- 1Doctorado en Ciencias Biológicas y de la Salud, Universidad Autónoma Metropolitana, Mexico City, Mexico
- 2Facultad de Medicina Veterinaria y Zootecnia, Universidad Autónoma de Tamaulipas, Ciudad Victoria, Mexico
- 3Clinical Pharmacology and Veterinary Anesthesia, Biological Sciences Department, FESC, Universidad Nacional Autónoma de México, Cuautitlán, Mexico
- 4Facultad de Estudios Superiores Cuautitlán, Universidad Nacional Autónoma de México, Cuautitlán Izcalli, Mexico
- 5Neurophysiology of Pain, Behavior and Assessment of Welfare in Domestic Animals, DPAA, Universidad Autónoma Metropolitana, Mexico City, Mexico
The negative effects of pain are a constant concern in the surgical management of animals, leading to the search for new drugs or more effective analgesic protocols to control this negative emotion. This study aimed to evaluate the nociceptive response of cannabidiol (CBD) alone and in combination with meloxicam using infrared pupillometry in female dogs undergoing elective ovariohysterectomy (OVH) under isoflurane anesthesia. A total of 60 female dogs of different breeds were included. These dogs were randomly assigned to four study groups according to the treatment: Control Group (G0: n = 15) receiving saline solution; group premedicated with meloxicam at a dose of 0.2 mg Kg−1 IV (GMelox: n = 15). Postoperatively this drug was used at 0.1 mg Kg−1 IV every 24 h; the CBD-treated Group (GCBD: n = 15) at a dose of 2 mg Kg−1 orally in the preoperative. Postoperatively was administrated every 12 h; and the Group premedicated with the combination of meloxicam and CBD (GMelox/CBD: n = 15) Meloxicam at a dose of 0.2 mg Kg−1 IV preoperatively, and 0.1 mg Kg−1 IV during the postoperative. CBD at a dose of 2 mg Kg−1 orally in the preoperative, and every 12 h in the postoperative. Treatments were administered for 48 postoperative hours. After OVH, the pupillary neurologic index, pupillary size, minimum diameter (MIN), percentage change, constriction latency (Lat), constriction velocity, and maximum constriction velocity were recorded as pupillometric variables in both eyes during events (E): Baseline (30 min before drug administration), E30 min, E1h, E2h, E3h, E4h, E8h, E12h, E24h, and E48h. The Short-Form of the Glasgow Composite Measure Pain Scale (GCMPS-SF) was used to assess pain during the same events. Overall, it was observed that the pupillometric variables Size, MIN., and Lat. were significantly higher in G0 compared to the other groups during E30 min, E1h, and E2h (p = 0.03), indicating greater pupil dilation in G0 animals. Additionally, no statistically significant differences were observed in GCMPS-SF between GMelox, GCBD, and GMelox/CBD during the postoperative period (p > 0.05). In contrast, the scores were statistically different compared to G0 (p = 0.00001), where all animals in this group received rescue analgesia at 2 h post-surgery. According to pupillometry and scores on the GCMPS-SF scale, it was observed that monotherapy with cannabidiol provides a similar analgesic effect to meloxicam alone or in combination with cannabidiol to manage acute pain in dogs. Similarly, these findings suggest that infrared pupillometry could be a tool for recognizing acute pain in dogs.
1 Introduction
Pain has physiological and emotional/behavioral negative outcomes in animals (1, 2). Therefore, it is a bioethical duty for the veterinarian to acknowledge and alleviate the perception of pain in animals under their care (3–5).
Pain management in companion animals relies on the use of analgesics such as opioids, non-steroidal analgesics (NSAIDs), and local analgesics. These drugs can prevent or decrease pain perception by interrupting some steps in the nociceptive neurobiology (6, 7). Despite the effectiveness of these analgesic drugs in several species, some authors state limitations in their use due to errors in clinical pain recognition, lack of pharmacological knowledge, or the risk of adverse effects (8, 9). For instance, opioids may cause respiratory depression and vasodilation, while NSAIDs may lead to adverse effects such as anorexia, vomiting, diarrhea, and negative consequences on renal and platelet function (10, 11).
An alternative to conventional analgesic drugs to manage pain in companion animals is the use of phytocannabinoid extracts, including cannabidiol (CBD) (12–14). In veterinary medicine, CBD is used as phytocannabinoid extracts (e.g., Sativex and Bedrocan) (15, 16), or synthetic cannabinoids such as CBD or tetrahydrocannabinol (THC). These highly liposoluble molecules interact with cannabinoid (CB) receptors 1 y CB2 (17, 18). Agonisms to CB1 receptors inhibit cAMP synthesis, inducing ion reduction. Consequently, the release of excitatory neurotransmitters (e.g., histamine, serotonin, dopamine, and glutamate) by the Central Nervous System (CSN) is reduced (19). Moreover, agonism of CB2 receptors reduces the inflammatory response induced by pro-inflammatory cytokines (20). It has been proposed that CBD can be used in combination with other drugs such as opioids to potentiate the analgesic effect due to shared mechanisms of action, reducing the dosage and minimizing the side effects of opioids (21–23). For multimodal analgesia, combining NSAIDs and CBD helps to prevent pain perception due to the action of each drug in different steps of the nociceptive pathway. However, there are limited studies evaluating the combination of NSAIDs with CBD during the perioperative period, although some reports indicate the reduction of pain perception in an osteoarthritis model (12, 24, 25).
Pupillometry is considered among the novel technological tools implemented to assess pain in dogs. It is suggested as a technique comparable to traditional methods that reduce evaluator subjectivity by quantitatively measuring pupillary diameter (26). In human medicine, this tool has been shown to objectively recognize pain and assess the efficacy of analgesic protocols to reduce their adverse effects (27). In veterinary medicine, although limited studies have been performed, Mills et al. (28) evaluated pupillometry in 126 healthy dogs to establish the pupillometric reference values for this species, which could help to develop pupillometric indices for pain assessment. Therefore, this study aimed to assess the nociceptive response of CBD alone or in combination with meloxicam through pupillometry in female dogs undergoing elective ovariohysterectomy under isoflurane anesthesia. It was hypothesized that animals receiving CBD alone or in combination with meloxicam would exhibit a lower nociceptive response compared to the use of meloxicam alone.
2 Materials and methods
2.1 Ethical considerations
Before carrying out the study, informed consent was obtained from the animals’ owners, authorizing the procedures. All work was performed under Mexico’s Official Norm NOM-062-ZOO-1999 guidelines on the technical specifications for animal production, care, and ethical use in applied ethological studies. This project was approved by the Academic Committee of the Ph.D. Program of Biological and Health Sciences (number CBS.066.21). Additionally, this study was conducted following the ARRIVE guidelines and ethical guidelines for the use of animals in experimentation (29, 30). No phase of the study during the surgical procedure or variable collection caused injury, mutilation, or overhandling of the animals.
2.2 Experimental design
Female dogs (n = 60) were randomly assigned into four groups according to the treatment: Control group (G0: n = 15) where 1 mL of saline solution was administered IV; Group premedicated with meloxicam (Meloxivet 5 mg/1 mL, Norvet, Mexico) (GMelox: n = 15) at a dose of 0.2 mg Kg−1 IV, 30 min before surgery. In the postoperative period, meloxicam was administered at 0.1 mg Kg−1 every 24 h (31); Group treated with CBD (extract of CBD with 1,000 mg/ 30 mL) (GCBD: n = 15) at a dose of 2 mg Kg−1 PO every 12 h (12); and Group medicated with the combination of meloxicam (0.2 mg Kg−1 IV and 0.1 mg Kg−1 every 24 h in the postoperative) and CBD (extract of CBD with 1,000 mg/ 30 mL) (2 mg Kg−1 PO every 12 h) (GMelox/CBD: n = 15). All treatments were administered 30 min before the start of surgery and in the immediate postoperative period for 48 h.
Pupillometry and the Glasgow Composite Measure Pain Scale (GCMPS-SF) scores were evaluated in the following events: Basal, 1 h before medical instrumentation (EBasal). Postoperative evaluations were performed at 30 min. (E30min), 1 h (E1h), 2 h (E2h), 3 h (E3h), 4 h (E4h), 8 h (E8h), 12 h (E12h), 24 h (E24h), and 48 h (E48h) after surgery.
2.3 Animals
Sixty female dogs of different breeds were included in the present study (21 mixed breed, 9 Chihuahua, 8 Poodle, 7 Pitbull, 5 Schnauzer, 2 Bobtail, 2 Cocker Spaniel, 2 Beagle, 1 Shiba, 1 Golden Retriever, 1 Teckel, and 1 Siberian Husky). Dogs had an average age, body condition score, and body weight of 2 ± 1.5 years, 3/5, and 12.1 ± 2.3 kg, respectively. The sample size was estimated using G*power 3.1.9.7 software (Heinrich-Heine-Universität Düsseldorf, Düsseldorf, Alemania) (32). To determine the sample size for four experimental groups and 10 measurements, an α error of 0.05 was established, with a confidence level of 95%, power (1- α error probability) of 0.95, and a correction among repeated measures of 0.5 (33).
All animals enrolled in the study underwent preanesthetic evaluation through a comprehensive general physical examination and laboratory tests, including complete blood cell count, serum biochemistry, and urinalysis, performed 24 h before surgery. Clinically healthy animals meeting the criteria for an ASA1 anesthetic risk according to the American Society of Anesthesiologists (34) were selected. Patients with ASA2 or higher anesthetic risk were excluded. Brachycephalic breeds, dogs medicated with anticholinergics, and with other conditions causing acute pain, with serious infectious or ocular diseases that could interfere with pupillometric evaluation were also excluded.
2.4 Anesthesia and perioperative management
Elective ovariohysterectomy (OVH) was performed with the previous informed consent of the owner. Dogs had 6-h fasting for food and 4-h fasting for water before the surgical procedure.
