- 1Food and Agriculture Vocational School, Çankırı Karatekin University, Çankırı, Türkiye
- 2Institute of Science, Hacettepe University, Ankara, Türkiye
- 3Division of Ecology, Department of Biology, Hacettepe University, Ankara, Türkiye
- 4Department of Biology, Sabire Yazıcı Faculty of Science and Letters, Aksaray University, Aksaray, Türkiye
- 5Institute of Preclinical Sciences, Veterinary Faculty, University of Ljubljana, Ljubljana, Slovenia
- 6Clinic for Birds, Small Mammals and Reptiles, Veterinary Faculty, University of Ljubljana, Ljubljana, Slovenia
Introduction: The aim of our study was to measure fecal glucocorticoid metabolite (FGM) concentrations in captive and free-ranging male and female mountain gazelles (Gazella gazella) during their circannual cycle. In addition, FGM concentrations were used to track the intensity of the adrenocortical response in mountain gazelles during the same period.
Methods: Fecal samples were collected from the ground in the Hatay Mountain Gazelle Wildlife Development Area in the Hatay Province of Türkiye (36°32’ N, 36°32′ E) in each season of the year (December, April, July, September). The sex of the animals was determined by detecting the SRY gene of the Y chromosome in DNA isolated from the fecal samples. FGM was extracted from dried fecal samples with methanol, and its concentration was measured using a previously partially validated ELISA.
Results and discussion: The results indicate that season is the most important factor explaining the variability in FGM concentrations in mountain gazelles. In animals of both sexes, the highest concentrations of FGM were observed in September. The values were significantly higher in the captive population, perhaps due to unpredictable stress. In July, FGM concentrations were low in both populations. As a result of the overall analysis across seasons, the comparison of FGM concentrations between captive and free-ranging animals revealed higher concentrations in captive animals only in September but not in other seasons, although higher concentrations have been previously reported for several wild captive species. Due to predation risk, the presence of offspring can be considered a critical point in the biological cycle for the welfare of free-ranging mountain gazelles, as suggested by the higher FGM concentrations in the free-ranging population in July. The high number of visitors could be a challenge for mountain gazelles in captivity, as indicated by higher FGM concentrations during September. Sex had no effect on the FGM concentrations of either population.
1 Introduction
The mountain gazelle (Gazella gazella) inhabits areas in Israel and Palestine, the Golan Heights (1), the Jordanian side of the Jordan Valley (2) and the Hatay Province of Türkiye (3, 4). The population of mountain gazelles in Türkiye is isolated from other populations. The first data on population size in Türkiye reported approximately two hundred individuals in 2008, while the most recent inventory studies showed that there were 1,387 individuals in 2024 (data were estimated by the Hatay Branch of Nature Conservation National Parks). For the Israeli population of the species, approximately 5,000 individuals were counted in 2020 based on data from Israel’s Nature and Parks Authority (1). The species is classified as endangered by the International Union for Conservation of Nature and Natural Resources (5).
When a species is declared endangered, it calls upon human society, nature conservation policy, and the veterinary profession to ensure its survival in its natural habitat. Every individual holds significance in the conservation of endangered species, necessitating efforts to protect injured animals and motherless offspring while prioritizing their care, treatment, and conservation. The veterinary profession, with its focus on animal welfare, plays a fundamental role (6), utilizing assessments of HPA axis activity as a crucial indicator (7). Physiological data are essential for maintaining species viability and population health and for accurately assessing ecological outcomes. The incorporation of measures of adrenocortical activity, such as fecal glucocorticoid metabolite (FGM) concentrations, can aid in understanding animal behavior, disease susceptibility, and population performance, guiding effective conservation strategies globally (8).
The assessment of adrenocortical activity in mountain gazelles is important from both general and intraspecies-specific perspectives. In general, seasonal fluctuations in adrenocortical activity may indicate critical points for animal welfare when external (environmental) and internal (physiological) factors are considered together. At the intraspecific level, the comparison of the seasonal adrenocortical activity between the sexes provides information on sex-dependent differences in the adrenocortical response during the annual biological cycle, adding to the knowledge of mountain gazelle physiology. Intraspecies differences can also be detected between free-ranging and captive populations, as captivity has been recognized as a risk factor for distress in various species (9).
As the mountain gazelle population in Türkiye is isolated from larger populations in Israel (and Jordan), its survival is an important challenge for relevant local authorities and specialized experts involved in conservation policy, such as conservation and environment scientists, wildlife veterinarians, conservation educators and journalists. Therefore, knowledge of the animals’ habitat, potential risk factors, identification of critical points and assessment of animal welfare is important to ensure basic living conditions. In light of species conservation, identifying environmental stressors and limiting their harmful effects play important roles. Well-known examples of the stressors that affect species are macroenvironmental factors such as climate change (10), weather conditions, habitat fragmentation and loss due to traffic and agricultural activities (11–13), and pollution due to environmental pollutants (14), including sound (15) and light pollution (16). At the microenvironmental level, gazelle populations are faced with predation risk, species-specific infections, parasite infestations, and cohabitation and competition with domestic livestock. Despite some studies on genetics (4, 17), parasite infestation (18, 19), population status and distribution (20), and appropriate conservation planning (21), mountain gazelles are attractive and endangered mammal species and remain one of the least studied species in Türkiye. Therefore, studies addressing metabolic, endocrine and reproductive characteristics are essential to ensure the survival of this species in Türkiye. The adrenocortical response, as a basic adaptive mechanism, is not well known, so information in this area is needed to elucidate the critical points in the biological cycle of animals.
Our study thus aimed to understand the seasonal patterns in adrenocortical activity in mountain gazelles. Based on several publications showing seasonality of cortisol synthesis activity in different animal species (22–28), we assessed circannual fluctuations in HPA axis activity by measuring FGM concentrations in free-ranging and captive mountain gazelles of both sexes. The sampling schedule included a whole-year period that allowed the biological cycle of the species to be accounted for. Since captivity is a risk factor for animal distress (9), we also compared the adrenocortical response of captive and free-ranging mountain gazelles using FGM concentrations. In view of the abovementioned findings on adrenocortical activity, seasonal differences in FGM concentrations are expected. When comparing captive and free-ranging animals, a more intense adrenocortical response is expected in the captive population.
2 Materials and methods
2.1 Study species
The mountain gazelle, a member of the genus Gazella (Antilopini, Bovidae), has a lifespan of 8 to 12 years (29). Females can become pregnant at 18 months but under favorable conditions as early as 6 to 12 months (30). Males reach reproductive maturity at 3 years or as soon as 15 to 20 months with good nutrition. They typically give birth to one offspring per year after a 180-day gestation (31). The offspring, weighing approximately 2 kg, start on solid foods after 3 to 6 weeks and are weaned by 3 months (32). The mating season of the studied population of G. gazella is between December and January (Mina C. Karaer and Tolga Kankılıç, pers. obs.). During the mating season and early gestation, female groups tend to be larger, and female group home ranges correspond to the territory of adult, territorial males (33, 34). Although there is a dominant male in each mating group (35), other males attempt to mate with the females in the group. However, the dominant male usually seeks to prevent these matings. After the mating season, mating groups typically start to spread out in late January (Tolga Kankılıç, pers. obs.), with females remaining together (matrilineal groups with a mother and her last born daughters) and completing the pregnancy process (36). This social organization mirrors the structure observed in female G. arabica groups (36). The fawning season falls between May and June. Considering the mating season, June, July, and August are subsequent lactating periods, as lactation lasts for approximately 3 months. During this period, most females form matrilineal groups (36). Adult males are territorial and solitary throughout the year (35). However, nonterritorial males live in bachelor groups, comprising yearlings and adult males without a territory (34). After the lactating period, female fawns always remain in the matrilineal groups, whereas male fawns leave this group at approximately 6 months of age (30). We refer to this period beyond the reproductive cycle as the nonreproductive period. During this period, females and males have no attempt to mate; they are not organized into mating groups, and females are neither pregnant nor lactating.
2.2 Study area
The study was conducted in the Hatay Mountain Gazelle Wildlife Development Area, located in Hatay Province, Türkiye, close to the Syrian border (36°32’ N, 36°32′ E, 200–450 m.a.s.l.). The mountain gazelle habitat that was included in our study covered 13,228 hectares, including grassland vegetation with a few patches of shrublands, large expanses of croplands, and rocky hills around the Kırıkhan district of Hatay Province in Türkiye. The estimated population of mountain gazelles in this area was 1,331 individuals in 2023 (data from the Hatay Branch of the General Directorate of Nature Conservation and National Parks). This habitat is actively used by the species throughout the year, and it is the only habitat known for mountain gazelles in Türkiye (17). The protected area comprises a free-ranging population as well as a small captive population in the Hatay Mountain Gazelle Production Center. Most of the Hatay Mountain Gazelle Wildlife Development Area falls within a strictly controlled military zone. For this reason, entry to the free-ranging population area is prohibited for everyone except military personnel and farmers.
