- 1Department of Parasitology, Faculty of Veterinary Medicine, Sivas Cumhuriyet University, Sivas, Türkiye
- 2Department of Parasitology, Faculty of Veterinary Medicine, Firat University, Elazig, Türkiye
- 3Faculty of Veterinary Medicine, Kyrgyz-Turkish Manas University, Bishkek, Kyrgyzstan
Introduction: Tick-borne pathogens (TBP) are an important group of organisms that can affect animals and humans all over the world. Equine piroplasmosis (EP), caused by Theileria equi and Babesia caballi, is considered one of the most important tick-borne diseases and can cause significant clinical symptoms and mortality in horses. Moreover, EP plays a restrictive role in international horse traditions and transportation. Although these species can cause similar symptoms, there are different 18S rRNA genotypes of T. equi (five genotypes) and B. caballi (three genotypes). Besides piroplasma species, Anaplasma and hemotropic mycoplasmas (HM) are known as other important tick-borne pathogens reported in horses.
Methods: In this study, we investigated the presence, prevalence, genetic diversity, and phylogenetic analyses of TBPs using PCRs and DNA sequencing in grazing horses in Kyrgyzstan. For these purposes, a total of 311 blood samples were collected from Chuy, Issyk-Kul, Naryn, Osh, Talas, and Jalal-Abad.
Results: DNA amplification of TBP revealed that 23 (7.40%) out of 311 samples were found to be positive for T. equi. However, B. caballi, HM, A. phagocytophilum, and A. capra were not detected in this study. The infection rate of T. equi was higher in males (8.11%) than in females (6.35%) (p=0.2880) and in those older than 5 years (9.02%) than in the 1-4 age group (6.35%) (p=0.1950). Phylogenetic analysis of 18S rRNA revealed that A and E genotypes of T. equi have circulated in grazing horses in Kyrgyzstan.
Discussion: Information about the genetic diversity of T. equi is important for understanding the population dynamics of the species and developing effective control strategies against this pathogen. This is the first molecular investigation of A. capra in horses in Kyrgyzstan. Although this pathogen has been detected in different hosts in Kyrgyzstan, it was not detected in this study. However, considering the wide host spectrum of A. capra, it is thought that more large-scale studies are needed to understand the effect of horses on the epidemiology of this pathogen.
1 Introduction
Tick-borne pathogens (TBP) are an important group of organisms that can affect animal and human health all over the World (1). Equine piroplasmosis (EP), equine anaplasmosis (formerly Equine Granulocytic Ehrlichiosis), and hemotropic mycoplasmas (HM) are the main TBPs of horses (2–4). EP is caused by Theileria equi (formerly Babesia equi), Babesia caballi, and the recently described T. haneyi. The disease is observed in horses, mules, donkeys, and zebras (2, 5, 6). EP is a global disease and a few countries such as Australia, Canada, Great Britain, Ireland, Japan, and New Zealand have EP-free status (5, 6). Ixodid ticks such as Dermacentor, Hyalomma, and Rhipicephalus are the biological vectors of EP, and it is mainly transmitted by these vectors. In addition, it has been reported that it is transmitted through contaminated needles, surgical operations, blood transfusion, and transplacental (2, 6, 7). EP exhibits a clinical course ranging from subclinical to acute. Theileria equi causes more severe clinical disease than B. caballi. The clinical signs of EP are fever, anemia, jaundice, and hemoglobinuria (6, 8). The mortality can reach up to 50% in T. equi infections, while 10% in B. caballi infections (6, 8). Horses that recover from the disease remain carriers for a long time. While in T. equi the carrier period lasts lifelong, in B. caballi this period is about 4 years (9). EP causes significant economic losses in the equine industry worldwide and even has a restrictive effect on international horse movements (2, 6).
Equine anaplasmosis (formerly Equine Granulocytic Ehrlichiosis) is a tick-borne disease of horses caused by Anaplasma phagocytophilum (formerly Ehrlichia equi). It has a very wide host spectrum and infects horses, cattle, sheep, goats dogs, cats, and humans (10). The clinical signs occur mostly in adult horses, which include fever, anorexia, depression, reluctance to move, limb edema, petechiation, and icterus (10–12). Anaplasma capra is discovered for the first time in goats from China in 2012 (13). In the short time so far, A. capra has been detected in many different countries including Kyrgyzstan from three continents (Africa, Asia, and Europe) (14–26). The studies show that the A. capra can infect a wide range of host groups including sheep, goats, cattle, water buffaloes, dogs, wild animals (e.g., deer, takin, Persian onegar, muntjac, serow), and humans (13–18, 20–25). Additionally, it was detected in ticks (19). There is no information regarding the presence of A. capra in horses. However, A. capra has been detected in wild onegars (Equus hemionus onager) in Iran (24).
Hemotropic mycoplasmas or hemoplasmas refers to the infection caused by small, cell wall-less Gram-negative bacteria (Mycoplasma spp., class Mollicutes) that attach to the surface of red blood cells, formerly known as eperythrozoonosis and haemobartonellosis. These species infect many animal species (26). The diseases may be transmitted by arthropod vectors such as ticks, lice, flies, and mosquitoes. Additionally, the disease can be transmitted through blood transfusion, contaminated needles or surgical equipment, and vertically (26). Information about HM in horses is scarce. The disease was first detected in horses by microscopic examination of blood smears in Nigeria in 1978 (4). In 2010, the first molecular diagnosis of the disease in horses was made and the species detected in horses from Germany were determined closely related to Mycoplasma haemofelis and Candidatus Mycoplasma haemobos (27). Apart from Germany (27) studies on molecular basis determination of HM in horses were conducted in Iran (28), Nigeria (29), and Brazil (30).
To date, no record of equine-TBPs has been found in the literature in Kyrgyzstan. Recently, it has been reported first molecular presence and prevalence of the important TBPs such as canine and bovine hemotropic mycoplasma species (31, 32), B. vogeli, B. vulpes in dogs (33), A. centrale, A. capra, A. phagocytophilum-like 1, B. major, T. annulata, and T. orientalis in cattle (14, 15, 34, 35), A. ovis, A. capra, and A. phagocytophilum-like 1 in sheep (36) in Kyrgyzstan. In this study, we aimed to survey of TBPs (EP, HM, Anaplasma phagocytophilum, and Anaplasma capra) in grazing horses in Kyrgyzstan and to detect the genetic diversity of the pathogens.
2 Materials and methods
2.1 Study area, collection of blood samples, and DNA extraction
The Republic of Kyrgyzstan is a Central Asia country bordered by Uzbekistan to the west, China to the east and southeast, Tajikistan to the south, and Kazakhstan to the north. The country is generally covered with high mountains and has a continental climate, with hot summers and cold winters. While the summers in low-altitude settlements can get quite hot, the high mountains remain colder even in the hottest months. It consists of seven geographical regions (Talas, Naryn, Batken, Issyk-Kul, Chuy, Osh, and Jalal-Abad) (Figure 1) (37).