Animals were aseptically catheterized in the cephalic vein with a number 20G intravenous catheter. Ringer lactate solution was administered at an infusion rate of 5 mL Kg−1 h−1 (BeneFusion VP1 Vet, Mindray, Germany) during the surgical procedure (35).
Once catheterized, the animals were premedicated with Dexmedetomidine (Dexdomitor 0.5 mg/ 1 mL, Zoetis, Mexico) at a dose of 1.5 μg Kg−1 intravenously (IV). Five minutes after premedication, the dogs presented moderate sedation according to Grint et al. (36)‘s sedation score. Anesthetic induction was performed with Propofol (Recofol 1%, Pisa, Mexico) at 2–4 mg Kg−1 IV (37). Once an adequate state of unconsciousness was observed (e.g., ventromedial deviation of the eyeball and decreased jaw tone), orotracheal intubation was performed. The orotracheal tube was connected to an anesthetic rebreathing circuit with an oxygen flow of 45 mL Kg−1 min−1. Anesthetic maintenance was performed with isoflurane (Sofloran, Pisa, Mexico) vaporized in 100% oxygen, regulating the vaporizer dial initially at 1.8% and modifying the concentration according to the anesthetic depth required to maintain a mean arterial pressure (MAP) between 60 to 90 mmHg, assessed through non-invasive blood pressure. All animals were ventilated with a mechanical ventilator into the anesthesia station (Wato-EX20 vet, Mindray, Germany), using a pressurometric ventilation method controlled at a mean airway pressure (Paw) of 10–15 cmH20 and an I:E ratio of 1:2 during surgery. A respiratory rate of 12 to 20 breaths per minute was established to maintain an EtCO2 of 35–45 mmHg (ePM12VETc/AA, Mindray, Alemania).
The surgical anesthetic depth was assessed through the recognition of clinical signs such as jaw tone relaxation, ventromedial deviation of the eyeball, and the absence of the palpebral reflex. All OVH surgeries were performed by the same surgeon using a midline approach and a triple hemostatic surgical technique. Similarly, all anesthetic procedures were carried out by the same anesthesiologist. The administration of inhalant anesthetics stopped 5 min before surgical wound closure. The end of the surgery was considered after the closure of the surgical incision. Extubating with the reappearance of the cough reflex was performed when patients could successfully sustain spontaneous ventilation and returned the ocular globe to the central position.
2.5 Infrared pupillometry
An automated and portable pupillometer (Neuroptics, NPi 200, United States) was used to measure pupillary size during 60 s in each eye (Figure 1). The following parameters were registered: neurological pupil index (NPi), size, minimum diameter (MIN), percentage of change (% CH), constriction latency (LAT), constriction velocity (CV) and maximum constriction velocity (MCV) (38). Pupillary assessment was performed once in each event. Assessments were performed by a single blinded evaluator.
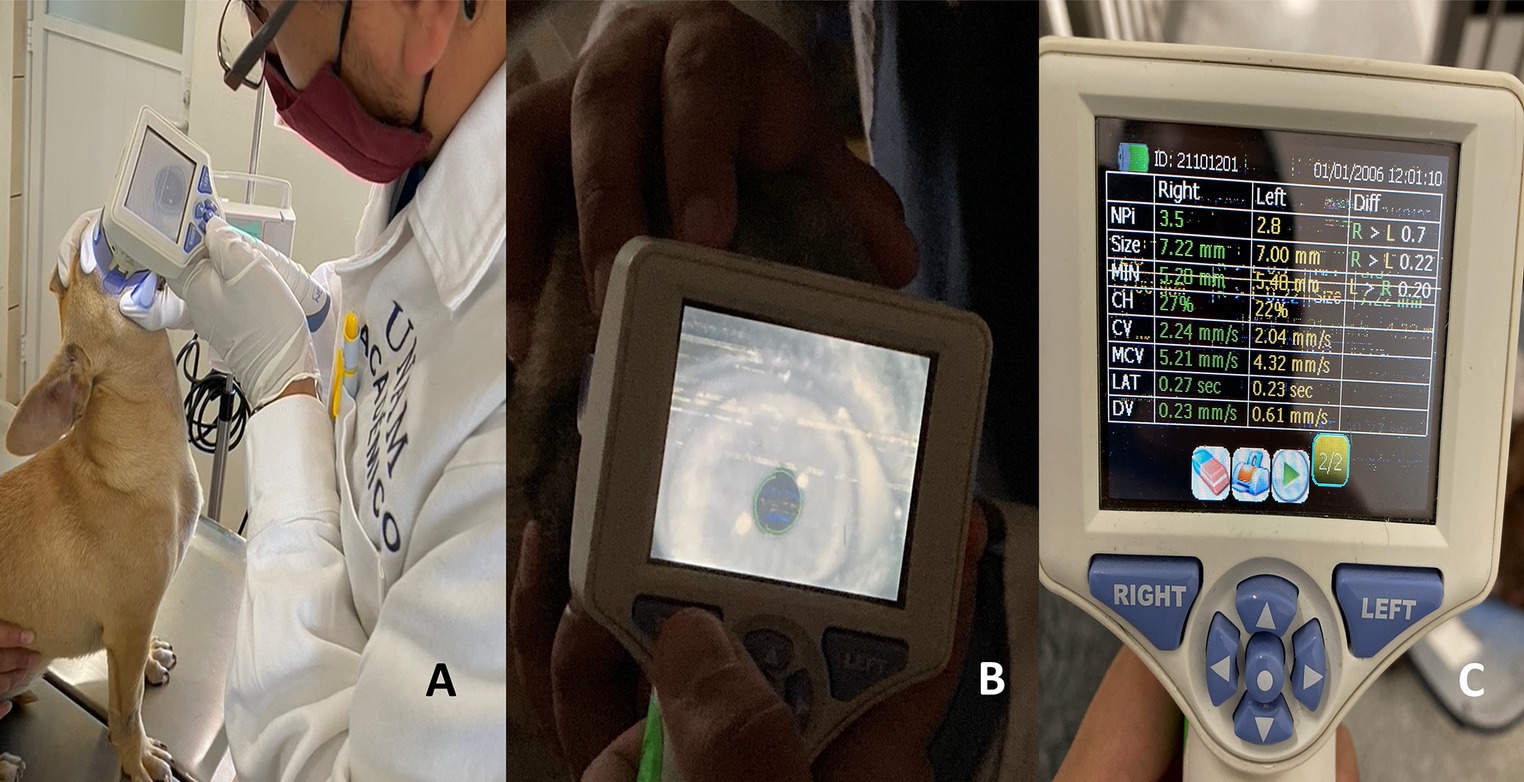
Figure 1. Methodology of the infrared pupilometry technique. (A) The placement of the pupilometer at a 90° angle in the ocular region is depicted. (B) The moment of measuring the pupil diameter using the infrared light camera is shown. From the pupil diameter measurement, 7 different variables are captured, including the neurological pupil index (NPi), size, minimum diameter (MIN), percentage change (% CH), constriction latency (LAT), constriction velocity (CV), and maximum constriction velocity (MCV), as shown in image C.
2.6 Assessment of acute pain
The Glasgow Composite Pain Score – Short Form (GCMPS-SF) was used to assess pain. This scale comprises different behavioral and physiological categories, as well as response to touch, facial expression, vocalization, and mobility. The maximum pain score is 24 points (39). A single and trained evaluator performed all measures. Rescue analgesia with Tramadol (Tramajet 50 mg/ 1 mL; Norvet, Mexico) at 4 mg kg −1 IV (40–42) was administered in the postsurgical period when GCMPS-SF score was ≥6 points.
2.7 Statistical analyses
Descriptive statistics were obtained using Graph Pad Prism (ver. 9.5) for all groups (G0, GMelox, GCBD, GMelox/CBD) and all events (EBasal, E30 min, E1h, E2h, E3h, E4h, E8h, E12h, E24h, and E48h). Normality tests were done with the Kolmogorov–Smirnov test for all the variables assessed.
The treatments were considered independent variables, while each of the pupillometric parameters and the post-surgical pain evaluation scores were considered dependent. To evaluate the effects of these variables, a linear mixed model was used.
A Tukey post hoc test was used to evaluate differences between means. The analysis of sensitivity and specificity was carried out using a receiver operating characteristics (ROC) test using the score obtained in GCMPS-SF as the gold standard. Finally, the linear relationship between study variables was performed using a Pearson correlation test. In all cases, the significance level was set at p < 0.05.
3 Results
In the present study, 64 dogs were considered. However, four dogs were excluded: two dogs due to the administration of anticholinergics, one dog due to pyometra, and one dog due to osteoarthritic chronic pain. A total of 60 dogs were included, 21 mixed breeds, 9 Chihuahuas, 8 Poodles, 7 Pitbulls, 5 Schnauzers, 2 Bobtail, 2 Cocker Spaniel, 2 Beagle, 1 Shiba, 1 Golden Retriever, 1 Teckel, and 1 Siberian Husky. In general, the average anesthesia time was 57 ± 8.4 min, surgical time was 24 ± 4.8 min, and extubating time was 13 ± 2.8 min. The main findings of the pupillary assessment show that Size, MIN, and Lat, had significant differences between groups (p < 0.05) particularly GMelox, GCBD, and GMelox/CBD with G0. These differences were observed during the first two postoperative hours. Moreover, all animals in G0 required rescue analgesia at E2h.
In Table 1, it can be observed that the Size of the right eye (maximum pupil diameter) significantly increased in GCBD during E2h (p = 0.006) when comparing basal values in the same group, registering 9.19 ± 0.26 mm. During E2h, the Size of GMelox/CBD was 8.59 ± 0.30 mm, a value that was not statistically significant (p = 0.47) in comparison with GCBD (9.19 ± 0.26 mm) and GMelox (9.35 ± 0.20 mm). However, the pupil diameter of G0 was 9.90 ± 0.07 mm, showing statistically significant differences (p = 0.003) with the other experimental groups GMelox, GCBD, GMelox/CBD.
