- 1College of Animal Science, Shanxi Agricultural University, Jinzhong, Shanxi, China
- 2Department of Medical Oncology, Dana-Farber Cancer Institute, Boston, MA, United States
- 3International Joint Research Laboratory in Universities of Jiangsu Province of China for Domestic Animal Germplasm Resources and Genetic Improvement, Yangzhou, Jiangsu, China
This study aimed to explore the effects of neonatal vitamin A (VA) supplementation on testis development and spermatogenesis. A total of 32 newborn lambs were intramuscularly injected with corn oil (control group) or corn oil + 2500 IU/kg BW VA (VA group). They were slaughtered and sampled at 3 weeks and 8 months of age to analyze spermatogenesis, cell proliferation, hormone secretion, antioxidant status of the testis, and adult sheep sperm parameters. Compared with the control group, the expression of spermatogonial differentiation-related genes in VA group was up-regulated (P < 0.05). Testis weight, seminiferous tubule diameter, number of spermatogonium and spermatocyte, and sperm density increased significantly in VA group at 8 months of age (P < 0.05). Neonatal VA injection upregulated the expression of the cell proliferation marker PCNA and cell cycle-related genes in the testis (P < 0.05). VA increased the concentrations of testosterone (T), luteinizing hormone (LH), and follicle-stimulating hormone (FSH) in the serum and upregulated steroidogenesis-related genes in the testis (P < 0.05). The antioxidant levels in the VA group were maintained at high levels. The total antioxidant capacity (T-AOC), antioxidant enzyme content and antioxidant-related genes were increased in the testis (P < 0.05). Furthermore, neonatal VA injection activated retinoic acid (RA) signaling to maintain the blood-testosterone barrier (BTB) in the testis of 3-week-old sheep. AMP-activated protein kinase (AMPK) and protein kinase B (AKT) signaling were also modulated in the sheep testis (P < 0.05). Taken together, VA supplementation in newborn rams promotes testis development and spermatogenesis to improve fertility.
Introduction
Vitamin A (VA), also known as retinol, is a fat-soluble vitamin that is necessary in animals and is involved in various physiological activities such as vision maintenance, embryonic development, immune regulation, metabolism, organ development, and reproductive regulation (1). It can be considered a feed additive to improve the growth performance of livestock. VA deficiency leads to the inhibition of spermatogonial differentiation, spermatogonial apoptosis, vacuolation, calcification of spermatogenic epithelium, spermatogenic stasis, interruption of the blood-testis barrier (BTB), and degeneration and atrophy of the testis, resulting in sterility in male animals (2). VA supplementation restarts spermatogenesis and restores BTB levels in VA-deficient mice (3).
Retinoic acid (RA) is the active metabolite of VA. VA binds to retinol-binding protein to form a complex for transport in the blood circulation, then enters the cells via plasma membrane diffusion. VA undergoes a two-step enzymatic reaction in cells, in which it is first converted to retinal by alcohol dehydrogenase (ADH) and retinol dehydrogenase, the retinal is then converted to RA by retinal dehydrogenase (also known as ALDH). RA binds to cellular RA-binding protein in the nucleus and then binds with the retinoic acid receptor (RARs)/retinoic acid X receptor (RXRs) dimer to dissociate the RAR/RXR dimer from the retinoic acid reaction elements of the genome and co-suppressors. The inhibitory effect of RAR/RXR dimer on target genes is competitively blocked, and coactivators are recruited to induce the expression of RA-responsive genes (4–6). The RA receptors play a key role in spermatogenesis. The symptoms of targeted knockout of RARA are similar to those of VA-deficient mice, which also lead to pre-meiotic spermatocyte apoptosis and an abnormal spermatogenesis cycle (7).
Spermatogenesis involves the proliferation and differentiation of spermatogonial stem cells (SSCs), spermatocyte meiosis, and spermiation. First, Asingle spermatogonia (As) in the SSCs proliferates to form Apaired spermatogonia (Apr) and Aaligned spermatogonia (Aal). Aal differentiates into A1-A4 spermatogonium, intermediate spermatogonium (In), type B spermatogonium and primary spermatocytes (pre-leptotene spermatocytes). Primary spermatocytes enter meiosis to form sperm cells that undergo morphological changes to form sperm. Immature sperm is released from the Sertoli cells into the lumen and through the testicular reticulum into the epididymis after spermatogenesis is complete. Germ cells at different stages in the testis are arranged successively from the basal to the apical compartment of seminiferous tubules and make up the seminiferous epithelium with Sertoli cells (8, 9). There is a BTB layer between the basal and apical compartments of the seminiferous epithelium. Spermatogonocytes develop into preleptotene spermatocytes and pass through the BTB for further meiosis. The BTB isolates germ cells of the basal compartment from the vasculature; therefore, meiosis is regulated periodically by the spermatogenic epithelium without interference from external factors (10, 11).
RA is a key factor in inducing SSC differentiation and initiating meiosis in males (12–14). However, synergistic regulation among multiple cell types in the testis and its regulatory mechanisms in downstream signaling pathways remain unclear. Moreover, the period after birth is the peak of various tissue and organ development in the body of animals, and the health status of a newborn continues to affect its entire life. Therefore, this study aimed to investigate the effects of neonatal VA supplementation on male animal fertility. The expression of cell proliferation-, steroidogenesis-, and antioxidation-related genes and the activity of key signaling pathways in the testes were determined. Various sperm parameters were tested after the sheep grew up, providing a theoretical basis for the effect of VA supplementation on the fertility of ruminants.
Materials and methods
Animals and treatments
A total of 32 hybrid F1 generation male lambs of Dorper sheep (♂) × Hu sheep (♀), which were newborns and had similar weights (3.5 ± 0.5 kg), were selected from a farm in Taigu county, Shanxi Province. All lambs were twins derived from the same father using artificial insemination technology. The lambs were randomly divided into the control and VA groups, with 16 in each group. They were reared with ewes and were free to drink their breast milk. The lambs were intramuscularly injected with corn oil (control group) or corn oil + 2500 IU/kg BW VA (PHR1235; Sigma, St. Louis, MO, USA) once a week at fixed times from birth to 3 weeks. The VA dose was selected based on our previous studies in mice (15) and cattle (16), which were converted to body weight. At 3 weeks of age, eight lambs from each group were randomly slaughtered to collect blood and testicular tissue. The remaining eight lambs in each group were weaned at 3 months of age, fed a backgrounding diet for 55 days, and then transferred to the finishing diet. From weaning to harvest, the daily intake of concentrate was increased from 0.3 to 1.75 kg, and grass hay was increased from 0.25 to 0.5 kg per lamb. The ingredients and nutrient composition of the diet, which contains 13,000 IU VA / kg concentrate, and the growth performance of the lambs have been previously published (17). They were slaughtered until 8 months of age for blood, testis, and epididymis tissue collection. Body and testis weights were measured to calculate the coefficients of the testes.
Testicular tissue from 3-week-old and 8-month-old sheep was used to obtain tissue slices, and the other was used to detect spermatogonial differentiation, cell cycle, steroidogenesis, antioxidation-related genes, RA, AMPK, AKT signaling, and the antioxidant enzyme levels. Blood was used to detect systemic steroid hormone. In addition, 8-month-old sheep epididymal sperm were collected to detect the expression of protein related to energy metabolism and sperm activity parameters.
Hematoxylin and eosin staining
The testicular samples were fixed in 4% paraformaldehyde for 24 h, dehydrated in an ethanol gradient, and made transparent in xylene. The samples were then embedded in paraffin, and each sample was sectioned at a thickness of 6 μm using a microtome (Leica, Wetzlar, Germany). The sample sections were serially dewaxed, rehydrated with xylene and ethanol, and stained with hematoxylin and eosin. The stained sections were dehydrated, made transparent, and imaged under a microscope (Olympus, Tokyo, Japan).
qRT-PCR analysis
The obtained testicular tissue was ground into a powder. Total RNA was extracted using RNAiso Plus (Takara, Tokyo, Japan) and reverse-transcribed into cDNA using the PrimeScript RT Reagent Kit with gDNA Eraser (Perfect Real Time) (Takara, Tokyo, Japan) according to the manufacturer's protocol. Primers were designed to span exons and were synthesized by Sangon Biotech Inc. (Shanghai, China). The primer sequences are shown in Table 1. qRT-PCR was conducted on a Bio-Rad Cfx system using the 2 × Real-time PCR Super mix (Mei5bio, Beijing, China). The procedure contained 38 cycles at 95°C for 15 s, 60°C for 15 s, and 72°C for 45 s. The amplification efficiency of all genes was calculated to ensure that they were in 90%−110%. The dissolution curves were unimodal. Ultimately, the relative abundance of mRNA expression was analyzed using the 2−ΔΔCt method and normalized to GAPDH.