The free-ranging and captive populations are subjected to the same macroenvironmental conditions. Thus, the photoperiod and climatic conditions (Table 1) were the same for both populations (21). The vegetation structure is similar in both areas, i.e., in the free-ranging and captive populations. The dominant plant species in the study area included Abutilon indicum, Sarcopoterium spinosum, Ziziphus jujuba, Zizyphus lotus, Plantago cretica, Asphodelus aestivus, and Arum dioscoridis (21).
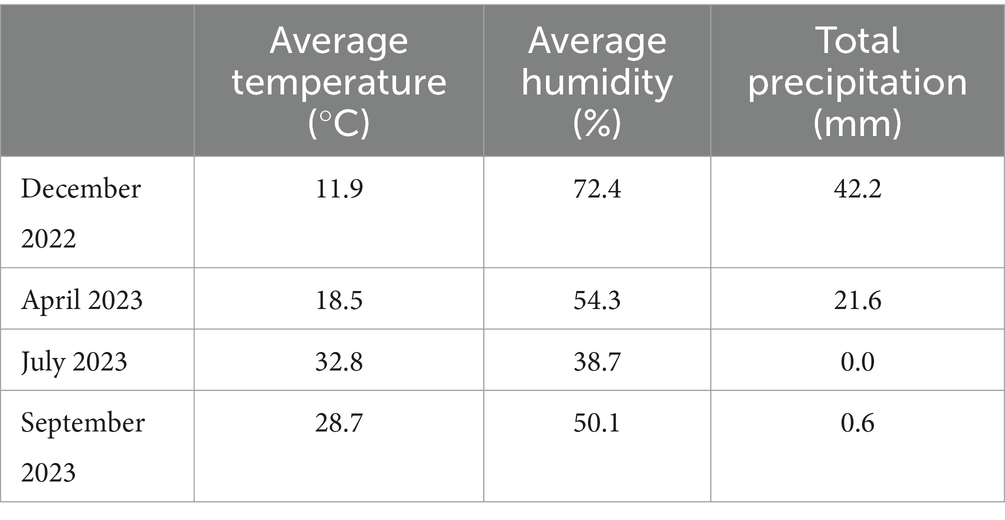
Table 1. Climatic conditions during the sampling periods in Hatay (the data are based on the Turkish State Meteorological Service data).
However, at the microenvironmental level, some conditions differ among populations (Table 2).
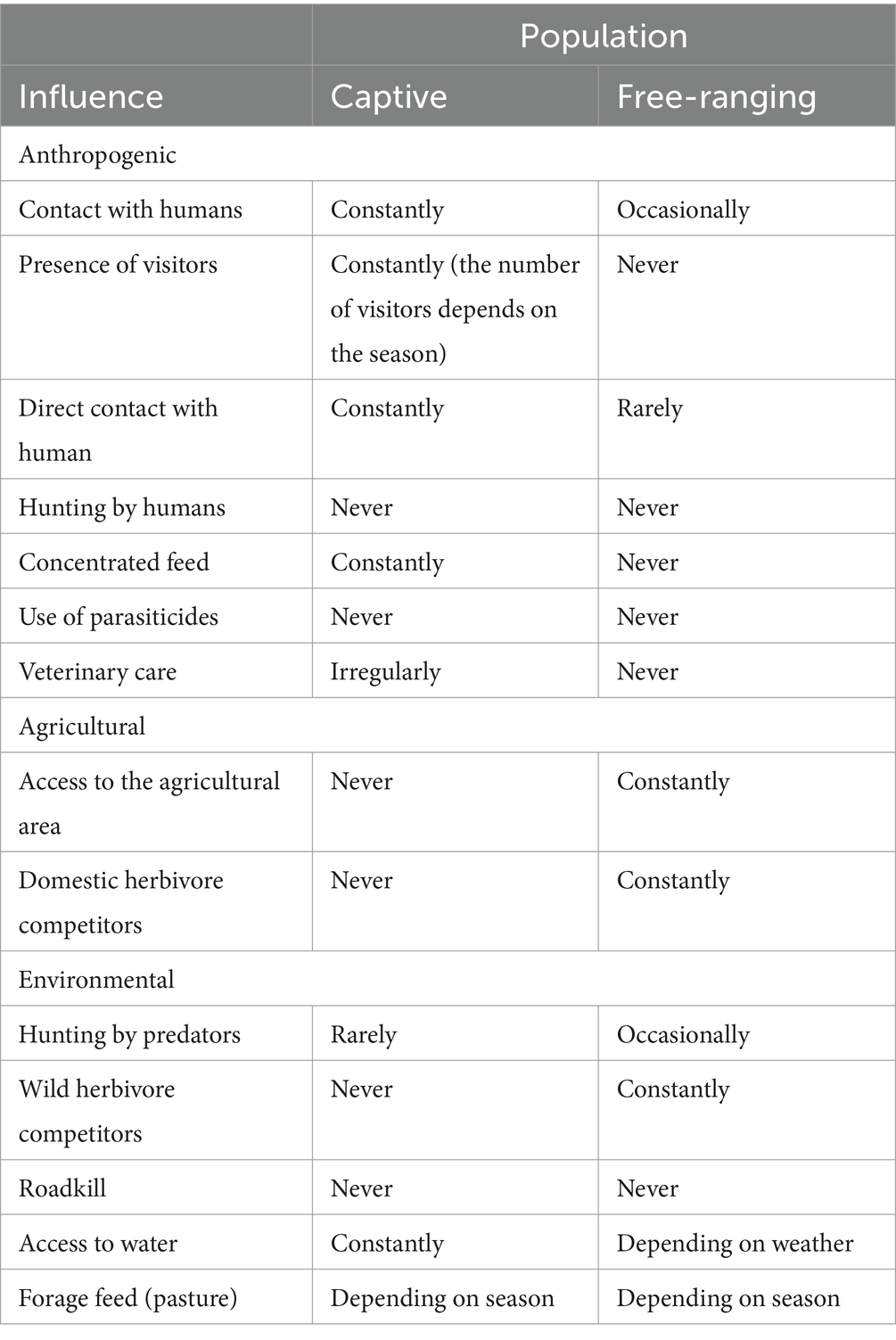
Table 2. Human-associated activities and environmental conditions in captive and free-ranging populations of the mountain gazelle in Hatay province, Türkiye.
The free-ranging population thus feeds to a small extent on agricultural areas, especially during cultivation periods, as there are many agricultural areas and olive groves (21). The access to water for the free-ranging population is limited. There are only artifactitious ponds and water troughs in the field; however, during our fieldwork, we observed that water troughs and a small pond were dry for three seasons (April, July, and September 2023). Furthermore, the free-ranging population is being increasingly impacted by habitat loss due to agricultural activities.
Captive mountain gazelles are located at the Hatay Mountain Gazelle Production Center, which is located within a 12-ha fenced site within the research area. During sampling, there were 39 females and 11 males within a 12-ha region. The Hatay Mountain Gazelle Production Center was established to support the existing small population and to reintroduce mountain gazelles to suitable areas where they were previously distributed. However, this goal has not yet been achieved. The center also cares for injured animals and newborn gazelles abandoned by their mothers. Animals from the Center are not released back into the wild because they were habituated to humans, particularly the centers’ care staff. In this area, members of the captive population reproduce, and the offspring remain with their parents, continuing to inhabit the same area without separation. In rare but necessary circumstances (injuries, infections, etc.), veterinary care is provided. Other than these unique situations, there is no veterinary care. For captive individuals, animal care staff provide additional fodder. The captive population has feeding and watering troughs year-round. Due to the presence of fences, the area is partially closed to animal entry; however, animal care staff have observed red foxes occasionally breaching the fence. Additionally, hundreds of visitors visit this center every month.
2.3 Sampling
Our sampling schedule included all seasons that matched the biological cycle of Gazella gazella. Field sampling was performed in December 2022 (mating period), April 2023 (gestation period), July 2023 (time of birth and lactation period) and September 2023 (nonreproductive period). We collected 262 fecal samples in total. The number of collected samples in each season is shown in Table 3.
