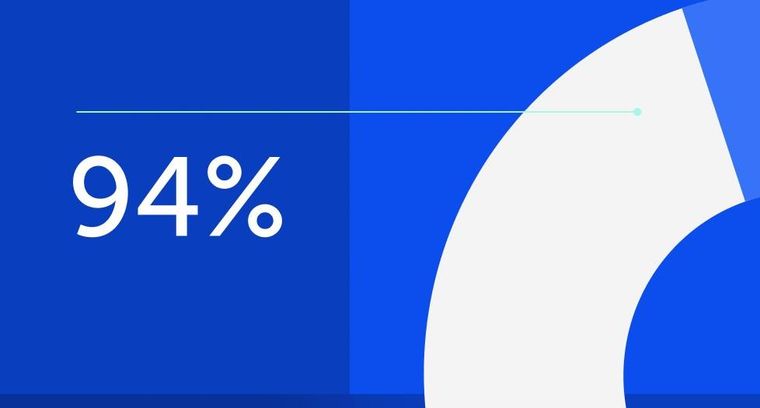
94% of researchers rate our articles as excellent or good
Learn more about the work of our research integrity team to safeguard the quality of each article we publish.
Find out more
ORIGINAL RESEARCH article
Front. Vet. Sci., 17 June 2024
Sec. Animal Reproduction - Theriogenology
Volume 11 - 2024 | https://doi.org/10.3389/fvets.2024.1389070
This article is part of the Research TopicUnraveling inflammaging : A pathway to Prevent Age-related Disease in AnimalsView all 11 articles
Introduction: Post-ovulatory aging is a time-dependent deterioration of ovulated oocytes and a major limiting factor reducing the fitness of offspring. This process may lead to the activation of cell death pathways like apoptosis in oocytes.
Methodology: We evaluated oocyte membrane integrity, egg developmental competency, and mRNA abundance of apoptosis-related genes by RT-qPCR. Oocytes from zebrafish Danio rerio were retained in vivo at 28.5°C for 24 h post-ovulation (HPO). Viability was assessed using trypan blue (TB) staining. The consequences of in vivo oocyte aging on the developmental competence of progeny were determined by the embryo survival at 24 h post fertilization, hatching, and larval malformation rates.
Results: The fertilization, oocyte viability, and hatching rates were 91, 97, and 65% at 0 HPO and dropped to 62, 90, and 22% at 4 HPO, respectively. The fertilizing ability was reduced to 2% at 8 HPO, while 72% of oocytes had still intact plasma membranes. Among the apoptotic genes bcl-2 (b-cell lymphoma 2), bada (bcl2-associated agonist of cell death a), cathepsin D, cathepsin Z, caspase 6a, caspase 7, caspase 8, caspase 9, apaf1, tp53 (tumor protein p53), cdk1 (cyclin-dependent kinase 1) studied, mRNA abundance of anti-apoptotic bcl-2 decreased and pro-apoptotic cathepsin D increased at 24 HPO. Furthermore, tp53 and cdk1 mRNA transcripts decreased at 24 HPO compared to 0 HPO.
Discussion: Thus, TB staining did not detect the loss of oocyte competency if caused by aging. TB staining, however, could be used as a simple and rapid method to evaluate the quality of zebrafish oocytes before fertilization. Taken together, our results indicate the activation of cell death pathways in the advanced stages of oocyte aging in zebrafish.
The fertilization rate and subsequent normal embryo and larval developmental stages can be used to define the quality of gametes (1). A delay in fertilization of ovulated oocytes leads to post-ovulatory aging or over-ripeness. Post-ovulatory aging is a factor that adversely affects the quality of oocytes and is complicated to manage. This aging process causes a loss of developmental competence of the egg, as well as deterioration, and may lead to oocyte death. Based on previous studies using murine, porcine and bovine models, oxidative stress (2, 3), mitochondrial dysfunction (4–6), degradation of maternal RNAs (7, 8), loss of survival factors (9, 10), epigenetic modifications (11, 12), and apoptosis (13–15) are probably involved in this process of oocyte aging. Mitochondrial microRNAs for cell death and signal transduction, stress response and DNA damage, RNA degradation, as well as energy and transcription regulation, were downregulated in rainbow trout (Oncorhynchus mykiss) due to post-ovulatory aging (16). However, oxidative stress does presumably not initiate oocyte aging in common carp (Cyprinus carpio) and goldfish (Carassius auratus) (17, 18). Histone acetylation pattern at histone H4 altered during the oocyte aging in common carp (19) indicating the involvement of epigenetic regulation. The first report of upregulation of apoptotic genes in aged oocytes from common carp speculated about the involvement of apoptosis in the degenerative process of fish oocytes (17).
The optimal time window for fertilization after ovulation highly varies among species and environmental conditions like temperature and storage (20). Several studies have been carried out to understand the in vitro and in vivo oocyte aging process using different model species. For example, the fertilization rate of in vivo aged curimata (Prochilodus marggravii) oocytes declined more prominently compared to in vitro aged at 26°C (21). The successful in vitro storage of Caspian brown trout (Salmo trutta caspius) oocytes was for 2 days at 2–3°C (22), whereas in vivo storage was for 30 days even at a higher temperature of 7°C (23). The most suitable duration for in vitro storage of rainbow trout oocytes was 9 days (24), and for in vivo storage was 30 days even at the same temperature, i.e., 2°C (25). The in vivo storage of northern pike (Esox lucius) oocytes retained the maximum fertilizing ability till 4 days post ovulation, whereas in vitro aging of 24 h resulted in a low fertilization rate at 10°C (26). In this study, we established a 24-h in vivo oocyte aging of zebrafish (Danio rerio) for the first time, as in vivo studies are important to understand the fate of oocytes retained inside the fish body after ovulation.