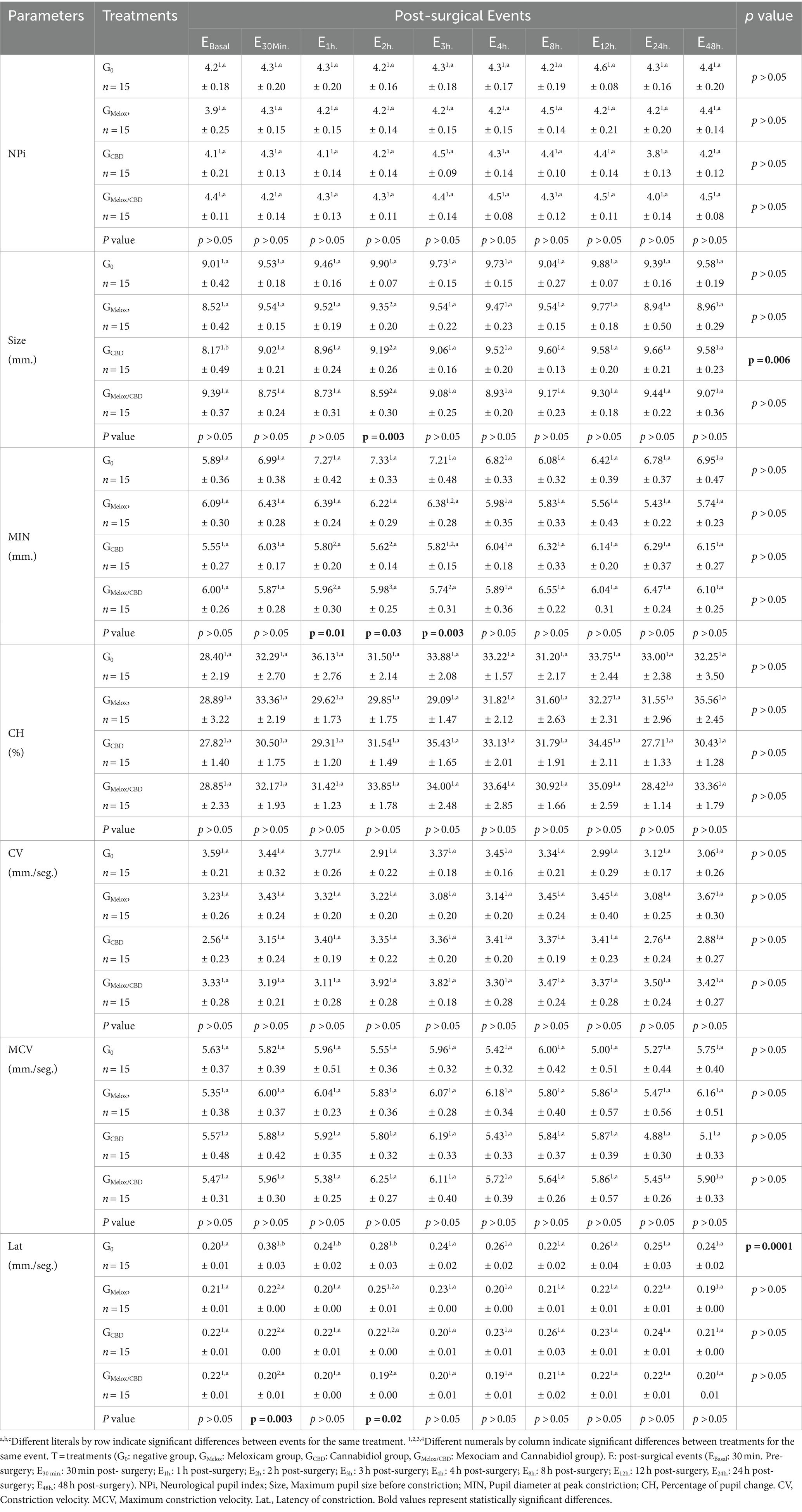
Table 1. Pupillometric values (Mean ± EE) of the right eye pupil in the evaluation events (E) of 60 bitches under elective ovariohysterectomy surgery distributed in 4 study groups: G0, GMelox, GCBD, GMelox/CBD.
In the case of the minimum pupil diameter (MIN) of the right eye, statistically significant differences were reported between study groups during E1h, (p = 0.01), E2h, (p = 0.03), and E3h, (p = 0.003). Animals in G0 recorded the highest values with 7.27 ± 0.42, 7.33 ± 0.33, and 7.21 ± 0.48 mm at E1h, E2h, and E3h, respectively.
Regarding the latency time of pupillary constriction (Lat), the Lat of G0 animals increased between 0.08 and 0.18 s compared to the rest of the postsurgical events and the EBasal. from the same experimental group (p = 0.0001). Likewise, at E30min and E2h, statistically significant differences between treatments were reported (p = 0.003 y p = 0.02 respectively). The latency time in G0 was 0.38 ± 0.03 s during E30min, while at E2h, Lat. was 0.28 ± 0.03. sec. In contrast, values recorded from GMelox, GCBD, GMelox/CBD decreased between 0.16–0.18 and 0.03–0.09 s, respectively, during the evaluation events. Also in Table 1, it can be observed that NPi, CH, CV, and MCV did not have significant differences between treatments and/or events (p > 0.05).
Table 2 shows the pupillometric variables of the left eye. Similar to the previously described results, CH, CV, and MCV had no statistical differences between events or between treatments (p > 0.05). However, NPi values increased in the left eye (between 0.70–1.00) in all postsurgical events when compared to EBasal., where a value of 3.60 ± 0.24 (p = 0.03) was recorded. For the Size variable in the left eye, the diameter of animals in GMelox at E24h was significantly smaller compared to the rest of the events (p = 0.006) and between treatments (p = 0.002).
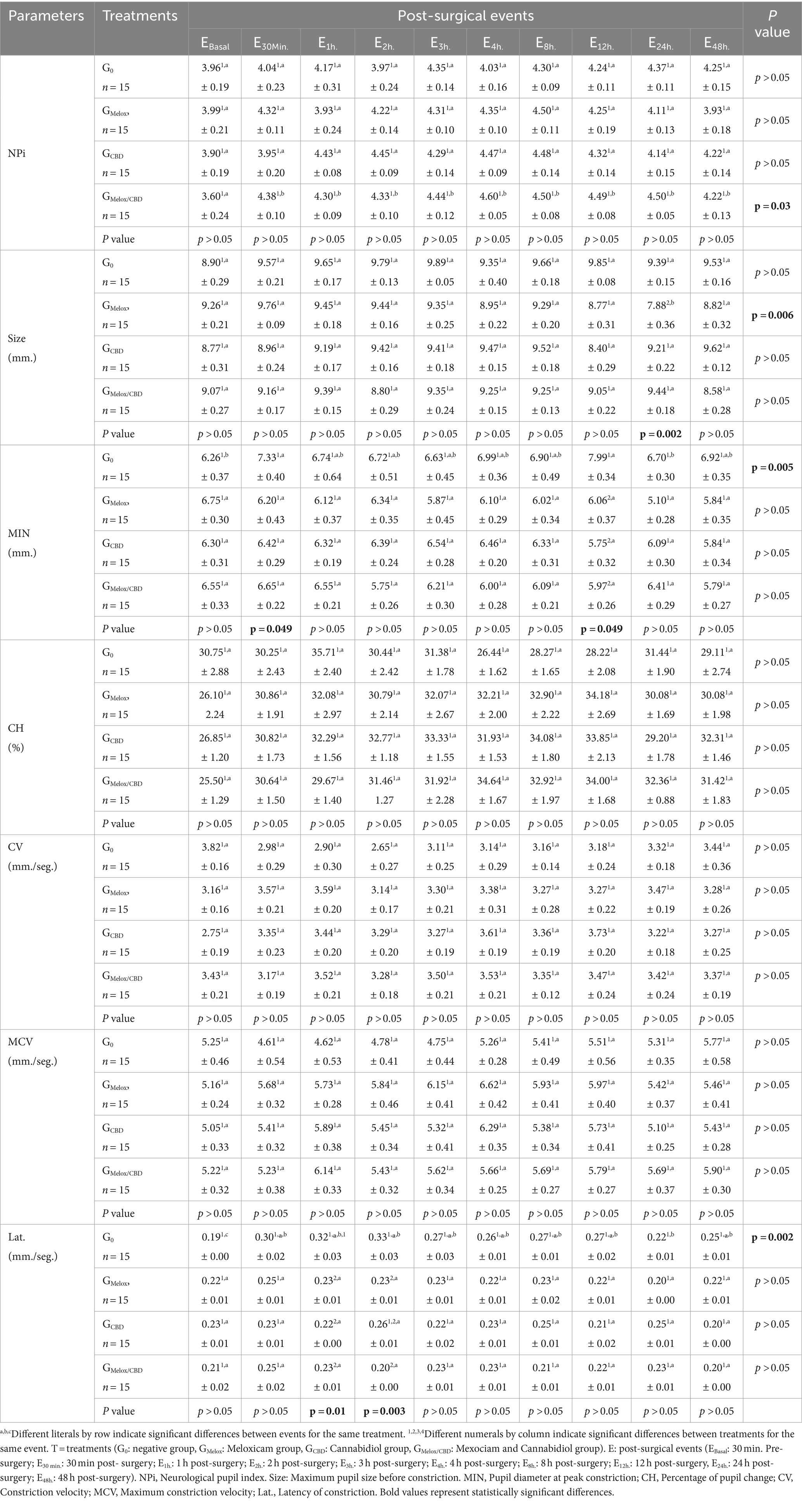
Table 2. Pupillometric values (Mean ± EE) of the left eye pupil in the evaluation events (E) of 60 bitches under elective ovariohysterectomy surgery distributed in 4 study groups: G0, GMelox, GCBD, GMelox/CBD.