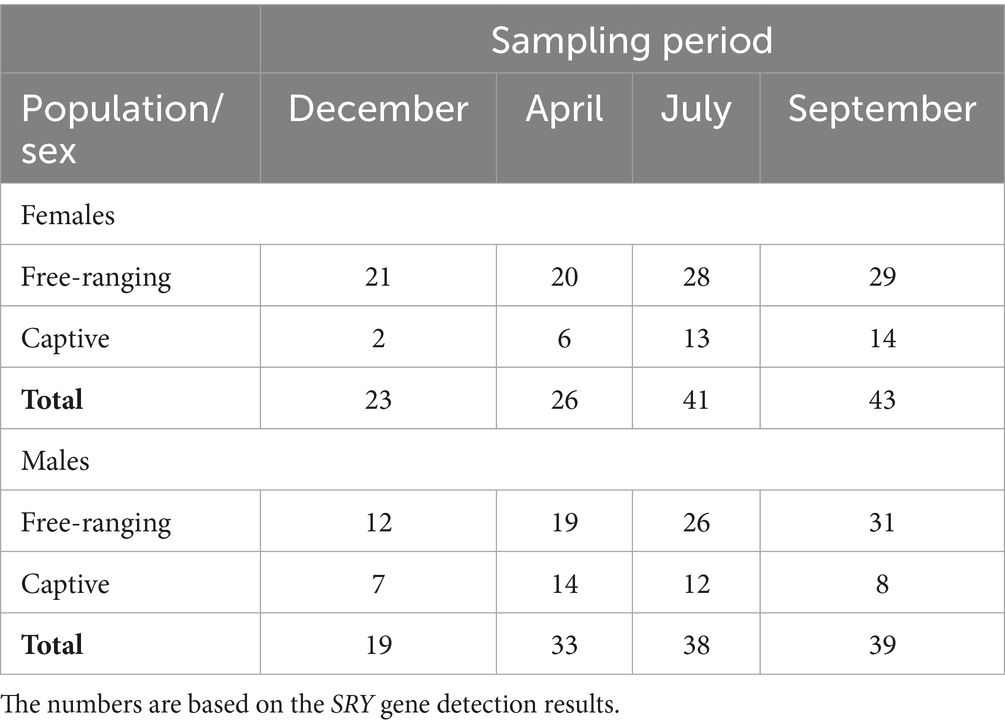
Table 3. Number of female and male samples included in the study during the sampling periods in the captive and free-ranging populations.
Before the fecal samples of mountain gazelles were collected from the ground, each individual was observed with binoculars from a minimum distance of 500 m for the free-ranging population and at a distance of 50 m for the captive population. When a group or individual defecated, the area was visually inspected (either by car or on foot), and fecal samples were collected as quickly as possible. Approximately 7 g of each fecal sample was collected from the ground and stored in polypropylene tubes, which were kept in a cooler (+4°C) during transportation to the laboratory.
2.4 Sex determination
The sex of the animals was determined by SRY gene detection in the DNA isolated from the fecal samples. Out of the 262 analyzed fecal samples, 133 were from females and 129 were from males (Table 3; Supplementary material). The results show the expected proportions and enable the formation of the animal groups studied.
For the preextraction procedure, all the samples were kept at −20°C. Before DNA extraction, each sample was weighed individually on a precision scale of 180–220 mg and transferred to 2 mL Eppendorf tubes with sample numbers written on them. Following preparation, the DNA extraction process was carried out, and genomic DNA was extracted from all 262 fecal samples. DNA was isolated using the QIAmp Fast DNA Stool Mini Kit (Qiagen, Hilden, Germany) according to the manufacturer’s instructions. After extraction, to detect sex, we used the SRY gene, which is the Y chromosomal gene that determines sex (37). PCR products were amplified in a 13.25 mL volume containing 11.25 mL of Platinum Supermix (Platinum PCR 2X MM; Invitrogen, Waltham, United States), 0.5 μL of each primer (diluted 10 pmol/μL) and 1 mL of template DNA. DNA was amplified via PCR using the following primers: SRY-BF (5′-GCA GCT GGG ATA TGA GTG GAA AAG-3′) and SRY-BR (3′-GCA GCT GGG ATA TGA GTG GAA AAG-5′). Primers flanking a target ruminant SRY gene were designed based on the NCBI GenBank reference assembly using Primer3 (38). PCR was performed using a SimpliAmp™ Thermal Cycler (Applied Biosystems, Waltham, United States) in a three-step process: first, denaturation for 10 min at 94°C, followed by 35 cycles of 30 s at 94°C, 30 s at 53°C, 1 min at 72°C, and finally, elongation for 10 min at 72°C. The PCR products were visualized by gel electrophoresis (2% agarose, TAE buffer). The expected amplicon size was 220 bp. We considered the presence of a band in a PCR result to be indicative of the sample belonging to a male; if no band was visible for the PCR product, we regarded this as indicating that the sample belonged to a female.
2.5 Measurement of the FGM concentrations in fecal samples
The extraction of FGM from fecal samples was performed according to previously described procedures (39–41) with slight modifications. The extraction was performed following this protocol. Approximately 2 g of each fecal sample was dried at 50°C for 24 h. A total of 0.5 g of the dry fecal sample was weighed and placed into a plastic tube. Five millilitres of absolute methanol was added, and the mixture was vortexed for 15 s and horizontally shaken at 300 rpm for 30 min. The extracts were centrifuged for 15 min at 4°C and 3,500 × g, after which two millilitres of the extract was added to a 4 mL tube. The extracts were dried under a stream of nitrogen at 40°C (nitrogen evaporator N-EVAP, Organomation Associates INC., Berlin, United States), and the dried extracts were dissolved in 1 mL of PBS. The dissolved samples were centrifuged for 15 min at 4°C and 3,500 × g. The supernatants were pipetted into 2 ml Eppendorf tubes and stored at −20°C until analysis.
The FGM concentrations in the fecal extracts were measured using a commercial ELISA kit (Demeditec, Kiel, Germany; ref. DES6611) following the manufacturer’s instructions. The absorbance was measured at 450 nm using a Multiskan FC microtiter plate reader (Thermo Fisher Scientific, Waltham, United States). The results obtained in ng FGM per mL extract were converted to ng FGM per g dry feces.
Prior to the measurement of the FGM concentrations in the fecal samples, partial analytical validation of the measurement procedure was performed. The validation included the calculation of intra- and interassay coefficients of variation (CVs) and the determination of recovery rates. Two samples of fecal extracts were run 16 times in one assay and repeated 4 times in the next assay. The recovery rate was determined by adding two known amounts of hydrocortisone (Sigma–Aldrich, St. Louis, United States) to two fecal samples before extraction. The FGM concentrations were also determined in these two fecal samples. The intra- and inter-CVs for the first sample were 8.72 and 13.95%, respectively, and those for the second sample were 7.62 and 12.20%, respectively. The recovery rates for the two samples were 88.6 and 85.2%, respectively. The data on the specificity of the method are given by the manufacturer of the ELISA kit. The cross-reactivity with other steroids was as follows (in %): testosterone <0.1, corticosterone 6.2, cortisone 0.8, 11-deoxycorticosterone 2.6, 11-deoxycortisol 50, dexamethasone <0.1, estriol <0.1, estrone 0.1, prednisolone 100, prednisone 0.9, progesterone <0.1, 17-hydroxyprogesterone 1.3, danazole <0.1, pregnenolone <0.1, estradiol <0.1, androstenedione <0.1. The abovementioned 11-deoxycortisol is one of the main FGMs (42). The range of the assay was between 1 and 300 ng/g.
In addition, biological evaluation of the FGM measurements was performed as demonstrated by Palme (7). The same fecal samples used for measurements of the FGM concentration in this study were also used to measure the fecal progesterone metabolite concentrations (Table 4). In April and July, we found high fecal progesterone metabolite concentrations (more than 4,000 ng/g) in some females. At this time, females are at the last phase of pregnancy, and high progesterone metabolite concentrations are most likely the result of pregnancy. Since females in the last stage of pregnancy are known to have elevated blood cortisol concentrations, we used 4 samples with high fecal progesterone metabolite concentrations for the biological evaluation of FGM measurements. In addition, we used four male samples collected in April with low fecal progesterone metabolite concentrations. Since April is not a mating season, the males remain in small groups or are solitary, which is why we expected lower FGM concentrations, as in pregnant females.
2.6 Statistics
To analyze the effects of sex (male versus female), season (four biological periods), and population (free-ranging versus captive) on FGM concentrations in G. gazella individuals, we performed analysis of variance (ANOVA) considering these factors as fixed factors. In this analysis, we tested each factor and the interactions between factors against a null model to obtain F and p values. In the case of the season, a Tukey HSD post hoc test was also performed to assess significant differences between seasons. The FGM concentration data were log-transformed before the analyses to ensure normality and heteroscedasticity. Additional analyses were also performed to determine the separate effects of sex on FGM concentrations by performing two linear model analyses for each sex, in which season and population were included as fixed factors. All the statistical analyses were conducted in the R environment (43).
3 Results
There was a statistically significant difference in FGM concentrations among seasons (p < 0.0001); however, the FGM concentrations did not significantly differ between sexes or between captive and free-ranging populations (p > 0.05). When season was accounted for, however, the interactions between sex and population with season became statistically significant (p < 0.0001; Table 5). These results indicated that season is the most important factor explaining the variability in FGM concentrations in mountain gazelles. Additionally, we also found a significant difference in FGM concentrations between free-ranging and captive individuals in September (p = 0.0003) and a slight difference in FGM concentrations between these populations in July (p = 0.061). Notably, the difference in September was especially due to the increased FGM concentrations in females (p = 0.016) but not in males (p = 0.173; Figures 1, 2).
