- 1College of Veterinary Medicine of Yunnan Agricultural University, Kunming, China
- 2The Faculty of Science and Mathematics, Auburn University, Auburn, AL, United States
- 3The Faculty of Veterinary Medicine, Chiang Mai University, Chiang Mai, Thailand
- 4Faculty of Veterinary Medicine, Khon Kaen University, Khon Kaen, Thailand
Pseudorabies virus (PRV) can cause fatal encephalitis in newborn pigs and escape the immune system. While there is currently no effective treatment for PRV, Scutellaria baicalensis Georgi polysaccharides (SGP) and Rodgersia sambucifolia Hemsl flavonoids (RHF) are traditional Chinese herbal medicines with potential preventive and therapeutic effects against PRV infection. In order to explore which one is more effective in the prevention and treatment of PRV infection in piglets. We investigate the therapeutic effects of RHF and SGP in PRV-infected piglets using clinical symptom and pathological injury scoring systems. The immune regulatory effects of RHF and SGP on T lymphocyte transformation rate, cytokines, T cells, and Toll-like receptors were also measured to examine the molecular mechanisms of these effects. The results showed that SGP significantly reduced clinical symptoms and pathological damage in the lungs, liver, spleen, and kidneys in PRV-infected piglets and the T lymphocyte conversion rate in the SGP group was significantly higher than that in the other treatment groups, this potential dose-dependent effect of SGP on T lymphocyte conversation. Serum immunoglobulin and cytokine levels in the SGP group fluctuated during the treatment period, with SGP treatment showing better therapeutic and immunomodulatory effects in PRV-infected piglets than RHF or the combined SGP + RHF treatment. In conclusion, RHF and SGP treatments alleviate the clinical symptoms of PRV infection in piglets, and the immunomodulatory effect of SGP treatment was better than that of the RHF and a combination of both treatments. This study provides evidence for SGP in controlling PRV infection in piglets.
1 Introduction
Pseudorabies virus (PRV) is extremely infectious in mammals and poses a risk of spill-over transmission to humans (1) and considerable public health challenges. PRV can inhibit host innate immunity and promote viral replication (2). Thus, focusing more on immunity regularity is important for investigating the effect of PRV. For humoral immunity, immunoglobulins IgG, IgA, and IgM are important for immunity against viral infections, with sIgA preventing the local invasion of the virus, and IgG and IgM blocking the spread of the virus through the blood by neutralizing and opsonizing viruses. For cellular immunity, The T lymphocytes have antiviral (3), antifungal, and immunomodulatory functions (4). The T lymphocyte transformation test is a definitive test for detecting the state of cellular immune function, where higher T lymphocyte conversion rates reflect a more pronounced role for T lymphocytes in enhanced immunity (5). Furthermore, CD4+ T cells coordinate the immune response and secrete different lymphocytes that act on the surrounding antigen-presenting cells (6), CD8+ T cells have killing and inhibitory functions that are crucial to the immune system (7); thus, the CD4+/CD8+ ratio can act as an indicator of immune capacity (8). There are also some differences among the different periods of the immune response, during the reactive phase, interleukin (IL)-2 and IL-4 promote the activation, proliferation, and differentiation of T and B cells (9), then, the practical phase, IFN-γ can activate macrophages, enhance their phagocytic and killing activities, and inhibit viral replication (10). In addition, Toll-like receptors (TLRs) possess antiviral biological activities and are significant components of the intrinsic immune system. TLR3 and TLR7 on endosomes recognize nucleic acids (11); TLR3 primarily recognizes double-stranded viral RNA, suggesting that it has important antiviral functions (12), while TLR7 recognizes single-stranded viral RNA. These TLRs are essential for protecting the body against infection (13). To sum up, this immunity helps us to evaluate the immunomodulatory effects of drugs.
Scutellaria baicalensis Georgi (SG) is also known as Chinese skullcap or Huangqin and is a perennial herb in the Lamiaceae Martinov (14). Recent clinical applications of Chinese skullcaps include the treatment of many diseases, including inflammation (15, 16), hypertension (17), cardiovascular disease (18), neurodegeneration (19), and tumors (20). Current studies have confirmed that polysaccharides have a wide range of therapeutic effects and disease-prevention properties, with most traditional Chinese medicinal polysaccharides acting as immune enhancers in vitro and in vivo. Moreover, the dried rhizome of Rodgersia sambucifolia Hemsl (RH), known as Yantuo, or Maoqinggang, is effective in clearing heat, detoxification, dispelling wind, and draining dampness; it has astringent properties and is often used to treat colds, headaches, rheumatism, bone pain, and traumatic bleeding (21). Flavonoids also have important immunomodulatory effects and can play antiviral and anti-infection roles. However, the effect of Scutellaria baicalensis Georgi polysaccharide (SGP) and Rodgersia sambucifolia Hemsl Flavonoid (RHF) on PRV has not been reported.
In order to screen out effective Chinese medicine against PRV, previous research from our group evaluated the safety and immune effects of extracts from RH, Angelica, SG, and the root of Isatis indigotica sourced from Yunnan. RHF and SGP with superior immunomodulatory effects were selected for this study to investigate the therapeutic and immunomodulatory effects of RHF and SGP on piglets infected with PRV.
2 Methods
2.1 Laboratory animals and virus sources
Fifty 15 ± 3 days-old Saba × Duroc hybrid pigs were selected from the Luquan Mountain area of Kunming City, Yunnan Province. Tests for porcine reproductive and respiratory syndrome (PRRSV), PRV, porcine circovirus type 2 (PCV2), and classical swine fever virus (CSFV) antigens were negative. All piglets were born from unvaccinated sows and tested negative for PRV, PCV2, PRRSV, CSFV, and porcine parvovirus using a polymerase chain reaction (PCR) method. The piglets were determined to be free of antibodies against PRV (gE) prior to the study using enzyme-linked immunosorbent assay (ELISA) kits. The piglets were reared under average daylight conditions and provided with standard commercial feed and unrestricted access to water. The PRV gD strain YN (22) was isolated from the lungs of infected pigs in Yunnan Province and used in this study. The PRV titre was 105.5 TCID50/0.1 mL (PK-15 cell line).