Regarding MIN, dogs in G0 registered 6.26 ± 0.37 mm during EBasal. This value increased from E30min (7.33 ± 0.40 mm) to 6.92 ± 0.35 mm at E48h, having statistically significant differences between events (p = 0.005). Differences between treatments were recorded during E30min (p = 0.049) and E12h (p = 0.049) where GMelox, GCBD, GMelox/CBD maintained a homogeneous pattern with mean peak constriction values of 5.75 to 6.65 mm. In G0, the pupil diameter increased from 0.68–1.13 mm during E30min up to 1.93–2.24 mm during E12h.
Lat. variable showed values of 0.19 ± 0.00 s in G0 animals during EBasal; however, this value increased during all postsurgical events, reaching 0.33 ± 0.03 and 0.27 ± 0.02 s at E2h and E12h, respectively. Therefore, as observed in the right eye, there was a statistically significant difference between postsurgical events (p = 0.002). Moreover, significant differences between treatments at E1h, and E2h were recorded (p = 0.01 y p = 0.003, respectively), where the Lat. of GMelox, GCBD, GMelox/CBD was lower than G0 during E1h (average of 0.09 s) and E2h (average of 0.1 s).
GCMPS-SF scores are presented in Table 3. In all groups, scores increased from EBasal to the post-operative period (p = 0.0001). However, the highest values were recorded in G0 during E30min, E1h, E2h, E3h, and E4h, in comparison to the rest of the treatments at the same events (p = 0.0001). Furthermore, it was found that the pain scores of GMelox, GCBD, GMelox/CBD did not present differences between groups (p > 0.05). Rescue analgesia was administered in one dog included in GMelox, GCBD, GMelox/CBD.
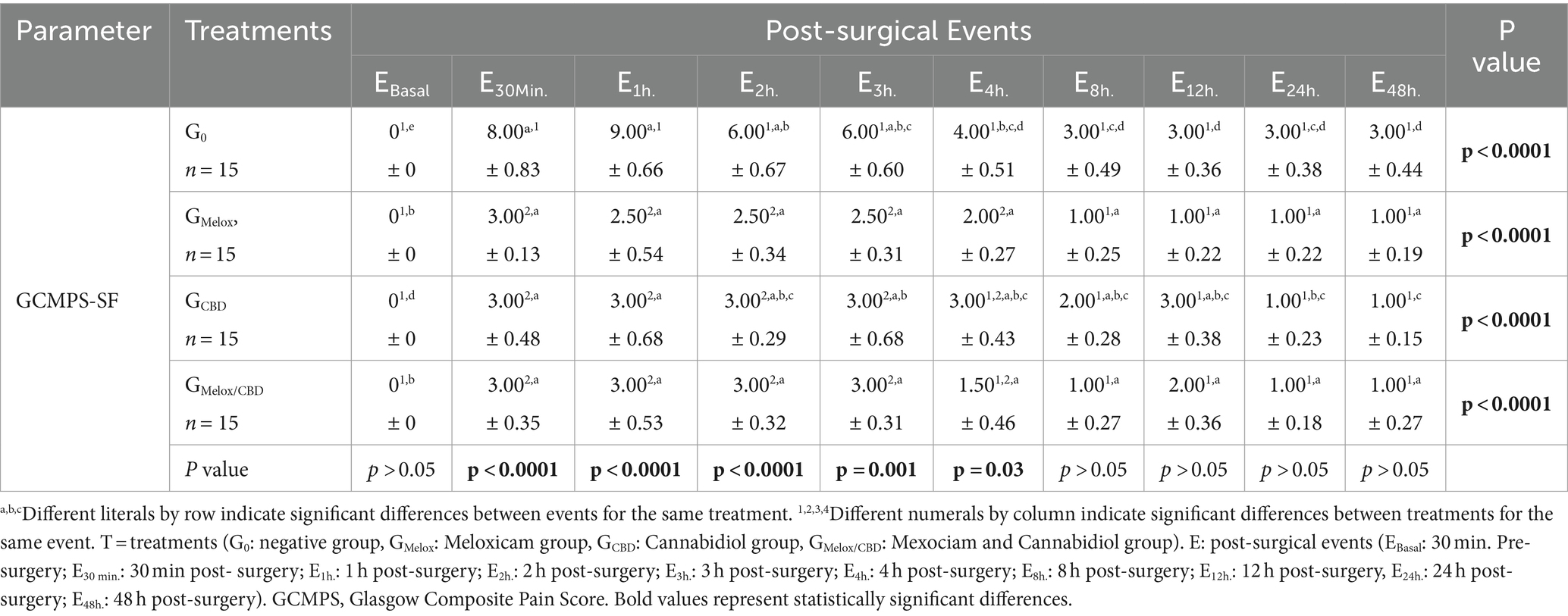
Table 3. Pain evaluation scale values (Median ± EE) in the evaluation events (E) of 60 bitches undergoing elective ovariohysterectomy surgeries distributed in 4 study groups: G0, GMelox, GCBD, GMelox/CBD.
Finally, in the ROC analysis, it was determined that the Size variable presented a sensitivity of 77.2% and specificity of 96.9% (p < 0.0001), while Lat., had a sensitivity of 94.5% and specificity of 88.1% was obtained (p < 0.001). Likewise, MIN had a sensitivity of 98.2% and a specificity of 95.6% was recorded (p < 0.0001). No significant correlation between the pupillometric indicators was found (Table 4).
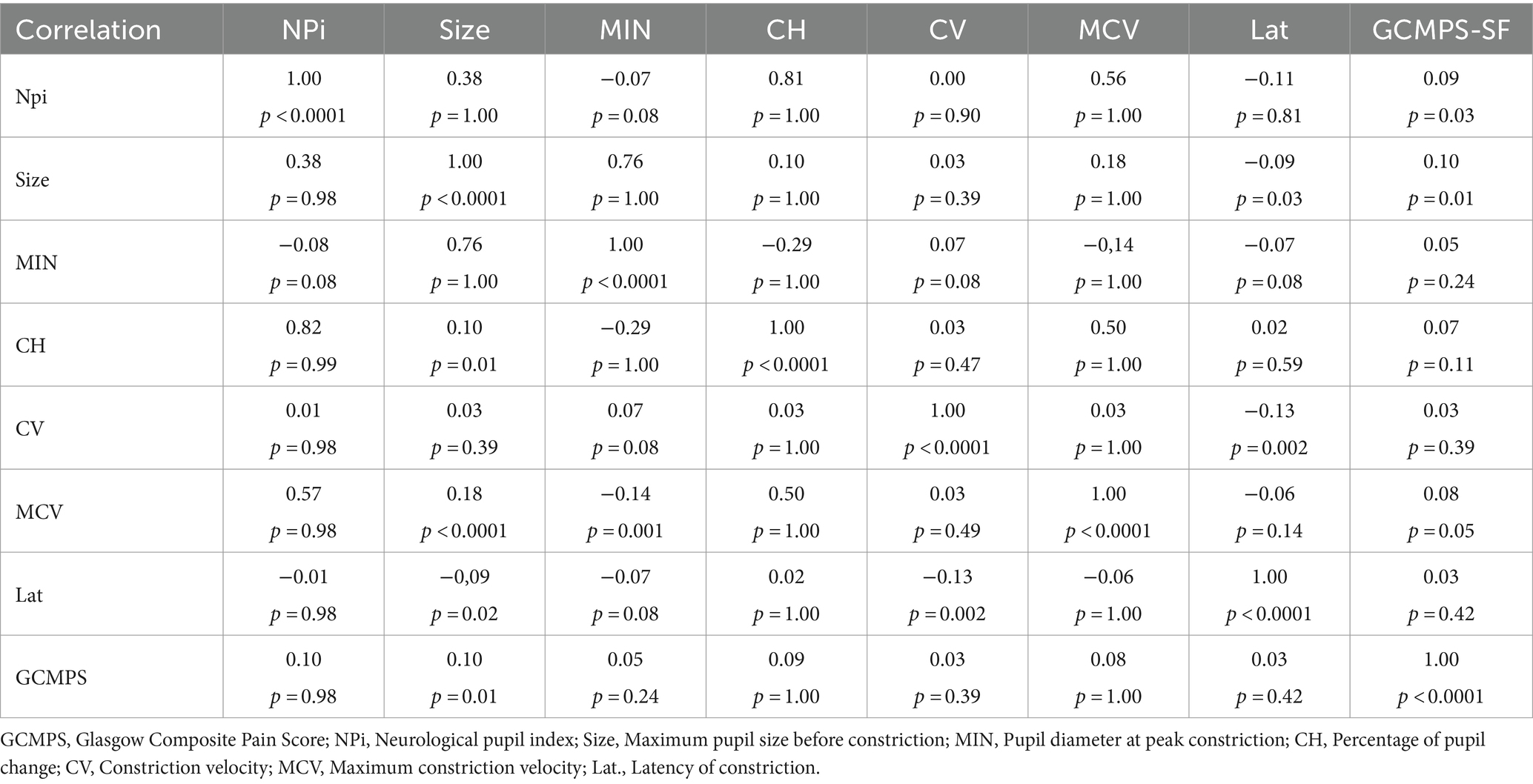
Table 4. Correlation matrix between Glasgow Composite Pain Scale (GCMPS) values of 60 bitches undergoing elective ovariohysterectomy surgeries distributed in 4 study groups: G0, GMelox, GCBD, GMelox/CBD.
4 Discussion
Among the most significant findings, the pupillometric variables Size, MIN., and Lat. showed higher sensitivity and specificity to identify pain during the postoperative period of dogs undergoing OVH. This suggests that pupillometry is an objective method to recognize acute pain in dogs. The neurophysiological control of the pupil diameter is related to the changes that can be observed in the pupil in animals experiencing pain. Both the sphincter and the dilator muscle control the pupil size. The dilator muscle has sympathetic fibers that increase the pupil diameter or the pupil dilator reflex (27). In humans, pupillometry is currently used to assess pain in pediatrics and traumatology (40–43).