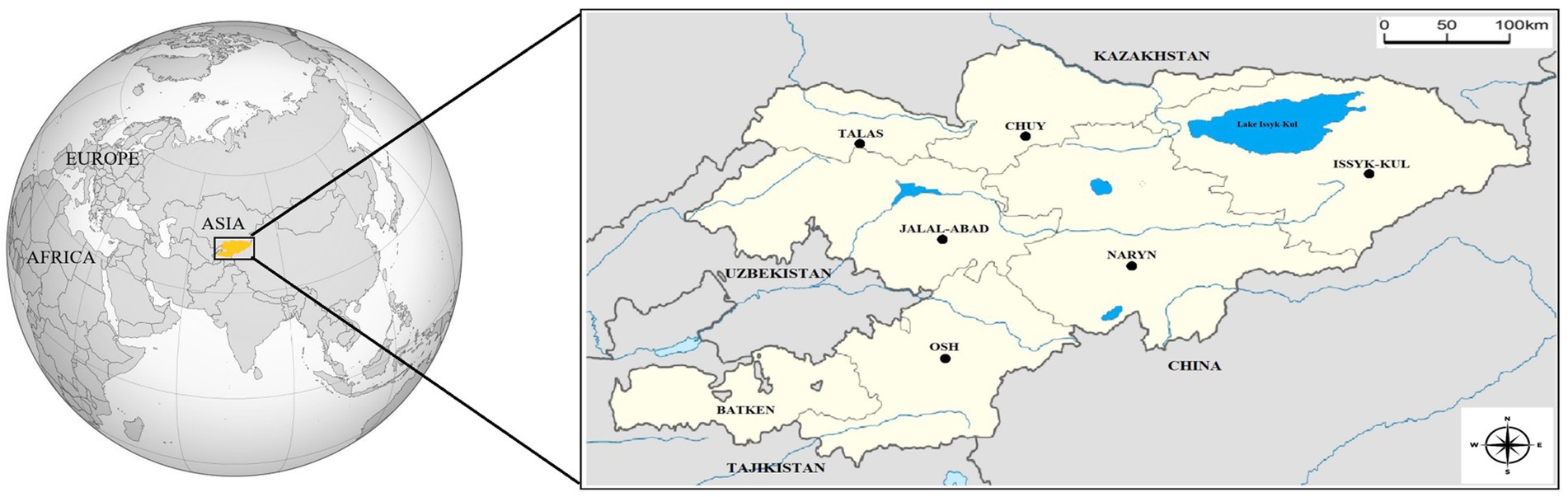
Figure 1. Location of Kyrgyzstan on the world map (Yellow). Neighbors and geographical regions of Kyrgyzstan. Sampling regions were shown with circles.
The blood samples were collected from 311 grazing horses from 35 flocks in 21 settlements in 6 geographical regions (Chuy, Osh, Naryn, Talas, Jalal-Abad, and Issyk-Kul) of Kyrgyzstan (Figure 1) between March 2022 and July 2023. Horse flocks in the settlements selected for the study and the animals from which blood was obtained in the flocks were randomly selected. The age and gender of the animals were recorded (Table 1). There were not any clinical signs on horses at first examination and they were recorded as healthy.
The total genomic DNAs were obtained from blood samples using a commercial DNA isolation kit (GeneAll Exgene™ Clinic SV kit, 108–152, GeneAll®, Seoul, South Korea). These gDNA samples were sored −20°C until use.
2.2 Survey of equine piroplasmosis, hemotropic mycoplasmas, Anaplasma phagocytophilum, and Anaplasma capra by polymerase chain reaction
The four different polymerase chain reaction (PCR) were used for the survey of equine piroplasmosis (EP), hemotropic mycoplasmas (HM), Anaplasma phagocytophilum, and Anaplasma capra DNAs in the study. A nested PCR was carried out for the amplification of Theileria spp. and Babesia spp. 18S rRNA gene. First, 1,600 bp of the 18S rRNA gene of Theileria spp. and Babesia spp. was amplified by PCR from the samples with Nbab-1F and Nbab-1R primers (38). In nested PCR using these PCR products as templates, ~500 bp fragments of the same gene was amplified with BJ1 and BN2 primers (39). To survey of HM species in the samples, the primers amplifying 192 bp fragments of 16S rRNA gene were used in the PCR (40). To survey of A. phagocytophilum in the samples SSAP2f and SSAP2r primers amplifying 641/642 bp fragments of 16S rRNA gene were used in the PCR (41). Anaplasma capra was researched by a nested PCR in the samples. First, 1,031 bp fragments of the gltA gene (20) and then 594 bp fragments of the same gene (19) were amplified by PCR. The detailed information about PCRs and the primers is presented in Table 2.
The PCR assays were performed as described before (19, 20, 38–41), and the genomic DNA of B. vogeli (Accession number: OR116199) (33), A. phagocytophilum (Accession number: OP828919) (42), A. capra (Accession number: ON783818) (22), and M. wenyonii (Accession number: OM468183) (43) were used as the positive controls, and DNase-RNase-free sterile water (Cat No.: 129114, Qiagen®, Germany) was used as the negative control in the PCRs.
PCR products were loaded on 1.5% agarose gel containing ethidium bromide and visualized under a UV transilluminator. The DNA extraction, PCR, and gel electrophoresis were performed in separate compartments of the laboratory to minimize the risk of contamination.
2.3 Sequencing and phylogenetic analysis
All positive samples obtained in the study were sequenced using the primers listed in Table 1.
The amplicons were purified from agarose gel using a commercial gel extraction kit (PCR Clean-Up & Gel Extraction Kit, GeneDireX®, Cat.No.: NA006-0300). The purified products were sent to bidirectionally sequence (ABI 3730XL analyzer, Applied Biosystems, Foster City, CA). The BigDye Terminator v3.1 Cycle Sequencing Kit was used in the reactions (Applied Biosystems, Foster City, CA).
The obtained sequences were aligned with each other with the MUSCLE algorithm of MEGA-11 software (44), and consensus sequences were constructed. Accession numbers were obtained by submitting them to GenBank. Phylogenetic analyses of gene sequences identified in this study were performed and genotypes of the T. equi were determined.
The best-fit model for maximum likelihood was determined as the TN93 + G parameter model (45) using the Find Best-Fit Substitution Model in MEGA-11 (44). The phylogenetic trees were created using maximum likelihood analysis in Mega 11 (44) (Figure 2).
