- 1College of Animal Science, South China Agricultural University, Guangzhou, China
- 2Guangdong Enterprise Key Laboratory for Animal Health and Environmental Control, Wen's Foodstuff Group Co. Ltd., Yunfu, China
Infection by the novel duck reovirus (NDRV) in ducklings causes high mortality, which leads to substantial economic losses in the duck industry in China. To date, no commercial vaccine is available for this disease. In this study, linear B cell epitopes of the σB protein of the NDRV were predicted and recombinant multiple linear B cell epitopes (MLBEs) were constructed through linkers. The recombinant MLBEs were then expressed and purified. One-day-old Muscovy ducklings were immunized with different doses of MLBEs and challenged with 5 × 104 ELD50 of the virulent CHY strain of NDRV 14 days after immunization. The ducklings vaccinated with 20 and 40 μg of MLBE performed no clinical signs or gross or histopathological lesions, indicating 100% protection against infection. The viral load in the liver and spleens of these birds was significantly lower than that in the control group. Additionally, these ducklings exhibited positive seroconversion at 7 days after vaccination on enzyme-linked immunosorbent assay. These results indicate that MLBE of σB could be used as a candidate for developing vaccines against NDRV infection.
1 Introduction
Avian orthoreoviruses (ARVs), belonging to the genus Orthoreovirus of the family Spinareoviridae, are critical etiological agents that can cause devastating diseases in various domestic and wild birds (1). ARVs can cause viral arthritis/tenosynovitis and are associated with several diseases in chickens, such as respiratory disease, enteric disease, and inclusion body hepatitis (2, 3). Muscovy duck reovirus (MDRV) infection mainly causes liver foci necrosis, resulting in high mortality in 1-day-old Muscovy ducklings (4, 5). Similar symptoms and 10–20% mortality have been recently reported in geese after ARV infection (6). Since 2011, a novel duck reovirus (NDRV) infection among different duck species, such as Peking, mallard, and Muscovy ducks, has caused severe hemorrhagic–necrotic lesions in the liver and spleen, resulting in 5–50% mortality in ducklings, which is different from MDRV infection (7–11). ARV infection has caused significant economic losses for the poultry industry in China.
The ARV genome consists of 10 double-stranded RNA segments, divided into three size classes: large (L1–L3), medium (M1–M3), and small (S1–S4) (1, 12), which encode at least 12 proteins. Sigma C and σB are the major surface proteins encoded by the S1 and S3 gene segments, respectively (13). Both sigma C and σB can induce specific neutralizing antibodies; hence, they are targets for vaccine development or disease diagnosis (13–16).
Vaccination is a major strategy for the prevention and control of infectious diseases. One commercial MDRV-CA strain live attenuated vaccine, is available to prevent the disease in China. However, it does not provide cross-protection against NDRV infection (17, 18). Therefore, developing an efficient vaccine to control NDRV infection is urgently needed. A naturally attenuated NDRV N20 strain provided sufficient protection (19), while a subunit vaccine consisting of sigma C protein produced in Sf9 cells induced 100% protection against the challenge with lethal NDRV (20).
In this study, the predicted linear B cell epitopes fused with sumo-tags were expressed in Escherichia coli. The humoral immune responses and protection provided by recombinant multiple linear B cell epitopes (rMLBEs) against NDRV challenge were evaluated in Muscovy ducklings, which can be used a reference for NDRV vaccine development.
2 Materials and methods
2.1 Ethics statement
The protocol was approved by the Committee of Animal Experiments of South China Agricultural University, Guangzhou, China (Approval ID: SYXK-2019-0136). All biological safety and sanitation measures were observed.
2.2 B cell epitope prediction and protein expression
The linear B cell epitopes of σB protein (TH11 strain, NCIB accession No. AFX68863.1) were predicted using Bepipred Linear Epitope Prediction 2.0 (http://tools.iedb.org/bcell/) (21). The multiple-epitope subunit vaccine was constructed using the B-cell epitopes through a GGGGG linker, and codon optimization was achieved using the Java Codon adaptation tool (http://www.jcat.de/) to improve its expression level in the BL21 (DE3) strain of E. coli (21). The optimized sequence was synthesized by Sangon Biotech (Shanghai, China) and cloned into the pSmart-I vector (Convenience Biology, Changzhou, China) downstream of the Sumo gene using the BamH I and Xho I restriction sites. The recombinant plasmid was used to transform the E. coli BL21 (DE3) cells; single colonies were selected and grown in LB medium with 50 μg/mL kanamycin at 37°C until an OD600 of 0.5–0.6 was reached. Protein expression was induced by adding isopropyl β-D-thiogalactopyranoside (IPTG) to a final concentration of 0.1 mM and incubated at 16°C overnight. Cells were collected, resuspended in buffer A [20 mM Tris, 250 mM NaCl, 5% (V/V) glycerine; pH ± 8.0], and ultrasonicated. The cell lysate was centrifuged at 13,000 g and 4°C for 30 min. The recombinant proteins in the supernatant were purified by nickel-nitrilotriacetate (Ni-NTA) affinity chromatography, as previously described (22). Briefly, the supernatants were loaded on the Ni-NTA agarose by gravity flow and washed with buffer B (20 mM Tris, 250 mM NaCl, 50 mM imidazole; pH 8.0). The protein of interest was eluted with buffer C (20 mM Tris, 250 mM NaCl, 250 mM imidazole; pH ± 8.0). The eluate was dialyzed in PBS buffer (pH 7.4) and analyzed via sodium dodecyl sulfate–polyacrylamide gel electrophoresis (SDS–PAGE) and western blotting.
2.3 Animal immunization and challenge
The purified rMLBE protein or PBS was mixed with oil adjuvant to obtain the rMLBE or control vaccines, respectively. For the animal studies, 60 one-day-old NDRV-free Muscovy ducks were supplied by the Wens Food Group Co. Ltd. and randomly divided into four groups of 15 each. Of them, three groups were immunized with 10, 20, and 40 μg of rMLBE vaccine on day 0; while one group was immunized with PBS. Sera from the immunized groups were collected at 0, 7, and 14 days post-vaccination (DPV) for antibody detection. The birds were challenged with 5 × 104 ELD50 of the NDRV CHY strain at 14-DPV. Three ducks from each group were sacrificed at 3, 5, and 7 days post-challenge (DPC), and their spleens and livers were collected for viral load determination.