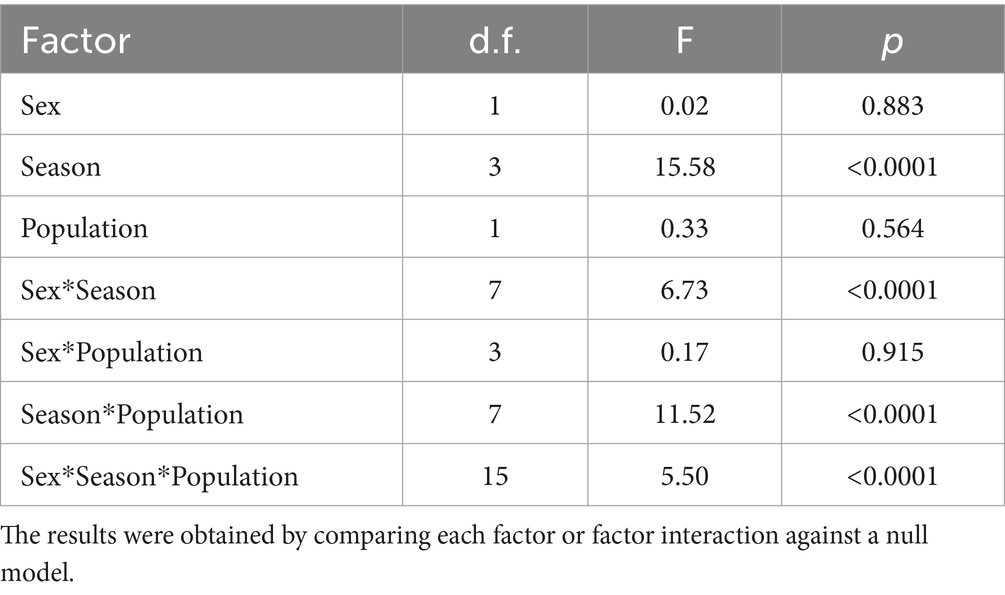
Table 5. Summary of the linear model analysis testing the effect of sex, season, and population on the fecal glucocorticoid metabolite concentrations in Gazella gazella.
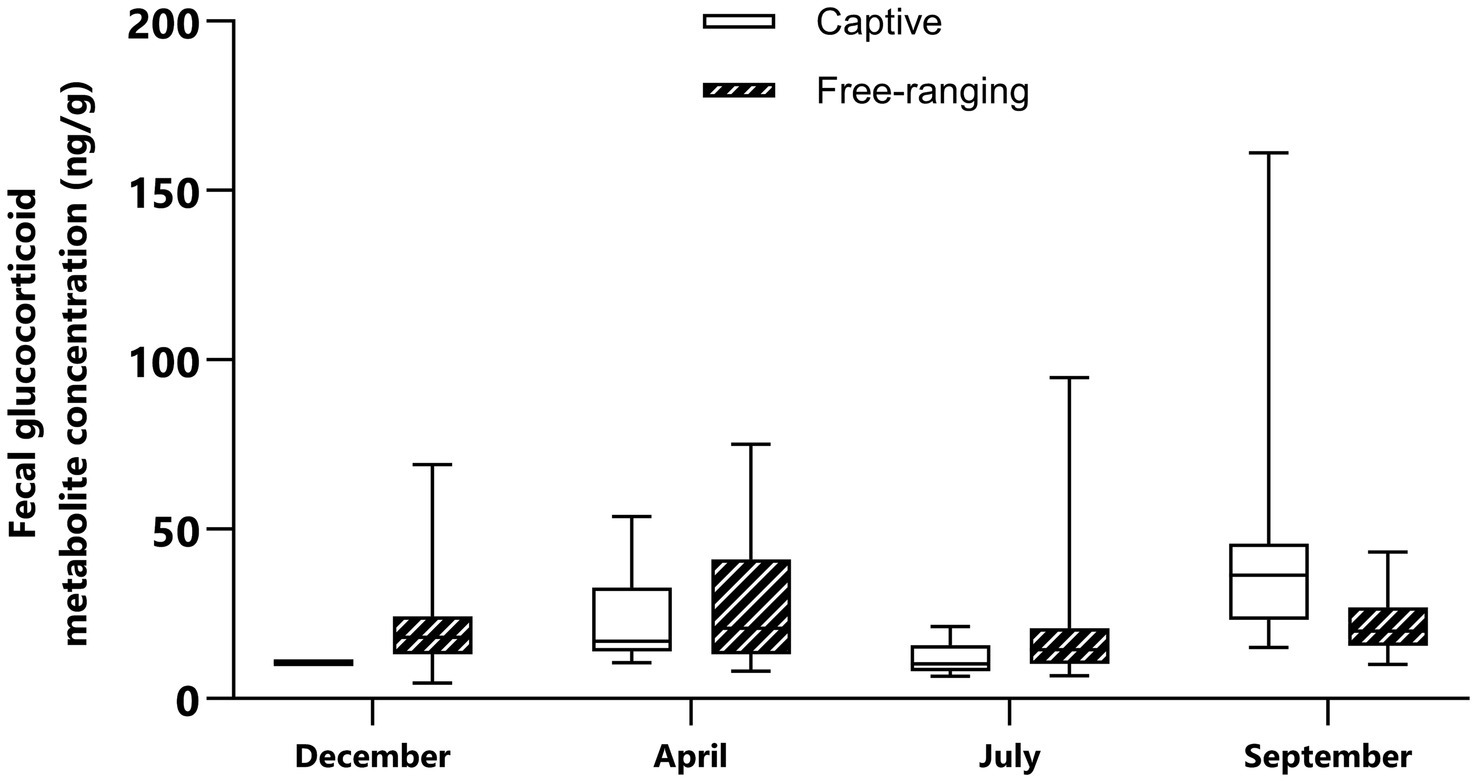
Figure 1. Median and interquartile range of the fecal glucocorticoid metabolite concentrations in captive and free-ranging female mountain gazelles (Gazella gazella) during different seasons.
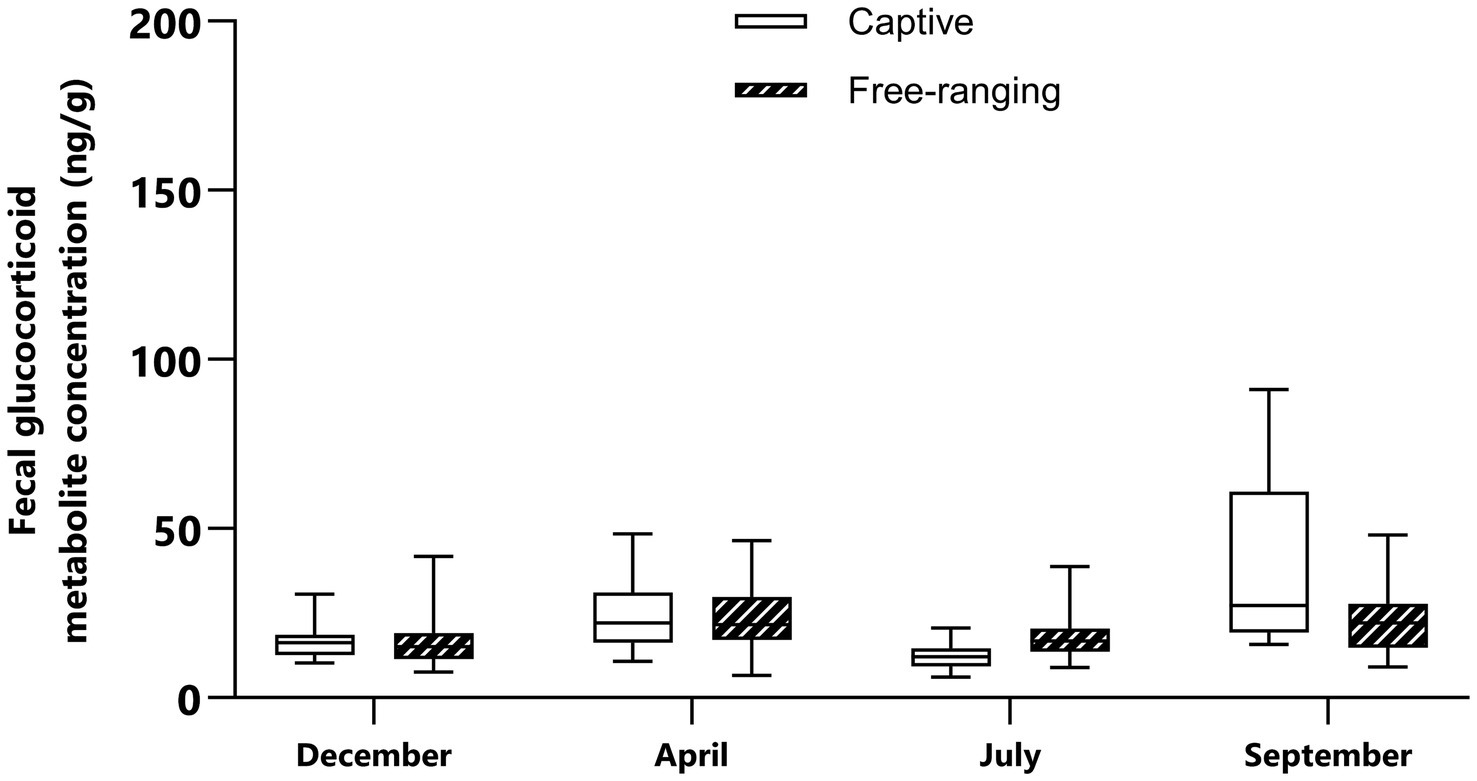
Figure 2. Median and interquartile range of the fecal glucocorticoid metabolite concentrations in captive and free-ranging male mountain gazelles (Gazella gazella) during different seasons.
