et al. Growth performance, carcass traits and gut health of broiler chickens fed diets incorporated with single cell protein. Anim Biosci. (2021) 34:1951–62. doi: 10.5713/ab.20.0844
15. Wei, H, Yu, H, Chen, X, Chao, W, Zou, F, Chen, P, et al. Effects of soybean meal replaced by Clostridium autoethanogenum protein on growth performance, plasma biochemical indexes and hepatopancreas and intestinal histopathology of grass carp (Ctenopharyngodon idllus). Chin J Anim Nutr. (2018) 30:4190–01. Available at: https://kns.cnki.net/kcms/detail/11.5461.S.20180731.1602.028.html
16. Norman, RO, Millat, T, Winzer, K, Minton, NP, and Hodgman, C. Progress towards platform chemical production using Clostridium autoethanogenum. Biochem Soc Trans. (2018) 46:523–35. doi: 10.1042/BST20170259
17. Yang, P, Li, X, Song, B, He, M, Wu, C, and Leng, X. The potential of Clostridium autoethanogenum, a new single cell protein, in substituting fish meal in the diet of largemouth bass (Micropterus salmoides): growth, feed utilization and intestinal histology. Aquac Fisher. (2023) 8:67–75. doi: 10.1016/j.aaf.2021.03.003
18. Ma, S, Liang, X, Chen, P, Wang, J, Gu, X, Qin, Y, et al. A new single-cell protein from Clostridium autoethanogenum as a functional protein for largemouth bass (Micropterus salmoides). Anim Nutr. (2022) 10:99–10. doi: 10.1016/j.aninu.2022.04.005
19. Zhou, Y-M, Liu, Y, Liu, W, and Shen, Y. Generation of microbial protein feed (MPF) from waste and its application in aquaculture in China. J Environ Chem Eng. (2023) 11:109297. doi: 10.1016/j.jece.2023.109297
20. Murgas Torrazza, R, Suryawan, A, Gazzaneo, MC, Orellana, RA, Frank, JW, Nguyen, HV, et al. Leucine supplementation of a low-protein meal increases skeletal muscle and visceral tissue protein synthesis in neonatal pigs by stimulating mTOR-dependent translation initiation. J Nutr. (2010) 140:2145–52. doi: 10.3945/jn.110.128421
21. Yao, K, Yin, Y-L, Chu, W, Liu, Z, Deng, D, Li, T, et al. Dietary arginine supplementation increases mTOR signaling activity in skeletal muscle of neonatal pigs. J Nutr. (2008) 138:867–72. doi: 10.1093/jn/138.5.867
22. Zhang, X, Zhao, F, Si, Y, Huang, Y, Yu, C, Luo, C, et al. GSK3β regulates milk synthesis in and proliferation of dairy cow mammary epithelial cells via the mTOR/S6K1 signaling pathway. Molecules. (2014) 19:9435–52. doi: 10.3390/molecules19079435
23. Dancey, J. mTOR signaling and drug development in cancer. Nat Rev Clin Oncol. (2010) 7:209–19. doi: 10.1038/nrclinonc.2010.21
24. Gundermann, DM, Walker, DK, Reidy, PT, Borack, MS, Dickinson, JM, Volpi, E, et al. Activation of mTORC1 signaling and protein synthesis in human muscle following blood flow restriction exercise is inhibited by rapamycin. Am J Physiol Endocrinol Metab. (2014) 306:E1198–204. doi: 10.1152/ajpendo.00600.2013
25. Laplante, M, and Sabatini, DM. mTOR signaling at a glance. J Cell Sci. (2009) 122:3589–94. doi: 10.1242/jcs.051011
26. Li, M, Liang, H, Xie, J, Chao, W, Zou, F, Ge, X, et al. Diet supplemented with a novel Clostridium autoethanogenum protein have a positive effect on the growth performance, antioxidant status and immunity in juvenile Jian carp (Cyprinus carpio var Jian). Aquac Rep. (2021) 19:100572. doi: 10.1016/j.aqrep.2020.100572
27. Maulu, S, Liang, H, Ge, X, Yu, H, Huang, D, Ke, J, et al. Effect of dietary Clostridium autoethanogenum protein on growth, body composition, plasma parameters and hepatic genes expression related to growth and AMPK/TOR/PI3K signaling pathway of the genetically improved farmed tilapia (GIFT: Oreochromis niloticus) juveniles. Anim Feed Sci Technol. (2021) 276:114914. doi: 10.1016/j.anifeedsci.2021.114914
28. Xue, R, Li, H, Liu, S, Hu, Z, Wu, Q, and Ji, H. Substitution of soybean meal with Clostridium autoethanogenum protein in grass carp (Ctenopharygodon idella) diets: effects on growth performance, feed utilization, muscle nutritional value and sensory characteristics. Anim Feed Sci Technol. (2023) 295:115547. doi: 10.1016/j.anifeedsci.2022.115547
29. AOAC. Official Methods of Analysis of AOAC International. Association of the Official Analytical Chemists (AOAC) International. MD Gaithersburg, 17th ed. Rockville: AOAC. (2003).