Western blotting analysis
Western blotting was performed as previously described (18). Briefly, the tissues were powdered and added to an ice-cold protein extraction buffer. The obtained samples were centrifuged to obtain the supernatant, which was denatured. Target proteins were separated using sodium dodecyl-sulfate polyacrylamide gel electrophoresis (80 V, 30 min; 120 V, 1 h) and transferred onto a nitrocellulose membrane (100 V, 1.5 h). The membranes were then soaked in skim milk powder (1 h), primary antibody (4°C, overnight), or fluorescent secondary antibody (1 h). Protein bands were scanned using an infrared imaging system (LI-COR Biosciences, Lincoln, NE, US). Protein density was analyzed using ImageJ software (National Institutes of Health, Bethesda, MD, USA) and normalized to β-actin.
The antibodies against p-AMP-activated protein kinase (AMPK)α1 (4184S, 1:1000), protein kinase B (AKT; 9272S, 1:1000), p-AKT (4060S, 1:2000), initiation factor 4E binding protein 1 alpha (4EBP1α; 9644S, 1:1000) and p-4EBP1α (2855S, 1:1000) were purchased from the Cell Signaling Technology (Danvers, MA, USA). AMPKα1 (bs-10344R, 1:500) and β-actin (bsm-33036M, 1:8000) were obtained from the Biosynthesis Biotechnology Co., Ltd. (Beijing, China). Fluorescent secondary antibodies goat anti-rabbit IgG (926-32211, 1:20000) and goat anti-mouse IgG (926-68070, 1:20000) were obtained from LI-COR Biosciences (Lincoln, NE, USA).
Enzyme-linked immunosorbent assay
The serum concentrations of testosterone (T), luteinizing hormone (LH), and follicle-stimulating hormone (FSH) were measured according to the manufacturer's instructions of the ELISA kits (MM-3460101, MM-3460401, and MM-124501, Jiangsu Meimian Industrial Co., Ltd, China). Briefly, serum was added to microtiter plate wells and incubated at 37°C for 30 min. Next, the horseradish peroxidase-conjugate reagent was added to the wells and incubated at 37°C for 30 min. The chromogen solution was added and incubated at 37°C for 10 min in the dark, and the reaction was stopped with a stop solution. The absorbance of each well was measured at 450 nm using a microplate reader (BioTek, Winooski, USA). The r2 (coefficient of regression) of fitted curves were all >0.996.
Antioxidant analysis
Testicular tissue was powdered in liquid nitrogen, and 1 g of the powder was added to 9 mL of normal saline to obtain a 10% tissue homogenate. The supernatant was obtained by centrifuging at 800 g for 10 min at 4°C. The total protein concentration in the supernatant was measured using a BCA kit (Solarbio, Beijing, China) according to the manufacturer's instructions. Multiple enzyme activity was detected using biochemical kits, according to the manufacturer's protocol. Superoxide dismutase (SOD, A001-3), glutathione peroxidase (GSH-PX, A005-1), catalase (CAT, A007-1), malondialdehyde (MDA, A003-1), and total antioxidant capacity (T-AOC, A015-1) kits were purchased from Nanjing Jiancheng Bioengineering Institute (Nanjing, China). Their intra-assay coefficients of variation (CV) were 5.50%, 3.10%, 1.90%, 3.50% and 3.20%, respectively. And their inter-assay CV were 3.32%, 4.34%, 4.94%, 4.11% and 6.83%.
Sperm evaluation
Computer-assisted sperm analysis
The right cauda epididymis of each sheep was cut into small pieces and soaked in 5 mL of sperm dilution solution containing 27 mg/mL Tris, 14 mg/mL citric acid, 10 mg/mL fructose, 100 IU/mL of penicillin, and 100 μg/mL of streptomycin, then it was gently shaken at 35°C for 30 min to let the sperm flow out freely. The sperm suspension was collected and placed onto preheated microscope slides. Sperm was observed under a phase contrast microscope (UB 200i Series, Olympus, Tokyo, Japan). Sperm motility, viability, density, average path velocity (VAP), straight-line velocity (VSL), and curvilinear velocity (VCL) were analyzed using the CASA system (ISAS®1.2, Proiser R&D, Valencia, Spain).
Sperm membrane integrity analysis
Sperm membrane integrity was assessed using a hypo-osmotic swelling test (HOST). First, 10 μL of a sperm suspension was added to 100 μL of a hypo-osmotic solution containing 13.5 mg/mL fructose and 7.35 mg/mL sodium citrate and incubated for 30 min at 35°C. Afterward, 10 μL of the mixture was evenly dripped onto microscope slides and air-dried. A total of 500 sperms were counted in randomly selected fields under a microscope (BX53, Olympus, Tokyo, Japan). The proportion of sperms with swollen and curved tails was calculated and regarded as the proportion of sperms with a completely functional membrane.
Sperm mitochondrial activity analysis
Sperm mitochondrial activity was assessed using 5,5',6,6'-tetrachloro-1,1',3,3'-tetraethylbenzimi-dazolylcarbocyanine iodide (JC-1) and propidium iodide (PI) double fluorescence staining. The sperm suspension was mixed with 1 mg/mL of JC-1 solution and 1 mg/mL of PI solution and incubated at 35°C for 30 min in the dark. Subsequently, Hancock's solution, containing 3.15 mg/mL of NaCl and 2.59% methanol, was added to the mixture to stop sperm movement. The samples were observed under a fluorescence microscope (BX53, Olympus, Tokyo, Japan), and 500 sperms were counted in multiple randomly selected fields. The yellow sperm tails indicated high mitochondrial membrane potential (MMP) and were regarded as having high mitochondrial activity.
Immunofluorescence
The sperm suspension was dripped evenly onto sticky glass slides and allowed to dry. The slides were immersed in 4% paraformaldehyde for 20 min, 1% Triton X-100 for 30 min, and 1% bovine serum albumin for 1 h. The primary antibody against CLUT3 (bs-1207R, 1:500, Bioss, Beijing, China) was added to the sperm surface at 4°C overnight. The next day, sperms were incubated with FITC-goat anti-rabbit IgG (BOSTER, Wuhan, China) for 1 h and DAPI solution (Sigma-Aldrich, St. Louis, MO, USA) for 20 min in the dark. Sperms were observed and photographed under a fluorescence microscope (BX53; Olympus, Tokyo, Japan).
Statistical analysis
All experiments were repeated three times. The normal distribution and homogeneity of data variance were tested using the Shapiro-Wilk and Browne-Forsythe test, and significance analysis was conducted using a t-test in SPSS (version 22.0; IBM, Armonk, NY, USA). All values are expressed as the mean ± standard error of the mean (SEM). A P < 0.05 was considered statistically significant for all data.
Results
Effects of neonatal VA injection on spermatogenesis in sheep
Through H&E staining, we observed that neonatal VA injection did not change the morphological structure of the testicular tissue or germ cells of 3-week-old sheep, but increased the diameter of seminiferous tubules and the number of spermatogonium and spermatocyte in 8-month-old sheep testis (Figure 1A). Neonatal VA injection promoted the mRNA expression of STRA8 in the testes of 3-week-old sheep, and the mRNA expression of STRA8 and KIT in the testes of 8-month -old sheep (P < 0.05) (Figure 1B). Immunofluorescence analysis showed that neonatal VA injection did not affect the expression of the energy metabolism-related protein glucose transporter 3 in the sperm of 8-month-old sheep (Figure 1C). Multiple sperm parameters were detected using the CASA, HOST, and JC-1/PI double fluorescence staining method (Table 2). Neonatal VA injection significantly increased sperm density (P < 0.05) but did not change sperm motility, viability, movement speed, membrane integrity, or MMP (P > 0.05) in the sperm suspension of 8-month-old sheep. In addition, testicular weight significantly increased in 8-month-old sheep in the VA group (P < 0.05), but there was no difference in the testicular coefficient (testicular weight/body weight) (P > 0.05).