2.4 Indirect enzyme-linked immunosorbent assay
The specific IgY antibody titer was determined by indirect enzyme-linked immunosorbent assay (ELISA) using sample sera diluted 100-fold in PBST with 2 mg of E. coli lysates and 10 μg of sumo-tag protein. Briefly, 96-well microtiter plates (Costar, USA) were coated with 1 μg/well of purified ΣB protein in 0.1 M carbonate/bicarbonate buffer (pH ± 9.6) and incubated at 4°C overnight. After washing thrice in PBST, the plates were blocked with 5% BSA in PBST for 1 h at 37°C. After washing thrice in PBST, 100 μL of the diluted serum was added to the microtiter wells and incubated for 1 h at 37°C. After washing thrice in PBST, 100 μL of horseradish (HRP)-conjugated anti-duck IgY antibody diluted to 1:3000 in PBST (KPL, USA) was added and incubated for 1 h at 37°C. After washing thrice in PBST, 100 μL of 3,3′,5,5′-Tetramethylbenzidine (TMB) was added to each well and incubated for 10 min at room temperature. The reaction was stopped by adding 50 μL of 2 M H2SO4, and the OD450 was measured. The S/P was calculated using the formula: S/P = (OD test serum-OD negative control serum)/(OD positive control serum-OD negative control serum). The test serum was considered positive when the S/P was > 0.2.
2.5 Fluorescent quantitative RT-PCR
Fluorescent quantitative (qRT-PCR) was used to determine the viral load of NDRV in the tissues as described previously (23). The tissues were homogenized, and viral RNA was extracted using MagPure Viral Nucleic Acid Micro LQ Kit (Magen, Guangzhou, China). qRT-PCR was performed as described previously (24) using a One Step RT-qPCR Probe Kit (Yeasen, Shanghai, China) with the forward primer: CCCGGATTCTCGATGAATGGT, reverse primer: CGACCCACTGCTGGATACAAG, and the probe: FAM-AACGCCTGTGCACGAGCTGAAC-3′-TAMRA under the following conditions: 50°C for 15 min, 95°C for 2 min, 40 cycles of 94°C for 15 s, and 60°C for 30 s. The viral load was determined using a standard curve. Each sample was run in triplicates.
2.6 Histopathology
Livers and kidneys of the ducks of different groups were collected 3, 5, and 7 DPC and fixed in 10% formalin for 48 h at 25°C. These tissues were embedded in paraffin wax, sliced into 4-μm-thick sections, stained with hematoxylin and eosin (HE), and then examined using light microscopy.
2.7 Statistical analysis
Statistical analyses of IgY antibodies were conducted using two-way ANOVA by GraphPad Prism 6 (www.graphpad.com). Statistical analyses of the results of other experiments were performed using an unpaired t-test. Differences were considered significant at *p < 0.05, **p < 0.01, or ***p < 0.001.
3 Results
3.1 Expression and purification of the recombinant σB protein
σB is an outer layer protein of NDRV. Therefore, it was proposed that sigma could induce immunological protection. The linear B cell epitopes of σB protein were predicted using Bepipred Linear Epitope Prediction 2.0. When the value was >0.5, the peptides with more than 15 residues were chosen for constructing the multiple linear B cell epitopes. As shown in Figure 1 and Table 1, five epitopes were selected and linked with the flexible peptide “GGGGG.” The expression and purification of MLBE were ascertained by SDS–PAGE and western blotting. As shown in Figures 2A, B, MLBE was successfully expressed and purified.
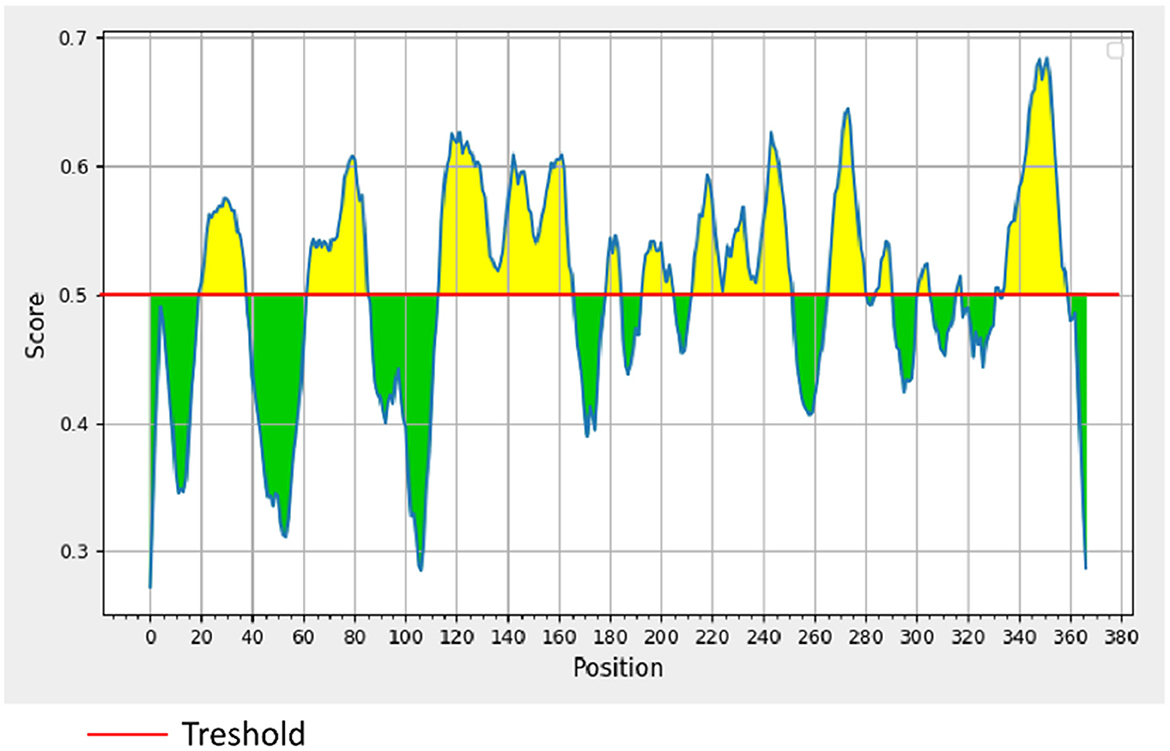
Figure 1. BepiPred linear prediction. Areas above the red line (threshold) are epitopes suggested to be binding to the B cells while the green areas are not.