2.2 Preparation of Rodgersia sambucifolia Hemsl flavonoids and Scutellaria baicalensis Georgi polysaccharide
2.2.1 Rodgersia sambucifolia Hemsl flavonoids
Dried clover roots of Rodgersia sambucifolia Hemsl. were ground and soaked in 60% ethanol for 24 h at a solid–liquid ratio of 1:60. The mixture was sonicated for 15 min before being placed in a water bath at 70°C for 3 h. The ethanol was evaporated, and the residue was dried. The flavonoid content of the extract was 41.1%, which was determined using the method described previously (23).
2.2.2 Scutellaria baicalensis Georgi polysaccharide
The roots of Scutellaria baicalensis was dried, crushed, and boiled in distilled water at a material-to-liquid ratio of 1:50 for 20 min. The residue was removed from the filtrate, which was then mixed with 95% ethanol and maintained at 4°C overnight. The supernatant was removed to obtain a flocculated precipitate, which was dried to a constant weight at 55°C to obtain the baicalin polysaccharides. The polysaccharide content was 40.3%, which was determined using a previously described method (24).
2.3 Lymphocyte proliferation assay
Peripheral lymphocytes were isolated from the PRV-and anti-PRV-antibody-free piglets as previously described (25). Trypan blue staining was used to perform cell counts from cells cultured in serum-free RPMI 1640 complete solution suspensions. We used a whole cell density of 5 × 109 cells/mL in our experiments. A total of four experimental groups were used (I, II, III, and IV; Table 1) and each experimental group was repeated four times. Cells were incubated for 44 h under 5% CO2 before 5 μL MTT were added to each well under dark conditions. After a 4 h incubation, the absorbance at 570 nm was measured with a UV spectrophotometer. The experiment was repeated three times.
The stimulus index (SI) was calculated as follows (26):
2.4 Piglet PRV infection experimental design
After 1 week of domestication, 50 piglets were randomly divided into five groups with 10 piglets in each group. The first group was the control, in which piglets were administered 1 mL saline solution intramuscularly. The second group included PRV-infected piglets (PRV), and the third and fourth groups were composed of PRV-infected piglets treated with either 200 mg/kg RHF (PRV + RHF) or 200 mg/kg SGP (PRV + SGP), respectively. The fifth group included PRV-infected piglets treated with 100 mg/kg RHF and 100 mg/kg SGP (PRV + RHF + RHF).
On day 0, piglets in groups 2–5 were administered 1 mL PRV nasal drops and the control group received PBS. After infection, groups 3, 4, and 5 received intranasal administration (i.g.) of the corresponding treatments once a day for 21 consecutive days, while distilled water was intragastrically administered to piglets in groups 1 and 2.
2.4.1 Animal ethics statement
All experiments performed in this study were approved by the International Animal Care and Use Committee of the Yunnan Agricultural University (permission code: YAUACUC06; date of publication: July 10, 2017). The study complied with the guidelines of the institutional administrative and ethics committees for laboratory animals.
2.5 Therapeutic effect
2.5.1 Evaluation of clinical symptoms
Either 1 mL PRV or PBS was intranasally administered to each piglet and clinical symptoms were recorded and evaluated daily (Table 2).
2.5.2 Evaluation of pathological injury
At 14 days post-infection (dpi), the piglets were euthanized with 100 mg/kg pentobarbital sodium intravenous injection and the liver, spleen, lung, and kidney tissues were collected and preserved in 10% paraformaldehyde. Each tissue sample was cut into 5 mm × 5 mm × 3 mm sections and processed following standard procedures. Formalin-fixed, paraffin-embedded tissue (FFPE) sections 5 μm in thickness were stained with haematoxylin and eosin (H&E) for microscopy (Motic China Group Co., Ltd.). Lung injury was scored using the Smith score (27) (Table 3), liver injury was scored using the Suzuki pathological score (28) (Table 4), and spleen injury was assessed using a scoring system for the pathological changes observed in PRV-infected piglets (Table 5). Renal injury was evaluated using the Haas grading system (Table 6).
2.6 Immune-regulation effect
2.6.1 Enzyme-linked immunosorbent assay
A specific enzyme-linked immunosorbent assay (ELISA) kit (Shanghai Enzyme-linked Biotechnology Co., Ltd., China) was used to detect the serum levels of IgA, IgM, IgG, IL-2, IL-4, IFN-γ, CD4+, CD8+, and sIgA antibodies following the manufacturer’s instructions.
2.6.2 Quantitative reverse transcriptase PCR
Total RNA was isolated from spleen tissue using TRIzol reagent (Takara, China) and cDNA was synthesised using the First-Strand cDNA Synthesis Kit (Takara, China). Quantitative reverse transcription PCR (qRT-PCR) was performed using a one-step SYBR PrimeScriptTM RT-PCR Kit II (Takara Biotech Co., Ltd., China). β-actin was used as a reference gene to detect TLR3 and TLR7 expression and quantified using the 2(−ΔΔCt) method for statistical analysis. Each assay was repeated three times. The primers used in this study were as follows:
β-actin: (F) TCTGGCACCACACCTTCT, (R) TGATCTGGGTCATCTTCTCAC
TLR3: (F) TCCAACTAACAAACCAGGC, (R) ACATCCTTCCACCATCT
TLR7: (F) TGCTTTCCAGTTGCGACATC, (R) CAGACAAGCCACACAGCGTC.
2.7 Statistical analysis
Data are expressed as the mean ± standard deviation. A one-way analysis of variance (ANOVA) was performed, followed by the Student–Newman–Keul multiple comparison test to compare the means. A p-value <0.01 was considered statistically significant. Analyses were performed using the SYSTAT 9 software package (SPSS 20).