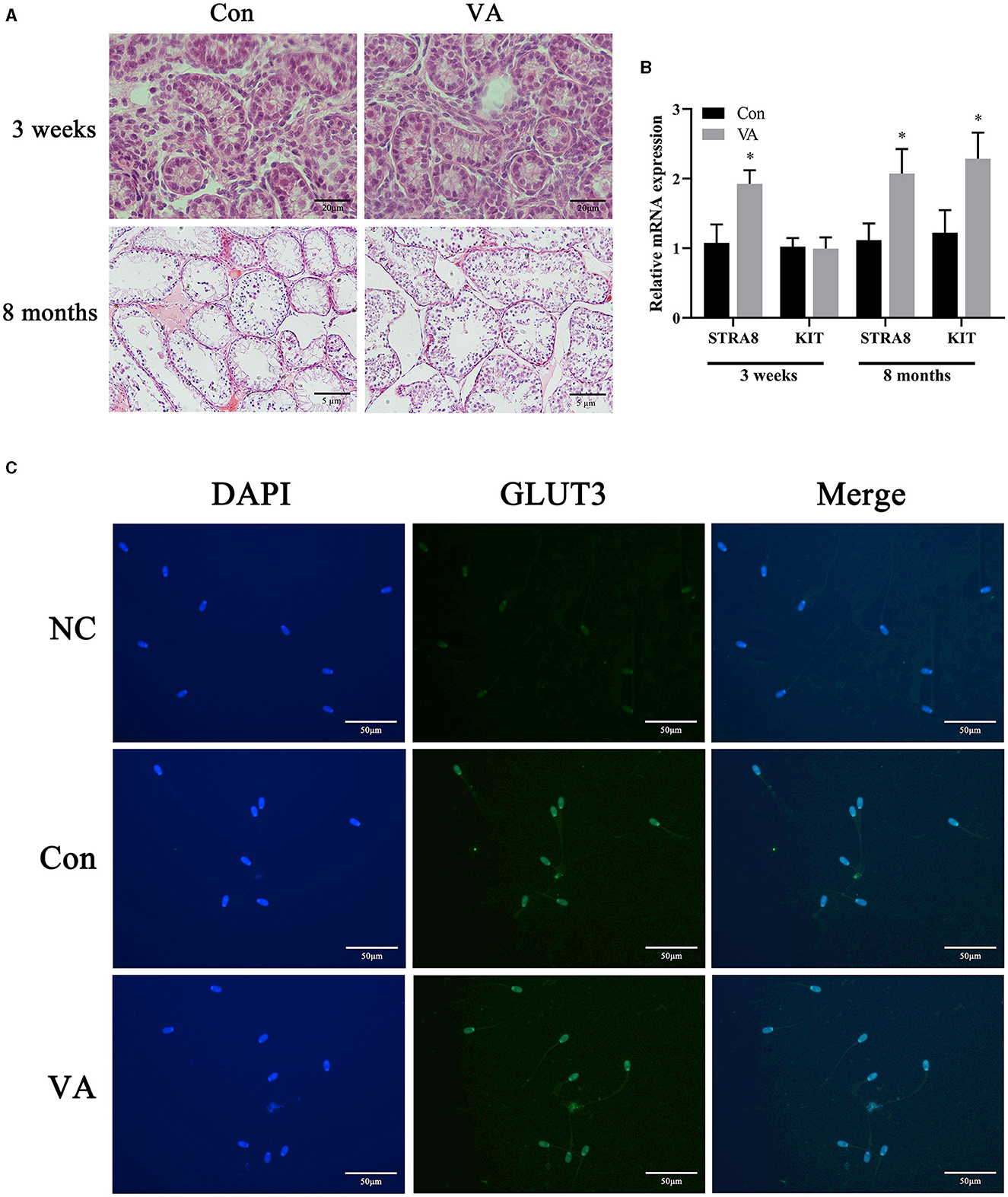
Figure 1. Spermatogonial differentiation and sperm energy metabolism related protein expression. HE staining of sheep testes (A). Relative mRNA expression of spermatogonial differentiation related genes in sheep testes (B). Immunofluorescence of sperm from 8-month-old sheep (C). *P < 0.05.
Effects of neonatal VA injection on testicular cell proliferation in sheep
The mRNA expression of cell cycle-related genes in the testicular tissue was tested after neonatal VA injection. The results showed that the mRNA expression of PCNA, CCND1, and CCND2 increased (P < 0.01), whereas the mRNA expression of P21 decreased (P < 0.05) at 3 weeks of age. There was no effect on the mRNA expression of CDK1, CDK4, and P53 at 3 weeks of age (Figure 2A). When the sheep grew to 8 months of age, the mRNA expression of PCNA, CDK1, and CDK4 increased (P < 0.05), whereas the mRNA expression of CCND1, CCND2, and P53 showed no difference in the testis (P > 0.05) compared with the control group (Figure 2B).
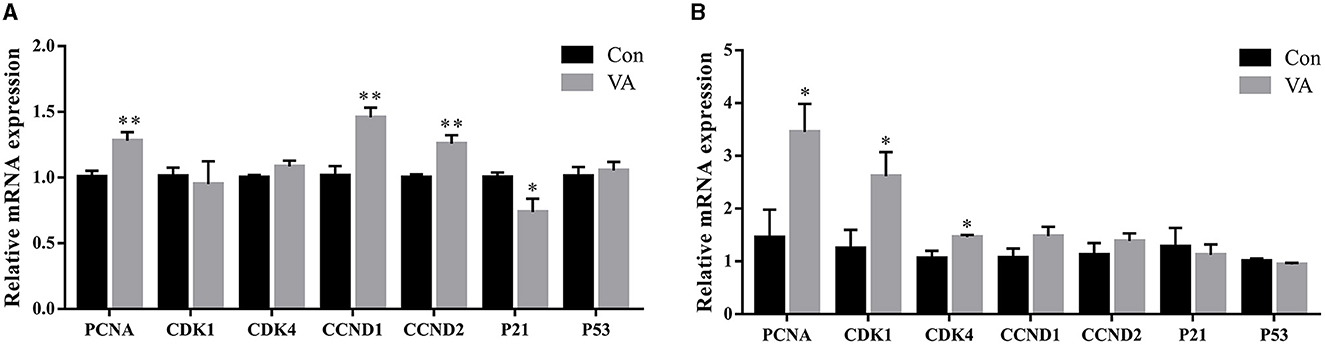
Figure 2. Proliferation of testicular cells. Relative mRNA expression of proliferative related genes in testes of 3-week-old sheep (A) and 8-month-old sheep (B). *P < 0.05, **P < 0.01.
Effects of neonatal VA injection on body hormone levels in sheep
The expression of steroidogenesis-related genes in the testicular tissue and the concentrations of hormones in the serum were measured. The results showed that the mRNA expression of the steroidogenesis-related gene HSD3B1 was upregulated (P < 0.01), whereas the mRNA expression of CYP11A1, CYP17A1, STAR, and HSD17B3 remained unchanged (P > 0.05) in the testes of 3-week-old sheep after neonatal VA injection (Figure 3A). The mRNA expression of HSD17B3 increased (P < 0.05), whereas there was no difference in the mRNA expression of CYP11A1, CYP17A1, STAR, or HSD3B1 (P > 0.05) in the testes of 8-month-old sheep after neonatal VA injection (Figure 3C). The levels of serum hormones T, LH, and FSH in the VA group at 3 weeks and 8 months of age were higher than those in the control group using ELISA (P < 0.05) (Figures 3B, D).