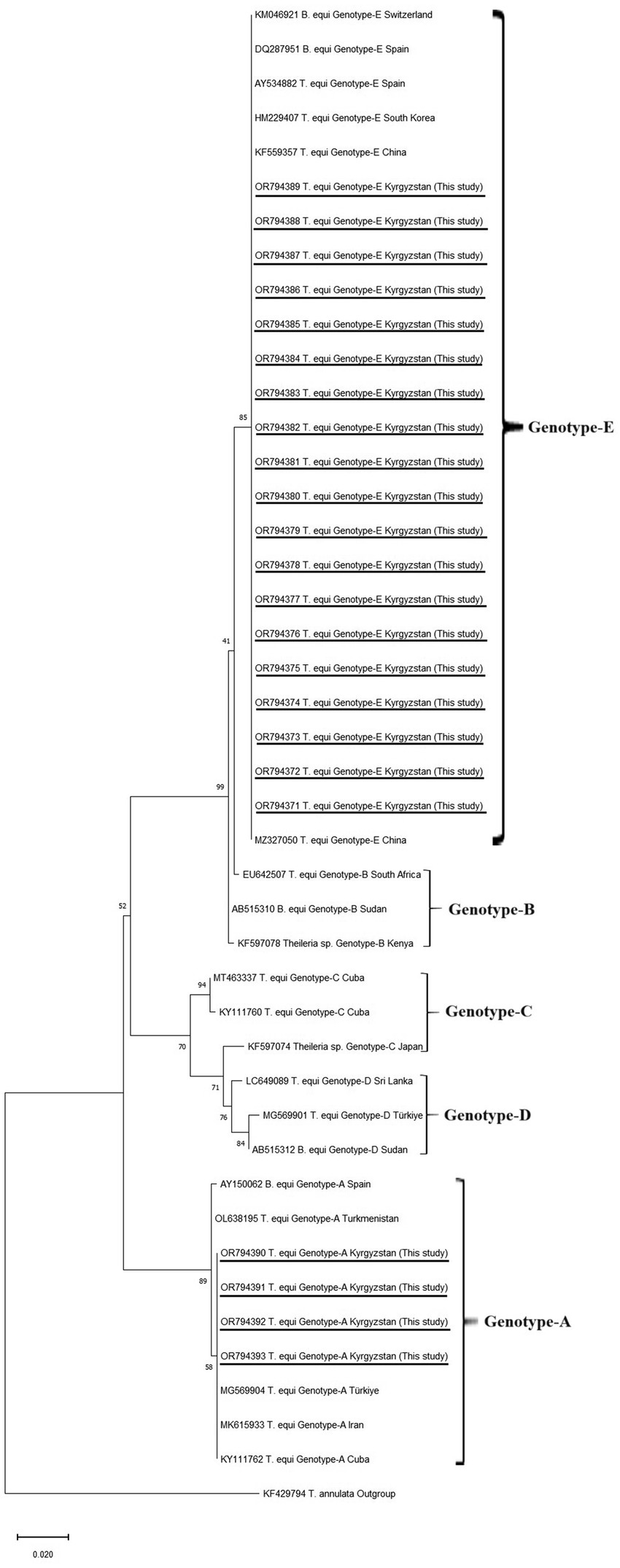
Figure 2. Phylogenetic trees according to 18S rRNA sequences of Theileria equi genotypes. The figure was created using the ML method. Bootstrap values were performed with 1,000 replicates. The evolutionary history was performed by using the ML method TN93 + G model were used of T. equi genotypes (45). Evolutionary analyses were conducted in MEGA-11 (44). T. annulata was used as an outgroup.
2.4 Statistical analysis
The chi-square test (χ2) was used to determine the differences among various parameters. p < 0.05 was accepted to be statistically significant.
2.5 Ethics statement
The ethical permission was granted from the Kyrgyz-Turkish Manas University Animal Experiments Local Ethics Committee with number 2023/12. The owners of the horses also gave their oral permission to collect the blood samples from their animals.
3 Results
3.1 The results of PCRs
A total of 311 horse blood samples were analyzed by equine piroplasmosis (EP), hemotropic mycoplasmas (HM), Anaplasma phagocytophilum, and Anaplasma capra specific-PCRs. As a result of PCRs, positivity was detected in 23 samples only with EP specific PCR, while no positivity was detected in the other three PCRs.
The 23 positive samples were identified as T. equi by sequencing (section of sequencing of 18S rRNA gene and phylogenetic analysis). Accordingly, PCR results based on sequence analyses are given in Table 3. The total prevalence of T. equi was determined as 7.40% (23/311). This rate was found to be 8.11% in males (15/185) and 6.35% in females (8/126) (p = 0.2880). It was determined as 6.35% (12/189) in the 1–4 age group and 9.02% (11/122) in those aged 5 and older (p = 0.1950) (Table 3).
3.2 Sequencing of 18S rRNA gene and phylogenetic analysis
Equine piroplasmos (EP) was detected in 23 samples by PCR. The partial sequence analyses of 18S rRNA revealed that 19 positive samples were matched with the T. equi E genotype, whereas four samples were T. equi A genotypes. The consensus sequences were uploaded to the GenBank under accession numbers T. equi E genotypes: OR794371-OR794389, T. equi A genotypes: OR794390-OR794393.
The sequences of T. equi E genotypes identified in this study were 100% identical to each other. High nucleotide similarities (99.78–100%) were seen between T. equi E genotypes obtained in this study and T. equi E genotype uploaded to the GenBank in different parts of the world. Furthermore, 100% nucleotide identities were present between our isolate and T. equi isolates identified from Ukraine (KP868757), China (OQ692565 and MZ327270), Russia (OM475525), Austria (MW446331), and Portugal (MT767169).
Theileria equi A genotypes obtained in this study had 100% nucleotide identities with each other. The 99.35–100% nucleotide similarities were determined between our sequence and T. equi A genotypes present in the GenBank deposited from various countries. The T. equi A genotype sequences obtained in this study showed 100% nucleotide similarities to those of T. equi A genotypes identified in Türkiye (MG569905), France (MK732476, MF510478), Portugal (MT767167), Israel (MK392060, MK063843), Saudi Arabia (LC431545, KJ801931), Egypt (MN625898), Chile (MT463613), Brazil (MG052917, KY952237), Cuba (KY111762), and United States (CP099438 and JX177673).
The phylogenetic tree showed that our T. equi Genotype-E and Genotype-A clustered with Genotype-E and Genotype-A identified from different countries, respectively.
4 Discussion
The factors such as global warming, increased use of rural areas, animal movements, and the contribution of migratory birds increase the spread and importance of TBPs (1). Knowing basic epidemiological information plays a critical role in the development and implementation of effective and widespread control methods for these diseases. Obtaining the most epidemiological data can undoubtedly be achieved through studies using methods with high specificity and sensitivity in different regions of the world. Equine piroplasmosis, an important TBP of horses, can be diagnosed by microscopic, serological, and molecular techniques (6). Microscopic examination of stained blood smears has the disadvantage of low sensitivity, especially in chronic infections with low parasitemia. The complement fixation technique, the indirect fluorescence antibody test, and the competitive inhibition enzyme-linked immunosorbent assay are used to detect parasite-specific antibodies (6). The most important disadvantage of serological methods is that they do not distinguish between current and previous infections (8). Molecular diagnostic techniques, especially PCR, stand out with their superiority in both specificity and sensitivity in the diagnosis of EP. In recent years, these methods have been widely used in epidemiological studies (46–50). Although the presence of different TBPs in cattle, sheep, and dogs (HM species, B. vogeli, B. vulpes, A. centrale, A. capra, A. phagocytophilum-like 1, A. ovis, B. major, T. annulata, and T. orientalis) has been previously reported in Kyrgyzstan (14, 15, 31–36), this study is the first molecular study investigating TBPs in horses in the country.