The results indicate that CBD and meloxicam offer equivalent perioperative analgesic quality, without either being superior when these drugs were administered together in the studied animals. Derived from the pupillometric data obtained, it was observed that CBD exhibited similar analgesic activity to meloxicam. This could be explained by the presence of CB1 receptors in neurons of the dorsal horn of the spinal cord (44) and CB2 receptors primarily found in cells of both the immune system and smooth muscle in viscera (45–47). The presence of CB1 and CB2 receptors in the retina, ciliary body, and sympathetic iris fibers has also been suggested (48, 49). Moreover, CBD has a high affinity to CB2 receptors (19).
The analgesic mechanism of action of cannabinoids is mainly by agonism to cannabidiol receptors. The first is the agonism of CB1 receptors, which can induce the activation of Gi/o proteins, inhibiting adenylate cyclase activity and reducing cAMP synthesis. CB1 receptor agonism induces the blockade of voltage-dependent N-type Ca2+ channels and an increase in G protein-related K+ channel conductance (19, 50). At the presynaptic level, these actions reduce the release of neurotransmitters such as norepinephrine, histamine, serotonin, dopamine, cholecystokinin, and glutamate in the central nervous system, thereby reducing the perception of nociceptive stimuli (14). Sagar et al. (44) reported that the use of a CB1 receptor agonist decreased Ca2+ conductance induced by capsaicin stimulation in dorsal horn neurons of the spinal cord, which could be an explanation for the antinociceptive effect observed in this study.
On the other hand, CB2 agonism could lead to the reduction of an inflammatory response (51) by mediating tumor necrosis factor-alpha (TNF-α) and interleukins from microglia or macrophages (14). Gugliandolo et al. (20) mentioned that the administration of cannabidiol in dogs receiving lipopolysaccharide reduced the presence of interleukin (IL)-10, nuclear factor-kappa B (NF), and the expression of cyclooxygenase 2 (COX-2). Therefore, the reduction in the expression and activity of COX-2 also inhibits the formation of prostaglandins such as prostaglandin E2 (PGE2) and lipoxygenases, subsequently decreasing the expression of proinflammatory metabolites (52). This mechanism of action is also associated with the reduction of proinflammatory cytokine synthesis such as IL-1, IL-8, NFκB, and TNF-α (53, 54). Hence, the evidence suggests that CBD can help to manage or reduce pain by reducing the inflammatory process, possibly being an additional mechanism of pain control.
The pupillometric data obtained in this study showed the analgesic activity of meloxicam due to the preferential inhibition of COX-2 (55, 56). This isoform of COX is the most active during an inflammatory process and is responsible for the production of prostaglandins (57). The inhibition of COX-2 prevents the increase in phospholipase A2 in dorsal horn neurons of the spinal cord, which can consequently prevent the expression of substance P, serotonin, histamine, PGE2, and proinflammatory cytokines (58–60). Preanesthetic administration of meloxicam can prevent peripheral and central sensitization phenomena during nociceptive events due to its pharmacodynamic properties (61, 62).
During the perception of pain, there is an increase in the activity of the sympathetic nervous system (SNS), so NSAIDs like meloxicam can reduce autonomic activity (63, 64). Hernández-Avalos et al. (65) reported that meloxicam increases parasympathetic tone or PTA index similarly to the use of carprofen and paracetamol by decreasing sympathetic nervous system (SNS) activity. The decrease in SNSi activity due to a predominant parasympathetic tone inhibits the stimulation in the Edinger-Westphal nucleus and, in turn, promotes miosis in the pupil (66), as observed in the present results. This effect explains that GMelox obtained the lowest value in the Size variable compared to the other study groups during E24h. (p < 0.05). However, it should be considered that, in dogs, meloxicam’s half-life is 24 h, which is why re-administration of meloxicam was necessary at this point to maintain adequate plasma levels and therapeutic effect (67), a situation that could have altered the pupillary response of the study subjects.
CBD, by its agonism to CB1 and CB2 receptors, prevents the transmission of nociceptive stimuli by inhibition of central neurotransmitters. On the other hand, meloxicam modulates PGE2 formation (6). Combining both drugs results in a multimodal analgesia that allows pain control at different points of the nociceptive pathway (55, 68). Thus, this could be the possible explanation for GMelox/CBD having a lower MIN compared to the other groups during E3hr (p < 0.05) and would reaffirm the fact that CBD exhibits analgesia similar to meloxicam. Therefore, based on our results, CBD can be used to control acute pain in dogs undergoing abdominal surgery and during the immediate postsurgical period. Similarly, according to the findings regarding infrared pupillometry, it can be suggested that the nociceptive response of dogs undergoing OVH and receiving CBD alone or in combination with meloxicam was similar.
Since pain is a subjective condition, its perception may differ among individuals (69). For this reason, it is suggested to use scales that integrate both behavioral and physiological indicators to recognize pain (70–73). In the present research, pain management during the immediate postoperative period could explain the differences observed in this study during the first hours of post-surgery evaluation, since the use of analgesics at the first signs of pain could help control long-term physiological changes and alter the scale scores (74). The scores obtained show the importance of using analgesics before surgery, which could prevent sensitization phenomena and, thus, pain perception (75).
On the other hand, the presence of a larger Size, MIN, and Lat value in G0 compared to GMelox, GCBD, and GMelox/CBD suggests that the pupillary response can be used as a method to recognize postoperative pain in dogs. This has been described in dogs, in whom a positive association between pupil diameter and the value obtained in the numerical rating scale was reported, highlighting that its assessment was limited to the presence or absence of the pupillary reflex (76). The possible neurobiological explanation for the increase in pupil diameter is the increase in SNS activity with catecholamine neurosecretion when animals perceive pain (77). Catecholamines have an effect on α1 adrenergic receptors present in the long ciliary fibers of the iris dilator muscle, which activation would lead to pupil dilation (78, 79). This was observed in G0 animals during E2hr, values that were also associated with increasing scores in the GCMPS-SF. A similar association between pupil diameter and pain scales has been reported in human medicine (43, 80, 81). Therefore, the present findings suggest a possible relationship between pain scales and the pupillary response in animals. Although further research is needed to establish the correlation between both methods to evaluate pain, the application of pupillometry could help to refine pain assessment in companion animals (4, 8).
Size and MIN represent an increase in the pupil diameter; however, the response to the light stimulus increased both in the left and right eye. This can also be evaluated through Lat, where the highest values were recorded in G0 in both the left and right eyes, in comparison with GMelox, GCBD, and GMelox/CBD. This indicates that the pupil speed is greater when faced with a light stimulus (27). In this sense, Mills et al. (28) suggested that the maximum value of Lat in dogs is 0.30 s, a value that was below the ones reported in the present study, possibly due to nociception. The pupillary response observed in animals during the perception of pain is related to the activation of the Locus Coeruleus, a region that contains pre-motor and excitatory sympathetic neurons that are projected to preganglionic neurons in the Edinger-Westphal nucleus and present in α2 adrenergic receptors. Through sympathoexcitation and parasympathetic inhibition, these fibers cause pupillary dilation, decreasing the response to light (82). Therefore, this could be the first time addressing the influence that these drugs have on the pupil diameter of dogs.
The increase in these values occurred at E30min, E1h, and E2h, when animals in G0 received rescue analgesia. In this sense, although there could be a residual effect of anesthetics, it is reported that pupil dilation has a positive relation with anesthetic depth (83, 84). This effect could only be observed in G0 at E30min, in contrast to the GMelox, GCBD, and GMelox/CBD groups, which was not observed at E1h. and E2h. It is necessary to mention that meloxicam has an elimination half-life of 24 h in dogs (67), while CBD has an elimination half-life of 3 to 5 days (14). This coincides with the increase in pain scores assessed with the behavioral-based scale in G0.
The present findings suggest that pupillometry could be used to recognize pain in dogs subjected to OVH. However, it is necessary to consider that increased values during the immediate postsurgical period where pain control is essential to avoid the physiological consequences of pain might coincide with these critical events (72, 73). This would explain why the pupillometric parameters and pain scores decreased in the subsequent events. Dyson (85) explains that pain control during the first hours after surgery reduces the risk of short- and long-term complications. Therefore, this evidence could lead to corroborating the theory that this tool can be used as an objective and quantitative way of acute pain in animals (86). Additionally, the sensitivity and specificity for MIN and Lat. were greater than 80%, possibly making it a reliable tool for assessing pain in animals. This has been reported in humans, recording a sensitivity of around 100% and a specificity of 77% (80). Regardless of the species, future studies need to consider the clinical application of pupillometry.
Regarding rescue analgesia, it was observed that GMelox and GCBD required more rescue analgesia than GMelox/CBD (GMelox = 1, GCBD = 1, GMelox/CBD = 0). This is due to the effect of multimodal analgesia in which CBD inhibits the nociceptive stimulus while meloxicam negatively alters the nociceptive signal at the peripheral level, preventing pain perception (68, 87). However, when comparing the number of animals that required rescue analgesia in GMelox and GCBD, these were significantly lower than G0, where all animals received rescue analgesia due to the lack of an analgesic protocol before the surgery. Thus, these observations add to the importance of providing analgesia to dogs before the surgical procedure to avoid pain-related complications during the postoperative period (88). Furthermore, at E30min the increase in pupillometry parameters was related to an increase in the GCMPS score. However, this might be attributed to the residual effect of general anesthetics and sedatives such as α2 agonists (89). Thus, this could be considered a limitation on the use of pupillometry in surgical patients.
One of the main limitations of the present study is that current pupillometry does not consider the anatomical and conformational characteristics of a dog’s eyes. For example, the iris pigmentation and morphology might affect the accuracy of pupillometric variables (90). This needs to be established in future research when implementing pupillometry as a complementary tool to assess pain. Another field of research would be implementing pupillometry during other surgical procedures such as trauma surgery where there is a greater risk of pain perception. Other limitation could be the level of fear that awake animals might experience, which needs further study to improve the application of pupillometry in veterinary medicine. Likewise, physiological parameters are not reported during the postoperative period, which can be modified due to the painful experience. This limitation arises from the incorporation of these parameters into another paper derived from the present research. Finally, another important perspective is the correlation with other methods that have been suggested to evaluate pain, such as the physiological parameters, the parasympathetic tone index monitor and infrared thermography (90–98).