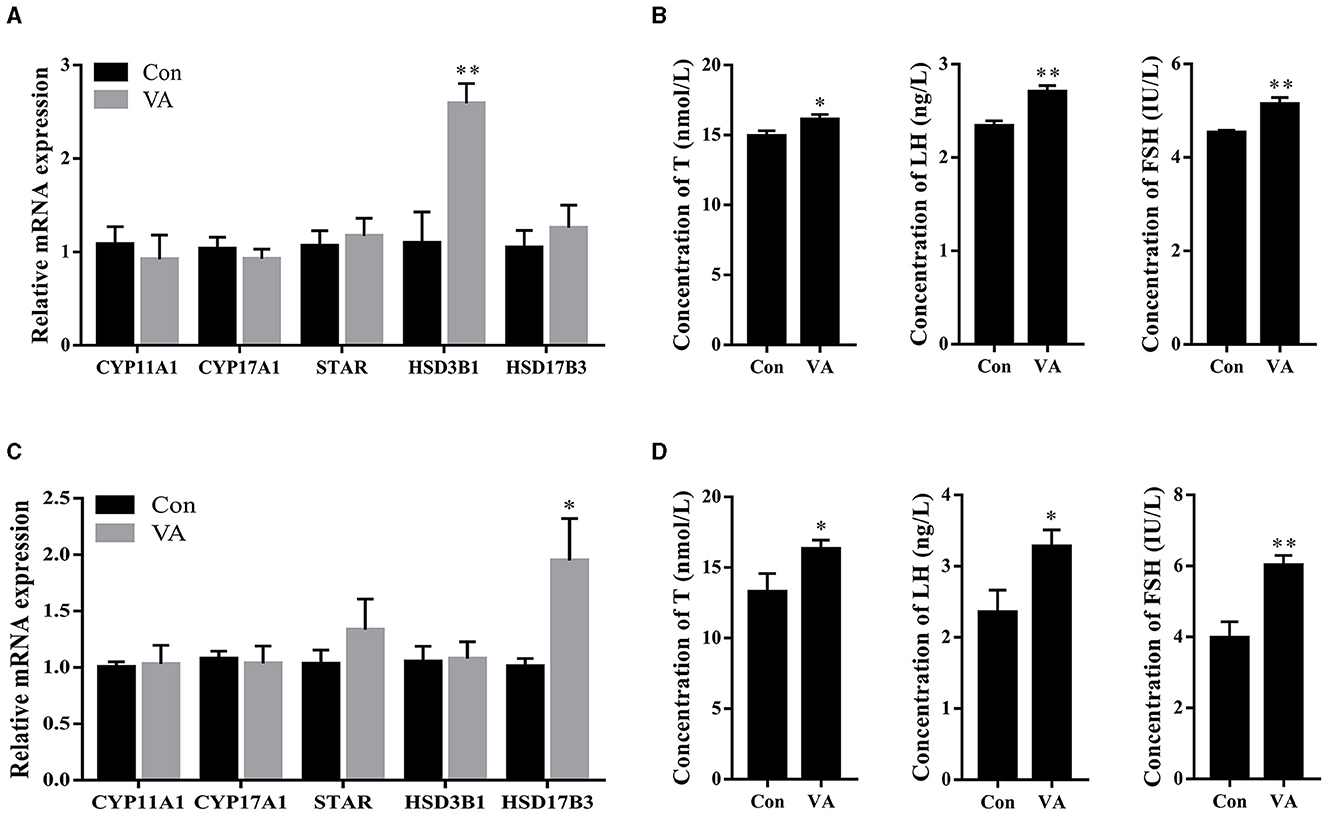
Figure 3. Synthesis and secretion of hormone. Expression of steroidogenesis related genes in testie of 3-week-old sheep (A). Concentrations of hormone T, LH and FSH in serum of sheep at 3 weeks of age (B). Expression of steroidogenesis related genes in testie of 8-month old sheep (C). Concentrations of hormone T, LH and FSH in serum of sheep at 8 month of age (D). *P < 0.05, **P < 0.01.
Effects of neonatal VA injection on antioxidant status in sheep testis
The abundance of antioxidant-related genes and antioxidant enzymes in sheep testes was detected. The results showed that the mRNA expression of SOD2 increased, whereas the mRNA expression of CAT and GPx4 showed no difference in the testes at 3 weeks of age after neonatal VA injection (P < 0.05) (Figure 4A). The abundance of the antioxidant enzymes SOD and T-AOC increased (P < 0.05), whereas the abundances of GSH-PX, CAT, and MDA were not affected (P > 0.05) in the testes of 3-week-old sheep (Figure 4B). Similarly, the mRNA expression levels of SOD2, CAT, and GPx4 increased (P < 0.05) in the testes of 8-month-old sheep in the VA group (Figure 4C). The levels of the antioxidant enzymes SOD, GSH-PX, and T-AOC increased (P < 0.05), MDA decreased (P < 0.05), and CAT remained unchanged in the testes at 8 months of age (P > 0.05) (Figure 4D).
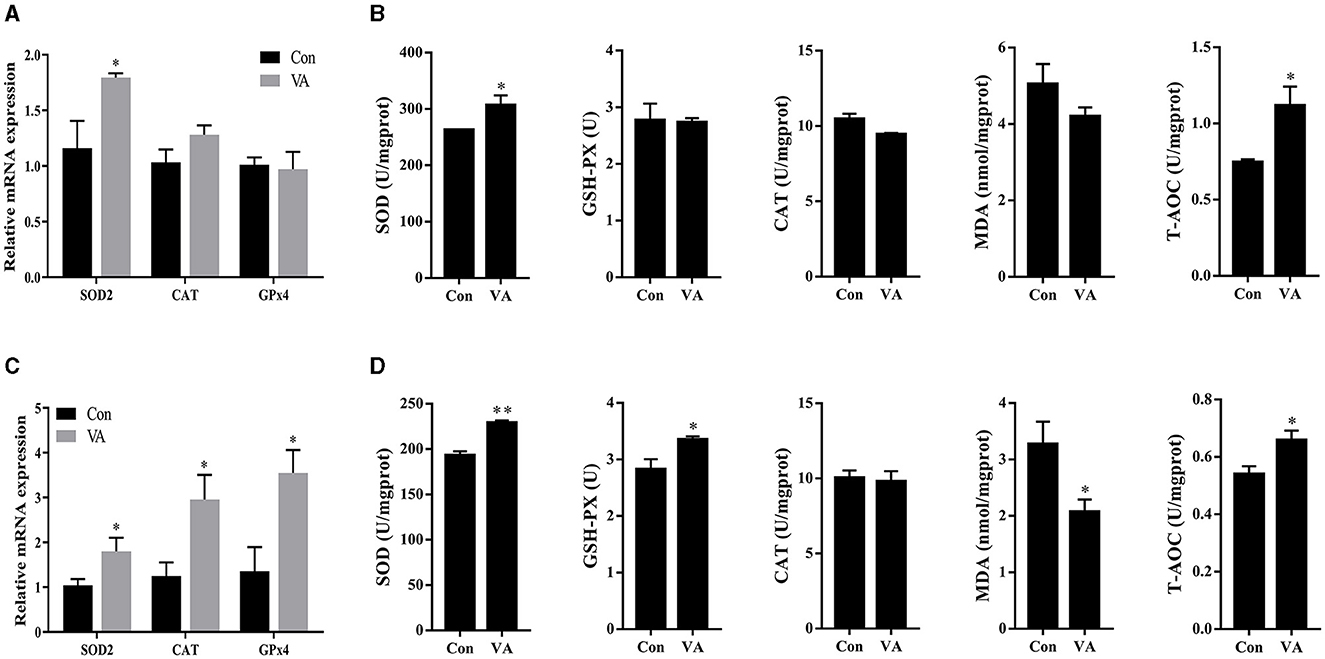
Figure 4. Antioxidant status of testis. Relative mRNA expression of antioxidant related genes in testes of 3-week-old sheep (A). Abundance of antioxidant enzymes in testes of 3-week-old sheep (B). Relative mRNA expression of antioxidant related genes in testes of 8-month-old sheep (C). Abundance of antioxidant enzymes in testes of 8-month-old sheep (D). *P < 0.05, **P < 0.01.
Effects of neonatal VA injection on RA signaling pathway in sheep testis
RA is an intermediate VA metabolite. To explore the regulation of VA in the sheep testes, we measured the expression of genes related to the RA signaling pathway. After neonatal VA injection, the mRNA expression of the RA receptors RARA and RAR-related orphan receptor A was upregulated (P < 0.01), whereas the mRNA expression of the RA receptor RARB and RXRA did not differ in the testes of 3-week-old sheep (P > 0.05) (Figure 5A). Moreover, the mRNA expression of RA synthetases ADH1C (P < 0.01) and ALDH1A1 (P < 0.05) was downregulated, whereas the mRNA expression of RA synthetases ADH4 and ALDH1A2 was not affected (P > 0.05) (Figure 5B). The mRNA expression of the RA lyase CYP26B1 was upregulated (P < 0.05), whereas the mRNA expression of the RA lyase CYP26A1 was not affected (P > 0.05) (Figure 5C). However, there were no significant differences in RA receptor, RA synthetase, or RA lyase expression in the testes of 8-month-old sheep between the two groups (P > 0.05) (Figures 5D–F).