3 Results
3.1 RHF and SGP relieve the clinical symptoms of pseudorabies
After 3 days post infection (dpi), the rectal temperature of the pigs in the four PRV-infected groups was higher than that of the control group (p ≤ 0.01). After 9 dpi, the rectal temperature decreased in the treated group (p ≤ 0.05) before it stabilised after 10 dpi (p > 0.05), whereas that in the PRV group remained higher than that of the control (p ≤ 0.01) (Figure 1A). At 14 dpi, all piglets in the PRV group died, with a mortality rate of 100%, while those in the treatment groups 60% (6/10) (Figure 1C). These results suggested that RHF and SGP alleviated fever symptoms in piglets after PRV infection, with no significant difference between the treatment conditions.
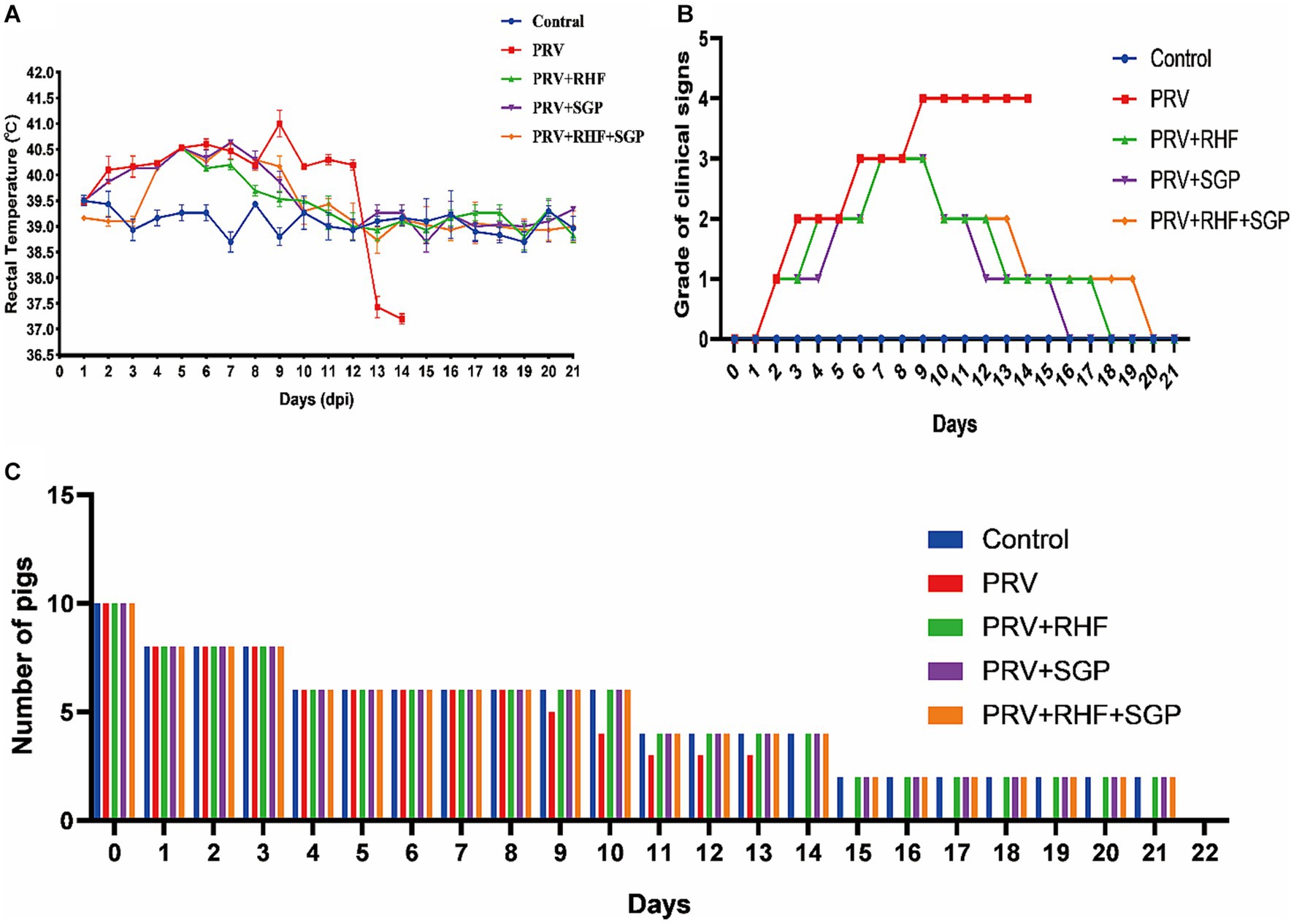
Figure 1. The clinical signs of pseudorabies virus-infected piglets. (A) The rectal temperature change of each group. (B) Clinical signs grade of piglets in each group. (C) The number of piglets surviving in each group.
At 9 dpi, the piglets in the PRV group were inability to stand, exhibited limb paralysis, and finally died at 14 dpi. In the RHF group, the clinical symptom scores initially decreased to 2 points 10 dpi and returned to normal 13 and 18 dpi. The score in the RHF + SGP group decreased to 2 points at 13 dpi, then to 1 point at 14 dpi before it returned to normal at 20 dpi. In contrast, the scores in the SGP group dropped to 1 at 12 dpi and then returned to normal at 16 dpi (Figure 1B). Overall, the clinical symptoms of the PRV-infected piglets were alleviated in all three drug groups, with the greatest effect observed in the SGP group when compared to that in the RHF or RHF + SGP treatment groups.
3.2 RHF and SGP treatments reduce pathological injury in PRV-infected piglets
The H&E-stained lung tissue sections from the PRV group showed focal bleeding, alveolar stenosis, serous exudation, tumour inflammatory cell infiltration, and epithelial cell shedding when compared with those from the control group. The liver tissue sections from the PRV group showed a disordered arrangement of the hepatocyte cords, severe haemorrhage between hepatocytes, hepatocyte necrosis, nucleolar vacuoles, and widening of the hepatic sinuses; while the spleen sections showed a scattered structure in the germinal centre, partial necrosis of lymphocytes, serous exudate in the interstitial tissue, and increased red myeloid erythrocytes; and the kidney sections showed inflammatory erythrocytes in the glomerulus and kidney capsule, shedding of the epithelial cells in the renal tubules, and structures of the glomerulus, distal tubules, and proximal tubules were disordered. In the treatment groups, lung exudation and bleeding were reduced, liver tissue bleeding was reduced, the hepatic cord was neatly arranged, the spleen structure was intact, the germinal centre was dense, and the kidney structure was intact. Together, these results showed that both RHF and SGP alleviated the pathological damage to the heart, liver, spleen, lungs, and kidneys of PRV-infected piglets (Figures 2A–D).