5 Conclusion
According to the results obtained through pupillometry and the GCMPS-SF scores, CBD alone or in combination with meloxicam has a similar analgesic effect for the control of acute pain in dogs. The findings of the present study suggest that infrared pupillometry could be implemented as a tool to recognize acute pain in ovariohysterectomized bitches.
Data availability statement
The original contributions presented in the study are included in the article/Supplementary material, further inquiries can be directed to the corresponding author/s.
Ethics statement
The animal studies were approved by the Ph.D. Program in the Biological and Health Science Academic Committee (number CBS.066.21). The studies were conducted in accordance with the local legislation and institutional requirements. Written informed consent was obtained from the owners for the participation of their animals in this study.
Author contributions
AC-A: Writing – review & editing, Writing – original draft, Supervision, Methodology, Formal analysis. JM-B: Writing – review & editing, Writing – original draft, Supervision. IH-Á: Writing – review & editing, Writing – original draft, Supervision, Methodology, Conceptualization. PM-M: Writing – review & editing, Writing – original draft, Supervision, Methodology. AM-C: Writing – review & editing, Writing – original draft, Supervision. AD-O: Writing – review & editing, Writing – original draft, Supervision. DM-R: Writing – review & editing, Writing – original draft, Supervision, Formal analysis, Conceptualization.
Funding
The author(s) declare that no financial support was received for the research, authorship, and/or publication of this article.
Acknowledgments
This is study is part of the PhD. Thesis by Alejandro Casas- Alvarado, a graduate student in the program of “Doctorado en Ciencias Biológicas y de la Salud” at UAM campus Xochimilco; (CONACYT scholarship no. 927304).
Conflict of interest
The authors declare that the research was conducted in the absence of any commercial or financial relationships that could be construed as a potential conflict of interest.
The author(s) declared that they were an editorial board member of Frontiers, at the time of submission. This had no impact on the peer review process and the final decision.
Publisher’s note
All claims expressed in this article are solely those of the authors and do not necessarily represent those of their affiliated organizations, or those of the publisher, the editors and the reviewers. Any product that may be evaluated in this article, or claim that may be made by its manufacturer, is not guaranteed or endorsed by the publisher.
Supplementary material
The Supplementary material for this article can be found online at: https://www.frontiersin.org/articles/10.3389/fvets.2024.1380022/full#supplementary-material
References
1. Landa, L . Pain in domestic animals and how to assess it: a review. Vet Med. (2012) 57:185–92. doi: 10.17221/5915-VETMED
2. Raja, SN, Carr, DB, Cohen, M, Finnerup, NB, Flor, H, Gibson, S, et al. The revised International Association for the Study of Pain definition of pain: concepts, challenges, and compromises. Pain. (2020) 161:1976–82. doi: 10.1097/j.pain.0000000000001939
3. Downing, R, and Della, RG. Pain in pets: beyond physiology. Animals. (2023) 13:355. doi: 10.3390/ani13030355
4. Hernandez-Avalos, I, Mota-Rojas, D, Mora-Medina, P, Martínez-Burnes, J, Casas Alvarado, A, Verduzco-Mendoza, A, et al. Review of different methods used for clinical recognition and assessment of pain in dogs and cats. Int J Vet Sci Med. (2019) 7:43–54. doi: 10.1080/23144599.2019.1680044
5. Sandgren, EP, Streiffer, R, Dykema, J, Assad, N, and Moberg, J. Influence of animal pain and distress on judgments of animal research justifiability among university undergraduate students and faculty. PLoS One. (2022) 17:e0272306. doi: 10.1371/journal.pone.0272306
6. Bradbrook, C, and Clark, L. State of the art analgesia—recent developments pharmacological approaches to acute pain management in dogs and cats: part 2. Vet J. (2018) 236:62–7. doi: 10.1016/j.tvjl.2018.04.009
7. Ruel, HLM, and Steagall, PV. Adjuvant analgesics in acute pain management. Vet Clin N Am Small Anim Pract. (2019) 49:1127–41. doi: 10.1016/j.cvsm.2019.07.005
8. Hugonnard, M, Leblond, A, Keroack, S, Cadoré, J, and Troncy, E. Attitudes and concerns of French veterinarians towards pain and analgesia in dogs and cats. Vet Anaesth Analg. (2004) 31:154–63. doi: 10.1111/j.1467-2987.2004.00175.x
9. Williams, V, Lascelles, B, and Robson, M. Current attitudes to, and use of, peri-operative analgesia in dogs and cats by veterinarians in New Zealand. N Z Vet J. (2005) 53:193–202. doi: 10.1080/00480169.2005.36504
10. Lomas, AL, and Grauer, GF. The renal effects of NSAIDs in dogs. J Am Anim Hosp Assoc. (2015) 51:197–203. doi: 10.5326/JAAHA-MS-6239
11. Monteiro-Steagall, BP, Steagall, PVM, and Lascelles, BDX. Systematic review of nonsteroidal anti-inflammatory drug-induced adverse effects in dogs. J Vet Intern Med. (2013) 27:1011–9. doi: 10.1111/jvim.12127
12. Gamble, L-J, Boesch, JM, Frye, CW, Schwark, WS, Mann, S, Wolfe, L, et al. Pharmacokinetics, safety, and clinical efficacy of Cannabidiol treatment in osteoarthritic dogs. Front Vet Sci. (2018) 5:165. doi: 10.3389/fvets.2018.00165
13. Landa, L, Sulcova, A, and Gbelec, P. The use of cannabinoids in animals and therapeutic implications for veterinary medicine: a review. Vet Med. (2016) 61:111–22. doi: 10.17221/8762-VETMED
14. Miranda-Cortés, A, Mota-Rojas, D, Crosignani-Outeda, N, Casas-Alvarado, A, Martínez-Burnes, J, Olmos-Hernández, A, et al. The role of cannabinoids in pain modulation in companion animals. Front Vet Sci. (2023) 9:884. doi: 10.3389/fvets.2022.1050884
15. Fernández-Trapero, M, Pérez-Díaz, C, Espejo-Porras, F, de Lago, E, and Fernández-Ruiz, J. Pharmacokinetics of Sativex® in dogs: towards a potential cannabinoid-based therapy for canine disorders. Biomol Ther. (2020) 10:279. doi: 10.3390/biom10020279
16. Łebkowska-Wieruszewska, B, Stefanelli, F, Chericoni, S, Owen, H, Poapolathep, A, Lisowski, A, et al. Pharmacokinetics of Bedrocan®, a cannabis oil extract, in fasting and fed dogs: an explorative study. Res Vet Sci. (2019) 123:26–8. doi: 10.1016/j.rvsc.2018.12.003
17. Pertwee, RG . Pharmacological actions of cannabinoids. Handb Exp Pharmacol. (2005) 168:1–51. doi: 10.1007/3-540-26573-2_1
18. McPartland, JM, Glass, M, and Pertwee, RG. Meta-analysis of cannabinoid ligand binding affinity and receptor distribution: interspecies differences. Br J Pharmacol. (2007) 152:583–93. doi: 10.1038/sj.bjp.0707399
20. Gugliandolo, E, Licata, P, Peritore, AF, Siracusa, R, D’Amico, R, Cordaro, M, et al. Effect of Cannabidiol (CBD) on canine inflammatory response: an ex vivo study on LPS stimulated whole blood. Vet Sci. (2021) 8:185. doi: 10.3390/vetsci8090185
21. Cichewicz, DL, and McCarthy, EA. Antinociceptive synergy between Δ 9-tetrahydrocannabinol and opioids after Oral administration. J Pharmacol Exp Ther. (2003) 304:1010–5. doi: 10.1124/jpet.102.045575
22. Cichewicz, DL . Synergistic interactions between cannabinoid and opioid analgesics. Life Sci. (2004) 74:1317–24. doi: 10.1016/j.lfs.2003.09.038
23. Welch, SP . Interaction of the cannabinoid and opioid systems in the modulation of nociception. Int Rev Psychiatry. (2009) 21:143–51. doi: 10.1080/09540260902782794
24. Valastro, C, Campanile, D, Marinaro, M, Franchini, D, Piscitelli, F, Verde, R, et al. Characterization of endocannabinoids and related acylethanolamides in the synovial fluid of dogs with osteoarthritis: a pilot study. BMC Vet Res. (2017) 13:309. doi: 10.1186/s12917-017-1245-7
25. Interlandi, C, Tabbì, M, Di Pietro, S, D’Angelo, F, Costa, GL, Arfuso, F, et al. Improved quality of life and pain relief in mature horses with osteoarthritis after oral transmucosal cannabidiol oil administration as part of an analgesic regimen. Front Vet Sci. (2024) 11:1396. doi: 10.3389/fvets.2024.1341396
26. Lussier, BL, Olson, DM, and Aiyagari, V. Automated Pupillometry in Neurocritical care: research and practice. Curr Neurol Neurosci Rep. (2019) 19:71. doi: 10.1007/s11910-019-0994-z
27. Packiasabapathy, S, Rangasamy, V, and Sadhasivam, S. Pupillometry in perioperative medicine: a narrative review. Can J Anesth/J Can Anesth. (2021) 68:566–78. doi: 10.1007/s12630-020-01905-z
28. Mills, EP, Combs-Ramey, K, Kwong, GPS, and Pang, DSJ. Development of reference intervals for pupillometry in healthy dogs. Front Vet Sci. (2022) 9:1020710. doi: 10.3389/fvets.2022.1020710
29. Kilkenny, C, Browne, WJ, Cuthill, IC, Emerson, M, and Altman, DG. Improving bioscience research reporting: the ARRIVE guidelines for reporting animal research. J Pharmacol Pharmacother. (2010) 1:94–9. doi: 10.4103/0976-500X.72351
30. Sherwin, CM, Christiansen, SB, Duncan, IJ, Erhard, HW, Lay, DC, Mench, JA, et al. Guidelines for the ethical use of animals in applied ethology studies. Appl Anim Behav Sci. (2003) 81:291–305. doi: 10.1016/S0168-1591(02)00288-5
31. Lascelles, BDX, McFarland, JM, and Swann, H. Guidelines for safe and effective use of NSAIDs in dogs. Vet Ther. (2005) 6:237–51.