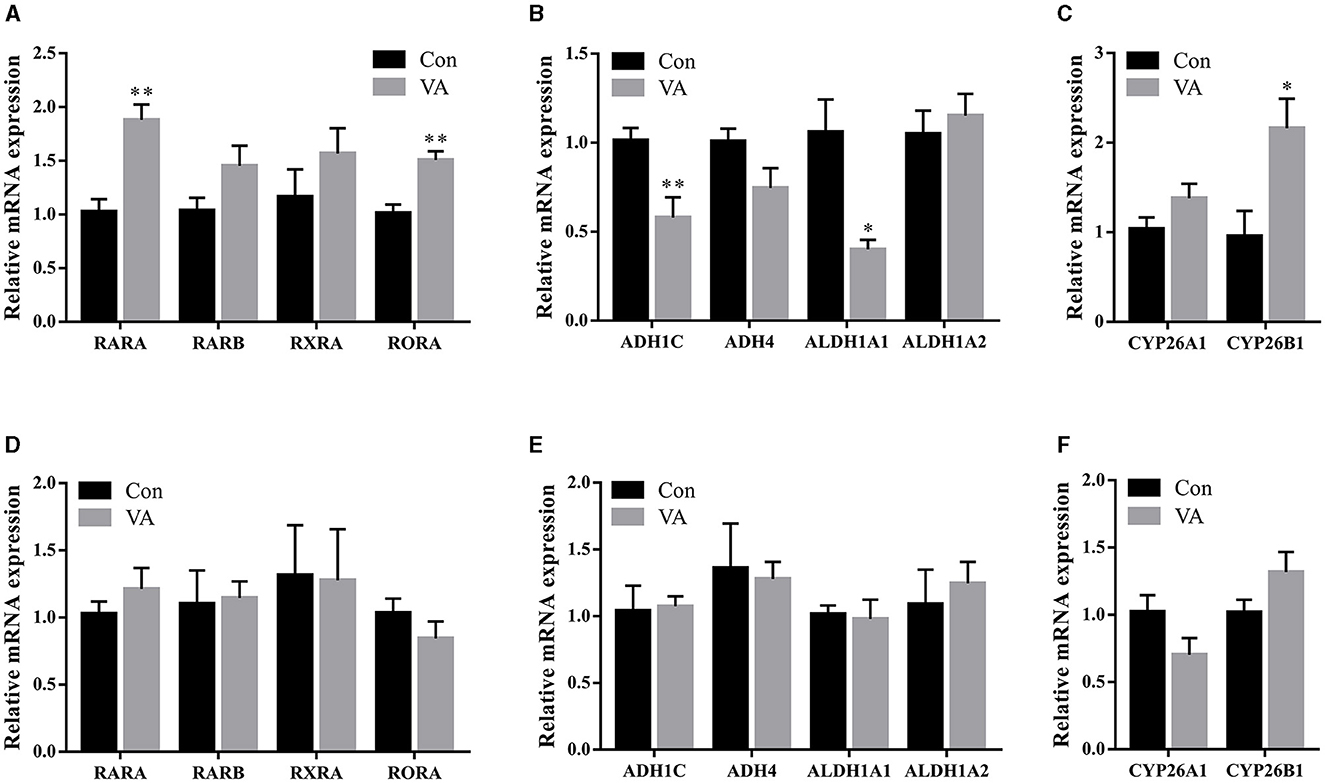
Figure 5. Expression of key genes in RA signaling pathway. Relative mRNA expression of RA receptor (A), RA synthetase (B), RA lyase (C) in testes of 3-week-old sheep. Relative mRNA expression of RA receptor (D), RA synthetase (E), RA lyase (F) in testes of 8-month-old sheep. *P < 0.05, **P < 0.01.
Effects of neonatal VA injection on AMPK/AKT signaling pathway in sheep testis
The protein abundances of AMPK and AKT signaling pathways in the testes of 3-week-old and 8-month-old sheep were measured after neonatal VA injection in neonates. The results showed no difference in the total protein abundance of AMPK, AKT, and 4EBP1α in the testes at 3 weeks or 8 months of age (Figures 6A, B). The abundance of phosphorylated protein p-AMPK increased (P < 0.01), p-AKT decreased (P < 0.05), and p-4EBP1α remained (P > 0.05) at 3 weeks of age (Figure 6A). The abundance of phosphorylated protein p-AMPK decreased (P < 0.05), p-AKT increased (P < 0.05), and p-4EBP1α was not affected (P > 0.05) at 8 months of age (Figure 6B).
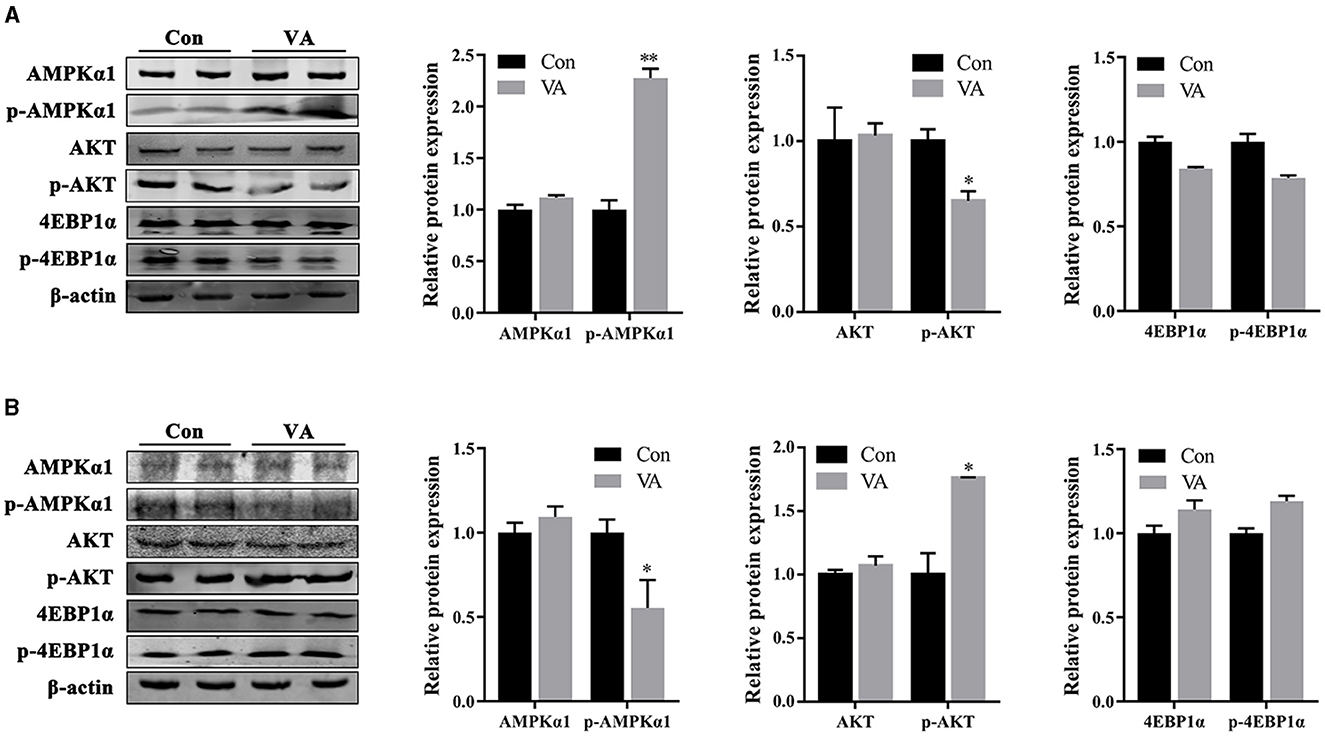
Figure 6. Protein abundance of AMPK and AKT signaling pathways. Western blot were performed to determine the activity of signaling pathway in testes of 3-week-old sheep (A) and 8-month-old sheep (B). *P < 0.05, **P < 0.01.
Discussion
In male mammals, germ cells in the testes are isolated from RA signaling, maintain mitotic activity, and stop at the G0/G1 phase of mitosis during the fetal stage. After birth, the developmental transitions of spermatogenesis include germ cell proliferation, transition to spermatogonia, spermatogonia differentiation, and initiation of primary spermatocyte meiosis, all of which are strictly regulated by RA (19). RA induces the expression of STRA8 and KIT in undifferentiated rodent spermatogonocytes (20–22). KIT is a marker of spermatogonial differentiation. STRA8 is a marker gene for meiosis initiation that promotes spermatogonial differentiation (23). Although neonatal VA supplementation did not cause morphological changes in the testes of lambs, the expression of STRA8 in the testis was increased. Neonatal VA supplementation may promote spermatogonial differentiation and primary spermatocyte meiosis in lamb testes. Compared with controls, testis weight, seminiferous tubule diameter, number of spermatogonium and spermatocyte, and sperm density increased significantly when VA-supplemented lambs grew, suggesting that neonatal VA supplementation promote the proliferation of testicular cells to improve the spermatogenic potential of sheep throughout their life. In addition, motility, viability, movement speed, membrane integrity, MMP, and expression of the energy metabolism-related protein glucose transporter 3 in sperm indicated that neonatal VA supplementation did not improve sperm activity in adult sheep.