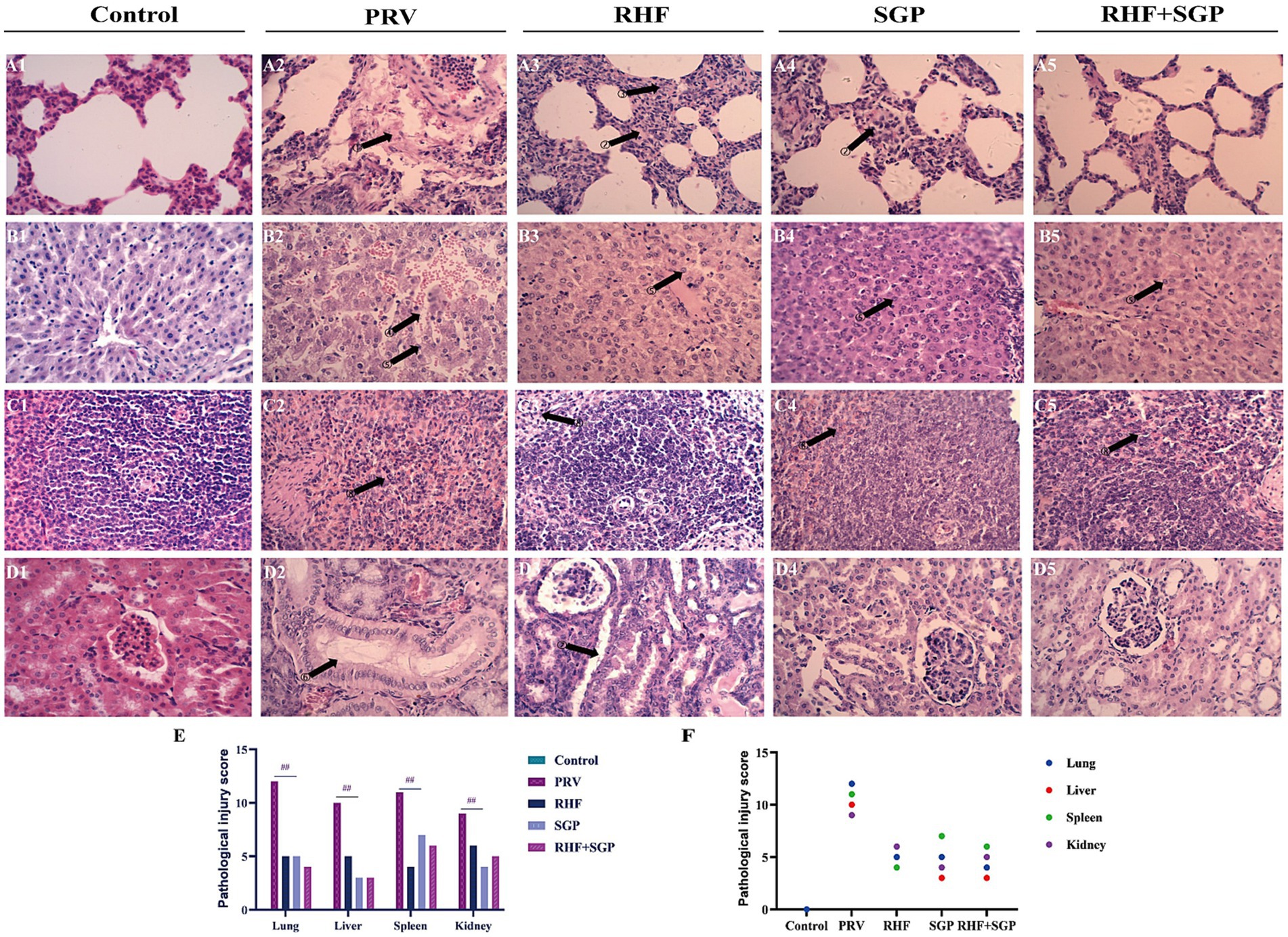
Figure 2. Histopathological pictures of lung, liver, spleen, and kidney tissues in different experimental groups and the pathological injury score. (A) Histopathological pictures of the lung. (B) Histopathological pictures of the liver. (C) Histopathological pictures of the spleen. (D) Histopathological pictures of the kidney. (E) The pathological injury scores. (F) Correlation between pathological damage and injury score within the same tissue. The arrow points to the lesion site. The numbers above the arrows indicate serous exudation in the alveolar interstitium; alveolar wall thickening; increased red blood cells; hepatocyte cord disorder, hepatic sinusoid enlargement; abundant plasma cells; distal convoluted tubule structure changes; the structure of distal convoluted tubules and proximal convoluted tubules are fuzzy; lymphocyte infiltration and erythrocytosis. (Hematoxylin and eosin staining, 400× magnification). (n = 10 in per group).
Pathological damage scores showed that damage to the lungs, liver, spleen, and kidneys of PRV-infected piglets was alleviated in the three drug groups when compared to those of the untreated control groups (p ≤ 0.01) (Figure 2E), and the pathological lesions correlated with damage scores within the same tissue (Figure 2F).
3.3 SGP effectively enhances T lymphocyte conversion rates
The effect of SGP and RHF on T lymphocyte conversion was investigated in PRV-infected piglets. The stimulation index (SI) of 1.25 mg/mL and 2.5 mg/mL SGP treatments was significantly higher than that of 5 mg/mL concentrations in PRV-infected piglets (p ≤ 0.01) (Figure 3A). These results indicated that SGP effectively enhances T lymphocyte conversion rates.