In recent studies, it has been shown that EP agents are divided into five genotypes (A, B, C, D, and E) based on the 18S rRNA gene (46, 51–53). Moreover, it has been reported that the T. equi-C genotype includes the species described as T. haneyi (5). In 2001, it was reported that T. equi has two genotypes (Florida (United States) and Pelotas (Southern Brazil) genotypes) based on the EMA-1 gene (54). The 18S rRNA gene has advantages in genotyping studies due to the fact that it has multiple copies in the genome and the hypervariable region is also located here (55, 56). It has been used extensively in studies on this subject. Firstly, B. equi Spain 1 and B. equi Spain 2 were reported in 2003, based on the 18S rRNA gene (57). In another conducted in Spain in 2004, B. equi Spain 1 and B. equi Spain 2 isolates were located in the same cluster, while T. equi-like was located in a different cluster, thus supporting the existence of two genotypes based on two 18S rRNA genes (58). In a more comprehensive study conducted in South Africa in 2009, a third genotype was detected, and the genotypes up to that time were named genotypes A, B, and C (59). According to the study, B. equi Spain-1 and B. equi Spain-2 isolates detected from horses and T. equi isolate detected from dogs in Spain (57) were collected under genotype A; T. equi-like isolates detected from horses in Spain and (58), and from zebra in South Africa (59) were collected genotype B, and T. equi isolates detected from horses in South Africa (59) were collected under genotype C (59). Following these, the presence of genotype D in horses in Sudan was revealed in 2010 (60), and genotype E was revealed in 2012 based on previous South Korean-T. equi sequences (61). In this study, 23 samples were sequenced. As a result of phylogenetic analysis based on 18S rRNA gene DNA sequences, we identified two genetically distinct T. equi genotypes (A—4 samples and E—19 samples) in grazing horses from Kyrgyzstan. This is the first study that reports of T. equi and its genotypes in Kyrgyzstan. Our results confirm that different genotypes of T. equi coexist within the same population and the heterogeneous nature of this species, in agreement with previous studies (46, 51–53, 62). However, there is still a need for molecular surveys to determine the geographical distribution of the genotypes and their impact on the course of clinical infections, as well as studies on differences in vectors.
The PCR-positivity for T. equi was found to be 7.40% (23/311). While it is close to the rate previously reported in horses in Türkiye (8.8%) (63), it is generally lower than the positivity rates reported in many parts of the world such as 19.7% in Türkiye (49), 38.9% in China (50), 44.5% in Spain (64), 61.9% in Gambia (65), 70.3% in Italy (66), 73.0% in Cuba (47). The differences among T. equi positivity rates may be related to sample numbers, the method used in the studies, age groups, animal management system, ecological factors, tick species in the region, and other risk factors.
The age of horses may be considered a risk factor for T. equi infections due to longer tick exposure. Additionally, the fact that T. equi is a lifelong carrier in horses may increase the percentage of positive older horses (6, 67). Bartolomé del Pino et al. (66) reported T. equi-PCR-positivity significantly decreases with age in Itay. On the other hand, Rueg et al. (68) reported that T. equi positivity increased with age in Mongolia. Unlike both cases, it has been reported that the T. equi-PCR-positivity rate has no connection with age in Türkiye (69). These different results may be due to multivariate factors related to long-term parasite host circulation processes, which need to be explained on the basis of host–parasite interaction, and the sensitivity of the methods used may also affect this situation. Although the prevalence of T. equi was high in horses older than 5 years of age in this study, this difference was found to be statistically insignificant (p = 0.1950). On the other hand, all animals examined in this study appear healthy. When all the data are evaluated together, it may contribute to the understanding of the long-term carrier status of horses. The decrease in prevalence with age in B. caballi is attributed to the clearance of the agents in 4 years and the loss of antibodies in the following period (66). This may be related to the overall low prevalence of B. caballi and the fact that it was not detected in this study.
While there are studies showing that gender is related to T. equi positivity (64, 70, 71), there are also studies showing that gender is not a risk factor (72, 73). In our study, T. equi prevalence by gender was found to be statistically insignificant (p = 0.2880). It is thought that this situation may be due to the husbandry techniques.
Equine anaplasmosis is mainly common in regions where Ixodes species occur, especially in northern America, and is also seen in Europe, Africa, and Southern America (3, 12, 74). It has been determined that the prevalence of A. phagocytophilum in Germany peaked in relation to the activity of I. ricinus (75). Anaplasma phagocytophilum-PCR positivity in horses was detected as 13% in the humid region of Tunisia (76), 8% in Italy (77), 4.3% in Pakistan (77), and 15% in the island of Sardinia (74). Although A. capra was detected in 2012 (13), it was included in this study because it was found in a very large host group and was found in wild onegar in Iranian (24), a species relatively close to horses. There has been no previous study investigating A. capra in horses. Anaplasma capra and A. phagocytophilum could not be detected in horses in our study. Unlike the above regions, Kyrgyzstan is a very mountainous country with no sea borders. The continental climate of the country and the related tick fauna may directly affect the presence and prevalence of TBPs. In our study, the animals whose blood was collected appeared healthy and no ticks were found. However, more comprehensive studies including tick species in the country are needed.
There is a paucity of information on the prevalence and distribution of hemotropic mycoplasma species in horses. Molecular-based studies have shown that the hemoplasma species detected in horses are species associated with different animal species (27–30). In these studies, Canditus M. haemobos and M. haemofelis, which are related to cattle and cats, respectively, in Germany (27), M. ovis which is mostly related to sheep in Brazil (30), Mycoplasma ovis-like and Candidatus M. haemocervae were detected in horses from Iran and Nigeria, respectively (28, 29). Besides this, in three different studies investigating TBPs in horses in Brazil, the presence of T. equi and B. caballi was reported, but hemotropic mycoplasma could not be detected in the horses (78–80). Additionally, some researchers have stated that hemoplasma infections may be accidental or an uncommon disease in horses (78). In this study, hemoplasma species were not detected in horses, and this may be related to the absence of hemoplasma species in other animals in the sampling areas.
5 Conclusion
The global importance of TBPs is increasing among hosts, and equine piroplasmosis is the most important TBD in horses. Although different genotypes of B. caballi and T. equi have been detected, the clinical relationship, geographical distribution, and vectors of these genotypes need to be revealed. This study revealed the existence of A and E genotypes of T. equi in grazing horses from Kyrgyzstan, and this information helps to understand the epidemiology of these T. equi genotypes. In this study, A. phagocytophilum, A. capra, and HM were detected in horses. A. capra, a relatively novel species, is thought to have a global distribution, and large-scale studies are still needed to understand the prevalence, distribution, and pathogenesis of this pathogen in horses.