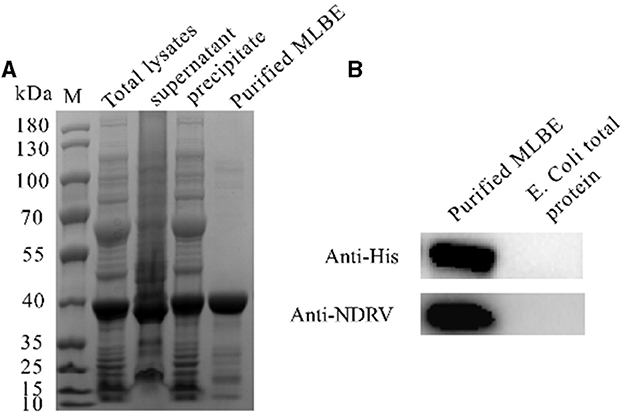
Figure 2. Expression and purification of MLEB of NDRV σB protein (A) and western-blotting analysis of the purified MLEB protein (B).
3.2 Humoral immune responses in ducklings immunized with MLBE
The birds were immunized with different dosages of MLBE, and sera were collected on the indicated dates. NDRV-specific antibodies produced in the birds were detected by indirect ELISA. As shown in Figure 3A, the serum conversion rate of ELISA antibodies was 100% in the 20 and 40 μg immunized birds on 7 DPV and 14 DPV, whereas only one bird was positive among those immunized with 10 μg on 7 DPV. The serum conversion rate was 100% in these birds on 14 DPV. Moreover, the OD450 values of 20- and 40-μg immunized birds were significantly higher than the 10 μg-immunized birds on 7 and 14 DPV. However, there was no statistical difference between the 20- and 40 μg-immunized birds. These data indicate that σB MLBE can induce positive immune responses, significantly increasing antibody levels.
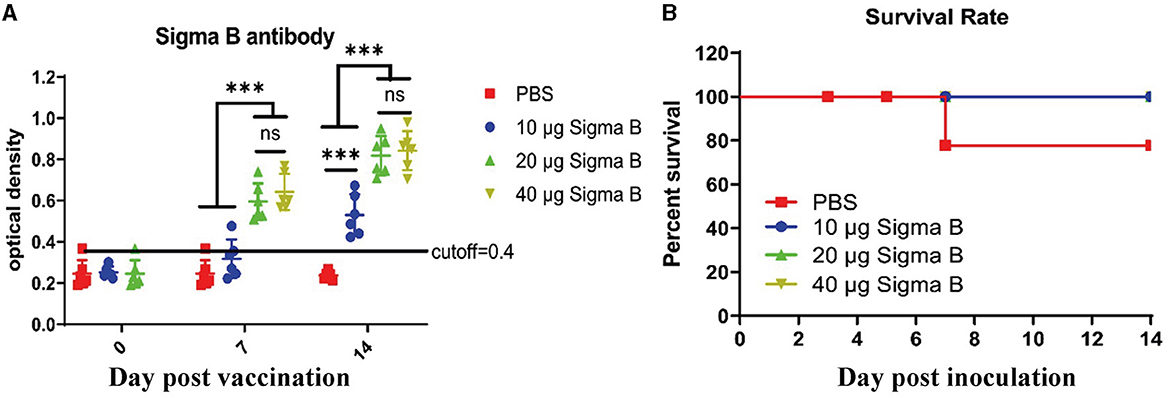
Figure 3. Antibodies analysis (A) and survival rate (B). IgY antibodies post MLEB of σB protein were detected by indirect ELISA (A). ns means no significant, *** means p < 0.001.
3.3 Protection of ducklings from the NDRV challenge
To assess the protective ability of MLBE, the birds were challenged at 14 DPV, and the clinical symptoms, gross lesions, and histologic microlesions were monitored. At 7 DPC, two birds died in the PBS group (Figure 3B), whereas no birds died during the challenge period in the immunized groups. Three birds were sacrificed for the examination of lesions. The liver and spleen are the primary target organs of NDRV. As shown in Figure 3, slight lesion spots in the liver were observed in the PBS group at 5 DPC (Figure 4E), whereas massive lesion spots were observed at 7 DPC (Figure 4J). Only slight blood spots were observed in the livers of the 10-μg MLBE group at 5 DPC (Figure 4F). No apparent lesions were observed in the livers of the 20- and 40-μg MLBE groups from 3 to 7 DPC (Figure 4). Lesion spots were also observed at 5 and 7 DPC in the PBS group (Figures 5E, J). Otherwise, the spleen was swollen in the 10-μg MLBE group at 5 and 7 DPC (Figures 5F, K). As expected, no noticeable lesions were observed in the spleen of 20- and 40-μg MLBE groups from 3 to 7 DPC (Figure 5).