The underlying mechanisms of the degeneration process of post-ovulatory aged oocytes in fish remain elusive. Therefore, in this study, we used zebrafish as the experimental animal to investigate the decrease of trypan blue (TB) -based viability during in vivo aging of zebrafish oocytes and their quality before fertilization. Furthermore, the developmental competence was analyzed by estimating fertilization capability, embryo survival, hatching, and larval malformation rates after fertilization. Moreover, we analyzed the mRNA abundance of the pro-survival factor bcl-2 (b-cell lymphoma 2), cell cycle regulator cdk1 (cyclin-dependent kinase 1), and the main pro-apoptotic protein bada (bcl2-associated agonist of cell death a) during oocyte aging. The pro-apoptotic regulators like cathepsin D and cathepsin Z, tp53 (tumor protein p53), and apaf1 (apoptosis peptidase activating factor 1) mRNA abundance were also investigated. In addition, caspase 6a, caspase 7, caspase 8, and caspase 9 were also studied. The successful in vivo storage of the zebrafish AB strain oocytes was identified.
The zebrafish (AB strain) used in this study were initially obtained from the European Zebrafish Resource Centre (Karlsruhe, Germany) and later donated to the Faculty of Fisheries and Protection of Waters, the University of South Bohemia, Vodnany, Czech Republic. The fish were maintained in the ZebTec Active Blue rearing system (Techniplast, UK) under optimal photoperiod (14:10 h for light:dark cycle) and temperature (28.5°C). The broodstock was fed an adequate amount of Skretting M-300 Gemma Micro (Skretting, UK), according to the manufacturers’ recommendation. The females were bred at two-week intervals to maintain a fresh reproductive cycle. For this experiment, approximately 12-month-old fish were used. The males and females were prepared for gamete Research Topic based on the protocol by Westerfield (27). Briefly, males and females were separated overnight in 1 L spawning tanks by a glass partition in the 2:1 ratio. The spawning was induced by the light on the subsequent morning.
Ovulation was detected by observing the release of a few eggs by the female fish. After that, the females were anaesthetized with 0.05% tricaine methanesulfonate (methyl-aminobenzoate, MS222). The hand-stripping method was followed for the Research Topic of oocytes. A smidgen of oocytes (approx. 150 oocytes) was stripped from each of the six experimental females and considered as the fresh group or 0 h post-ovulation (HPO). The remaining oocytes were retained in the fish body for in vivo aging at 28.5°C. The cycle of collecting oocytes was continued at 2, 4, 8, and 24 HPO. Six individual females were used to strip at 0, 2 and 24 HPO, and another set of six females were used to strip at 0, 4 and 8 HPO since stripping the same females five times is unfeasible. The oocytes collected at each time point were divided into two different 35 mm petri dishes to assess viability loss using TB and for in vitro fertilization. The oocyte samples from 0, 8, and 24 HPOs were immediately frozen in liquid nitrogen and stored at −80°C till further use for total RNA extraction.
Trypan blue dye exclusion test was used to analyze the oocytes’ membrane integrity. The stock solution of 0.2% trypan blue (Sigma-Aldrich, Missouri, United States) was prepared in 1X Phosphate Buffered Saline (PBS, Sigma-Aldrich). 30–60 oocytes were stained with TB staining solution (0.1% in PBS) for 2 min at room temperature in a 35 mm petri dish immediately after stripping. Following staining, the oocytes were rinsed with PBS before visualization. TB stain, a large negatively charged molecule, is impermeable to cells with an intact plasma membrane and permeable to a cell with a disrupted membrane and enters the cytoplasm, making non-viable cells dark blue (28). The total and the viable number of oocytes were counted under a stereo microscope (Olympus SZX16, Olympus Life Science, Japan). The number of viable oocytes to the total number of oocytes used for staining was used to calculate the viability rates.
The males were anaesthetized in MS222 (0.05%), and milt was collected from them in the immobilizing solution (Kurokura 180) (29). While stripping the male fish, a 2.5 μL pipette was used to collect the milt simultaneously. The milt collected from 5 males were pooled together in 50 μL of Kurokura 180 solution. The milt was pooled to eliminate the effect of individual male sperm quality and to have an equal volume of sperm for fertilization of all females. The motility of pooled sperm was analyzed using an Olympus CX31 light microscope (Olympus Life Science, Japan) before fertilization, according to Fauvel et al. (30). The pooled milt showed >75% of active spermatozoa movement. The oocytes stripped at 0, 2, and 24 HPO from six individual females, and 0, 4, and 8 HPO from another set of six females were fertilized immediately. Fertilization was carried out by adding 200 μL of water and 10 μL of sperm to 50–90 oocytes, as the number varied at different time points of aging in a 35 mm glass petri plate. The eggs were thereafter transferred into a Pyrex® Petri plate (9 cm in diameter, Germany) (31). After 90 min of incubation at 28.5°C, the fertilization rate was assessed by examining the cleavage pattern of embryos. The eggs showing cleavage were counted under a Nikon SMZ745T stereo microscope (Nikon, Japan). The fertilization rate was defined by the number of eggs showing cleavage to the total number of oocytes inseminated.
Embryo survival, mortality, hatching, and malformation rates were calculated to determine the developmental competence of differently aged oocytes, according to Waghmare et al. (31). Briefly, the fertilized eggs were re-examined after 24 h of fertilization. The number of viable embryos after 24 h of fertilization to the total number of inseminated oocytes was used to determine the embryo survival rate. The successful development of fertilized eggs was examined by calculating embryo mortality (between 24 h and the hatching period) and hatching (48-72 h after fertilization) rates. The embryo mortality rate was determined by counting the viable embryos between 24 h and the hatching period to the total number of 24 h survived embryos. The number of hatched larvae to the total number of inseminated eggs was used to determine the hatching rate. After hatching, the larvae were observed under the Nikon SMZ745T stereomicroscope (Nikon, Japan) for the body shape. The larvae with body shape curved, bent, or curled were counted (72 h after fertilization) to the total number of hatched larvae for malformation rate.