Data availability statement
The datasets presented in this study can be found in online repositories. The names of the repository/repositories and accession number(s) can be found below: https://www.ncbi.nlm.nih.gov/genbank/, OR794390; https://www.ncbi.nlm.nih.gov/genbank/, OR794391; https://www.ncbi.nlm.nih.gov/genbank/, OR794392; https://www.ncbi.nlm.nih.gov/genbank/, OR794393.
Ethics statement
The animal studies were approved by Kyrgyzs-Turkish Manas Unıversity Animal Experiments Local Ethics Committee with decision number 2023/12. The studies were conducted in accordance with the local legislation and institutional requirements. Written informed consent was obtained from the owners for the participation of their animals in this study.
Author contributions
KA: Conceptualization, Data curation, Formal analysis, Investigation, Methodology, Project administration, Resources, Software, Supervision, Validation, Visualization, Writing – original draft, Writing – review & editing. UE: Conceptualization, Data curation, Formal analysis, Investigation, Methodology, Validation, Writing – review & editing. OS: Data curation, Formal analysis, Investigation, Methodology, Writing – review & editing. MU: Data curation, Formal analysis, Investigation, Writing – review & editing. AA: Data curation, Formal analysis, Investigation, Methodology, Writing – review & editing. MA: Conceptualization, Data curation, Formal analysis, Investigation, Methodology, Resources, Writing – original draft, Writing – review & editing.
Funding
The author(s) declare that no financial support was received for the research, authorship, and/or publication of this article.
Acknowledgments
The material for this study was obtained from horses owned in Kyrgyzstan. We would like to thank the animal owners for their cooperation.
Conflict of interest
The authors declare that the research was conducted in the absence of any commercial or financial relationships that could be construed as a potential conflict of interest.
The author(s) declared that they were an editorial board member of Frontiers, at the time of submission. This had no impact on the peer review process and the final decision.
Publisher’s note
All claims expressed in this article are solely those of the authors and do not necessarily represent those of their affiliated organizations, or those of the publisher, the editors and the reviewers. Any product that may be evaluated in this article, or claim that may be made by its manufacturer, is not guaranteed or endorsed by the publisher.
References
1. Inci, A, Yildirim, A, Duzlu, O, Doganay, M, and Aksoy, S. Tick-borne diseases in Turkey: a review based on one health perspective. PLoS Negl Trop Dis. (2016) 10:e0005021. doi: 10.1371/journal.pntd.0005021
2. Scoles, GA, and Ueti, MW. Vector ecology of equine piroplasmosis. Annu Rev Entomol. (2015) 60:561–80. doi: 10.1146/annurev-ento-010814-021110
3. Korbutiak, E, and Schneiders, D. Equine granulocytic ehrlichiosis in the UK. Vet Rec. (1994) 135:387–8. doi: 10.1136/vr.135.16.387
4. Fard, RMN, Vahedi, SM, and Mohammadkhan, F. Haemotropic mycoplasmas (haemoplasmas): a review. Int J Adv Bio Biomed Res. (2014) 2:1484–503.
5. Knowles, DP, Kappmeyer, LS, Haney, D, Herndon, DR, Fry, LM, Munro, JB, et al. Discovery of a novel species, Theileria haneyi n. sp., infective to equids, highlights exceptional genomic diversity within the genus Theileria: implications for apicomplexan parasite surveillance. Int J Parasitol. (2018) 48:679–90. doi: 10.1016/j.ijpara.2018.03.010
6. Tamzali, Y. Equine piroplasmosis: an updated review. Equine Vet Educ. (2013) 25:590–8. doi: 10.1111/eve.12070
7. Allsopp, MTEP, Lewis, BD, and Penzhorn, BL. Molecular evidence for transplacental transmission of Theileria equi from carrier mares to their apparently healthy foals. Vet Parasitol. (2007) 148:130–6. doi: 10.1016/j.vetpar.2007.05.017
8. Wise, LN, Kappmeyer, LS, Mealey, RH, and Knowles, DP. Review of equine piroplasmosis. J Vet Intern Med. (2013) 27:1334–46. doi: 10.1111/jvim.12168
9. Rothschild, CM. Equine piroplasmosis. J Equine Vet. (2013) 33:497–508. doi: 10.1016/j.jevs.2013.03.189
10. Karshima, SN, Ahmed, MI, Kogi, CE, and Iliya, PS. Anaplasma phagocytophilum infection rates in questing and host-attached ticks: a global systematic review and meta-analysis. Acta Trop. (2022) 228:106299. doi: 10.1016/j.actatropica.2021.106299
11. Pusterla, N, Huder, JB, Feige, K, and Lutz, H. Identification of a eranulocytic Ehrlichia strain isolated from a horse in Switzerland and comparison with other Rickettsiae of the Ehrlichia phagocytophila genogroup. J Clin Microbiol. (1998) 36:2035–7. doi: 10.1128/JCM.36.7.2035-2037.1998
12. Woldehiwet, Z. The natural history of Anaplasma phagocytophilum. Vet Parasitol. (2010) 167:108–22. doi: 10.1016/j.vetpar.2009.09.013
13. Liu, Z, Ma, M, Wang, Z, Wang, J, Peng, Y, Li, Y, et al. Molecular survey and genetic identification of Anaplasma species in goats from central and southern China. Appl Environ Microbiol. (2012) 78:464–70. doi: 10.1128/AEM.06848-11
14. Altay, K, and Erol, USahin OF. The first molecular detection of Anaplasma capra in domestic ruminants in the central part of Turkey, with genetic diversity and genotyping of Anaplasma capra. Trop Anim Health Prod. (2022) 54:129–8. doi: 10.1007/s11250-022-03125-7
15. Altay, K, Erol, U, and Sahin OFAytmirzakizi, A. First molecular detection of Anaplasma species in cattle from Kyrgyzstan; molecular identification of human pathogenic novel genotype Anaplasma capra and Anaplasma phagocytophilum related strain. Ticks Tick Borne Dis. (2022) 13:101861. doi: 10.1016/j.ttbdis.2021.101861