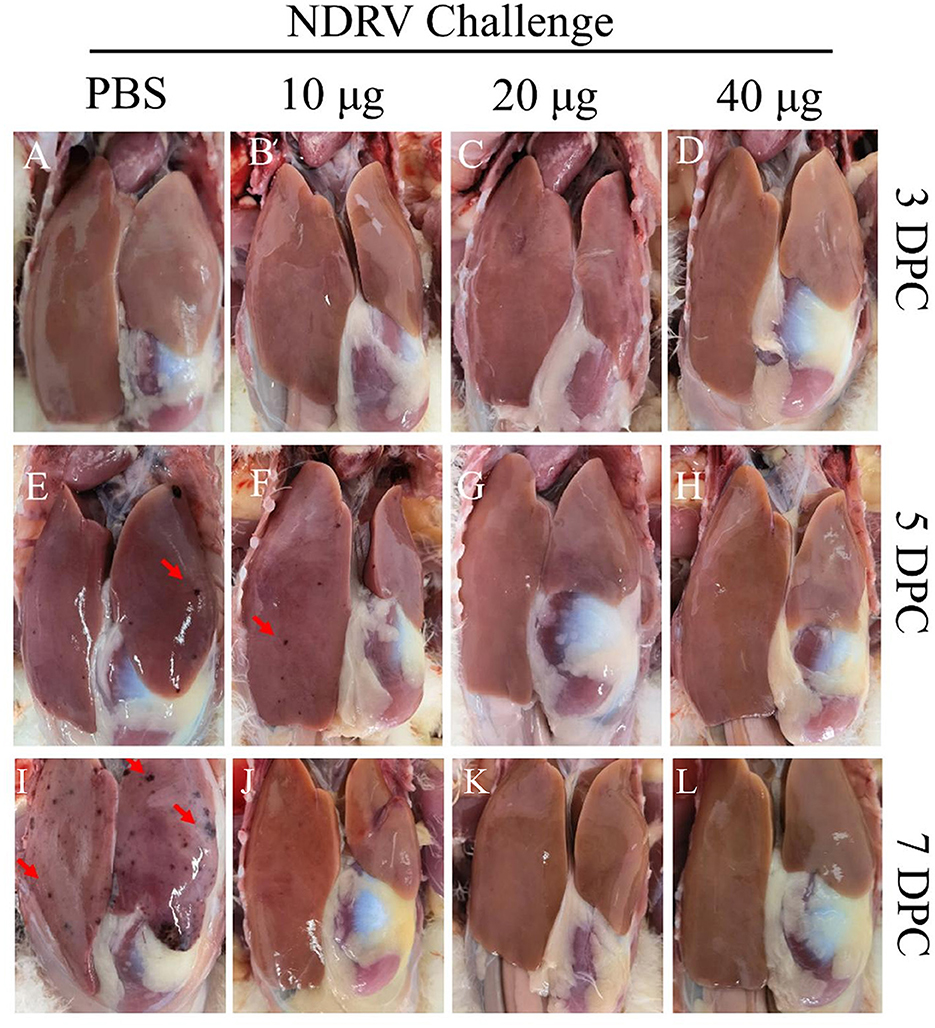
Figure 4. (A–D) Clinical changes of the livers on 3 DPC. (E–H) Clinical changes of the livers on 5 DPC. (I–L) Clinical changes of the livers on 7 DPC. Red arrowheads means Lesion spots.
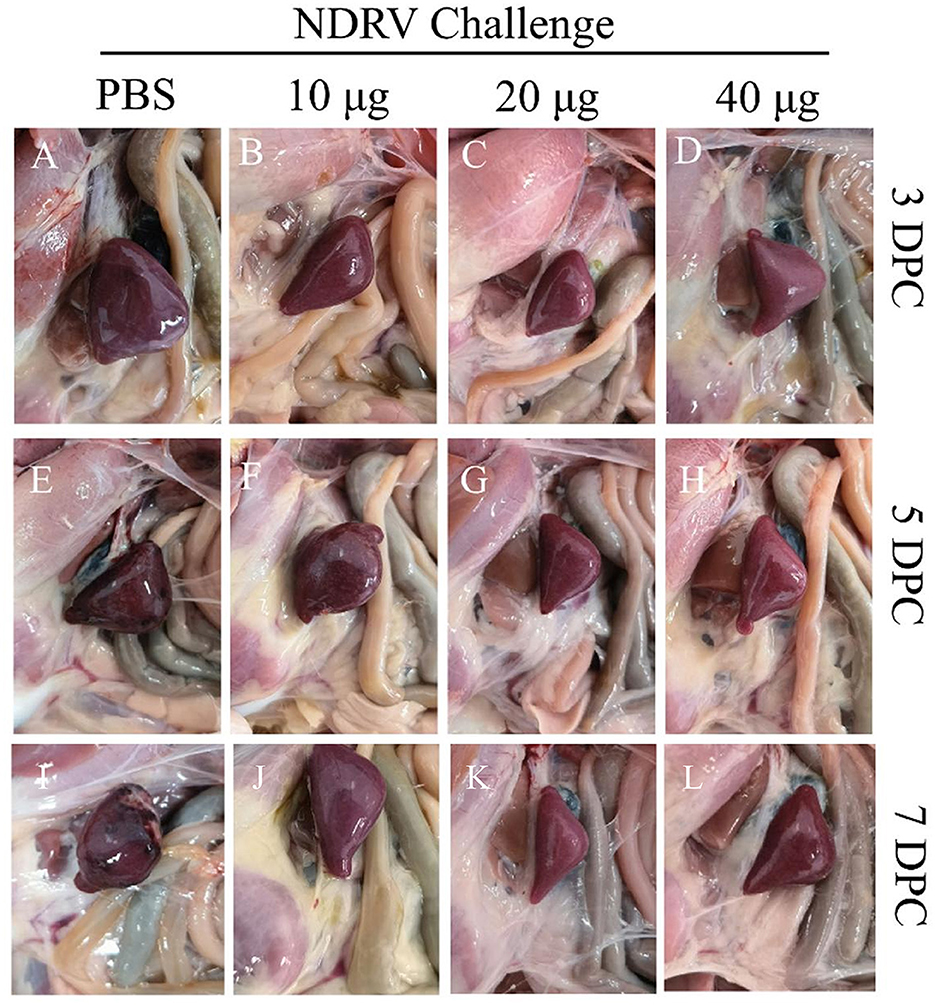
Figure 5. (A–D) Clinical changes of the spleens on 3 DPC. (E–H) Clinical changes of the spleens on 5 DPC. (I–L) Clinical changes of the spleens on 7 DPC.