30. Li, L, Liu, X, Wang, Y, Huang, Y, and Wang, C. Effects of alternate feeding between fish meal and novel protein diets on the intestinal health of juvenile largemouth bass (Micropterus salmoides). Aquac Rep. (2022) 23:101023. doi: 10.1016/j.aqrep.2022.101023
31. Maulu, S, Hualiang, L, Ke, J, Ren, M, Ge, X, Huang, D, et al. Dietary Clostridium autoethanogenum protein modulates intestinal absorption, antioxidant status, and immune response in GIFT (Oreochromis niloticus) juveniles. Aquac Res. (2021) 52:5787–99. doi: 10.1111/are.15454
32. Yao, W, Yang, P, Zhang, X, Xu, X, Zhang, C, Li, X, et al. Effects of replacing dietary fish meal with Clostridium autoethanogenum protein on growth and flesh quality of Pacific white shrimp (Litopenaeus vannamei). Aquaculture. (2022) 549:737770. doi: 10.1016/j.aquaculture.2021.737770
33. Jiang, X, Yao, W, Yang, H, Tan, S, Leng, X, and Li, X. Dietary effects of Clostridium autoethanogenum protein substituting fish meal on growth, intestinal histology and immunity of Pacific white shrimp (Litopenaeus vannamei) based on transcriptome analysis. Fish Shellfish Immunol. (2021) 119:635–44. doi: 10.1016/j.fsi.2021.10.005
34. Wu, Y, Wang, J, Jia, M, Huang, S, Cao, Y, Yao, T, et al. Clostridium autoethanogenum protein inclusion in the diet for broiler: enhancement of growth performance, lipid metabolism, and gut microbiota. Front Vet Sci. (2022) 9:1028792. doi: 10.3389/fvets.2022.1028792
35. Najib, H. Feeding value of single cell protein produced from date palm (Phoenix dactylifera) fruits for broiler chickens. Ann Res Rev Biol. (2014) 4:2406–13. doi: 10.9734/ARRB/2014/9524
36. Li, H-H, Jiang, X-R, and Qiao, J-Y. Effect of dietary Bacillus subtilis on growth performance and serum biochemical and immune indexes in weaned piglets. J Appl Anim Res. (2021) 49:83–8. doi: 10.1080/09712119.2021.1877717
37. Torres-Pitarch, A, Hermans, D, Manzanilla, EG, Bindelle, J, Everaert, N, Beckers, Y, et al. Effect of feed enzymes on digestibility and growth in weaned pigs: a systematic review and meta-analysis. Anim Feed Sci Technol. (2017) 233:145–59. doi: 10.1016/j.anifeedsci.2017.04.024
38. Aldecoa, C, Llau, JV, Nuvials, X, and Artigas, A. Role of albumin in the preservation of endothelial glycocalyx integrity and the microcirculation: a review. Ann Intens Care. (2020) 10:1–12. doi: 10.1186/s13613-020-00697-1
39. Zou, F, Zhao, X, and Wang, F. A review on the fruit components affecting uric acid level and their underlying mechanisms. J Food Biochem. (2021) 45:e13911. doi: 10.1111/jfbc.13911
40. Li, Y, Li, W, Luo, L, Ren, Y, Xing, W, Xu, G, et al. Dietary lipid levels affect growth performance, lipid metabolism, antioxidant and immune status of Amur sturgeon Acipenser schrenckii. Aquac Rep. (2023) 33:101796. doi: 10.1016/j.aqrep.2023.101796
41. Pan, S, Yan, X, Dong, X, Li, T, Suo, X, Liu, H, et al. Influence of dietary inositol supplementation on growth, liver histology, lipid metabolism, and related genes expression on juvenile hybrid grouper (♀ epinephelus fuscoguttatus×♂ e. lanceolatu) fed high-lipid diets. Aquac Nutr. (2022) 1–13. doi: 10.1155/2022/6743690
42. Amirabagya, F, Hapsari, RAF, and Wulandari, E. The effect of Jatropha curcas L seed extract on AST/ALT activity and the central vein thickness in liver. Pharm J. (2021) 13:66–72. doi: 10.5530/pj.2021.13.10