The differentiation ability of spermatogonocytes is closely associated with their proliferative activity. To determine whether neonatal VA supplementation affected the proliferative ability of testicular cells, cell proliferation-related genes were examined. As a marker gene for cell proliferation, PCNA regulates the formation of chromatin, DNA damage repair, and separation of sister chromatids, enabling rapid and accurate replication of genomic DNA (24). The cell cycle-related genes CDK1, CDK4, CCND1, and CCND2 induce mitosis (25). CCNDs bind to CDKs to form complexes that promote the cell cycle from G1 to S phase (26, 27). P21 and P53 are cell cycle inhibitors that lead to cell cycle arrest in response to various stimuli by inhibiting retinoblastoma protein phosphorylation and E2F transcription factor release (28–30). The results revealed that neonatal VA supplementation increased the proliferation of testicular cells and promoted the growth of the testes by synergistically regulating PCNA, CCND1, CCND2, and P21 in lamb testes, thus enlarging the testes after sheep growth. Higher expression of PCNA, CDK1, and CDK4 in the testes of adult sheep may be associated with stronger spermatogenic ability.
Hormones regulate spermatogenesis in the testes. Higher levels of FSH, LH, and T were detected in the serum of both lambs and adults following neonatal VA supplementation. FSH secreted by the pituitary gland is transported to the testes and directly promotes the proliferation of Sertoli cells. LH secreted by the pituitary gland stimulates Leydig cells to secrete T, which binds to the androgen receptor on Sertoli cells to further regulate Sertoli cell proliferation (2, 31). Sertoli cells provide nutritional support in seminiferous tubules and spontaneously synthesize endogenous RA, which regulates the expression of STRA8 and meiosis in spermatocytes through paracrine signals (8). FSH, LH, and T indirectly promote spermatocyte meiosis by acting on Sertoli or Leydig cells. The higher levels of gonadotropin and T suggested that neonatal VA supplementation led to a higher long-term meiotic capacity of ovine testis spermatocytes. Cholesterol, a substrate for T biosynthesis, is converted to pregnenolone by CYP11A1, which is subsequently converted to progesterone by HSD3B1. Progesterone is converted to androstenadione by CYP17A1, and T is synthesized from androstenedione by HSD17B3. STAR is an intracellular cholesterol carrier (32). The expression of HSD3B1 in lamb testes and HSD17B3 in adult sheep testes was upregulated by neonatal VA supplementation, which was consistent with the increased T levels. However, in vitro studies have reported that VA and RA regulate T synthesis by promoting the expression of STAR and CYP17A1 in Leydig cells. Specific blocking of RA signaling in mouse Leydig cells results in decreased expression of CYP17A1 and T levels, and VA deficiency in rats results in decreased expression of HSD3B1 and CYP11A1 (33, 34). Therefore, the regulation of steroidogenesis-related genes by RA may differ between sheep and rodent testis Leydig cells.
Vitamins may protect the sperm against oxidative stress and affect male fertility (35, 36). Serum VA levels are significantly decreased in men with infertility, and VA reduces sperm DNA breakage and lipid peroxidation through its antioxidant action (37). To further investigate whether the improvement in the reproductive ability of sheep by neonatal VA supplementation is related to the level of oxidative stress in the testes, we measured the antioxidant enzymes in the testes and found that neonatal VA supplementation increased the concentration of antioxidant enzymes and total antioxidant levels in both lamb and adult sheep testes. SOD catalyzes the conversion of superoxide free radicals to hydrogen peroxide, and loss of SOD activity is related to male sterility (38). GSH-Px and CAT break down hydrogen peroxide, protecting cells from oxidative damage caused by peroxides and maintaining cell membrane integrity (39). MDA is the final product of lipid peroxidation; therefore, changes in the MDA concentration can be used as a sign of oxidative stress and reflect the degree of cell damage (40). In addition, the increased expression of antioxidant-related genes confirmed the improved antioxidant capacity.
VA regulates downstream functional genes through the RA signaling pathway after diffusion into the cells. The RA signaling pathway is composed of the RA receptors (RAR, RXR), RA synthetases (ADH, ALDH), and RA lyase (CYP26). Periodic changes in RA levels in the seminiferous tubules of adult mammals are co-regulated by ADH and ALDH, which catalyze RA synthesis, and CYP26, which degrades RA. Periodic RA signals induce meiosis initiation to ensure that sperms are produced at a constant rate throughout reproductive life (19). ALDH1A knockout in mouse Sertoli cells prevents local RA production and blocks spermatogonal differentiation and meiosis initiation (41). CYP26B1 forms a catabolic barrier in Sertoli cells by degrading RA, preventing RA outside the spermatogenic tubules from reaching germ cells, and maintaining stable endogenous RA levels. CYP26B1 knockout in Sertoli cells increased endogenous RA in spermatogenic tubules and meiosis-related gene expression (42). The RA receptor RARA was upregulated in the testes of lambs after neonatal VA supplementation, suggesting that RA signaling in the testis was activated. The mRNA expression of the RAR-related orphan receptor A was also upregulated. ROR is involved in T biosynthesis and the regulation of semen quality and male fertility (43, 44). RAR activates its transcription (45). In addition, decreased RA synthetase and increased RA lyase levels indicated that the RA concentration was also elevated on the lateral side of the spermatogenic tubules. RA synthetase and lyase function together as the BTB. However, there was no difference in RA signaling between the control and VA groups after the sheep grew. Neonatal VA supplementation did not continuously activate the RA signaling pathway for a long time.
The AMPK and AKT signaling pathways have been proven to be two extremely important regulatory pathways in testicular development and spermatogenesis (46). AKT positively regulates the downstream proteins, mTOR, 4EBP1, and p70S6K, whereas AMPK inhibits their activities (47). AMPK participates in the regulation of tight junctions in testis cells (48). Tight junctions between the Sertoli cells constitute the BTB, providing a suitable microenvironment for spermatogenesis. Inhibition of AMPK signaling in Sertoli cells leads to the destruction of tight junctions between Sertoli and germ cells, the shedding of germ cells, and a decline in the fertility of mice (49). AKT signaling disrupts BTB structure by regulating protein synthesis and the cytoskeleton in Sertoli cells (50–52). Neonatal VA supplementation stimulates the activity of the AMPK signaling pathway and inhibits the activity of the AKT signaling pathway in the testis. It has been speculated that AMPK and AKT jointly enhance the junctions between Sertoli cells to form a stronger BTB and maintain periodic RA levels in the spermatogenic tubules. This result is consistent with the changes in the expression of RA synthetase and lyase, the key proteins that constitute the BTB. In addition to regulating intercellular junctions, AMPK inhibits the proliferation of Sertoli cells by mediating G1/S phase cell cycle arrest (53, 54). AKT also promotes the proliferation and differentiation of spermatogonia and Sertoli cells in the testes (50, 55). RA signaling initiated efficient mRNA translation associated with spermatogonal differentiation via the AKT signaling pathway (56). The phosphorylation of AMPK decreased, and that of AKT increased in the testes of adult sheep in the VA group, suggesting that these two signaling pathways synergistically regulate the maintenance of high spermatogenic ability in adult sheep. There was no significant difference in the expression of the downstream protein p-4EBP1 in the VA group in either the lamb or adult sheep testes; however, this slight trend was consistent with the regulatory effects of p-AMPK and p-AKT. Therefore, we speculate that changes in AMPK and AKT signaling pathway activity caused by neonatal VA supplementation may play different regulatory roles in the testes of lambs and adult sheep.
Conclusions
In conclusion, neonatal VA supplementation promotes testis development and improves spermatogenesis by regulating spermatogonial differentiation, spermatocyte meiosis, cell proliferation, hormone levels, and antioxidant levels in sheep testes. VA activates the RA signaling pathway and enhances BTB and spermatogenesis by regulating the AMPK and AKT signaling pathways in sheep testes. The rapid replenishment and renewal of spermatogenic cells in the testis of adult sheep promote the continuous production and release of sperm, and the increase in serum testosterone levels also stimulates male sexual behavior. The higher semen density and libido of rams are conducive to improving the mating rate in the actual breeding process. Therefore, VA supplementation may improve the reproductive potential of male lambs.
Data availability statement
The original contributions presented in the study are included in the article/Supplementary material, further inquiries can be directed to the corresponding author.
Ethics statement
The animal studies were approved by the Animal Protection and Utilization Committee of Shanxi Agricultural University. The studies were conducted in accordance with the local legislation and institutional requirements. Written informed consent was obtained from the owners for the participation of their animals in this study.