Total RNA was isolated from 10 mg of oocytes sampled at 0, 8, and 24 HPOs from four individual females by the Trizol (Invitrogen, United States) method. The RNA concentration and quality were assessed using Nanodrop 2000 (Thermo Scientific, United States). The isolated RNA was treated with DNAse I, Amplification Grade (Invitrogen, United States) to digest residual DNA. Maxima First Strand cDNA Synthesis Kit (Thermo Scientific™, United States) was used for reverse transcription of 1,000 ng of RNA to first strand cDNA according to the manufacturer’s instructions. Reverse transcription was carried out by incubating samples for 10 min at 25°C followed by 15 min at 50°C and final termination at 85°C for 5 min.
Real-time quantitative expression analysis was performed to determine the apoptosis-related gene (bcl-2, bada, cathepsin D, cathepsin Z, caspase 6a, caspase 7, caspase 8, caspase 9, apaf1, p53, cdk1) expression using LightCycler 480 (Roche Applied Science, Germany). The primers of targeted genes were designed by Primer3web (https://primer3.ut.ee/) and were provided by Eurofins Genomics, Germany (Table 1). The reaction mixture contained 2 μL of diluted cDNA (1:10), 1 μL forward and reverse primers (500 nM), 2 μL of nuclease-free water, and 5 μL PowerUp™ SYBR™ Green Master Mix (Thermo scientific™, United States). 18S ribosomal RNA (18 s), beta-actin (βactin), and elongation factor 1 alpha (ef1α) were tested as reference genes. Among those, 18S was found to be the most stable reference gene to normalize the expression of selected genes. All data were presented as mean ± SD from three samples with three parallel repetitions, and all the primers were validated by melt curve analysis. The relative expression levels were analyzed by the 2-ΔΔCt method (32).
The statistical evaluation and graph generation were performed using GraphPad Prism 9.4.1 (GraphPad Software, San Diego, CA, United States). The viability, fertilization, egg development indices, and relative gene expression were analyzed for variance by one-way ANOVA followed by Tukey’s multiple comparisons tests for post hoc comparisons. Viability and fertilization rates between the high- and low-quality oocytes were analyzed by Mann Whitney test as data were not normally distributed. Statistical significance was considered with *p ≤ 0.05, **p ≤ 0.01, ***p ≤ 0.001, and, ****p ≤ 0.0001.
All methodological protocols, experimental manipulations, and sampling procedures used in the present study were approved by the expert committee of the Institutional Animal Care and Use Committee of the University of South Bohemia, Czech Republic. The co-authors of this study deal with the manipulation and artificial reproduction of fish and hold certificates authorizing them to work with laboratory animals according to section 15d paragraph 3 of Act no. 246/1992 Coll. For the purposes of stripping gametes, fish were anesthetized with 0.05% tricaine methanesulfonate (MS-222; Sigma-Aldrich, United States) to ensure their welfare and minimize any associated stress.
To account for quality eggs, we estimated oocyte viability by measuring the TB-based staining rate. An oocyte batch from a female with less than 50% viability rate at 0 HPO was considered poor and excluded from the study at further time points (Figure 1A). Those oocytes also exhibited very low fertilization rates of 27% at 0 HPO and were categorized as low-quality oocytes (Figure 1B). We observed hardly any impact during the first 4 h of in vivo oocyte aging with approximately 97% viability at 0 and 2 HPO, and 90% at 4 HPO without differences compared to 0 HPO (Figure 2A). The viability rate significantly decreased to 72% at 8 HPO and drastically to 16% at 24 HPO. The fertilization rate for oocytes fertilized immediately after ovulation was 91% and slightly decreased at 2 HPO to 78%, not reaching statistical significance (Figure 2B). However, this decrease significantly intensified at 4 HPO and 8 HPO to 62 and 2%, respectively. As expected, the fertilization rate at 24 HPO was nil.
Figure 1. Evaluation of TB-based viability (A) and fertilization rates (B) during post-ovulatory aging in zebrafish. Data represents mean ± SD. Statistical significance calculated by one-way ANOVA followed by Tukeys multiple comparison test are shown by ns, non-significant; *p ≤ 0.05; **p < 0.01; ***p < 0.001; ****p < 0.0001.
Figure 2. Oocyte quality of zebrafish assessed by TB-based viability (A) and fertilization rates (B). Data represents mean ± SD. Statistical significance calculated by Mann Whitney test are shown by *p ≤ 0.05; **p < 0.01.
To further analyze the impact of post-ovulatory aging on oocytes, we measured the survival and hatching of the fertilized eggs. We found that within 2 h of oocyte aging, embryo survival, and hatching rates had significantly declined from 67 and 65% at 0 HPO to 28 and 26% at 2 HPO, respectively. We did not reveal a further drop toward 4 HPO, which showed values of 27 and 22% (Figures 3A,B). Beyond that, the developmental indices are only shown up to 4 HPO, as no embryos developed from 8 HPO. Embryo mortality and larval malformation rates were assessed to further specify oocyte quality. Therefore, larva morphology was examined and classified as malformed if a curled, curved, or bent body shape was observed. The embryo mortality and larval malformation rates barely occurred at 0 HPO with 1 and 9%, respectively (Figures 3C,D). Although the mortality and malformation rates slightly rose to 5 and 33%, respectively, we did not detect significant differences for 2 HPO in either parameter (Figures 3C,D). However, at 4 HPO, a significant increase in the mortality and malformation rates to 12 and 41% was found (Figures 3C,D).
Figure 3. Embryo survival (A), embryo mortality (B), hatching (C), and larval malformation (D) rates during post-ovulatory aging in zebrafish. Data represents mean ± SD. Statistical significance calculated by one-way ANOVA followed by Tukeys multiple comparison tests is shown by ns, non-significant; *p ≤ 0.05; **p < 0.01; ***p < 0.001; ****p < 0.0001.