32. Althubaiti, A . Sample size determination: a practical guide for health researchers. J Gen Fam Med. (2023) 24:72–8. doi: 10.1002/jgf2.600
33. Charan, J, and Biswas, T. How to calculate sample size for different study designs in medical research? Indian J Psychol Med. (2013) 35:121–6. doi: 10.4103/0253-7176.116232
34. Doyle, DJ, Goyal, A, Bansal, P, and Garmon, EH. American Society of Anesthesiologists Classification (ASA Class). Treasure Island, FL: StatPearls Publishing (2020).
35. Chohan, AS, and Davidow, EB. Clinical pharmacology and Administration of Fluid, electrolyte, and blood component solutions In: AS Chohan , editor. Veterinary Anesthesia and Analgesia. Chichester, UK: John Wiley and Sons, Ltd. (2017). 386–413.
36. Grint, NJ, Burford, J, and Dugdale, AHA. Does pethidine affect the cardiovascular and sedative effects of dexmedetomidine in dogs? J Small Anim Pract. (2009) 50:62–6. doi: 10.1111/j.1748-5827.2008.00670.x
37. Branson, KR . Injectable and alternative anesthniques In: WJ Traquilli, JC Thurmon, and KA Grimm, editors. Lumbs and Jones Veterinary Anesthesia and analgesia. Hoboken, NJ: Blackwell Publishing Ltd. (2007). 273–99.
38. Kim, J, Heo, J, Ji, D, and Kim, M-S. Quantitative assessment of pupillary light reflex in normal and anesthetized dogs: a preliminary study. J Vet Med Sci. (2015) 77:475–8. doi: 10.1292/jvms.14-0387
39. Platt, SR, Radaelli, ST, and McDonnell, JJ. The prognostic value of the modified Glasgow coma scale in head trauma in dogs. J Vet Intern Med. (2001) 15:581–4. doi: 10.1892/0891-6640(2001)015<0581:TPVOTM>2.3.CO;2
40. de Audícana-Jimenez, L, de Aberasturi, Y, Vallejo-De la Cueva, A, Aretxabala-Cortajarena, N, Quintano-Rodero, A, Rodriguez-Nuñez, C, et al. Pupillary dilation reflex and behavioural pain scale: study of diagnostic test. Intens Crit Care Nurs. (2023) 74:103332. doi: 10.1016/j.iccn.2022.103332
41. Tosi, F, Gatto, A, Capossela, L, Ferretti, S, Mancino, A, Curatola, A, et al. Role of the pupillometer in the assessment of pain in the sedation of pediatric patients. Eur Rev Med Pharmacol Sci. (2021) 25:6349–55. doi: 10.26355/eurrev_202110_27008
42. Isnardon, S, Vinclair, M, Genty, C, Hebrard, A, Albaladejo, P, and Payen, J-F. Pupillometry to detect pain response during general anaesthesia following unilateral popliteal sciatic nerve block. Eur J Anaesthesiol. (2013) 30:429–34. doi: 10.1097/EJA.0b013e32835f0030
43. Guglielminotti, J, Mentré, F, Gaillard, J, Ghalayini, M, Montravers, P, and Longrois, D. Assessment of pain during labor with Pupillometry. Anesth Analg. (2013) 116:1057–62. doi: 10.1213/ANE.0b013e31828a7218
44. Sagar, DR, Kelly, S, Millns, PJ, O’Shaughnessey, CT, Kendall, DA, and Chapman, V. Inhibitory effects of CB1 and CB2 receptor agonists on responses of DRG neurons and dorsal horn neurons in neuropathic rats. Eur J Neurosci. (2005) 22:371–9. doi: 10.1111/j.1460-9568.2005.04206.x
45. Freundt-Revilla, J, Kegler, K, Baumgärtner, W, and Tipold, A. Spatial distribution of cannabinoid receptor type 1 (CB1) in normal canine central and peripheral nervous system. PLoS One. (2017) 12:e0181064. doi: 10.1371/journal.pone.0181064
46. Galiazzo, G, Giancola, F, Stanzani, A, Fracassi, F, Bernardini, C, Forni, M, et al. Localization of cannabinoid receptors CB1, CB2, GPR55, and PPARα in the canine gastrointestinal tract. Histochem Cell Biol. (2018) 150:187–205. doi: 10.1007/s00418-018-1684-7
47. Turcotte, C, Blanchet, M-R, Laviolette, M, and Flamand, N. The CB2 receptor and its role as a regulator of inflammation. Cell Mol Life Sci. (2016) 73:4449–70. doi: 10.1007/s00018-016-2300-4
48. Aebersold, A, Duff, M, Sloan, L, and Song, Z-H. Cannabidiol signaling in the eye and its potential as an ocular therapeutic agent. Cell Physiol Biochem. (2021) 55:1–14. doi: 10.33594/000000371
49. Porcella, A, Maxia, C, Gessa, GL, and Pani, L. The human eye expresses high levels of CB1 cannabinoid receptor mRNA and protein. Eur J Neurosci. (2000) 12:1123–7. doi: 10.1046/j.1460-9568.2000.01027.x
50. Grotenhermen, F . Cannabinoids. Curr Drug Target CNS Neurol Disord. (2005) 4:507–30. doi: 10.2174/156800705774322111
51. Silver, RJ . The endocannabinoid system of animals. Animals. (2019) 9:686. doi: 10.3390/ani9090686
52. Ruhaak, LR, Felth, J, Karlsson, PC, Rafter, JJ, Verpoorte, R, and Bohlin, L. Evaluation of the cyclooxygenase inhibiting effects of six major cannabinoids isolated from Cannabis sativa. Biol Pharm Bull. (2011) 34:774–8. doi: 10.1248/bpb.34.774
53. Takeda, S, Misawa, K, Yamamoto, I, and Watanabe, K. Cannabidiolic acid as a selective Cyclooxygenase-2 inhibitory component in Cannabis. Drug Metab Dispos. (2008) 36:1917–21. doi: 10.1124/dmd.108.020909
54. Zurier, RB, and Burstein, SH. Cannabinoids, inflammation, and fibrosis. FASEB J. (2016) 30:3682–9. doi: 10.1096/fj.201600646R
55. Jin, F, and Chung, F. Multimodal analgesia for postoperative pain control. J Clin Anesth. (2001) 13:524–39. doi: 10.1016/S0952-8180(01)00320-8
56. Pieper, K . Perioperative Schmerztherapie bei Hund und Katze – eine Übersicht. Tierärztliche Praxis Ausgabe K. (2016) 44:200–8. doi: 10.15654/TPK-160084
57. Caulkett, N, Read, M, Fowler, D, and Waldner, C. A comparison of the analgesic effects of butorphanol with those of meloxicam after elective ovariohysterectomy in dogs. Can Vet J. (2003) 44:565–70.
58. Maihöfner, C, Tegeder, I, Euchenhofer, C, DeWitt, D, Brune, K, Bang, R, et al. Localization and regulation of cyclo-oxygenase-1 and-2 and neuronal nitric oxide synthase in mouse spinal cord. Neuroscience. (2000) 101:1093–108. doi: 10.1016/S0306-4522(00)00361-4
59. Tegeder, I, Niederberger, E, Vetter, G, Bräutigam, L, and Geisslinger, G. Effects of selective COX-1 and-2 inhibition on formalin-evoked nociceptive behaviour and prostaglandin E2 release in the spinal cord. J Neurochem. (2008) 79:777–86. doi: 10.1046/j.1471-4159.2001.00613.x
60. Vanegas, H, and Schaible, H-G. Prostaglandins and cycloxygenases in the spinal cord. Prog Neurobiol. (2001) 64:327–63. doi: 10.1016/S0301-0082(00)00063-0
61. Brenneis, C, Maier, TJ, Schmidt, R, Hofacker, A, Zulauf, L, Jakobsson, P-J, et al. Inhibition of prostaglandin E 2 synthesis by SC-560 is independent of cyclooxygenase 1 inhibition. FASEB J. (2006) 20:1352–60. doi: 10.1096/fj.05-5346com
62. Ghilardi, JR . Constitutive spinal Cyclooxygenase-2 participates in the initiation of tissue injury-induced hyperalgesia. J Neurosci. (2004) 24:2727–32. doi: 10.1523/JNEUROSCI.5054-03.2004
63. Larson, CM, Wilcox, GL, and Fairbanks, CA. The study of pain in rats and mice. Comp Med. (2019) 69:555–70. doi: 10.30802/AALAS-CM-19-000062
64. Rollins, M, Feiner, JR, Lee, JM, Shah, S, and Larson, M. Infrared Pupillometry. Anesthesiology. (2014) 121:1037–44. doi: 10.1097/ALN.0000000000000384
65. Hernández-Avalos, I, Valverde, A, Ibancovichi-Camarillo, JA, Sánchez-Aparicio, P, Recillas-Morales, S, Rodríguez-Velázquez, D, et al. Clinical use of the parasympathetic tone activity index as a measurement of postoperative analgaesia in dogs undergoing ovariohysterectomy. J Vet Res. (2021) 65:117–23. doi: 10.2478/jvetres-2021-0004
66. Larson, MD . Mechanism of opioid-induced pupillary effects. Clin Neurophysiol. (2008) 119:1358–64. doi: 10.1016/j.clinph.2008.01.106
67. Poulsen Nautrup, B, and Hörstermann, D. Pharmacodynamic and pharmacokinetic aspects of the non-inflammatory non-steroidal agent meloxicam in dogs. Dtsch Tierarztl Wochenschr. (1999) 106:94–100. http://www.ncbi.nlm.nih.gov/pubmed/10220944.