The corresponding tissues were fixed, sectioned, and stained with HE (Figures 6, 7). Massive pathological damage was observed in the livers of the birds in the PBS group (Figure 6). Necrosis of local hepatocytes and cytolysis were observed at 5 DPC, around which massive inflammatory cell infiltration and hyperpigmentation were evident (Figure 6E). Focal necrosis of hepatocytes and nucleus fragmentation and nucleolysis were also observed at 7 DPC (Figure 6J). No noticeable pathological change was observed in the immunized groups (Figure 6). Massive pathological damage was also observed in the spleens of the PBS group ducks (Figure 7). An enlarged spleen and an unclear boundary were observed at 3 and 5 DPC (Figures 7A, E), whereas massive necrosis with hemorrhage was observed at 7 DPC (Figure 7J). Spleen and lymphatic nodule enlargement were also observed at 5 and 7 DPC in the 10-μg MLBE group (Figures 7F, K), but no evident pathological change was observed in the 20 and 40-μg MLBE groups. These results demonstrated that the MLBE of σB protein protected birds against virulent NDRV-induced pathological changes.
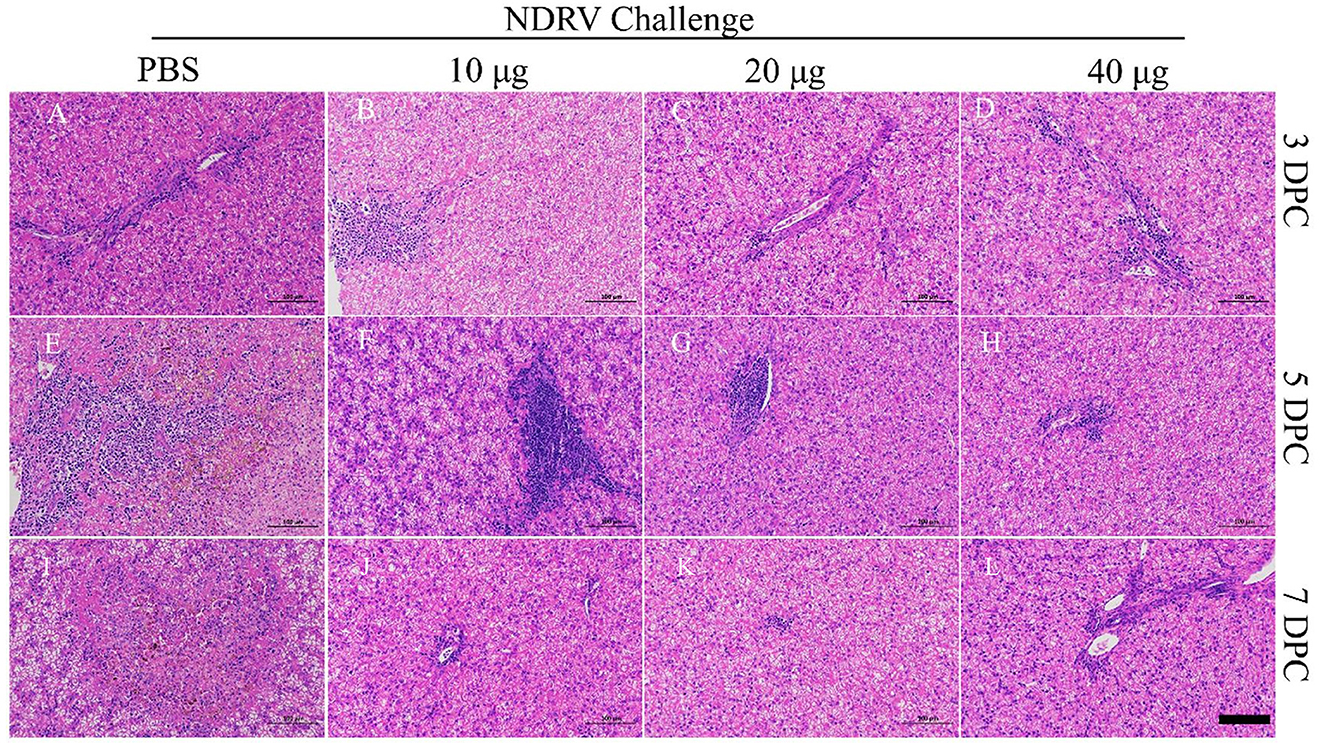
Figure 6. (A–D) Histological changes of the livers on 3 DPC. (E–H) Histological changes of the livers on 5 DPC. (I–L) Histological changes of the livers on 7 DPC.