To investigate possible mechanisms of post-ovulatory oocyte degradation, we studied the mRNA abundance of apoptosis-related genes during oocyte aging. Based on previous findings (17, 18, 33), mRNA abundance of pro- and anti-apoptotic genes were quantified at 0, 8, and 24 HPO. We observed a strong increase in the pro-apoptotic gene cathepsin D at 24 HPO compared to 0 and 8 HPO (Figure 4A). In line with the induction of apoptosis during oocyte aging, we also revealed a significant downregulation of the anti-apoptotic gene bcl-2 at 24 HPO (Figure 4C). The pro-apoptotic gene bada showed constant transcript levels during all time points (Figure 4D). In contrast, the transcript level of tp53 was significantly decreased at 8 and 24 HPO compared to 0 HPO (Figure 4E). The transcript level of the cell cycle-related gene cdk1 significantly decreased at 24 HPO (Figure 4K). Furthermore, cathepsin Z, caspase 6a, caspase 7, caspase 8, caspase 9, and apaf-1 enhibited no significant change at the examined time points (Figures 4B,F–J).
Figure 4. Regulation of pro- and anti-apoptotic genes during post-ovulatory oocyte aging. Depicted are the pro- and anti-apoptotic genes cathepsin D (A), cathepsin Z (B), bcl2 (C), bada (D), tp53 (E), caspase 6a (F), caspase7 (G), caspase 8 (H), caspase 9 (I), apaf 1 (J) and cdk1 (K). A.U. represents arbitrary units. Data represents mean ± SD. Statistical significance calculated by one-way ANOVA followed by Tukeys multiple comparison test is shown by ns, non-significant; *p ≤ 0.05; **p < 0.01; ***p < 0.001; ****p < 0.0001.
The observed decrease in the fertilization rates during in vivo aging of zebrafish oocytes is consistent with findings in other species, such as rainbow trout, Japanese eel (Anguilla japonica), common carp, northern pike, and yellowtail tetra (Alestopetersius caudalis) (26, 34–37). However, the duration of oocyte storage assuring the fertilization success is relatively short in zebrafish compared to those other species. Zebrafish oocytes from certain females exhibited low TB-based viability rates at the time of ovulation, and hence, this decrease in viability was not solely attributed to aging. Various factors, such as nutrition, temperature, photoperiod, salinity, captivity management, stress, chemical elements, and diseases, can affect the oocyte quality (1) thus contributing to low viability rates at the time of ovulation. The in vitro aging of zebrafish AB strain oocytes at 26°C had no significant difference in fertilization rates until 2 h post-stripping, which aligns with our findings (31). However, it’s worth noting that the fertilization capacity of oocytes at 4 h of in vivo aging was higher, even at a higher storage temperature, compared to in vitro aging (31). Zebrafish Tubingen strain oocytes stored in Chinook Salmon ovarian fluid retained fertilizing ability for a longer duration (38) compared to our study. Conversely, gold-strain zebrafish oocytes stored at 8°C in a modified Hanks medium lost their fertilizing ability (39) earlier than in vivo storage of AB strain oocytes in our study. These variations in fertilization rates may be attributed to specific storage conditions (e.g., temperature and medium) as well as genetic strain differences. Although we observed high TB-based viability rates after 4 h in vivo aging, their fertilization rates significantly decreased. Hence, aging may cause the developmental capacity loss of oocytes at the early stage and viability loss in the later stage of aging.
The current results showed that the embryo mortality and larval malformation rates increased significantly with in vivo oocyte aging. However, it’s noteworthy that only 50% of the larvae were found to be malformed at 4 HPO, which contrasts with the findings from in vitro aging experiment where all larvae developed from 4-h-aged oocytes exhibited morphological malformations (31). Studies involving other fish species, such as northern pike, common carp, and rainbow trout, have consistently reported a higher occurrence of malformations in larvae originating from in vitro aged oocytes compared to those aged in vivo (17, 25, 26). This suggests that in vitro oocyte aging is more likely to result in malformation in the resulting larvae compared to in vivo aging. While it’s known that ovarian fluid components may contribute to maintaining the fertilizing ability and embryo development during oocyte aging (40), the precise role of ovarian fluid in the oocyte aging process remains elusive and requires further investigation.
The lysosomal proteases, including cathepsin D, cathepsin Z, and cathepsin B, can indirectly act as pro-apoptotic modulators (41). These cathepsins disrupt mitochondrial membrane potential, thereby triggering the mitochondrial pathway of apoptosis (42). In our study, we observed a significant increase in cathepsin D in 24-h-aged oocytes, while cathepsin Z showed a non-significant upward trend. Studies with other fish species have shown variability in cathepsin activity during oocyte aging. For example, sea bream (Sparus aurata) eggs with high cathepsin D activity exhibited activation of apoptosis (43), while mRNA abundance of cathepsin D showed no significant change during in vivo aging of rainbow trout oocytes (44). Similarly, studies with African catfish (Clarias gariepinus) and common carp showed varying trends in cathepsin D and cathepsin Z mRNA level (17, 33). Considering that matured oocytes are transcriptionally silent until the initiation of zygotic transcription and early embryo development relies on stored mRNAs (45), the increase in cathepsin D transcripts at 24 h post-ovulation in our study could be linked to the spontaneous activation of oocytes, which ceases transcriptional silence and presumably triggering cell death. Cathepsins are also known to potentially activate caspases, which are key regulators of apoptosis (46). In our study, we did not find a significant difference in the mRNA level of caspase 6a, caspase 7, caspase 8, and caspase 9 in zebrafish aged oocytes. This contrasts with findings from studies involving common carp, where the mRNA abundance of caspase 9 increased during oocyte aging (17). However, future research focusing on cathepsin and caspase enzyme activity may provide a clearer understanding of their roles and interactions during oocyte aging.