There were significant differences in FGM concentrations among female gazelle seasons (Figure 1), specifically between September and July (p < 0.0001), between September and December (p = 0.032), and between April and July (p = 0.012). Among the captive female animals, the highest concentration was found in September, which was significantly higher than that in December (p = 0.048) and July (p < 0.0001). However, there was no significant difference in the FGM concentrations in free-ranging females among the seasons (p > 0.05).
There were significant differences (p < 0.05) in FGM concentrations between male gazelle and female gazelle in the same seasons. In captive male animals, the highest concentration was found in September, and this value was significantly higher than the values in December (p = 0.046) and July (p < 0.0001). In free-ranging males, however, there was no significant difference in the FGM concentrations between seasons (p > 0.05) (Table 6). The raw data on the FGM concentrations are shown in the Supplementary material.
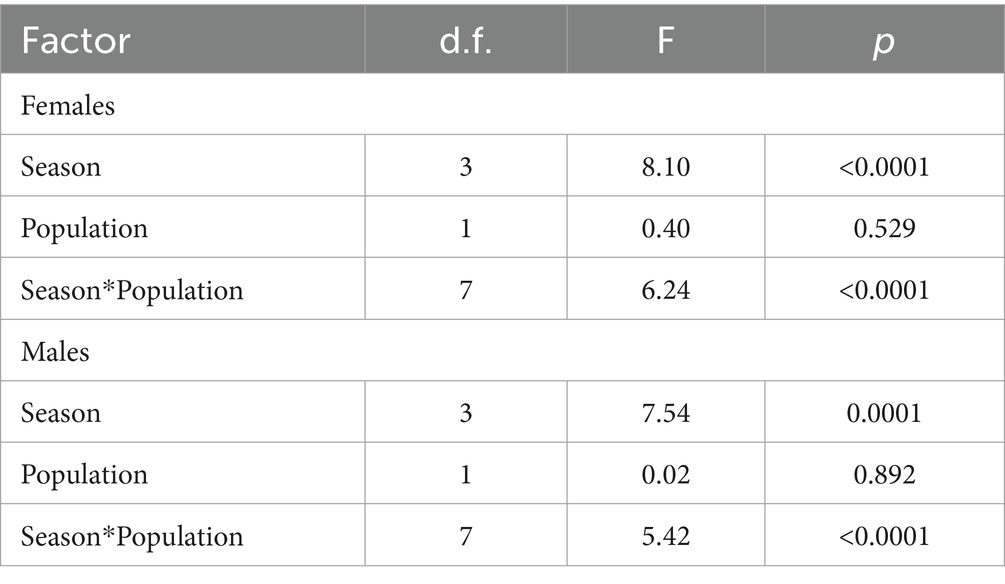
Table 6. Summary of the linear model analysis testing the effect of season and population on the fecal glucocorticoid metabolite concentrations in Gazella gazella of both sexes.
4 Discussion
In this study, we aimed to gain insight into adrenocortical activity during the circannual cycle by measuring FGM concentrations in mountain gazelles. Two gazelle populations, free-ranging and captive, living under the same macroinveronmental conditions were investigated to determine how a population-specific microenvironment can influence their adrenocortical response. The assessment of FGM concentrations in mountain gazelles revealed seasonal fluctuations, as was also reported for other ruminants (24–27). Although several endogenous and exogenous factors influence adrenocortical response changes across seasons, it is difficult to identify the factors that are more important or less important for the adrenocortical response. We believe that the combination of all exogenous and endogenous factors that have a positive or negative effect on an individual can lead to adaptation processes that are controlled by the adrenocortical response. The well-being of an animal is ultimately a consequence of the sum of all negative and positive factors affecting it.
In the free-ranging population and captive population, lower FGM concentrations were detected in December than in April and September (Figures 1, 2). In December, vegetation provides sufficient high-quality forage feed for both populations. We also assumed that most females were at the beginning of their pregnancy at this time. Considering this, it seems that gazelles in December are not subjected to significant climatic and reproductive events, and their adaptation processes do not require a significant adrenocortical response. During this period, our study also revealed no significant differences in FGM concentrations between captive and free-ranging populations; thus, although there were some differences in the microenvironment (Table 2), these differences had no effect on the intensity of the adaptive processes assessed by FGM concentrations.
In April, the FGM concentrations were higher than those in December and July (Figures 1, 2). At this time, most of the females were in the 4th or 5th month of pregnancy. It is important to highlight that despite the lack of statistically significant differences in females between April and other studied seasons, many samples with higher FGM concentrations were identified during the analysis of April sample data. As supported by previous studies in wild ungulates, glucocorticoid concentrations increase in the late phase of pregnancy (44, 45). FGM concentrations are higher in the later phases of pregnancy because the production of glucocorticoids increases to meet the increased energy demand during intense fetal growth and because of intensive glucocorticoid synthesis by the fetus (46, 47). However, in some fecal samples, FGM concentrations were low, and these samples most likely represented early pregnant or nonpregnant females. When we compared the FGM concentrations of captive and free-ranging mountain gazelle in April, we did not observe a significant difference. Nonterritorial males from both populations form bachelor groups, while territorial males in each population live solitarily during this period. Since it has been reported that the adrenocortical response differs between territorial and nonterritorial males and that concentrations of fecal cortisol metabolites are higher in nonterritorial males (48), this could be the reason for the considerable variation in our data, the inconsistent results and the lack of significant differences.
In both populations, FGM concentrations were lowest in July compared to those in the other sampling periods. At this time of year, females and fawns stay in groups, while males remain solitary or in bachelor groups; however, some females are still pregnant. Although the ambient temperature was high in July, this apparently did not cause an intensive adrenocortical response. There are no published data on the thermoneutral zone of mountain gazelles, but it is likely that such environmental conditions are favorable for this species and that high temperatures do not cause heat stress. However, higher FGM concentrations were observed in the free-ranging population than in the captive population. The difference was very close to statistical significance (p = 0.061). The reason for this could be that weather conditions (Table 1) lead to limited food and water availability, which could be the reason for the slightly higher FGM concentrations in the free-ranging population. In contrast, the captive population has constant access to high-quality food and water, which may decrease the adrenocortical response of the animals. Furthermore, predators are more likely to exploit the presence of mothers with offspring, as they are less capable of detecting and avoiding predators, as has been reported for red deer (Cervus elaphus) (49), Przewalski’s gazelle (Procapra przewalskii) (50), Apennine chamois (Rupicapra pyrenaica) (51), fallow deer Dama dama (52) and roe deer (Capreolus capreolus) (53). In the captive population, fences provide protection and a safe place for mothers and fawns, which may also be reflected in lower FGM concentrations.
In September, FGM concentrations were found higher than those in the other seasons. In the captive population, FGM concentrations were high compared to those in other seasons (Figures 1, 2). According to research conducted on captive populations of G. mohor, G. cuvieri and G. dorcas, when G. cuvieri males were alone, higher glucocorticoid levels were detected, and they had lower glucocorticoid levels when they were with other males. G. dorcas and G. mohor males had higher glucocorticoid levels than females and lower values when they were alone (54). Therefore, it seems that the adrenocortical response depends on the species or even on the individual. Since we could not categorize and evaluate singleton individuals in the captive male populations, the reason for the high FGM concentrations measured in the captive populations in our study is not clear. In addition, the FGM concentrations in captive animals were significantly higher than those in free-ranging populations (p = 0.0003), since September is the month with the highest number of visitors. However, in the area of the captive population, the highest number of visitors was recorded in September. This could be a challenge and could be the reason for the high concentrations of FGM in the captive population.