Author contributions
YL: Conceptualization, Data curation, Investigation, Methodology, Writing – original draft. PS: Conceptualization, Methodology, Writing – original draft. JiZ: Methodology, Writing – original draft. WZ: Methodology, Writing – original draft. XLi: Conceptualization, Methodology, Writing – original draft. XLv: Conceptualization, Funding acquisition, Writing – original draft. JuZ: Funding acquisition, Resources, Supervision, Writing – review & editing.
Funding
The author(s) declare financial support was received for the research, authorship, and/or publication of this article. This work was supported by the National Natural Science Foundation of China (31972559), Distinguished and Excellent Young Scholar Cultivation Project of Shanxi Agricultural University (2022JQPYGC01), and Open Project Program of International Joint Research Laboratory in Universities of Jiangsu Province of China for Domestic Animal Germplasm Resources and Genetic Improvement (IJRLD-KF202206).
Acknowledgments
The authors thank Baosen Farm in Taigu County for providing a breeding base for experimental animals.
Conflict of interest
The authors declare that the research was conducted in the absence of any commercial or financial relationships that could be construed as a potential conflict of interest.
The reviewer JW declared a shared affiliation with the authors YL, PS, JiZ, WZ, and JuZ to the handling editor at the time of review.
Publisher's note
All claims expressed in this article are solely those of the authors and do not necessarily represent those of their affiliated organizations, or those of the publisher, the editors and the reviewers. Any product that may be evaluated in this article, or claim that may be made by its manufacturer, is not guaranteed or endorsed by the publisher.
Supplementary material
The Supplementary Material for this article can be found online at: https://www.frontiersin.org/articles/10.3389/fvets.2024.1370576/full#supplementary-material
Supplementary Data Sheet 1. Original images of gels.
Supplementary Data Sheet 2. Microscopy images.
References
1. Polcz ME, Barbul A. The role of vitamin A in wound healing. Nutr Clin Pract. (2019) 34:695–700. doi: 10.1002/ncp.10376
2. Hogarth CA, Griswold MD. The key role of vitamin A in spermatogenesis. J Clin Invest. (2010) 120:956–62. doi: 10.1172/JCI41303
3. Chihara M, Otsuka S, Ichii O, Kon Y. Vitamin A deprivation affects the progression of the spermatogenic wave and initial formation of the blood-testis barrier, resulting in irreversible testicular degeneration in mice. J Reprod Dev. (2013) 59:525–35. doi: 10.1262/jrd.2013-058
4. Chambon P. A decade of molecular biology of retinoic acid receptors. FASEB J. (1996) 10:940–54. doi: 10.1096/fasebj.10.9.8801176
5. do Nascimento MAW, Cavalari FC, Oliveria VS, Gonçalves R, Menegaz D, Loss E, et al. Crosstalk in the non-classical signal transduction of testosterone and retinol in immature rat testes. Steroids. (2020) 153:108522. doi: 10.1016/j.steroids.2019.108522
6. Nourashrafeddin S, Rashidi BH. Gonadotropin regulation of retinoic acid activity in the testis. Acta Med Iran. (2018) 56:34–42.
7. Doyle TJ, Oudes AJ, Kim KH. Temporal profiling of rat transcriptomes in retinol-replenished vitamin A-deficient testis. Syst Biol Reprod Med. (2009) 55:145–63. doi: 10.3109/19396360902896844
8. Chen SR, Liu YX. Regulation of spermatogonial stem cell self-renewal and spermatocyte meiosis by Sertoli cell signaling. Reproduction. (2015) 149:159–67. doi: 10.1530/REP-14-0481
9. Cheng CY, Mruk DD. The blood-testis barrier and its implications for male contraception. Pharmacol Rev. (2012) 64:16–64. doi: 10.1124/pr.110.002790
10. Mruk DD, Cheng CY. The mammalian blood-testis barrier: its biology and regulation. Endocr Rev. (2015) 36:564–91. doi: 10.1210/er.2014-1101
11. Nishimura H, L'Hernault SW. Spermatogenesis. Curr Biol. (2017) 27:988–94. doi: 10.1016/j.cub.2017.07.067
12. Griswold M, Hogarth C. Synchronizing spermatogenesis in the mouse. Biol Reprod. (2022) 107:1159–65. doi: 10.1093/biolre/ioac148
13. Khanehzad M, Abbaszadeh R, Holakuyee M, Modarressi MH, Nourashrafeddin SM. FSH regulates RA signaling to commit spermatogonia into differentiation pathway and meiosis. Reprod Biol Endocrinol. (2021) 19:4. doi: 10.1186/s12958-020-00686-w
14. Zhou Y, Wang Y. Action and interaction between retinoic acid signaling and blood-testis barrier function in the spermatogenesis cycle. Cells. (2022) 11:352. doi: 10.3390/cells11030352
15. Wang B, Fu X, Zhang S, Liang X, Zhu M, Du M. Maternal vitamin A supplementation expands adipose progenitor population through promoting vascular development. FASEB J 30. (2016) 122–4. doi: 10.1096/fasebj.30.1_supplement.124.2
16. Wang B, Nie W, Fu X, Avila JM, Ma Y, Zhu MJ, et al. Neonatal vitamin A injection promotes cattle muscle growth and increases oxidative muscle fibers. J Anim Sci Biotechnol. (2018) 9:82. doi: 10.1186/s40104-018-0296-3
17. Song P, Chen X, Zhao J, Li Q, Li X, Wang Y, et al. Vitamin A injection at birth improves muscle growth in lambs. Anim Nutr. (2023) 14:204–12. doi: 10.1016/j.aninu.2023.05.011
18. Li Y, Jing J, Dang W, Jia K, Guo X, Kebreab E, et al. Cross-talk between NOTCH2 and BMP4/SMAD signaling pathways in bovine follicular granulosa cells. Theriogenology. (2022) 187:74–81. doi: 10.1016/j.theriogenology.2022.04.016
19. Endo T, Mikedis MM, Nicholls PK, Page DC, Rooij DG. Retinoic acid and germ cell development in the ovary and testis. Biomolecules. (2019) 9:775. doi: 10.3390/biom9120775
20. Bowles J, Feng CW, Ineson J, Miles K, Spiller CM, Harley VR, et al. Retinoic acid antagonizes testis development in mice. Cell Rep. (2018) 24:1330–41. doi: 10.1016/j.celrep.2018.06.111
21. Long C, Zhou Y, Shen L, Yu Y, Hu D, Liu X, et al. Retinoic acid can improve autophagy through depression of the PI3K-Akt-mTOR signaling pathway via RARα to restore spermatogenesis in cryptorchid infertile rats. Genes Dis. (2021) 9:1368–77. doi: 10.1016/j.gendis.2021.03.006
22. Tedesco M, Desimio MG, Klinger FG, Felici MD, Farini D. Minimal concentrations of retinoic acid induce stimulation by retinoic acid 8 and promote entry into meiosis in isolated pregonadal and gonadal mouse primordial germ cells. Biol Reprod. (2013) 88:145. doi: 10.1095/biolreprod.112.106526
23. Sahin P, Sahin Z, Gungor-Ordueri NE, Donmez BO, Celik-Ozenci C. Inhibition of mammalian target of rapamycin signaling pathway decreases retinoic acid stimulated gene 8 expression in adult mouse testis. Fertil Steril. (2014) 102:1482–90. doi: 10.1016/j.fertnstert.2014.08.004
24. Arbel M, Choudhary K, Tfilin O, Kupiec M. PCNA loaders and unloaders-one ring that rules them all. Genes. (2021) 12:1812. doi: 10.3390/genes12111812
25. Mohamed TMA, Ang YS, Radzinsky E, Zhou P, Huang Y, Elfenbein A, et al. Regulation of cell cycle to stimulate adult cardiomyocyte proliferation and cardiac regeneration. Cell. (2018) 173:104–16. doi: 10.1016/j.cell.2018.02.014
26. Büschges R, Weber RG, Actor B, Lichter P, Collins VP, Reifenberger G. Amplification and expression of cyclin D genes (CCND1, CCND2 and CCND3) in human malignant gliomas. Brain Pathol. (1999) 9:435–42. doi: 10.1111/j.1750-3639.1999.tb00532.x
27. Gao X, Leone GW, Wang H. Cyclin D-CDK4/6 functions in cancer. Adv Cancer Res. (2020) 148:147–69. doi: 10.1016/bs.acr.2020.02.002