Another critical factor in cell fate determination is the ratio of pro-apoptotic to anti-apoptotic Bcl-2 family proteins. An excess of bcl-2 typically results in cell survival, while an excess of bax leads to cell death (47). In our study, we observed a significant decrease in bcl-2 abundance in 24-h in vivo aged oocytes, which is consistent with findings in aged oocytes of pigs and mice (9, 10). However, the bcl-2 transcript level remained unchanged in common carp aged oocytes during 14 h of in vivo aging (17). The bax gene is upregulated during oocyte aging in common carp (17), whereas it remains unchanged in mouse oocytes during in vitro aging (14). Additionally, in the current study, the expression of bada, which has a similar function to bax, showed no statistical difference during oocyte aging in zebrafish. This could be due to low levels of maturation promoting factor (MPF) and mitogen-activated protein kinase (MAPK) in aged oocytes (48) potentially influencing the downregulation of bcl-2 without affecting bada expression (14). The decrease in the pro-survival gene bcl-2 and the increase in pro-apoptotic cathepsin D might make aged oocytes prone to apoptosis.
p53can directly bind to PUMA/NOXA (49) and increase mitochondrial membrane permeability, resulting in leakage of pro-apoptotic proteins and cytochrome c, or indirectly activate pro-apoptotic genes, such as bax, puma, bbc3, igfbp3, noxa, and apaf1 (50–52). The mRNA level of tp53 decreased significantly at 24 HPO, which is consistent with the results observed in post-ovulatory aged rainbow trout oocytes (53). The transcript levels of tp53 orthologous decreased in maternally aged oocytes (54). The tp53 protein level decreased significantly in mouse oocytes (55), whereas the mRNA level remained constant in pig oocytes during post-ovulatory aging (56). Maternally derived tp53 mRNA is essential for embryonic development in mice and frog (57, 58). Thus, a decrease in tp53 transcripts along with bcl-2 might contribute to abnormal cleavage, increased mortality, and increased malformations in embryos developed from the aged oocytes. However, the detailed role of tp53 in oocyte developmental competence and aging is yet unexplored.
Cdk1 kinase is a major component of the maturation-promoting factor, MPF that maintains the ovulated oocytes at the metaphase arrest (59). Deficiency of cdk1 in mouse ovulated oocytes prevents the cells from resuming the meiotic cycle and resulting in infertility (60). Post-ovulatory aged frog oocytes exhibited low cdk1 activity followed by spontaneous meiotic exit (61), which is a prerequisite for the activation of apoptosis (62). The drastic decrease in cdk1 transcript at 24 HPO in our study might be due to the spontaneous activation to exit metaphase arrest and execute the cell death. In the current study, the 8-h-aged oocytes lost their fertilizing capacity and exhibited membrane blebbing phenotype after activation with water (unpublished data), which is a morphological indicator of apoptosis. Additional analyzes are required to elaborate on the mechanism behind spontaneous activation followed by cell death in post-ovulatory aged oocytes.
Zebrafish AB strain oocytes can be stored in vivo for 2 h with minimal loss of competency, and complete loss of egg fertilizing ability occurs at 8 h of ovulation. TB staining does not accurately detect oocyte post-ovulatory aging. However, it can be used as a simple and rapid method to estimate the quality of oocytes before fertilization in zebrafish. The upregulation of cathepsin D and downregulation of bcl-2 and cdk1 after 24 h of in vivo oocyte aging was observed. Based on the results obtained in the current study, the apoptotic pathway is activated in the advanced stage of aging, attributing to viability loss. Complementary analyses like DNA fragmentation, detection of proteins involved in the activation of cell death pathway, and examining mitochondrial changes would contribute to thoroughly understanding the involvement of apoptosis in the oocyte aging process.
The raw data supporting the conclusions of this article will be made available by the authors, without undue reservation.
The experiment was carried out under controlled conditions of RAS in the Laboratory of Intensive Aquaculture (LIA), which is part of the University of South Bohemia, Faculty of Fisheries and Protection of Waters (USB FFPW) (Vodňany, Czech Republic). All fish manipulations during the experiment were governed by valid legislative regulations of the Czech Republic (Act No. 166/1996 and No. 246/1992); the permit was issued No. 58672/2020-MZE-18134 and No. 33446/2020-MZE-18134 in the NAZV QK22020144 project.
EK: Conceptualization, Formal analysis, Investigation, Methodology, Writing – original draft. KM: Writing – review & editing. SB: Writing – review & editing. SW: Formal analysis, Investigation, Methodology, Writing – review & editing. AAS: Formal analysis, Investigation, Methodology, Writing – review & editing. TP: Funding acquisition, Writing – review & editing. AIS: Conceptualization, Formal analysis, Funding acquisition, Investigation, Methodology, Supervision, Writing – review & editing.
The author(s) declare that financial support was received for the research, authorship, and/or publication of this article. This study was financially supported by the Ministry of Agriculture of the Czech Republic (NAZV QK22020144) and by the Czech Science Foundation (GACR No. 20-01251S).
We thank Diana Woellner, Department of Endocrinology and Metabolism, Charité – Universitätsmedizin Berlin, for excellent technical assistance.
The authors declare that the research was conducted in the absence of any commercial or financial relationships that could be construed as a potential conflict of interest.
All claims expressed in this article are solely those of the authors and do not necessarily represent those of their affiliated organizations, or those of the publisher, the editors and the reviewers. Any product that may be evaluated in this article, or claim that may be made by its manufacturer, is not guaranteed or endorsed by the publisher.