When comparing captive and free-ranging populations, we generally expected higher FGM concentrations in captive animals, as several studies have shown similar results in some herbivores and carnivores (9), such as spotted hyenas (Crocuta crocuta) (55, 56), cheetah (Acinonyx jubatus) (57), fallow deer (Dama dama) (58, 59), and Canada lynx (Lynx canadensis) (60). The FGM concentration in captive animals could increase due to limited space, more socio-negative interactions, and the presence of humans. Our study revealed that the reactions of mountain gazelles are different. Compared to those in the free-ranging population, we found lower FGM concentrations in the captive population in December, April and July. We believe that for gazelle captivity offers several advantages, such as constant feed and water availability and protection from predators because of the protection provided by fences and animal care staff. As observed during fieldwork, some captive gazelles had no difficulties contacting humans, as some individuals were habituated and sought contact with humans (Figure 3), which has not been observed in free-ranging gazelles. It seems that contact with the care staff does not cause an intense adrenocortical response in captive mountain gazelles; on the other hand, groups of visitors may be the reason for this. Moreover, a constant supply of feed and water might have been particularly beneficial.
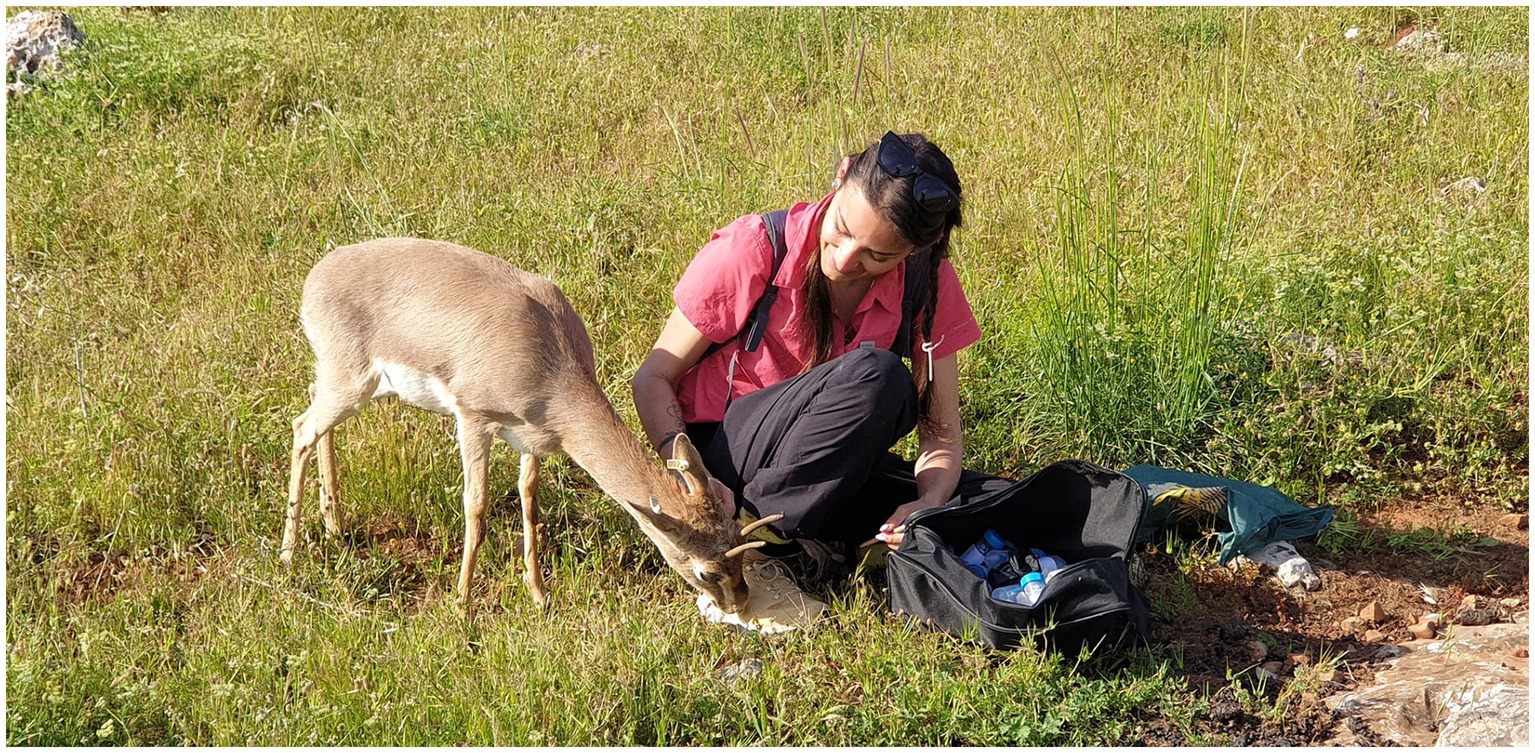
Figure 3. A female mountain gazelle, transferred to the Hatay Mountain Gazelle Production Center at an early age, was habituated to human presence.
Since we were not able to track all the endogenous and exogenous factors that influence the response of the HPA axis, only internal factors, such as sex and the phase of the biological cycle, and external factors, such as food and water availability, intraspecific conflict, the presence of humans, human care and climate conditions, were considered and discussed. We are aware that the exact status of the animals examined is not known in detail. Although we have determined sex, several factors, such as age, health and reproductive status, as well as several external factors, remain unknown and have therefore not been accounted for when interpreting the results. We believe that these factors may also influence the activity of the HPA axis and could be a reason for the inconsistent data.
Although our study revealed seasonal variation in the adrenocortical response in mountain gazelles, there are some shortcomings that need to be addressed in future studies. For instance, our sampling schedule included only four samplings over 1 year. Consequently, the results do not provide insight into HPA axis activity and adrenocortical response between sampling periods. In addition, we did not identify the individual animals in our study, so age, health status, reproductive status, and social status were unknown. Future research should collect these data and take them into account when interpreting the results. Moreover, we conducted a partial analytical validation and biological evaluation of the FGM detection method for this study. However, for ethical and conservation reasons, pharmacological validation of the detection method was not performed, as it requires an ACTH challenge test and a dexamethasone suppression test (7), which cannot be performed on individuals of endangered species. However, a detailed analytical validation together with biological and pharmacological validation would increase the reliability of the detection method used.
In conclusion, FGM concentrations in mountain gazelles are subjected to seasonal fluctuations. The results suggest that the limited availability of food and water in summer can be regarded as a critical point for the free-ranging population. High visitor numbers can disturb the captive population. FGM concentrations do not differ between the sexes.
Data availability statement
The original contributions presented in the study are included in the article/Supplementary material, further inquiries can be directed to the corresponding author.
Ethics statement
Ethical approval was not required for the study involving animals in accordance with the local legislation and institutional requirements because the fecal samples were collected from the ground, there was no direct contact with the animals. The work was approved by the General Directorate of Nature Conservation and National Parks of Türkiye with a document no: 8037146, dated 08.12.2022. Written informed consent was obtained from the individual(s) for the publication of any potentially identifiable images or data included in this article.
Author contributions
MCK: Conceptualization, Formal analysis, Investigation, Writing – original draft, Writing – review & editing. TK: Investigation, Supervision, Writing – review & editing. ÇT: Conceptualization, Formal analysis, Investigation, Writing – original draft, Writing – review & editing. MC: Investigation, Writing – review & editing, Methodology. NČK: Investigation, Writing – review & editing. AD: Formal analysis, Writing – review & editing, Data curation. TS: Formal analysis, Writing – review & editing, Conceptualization, Investigation, Writing – original draft.
Funding
The author(s) declare financial support was received for the research, authorship, and/or publication of this article. The work was financially supported by the Slovenian Research and Innovation Agency Research Program P4-0053 and Hacettepe University Scientific Research Unit (grant no: FUK-2023-20841).
Acknowledgments
The authors would like to thank Katarina Babnik, Magdalena Dobravec, Boštjan Drolc, and Tilen Vake for their technical and professional support. We also thank the Provincial Directorate of Nature Conservation and National Parks of Hatay for permissions, and the Hatay Nature Conservation Association, especially Abdullah Öğünç, for their logistic support. The fieldwork of the study was conducted with the permission of the General Directorate of Nature Conservation and National Parks of Türkiye (no: 8037146, date: 08.12.2022). This study is a part of the PhD dissertation of the first author. The English was edited by the American Journal Experts.
Conflict of interest
The authors declare that the research was conducted in the absence of any commercial or financial relationships that could be construed as a potential conflict of interest.
Publisher’s note
All claims expressed in this article are solely those of the authors and do not necessarily represent those of their affiliated organizations, or those of the publisher, the editors and the reviewers. Any product that may be evaluated in this article, or claim that may be made by its manufacturer, is not guaranteed or endorsed by the publisher.