43. Tang, Z, Chen, H, He, H, and Ma, C. Assays for alkaline phosphatase activity: Progress and prospects. TrAC Trends Anal Chem. (2019) 113:32–43. doi: 10.1016/j.trac.2019.01.019
44. Jie, X, Wu, M, Yang, H, and Wei, W. Red–near-infrared fluorescent probe for time-resolved in vivo alkaline phosphatase detection with the assistance of a Photoresponsive Nanocontainer. Anal Chem. (2019) 91:13174–82. doi: 10.1021/acs.analchem.9b03497
45. Zhang, P, Fu, C, Zhang, Q, Li, S, and Ding, C. Ratiometric fluorescent strategy for localizing alkaline phosphatase activity in mitochondria based on the ESIPT process. Anal Chem. (2019) 91:12377–83. doi: 10.1021/acs.analchem.9b02917
46. Wang, K, Wang, W, Zhang, X-Y, Jiang, A-Q, Yang, Y-S, and Zhu, H-L. Fluorescent probes for the detection of alkaline phosphatase in biological systems: recent advances and future prospects. TrAC Trends Anal Chem. (2021) 136:116189. doi: 10.1016/j.trac.2021.116189
47. Benhar, M. Roles of mammalian glutathione peroxidase and thioredoxin reductase enzymes in the cellular response to nitrosative stress. Free Radic Biol Med. (2018) 127:160–4. doi: 10.1016/j.freeradbiomed.2018.01.028
48. Ma, Q-W, Guo, H-Y, Zhu, K-C, Guo, L, Liu, B-S, Zhang, N, et al. Dietary taurine intake affects growth and taurine synthesis regulation in golden pompano, Trachinotus ovatus (Linnaeus 1758). Aquaculture. (2021) 530:735918. doi: 10.1016/j.aquaculture.2020.735918
49. Muhammed, Y. The best IgG subclass for the development of therapeutic monoclonal antibody drugs and their commercial production: a review. Immunome Res. (2020) 16:1–12. doi: 10.35248/1745-7580.20.16.173
50. Chen, K, Magri, G, Grasset, EK, and Cerutti, A. Rethinking mucosal antibody responses: IgM, IgG and IgD join IgA. Nat Rev Immunol. (2020) 20:427–41. doi: 10.1038/s41577-019-0261-1
51. Bouquet, M, Passmore, MR, Hoe, LES, Tung, J-P, Simonova, G, Boon, A-C, et al. Development and validation of ELISAs for the quantitation of interleukin (IL)-1β, IL-6, IL-8 and IL-10 in ovine plasma. J Immunol Methods. (2020) 486:112835. doi: 10.1016/j.jim.2020.112835
52. Hao, G, Duan, W, Sun, J, Liu, J, and Peng, B. Effects of febuxostat on serum cytokines IL-1, IL-4, IL-6, IL-8, TNF-α and COX-2. Exp Ther Med. (2019) 17:812–6. doi: 10.3892/etm.2018.6972
53. Tang, Z-L, Zhang, K, Lv, S-C, Xu, G-W, Zhang, J-F, and Jia, H-Y. LncRNA MEG3 suppresses PI3K/AKT/mTOR signalling pathway to enhance autophagy and inhibit inflammation in TNF-α-treated keratinocytes and psoriatic mice. Cytokine. (2021) 148:155657. doi: 10.1016/j.cyto.2021.155657
54. Sabatini, DM. Twenty-five years of mTOR: uncovering the link from nutrients to growth. Proc Natl Acad Sci. (2017) 114:11818–25. doi: 10.1073/pnas.1716173114
55. Goldspink, D. The influence of immobilization and stretch on protein turnover of rat skeletal muscle. J Physiol. (1977) 264:267–82. doi: 10.1113/jphysiol.1977.sp011667
56. Liu, GY, and Sabatini, DM. mTOR at the nexus of nutrition, growth, ageing and disease. Nat Rev Mol Cell Biol. (2020) 21:183–03. doi: 10.1038/s41580-019-0199-y
57. She, Y, Deng, H, Cai, H, and Liu, G. Regulation of the expression of key signalling molecules in mTOR pathway of skeletal muscle satellite cells in neonatal chicks: effects of leucine and glycine–leucine peptide. J Anim Physiol Anim Nutr. (2019) 103:786–90. doi: 10.1111/jpn.13090