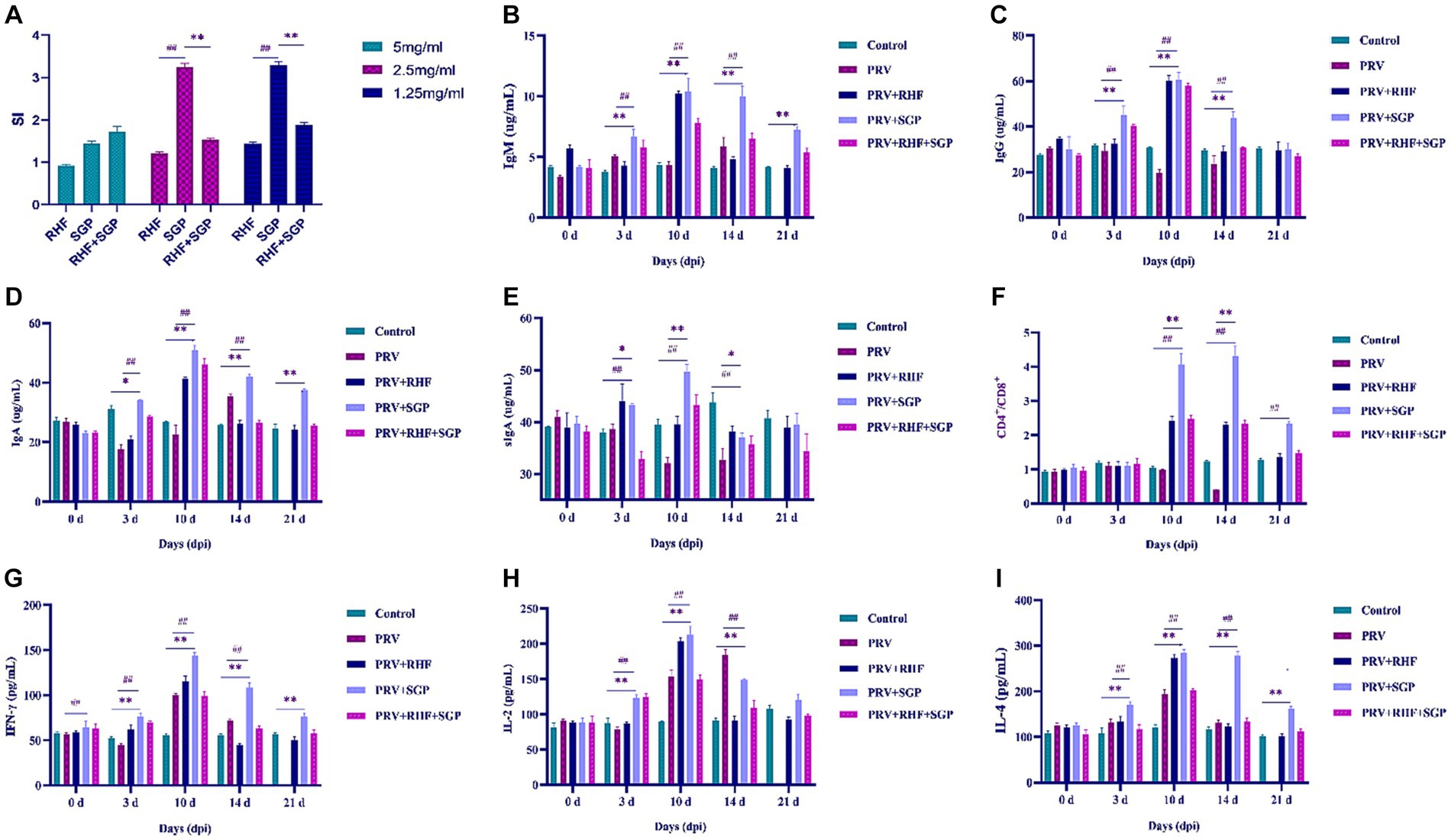
Figure 3. (A) The stimulation index in each group. (B) IgM level in each group. (C) IgG level in each group. (D) IgA level in each group. (E) sIgA level in each group. (F) CD4+/CD8+ ratio in each group. (G) IFN-γ level in each group. (H) IL-2 level in each group. (I) IL-4 level in each group. (At each selected time point, all the living piglets were tested, n = 10 in per group).
3.4 RHF and SGP treatments increase immunoglobulin concentration
The levels of IgM, IgG, IgA, and sIgA in the experimental groups were significantly different from those at 3 dpi (p ≤ 0.01 or p ≤ 0.05). These differences were greatest at 10 dpi (p ≤ 0.01), after which IgM, IgG, and IgA decreased, while sIgA first decreased and then increased when compared with control group (Figures 3B–E). Both RHF and SGP increased the concentration of immunoglobulins in the blood and lung tissue, with SGP showing a greater ability to boost immunoglobulin levels, indicating an improved immunity and mucosal anti-infection immunity in piglets infected with PRV.
3.5 SGP improves the serum CD4+/CD8+ ratio and cytokine production in PRV-infected piglets
Significant differences in the CD4+/CD8+ ratio in the SGP group were observed at 10 dpi when compared with control group (p ≤ 0.01) (Figure 3F). Significant differences in the levels of IFN-γ, IL-2, and IL-4 in all experimental groups were observed at 3 dpi, with the largest differences observed at 10 dpi when compared with control group (p ≤ 0.01). These results showed that SGP improved the serum CD4+/CD8+ ratio and cytokine production in PRV-infected piglets (Figures 3G–I).
3.6 SGP increases TLR3 and TLR7 expression in PRV-infected piglet spleens
A significant increase in the TLR3 mRNA levels in PRV-infected piglets treated with and without SGP and RHF were observed at 3 dpi (p ≤ 0.01), with the largest increase observed at 10 dpi when compared with the controls (p ≤ 0.01). These increases were followed by a gradual decrease in TLR3 levels. The TLR7 levels first decreased, then increased, and then decreased again after SGP and RHF treatment, with the highest expression observed at 10 dpi when compared with the controls. Both RHF and SGP treatment increased the relative expression levels of TLR3 and TLR7 in the spleens of PRV-infected piglets; however, this effect was more significant in the SGP group (Figures 4A,B).