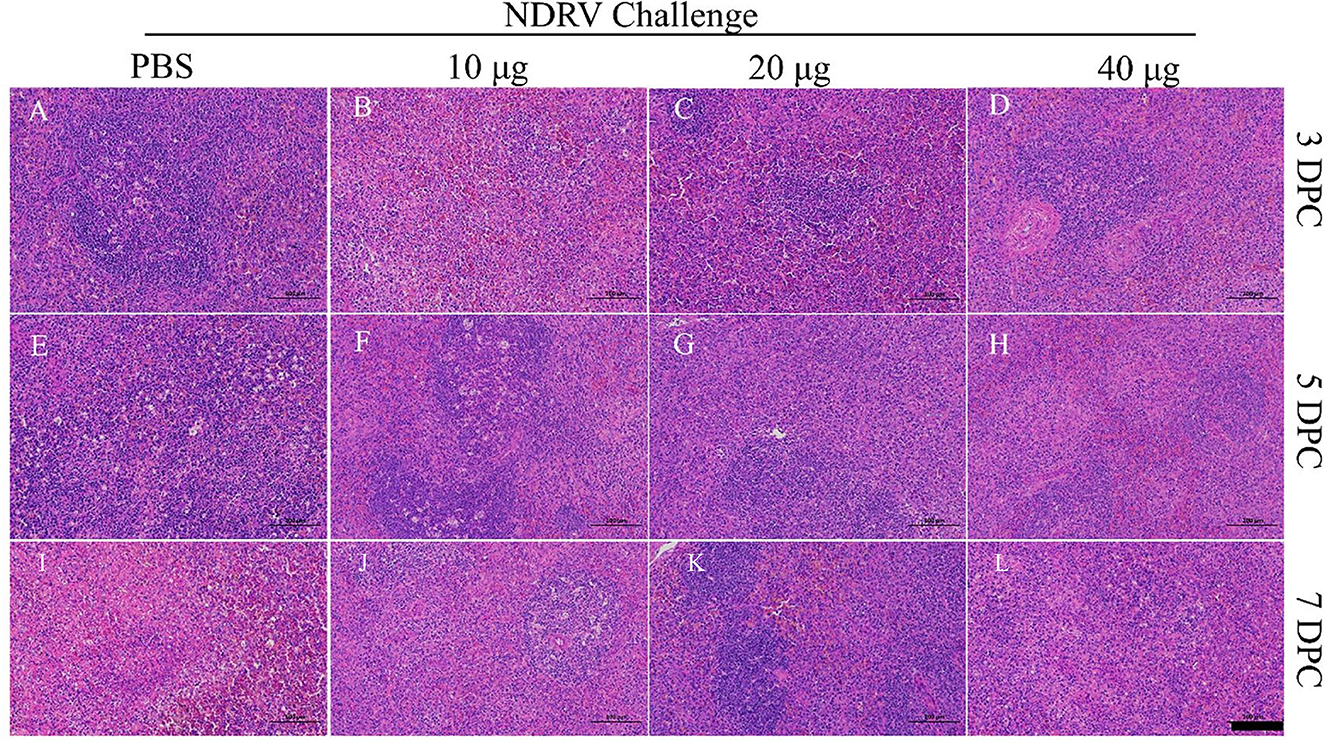
Figure 7. (A–D) Histological changes of the spleens on 3 DPC. (E–H) Histological changes of the spleens on 5 DPC. (I–L) Histological changes of the spleens on 7 DPC.
3.4 Detection of viral shedding and load in the spleens and livers
From each group, six cloacal swabs were collected at 3, 5, and 7 DPCs for detecting viral shedding using qRT-PCR. As shown in Table 2, viral shedding in a high proportion of birds in the PBS and 10-μg MLBE groups was observed at 5 and 7 DPC. A low proportion of birds in the 20- and 40-μg MLBE groups demonstrated viral shedding at 5 DPC, while only 3/6 birds in the 20 μg and 2/6 birds in the 40-μg MLBE group shed virus particles (Table 2). These results indicated that MLBE can impede viral shedding.
The liver and spleen tissue from each group at 3, 5, and 7 DPC were collected for viral load detection. As shown in Figure 8A, the viral load in the livers from the 20-μg MLBE group was markedly lower than that from the PBS group (p < 0.05 or p < 0.01) at 3, 5, and 7 DPC, and the viral load in the livers from the 40-μg MLBE group was also remarkably lesser than that from the PBS group (p < 0.01). The virus load was not significantly different between the PBS and 10-μg MLBE groups. Similarly, the viral load in the spleens from the 20-μg MLBE group was substantially lower than that from the PBS group (p < 0.05 or p < 0.01) at 3, 5, and 7 DPC, and the viral load in the spleens from the 40-μg MLBE group was also significantly lower than that from the PBS group (p < 0.01). The viral load in the spleens from the 10-μg MLBE groups was also considerably lower than that from the PBS group at 7 DPC (p < 0.01), and there was no marked variation at 3 and 5 DPC (Figure 8B). These results indicate that MLBE vaccination can reduce the replication of NDRV in the livers and spleens.
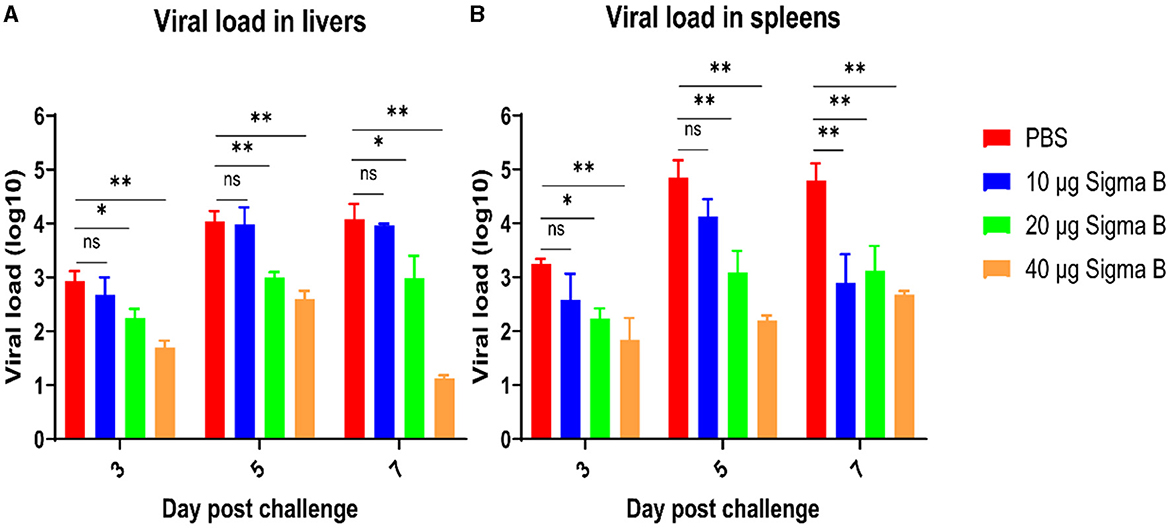
Figure 8. The viral RNA amount in the livers (A) and spleens (B) after NDRV challenge. Data are represented as means ± SD. ns means no significant, *means p ≤ 0.05, **means p ≤ 0.01.