Supplementary material
The Supplementary material for this article can be found online at: https://www.frontiersin.org/articles/10.3389/fvets.2024.1386009/full#supplementary-material
References
1. Yom-Tov, Y, Balaban, A, Hadad, E, Weil, G, and Roll, U. The plight of the endangered mountain gazelle Gazella gazella. Oryx. (2021) 55:771–8. doi: 10.1017/S003060531900108X
2. Munro, ND, Lebenzon, R, and Sapir-Hen, L. Revisiting late Pleistocene-early Holocene mountain gazelle (Gazella gazella) body size change in the southern Levant: a case for anthropogenic impact. PLoS One. (2022) 17:e0273024. doi: 10.1371/journal.pone.0273024
3. Hadas, L, Hermon, D, Boldo, A, Arieli, G, Gafny, R, King, R, et al. Wild gazelles of the southern Levant: genetic profiling defines new conservation priorities. PLoS One. (2015) 10:e0116401. doi: 10.1371/journal.pone.0116401
4. İlaslan, E. Türkiye'de yayılış gösteren Gazella gazella (Pallas, 1766) türünün filocoğrafyası. MS thesis. Aksaray Üniversitesi Fen Bilimleri Enstitüsü, Aksaray, Türkiye (2019).
5. IUCN SSC Antelope Specialist Group . Gazella gazella. In: The IUCN red list of threatened species 2017:e.T8989A50186574 International Union for Conservation of Nature (2017). Available at: https://www.iucnredlist.org/species/8989/50186574
6. Fernandes, DRN, and Pinto, MDLR. Veterinarian’s role in conservation medicine and animal welfare. Vet Anat Physiol. (2019):119–33. doi: 10.5772/intechopen.84173
7. Palme, R . Non-invasive measurement of glucocorticoids: advances and problems. Physiol Behav. (2019) 199:229–43. doi: 10.1016/j.physbeh.2018.11.021
8. Dantzer, B, Fletcher, QE, Boonstra, R, and Sheriff, MJ. Measures of physiological stress: a transparent or opaque window into the status, management and conservation of species. Conserv Physiol. (2014) 2:cou023. doi: 10.1093/conphys/cou023
9. Karaer, MC, Čebulj-Kadunc, N, and Snoj, T. Stress in wildlife: comparison of the stress response among domestic, captive, and free-ranging animals. Front Vet Sci. (2023) 10:1167016. doi: 10.3389/fvets.2023.1167016
10. Fuller, A, Maloney, SK, Blache, D, and Cooper, C. Endocrine and metabolic consequences of climate change for terrestrial mammals. Curr Opin Endocrinol Diabetes Obes. (2020) 11:9–14. doi: 10.1016/j.coemr.2019.12.003
11. Johnstone, CP, Lill, A, and Reina, RD. Habitat loss, fragmentation and degradation effects on small mammals: analysis with conditional inference tree statistical modelling. Biol Conserv. (2014) 176:80–98. doi: 10.1016/j.biocon.2014.04.025
12. Carroll, C . Interacting effects of climate change, landscape conversion, and harvest on carnivore populations at the range margin: marten and lynx in the northern Appalachians. Biol Conserv. (2007) 21:1092–104. doi: 10.1111/j.1523-1739.2007.00719.x
13. Mathews, F . Wild animal conservation and welfare in agricultural systems. Anim Welf. (2010) 19:159–70. doi: 10.1017/S096272860000141X
14. Preeti, JKR, Thakur, M, Suman, M, and Kumar, R. Consequences of pollution in wildlife: a review. Pharma Innov J. (2018) 7:94–102.
15. Arcangeli, G, Lulli, LG, Traversini, V, De Sio, S, Cannizzaro, E, Galea, RP, et al. Neurobehavioral alterations from noise exposure in animals: a systematic review. Int J Environ Res Public Health. (2022) 20:591. doi: 10.3390/ijerph20010591
16. Bennie, J, Davies, TW, Cruse, D, Inger, R, and Gaston, KJ. Cascading effects of artificial light at night: resource-mediated control of herbivores in a grassland ecosystem. Philos Trans R Soc Lond B Biol Sci. (2015) 370:20140131. doi: 10.1098/rstb.2014.0131
17. Kankiliç, T, Özüt, D, Gürler, Ş, Kence, M, Bozkaya, F, and Kence, A. Rediscovery of a new mountain gazelle population and clarification of taxonomic status of the genus Gazella in Turkey using mtDNA sequencing. Folia Zool. (2012) 61:129–37. doi: 10.25225/fozo.v61.i2.a6.2012
18. Zerek, A, Erdem, İ, Yaman, M, Aydenizöz, M, Şimşek, FN, and Altuğ, ME. Parasites detected in Hatay Mountain gazelles (Gazella gazella). Uluslararası Tarım ve Yaban Hayatı Bilimleri Dergisi. (2022) 8:520–7. doi: 10.24180/ijaws.1122022
19. Karaer, MC, Sönmez, Hİ, Madak, E, Kankılıç, T, Tavşanoğlu, Ç, and Sarımehmetoğlu, HO. Helminths of captive and free-ranging populations of the mountain gazelle (Gazella gazella): evidence from fecal examination. Vet Med Sci. (2024) 10:e1429. doi: 10.1002/vms3.1429
20. Çoğal, M, and Sözen, M. The status and distribution of Gazella gazella (Artiodactyla: Mammalia), and other mammals of the Hatay province in southern Turkey. Isr J Ecol Evol. (2017) 63:44–9. doi: 10.1163/22244662-06301003
21. Akman, B, Biler, L, Bilgin, C, Çoğal, M, Kılın, N, and Muratlı, S. Hatay dağ ceylanı (Gazella Gazella) türünün Hatay ili sınırlarında yaşayan popülasyonlarının ve yaşam alanlarının korunmasına yönelik tür eylem planı, 7, T.C. Orman ve Su Işleri Bakanlığı. (2017). p. 2–20. Doğa Koruma ve Milli Parklar Genel Müdürlüğü Beştepe Mah.
22. Nilssen, KJ, Bye, K, Sundsfjord, JA, and Blix, AS. Seasonal changes in T3, FT4, and cortisol in free-ranging Svalbard reindeer (Rangifer tarandus platyrhynchus). Gen Comp Endocrinol. (1985) 59:210–3. doi: 10.1016/0016-6480(85)90371-5
23. Liu, R, Shi, J, Liu, D, Dong, S, Zhang, Y, Wu, Y, et al. Effect of group size and reproductive status on faecal glucocorticoid concentration and vigilance in a free-ranging population of Przewalski’s gazelle. Conserv Physiol. (2020) 8:coaa027. doi: 10.1093/conphys/coaa027
24. Ogino, M, Matsuura, A, Yamazaki, A, Irimajiri, M, Suzuki, Y, Kushibiki, S, et al. Plasma cortisol and prolactin secretion rhythms in cattle under varying external environments and management techniques. Anim Sci. (2014) 85:58–68. doi: 10.1111/asj.12090
25. Snoj, T, Jenko, Z, and Cebulj-Kadunc, N. Fluctuations of serum cortisol, insulin and non-esterified fatty acid concentrations in growing ewes over the year. Ir Vet J. (2014) 67:22. doi: 10.1186/2046-0481-67-22
26. Chergui, N, Mormède, P, Foury, A, Khammar, F, and Amirat, Z. Seasonal effects on plasma cortisol concentrations in the Bedouin buck: circadian studies and response to ACTH. Animal. (2017) 11:445–51. doi: 10.1017/S1751731116001671
27. Huber, S, Palme, R, and Arnold, W. 2003 effects of season, sex, and sample collection on concentrations of fecal cortisol metabolites in red deer (Cervus elaphus). Gen Comp Endocrinol. (2003) 130:48–54. doi: 10.1016/s0016-6480(02)00535-x
28. Jachowski, DS, Washburn, BE, and Millspaugh, JJ. Revisiting the importance of accounting for seasonal and diel rhythms in fecal stress hormone studies. Wildl Soc Bull. (2015) 39:738–45. doi: 10.1002/wsb.592
29. Bärmann, EV, Rössner, GE, and Wörheide, G. A revised phylogeny of Antilopini (Bovidae, Artiodactyla) using combined mitochondrial and nuclear genes. Mol Phylogenet Evol. (2013) 67:484–93. doi: 10.1016/j.ympev.2013.02.015
30. Baharav, D . Notes on the population structure and biomass of the mountain gazelle, Gazella gazella gazella. Isr J Ecol Evol J. (1974) 23:39–44. doi: 10.1080/00212210.1974.10688395
31. Mohammed, OB, Green, DI, and Holt, WV. Fecal progesterone metabolites and ovarian activity in cycling and pregnant mountain gazelles (Gazella gazella). Theriogenology. (2011) 75:542–8. doi: 10.1016/j.theriogenology.2010.09.023
32. Mendelssohn, H, Yom-Tov, Y, and Groves, CP. Gazella gazella. Mamm Species. (1995) 490:1–7. doi: 10.2307/0.490.1
33. Geffen, H, Perevolotsky, A, Geffen, E, and Yom-Tov, Y. Use of space and social organization of female mountain gazelles (Gazella gazella gazella) in Ramat HaNadiv, Israel. J Zool. (1999) 247:113–9. doi: 10.1111/j.1469-7998.1999.tb00198.x
34. Wronski, T, Apio, A, Plath, M, and Ziege, M. Sex difference in the communicatory significance of localized defecation sites in Arabian gazelles (Gazella arabica). J Ethol. (2013) 31:129–40. doi: 10.1007/s10164-012-0357-6
35. Dunham, KM . The social organization of mountain gazelles Gazella gazella in a population reintroduced to Central Arabia. J Arid Environ. (1999) 43:251–66. doi: 10.1006/jare.1999.0528
36. Shalmon, B, and Wronski, T. The conservation history of Arabian gazelles (Gazella arabica) in Israel – do fifty-five years monitoring help to define future incentives In: SW Hung , editor. Animal Science. Reno: MedDocs Publishers (2021). 21–38.