4 Discussion
Several studies have shown that traditional Chinese medicines have antiviral and immune-enhancing effects (29). RH is used to treat various immune diseases, such as tumours, asthma, and rheumatoid arthritis (21), although the medicinal value of these active ingredients has rarely been reported (30). SG is the dried S. scutellariae root from which baicalin is extracted from the by-product of Scutellaria flavones. These compounds are relatively easy to obtain and demonstrate anti-tumour activities, blood glucose regulatory effects, lipid regulation, antioxidant properties, antibacterial activates, and anti-inflammatory and immunomodulatory effects (31, 32). The prevention and treatment of PRV pose considerable challenges to industrial pig farming and the effects of RSH and SGP on PRV-infected piglets have not been reported (see Figure 4).
We initially fermented and extracted the effective components from four native Yunnan herbs (SG, RH, Angelica, and the root of Isatis indigotica) and found that SG and RH were the most effective medicinal herbs. We sought to identify the medicinal herb with optimal therapeutic and immunomodulatory effects on PRV-infected piglets and determine whether their individual or combined administration is most effective at treating PRV infection. Clinical symptom and pathological injury scores were used to evaluate the therapeutic effects of the experimental treatments in PRV-infected piglets and lymphocyte transformation assays and relevant immunoglobulins, cytokines, T cells, and Toll-like receptors were evaluated to demonstrate the immunomodulatory effects of the tested treatments.
The clinical symptom and pathological injury scores showed that all three treatment groups alleviated the clinical symptoms and pathological injury of pseudorabies in PRV-infected piglets. Xiong et al. (33) calculated the clinical cure rate of COVID-19 patients using a Chinese herbal formula containing Scutellaria and found that the clinical and fever symptom scores and inflammatory biomarkers were reduced. Ming et al. (21) also found that Astragalus polysaccharides alleviated lipopolysaccharide-induced inflammatory lung injury by altering the intestinal microbiota in mice, while Li et al. (31) reported that the flavonoid compound, kaverol, inhibits PRV replication in the brain, lungs, kidneys, heart, and spleen, alleviating pathological changes in these organs.
The levels of IgM, IgG, IgA, sIgA, IL-2, IL-4, TLR3, and TLR7 showed that both RHF and SGP enhanced the immunity of PRV-infected piglets; however, SGP had a stronger effect than RHF. In lymphocyte transformation experiments, we found that 1.25 mg/mL and 2.5 mg/mL SGP treatments could improve lymphocyte conversion, indicated by the increased immunoglobulin concentration in piglets that received SGP treatment than that of untreated piglets. Baicalin can significantly increase IgA, IgG, and IgM levels in the serum of mice (23), which is similar to the results of the present study. Pro-inflammatory cytokines and high levels of interferon in the airway mucosa can trigger and maintain local inflammation and can lead to disturbances in the protective mucosal immune response. We found that sIgA levels in treated piglets at 21 dpi increased instead of decreased. We hypothesized that this was related to higher levels of interferon that aggravated the mucosal inflammatory response (26, 34). Both RHF and SGP increased the ratio of CD4+/CD8+ cells and cytokine levels in the serum of PRV-infected piglets, although SGP was more effective. Future studies will examine the relationship between interferon and PRV infection.
The SGP treatment of PRV-infected piglets showed a greater increase in the relative RNA expression of TLR3 and TLR7 in isolated spleen tissues, further indicating that RHF and SGP treatment improved the immunomodulatory function in PRV-infected piglets and that the effect of SGP treatment was greater than that of RHF. There are also some current articles suggesting that the rise of TLR4 in herbal formulations containing baicalin is also associated with inflammatory bursts (35).
Most markers for inflammation showed higher elevation at 10 dpi, which may be related to the immune response against PRV. Previous study have shown that PRV has the highest viral load between 7 and 10 dpi and that antibody detection in the humoral immune response after immunisation occurs after 5 to 10 dpi (36). Therefore, we hypothesised that administration of RHF and SGP within 10 dpi of infection would increase the levels of PRV-related immune factors in piglets, promote lymphocyte proliferation, and enhance piglet resistance to PRV. Our results showed that RHF and SGP can reduce the clinical symptoms and pathological damage in PRV-infected piglets by improving their immunity.
In this study, the in vitro tests had some limitations and in the future, primary cells can be isolated from challenged tissue and transcriptomic analysis can be used to search for proteins with significant differences. Validation was performed on primary cells and the safety of these two extracts on cells needs further investigation. Network pharmacological analysis of the baicalin monomer and PRV will be conducted in the future to find targets and functional pathways that will provide information to produce more effective drugs suitable for treating PRV.
5 Conclusion
RHF and SGP treatments alleviate the clinical symptoms of PRV infection in piglets. The immunomodulatory effect of SGP treatment was better than that in the RHF and a combination of both treatments. This study has important implications in the search for effective herbal medicines that can control PRV transmission and infection.
Data availability statement
The original contributions presented in the study are included in the article/supplementary material, further inquiries can be directed to the corresponding author.
Ethics statement
The animal study was approved by International Animal Care and Use Committee of the Yunnan Agricultural University (permission code: YAUACUC06; date of publication: July 10, 2017). The study was conducted in accordance with the local legislation and institutional requirements.
Author contributions
XS: Writing – review & editing. YZ (2nd author): Writing – original draft. XZ: Visualization, Writing – review & editing. YZ (4th author): Methodology, Writing – review & editing. YS: Conceptualization, Writing – review & editing. YW: Data curation, Writing – review & editing. ZZ: Software, Writing – review & editing. CS: Supervision, Writing – review & editing.
Funding
The author(s) declare that financial support was received for the research, authorship, and/or publication of this article. This study was supported by National Key R&D Project “Integration and Demonstration of Green and Healthy Breeding Technology of Zhaotong Wugen Pig in Zhenxiong County” (grant no. 2022YFD1100407).