68. Lamont, LA . Multimodal pain Management in Veterinary Medicine: the physiologic basis of pharmacologic therapies. Vet Clin N Am Small Anim Pract. (2008) 38:1173–86. doi: 10.1016/j.cvsm.2008.06.005
69. Mader, TL, Davis, MS, and Brown-Brandl, T. Environmental factors influencing heat stress in feedlot cattle. J Anim Sci. (2006) 84:712–9. doi: 10.2527/2006.843712x
70. Srithunyarat, T, Höglund, OV, Hagman, R, Olsson, U, Stridsberg, M, Lagerstedt, A-S, et al. Catestatin, vasostatin, cortisol, temperature, heart rate, respiratory rate, scores of the short form of the Glasgow composite measure pain scale and visual analog scale for stress and pain behavior in dogs before and after ovariohysterectomy. BMC Res Notes. (2016) 9:381. doi: 10.1186/s13104-016-2193-1
71. Reid, J, Nolan, AM, and Scott, EM. Measuring pain in dogs and cats using structured behavioural observation. Vet J. (2018) 236:72–9. doi: 10.1016/j.tvjl.2018.04.013
72. Saberi Afshar, F, Shekarian, M, Baniadam, A, Avizeh, R, Najafzadeh, H, and Pourmehdi, M. Comparison of different tools for pain assessment following ovariohysterectomy in bitches. Iran J Vet Med. (2017) 11:255–65. doi: 10.22059/ijvm.2017.138815.1004701
73. Matičić, D, Stejskal, M, Pećin, M, Kreszinger, M, Pirkić, B, Vnuk, D, et al. Correlation of pain assessment parameters in dogs with cranial cruciate surgery. Vet Arhiv. (2010) 80:597–609.
74. Fazio, E, Medica, P, Cravana, C, Pupillo, A, and Ferlazzo, A. Effects of ovariohysterectomy in dogs and cats on adrenocortical, haematological and behavioural parameters. Acta Sci Vet. (2015) 43:1–8.
75. Hernández-Avalos, I, Valverde, A, Ibancovichi-Camarillo, JA, Sánchez-Aparicio, P, Recillas-Morales, S, Osorio-Avalos, J, et al. Clinical evaluation of postoperative analgesia, cardiorespiratory parameters and changes in liver and renal function tests of paracetamol compared to meloxicam and carprofen in dogs undergoing ovariohysterectomy. PLoS One. (2020) 15:e0223697. doi: 10.1371/journal.pone.0223697
76. Holton, LL, Scott, EM, Nolan, AM, Reid, J, and Welsh, E. Relationship between physiological factors and clinical pain in dogs scored using a numerical rating scale. J Small Anim Pract. (1998) 39:469–74. doi: 10.1111/j.1748-5827.1998.tb03681.x
77. Hall, CA, and Chilcott, RP. Eyeing up the future of the pupillary light reflex in neurodiagnostics. Diagnostics. (2018) 8:19. doi: 10.3390/diagnostics8010019
78. Pupillometry, MS . Psychology, physiology, and function. J Cogn. (2018) 1:18. doi: 10.5334/joc.18
79. Zele, AJ, and Gamlin, PD. Editorial: the pupil: behavior, anatomy, physiology and clinical biomarkers. Front Neurol. (2020) 11:211. doi: 10.3389/fneur.2020.00211
80. Lukaszewicz, A-C, Dereu, D, Gayat, E, and Payen, D. The relevance of pupillometry for evaluation of analgesia before noxious procedures in the intensive care unit. Anesth Analg. (2015) 120:1297–300. doi: 10.1213/ANE.0000000000000609
81. Charier, D, Vogler, M-C, Zantour, D, Pichot, V, Martins-Baltar, A, Courbon, M, et al. Assessing pain in the postoperative period: analgesia nociception IndexTM versus pupillometry. Br J Anaesth. (2019) 123:e322–7. doi: 10.1016/j.bja.2018.09.031
82. Szabadi, E . Functional Organization of the Sympathetic Pathways Controlling the pupil: light-inhibited and light-stimulated pathways. Front Neurol. (2018) 9:1069. doi: 10.3389/fneur.2018.01069
83. Yang, LL, Niemann, CU, and Larson, MD. Mechanism of pupillary reflex dilation in awake volunteers and in organ donors. Anesthesiology. (2003) 99:1281–6. doi: 10.1097/00000542-200312000-00008
84. Larson, MD, Tayefeh, F, Sessler, DI, Daniel, M, and Noorani, M. Sympathetic nervous system does not mediate reflex pupillary dilation during Desflurane anesthesia. Anesthesiology. (1996) 85:748–54. doi: 10.1097/00000542-199610000-00009
85. Dyson, DH . Perioperative pain Management in Veterinary Patients. Vet Clin N Am Small Anim Pract. (2008) 38:1309–27. doi: 10.1016/j.cvsm.2008.06.006
86. Johnson, C . Research tools for the measurement of pain and nociception. Animals. (2016) 6:10071. doi: 10.3390/ani6110071
87. Slingsby, L . Multimodal analgesia for postoperative pain relief. In Pract. (2008) 30:208–12. doi: 10.1136/inpract.30.4.208
88. Miranda-Cortés, AE, Ruiz-García, AG, Olivera-Ayub, AE, Garza-Malacara, G, Ruiz-Cervantes, JG, Toscano-Zapien, JA, et al. Cardiorespiratory effects of epidurally administered ketamine or lidocaine in dogs undergoing ovariohysterectomy surgery: a comparative study. Iran J Vet Res. (2020) 21:92–6.
89. Steagall, PV, and Monteiro, BP. Acute pain in cats: recent advances in clinical assessment. J Feline Med Surg. (2019) 21:25–34. doi: 10.1177/1098612X18808103
90. Malmström, T, and Kröger, RHH. Pupil shapes and lens optics in the eyes of terrestrial vertebrates. J Exp Biol. (2006) 209:18–25. doi: 10.1242/jeb.01959
91. Mota-Rojas, D, Napolitano, F, Strappini, A, Orihuela, A, Ghezzi, MD, Hernández-Ávalos, I, et al. Pain at the slaughterhouse in ruminants with a focus on the neurobiology of sensitisation. Animals. (2021) 11:1085. doi: 10.3390/ani11041085
92. Ruíz-López, P, Domínguez, JM, and Granados, MM. Intraoperative nociception-antinociception monitors: a review from the veterinary perspective. Vet Anaesth Analg. (2020) 47:152–9. doi: 10.1016/j.vaa.2019.09.006
93. Mota-Rojas, D, Velarde, A, Marcet-Rius, M, Orihuela, A, Bragaglio, A, Hernández-Ávalos, I, et al. Analgesia during parturition in domestic animals: perspectives and controversies on its use. Animals. (2022) 12:2686. doi: 10.3390/ani12192686
94. Whittaker, AL, Muns, R, Wang, D, Martínez-Burnes, J, Hernández-Ávalos, I, Casas-Alvarado, A, et al. Assessment of pain and inflammation in domestic animals using infrared thermography. Animals. (2023) 13:2065. doi: 10.3390/ani13132065
95. Mota-Rojas, D, Olmos-Hernández, A, Verduzco-Mendoza, A, Lecona-Butrón, H, Martínez-Burnes, J, Mora-Medina, P, et al. Infrared thermal imaging associated with pain in laboratory animals. Exp Anim. (2021) 70:1–12. doi: 10.1538/expanim.20-0052
96. Casas-Alvarado, A, Mota-Rojas, D, Hernández-Avalos, I, Martínez-Burnes, J, Rosas, M, Miranda-Cortés, AE, et al. Assessment of thermal response, cardiorespiratory parameters and postoperative analgesia in dogs undergoing ovariohysterectomy with different combinations of epidural anesthesia and isoflurane. J Anim Behav Biometeorol. (2023) 11:e2023009. doi: 10.31893/jabb.23009
97. Domínguez-Oliva, A, Casas-Alvarado, A, Miranda-Cortes, AE, and Hernández-Avalos, I. Clinical pharmacology of tramadol and tapentadol, and their therapeutic efficacy in different models of acute and chronic pain in dogs and cats. J Adv Vet Anim Res. (2021) 8:404. doi: 10.5455/javar.2021.h529
Keywords: pain, pupillometry, dogs, nociception, CBD, meloxicam
Citation: Casas-Alvarado A, Martínez-Burnes J, Hernández-Ávalos I, Mora-Medina P, Miranda-Cortés A, Domínguez-Oliva A and Mota-Rojas D (2024) Assessment of the nociceptive response to the use of cannabidiol alone and in combination with meloxicam through infrared pupillometry in female dogs undergoing elective ovariohysterectomy. Front. Vet. Sci. 11:1380022. doi: 10.3389/fvets.2024.1380022
Edited by:
Caterina Di Bella, University of Camerino, ItalyReviewed by:
Claudia Interlandi, University of Messina, ItalyFederica Serino, University of Camerino, Italy
Copyright © 2024 Casas-Alvarado, Martínez-Burnes, Hernández-Ávalos, Mora-Medina, Miranda-Cortés, Domínguez-Oliva and Mota-Rojas. This is an open-access article distributed under the terms of the Creative Commons Attribution License (CC BY). The use, distribution or reproduction in other forums is permitted, provided the original author(s) and the copyright owner(s) are credited and that the original publication in this journal is cited, in accordance with accepted academic practice. No use, distribution or reproduction is permitted which does not comply with these terms.
*Correspondence: Daniel Mota-Rojas, ZG1vdGExMDBAeWFob28uY29tLm14