The Supplementary material for this article can be found online at: https://www.frontiersin.org/articles/10.3389/fvets.2024.1389070/full#supplementary-material
1. Bobe, J, and Labbé, C. Egg and sperm quality in fish. Gen Comp Endocrinol. (2010) 165:535–48. doi: 10.1016/j.ygcen.2009.02.011
2. Tarin, JJ, Perez-Albala, S, and Cano, A. Consequences on offspring of abnormal function in ageing gametes. Hum Reprod Update. (2000) 6:532–49. doi: 10.1093/humupd/6.6.532
3. Takahashi, T, Takahashi, E, Igarashi, H, Tezuka, N, and Kurachi, H. Impact of oxidative stress in aged mouse oocytes on calcium oscillations at fertilization. Mol Reprod Dev. (2003) 66:143–52. doi: 10.1002/mrd.10341
4. Perez, GI, Trbovich, AM, Gosden, RG, and Tilly, JL. Mitochondria and the death of oocytes. Nature. (2000) 403:500–1. doi: 10.1038/35000651
5. Hamatani, T, Falco, G, Carter, MG, Akutsu, H, Stagg, CA, Sharov, AA, et al. Age-associated alteration of gene expression patterns in mouse oocytes. Hum Mol Genet. (2004) 13:2263–78. doi: 10.1093/hmg/ddh241
6. Takahashi, T, Igarashi, H, Amita, M, Hara, S, and Kurachi, H. Cellular and molecular mechanisms of various types of oocyte aging. Reprod Med Biol. (2011) 10:239–49. doi: 10.1007/s12522-011-0099-0
7. Kosubek, A, Klein-Hitpass, L, Rademacher, K, Horsthemke, B, and Ryffel, GU. Aging of Xenopus tropicalis eggs leads to deadenylation of a specific set of maternal mRNAs and loss of developmental potential. PLoS One. (2010) 5:e13532. doi: 10.1371/journal.pone.0013532
8. Dankert, D, Demond, H, Trapphoff, T, Heiligentag, M, Rademacher, K, Eichenlaub-Ritter, U, et al. Pre-and postovulatory aging of murine oocytes affect the transcript level and poly (a) tail length of maternal effect genes. PLoS One. (2014) 9:e108907. doi: 10.1371/journal.pone.0108907
9. Ma, W, Zhang, D, Hou, Y, Li, Y-H, Sun, Q-Y, Sun, X-F, et al. Reduced expression of MAD2, BCL2, and MAP kinase activity in pig oocytes after in vitro aging are associated with defects in sister chromatid segregation during meiosis II and embryo fragmentation after activation. Biol Reprod. (2005) 72:373–83. doi: 10.1095/biolreprod.104.030999
10. Tatone, C, Carbone, MC, Gallo, R, Delle Monache, S, Di Cola, M, Alesse, E, et al. Age-associated changes in mouse oocytes during postovulatory in vitro culture: possible role for meiotic kinases and survival factor BCL21. Biol Reprod. (2006) 74:395–402. doi: 10.1095/biolreprod.105.046169
11. Huang, J-C, Yan, L-Y, Lei, Z-L, Miao, Y-L, Shi, L-H, Yang, J-W, et al. Changes in histone acetylation during postovulatory aging of mouse oocyte. Biol Reprod. (2007) 77:666–70. doi: 10.1095/biolreprod.107.062703
12. Ge, Z-J, Schatten, H, Zhang, C-L, and Sun, Q-Y. Oocyte ageing and epigenetics. Reproduction. (2015) 149:R103–14. doi: 10.1530/REP-14-0242
13. Perez, GI, Tao, X-J, and Tilly, JL. Fragmentation and death (aka apoptosis) of ovulated oocytes. Mol Hum Reprod. (1999) 5:414–20. doi: 10.1093/molehr/5.5.414
14. Gordo, AC, Rodrigues, P, Kurokawa, M, Jellerette, T, Exley, GE, Warner, C, et al. Intracellular calcium oscillations signal apoptosis rather than activation in in vitro aged mouse eggs. Biol Reprod. (2002) 66:1828–37. doi: 10.1095/biolreprod66.6.1828
15. Tokmakov, AA, Sato, K-I, and Stefanov, VE. Postovulatory cell death: why eggs die via apoptosis in biological species with external fertilization. J Reprod Dev. (2017) 64:1–6. doi: 10.1262/jrd.2017-100
16. Ma, H, Weber, GM, Hostuttler, MA, Wei, H, Wang, L, and Yao, J. MicroRNA expression profiles from eggs of different qualities associated with post-ovulatory ageing in rainbow trout (Oncorhynchus mykiss). BMC Genomics. (2015) 16:201. doi: 10.1186/s12864-015-1400-0
17. Samarin, A, Samarin, A, Østbye, T-KK, Ruyter, B, Sampels, S, Burkina, V, et al. Alteration of mRNA abundance, oxidation products and antioxidant enzyme activities during oocyte ageing in common carp Cyprinus carpio. PLoS One. (2019) 14:e0212694. doi: 10.1371/journal.pone.0212694
18. Samarin, AM, Samarin, AM, Østbye, T-KK, Ruyter, B, Sampels, S, Burkina, V, et al. The possible involvement of oxidative stress in the oocyte ageing process in goldfish Carassius auratus (Linnaeus, 1758). Sci Rep. (2019) 9:10469. doi: 10.1038/s41598-019-46895-1
19. Waghmare, SG, Samarin, AM, Samarin, AM, Danielsen, M, Møller, HS, Policar, T, et al. Histone acetylation dynamics during in vivo and in vitro oocyte aging in common carp Cyprinus carpio. Int J Mol Sci. (2021) 22:6036. doi: 10.3390/ijms22116036
20. Samarin, AM, Policar, T, and Lahnsteiner, F. Fish oocyte ageing and its effect on egg quality. Rev Fish Sci Aquacul. (2015) 23:302–14. doi: 10.1080/23308249.2015.1053560
21. Rizzo, E, Godinho, HP, and Sato, Y. Short-term storage of oocytes from the neotropical teleost fish Prochilodus marggravii. Theriogenology. (2003) 60:1059–70. doi: 10.1016/S0093-691X(03)00108-0
22. Niksirat, H, Sarvi, K, Amiri, BM, Karami, M, and Hatef, A. In vitro storage of unfertilized ova of endangered Caspian brown trout (Salmo trutta caspius) in artificial media. Anim Reprod Sci. (2007) 100:356–63. doi: 10.1016/j.anireprosci.2006.08.019
23. Bahre Kazemi, M, Soltani, M, Matinfar, A, Abtahi, B, Pusti, I, SamarinA, M, et al. Biochemical and histological studies of over-ripened oocyte in the Caspian brown trout (Salmo trutta caspius) to determine biomarkers for egg quality. Iran J Fish Sci. (2010) 9:33–48. doi: 10.22092/IJFS.2018.114081
24. Niksirat, H, Sarvi, K, Amiri, BM, and Hatef, A. Effects of storage duration and storage media on initial and post-eyeing mortality of stored ova of rainbow trout Oncorhynchus mykiss. Aquaculture. (2007) 262:528–31. doi: 10.1016/j.aquaculture.2006.10.031
25. Samarin, AM, Ahmadi, MR, Azuma, T, Rafiee, GR, Amiri, BM, and Naghavi, MR. Influence of the time to egg stripping on eyeing and hatching rates in rainbow trout Oncorhynchus mykiss under cold temperatures. Aquaculture. (2008) 278:195–8. doi: 10.1016/j.aquaculture.2008.03.034
26. Samarin, AM, Blecha, M, Uzhytchak, M, Bytyutskyy, D, Zarski, D, Flajshans, M, et al. Post-ovulatory and post-stripping oocyte ageing in northern pike, Esox lucius (Linnaeus, 1758), and its effect on egg viability rates and the occurrence of larval malformations and ploidy anomalies. Aquaculture. (2016) 450:431–8. doi: 10.1016/j.aquaculture.2015.08.017
27. Westerfield, M. The zebrafish book. A guide for the laboratory use of Zebrafish (Danio rerio), 4th Edition. Eugene: University of Oregon Press (2000).