16. Barradas, PF, Mesquita, JR, Ferreira, P, Gartner, F, Carvalho, M, Inacio, E, et al. Molecular identification and characterization of Rickettsia spp. and other tick-borne pathogens in cattle and their ticks from Huambo, Angola. Ticks Tick Borne Dis. (2021) 12:101583. doi: 10.1016/j.ttbdis.2020.101583
17. Jouglin, M, Blanc, B, de la Cotte, N, Bastian, S, Ortiz, K, and Malandrin, L. First detection and molecular identification of the zoonotic Anaplasma capra in deer in France. PLoS One. (2019) 14:e0219184. doi: 10.1371/journal.pone.0219184
18. Remesar, S, Prieto, A, Garcia-Dios, D, Lopez-Lorenzo, G, Martinez-Calabuig, N, Diaz-Cao, JM, et al. Diversity of Anaplasma species and importance of mixed infections in roe deer from Spain. Transbound Emerg Dis. (2022) 69:e374–85. doi: 10.1111/tbed.14319
19. Yang, J, Liu, Z, Niu, Q, Liu, J, Han, R, Liu, G, et al. Molecular survey and characterization of a novel Anaplasma species closely related to Anaplasma capra in ticks, northwestern China. Parasit Vectors. (2016) 9:603–5. doi: 10.1186/s13071-016-1886-6
20. Li, H, Zheng, YC, Ma, L, Jia, N, Jiang, BG, Jiang, RR, et al. Human infection with a novel tick-borne Anaplasma species in China: a surveillance study. Lancet Infect Dis. (2015) 15:663–70. doi: 10.1016/S1473-3099(15)70051-4
21. Peng, Y, Wang, K, Zhao, S, Yan, Y, and Wang, H. Jing J, et alp detection and phylogenetic characterization of Anaplasma capra: an emerging pathogen in sheep and goats in China. Front Cell Infect Microbiol. (2018) 8:283. doi: 10.3389/fcimb.2018.00283
22. Sahin OFErol, U, and Altay, K. Buffaloes as new hosts for Anaplasma capra: molecular prevalence and phylogeny based on gtlA, groEL, and 16S rRNA genes. Res Vet Sci. (2022) 152:458–64. doi: 10.1016/j.rvsc.2022.09.008
23. Shi, K, Li, J, Yan, Y, Chen, Q, Wang, K, Zhou, Y, et al. Dogs as new hosts for the emerging zoonotic pathogen Anaplasma capra in China. Front Cell Infect Microbiol. (2019) 9:394. doi: 10.3389/fcimb.2019.00394
24. Staji, H, Yousefi, M, Hamedani, MA, Tamai, IA, and Khaligh, SG. Genetic characterization and phylogenetic of Anaplasma capra in Persian onagers (Equus hemionus onager). Vet Microbiol. (2021) 261:109199. doi: 10.1016/j.vetmic.2021.109199
25. Yang, J, Li, Y, Liu, Z, Liu, J, Niu, Q, Ren, Q, et al. Molecular detection and characterization of Anaplasma spp. in sheep and cattle from Xinjiang, Northwest China. Parasit Vectors. (2015) 8:108. doi: 10.1186/s13071-015-0727-3
26. Matwari, HF, Ahmed, JA, and Saad, KM. Hemomycoplasmosis (Eperythrozoonosis) in domestic animals (a review). Iosr-Javs. (2022) 15:14–9. doi: 10.9790/2380-1507011419
27. Dieckmann, SM, Winkler, M, Groebel, K, Dieckmann, MP, Hofmann-Lehmann, R, Hoelzle, K, et al. Haemotrophic Mycoplasma infection in horses. Vet Microbiol. (2010) 145:351–3. doi: 10.1016/j.vetmic.2010.04.009
28. Kalantari, M, Sharifiyazdi, H, Ghane, M, and Nazifi, S. The occurrence of hemotropic Mycoplasma ovis-like species in horses. Prev Vet Med (2020) 175:104877:104877, doi: 10.1016/j.prevetmed.2019.104877
29. Happi, AN, and Oluniyi, PE. A rare case of equine Haemotropic Mycoplasma infection in Nigeria. Niger Vet J. (2020) 41:274–86. doi: 10.4314/nvj.v41i3.8
30. Kakimori, MTA, Barros, LD, Collere, FCM, Ferrari, LDR, Matos, A, Lucas, JI, et al. First molecular detection of Mycoplasma ovis in horses from Brazil. Acta Trop. (2023) 237:106697. doi: 10.1016/j.actatropica.2022.106697
31. Altay, K, Aydın, MF, Aytmirzakizi, A, Jumakanova, Z, Cunusova, A, and Dumanlı, N. First molecular evidence for Mycoplasma haemocanis and Candidatus Mycoplasma haematoparvum in asymptomatic shelter dogs in Kyrgyzstan. Kafkas Univ Vet Fak Derg. (2020) 26:143–6. doi: 10.9775/kvfd.2019.22196
32. Altay, K, Sahin OFErol, U, and Aytmirzakizi, A. First molecular detection and phylogenetic analysis of Mycoplasma wenyonii and Candidatus Mycoplasma haemobos in cattle in different parts of Kyrgyzstan. Biologia. (2023) 78:633–40. doi: 10.1007/s11756-022-01292-4
33. Altay, K, Erol, U, OF, S, Aydin, MF, Aytmirzakizi, A, and Dumanli, N. First molecular evidence of Babesia vogeli, Babesia vulpes, and Theileria ovis in dogs from Kyrgyzstan. Pathogens. (2023) 12:1046. doi: 10.3390/pathogens12081046
34. Aktas, M, Kisadere, I, Ozubek, S, Cihan, H, Salıkov, R, and Cirak, VY. First molecular survey of piroplasm species in cattle from Kyrgyzstan. Parasitol Res. (2019) 118:2431–5. doi: 10.1007/s00436-019-06370-2
35. Ozubek, S, Ulucesme, MC, Cirak, VY, and Aktas, M. Detection of Theileria orientalis genotypes from cattle in Kyrgyzstan. Pathogens. (2022) 11:1185. doi: 10.3390/pathogens11101185
36. Altay, K, Erol, U, OF, S, Aytmirzakizi, A, Temizel, EM, Aydin, MF, et al. The detection and phylogenetic analysis of Anaplasma phagocytophilum-like 1, A. ovis and A. capra in sheep: A. capra divides into two genogroups. Vet Res Commun. (2022) 46:1271–9. doi: 10.1007/s11259-022-09998-1
37. Frenken, K. Irrigation in Central Asia in figures: AQUASTAT Survey-2012. FAO Water Reports. Roma: (2013).
38. Oosthuizen, MC, Zweygarth, E, Collins, NE, Troskie, M, and Penzhorn, BL. Identification of a novel Babesia sp. from a sable antelope (Hippotragus niger Harris, 1838). J Clin Microbiol. (2008) 46:2247–51. doi: 10.1128/JCM.00167-08
39. Casati, S, Sager, H, Gern, L, and Piffaretti, JC. Presence of potentially pathogenic Babesia sp. for human in Ixodes ricinus in Switzerland. Ann Agric Environ Med. (2006) 13:65–70.