4 Discussion
NDRV infection in ducklings causes high mortality, resulting in substantial economic losses in the poultry industry in China. Since its first identification, NDRV has widely infected duck flocks in China (25). NDRV is highly different from MDRV, which results in poor cross-protection of the MDRV vaccine against NDRV infection. A commercial live attenuated vaccine of the MDRV CA strain has been used to prevent MDRV. The naturally attenuated NDRV N20 strain also effectively protected against virulent NDRV challenge (19). However, the safety of sN20 requires further evaluation. A subunit vaccine based on the Sigma C protein produced in Sf9 cells was developed, which provided sufficient protection against infection by the Th11 strain (20). However, no commercial vaccine is currently available for preventing NDRV infection. Thus, there is an urgent need to develop a safe and effective vaccine against NDRV for the poultry industry. In the current study, the predicted linear B Cell epitopes of the σB protein of NDRV were expressed in E. coli and used as subunit vaccines in ducks. The protective efficacy of the rMLBE was evaluated, with the results suggesting that rMLBE is an attractive candidate for subunit vaccines against virulent NRDV. These results were similar to the research findings on multi-epitope subunit vaccine of influenza viruses (26, 27).
Humoral immunity plays a critical role in disease prevention (28). In this study, the Igγ titers induced by 20 and 40 μg rMLBE were conspicuously higher than those in the PBS group at 7 and 14 days post-inoculation (DPI), while the titers induced by 10 μg rMLBE were significantly higher than those in the PBS group at 14 DPI. These results indicated that the immunization dose was critical for generating Igγ in ducklings. Furthermore, the protective efficacy was evaluated based on the mortality, virus load, and histopathological examinations after challenge with NDRV. Like Igγ titers, immunization with 20 or 40 μg rMLBE provided sufficient protection against NDRV challenge.
In summary, the rMLEB of σB protein prepared using an E. coli expression system had good antigenicity and immunogenicity in ducklings, indicating that it can be a vaccine candidate against NDRV infection.
Data availability statement
The original contributions presented in the study are included in the article/supplementary material, further inquiries can be directed to the corresponding authors.
Ethics statement
The animal study was approved by the Committee of Animal Experiments of South China Agricultural University, Guangzhou, China (Approval ID: SYXK-2019-0136). The study was conducted in accordance with the local legislation and institutional requirements.
Author contributions
CL: Conceptualization, Writing – original draft. YC: Methodology, Writing – review & editing. ZY: Writing – review & editing, Supervision. YS: Writing – review & editing, Project administration. QZ: Writing – review & editing, Data curation. HS: Writing – review & editing, Formal analysis. FC: Writing – review & editing, Supervision.
Funding
The author(s) declare financial support was received for the research, authorship, and/or publication of this article. This work was supported by the China Postdoctoral Science Foundation (grant number: 2021M692455).
Conflict of interest
ZY, CL, YS, QZ, and HS were employed by Wen's Foodstuff Group Co. Ltd.
The remaining authors declare that the research was conducted in the absence of any commercial or financial relationships that could be construed as a potential conflict of interest.
Publisher's note
All claims expressed in this article are solely those of the authors and do not necessarily represent those of their affiliated organizations, or those of the publisher, the editors and the reviewers. Any product that may be evaluated in this article, or claim that may be made by its manufacturer, is not guaranteed or endorsed by the publisher.
References
1. Benavente J, Martinez-Costas J. Avian reovirus: structure and biology. Virus Res. (2007) 123:105–19. doi: 10.1016/j.virusres.2006.09.005
2. Egana-Labrin S, Jerry C, Roh HJ, da Silva AP, Corsiglia C, Crossley B, et al. Avian reoviruses of the same genotype induce different pathology in chickens. Avian Dis. (2021) 65:530–40. doi: 10.1637/0005-2086-65.4.529
3. Glass SE, Naqi SA, Hall CF, Kerr KM. Isolation and characterization of a virus associated with arthritis of chickens. Avian Dis. (1973) 17:415–24. doi: 10.2307/1589226
4. Heffels-Redmann U, Muller H, Kaleta EF. Structural and biological characteristics of reoviruses isolated from Muscovy ducks (Cairina moschata). Avian Pathol. (1992) 21:481–91. doi: 10.1080/03079459208418866
5. Yun T, Yu B, Ni Z, Ye W, Chen L, Hua J, et al. Isolation and genomic characterization of a classical Muscovy duck reovirus isolated in Zhejiang, China. Infect Genet Evol. (2013) 20:444–53. doi: 10.1016/j.meegid.2013.10.004
6. Huang Y, Zhang J, Dong J, Li L, Kuang R, Sun M, et al. Isolation and characterization of a new goose orthoreovirus causing liver and spleen focal necrosis in geese, China. Transbound Emerg Dis. (2022) 695:3028–34. doi: 10.1111/tbed.14236
7. Yu K, Li Y, Han H, Song M, Ma X, Liu C, et al. Complete genome sequence of an avian reovirus isolated from wild mallard ducks in china. Genome Announc. (2014) 25:e00813–14. doi: 10.1128/genomeA.00813-14
8. Farkas SL, Varga-Kugler R, Marton S, Lengyel G, Palya V, Banyai K. Genomic sequence and phylogenetic analyses of two novel orthoreovirus strains isolated from Pekin ducks in 2014 in Germany. Virus Res. (2018) 257:57–62. doi: 10.1016/j.virusres.2018.09.001