28. Strober, W . Trypan blue exclusion test of cell viability. Curr Protoc Immunol. (2015) 111:A–3B. doi: 10.1002/0471142735.ima03bs111
29. Rodina, M, Cosson, J, Gela, D, and Linhart, O. Kurokura solution as immobilizing medium for spermatozoa of Tench (Tinca tinca L.). Aquac Int. (2004) 12:119–31. doi: 10.1023/B:AQUI.0000017192.75993.e3
30. Fauvel, C, Suquet, M, and Cosson, J. Evaluation of fish sperm quality. J Appl Ichthyol. (2010) 26:636–43. doi: 10.1111/j.1439-0426.2010.01529.x
31. Waghmare, SG, Samarin, AM, Franěk, R, Pšenička, M, Policar, T, Linhart, O, et al. Oocyte ageing in zebrafish Danio rerio (Hamilton, 1822) and its consequence on the viability and ploidy anomalies in the progeny. Animals. (2021) 11:912. doi: 10.3390/ani11030912
32. Livak, KJ, and Schmittgen, TD. Analysis of relative gene expression data using real-time quantitative PCR and the 2(-Delta Delta C(T)) method. Methods. (2001) 25:402–8. doi: 10.1006/meth.2001.1262
33. Samarin, AM, Sampels, S, Policar, T, Rodina, M, Hematyar, N, and Samarin, AM. mRNA abundance changes during in vitro oocyte ageing in African catfish Clarias gariepinus (Burchell, 1822). Aquac Res. (2018) 49:1037–45. doi: 10.1111/are.13552
34. Azuma, T, Ohta, H, Oda, S, Muto, K, Yada, T, and Unuma, T. Changes in fertility of rainbow trout eggs retained in coelom. Fish Sci. (2003) 69:131–6. doi: 10.1046/j.1444-2906.2003.00597.x
35. Nomura, K, Takeda, Y, Unuma, T, Morishima, K, Tanaka, H, Arai, K, et al. Post-ovulatory oocyte aging induces spontaneous occurrence of polyploids and mosaics in artificial fertilization of Japanese eel, Anguilla japonica. Aquaculture. (2013) 404-405:15–21. doi: 10.1016/j.aquaculture.2013.04.016
36. Samarin, AM, Blecha, M, Bytyutskyy, D, and Policar, T. Post-ovulatory oocyte ageing in pikeperch (Sander lucioperca L.) and its effect on egg viability rates and the occurrence of larval malformations and ploidy anomalies. Turk J Fish Aquat Sci. (2015) 15:429–35. doi: 10.4194/1303-2712-v15_2_29
37. do Nascimento, NF, Lázaro, TM, de Alcântara, NR, Senhorini, JA, dos Santos, SCA, Nakaghi, LSO, et al. In vivo storage of oocytes leads to lower survival, increased abnormalities and may affect the ploidy status in the yellowtail tetra Astyanax altiparanae. Zygote. (2018) 26:471–5. doi: 10.1017/S0967199418000527
38. Siripattarapravat, K, Busta, A, Steibel, JP, and Cibelli, J. Characterization and in vitro control of MPF activity in zebrafish eggs. Zebrafish. (2009) 6:97–105. doi: 10.1089/zeb.2008.0527
39. Cardona-Costa, J, Perez-Camps, M, Garcia-Ximenez, F, and Espinos, FJ. Effect of gametes aging on their activation and fertilizability in zebrafish (Danio rerio). Zebrafish. (2009) 6:93–5. doi: 10.1089/zeb.2008.0578
40. Rime, H, Guitton, N, Pineau, C, Bonnet, E, Bobe, J, and Jalabert, B. Post-ovulatory ageing and egg quality: a proteomic analysis of rainbow trout coelomic fluid. Reprod Biol Endocrinol. (2004) 2:26. doi: 10.1186/1477-7827-2-26
41. Kågedal, K, Johansson, U, and Öllinger, K. The lysosomal protease cathepsin D mediates apoptosis induced by oxidative stress. FASEB J. (2001) 15:1592–4. doi: 10.1096/fj.00-0708fje
42. Paquet, C, Sane, A, Beauchemin, M, and Bertrand, R. Caspase-and mitochondrial dysfunction-dependent mechanisms of lysosomal leakage and cathepsin B activation in DNA damage-induced apoptosis. Leukemia. (2005) 19:784–91. doi: 10.1038/sj.leu.2403717
43. Carnevali, O, Polzonetti, V, Cardinali, M, Pugnaloni, A, Natalini, P, Zmora, N, et al. Apoptosis in sea bream Sparus aurata eggs. Mol Reprod Dev. (2003) 66:291–6. doi: 10.1002/mrd.10356
44. Aegerter, S, Jalabert, B, and Bobe, J. Large scale real-time PCR analysis of mRNA abundance in rainbow trout eggs in relationship with egg quality and post-ovulatory ageing. Mol Reprod Dev. (2005) 72:377–85. doi: 10.1002/mrd.20361
45. Lubzens, E, Bobe, J, Young, G, and Sullivan, CV. Maternal investment in fish oocytes and eggs: the molecular cargo and its contributions to fertility and early development. Aquaculture. (2017) 472:107–43. doi: 10.1016/j.aquaculture.2016.10.029
46. Conus, S, Perozzo, R, Reinheckel, T, Peters, C, Scapozza, L, Yousefi, S, et al. Caspase-8 is activated by cathepsin D initiating neutrophil apoptosis during the resolution of inflammation. J Exp Med. (2008) 205:685–98. doi: 10.1084/jem.20072152
47. Adams, JM, and Cory, S. The Bcl-2 protein family: arbiters of cell survival. Science. (1998) 281:1322–6. doi: 10.1126/science.281.5381.1322
48. Xu, Z, Abbott, A, Kopf, GS, Schultz, RM, and Ducibella, T. Spontaneous activation of ovulated mouse eggs: time-dependent effects on M-phase exit, cortical granule exocytosis, maternal messenger ribonucleic acid recruitment, and inositol 1, 4, 5-trisphosphate sensitivity. Biol Reprod. (1997) 57:743–50. doi: 10.1095/biolreprod57.4.743
49. Nakano, K, and Vousden, KH. PUMA, a novel proapoptotic gene, is induced by p53. Mol Cell. (2001) 7:683–94. doi: 10.1016/S1097-2765(01)00214-3
50. Miyashita, T, and Reed, JC. Tumor suppressor p53 is a direct transcriptional activator of the human bax gene. Cell. (1995) 80:293–9. doi: 10.1016/0092-8674(95)90412-3
51. Robles, AI, Bemmels, NA, Foraker, AB, and Harris, CC. APAF-1 is a transcriptional target of p53 in DNA damage-induced apoptosis. Cancer Res. (2001) 61:6660–4.