40. Jensen, WA, Lappin, MR, Kamkar, S, and Reagan, WJ. Use of a polymerase chain reaction assay to detect and differentiate two strains of Haemobartonella felis in naturally infected cats. Am J Vet Res. (2001) 62:604–8. doi: 10.2460/ajvr.2001.62.604
41. Kawahara, M, Rikihisa, Y, Lin, Q, Isogai, E, Tahara, K, Itagaki, A, et al. Novel genetic variants of Anaplasma phagocytophilum, Anaplasma bovis, Anaplasma Centrale, and a novel Ehrlichia sp. in wild deer and ticks on two major islands in Japan. Appl Environ Microbiol. (2006) 72:1102–9. doi: 10.1128/AEM.72.2.1102-1109.2006
42. Sahin OFErol, U, Duzlu, O, and Altay, K. Molecular survey of Anaplasma phagocytophilum and related variants in water buffaloes: the first detection of Anaplasma phagocytophilum-like 1. Comp Immunol Microbiol Infect Dis. (2023) 98:102004. doi: 10.1016/j.cimid.2023.102004
43. Erol, U, and Sahin OFAltay, K. Molecular prevalence of bovine hemoplasmosis in Turkey with first detection of Mycoplasma wenyonii and Candidatus Mycoplasma haemobos in cattle and water buffalo. Vet Res Commun. (2023) 47:207–15. doi: 10.1007/s11259-022-09943-2
44. Tamura, K, Stecher, G, and Kumar, S. MEGA11: molecular evolutionary genetics analysis version 11. Mol Biol Evol. (2021) 38:3022–7. doi: 10.1093/molbev/msab120
45. Tamura, K, and Nei, M. Estimation of the number of nucleotide substitutions in the control region of mitochondrial DNA in humans and chimpanzees. Mol Biol Evol. (1993) 10:512–26. doi: 10.1093/oxfordjournals.molbev.a040023
46. Camino, E, Cruz-Lopez, F, de Juan, L, Dominguez, L, Shiels, B, and Coultous, RM. Phylogenetic analysis and geographical distribution of Theileria equi and Babesia caballi sequences from horses residing in Spain. Ticks Tick Borne Dis. (2020) 11:101521. doi: 10.1016/j.ttbdis.2020.101521
47. Diaz-Sanchez, AA, Pires, MS, Estrada, CY, Canizares, EV, del Castillo Dominguez, SL, Cabezas-Cruz, A, et al. First molecular evidence of Babesia caballi and Theileria equi infections in horses in Cuba. Parasitol Res. (2018) 117:3109–18. doi: 10.1007/s00436-018-6005-5
48. Idoko, IS, Levy, ST, Mazuz, ML, Adam, BM, Garba, BS, Nafarnda, DW, et al. Genetic characterization of piroplasms in donkeys and horses from Nigeria. Animals. (2020) 10:324. doi: 10.3390/ani10020324
49. Ozubek, S, and Aktas, M. Genetic diversity and prevalence of piroplasm species in equids from Turkey. Comp Immunol Microbiol Infect Dis. (2018) 59:47–51. doi: 10.1016/j.cimid.2018.08.005
50. Wu, J, Cui, Y, Yu, F, Muhatai, G, Tao, D, Zhao, A, et al. Prevalence and genetic characterization of Theileria equi and Babesia caballi in grazing horses in Xinjiang, northwestern China. Parasitol Res. (2023) 122:387–94. doi: 10.1007/s00436-022-07749-4
51. Peckle, M, Pires, MS, da Silva, CB, da Costa, RL, Vitari, GLV, Senra, MVX, et al. Molecular characterization of Theileria equi in horses from the state of Rio de Janeiro. Brazil Ticks Tick Borne Dis. (2018) 9:349–53. doi: 10.1016/j.ttbdis.2017.11.011
52. Qablan, MA, Obornik, M, Petrzelkova, KJ, Sloboda, M, Shudiefat, MF, Horin, P, et al. Infections by Babesia caballi and Theileria equi in Jordanian equids: epidemiology and genetic diversity. Parasitology. (2013) 140:1096–103. doi: 10.1017/S0031182013000486
53. Wang, J, Liu, J, Yang, J, Wang, X, Li, Z, Jianlin, X, et al. The first molecular detection and genetic diversity of Babesia caballi and Theileria equi in horses of Gansu province, China. Ticks Tick Borne Dis. (2019) 10:528–32. doi: 10.1016/j.ttbdis.2019.01.003
54. Nicolaiewsky, TB, Richter, MF, Lunge, VR, Cunha, CW, Delagostin, O, Ikuta, N, et al. Detection of Babesia equi (Laveran, 1901) by nested polymerase chain reaction. Vet Parasitol. (2001) 101:9–21. doi: 10.1016/s0304-4017(01)00471-x
55. Allsopp, MTEP, and Allsopp, BA. Molecular sequence evidence for the reclassification of some Babesia species. Ann N Y Acad Sci. (2006) 1081:509–17. doi: 10.1196/annals.1373.076
56. Hall, CM, Busch, JD, Scoles, GA, Palma-Cagle, KA, Ueti, MW, Kappmeyer, LS, et al. Genetic characterization of Theileria equi infecting horses in North America: evidence for a limited source of US introductions. Parasit Vectors. (2013) 6:1–12. doi: 10.1186/1756-3305-6-35
57. Criado-Fornelio, A, Martinez-Marcos, A, Buling-Sarana, A, and Barba-Carretero, JC. Molecular studies on Babesia, Theileria and Hepatozoon in southern Europe part I. Epizootiological aspects. Vet Parasitol. (2003) 113:189–201. doi: 10.1016/s0304-4017(03)00078-5
58. Nagore, D, Garcia-Sanmartin, J, Garcia-Perez, AL, Juste, RA, and Hurtado, A. Detection and identification of equine Theileria and Babesia species by reverse line blotting: epidemiological survey and phylogenetic analysis. Vet Parasitol. (2004) 123:41–54. doi: 10.1016/j.vetpar.2004.04.010
59. Bhoora, R, Franssen, L, Oosthuizen, MC, Guthrie, AJ, Zweygarth, E, Penzhorn, BL, et al. Sequence heterogeneity in the 18S rRNA gene within Theileria equi and Babesia caballi from horses in South Africa. Vet Parasitol. (2009) 159:112–20. doi: 10.1016/j.vetpar.2008.10.004
60. Salim, B, Bakheit, MA, Kamau, J, Nakamura, I, and Sugimoto, C. Nucleotide sequence heterogeneity in the small subunit ribosomal RNA gene within Theileria equi from horses in Sudan. Parasitol Res. (2010) 106:493–8. doi: 10.1007/s00436-009-1691-7
61. Qablan, MA, Sloboda, M, Jirku, M, Obornik, M, Dwairi, S, Amr, ZS, et al. Quest for the piroplasms in camels: identification of Theileria equi and Babesia caballi in Jordanian dromedaries by PCR. Vet Parasitol. (2012) 186:456–60. doi: 10.1016/j.vetpar.2011.11.070
62. Liu, Q, Meli, ML, Zhang, Y, Meili, T, Stirn, M, Riond, B, et al. Sequence heterogeneity in the 18S rRNA gene in Theileria equi from horses presented in Switzerland. Vet Parasitol. (2016) 221:24–9. doi: 10.1016/j.vetpar.2016.03.003