9. Wang S, Lin F, Cheng X, Wang J, Zhu X, Xiao S, et al. The genomic constellation of a novel duck reovirus strain associated with hemorrhagic necrotizing hepatitis and splenitis in Muscovy ducklings in Fujian, China. Mol Cell Probes. (2020) 53:101604. doi: 10.1016/j.mcp.2020.101604
10. Wang W, Liang J, Shi M, Chen G, Huang Y, Zhang Y, et al. The diagnosis and successful replication of a clinical case of Duck Spleen Necrosis Disease: an experimental co-infection of an emerging unique reovirus and Salmonella indiana reveals the roles of each of the pathogens. Vet Microbiol. (2020) 246:108723. doi: 10.1016/j.vetmic.2020.108723
11. Wang H, Gao B, Chen H, Diao Y, Tang Y. Isolation and characterization of a variant duck orthoreovirus causing spleen necrosis in Peking ducks, China. Transbound Emerg Dis. (2019) 66:2033–44. doi: 10.1111/tbed.13252
12. Spandidos DA, Graham AF. Physical and chemical characterization of an avian reovirus. J Virol. (1976) 19:968–76. doi: 10.1128/jvi.19.3.968-976.1976
13. Shih WL, Hsu HW, Liao MH, Lee LH, Liu HJ. Avian reovirus sigmaC protein induces apoptosis in cultured cells. Virology. (2004) 321:65–74. doi: 10.1016/j.virol.2003.12.004
14. Wickramasinghe R, Meanger J, Enriquez CE, Wilcox GE. Avian reovirus proteins associated with neutralization of virus infectivity. Virology. (1993) 194:688–96. doi: 10.1006/viro.1993.1309
15. Goldenberg D, Pasmanik-Chor M, Pirak M, Kass N, Lublin A, Yeheskel A, et al. Genetic and antigenic characterization of sigma C protein from avian reovirus. Avian Pathol. (2010) 39:189–99. doi: 10.1080/03079457.2010.480969
16. Lin YH, Lee LH, Shih WL, Hu YC, Liu HJ. Baculovirus surface display of sigmaC and sigmaB proteins of avian reovirus and immunogenicity of the displayed proteins in a mouse model. Vaccine. (2008) 26:6361–7. doi: 10.1016/j.vaccine.2008.09.008
17. Chen S, Lin F, Chen S, Hu Q, Cheng X, Jiang B, et al. Development of a live attenuated vaccine against Muscovy duck reovirus infection. Vaccine. (2018) 3652:8001–7. doi: 10.1016/j.vaccine.2018.10.102
18. Chen X, Zheng M, Lin F, Cheng X, Xiao S, Chen S, et al. Impacts of novel duck reovirus infection on the composition of intestinal microbiota of Muscovy ducklings. Microb Pathog. (2019) 137:103764. doi: 10.1016/j.micpath.2019.103764
19. Yan H, Xu G, Zhu Y, Xie Z, Zhang R, Jiang S. Isolation and characterization of a naturally attenuated novel duck reovirus strain as a live vaccine candidate. Vet Microbiol. (2021) 261:109214. doi: 10.1016/j.vetmic.2021.109214
20. Bi Z, Zhu Y, Chen Z, Li C, Wang Y, Wang G, et al. Induction of a robust immunity response against novel duck reovirus in ducklings using a subunit vaccine of sigma C protein. Sci Rep. (2016) 6:39092. doi: 10.1038/srep39092
21. Jespersen MC, Peters B, Nielsen M, Marcatili P. BepiPred-20: improving sequence-based B-cell epitope prediction using conformational epitopes. Nucleic Acids Res. (2017) 45:W24–9. doi: 10.1093/nar/gkx346
22. Yin L, Chen L, Luo Y, Lin L, Li Q, Peng P, et al. Recombinant fiber-2 protein protects Muscovy ducks against duck adenovirus 3 (DAdV-3). Virology. (2019) 526:99–104. doi: 10.1016/j.virol.2018.10.011
23. Zhang S, Li W, Liu X, Li X, Gao B, Diao Y, et al. A TaqMan-based real-time PCR assay for specific detection of novel duck reovirus in China. BMC Vet Res. (2020) 16:306. doi: 10.1186/s12917-020-02523-z
24. Wang H, Gao B, Liu X, Zhang S, Diao Y, Tang Y. Pathogenicity of a variant duck orthoreovirus strain in Cherry Valley Ducklings. Vet Microbiol. (2020) 242:108546. doi: 10.1016/j.vetmic.2019.108546
25. Zhang W, Wang M, Yuan S, Li W, Huang J, Sun J, et al. Genetic variation analysis of the sigma B protein gene of novel duck reovirus in southeastern China from 2011 to 2020. J Virol Methods. (2022) 303:114479. doi: 10.1016/j.jviromet.2022.114479
26. Mia MM, Hasan M, Ahmed S, Rahman MN. Insight into the first multi-epitope-based peptide subunit vaccine against avian influenza A virus (H5N6): an immunoinformatics approach. Infect Genet Evol. (2022) 104:105355. doi: 10.1016/j.meegid.2022.105355
27. Sharma S, Kumari V, Kumbhar BV, Mukherjee A, Pandey R, Kondabagil K. Immunoinformatics approach for a novel multi-epitope subunit vaccine design against various subtypes of Influenza A virus. Immunobiology. (2021) 2262:152053. doi: 10.1016/j.imbio.2021.152053
Keywords: novel duck reovirus (NDRV), σB protein, linear B cell epitopes, vaccine, immunization
Citation: Chen Y, Yan Z, Liao C, Song Y, Zhou Q, Shen H and Chen F (2024) Recombinant linear multiple epitopes of σB protein protect Muscovy ducks against novel duck reovirus infection. Front. Vet. Sci. 11:1360246. doi: 10.3389/fvets.2024.1360246
Received: 22 December 2023; Accepted: 11 March 2024;
Published: 13 May 2024.
Edited by:
Shilong Chen, Fujian Academy of Agricultural Sciences, ChinaReviewed by:
Mohsan Ullah Goraya, Huaqiao University, ChinaDipak Deka, Assam Agricultural University, India
Copyright © 2024 Chen, Yan, Liao, Song, Zhou, Shen and Chen. This is an open-access article distributed under the terms of the Creative Commons Attribution License (CC BY). The use, distribution or reproduction in other forums is permitted, provided the original author(s) and the copyright owner(s) are credited and that the original publication in this journal is cited, in accordance with accepted academic practice. No use, distribution or reproduction is permitted which does not comply with these terms.
*Correspondence: Zhuanqiang Yan, Zqyan-2015@wens.com.cn; Hanqin Shen, hqshen@wens.com.cn; Feng Chen, fengch@scau.edu.cn