52. Yu, J, and Zhang, L. The transcriptional targets of p53 in apoptosis control. Biochem Biophys Res Commun. (2005) 331:851–8. doi: 10.1016/j.bbrc.2005.03.189
53. Aegerter, S, Jalabert, B, and Bobe, J. Messenger RNA stockpile of cyclin B, insulin-like growth factor I, insulin-like growth factor II, insulin-like growth factor receptor Ib, and p53 in the rainbow trout oocyte in relation with developmental competence. Mol Reprod Dev. (2004) 67:127–35. doi: 10.1002/mrd.10384
54. Guglielmino, MR, Santonocito, M, Vento, M, Ragusa, M, Barbagallo, D, Borzì, P, et al. TAp73 is downregulated in oocytes from women of advanced reproductive age. Cell Cycle. (2011) 10:3253–6. doi: 10.4161/cc.10.19.17585
55. Jeon, H-J, Cui, X-S, Guo, J, Lee, JM, Kim, J-S, and Oh, JS. TCTP regulates spindle assembly during postovulatory aging and prevents deterioration in mouse oocyte quality. Biochimica et Biophysica Acta (BBA) - molecular. Cell Res. (2017) 1864:1328–34. doi: 10.1016/j.bbamcr.2017.05.002
56. Wang, T, Gao, YY, Chen, L, Nie, ZW, Cheng, W, Liu, X, et al. Melatonin prevents postovulatory oocyte aging and promotes subsequent embryonic development in the pig. Aging. (2017) 9:1552–64. doi: 10.18632/aging.101252
57. Armstrong, JF, Kaufman, MH, Harrison, DJ, and Clarke, AR. High-frequency developmental abnormalities in p53-deficient mice. Curr Biol. (1995) 5:931–6. doi: 10.1016/S0960-9822(95)00183-7
58. Wallingford, JB, Seufert, DW, Virta, VC, and Vize, PD. p53 activity is essential for normal development in Xenopus. Curr Biol. (1997) 7:747–57. doi: 10.1016/S0960-9822(06)00333-2
59. Masui, Y, and Markert, CL. Cytoplasmic control of nuclear behavior during meiotic maturation of frog oocytes. J Exp Zool. (1971) 177:129–45. doi: 10.1002/jez.1401770202
60. Adhikari, D, Zheng, W, Shen, Y, Gorre, N, Ning, Y, Halet, G, et al. Cdk1, but not Cdk2, is the sole Cdk that is essential and sufficient to drive resumption of meiosis in mouse oocytes. Hum Mol Genet. (2012) 21:2476–84. doi: 10.1093/hmg/dds061
61. Iguchi, S, Iwasaki, T, Fukami, Y, and Tokmakov, AA. Unlaid Xenopus eggs degrade by apoptosis in the genital tract. BMC Cell Biol. (2013) 14:11. doi: 10.1186/1471-2121-14-11
Keywords: apoptosis, cell death, fertilization, membrane integrity, trypan blue, zebrafish
Citation: Konar ESM, Mai K, Brachs S, Waghmare SG, Samarin AM, Policar T and Samarin AM (2024) Evaluation of viability, developmental competence, and apoptosis-related transcripts during in vivo post-ovulatory oocyte aging in zebrafish Danio rerio (Hamilton, 1822). Front. Vet. Sci. 11:1389070. doi: 10.3389/fvets.2024.1389070
Received: 20 February 2024; Accepted: 30 May 2024;
Published: 17 June 2024.
Edited by:
Yatta Linhares Boakari, Texas A&M University System, United StatesReviewed by:
Charles Bradley Shuster, New Mexico State University, United StatesCopyright © 2024 Konar, Mai, Brachs, Waghmare, Samarin, Policar and Samarin. This is an open-access article distributed under the terms of the Creative Commons Attribution License (CC BY). The use, distribution or reproduction in other forums is permitted, provided the original author(s) and the copyright owner(s) are credited and that the original publication in this journal is cited, in accordance with accepted academic practice. No use, distribution or reproduction is permitted which does not comply with these terms.
*Correspondence: Essaikiammal Sodalai Muthu Konar, ZWtvbmFyQGZyb3YuamN1LmN6
Disclaimer: All claims expressed in this article are solely those of the authors and do not necessarily represent those of their affiliated organizations, or those of the publisher, the editors and the reviewers. Any product that may be evaluated in this article or claim that may be made by its manufacturer is not guaranteed or endorsed by the publisher.
Research integrity at Frontiers
Learn more about the work of our research integrity team to safeguard the quality of each article we publish.