Acknowledgments
The paper was coauthored by XS, YZ, XZ, YZ, YS, YW, and ZZ.
Conflict of interest
The authors declare that the research was conducted in the absence of any commercial or financial relationships that could be construed as a potential conflict of interest.
Publisher’s note
All claims expressed in this article are solely those of the authors and do not necessarily represent those of their affiliated organizations, or those of the publisher, the editors and the reviewers. Any product that may be evaluated in this article, or claim that may be made by its manufacturer, is not guaranteed or endorsed by the publisher.
References
1. Chen, M, Wang, MH, Shen, XG, Liu, H, Zhang, YY, Peng, JM, et al. Neuropilin-1 facilitates pseudorabies virus replication and viral glycoprotein B promotes its degradation in a furin-dependent manner. J Virol. (2022) 96:e0131822. doi: 10.1128/jvi.01318-22
2. Nie, Z, Zhu, S, Wu, L, Sun, R, Shu, J, He, Y, et al. Progress on innate immune evasion and live attenuated vaccine of pseudorabies virus. Front Microbiol. (2023) 14:1138016. doi: 10.3389/fmicb.2023.1138016
3. Malyshkina, A, Brüggemann, A, Paschen, A, and Dittmer, U. Cytotoxic CD4+ T cells in chronic viral infections and cancer. Front Immunol. (2023) 14:1271236. doi: 10.3389/fimmu.2023.1271236
4. Dunne, MR, Wagener, J, Loeffler, J, Doherty, DG, and Rogers, TR. Unconventional T cells—new players in antifungal immunity. Clin Immunol. (2021) 227:108734. doi: 10.1016/j.clim.2021.108734
5. Sachs, B, Fatangare, A, Sickmann, A, and Glässner, A. Lymphocyte transformation test: history and current approaches. J Immunol Methods. (2021) 493:113036. doi: 10.1016/j.jim.2021.113036
6. Koskinen, R, Salomonsen, J, Tregaskes, CA, Young, JR, Goodchild, M, Bumstead, N, et al. The chicken CD4 gene has remained conserved in evolution. Immunogenetics. (2002) 54:520–5. doi: 10.1007/s00251-002-0490-4
7. Quere, P, Cooper, MD, and Thorbecke, GJ. Characterization of suppressor T cells for antibody production by chicken spleen cells. I. Antigen-induced suppressor cells are CT8+, TcR1+ (gamma delta) T cells. Immunology. (1990) 71:517–22.
8. Sant, AJ, and McMichael, A. Revealing the role of CD4+ T cells in viral immunity. J Exp Med. (2012) 209:1391–5. doi: 10.1084/jem.20121517
9. Lee, J, Park, JY, Yang, SJ, Lee, JY, Kim, DG, Joo, DJ, et al. Renal safety of tenofovir disoproxil fumarate and entecavir with hepatitis B immunoglobulin in liver transplant patients. J Viral Hepat. (2020) 27:818–25. doi: 10.1111/jvh.13291
10. Kawai, T, and Akira, S. The role of pattern-recognition receptors in innate immunity: update on Toll-like receptors. Nat Immunol. (2010) 11:373–84. doi: 10.1038/ni.1863
11. Sen, GC, and Sarkar, SN. Transcriptional signaling by double-stranded RNA: role of TLR3. Cytokine Growth Factor Rev. (2005) 16:1–14. doi: 10.1016/j.cytogfr.2005.01.006
12. Brownlie, R, and Allan, B. Avian Toll-like receptors. Cell Tissue Res. (2011) 343:121–30. doi: 10.1007/s00441-010-1026-0
13. Liao, H, Ye, J, Gao, L, and Liu, Y. The main bioactive compounds of Scutellaria baicalensis Georgi. for alleviation of inflammatory cytokines: a comprehensive review. Biomed Pharmacother. (2021) 133:110917. doi: 10.1016/j.biopha.2020.110917
14. Ma, XG, Sun, WG, and Sun, H. Historical introgression among the species of Rodgersia (Saxifragaceae) in mountainous forests of southwest China. Mol Phylogenet Evol. (2018) 125:93–9. doi: 10.1016/j.ympev.2018.03.002
15. Wang, L, Zhang, D, Wang, N, Li, S, Tan, HY, and Feng, Y. Polyphenols of Chinese skullcap roots: from chemical profiles to anticancer effects. RSC Adv. (2019) 9:25518–32. doi: 10.1039/c9ra03229k
16. Hong, GE, Kim, JA, Nagappan, A, Yumnam, S, Lee, HJ, Kim, EH, et al. Flavonoids identified from Korean Scutellaria baicalensis Georgi inhibit inflammatory signaling by suppressing activation of NF- kappa B and MAPK in RAW 264.7 cells. Evid Based Complement Alternat Med. (2013) 2013:912031. doi: 10.1155/2013/912031
17. Ayeka, PA, Bian, Y, Githaiga, PM, and Zhao, Y. The immunomodulatory activities of licorice polysaccharides (Glycyrrhiza uralensis Fisch.) in CT 26 tumor-bearing mice. BMC Complement Altern Med. (2017) 17:536. doi: 10.1186/s12906-017-2030-7
18. Tian, H, Liu, Z, Pu, Y, and Bao, Y. Immunomodulatory effects exerted by Poria cocos polysaccharides via TLR4/TRAF6/NF-kappaB signaling in vitro and in vivo. Biomed Pharmacother. (2019) 112:108709. doi: 10.1016/j.biopha.2019.108709
19. Wu, Y, Zhou, H, Wei, K, Zhang, T, Che, Y, Nguyễn, AD, et al. Structure of a new Glycyrrhiza polysaccharide and its immunomodulatory activity. Front Immunol. (2022) 13:1007186. doi: 10.3389/fimmu.2022.1007186
20. Huang, X, Tang, W, Lin, C, Sa, Z, Xu, M, Liu, J, et al. Protective mechanism of Astragalus Polysaccharides against Cantharidin-induced liver injury determined in vivo by liquid chromatography/mass spectrometry metabolomics. Basic Clin Pharmacol Toxicol. (2021) 129:61–71. doi: 10.1111/bcpt.13585
21. Ming, K, Zhuang, S, Ma, N, Nan, S, Li, Q, Ding, M, et al. Astragalus polysaccharides alleviates lipopolysaccharides-induced inflammatory lung injury by altering intestinal microbiota in mice. Front Microbiol. (2022) 13:1033875. doi: 10.3389/fmicb.2022.1033875
22. Song, C. (2016) Pathogenic characteristics of piglets infected with PRV and PCV2 and control techniques of pseudorabies in pigs.