63. Guven, E, Avcioglu, H, Ahmet, D, Balkaya, I, Abay, U, Yavuz, S, et al. Prevalence and molecular characterization of Theileria equi and Babesia caballi in jereed horses in Erzurum, Turkey. Acta Trop. (2017) 62:207–13. doi: 10.1515/ap-2017-0025
64. Montes Cortes, MG, Fernandez-Garcia, JL, and Martinez-Estellez, MAH. Seroprevalence of Theileria equi and Babesia caballi in horses in Spain. Parasite. (2017) 24:14. doi: 10.1051/parasite/2017015
65. Coultous, RM, McDonald, M, Raftery, AG, Shiels, BR, Sutton, DGM, and Weir, W. Analysis of Theileria equi diversity in the Gambia using a novel genotyping method. Transbound Emerg Dis. (2020) 67:1213–21. doi: 10.1111/tbed.13454
66. Bartolomé del Pino, LE, Nardini, R, Veneziano, V, Iacoponi, F, Cersini, A, Autorino, GL, et al. Babesia caballi and Theileria equi infections in horses in central-southern Italy: Sero-molecular survey and associated risk factors. Ticks Tick Borne Dis. (2016) 7:462–9. doi: 10.1016/j.ttbdis.2016.01.011
67. De Waal, DT. Equine piroplasmosis: a review. Br Vet J. (1992) 148:6–14. doi: 10.1016/0007-1935(92)90061-5
68. Ruegg, SR, Heinzmann, D, Barbour, AD, and Torgerson, PR. Estimation of the transmission dynamics of Theileria equi and Babesia caballi in horses. Parasitology. (2008) 135:555–65. doi: 10.1017/S0031182008004204
69. Kizilarslan, F, Yildirim, A, Duzlu, O, Inci, A, Onder, Z, and Ciloglu, A. Molecular detection and characterization of Theileria equi and Babesia caballi in horses (Equus ferus caballus) in Turkey. J Equine Vet. (2015) 35:830–5. doi: 10.1016/j.jevs.2015.08.002
70. Moretti, A, Mangili, V, Salvatori, R, Maresca, C, Scoccia, E, Torina, A, et al. Prevalence and diagnosis of Babesia and Theileria infections in horses in Italy: a preliminary study. Vet J. (2010) 184:346–50. doi: 10.1016/j.tvjl.2009.03.021
71. Sevinc, F, Maden, M, Kumas, C, Sevnic, M, and Ekici, OC. A comparative study on the prevalence of Theileria equi and Babesia caballi infections in horse sub-populations in Turkey. Vet Parasitol. (2008) 156:173–7. doi: 10.1016/j.vetpar.2008.06.006
72. Mujica, FF, Perrone, T, Forlano, M, Coronado, A, Melendez, RD, Barrios, N, et al. Serological prevalence of Babesia caballi and Theileria equi in horses of Lara state, Venezuela. Vet Parasitol. (2011) 178:180–3. doi: 10.1016/j.vetpar.2010.12.036
73. Santos, TM, Roier, EC, Santos, HA, Pires, MS, Vilela, JA, Moraes, LM, et al. Factors associated to Theileria equi in equids of two microregions from Rio de Janeiro, Brazil. Rev Bras Parasitol Vet. (2011) 20:235–41. doi: 10.1590/s1984-29612011000300011
74. Alberti, A, Zobba, R, Chessa, B, Addis, MF, Sparagano, O, Parpaglia, MLP, et al. Equine and canine Anaplasma phagocytophilum strains isolated on the island of Sardinia (Italy) are phylogenetically related to pathogenic strains from the United States. Appl Env Microbiol. (2005) 71:6418–22. doi: 10.1128/AEM.71.10.6418-6422.2005
75. Schafer, I, Silaghi, C, Fischer, S, Marsboom, C, Hendrickx, G, Gehlen, H, et al. Detection of Anaplasma phagocytophilum in horses from Germany by molecular and serological testing (2008–2021). Vet Parasitol. (2022) 312:109840. doi: 10.1016/j.vetpar.2022.109840
76. Mghirby, Y, Yaich, H, Ghorbel, A, and Bouattour, A. Anaplasma phagocytophilum in horses and ticks in Tunisia. Parasit Vectors. (2012) 5:180. doi: 10.1186/1756-3305-5-180
77. Passamonti, F, Veronesi, F, Cappelli, K, Capomaccio, S, Coppola, G, Marenzoni, ML, et al. Anaplasma phagocytophilum in horses and ticks: a preliminary survey of Central Italy. Comp Immunol Microbiol Infect Dis. (2010) 33:73–83. doi: 10.1016/j.cimid.2008.08.002
78. Valentea, JDM, Mongruela, ACB, Machadoa, CAL, Chiyob, L, Leandrob, AS, Brittob, AS, et al. Tick-borne pathogens in carthorses from Foz Do Iguaçu City, Paraná state, southern Brazil: a tri-border area of Brazil, Paraguay and Argentina. Vet Parasitol. (2019) 273:71–9. doi: 10.1016/j.vetpar.2019.08.008
79. Vieira, TSWJ, Vidotto, O, Guimaraes, AMS, Santos, AP, Nascimento, NC, Finger, MAP, et al. Use of pan-hemoplasma PCR for screening horses highly exposed to tick bites from southern Brazil. Semin Ciencias Agrarias. (2015) 36:291–4. doi: 10.5433/1679-0359.2015v36n1p291
80. Vieira, RFC, Vidotto, O, Vieira, TSWJ, Guimaraes, AMS, Santos, AP, Nascimento, NC, et al. Molecular investigation of hemotropic mycoplasmas in human beings, dogs and horses in a rural settlement in southern Brazil. Rev Inst Med Trop São Paulo. (2015) 57:353–7. doi: 10.1590/S0036-46652015000400014
Keywords: tick borne pathogens, Theileria equi, genotypes, horse, Kyrgyzstan
Citation: Altay K, Erol U, Sahin OF, Ulucesme MC, Aytmirzakizi A and Aktas M (2024) Survey of tick-borne pathogens in grazing horses in Kyrgyzstan: phylogenetic analysis, genetic diversity, and prevalence of Theileria equi. Front. Vet. Sci. 11:1359974. doi: 10.3389/fvets.2024.1359974
Edited by:
Mingming Liu, Hubei University of Arts and Science, ChinaReviewed by:
Mohammed Hocine Benaissa, Scientific and Technical Research Center on Arid Regions (CRSTRA), AlgeriaJixu Li, Qinghai University, China
Copyright © 2024 Altay, Erol, Sahin, Ulucesme, Aytmirzakizi and Aktas. This is an open-access article distributed under the terms of the Creative Commons Attribution License (CC BY). The use, distribution or reproduction in other forums is permitted, provided the original author(s) and the copyright owner(s) are credited and that the original publication in this journal is cited, in accordance with accepted academic practice. No use, distribution or reproduction is permitted which does not comply with these terms.
*Correspondence: Kursat Altay, a2FsdGF5QGN1bWh1cml5ZXQuZWR1LnRy
†ORCID: Kursat Altay, https://orcid.org/0000-0002-5288-1239
Ufuk Erol, https://orcid.org/0000-0002-6766-1335
Omer Faruk Sahin, https://orcid.org/0000-0002-3230-504X
Mehmet Can Ulucesme, https://orcid.org/0000-0002-4492-143X
Ayperi Aytmirzakizi, https://orcid.org/0000-0003-3363-0941
Munir Aktas, https://orcid.org/0000-0002-3188-8757