37. Wallis, MC, Waters, PD, and Graves, JAM. Sex determination in mammals—before and after the evolution of SRY. Cell Mol Life Sci. (2008) 65:3182–95. doi: 10.1007/s00018-008-8109-z
38. Untergasser, A, Cutcutache, I, Koressaar, T, Ye, J, Faircloth, BC, Remm, M, et al. Primer3—new capabilities and interfaces. Nucleic Acids Res. (2012) 40:e115–5. doi: 10.1093/nar/gks596
39. Schwarzenberger, F, Mostl, E, Bamberg, E, Pammer, J, and Schmehlik, O. Concentrations of progestagens and oestrogens in the faeces of pregnant Lipizzan, trotter and thoroughbred mares. J Reprod Fertil Suppl. (1991) 44:489–99.
40. Wasser, SK, Hunt, KE, Brown, JL, Cooper, K, Crockett, CM, Bechert, U, et al. A generalized fecal glucocorticoid assay for use in a diverse array of nondomestic mammalian and avian species. Gen Comp Endocrinol. (2000) 120:260–75. doi: 10.1006/gcen.2000.7557
41. Mostl, E, and Palme, R. Hormones as indicators of stress. Domest Anim Endocrinol. (2002) 23:67–74. doi: 10.1016/S0739-7240(02)00146-7
42. Kothmann, KH, Jons, A, Wilhelmi, B, Kasozi, N, Graham, L, Gent, R, et al. Non-invasive assessment of fecal glucocorticoid, progesterone, and androgen metabolites and microbiome in free-ranging southern white rhinoceros (Ceratotherium simum simum) in South Africa. Gen Comp Endocrinol. (2022) 329:114099. doi: 10.1016/j.ygcen.2022.114099
43. R Core Team. R: a language and environment for statistical computing. Vienna, Austria: R Foundation for Statistical Computing (2021) Available at: https://www.R-project.org/.
44. Caslini, C, Comin, A, Peric, T, Prandi, A, Pedrotti, L, and Mattiello, S. Use of hair cortisol analysis for comparing population status in wild red deer (Cervus elaphus) living in areas with different characteristics. Eur J Wildl Res. (2016) 62:713–23. doi: 10.1007/s10344-016-1049-2
45. Ventrella, D, Elmi, A, Bertocchi, M, Aniballi, C, Parmeggiani, A, Govoni, N, et al. Progesterone and cortisol levels in blood and hair of wild pregnant red deer (Cervus elaphus) hinds. Animals. (2020) 10:143. doi: 10.3390/ani10010143
46. Wieczorek, A, Perani, CV, Nixon, M, Constancia, M, Sandovici, I, Zazara, DE, et al. Sex-specific regulation of stress-induced fetal glucocorticoid surge by the mouse placenta. Am J Physiol Endocrinol Metab. (2019) 317:E109–20. doi: 10.1152/ajpendo.00551.2018
47. Solano, ME, and Arck, PC. Steroids, pregnancy and fetal development. Front Immunol. (2020) 10:3017. doi: 10.3389/fimmu.2019.03017
48. Corlatti, L, Palme, R, and Lovari, S. Physiological response to etho-ecological stressors in male alpine chamois: timescale matters! Sci Nat. (2014) 101:577–86. doi: 10.1007/s00114-014-1195-x
49. Childress, MJ, and Lung, MA. Predation risk, gender and the group size effect: does elk vigilance depend upon the behaviour of conspecifics? Anim Behav. (2003) 66:389–98. doi: 10.1006/anbe.2003.2217
50. Li, C, Jiang, Z, Feng, Z, Yang, X, Yang, J, and Chen, L. Effects of highway traffic on diurnal activity of the critically endangered Przewalski’s gazelle. Wildl Res. (2009) 36:379–85. doi: 10.1071/WR08117
51. Fattorini, N, Lovari, S, Brunetti, C, Baruzzi, C, Cotza, A, Macchi, E, et al. Age, seasonality, and correlates of aggression in female Apennine chamois. Behav Ecol Sociobiol. (2018) 72:1–17. doi: 10.1007/s00265-018-2584-5
52. Pecorella, I, Fattorini, N, Macchi, E, and Ferretti, F. Sex/age differences in foraging, vigilance and alertness in a social herbivore. Acta Ethol. (2019) 22:1–8. doi: 10.1007/s10211-018-0300-0
53. Fattorini, N, and Ferretti, F. To scan or not to scan? Occurrence of the group-size effect in a seasonally nongregarious forager. Ethology. (2019) 125:263–75. doi: 10.1111/eth.12844
54. Arregui, L, Garde, JJ, Soler, AJ, Espeso, G, and Roldan, ER. Effect of season and social environment on semen quality and endocrine profiles of three endangered ungulates (Gazella cuvieri, G. dorcas and Nanger dama). Animals. (2021) 11:901. doi: 10.3390/ani11030901
55. Goymann, W, East, ML, Wachter, B, Honer, OP, Mostl, E, Van't Holf, TJ, et al. Social, state-dependent and environmental modulation of faecal corticosteroid levels in free-ranging female spotted hyenas. Proc R Soc B Biol Sci. (2001) 268:2453–9. doi: 10.1098/rspb.2001.1828
56. Benhaiem, S, Dehnhard, M, Bonanni, R, Hofer, H, Goymann, W, Eulenberger, K, et al. Validation of an enzyme immunoassay for the measurement of faecal glucocorticoid metabolites in spotted hyenas (Crocuta crocuta). Gen Comp Endocrinol. (2012) 178:265–71. doi: 10.1016/j.ygcen.2012.05.006
57. Terio, KA, Marker, L, and Munson, L. Evidence for chronic stress in captive but not free-ranging cheetahs (Acinonyx jubatus) based on adrenal morphology and function. J Wildl Dis. (2004) 40:259–66. doi: 10.7589/0090-3558-40.2.259
58. Konjević, D, Janicki, Z, Slavica, A, Severin, K, Krapinec, K, Božić, F, et al. Non-invasive monitoring of adrenocortical activity in free-ranging fallow deer (Dama dama L.). Eur J Wildl Res. (2011) 57:77–81. doi: 10.1007/s10344-010-0401-1
59. Konjević, D, Janicki, Z, Slavica, A, Severin, K, Krapinec, K, Želježić, D, et al. Monitoring cortisol metabolites in the faeces of captive fallow deer (Dama dama L.). Veterinarski arhiv. (2016) 86:363–71.
Keywords: mountain gazelle (Gazella gazella), fecal glucocorticoid metabolite concentrations, adrenocortical response, biological cycle, sex determination
Citation: Karaer MC, Kankılıç T, Tavşanoğlu &, Cotman M, Čebulj-Kadunc N, Dovč A and Snoj T (2024) Effects of season and sex on the concentrations of fecal glucocorticoid metabolites in captive and free-ranging endangered mountain gazelles (Gazella gazella). Front. Vet. Sci. 11:1386009. doi: 10.3389/fvets.2024.1386009
Edited by:
Jianzhu Liu, Shandong Agricultural University, ChinaReviewed by:
Torsten Wronski, Liverpool John Moores University, United KingdomMichał Dzięcioł, Wroclaw University of Environmental and Life Sciences, Poland
Copyright © 2024 Karaer, Kankılıç, Tavşanoğlu, Cotman, Čebulj-Kadunc, Dovč and Snoj. This is an open-access article distributed under the terms of the Creative Commons Attribution License (CC BY). The use, distribution or reproduction in other forums is permitted, provided the original author(s) and the copyright owner(s) are credited and that the original publication in this journal is cited, in accordance with accepted academic practice. No use, distribution or reproduction is permitted which does not comply with these terms.
*Correspondence: Tomaž Snoj, dG9tYXouc25vakB2Zi51bmktbGouc2k=