58. Zhong, H, Yuan, P, Li, Y, Batonon-Alavo, D, Deschamps, C, Feng, B, et al. Methionine protects mammary cells against oxidative stress through producing S-Adenosylmethionine to maintain mTORC1 signaling activity. Oxidative Med Cell Longev. (2021) 2021:5550196. doi: 10.1155/2021/5550196
59. Batool, A, Majeed, ST, Aashaq, S, Majeed, R, Shah, G, Nazir, N, et al. Eukaryotic initiation factor 4E (eIF4E) sequestration mediates 4E-BP1 response to rapamycin. Int J Biol Macromol. (2019) 125:651–9. doi: 10.1016/j.ijbiomac.2018.12.102
60. Huang, B, Shi, M, Pang, A, Tan, B, and Xie, S. Effects of fishmeal replacement by Clostridium Autoethanogenum protein meal on cholesterol bile acid metabolism, antioxidant capacity, hepatic and intestinal health of pearl gentian grouper (Epinephelus Fuscoguttatus♀× Epinephelus Lanceolatus♂). Animals. (2023) 13:1090. doi: 10.3390/ani13061090
61. Fan, Z, Li, C, Wu, D, Li, J, Wang, L, Cao, D, et al. Evaluation of four novel protein sources as alternatives to soybean meal for two specifications of cage-farmed grass carp (Ctenopharyngodon idellus) deeds: effect on growth performance, flesh quality, and expressions of muscle-related genes. Front Mar Sci. (2022) 9:935651. doi: 10.3389/fmars.2022.935651
62. Komorowski, JR, Ojalvo, SP, Sylla, S, Tastan, H, Orhan, C, Tuzcu, M, et al. The addition of an amylopectin/chromium complex to branched-chain amino acids enhances muscle protein synthesis in rat skeletal muscle. J Int Soc Sports Nutr. (2020) 17:26. doi: 10.1186/s12970-020-00355-8
63. Rezaei, R, and Wu, G. Branched-chain amino acids regulate intracellular protein turnover in porcine mammary epithelial cells. Amino Acids. (2022) 54:1491–04. doi: 10.1007/s00726-022-03203-y
64. Columbus, DA, Fiorotto, ML, and Davis, TA. Leucine is a major regulator of muscle protein synthesis in neonates. Amino Acids. (2015) 47:259–70. doi: 10.1007/s00726-014-1866-0
65. Zou, T, Cao, SP, Xu, WJ, Han, D, Liu, HK, Yang, YX, et al. Effects of dietary leucine levels on growth, tissue protein content and relative expression of genes related to protein synthesis in juvenile gibel carp (Carassius auratus gibelio var. CAS III). Aquac Res. (2018) 49:2240–8. doi: 10.1111/are.13682
Keywords: Clostridium autoethanogenum protein, broiler, growth performance, mTOR, antioxidant activity, immune function
Citation: Shan C, Liu Y, Ma C, Li C, Liu Q, Liu S, Jiang G and Tian J (2024) Dietary supplementation with Clostridium autoethanogenum protein improves growth performance and promotes muscle protein synthesis by activating the mTOR signaling pathway of the broiler. Front. Vet. Sci. 11:1389738. doi: 10.3389/fvets.2024.1389738
Edited by:
Yangchun Cao, Northwest A&F University, ChinaReviewed by:
Sabreen Ezzat Fadl, Matrouh University, EgyptWeiwei Wang, Academy of National Food and Strategic Reserves Administration, China
Copyright © 2024 Shan, Liu, Ma, Li, Liu, Liu, Jiang and Tian. This is an open-access article distributed under the terms of the Creative Commons Attribution License (CC BY). The use, distribution or reproduction in other forums is permitted, provided the original author(s) and the copyright owner(s) are credited and that the original publication in this journal is cited, in accordance with accepted academic practice. No use, distribution or reproduction is permitted which does not comply with these terms.
*Correspondence: Guotuo Jiang, jgt600@126.com; Jing Tian, Tianjing@dlpu.edu.cn
†These authors have contributed equally to this work and share first authorship