23. Dou, T, Wang, J, Liu, Y, Jia, J, Zhou, L, Liu, G, et al. A combined transcriptomic and proteomic approach to reveal the effect of mogroside V on OVA-induced pulmonary inflammation in mice. Front Immunol. (2022) 13:800143. doi: 10.3389/fimmu.2022.800143
24. Zeinali, M, Rezaee, SA, and Hosseinzadeh, H. An overview on immunoregulatory and anti-inflammatory properties of chrysin and flavonoids substances. Biomed Pharmacother. (2017) 92:998–1009. doi: 10.1016/j.biopha.2017.06.003
25. Ninfali, P, Antonelli, A, Magnani, M, and Scarpa, ES. Antiviral properties of flavonoids and delivery strategies. Nutrients. (2020) 12:2534. doi: 10.3390/nu12092534
26. Wang, YL, Shu, XH, Zhang, X, Liu, YB, Zhang, YJ, Lv, T, et al. Effects of two polysaccharides from traditional Chinese medicines on rat immune function. Front Vet Sci. (2021) 8:703956. doi: 10.3389/fvets.2021.703956
27. Xie, L, Fang, L, Wang, D, Luo, R, Cai, K, Chen, H, et al. Molecular cloning and functional characterization of porcine DNA-dependent activator of IFN-regulatory factors (DAI). Dev Comp Immunol. (2010) 34:293–9. doi: 10.1016/j.dci.2009.10.007
28. Zhu, X, Wang, D, Zhang, H, Zhou, Y, Luo, R, Chen, H, et al. Molecular cloning and functional characterization of porcine DEAD (Asp–Glu–Ala–Asp) box polypeptide 41 (DDX41). Dev Comp Immunol. (2014) 47:191–6. doi: 10.1016/j.dci.2014.07.020
29. Wang, J, Chu, B, Du, L, Han, Y, Zhang, X, Fan, S, et al. Molecular cloning and functional characterization of porcine cyclic GMP-AMP synthase. Mol Immunol. (2015) 65:436–45. doi: 10.1016/j.molimm.2015.02.002
30. Laval, K, Van Cleemput, J, Vernejoul, JB, and Enquist, LW. Alphaherpesvirus infection of mice primes PNS neurons to an inflammatory state regulated by TLR2 and type I IFN signaling. PLoS Pathog. (2019) 15:e1008087. doi: 10.1371/journal.ppat.1008087
31. Li, GL, Ding, GX, Zeng, L, Ming, SL, Fu, PF, Wang, Q, et al. Inhibition of PARP1 dampens pseudorabies virus infection through DNA damage-induced antiviral innate immunity. J Virol. (2021) 95:e0076021. doi: 10.1128/JVI.00760-21
32. Liu, X, Zhang, M, Ye, C, Ruan, K, Xu, A, Gao, F, et al. Inhibition of the DNA-sensing pathway by pseudorabies virus UL24 protein via degradation of interferon regulatory factor 7. Vet Microbiol. (2021) 255:109023. doi: 10.1016/j.vetmic.2021.109023
33. Xiong, X, Wang, P, Su, K, William, C, and Xing, Y. Chinese herbal medicine for coronavirus disease 2019: a systematic review and meta-analysis. Pharmacol Res. (2020) 160:105056. doi: 10.1016/j.phrs.2020.105056
34. Sitz, KV, and Birx, DL. Lymphocyte proliferation assay. Methods Mol Med. (1999) 17:343–53. doi: 10.1385/0-89603-369-4:343
35. Wen, Y, Han, C, Liu, T, Wang, R, Cai, W, Yang, J, et al. Chaiqin chengqi decoction alleviates severity of acute pancreatitis via inhibition of TLR4 and NLRP3 inflammasome: identification of bioactive ingredients via pharmacological sub-network analysis and experimental validation. Phytomedicine. (2020) 79:153328. doi: 10.1016/j.phymed.2020.153328
Keywords: piglets, therapeutic effect, immune-regulation effect, pseudorabies virus, Scutellaria baicalensis Georgi polysaccharide, Rodgersia sambucifolia Hemsl flavonoids
Citation: Shu X, Zhang Y, Zhang X, Zhang Y, Shu Y, Wang Y, Zhang Z and Song C (2024) Therapeutic and immune-regulation effects of Scutellaria baicalensis Georgi polysaccharide on pseudorabies in piglets. Front. Vet. Sci. 11:1356819. doi: 10.3389/fvets.2024.1356819
Edited by:
Rao Zahid Abbas, University of Agriculture, Faisalabad, PakistanReviewed by:
Amjad Islam Aqib, Cholistan University of Veterinary and Animal Sciences, PakistanYun peng Fan, Northwest A&F University, China
Copyright © 2024 Shu, Zhang, Zhang, Zhang, Shu, Wang, Zhang and Song. This is an open-access article distributed under the terms of the Creative Commons Attribution License (CC BY). The use, distribution or reproduction in other forums is permitted, provided the original author(s) and the copyright owner(s) are credited and that the original publication in this journal is cited, in accordance with accepted academic practice. No use, distribution or reproduction is permitted which does not comply with these terms.
*Correspondence: Chunlian Song, Q2h1bmxpYW4tU29uZ0BvdXRsb29rLmNvbQ==
†These authors have contributed equally to this work