28. Engeland K. Cell cycle regulation: p53-p21-RB signaling. Cell Death Differ. (2022) 29:946–60. doi: 10.1038/s41418-022-00988-z
29. Karimian A, Ahmadi Y, Yousefi B. Multiple functions of p21 in cell cycle, apoptosis and transcriptional regulation after DNA damage. DNA Repair. (2016) 42:63–71. doi: 10.1016/j.dnarep.2016.04.008
30. Kuganesan N, Dlamini S, Tillekeratne LMV, Taylor WR. Tumor suppressor p53 promotes ferroptosis in oxidative stress conditions independent of modulation of ferroptosis by p21, CDKs, RB, and E2F. J Biol Chem. (2021) 297:101365. doi: 10.1016/j.jbc.2021.101365
31. Deng CY, Lv M, Luo BH, Zhao SZ, Mo ZC, Xie YJ. The role of the PI3K/AKT/mTOR signalling pathway in male reproduction. Curr Mol Med. (2021) 21:539–48. doi: 10.2174/1566524020666201203164910
32. Dong Y, Wang Y, Zhu Q, Li X, Huang T, Li H, et al. Dimethoate blocks pubertal differentiation of Leydig cells in rats. Chemosphere. (2020) 241:125036. doi: 10.1016/j.chemosphere.2019.125036
33. Jauregui EJ, Mitchell D, Topping T, Hogarth CA, Griswold MD. Retinoic acid receptor signaling is necessary in steroidogenic cells for normal spermatogenesis and epididymal function. Development. (2018). 145:dev160465. doi: 10.1242/dev.160465
34. Udhane SS, Pandey AV, Hofer G, Mullis PE, Flück CE. Retinoic acid receptor beta and angiopoietin-like protein 1 are involved in the regulation of human androgen biosynthesis. Sci Rep. (2015) 5:10132. doi: 10.1038/srep10132
35. Calderón B, Hevia V, Vega-Piñero B, Martín-Hidalgo A, Sol HMD, Escobar-Morreale HF, et al. Serum retinol, folic acid, and copper are associated with sperm abnormalities in men with obesity. J Am Coll Nutr. (2018) 37:194–200. doi: 10.1080/07315724.2017.1387877
36. Ebisch IMW, Thomas CMG, Peters WHM, Braat DDM, Steegers-Theunissen RPM. The importance of folate, zinc and antioxidants in the pathogenesis and prevention of subfertility. Hum Reprod Update. (2007) 13:163–74. doi: 10.1093/humupd/dml054
37. Ghyasvand T, Goodarzi MT, Amiri I, Karimi J, Ghorbani M. Serum levels of lycopene, beta-carotene, and retinol and their correlation with sperm DNA damage in normospermic and infertile men. Int J Reprod Biomed. (2015) 13:787–92. doi: 10.29252/ijrm.13.12.787
38. Sakamoto T, Imai H. Hydrogen peroxide produced by superoxide dismutase SOD-2 activates sperm in Caenorhabditis elegans. J Biol Chem. (2017) 292:14804–13. doi: 10.1074/jbc.M117.788901
39. Yang XH, Li L, Xue YB, Zhou XX, Tang JH. Flavonoids from Epimedium pubescens: extraction and mechanism, antioxidant capacity and effects on CAT and GSH-Px of Drosophila melanogaster. PeerJ. (2020) 8:e8361. doi: 10.7717/peerj.8361
40. Colakoglu HE, Yazlik MO, Kaya U, Colakoglu EC, Kurt S, Oz B, et al. MDA and GSH-Px activity in transition dairy cows under seasonal variations and their relationship with reproductive performance. J Vet Res. (2017) 61:497–502. doi: 10.1515/jvetres-2017-0067
41. Gewiss R, Topping T, Griswold MD. Cycles, waves, and pulses: Retinoic acid and the organization of spermatogenesis. Andrology. (2020) 8:892–7. doi: 10.1111/andr.12722
42. Bowles J, Feng CW, Miles K, Ineson J, Spiller C, Koopman P. ALDH1A1 provides a source of meiosis-inducing retinoic acid in mouse fetal ovaries. Nat Commun. (2016) 7:10845. doi: 10.1038/ncomms10845
43. Mandal K, Sarkar RK, Sharma SS, Jain A, Majumdar SS. Sertoli cell specific knockdown of RAR-related orphan receptor (ROR) alpha at puberty reduces sperm count in rats. Gene. (2018) 641:18–24. doi: 10.1016/j.gene.2017.10.032
44. Qin F, Liu N, Nie J, Shen T, Xu Y, Pan S, et al. Circadian effects of ionizing radiation on reproductive function and clock genes expression in male mouse. Environ Health Prev Med. (2021) 26:103. doi: 10.1186/s12199-021-01021-4
45. Matsui T. Transcriptional regulation of a Purkinje cell-specific gene through a functional interaction between ROR alpha and RAR. Genes Cells. (1997) 2:263–72. doi: 10.1111/j.1365-2443.1997.119gc0317.x
46. Ni FD, Hao SL, Yang WX. Multiple signaling pathways in Sertoli cells: recent findings in spermatogenesis. Cell Death Dis. (2019) 10:541. doi: 10.1038/s41419-019-1782-z
47. Yang T, Yang WX. The dynamics and regulation of microfilament during spermatogenesis. Gene. (2020) 744:144635. doi: 10.1016/j.gene.2020.144635
48. Yang W, Wang L, Wang F, Yuan S. Roles of AMP-activated protein kinase (AMPK) in mammalian reproduction. Front Cell Dev Biol. (2020) 8:593005. doi: 10.3389/fcell.2020.593005
49. Bertoldo MJ, Guibert E, Faure M, Guillou F, Ramé C, Nadal-Desbarats L, et al. Specific deletion of AMP-activated protein kinase (α1AMPK) in mouse Sertoli cells modifies germ cell quality. Mol Cell Endocrinol. (2016) 423:96–112. doi: 10.1016/j.mce.2016.01.001
50. Chen KQ, Wei BH, Hao SL, Yang WX. The PI3K/AKT signaling pathway: How does it regulate development of Sertoli cells and spermatogenic cells? Histol. Histopathol. (2022) 37:621–36. doi: 10.14670/HH-18-457
51. Zhou Y, Chen Y, Hu X, Guo J, Shi H, Yu G, et al. Icariin attenuate microcystin-LR-induced gap junction injury in Sertoli cells through suppression of Akt pathways. Environ Pollut. (2019) 251:328–37. doi: 10.1016/j.envpol.2019.04.114
52. Zhou Y, Sun M, Tang Y, Chen Y, Zhu C, Yang Y, et al. Responses of the proteome in testis of mice exposed chronically to environmentally relevant concentrations of Microcystin-LR. Ecotoxicol Environ Saf. (2020) 187:109824. doi: 10.1016/j.ecoenv.2019.109824
53. Meroni SB, Galardo MN, Rindone G, Gorga A, Riera MF, Cigorraga SB. Molecular mechanisms and signaling pathways involved in sertoli cell proliferation. Front Endocrinol. (2019) 10:224. doi: 10.3389/fendo.2019.00224
54. Riera MF, Regueira M, Galardo MN, Pellizzari EH, Meroni SB, Cigorraga SB. Signal transduction pathways in FSH regulation of rat Sertoli cell proliferation. Am J Physiol Endocrinol Metab. (2012) 302:914–23. doi: 10.1152/ajpendo.00477.2011
55. Wang S, Kang J, Song Y, Zhang A, Pan Y, Zhang Z, et al. Long noncoding RNAs regulated spermatogenesis in varicocele-induced spermatogenic dysfunction. Cell Prolif. (2022) 55:e13220. doi: 10.1111/cpr.13220
Keywords: sheep, vitamin A, testis, sperm, hormone, antioxidant
Citation: Li Y, Song P, Zhao J, Zhang W, Liu X, Lv X and Zhao J (2024) Neonatal vitamin A supplementation improves sheep fertility potential. Front. Vet. Sci. 11:1370576. doi: 10.3389/fvets.2024.1370576
Received: 15 January 2024; Accepted: 15 April 2024;
Published: 02 May 2024.
Edited by:
Gemma Gaitskell-Phillips, University of Extremadura, SpainReviewed by:
Jinming Wang, Shanxi Agricultural University, ChinaYunsheng Li, Anhui Agricultural University, China
Elisabeth Pinart, University of Girona, Spain
Copyright © 2024 Li, Song, Zhao, Zhang, Liu, Lv and Zhao. This is an open-access article distributed under the terms of the Creative Commons Attribution License (CC BY). The use, distribution or reproduction in other forums is permitted, provided the original author(s) and the copyright owner(s) are credited and that the original publication in this journal is cited, in accordance with accepted academic practice. No use, distribution or reproduction is permitted which does not comply with these terms.
*Correspondence: Junxing Zhao, SnVueHpoJiN4MDAwNDA7c3hhdS5lZHUuY24=